Abstract
1. The inhibition of acid extrusion via Na(+)-H+ exchange caused by reducing pHo (extracellular pH) was examined in the sheep cardiac Purkinje fibre. Intracellular pH (pHi) and intracellular Na+ activity (alpha 1 Na) were recorded using ion-selective microelectrodes. Acid extrusion via Na(+)-H+ exchange was estimated from the pHi recovery rate (multiplied by intracellular buffering power, beta) in response to an internal acid load induced by 20 mM-NH4Cl removal (nominally CO2-HCO3-free media). 2. At a given pHi, acid extrusion decreased sigmoidally with decreases of pHo in the range 8.5 to 6.5 (50% inhibition of efflux occurred at a pHo between 7.0 and 7.5). This inhibition was associated with a parallel decrease in Na+ influx as evidenced from a decrease in the rise of alpha i Na measured during acid extrusion, suggesting inhibition of Na(+)-H+ exchange. 3. The background acid-loading rate (estimated by adding 1 mM-amiloride to inhibit Na(+)-H+ exchange and recording the initial rate of fall of pHi) was found to be unaffected in the steady state by changes of pHo. We therefore conclude that the slowing of pHi recovery at low pHo is due to direct inhibition of Na(+)-H+ exchange rather than to an increase of background acid loading. 4. Reducing pHo (constant pHi) inhibited acid efflux by producing a parallel shift of the efflux versus pHi relationship to lower values of pHi, consistent with a decrease in the apparent internal H+ ion affinity (pKi) of the system. 5. Raising pHi (constant pHo) also inhibited acid efflux, but this was associated with a rise in the pHo required for 50% maximal inhibition of acid efflux (pKo), consistent with an increase in apparent affinity for external H ions. Thus reduction of pHo reduces pKi (point 4) while reduction of pHi reduces pKo (point 5). 6. Inhibition by elevated Ho+ was not linearly related to the decrease in chemical driving force for Na(+)-H+ exchange, nor was it related to a reversal of the transmembrane H+ gradient. We found that efflux still occurred when pHo less than pHi. 7. Efflux was not a unique function of the transmembrane H+ ratio (i.e. pHo-pHi). At appropriate values of pHi and pHo, acid efflux could be kept constant despite a four-fold change in the transmembrane H+ ratio. 8. Inhibition by low pHo was a saturating function of Ho+ ions with a Hill coefficient of 1.2.(ABSTRACT TRUNCATED AT 400 WORDS)
Full text
PDF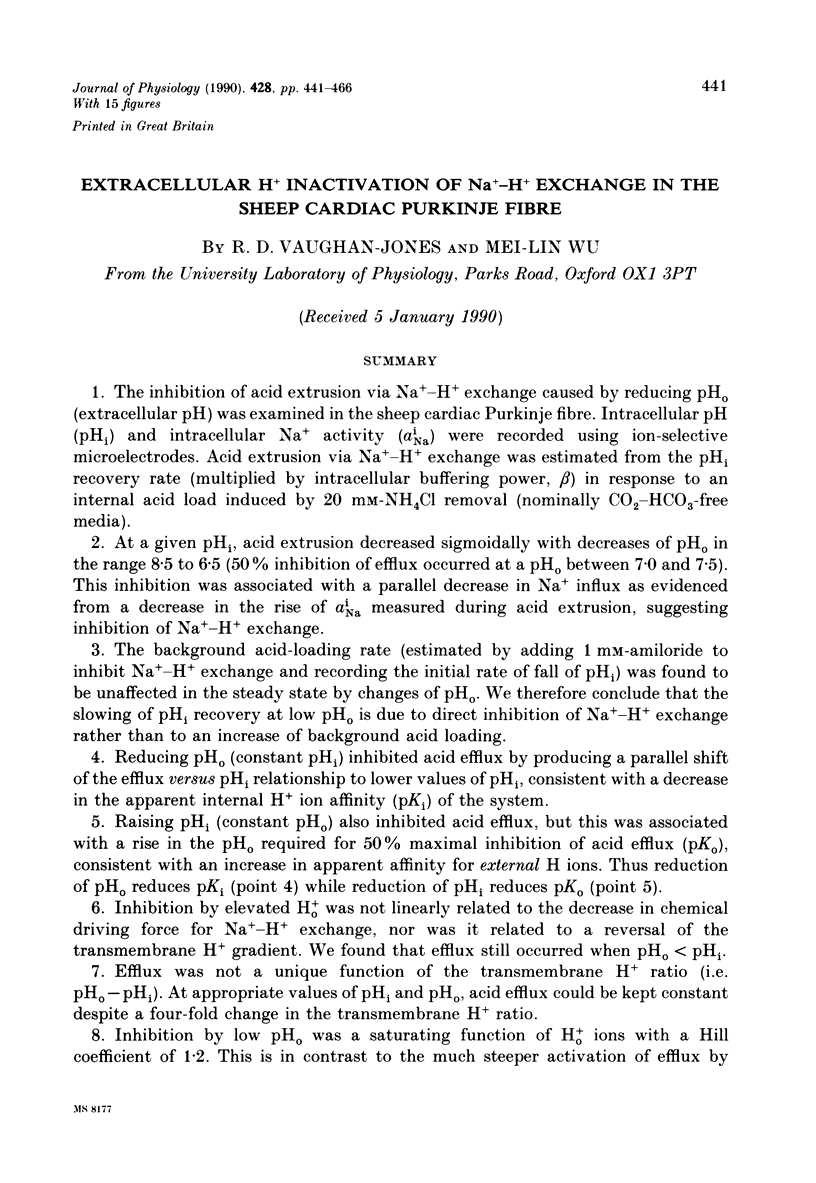
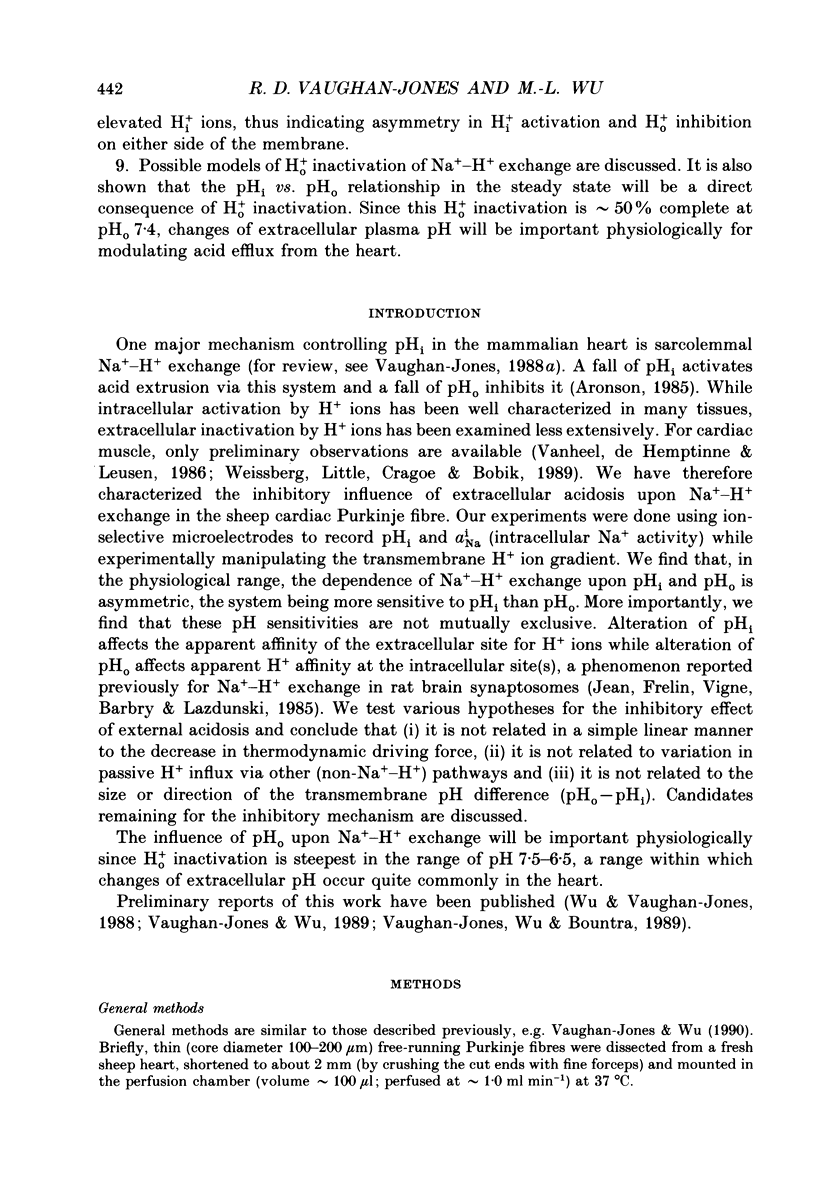
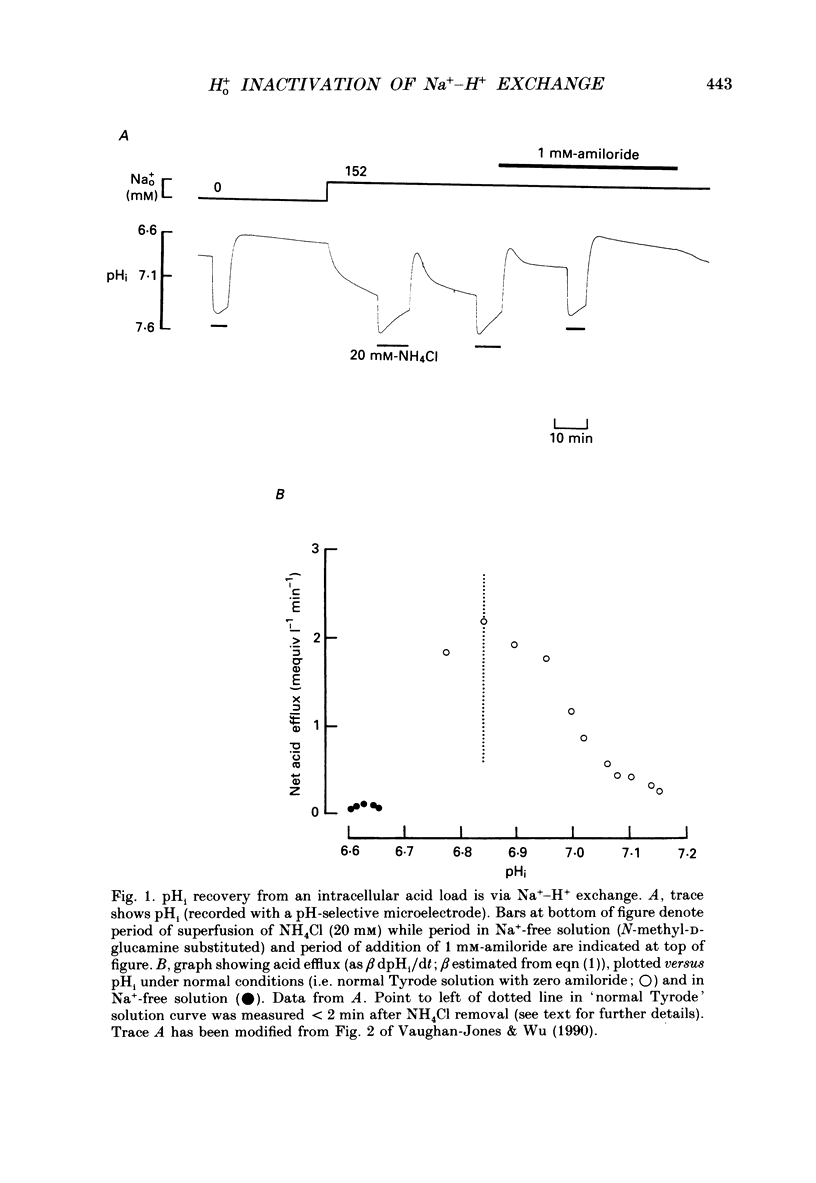
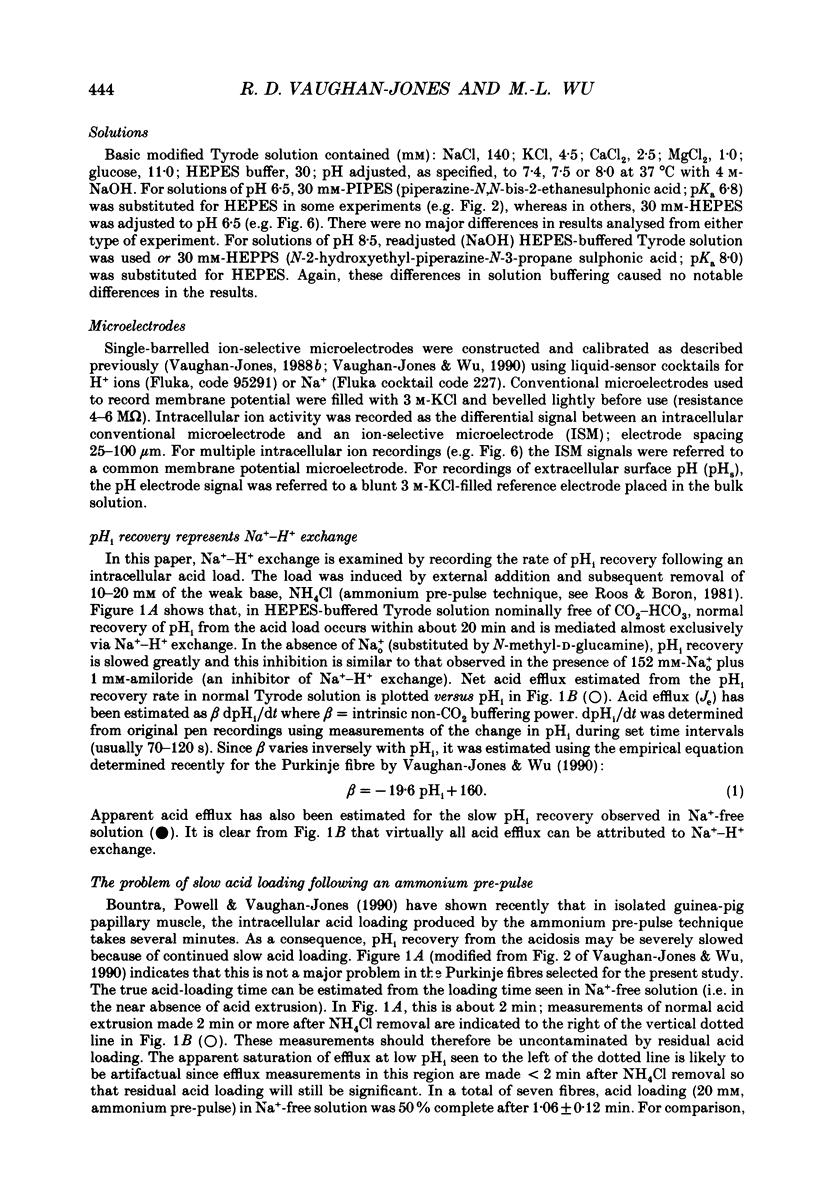
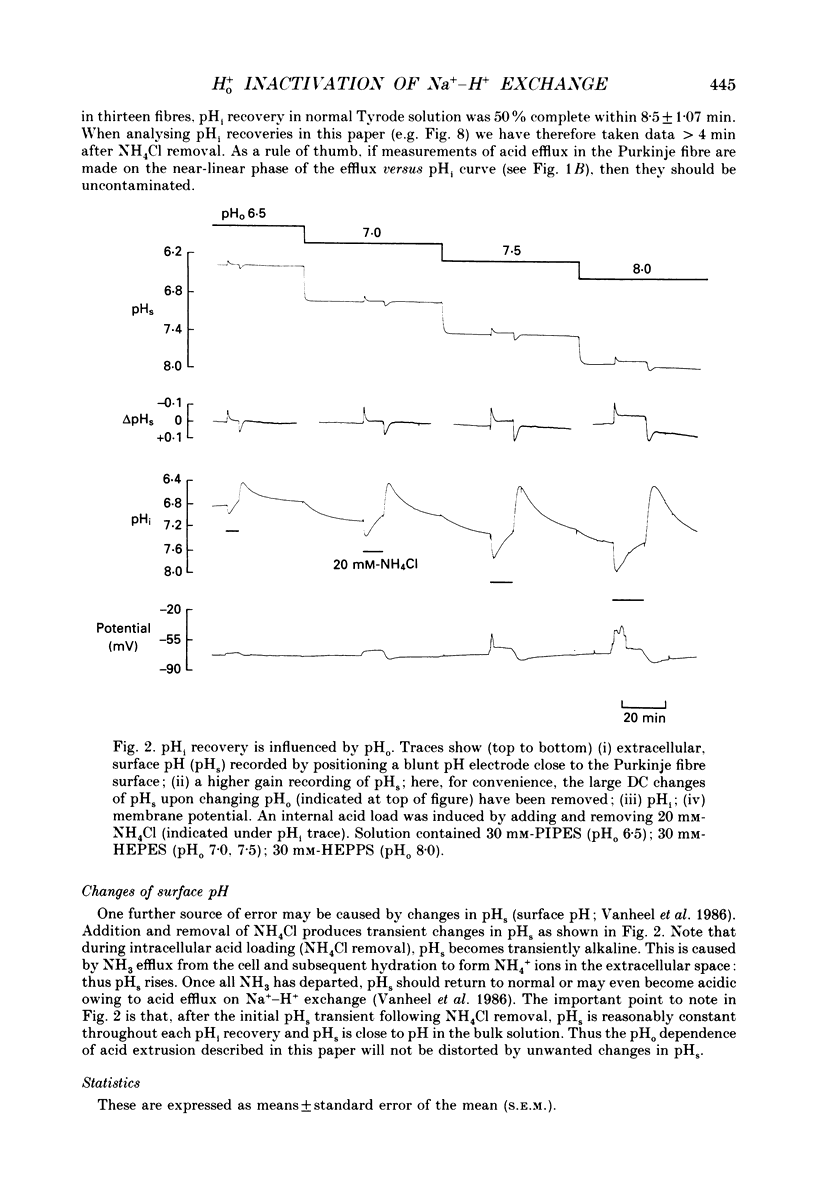
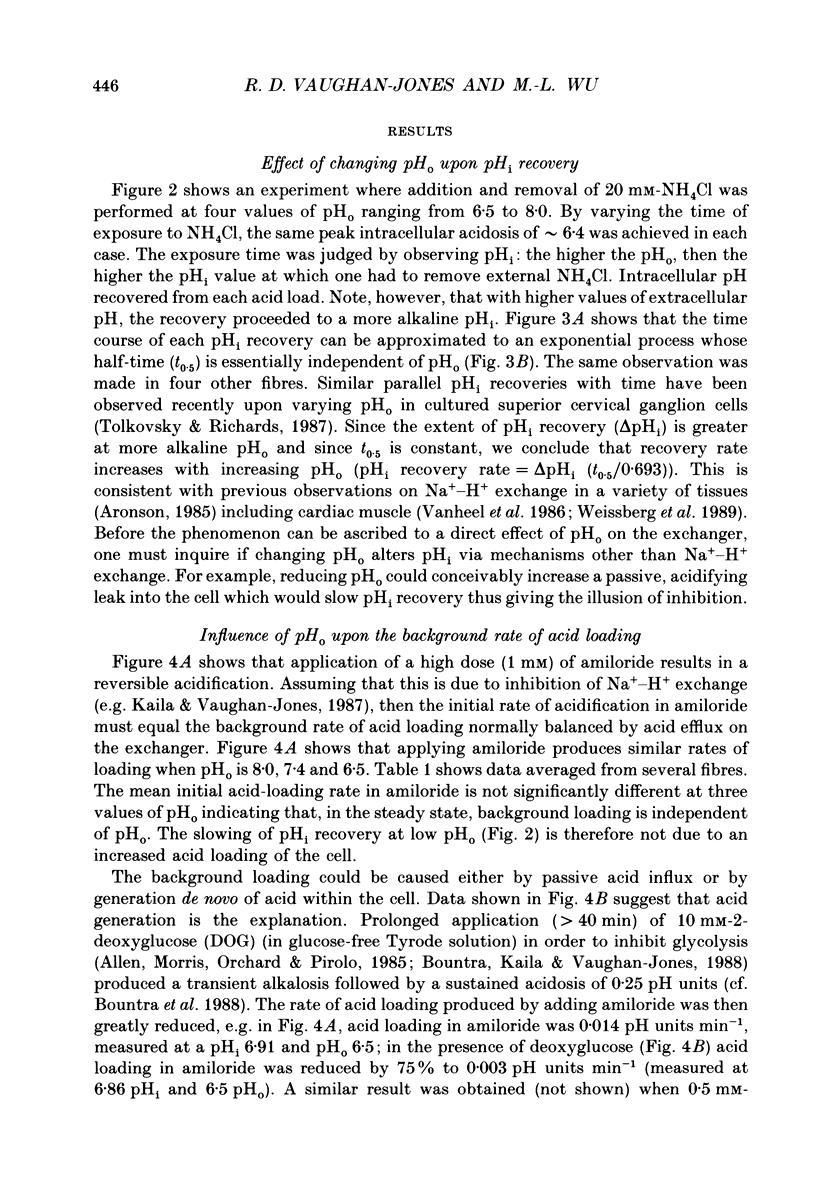
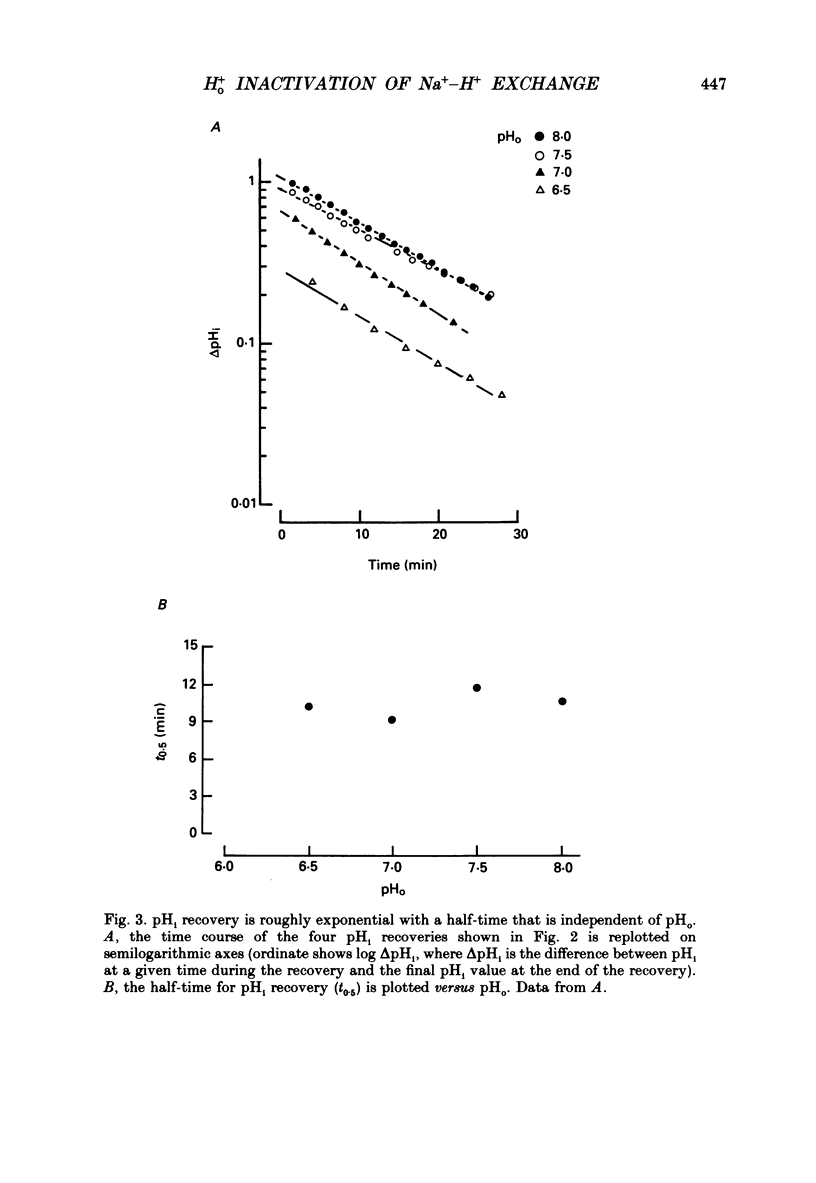
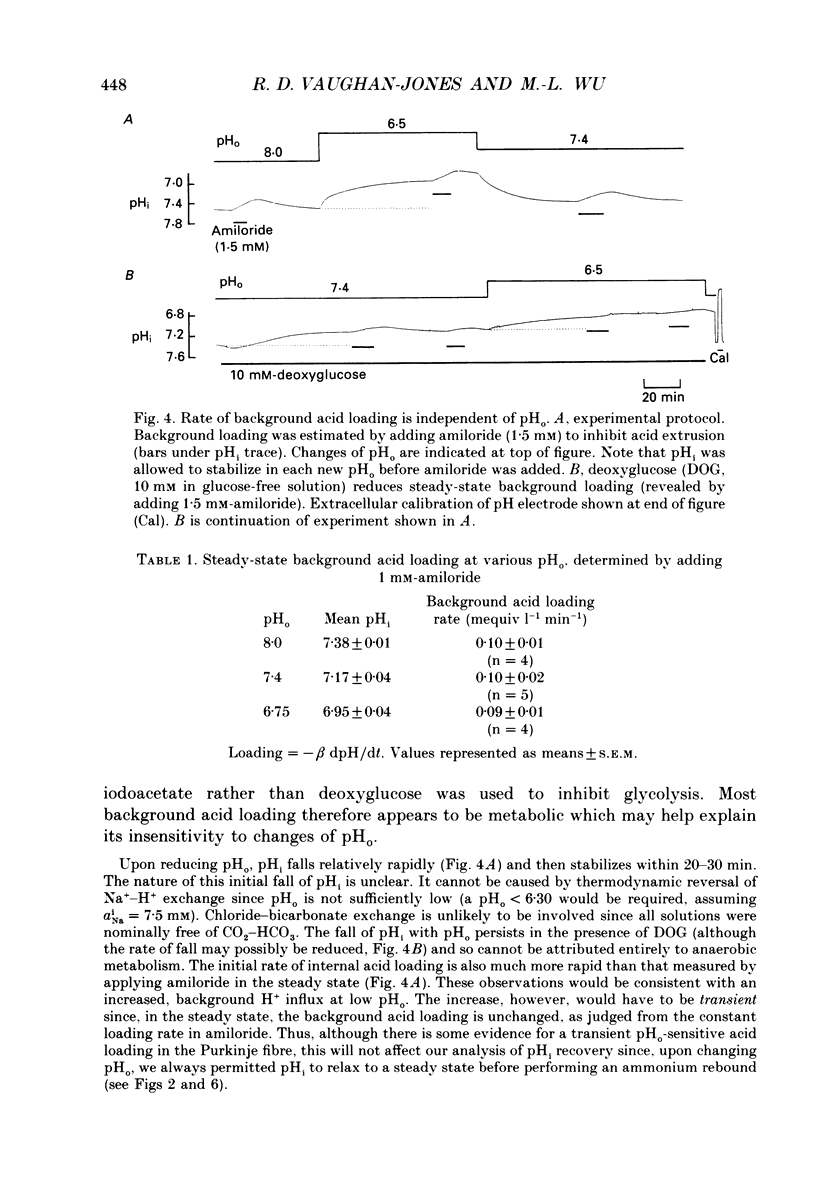
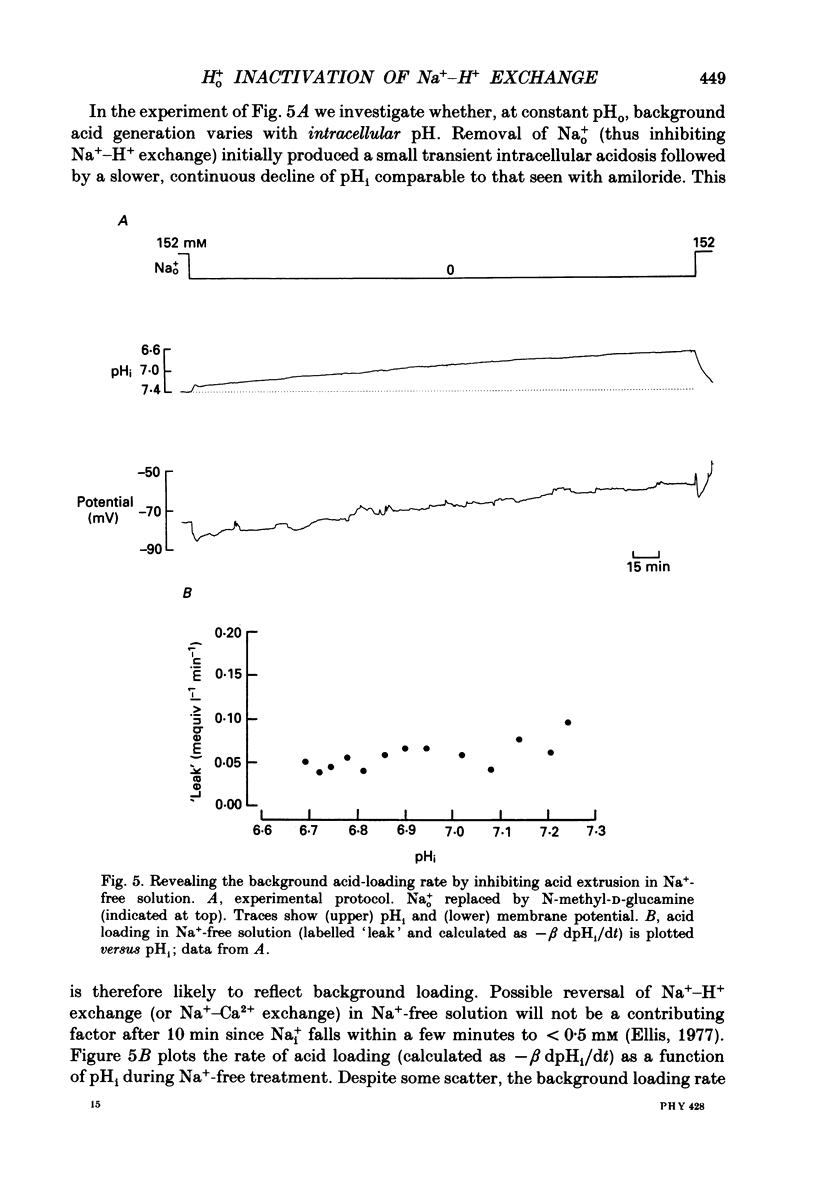
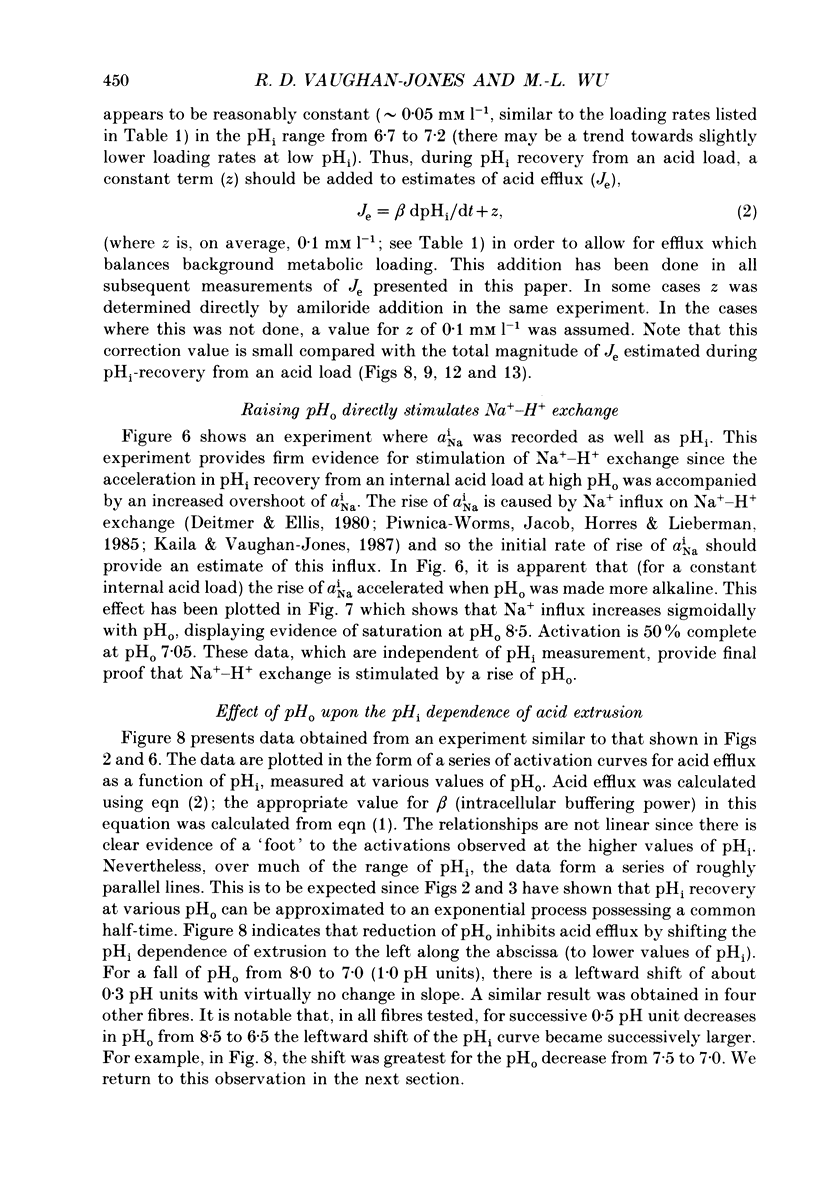
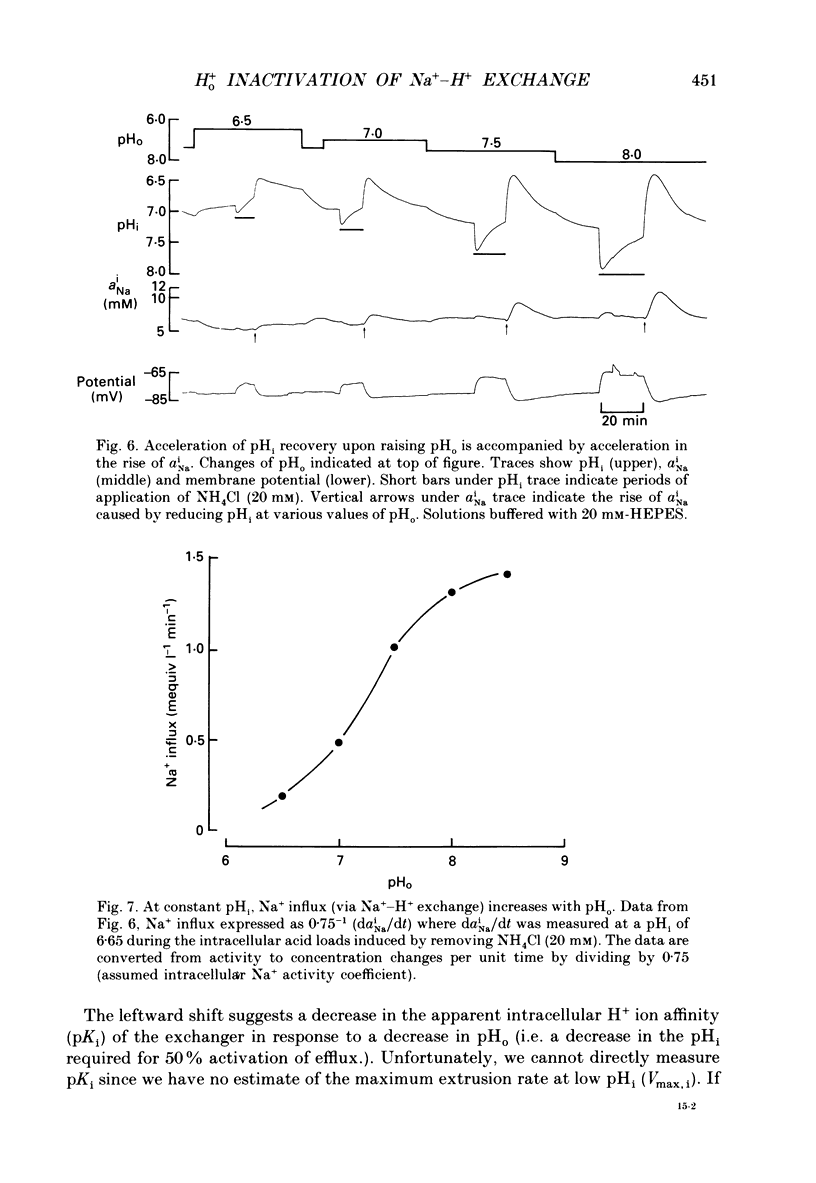
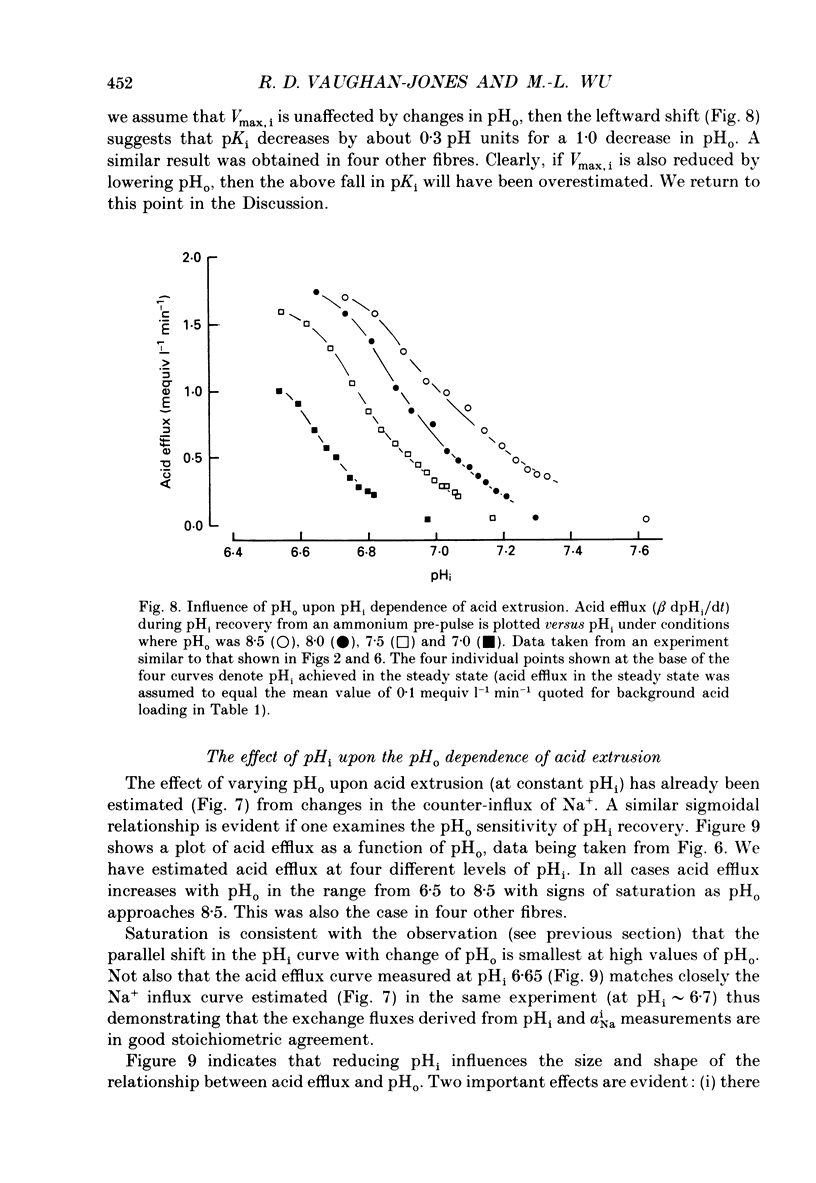
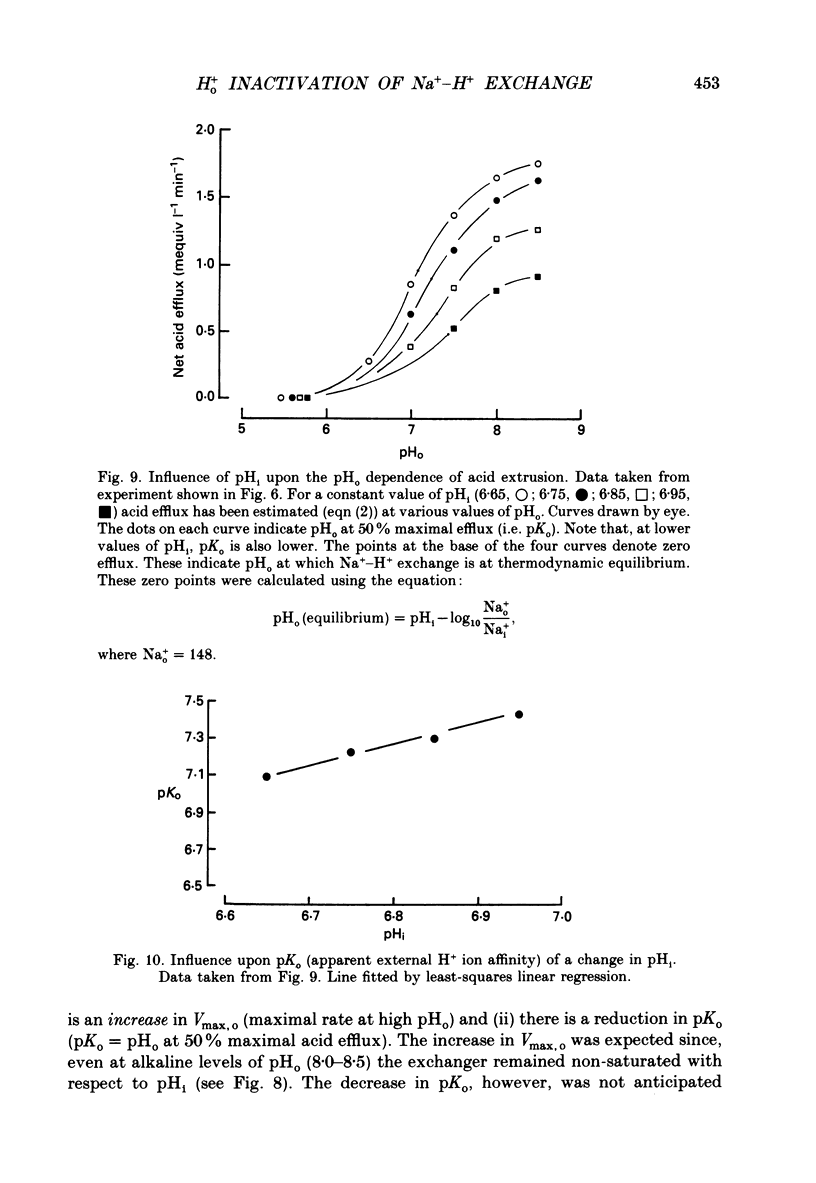
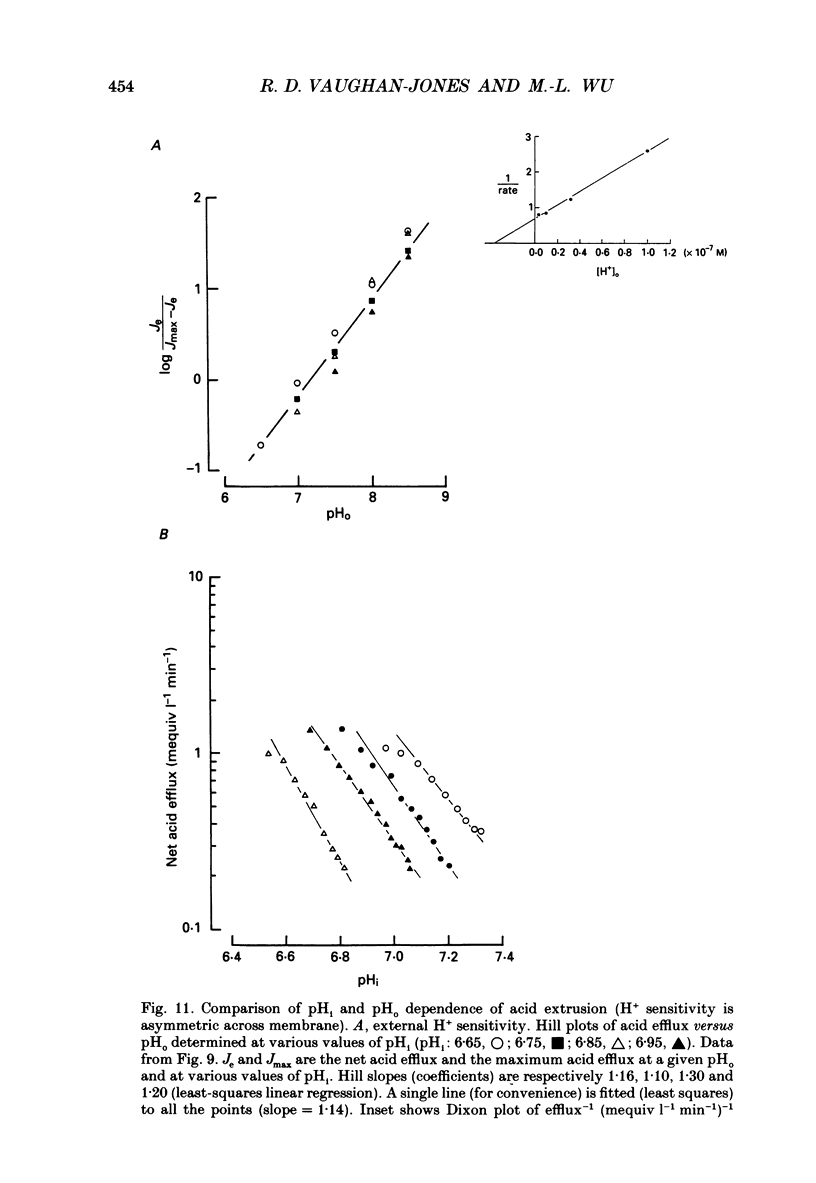
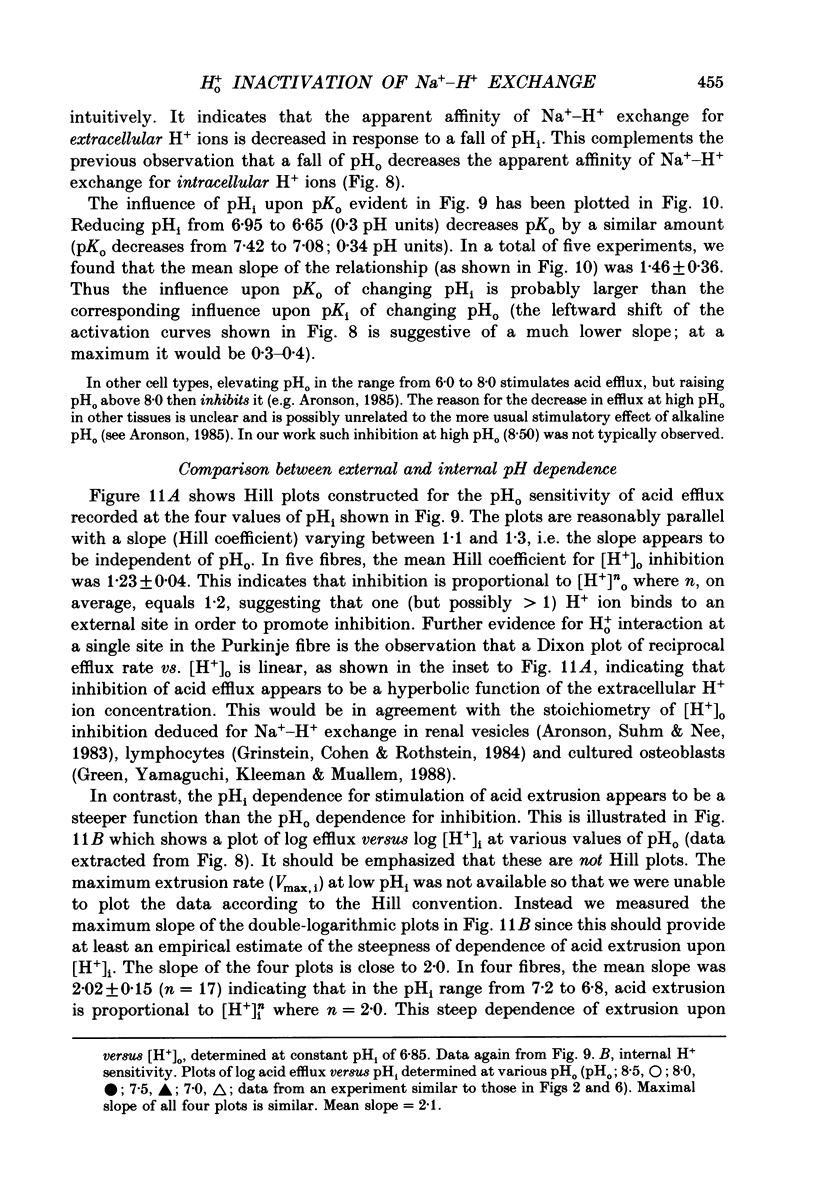
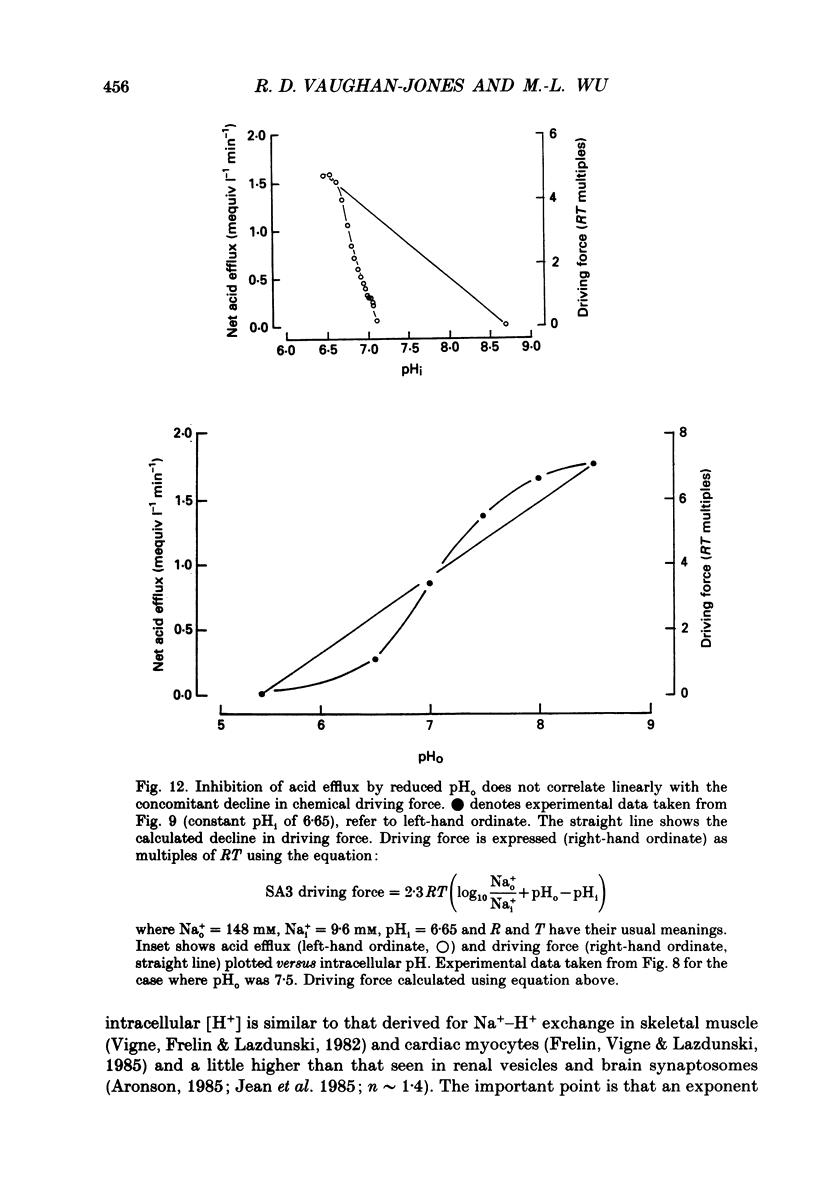
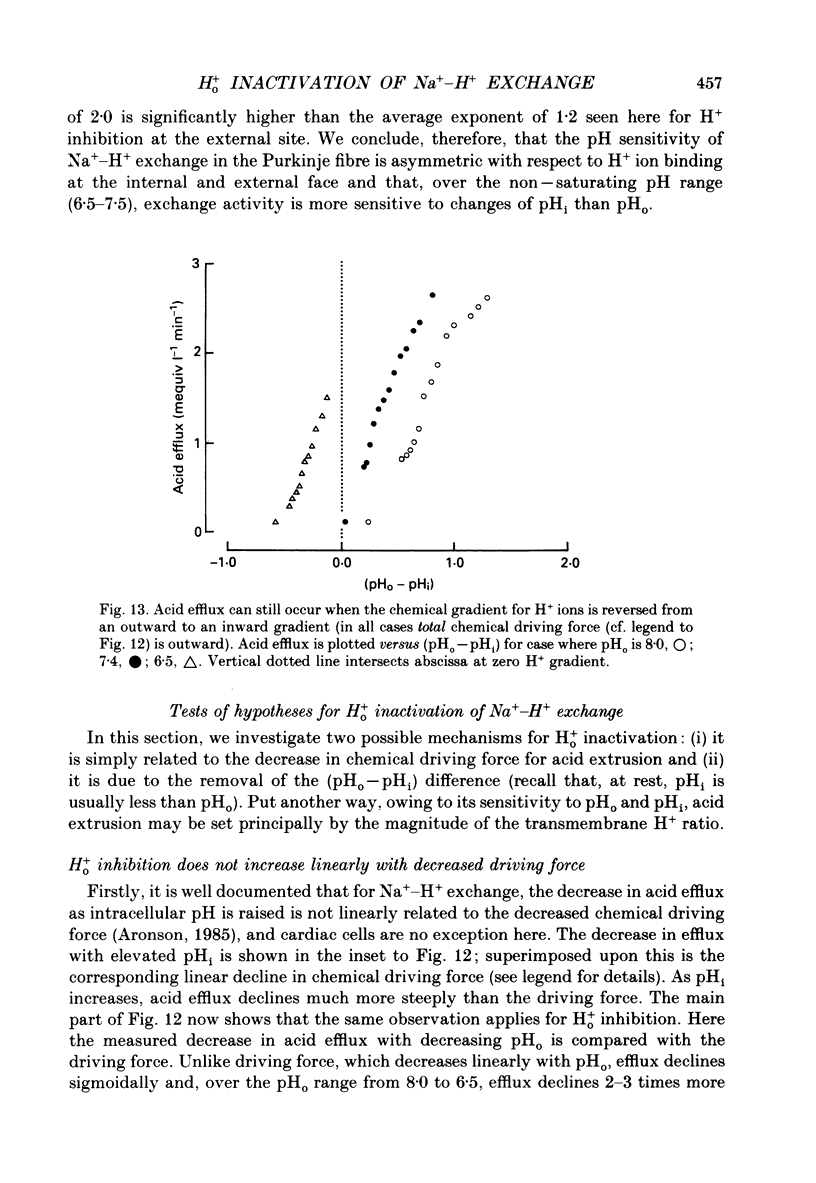
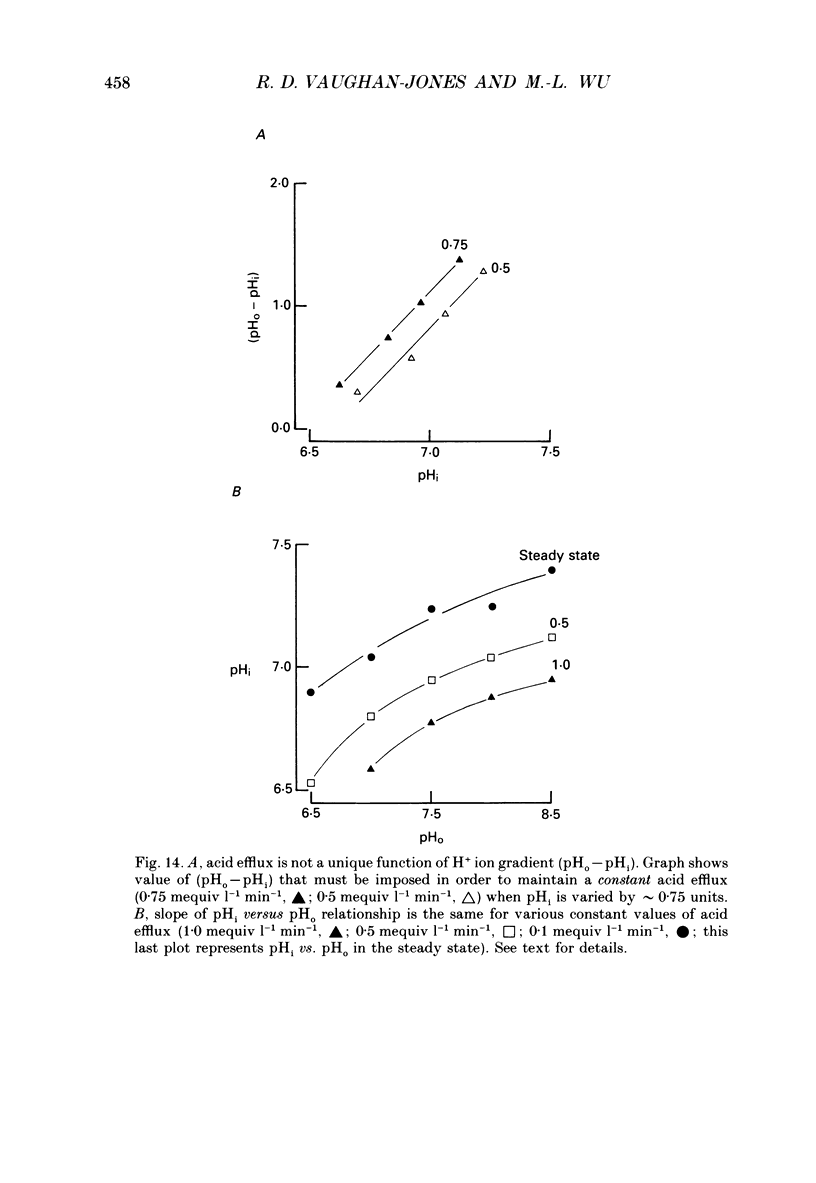
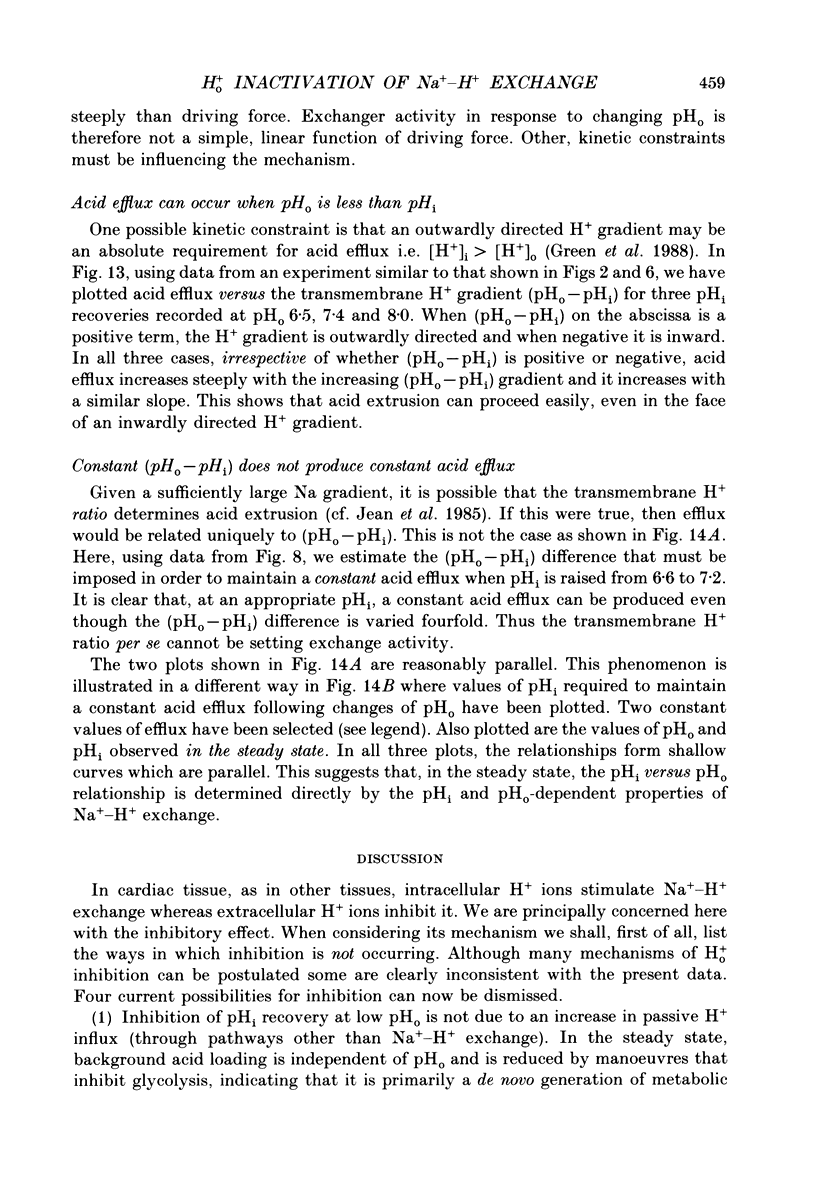
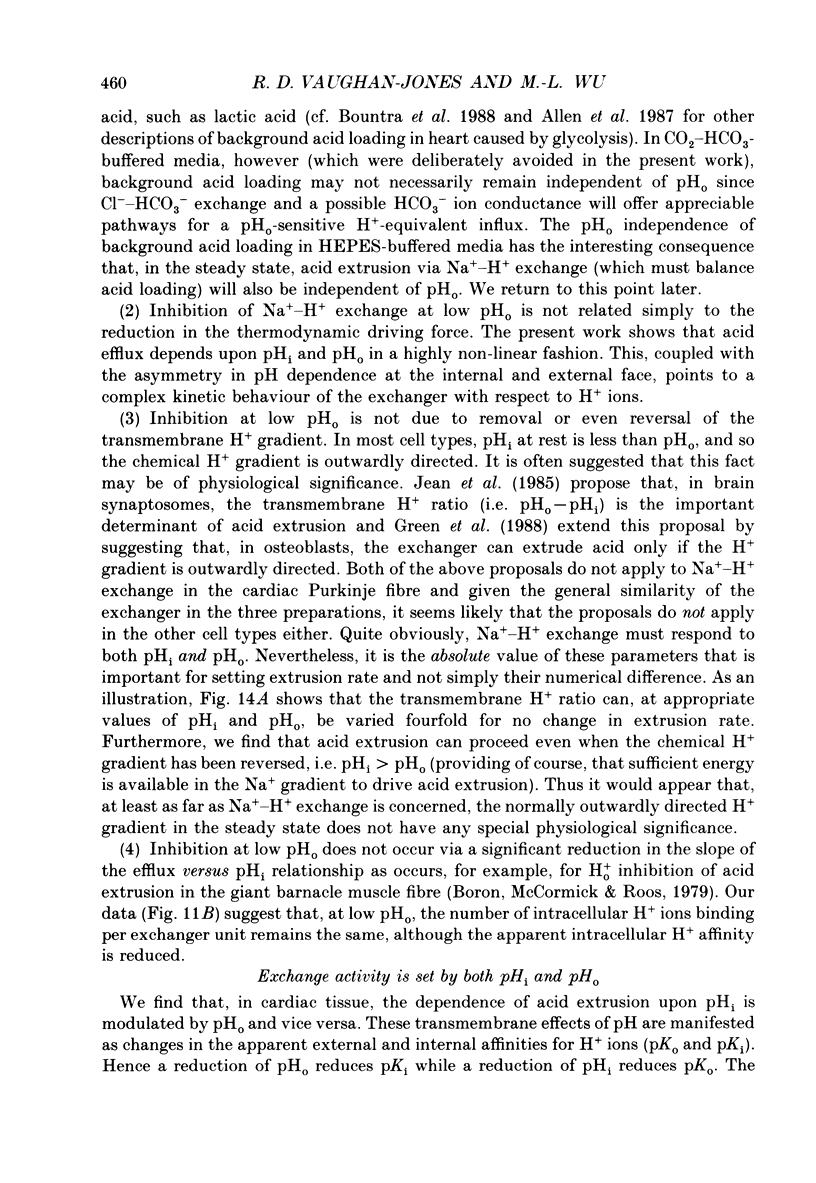
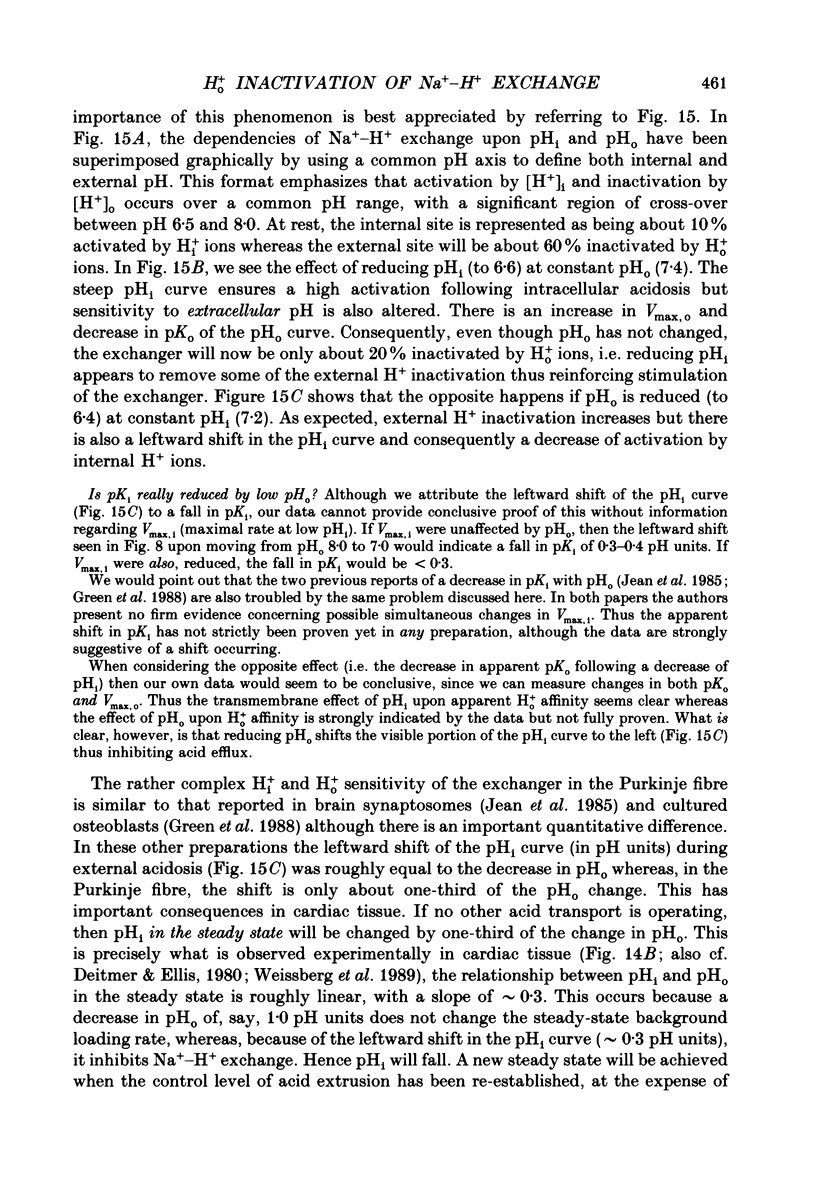
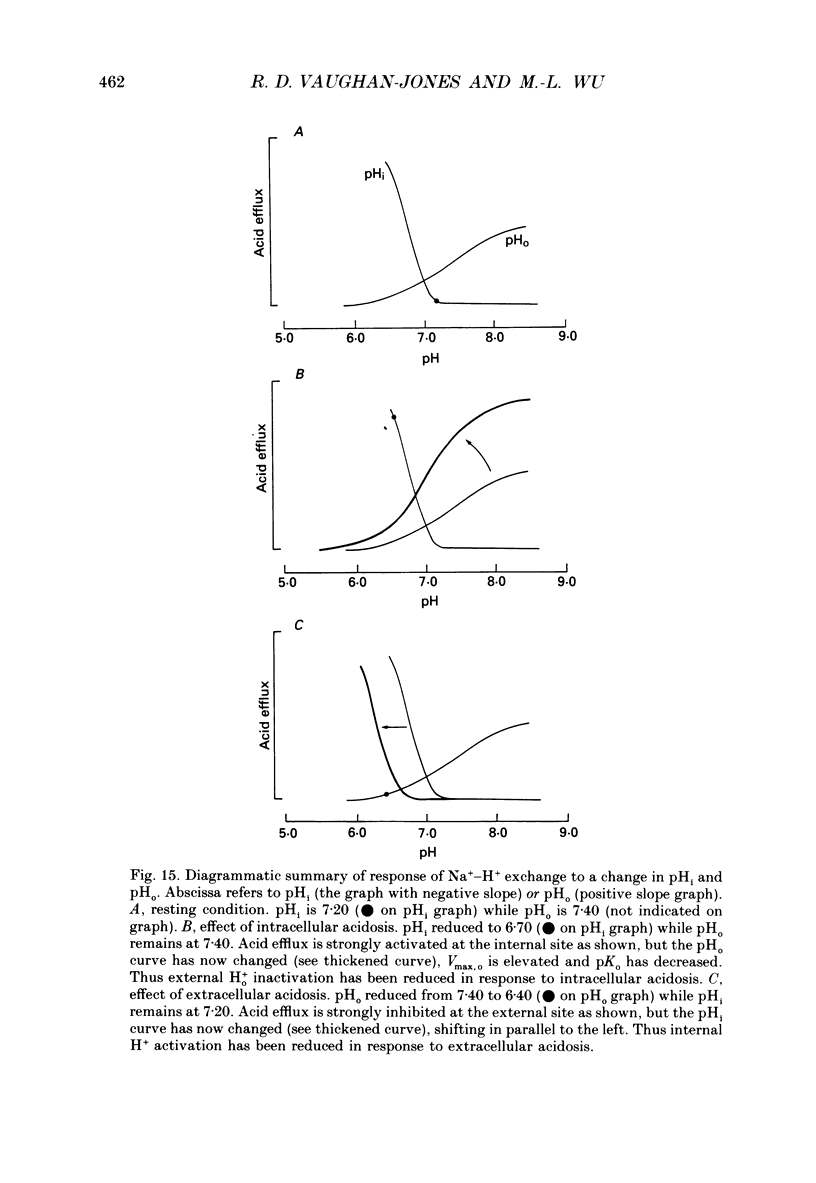
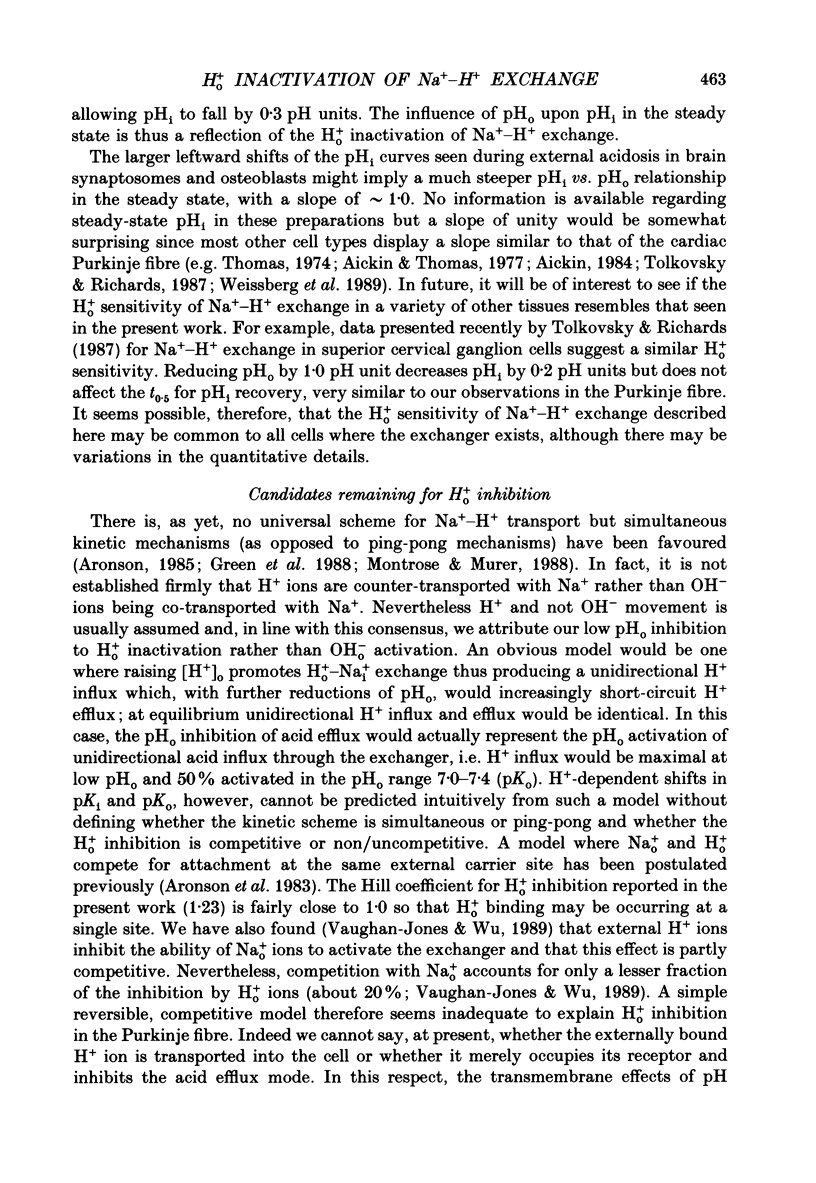
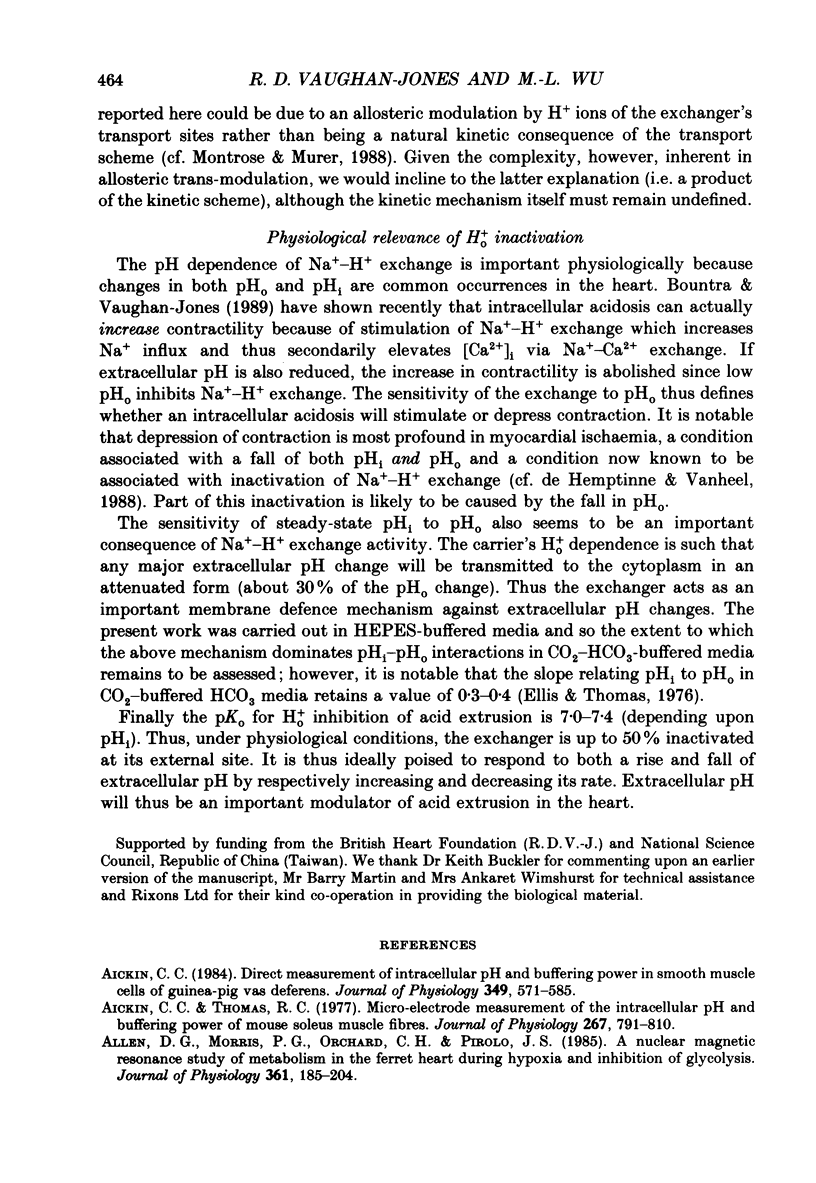
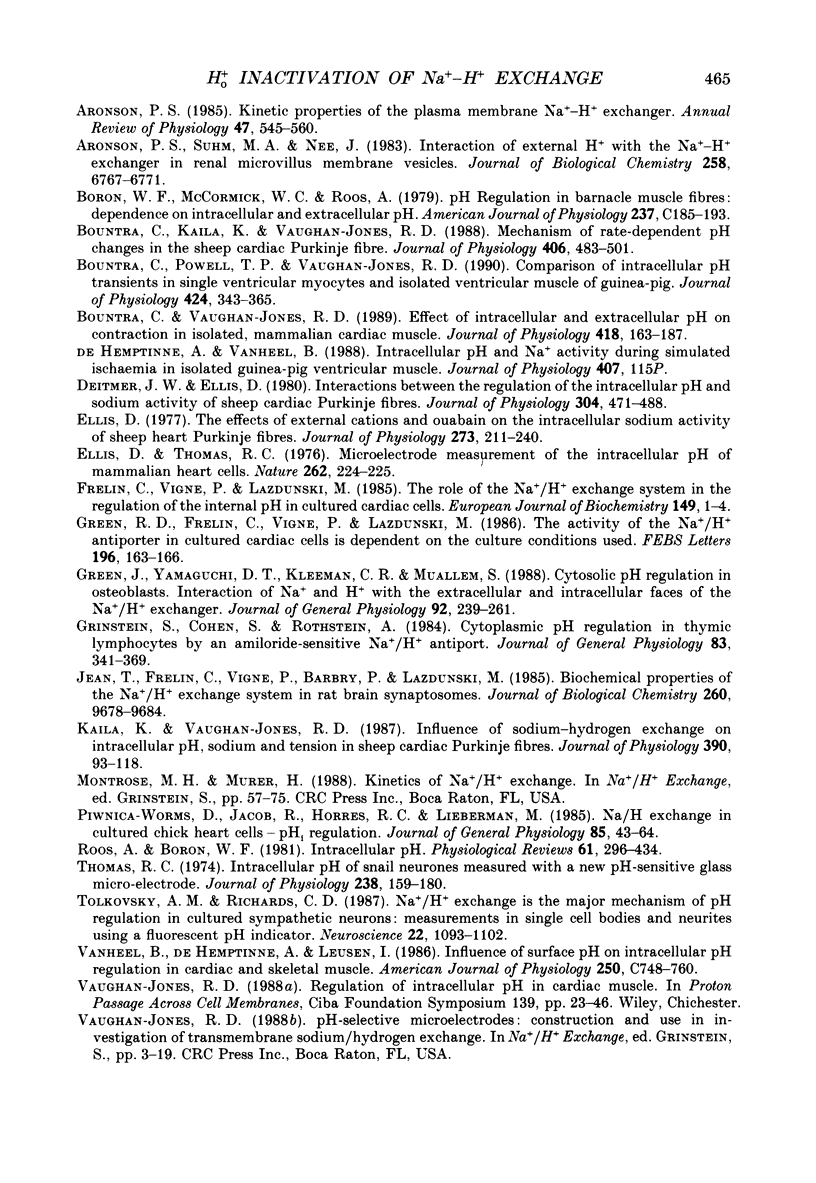
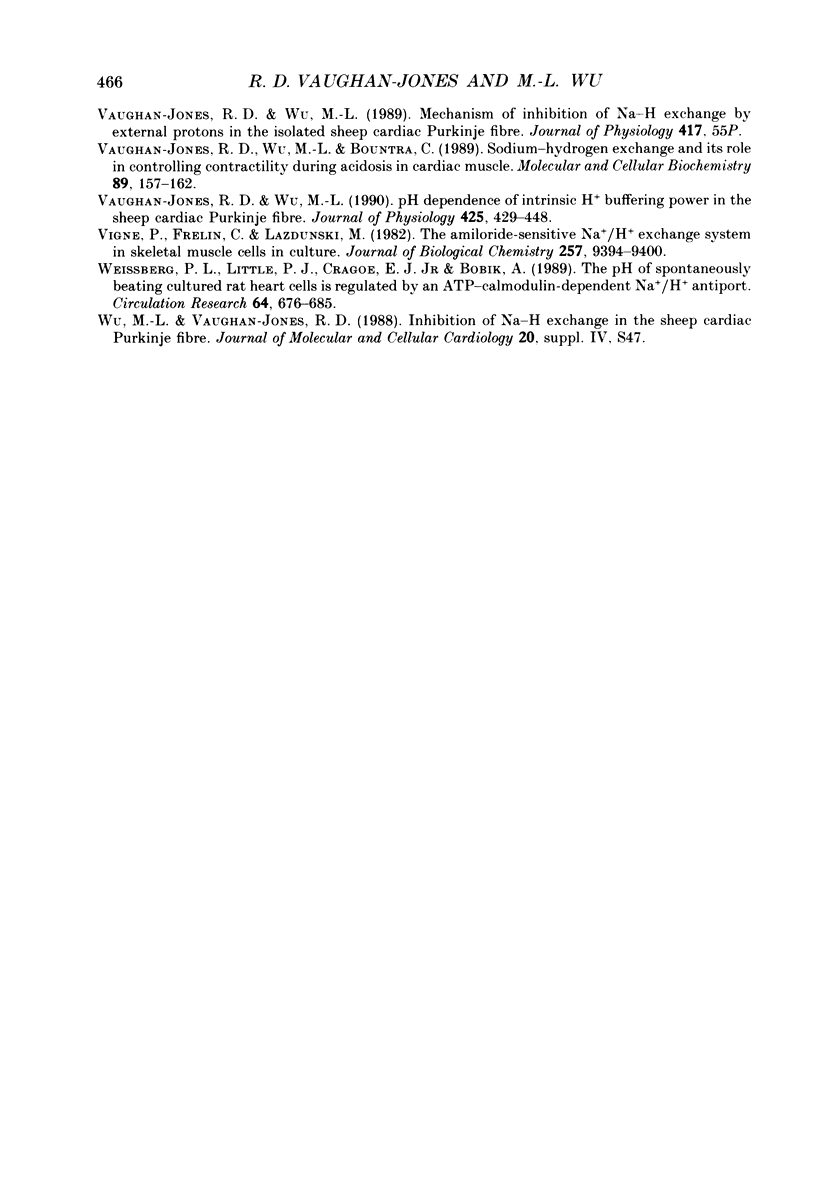
Selected References
These references are in PubMed. This may not be the complete list of references from this article.
- Aickin C. C. Direct measurement of intracellular pH and buffering power in smooth muscle cells of guinea-pig vas deferens. J Physiol. 1984 Apr;349:571–585. doi: 10.1113/jphysiol.1984.sp015174. [DOI] [PMC free article] [PubMed] [Google Scholar]
- Allen D. G., Morris P. G., Orchard C. H., Pirolo J. S. A nuclear magnetic resonance study of metabolism in the ferret heart during hypoxia and inhibition of glycolysis. J Physiol. 1985 Apr;361:185–204. doi: 10.1113/jphysiol.1985.sp015640. [DOI] [PMC free article] [PubMed] [Google Scholar]
- Aronson P. S. Kinetic properties of the plasma membrane Na+-H+ exchanger. Annu Rev Physiol. 1985;47:545–560. doi: 10.1146/annurev.ph.47.030185.002553. [DOI] [PubMed] [Google Scholar]
- Aronson P. S., Suhm M. A., Nee J. Interaction of external H+ with the Na+-H+ exchanger in renal microvillus membrane vesicles. J Biol Chem. 1983 Jun 10;258(11):6767–6771. [PubMed] [Google Scholar]
- Bountra C., Kaila K., Vaughan-Jones R. D. Mechanism of rate-dependent pH changes in the sheep cardiac Purkinje fibre. J Physiol. 1988 Dec;406:483–501. doi: 10.1113/jphysiol.1988.sp017392. [DOI] [PMC free article] [PubMed] [Google Scholar]
- Bountra C., Powell T., Vaughan-Jones R. D. Comparison of intracellular pH transients in single ventricular myocytes and isolated ventricular muscle of guinea-pig. J Physiol. 1990 May;424:343–365. doi: 10.1113/jphysiol.1990.sp018071. [DOI] [PMC free article] [PubMed] [Google Scholar]
- Bountra C., Vaughan-Jones R. D. Effect of intracellular and extracellular pH on contraction in isolated, mammalian cardiac muscle. J Physiol. 1989 Nov;418:163–187. doi: 10.1113/jphysiol.1989.sp017833. [DOI] [PMC free article] [PubMed] [Google Scholar]
- Deitmer J. W., Ellis D. Interactions between the regulation of the intracellular pH and sodium activity of sheep cardiac Purkinje fibres. J Physiol. 1980 Jul;304:471–488. doi: 10.1113/jphysiol.1980.sp013337. [DOI] [PMC free article] [PubMed] [Google Scholar]
- Ellis D. The effects of external cations and ouabain on the intracellular sodium activity of sheep heart Purkinje fibres. J Physiol. 1977 Dec;273(1):211–240. doi: 10.1113/jphysiol.1977.sp012090. [DOI] [PMC free article] [PubMed] [Google Scholar]
- Ellis D., Thomas R. C. Microelectrode measurement of the intracellular pH of mammalian heart cells. Nature. 1976 Jul 15;262(5565):224–225. doi: 10.1038/262224a0. [DOI] [PubMed] [Google Scholar]
- Frelin C., Vigne P., Lazdunski M. The role of the Na+/H+ exchange system in the regulation of the internal pH in cultured cardiac cells. Eur J Biochem. 1985 May 15;149(1):1–4. doi: 10.1111/j.1432-1033.1985.tb08884.x. [DOI] [PubMed] [Google Scholar]
- Green J., Yamaguchi D. T., Kleeman C. R., Muallem S. Cytosolic pH regulation in osteoblasts. Interaction of Na+ and H+ with the extracellular and intracellular faces of the Na+/H+ exchanger. J Gen Physiol. 1988 Aug;92(2):239–261. doi: 10.1085/jgp.92.2.239. [DOI] [PMC free article] [PubMed] [Google Scholar]
- Green R. D., Frelin C., Vigne P., Lazdunski M. The activity of the Na+/H+ antiporter in cultured cardiac cells is dependent on the culture conditions used. FEBS Lett. 1986 Feb 3;196(1):163–166. doi: 10.1016/0014-5793(86)80234-4. [DOI] [PubMed] [Google Scholar]
- Grinstein S., Cohen S., Rothstein A. Cytoplasmic pH regulation in thymic lymphocytes by an amiloride-sensitive Na+/H+ antiport. J Gen Physiol. 1984 Mar;83(3):341–369. doi: 10.1085/jgp.83.3.341. [DOI] [PMC free article] [PubMed] [Google Scholar]
- Jean T., Frelin C., Vigne P., Barbry P., Lazdunski M. Biochemical properties of the Na+/H+ exchange system in rat brain synaptosomes. Interdependence of internal and external pH control of the exchange activity. J Biol Chem. 1985 Aug 15;260(17):9678–9684. [PubMed] [Google Scholar]
- Kaila K., Vaughan-Jones R. D. Influence of sodium-hydrogen exchange on intracellular pH, sodium and tension in sheep cardiac Purkinje fibres. J Physiol. 1987 Sep;390:93–118. doi: 10.1113/jphysiol.1987.sp016688. [DOI] [PMC free article] [PubMed] [Google Scholar]
- Micro-electrode measurement of the intracellular pH and buffering power of mouse soleus muscle fibres. J Physiol. 1977 Jun;267(3):791–810. doi: 10.1113/jphysiol.1977.sp011838. [DOI] [PMC free article] [PubMed] [Google Scholar]
- Piwnica-Worms D., Jacob R., Horres C. R., Lieberman M. Na/H exchange in cultured chick heart cells. pHi regulation. J Gen Physiol. 1985 Jan;85(1):43–64. doi: 10.1085/jgp.85.1.43. [DOI] [PMC free article] [PubMed] [Google Scholar]
- Roos A., Boron W. F. Intracellular pH. Physiol Rev. 1981 Apr;61(2):296–434. doi: 10.1152/physrev.1981.61.2.296. [DOI] [PubMed] [Google Scholar]
- Thomas R. C. Intracellular pH of snail neurones measured with a new pH-sensitive glass mirco-electrode. J Physiol. 1974 Apr;238(1):159–180. doi: 10.1113/jphysiol.1974.sp010516. [DOI] [PMC free article] [PubMed] [Google Scholar]
- Tolkovsky A. M., Richards C. D. Na+/H+ exchange is the major mechanism of pH regulation in cultured sympathetic neurons: measurements in single cell bodies and neurites using a fluorescent pH indicator. Neuroscience. 1987 Sep;22(3):1093–1102. doi: 10.1016/0306-4522(87)92984-8. [DOI] [PubMed] [Google Scholar]
- Vanheel B., de Hemptinne A., Leusen I. Influence of surface pH on intracellular pH regulation in cardiac and skeletal muscle. Am J Physiol. 1986 May;250(5 Pt 1):C748–C760. doi: 10.1152/ajpcell.1986.250.5.C748. [DOI] [PubMed] [Google Scholar]
- Vaughan-Jones R. D. Regulation of intracellular pH in cardiac muscle. Ciba Found Symp. 1988;139:23–46. doi: 10.1002/9780470513699.ch3. [DOI] [PubMed] [Google Scholar]
- Vaughan-Jones R. D., Wu M. L., Bountra C. Sodium-hydrogen exchange and its role in controlling contractility during acidosis in cardiac muscle. Mol Cell Biochem. 1989 Sep 7;89(2):157–162. doi: 10.1007/BF00220769. [DOI] [PubMed] [Google Scholar]
- Vaughan-Jones R. D., Wu M. L. pH dependence of intrinsic H+ buffering power in the sheep cardiac Purkinje fibre. J Physiol. 1990 Jun;425:429–448. doi: 10.1113/jphysiol.1990.sp018112. [DOI] [PMC free article] [PubMed] [Google Scholar]
- Vigne P., Frelin C., Lazdunski M. The amiloride-sensitive Na+/H+ exchange system in skeletal muscle cells in culture. J Biol Chem. 1982 Aug 25;257(16):9394–9400. [PubMed] [Google Scholar]
- Weissberg P. L., Little P. J., Cragoe E. J., Jr, Bobik A. The pH of spontaneously beating cultured rat heart cells is regulated by an ATP-calmodulin-dependent Na+/H+ antiport. Circ Res. 1989 Apr;64(4):676–685. doi: 10.1161/01.res.64.4.676. [DOI] [PubMed] [Google Scholar]