Abstract
1. The dark current and responses to dim flashes were recorded with the suction pipette technique from single rods in pieces of bull-frog retina taken from either the dorsal porphyropsin or the ventral rhodopsin field. 2. The composition of visual pigment in the rods was determined by microspectrophotometry. Rods from the dorsal pieces contained 70-88% porphyropsin523 mixed with rhodopsin502. The ventral rods contained almost pure rhodopsin, any possible admixture of porphyropsin being below the level of detectability (less than 5%). 3. In most cells, the responses to dim flashes were well fitted by a four-stage linear filter model, with no systematic differences in the response kinetics of porphyropsin and rhodopsin rods. The amplitude of saturated responses varied between 8 and 55 pA and that of responses to single isomerizations between 0.4 and 3.5 pA. 4. In porphyropsin rods, discrete events similar to the response to one photoisomerization were clearly seen in complete darkness. The dark current amplitude histogram was fitted by a convolution of the probability densities for the Gaussian continuous noise component and the averaged dim-flash response waveform. This allows estimation of the frequency and amplitude of discrete events and the standard deviation of the continuous component. The mean frequency of discrete dark events thus obtained from six porphyropsin cells was 0.057 rod-1 s-1 at 18 degrees C. 5. In rhodopsin rods, the dark current amplitude histogram appeared completely symmetrical, indicating that the frequency of discrete events must be lower than 0.005 rod-1 s-1 (except in one rod where it was 0.006 events rod-1 s-1). Per molecule of rhodopsin, the events are then at least 5 times rarer than reported for toad rhodopsin rods at the same temperature. 6. The low rate of isomerization-like 'dark' events in bull-frog rhodopsin rods shows, firstly, that results cannot be generalized across species even for rhodopsins which appear spectrally identical. Secondly, it suggests that these events need not (in an evolutionary sense) constitute an irreducible noise factor which must set the ultimate limit to the sensitivity of dark-adapted vision. 7. The difference between porphyropsin and rhodopsin rods shows that, given (presumably) the same opsin, the pigment utilizing retinal2 and absorbing maximally at longer wavelengths produces more noise. The signal/noise ratio attained in the photoreceptor may be an important factor in the natural selection of visual pigments.
Full text
PDF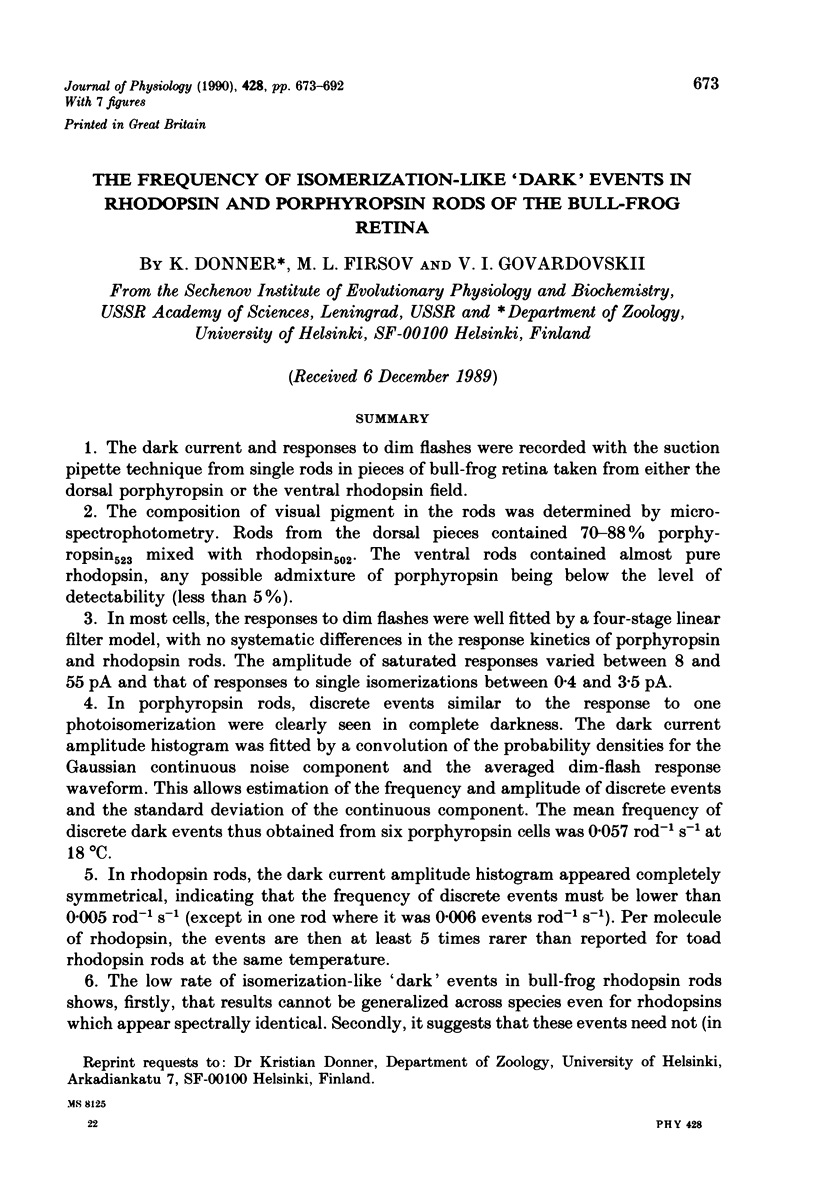
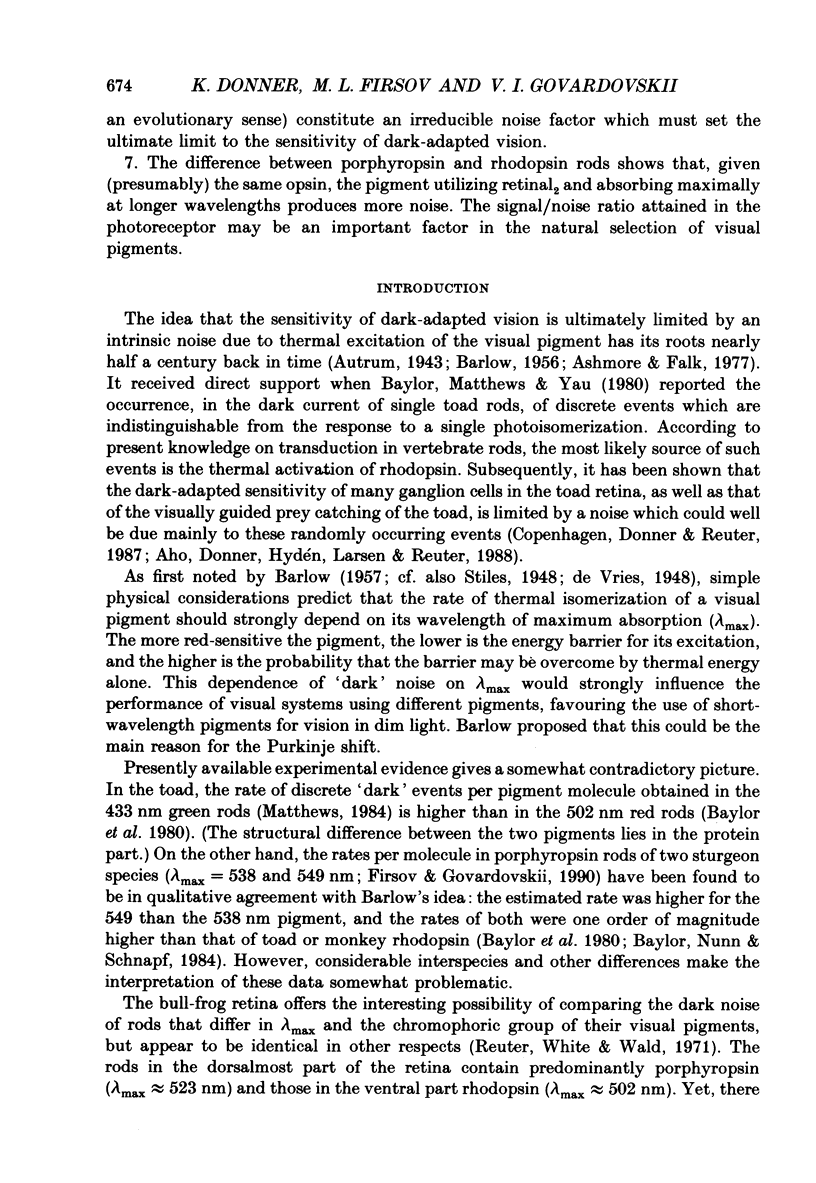
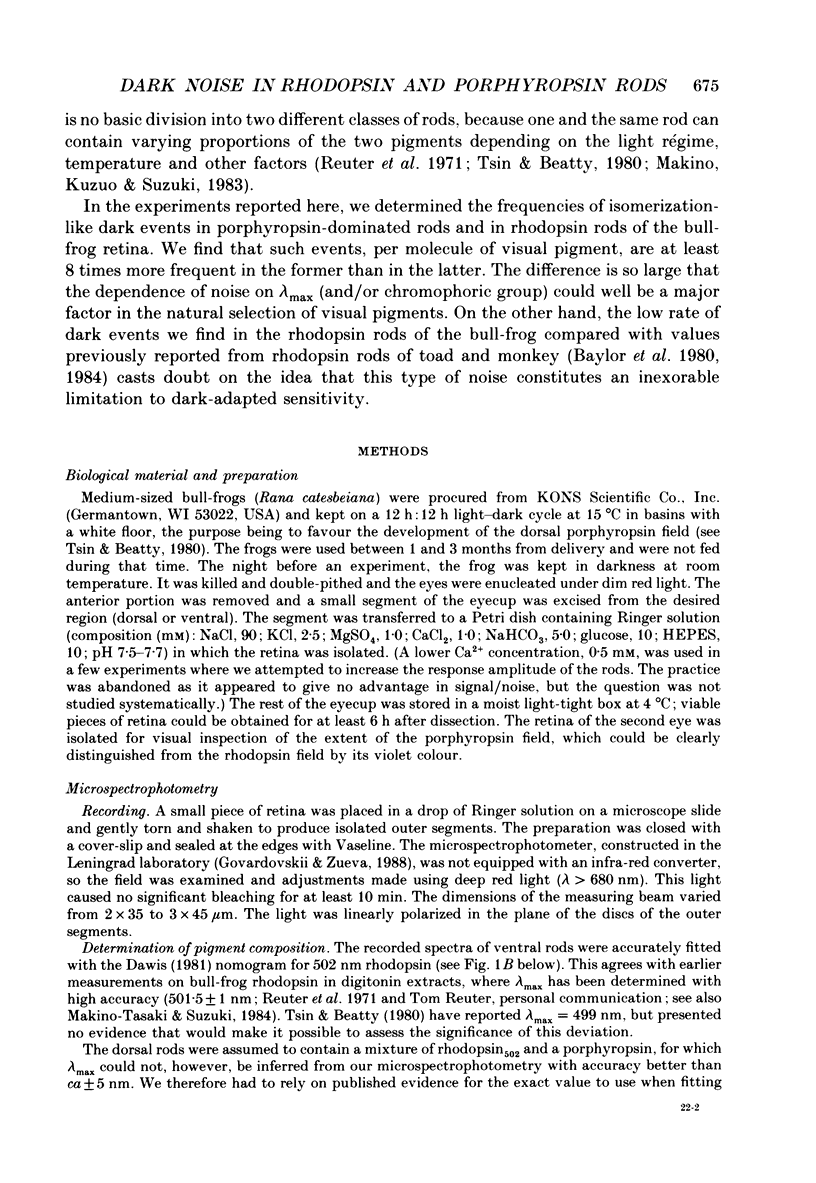
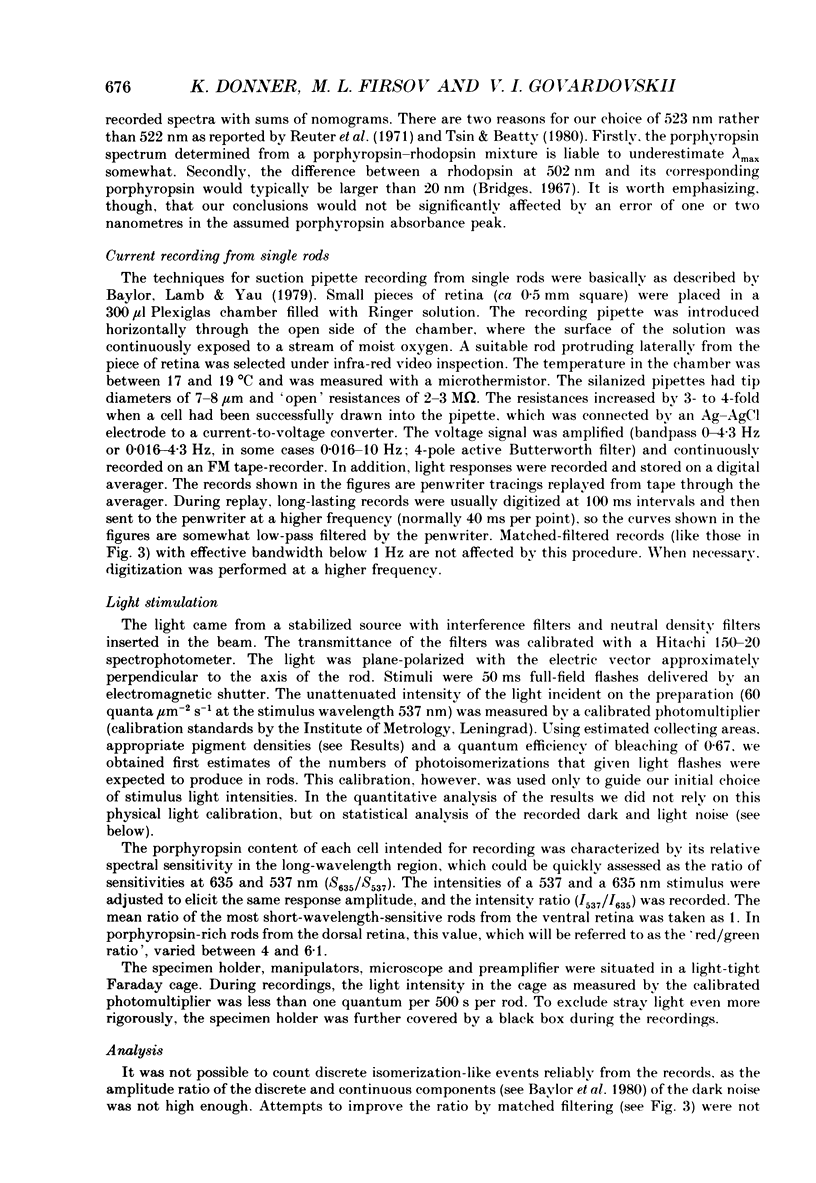
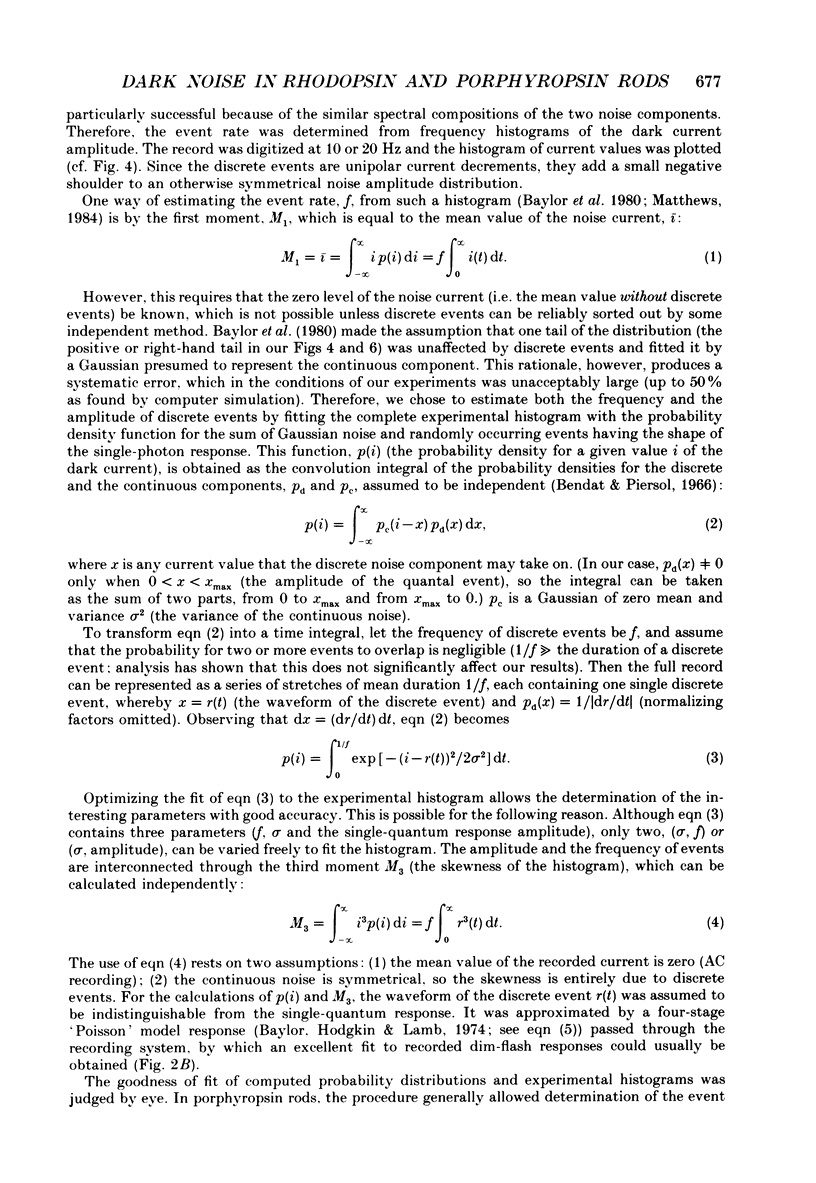
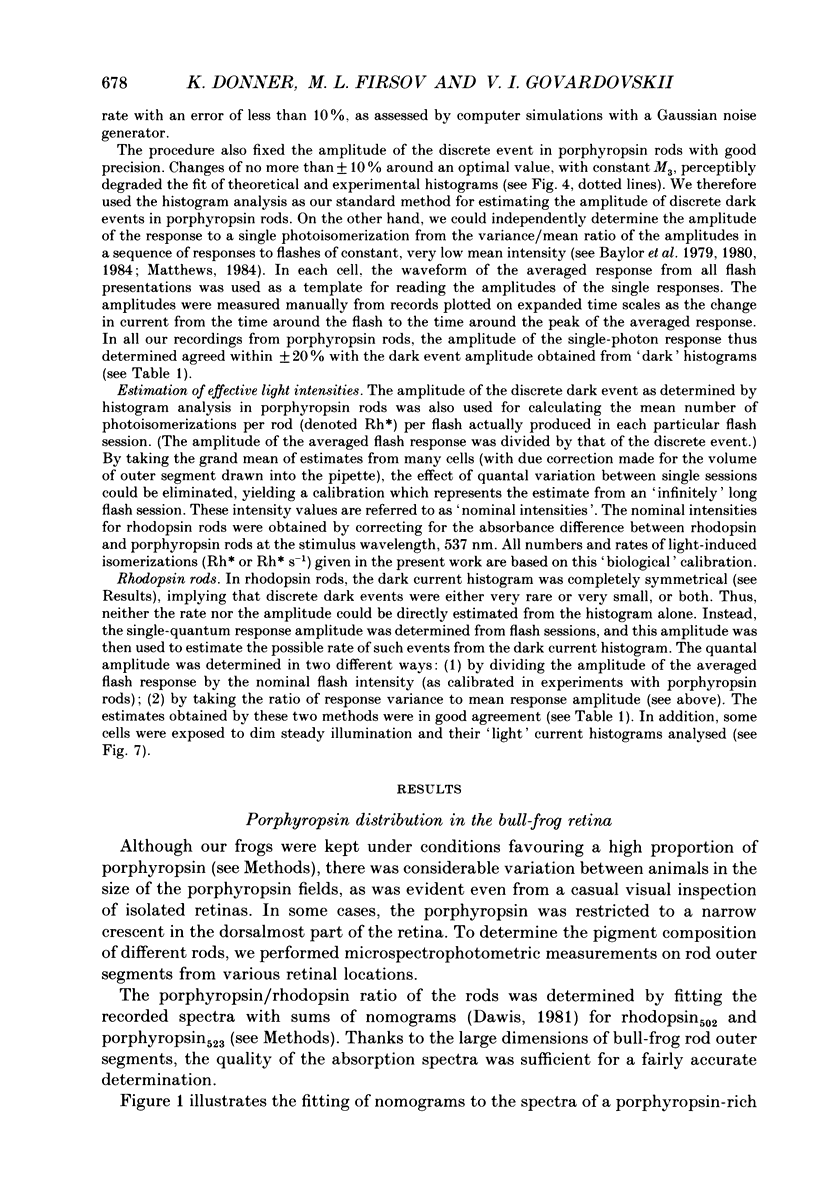
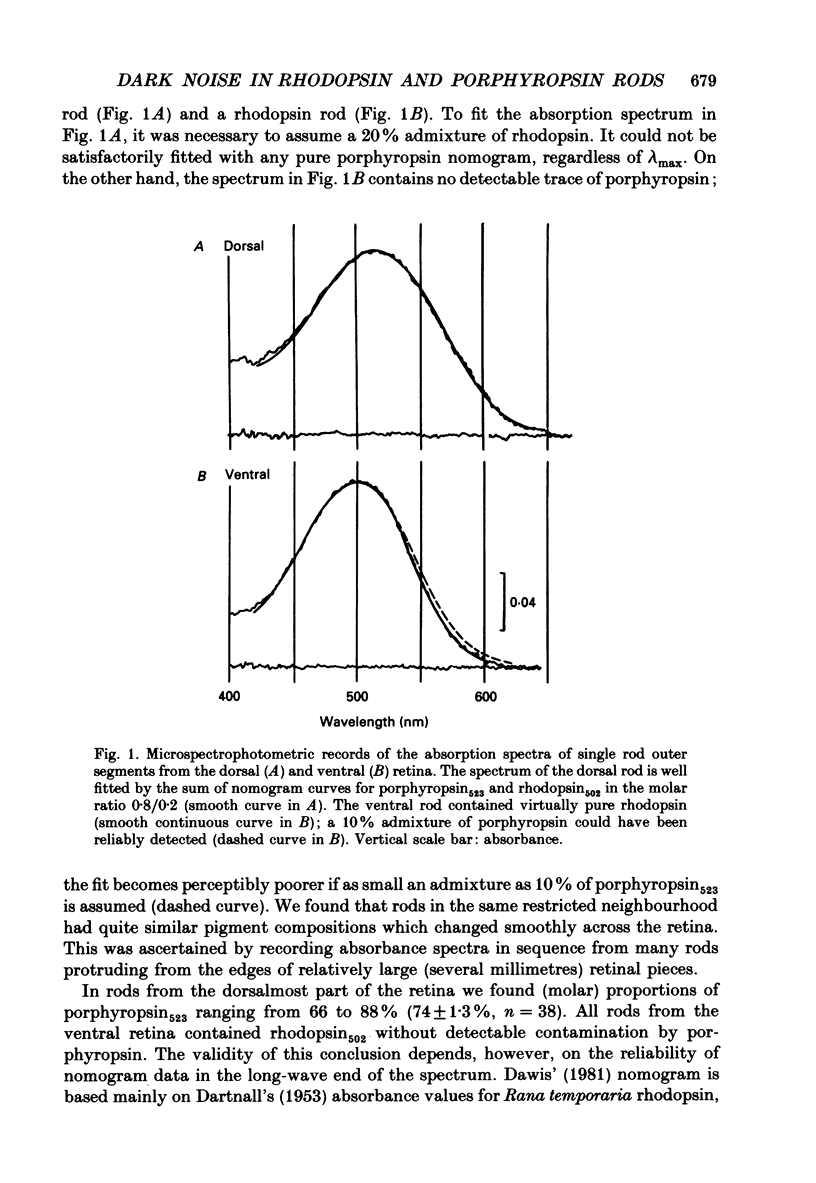
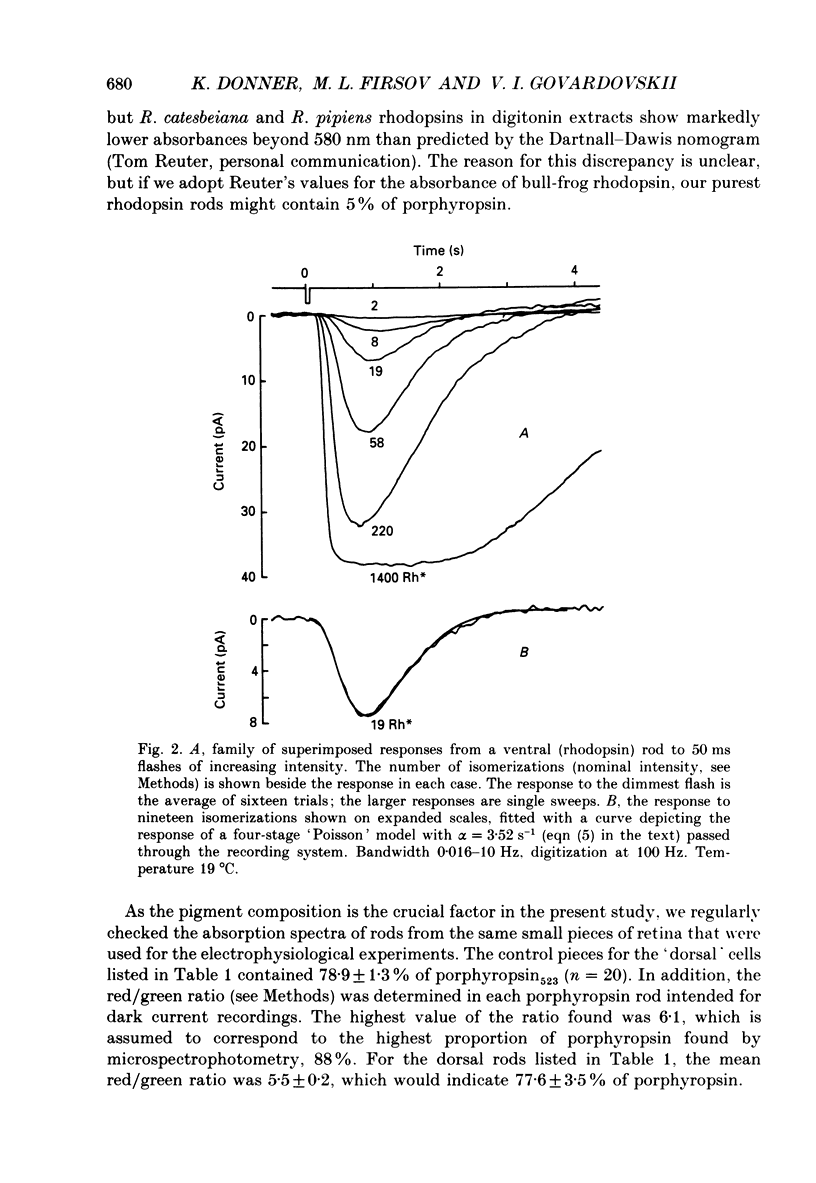
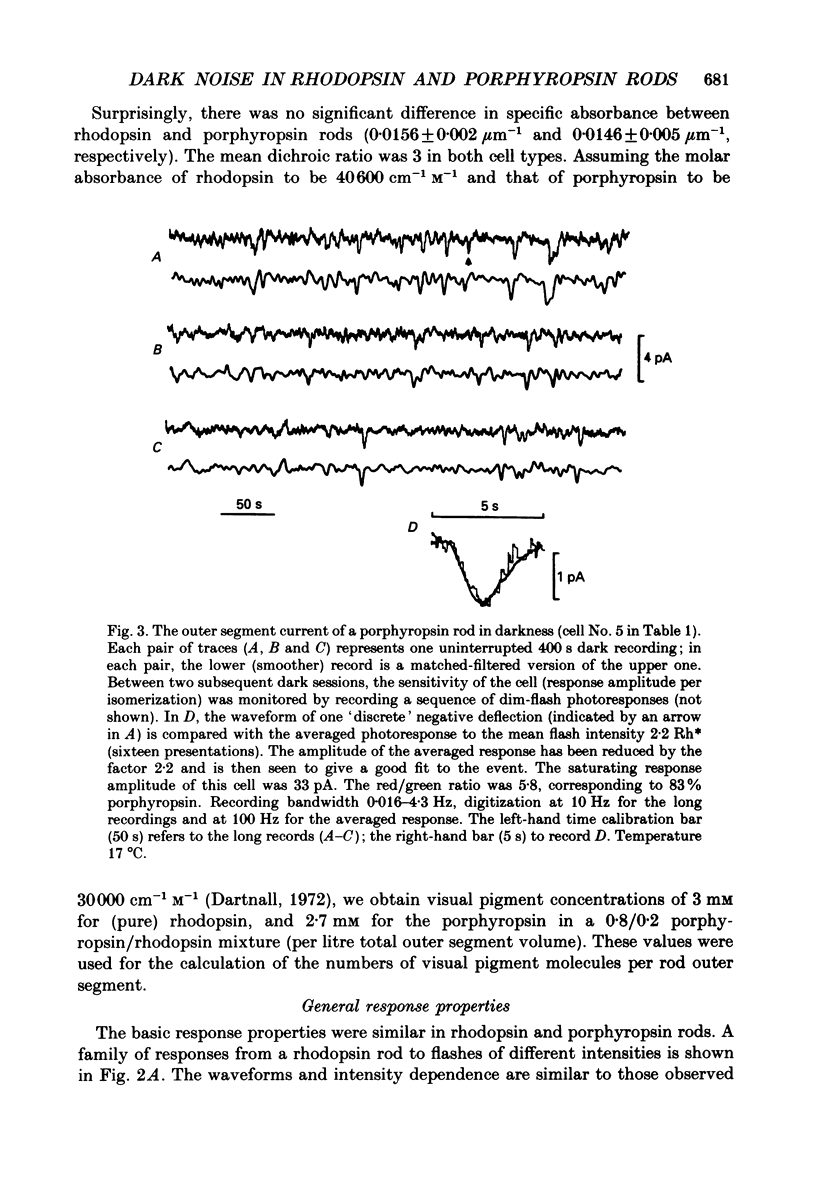
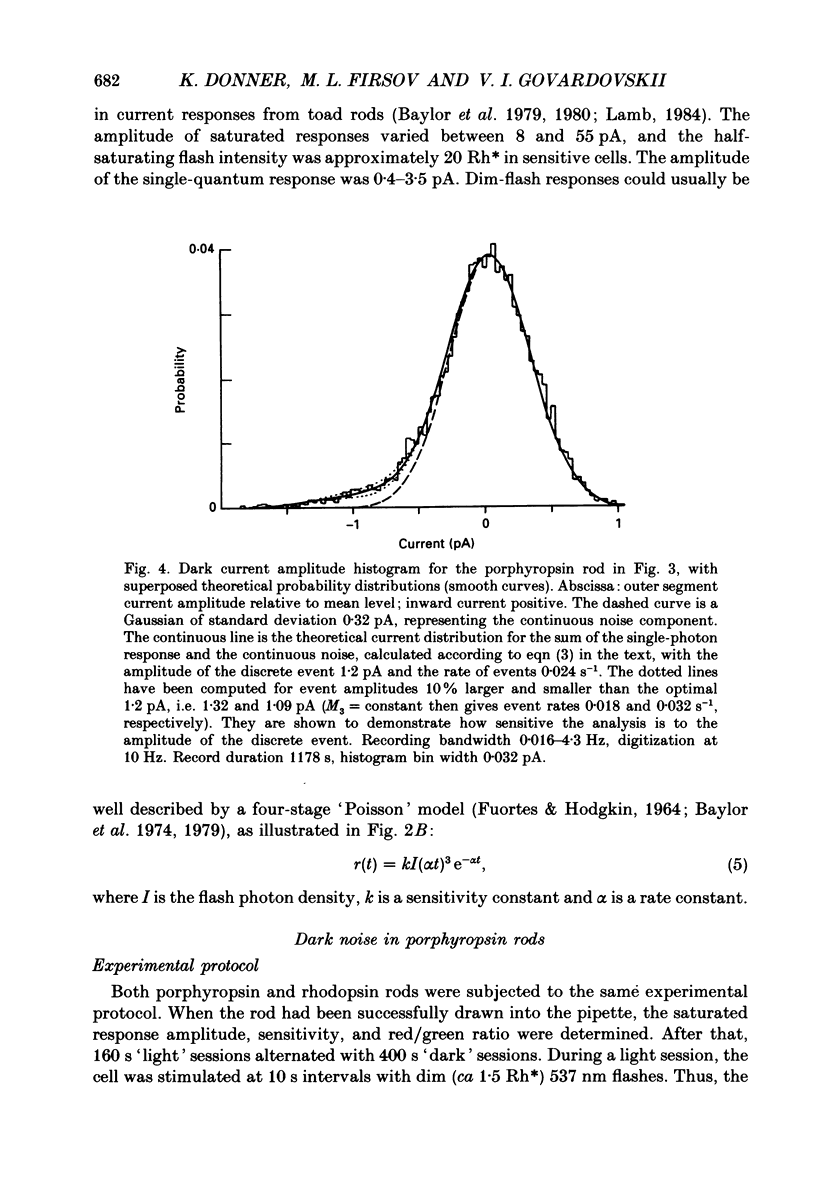
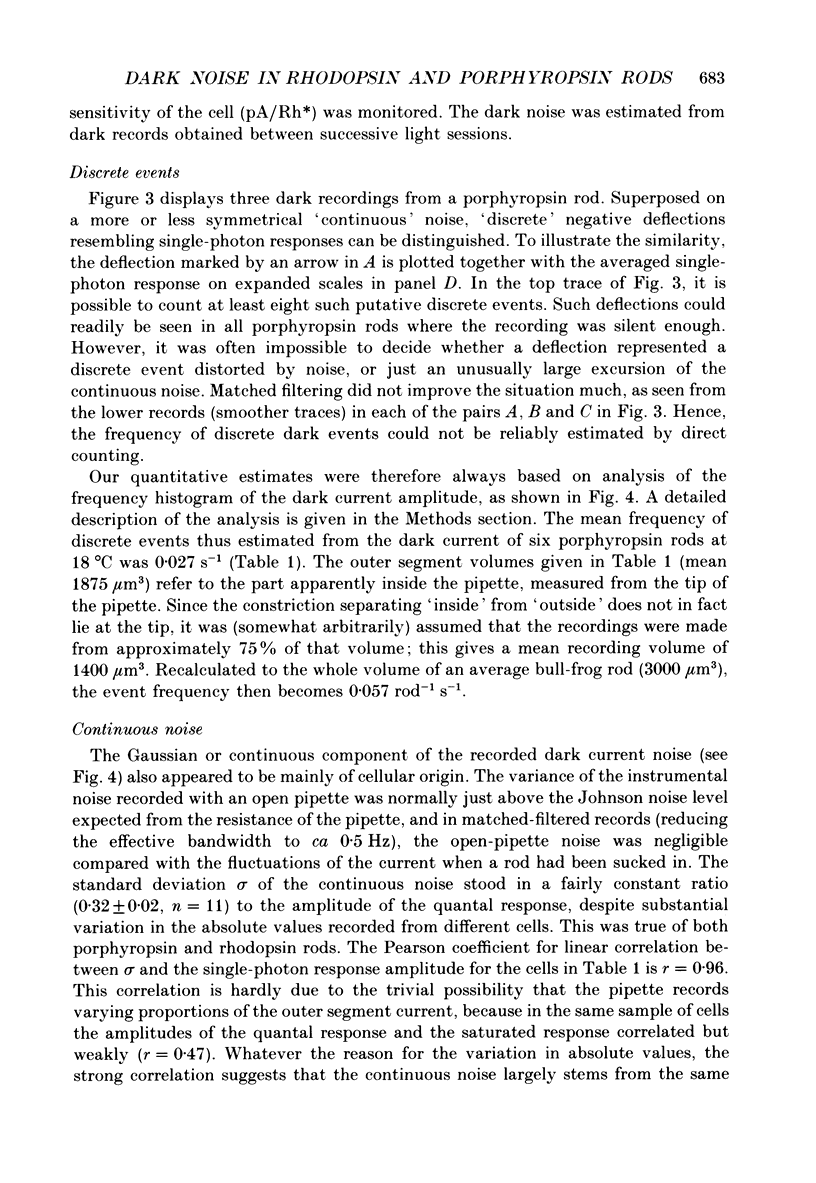
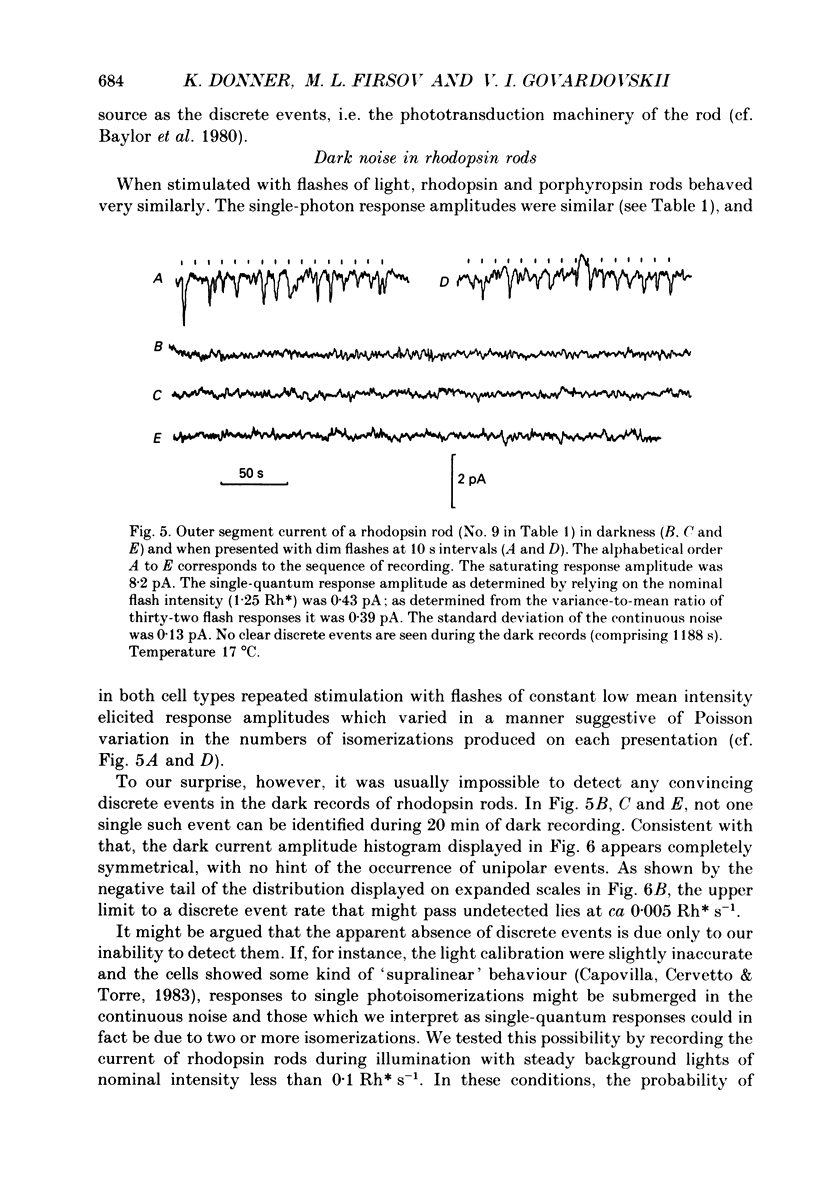
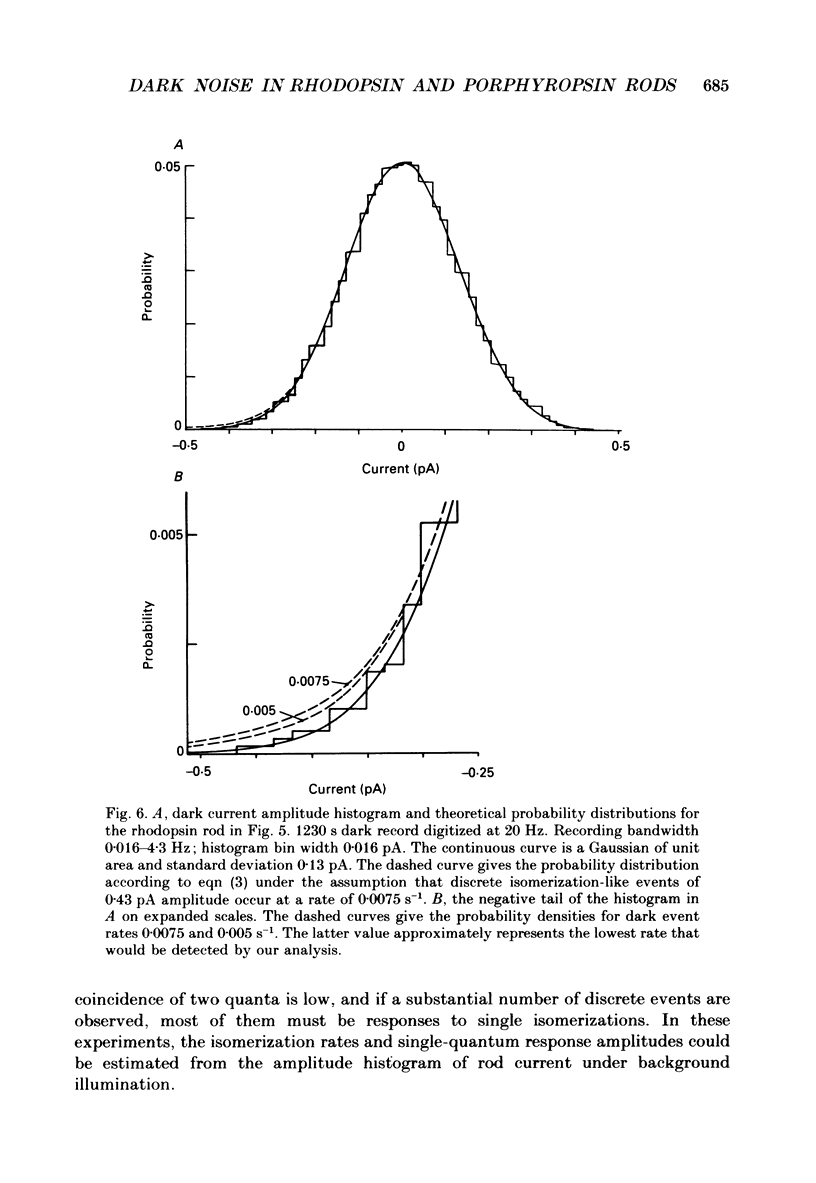
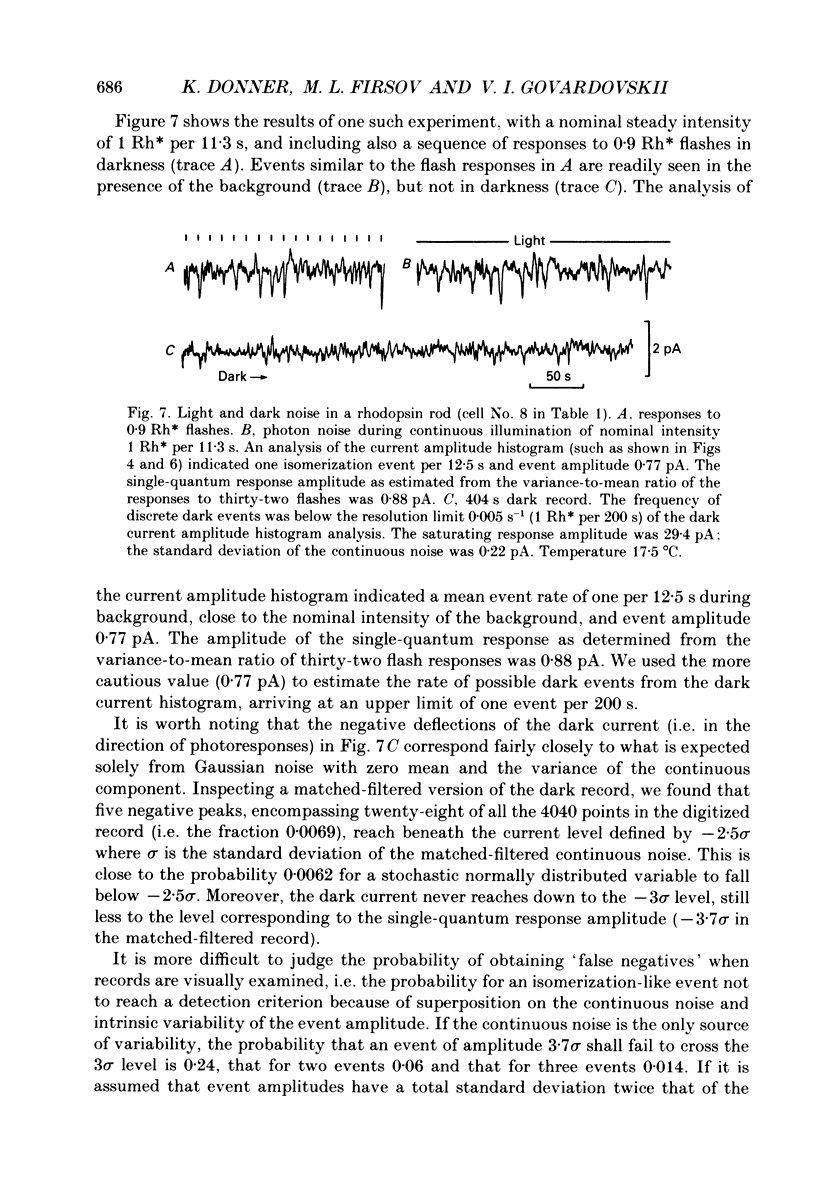
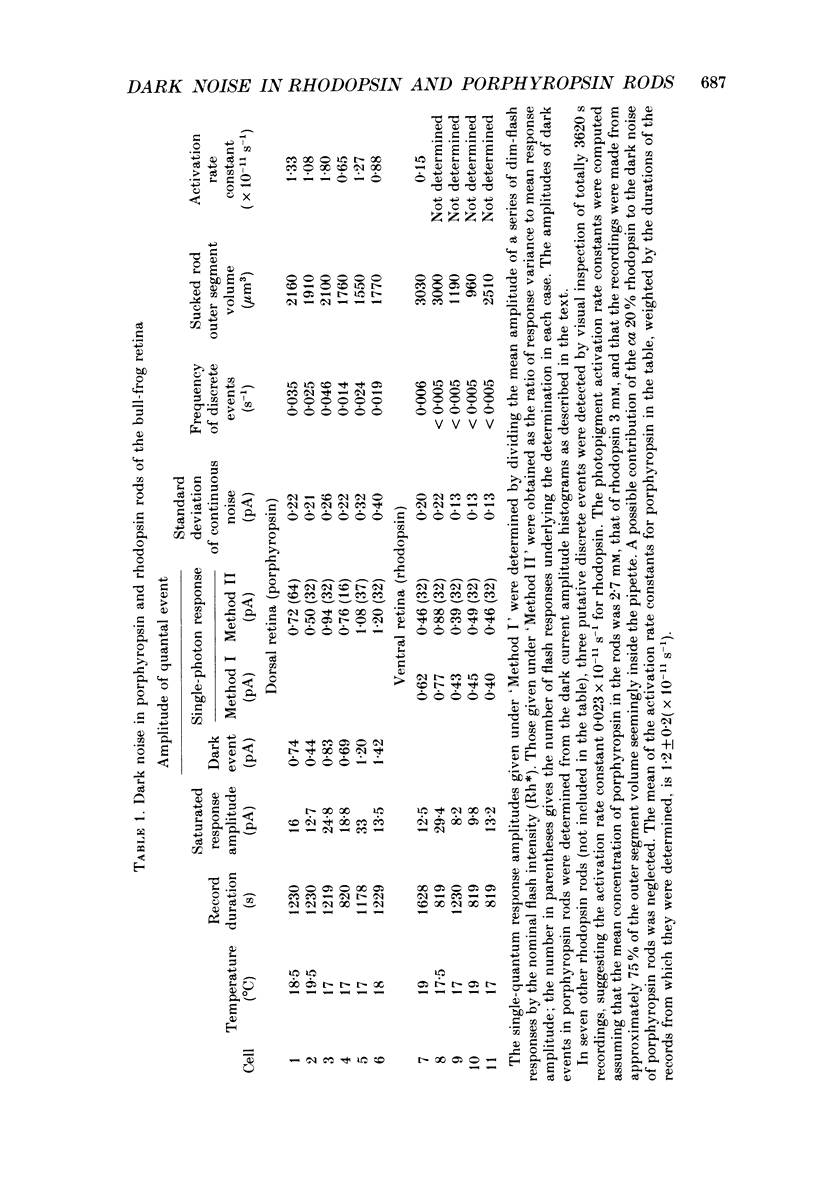
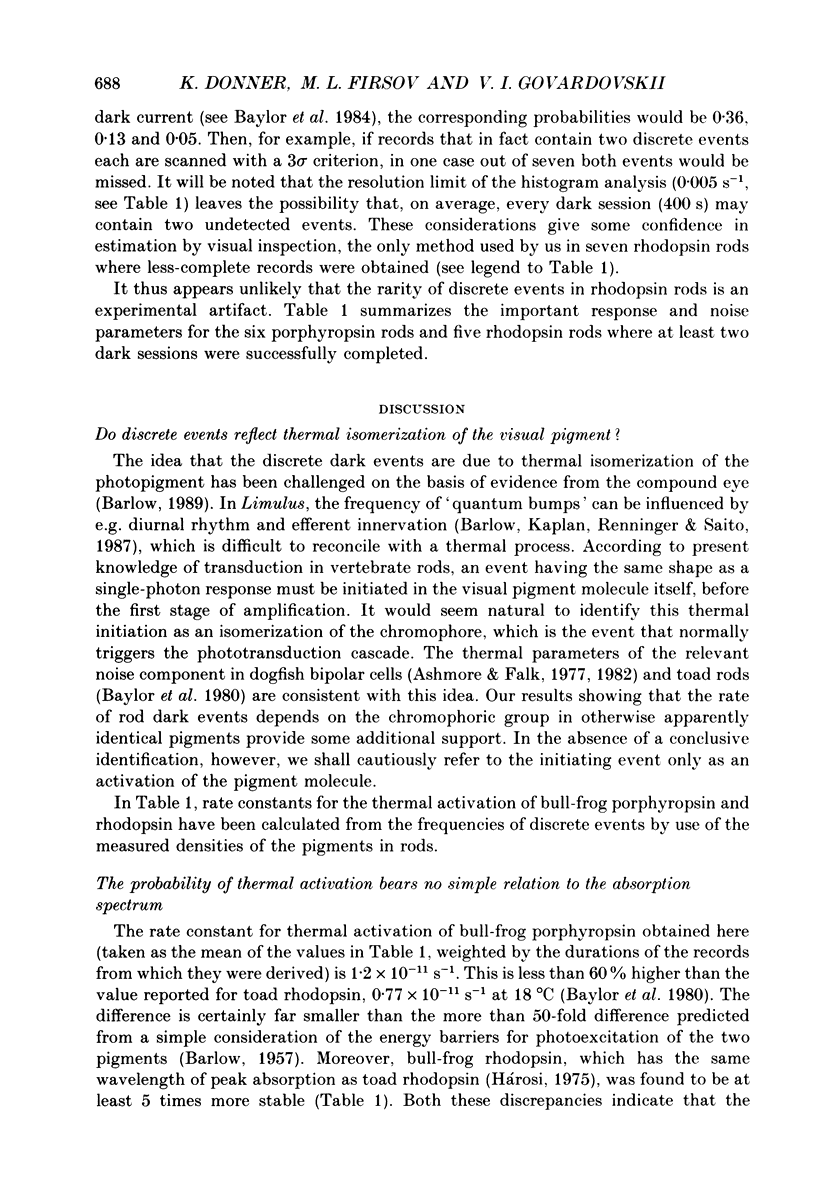
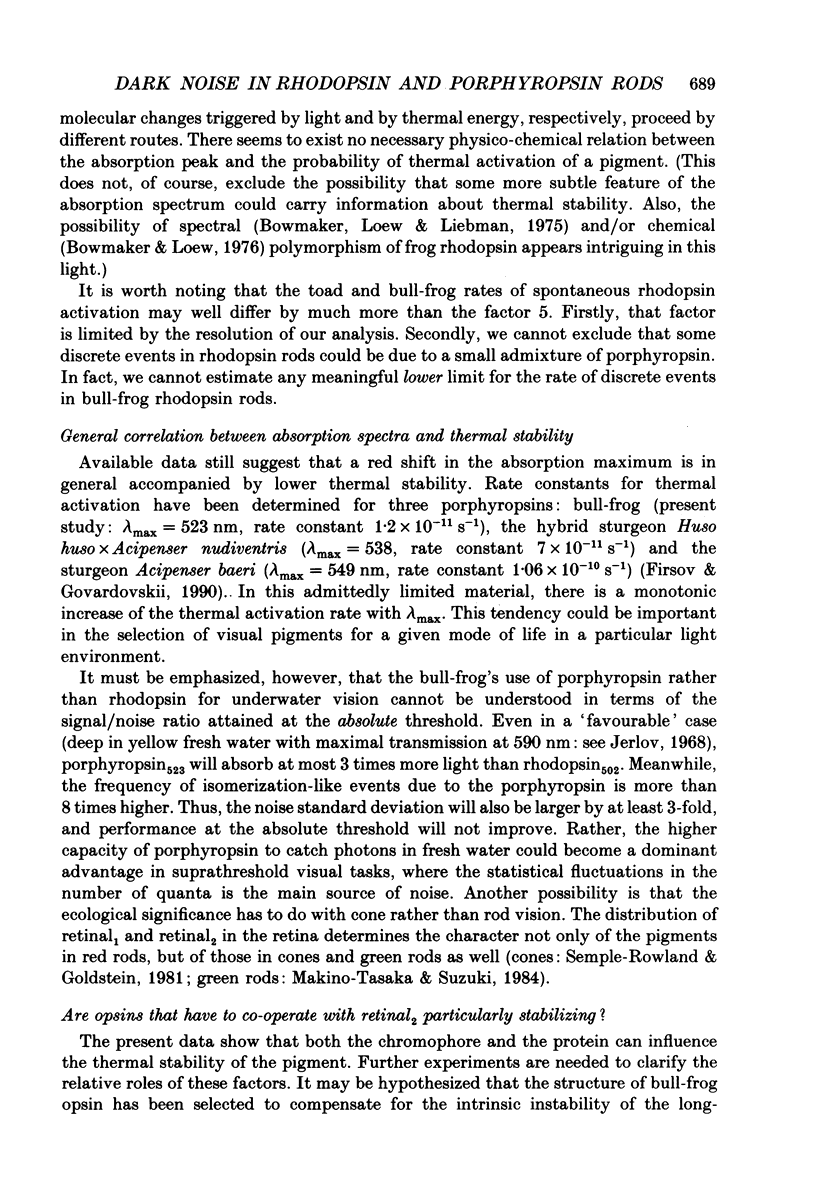
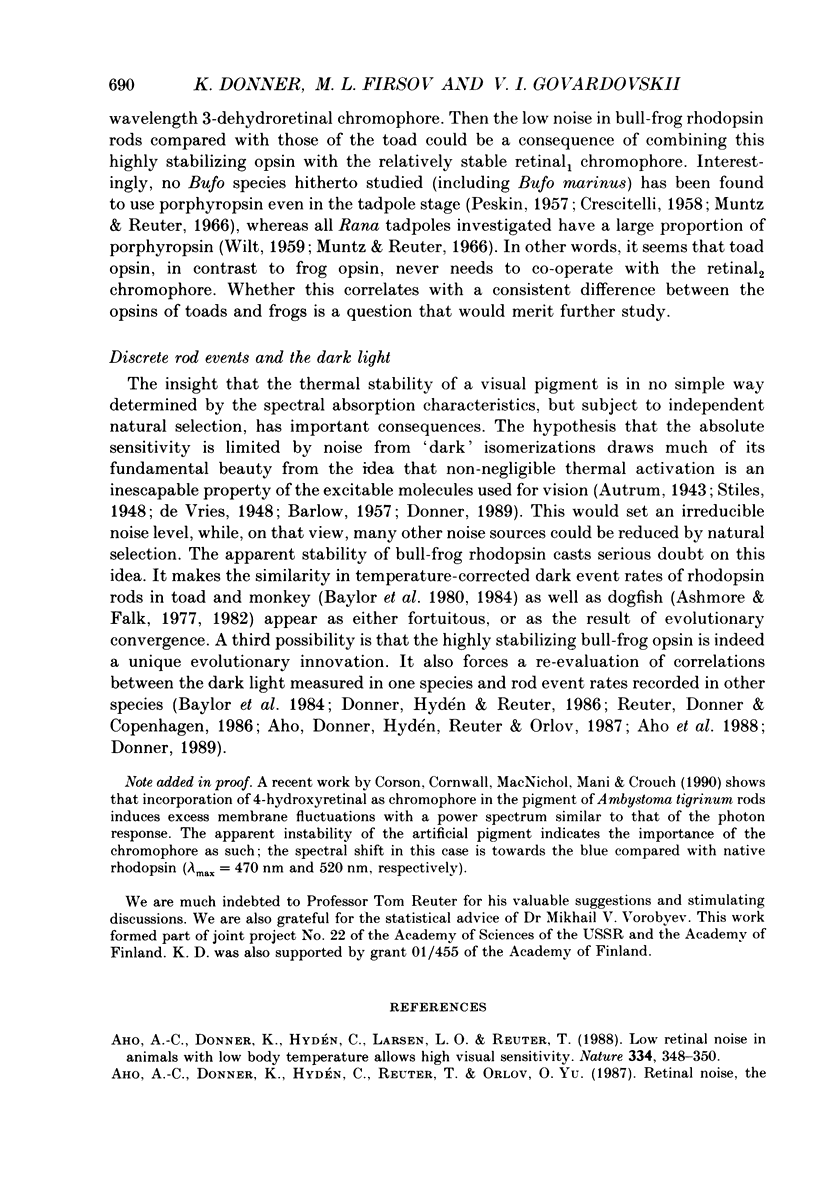
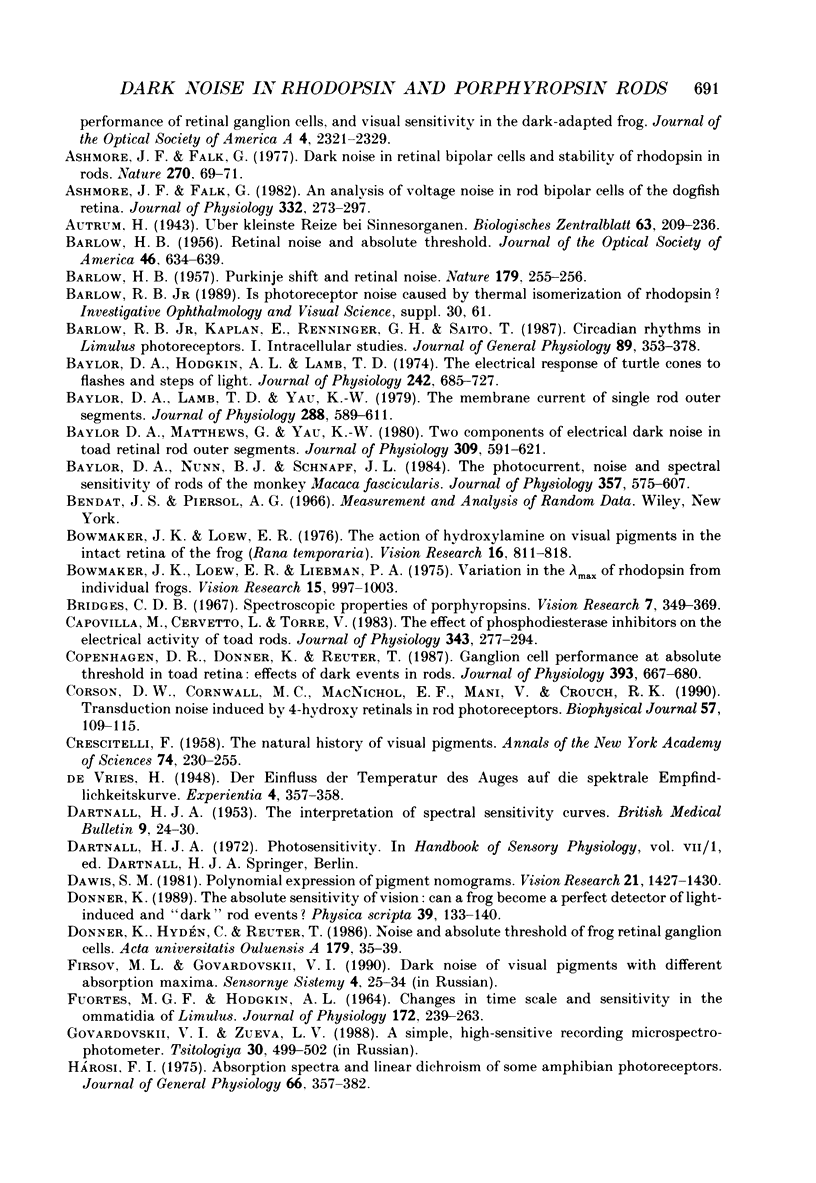
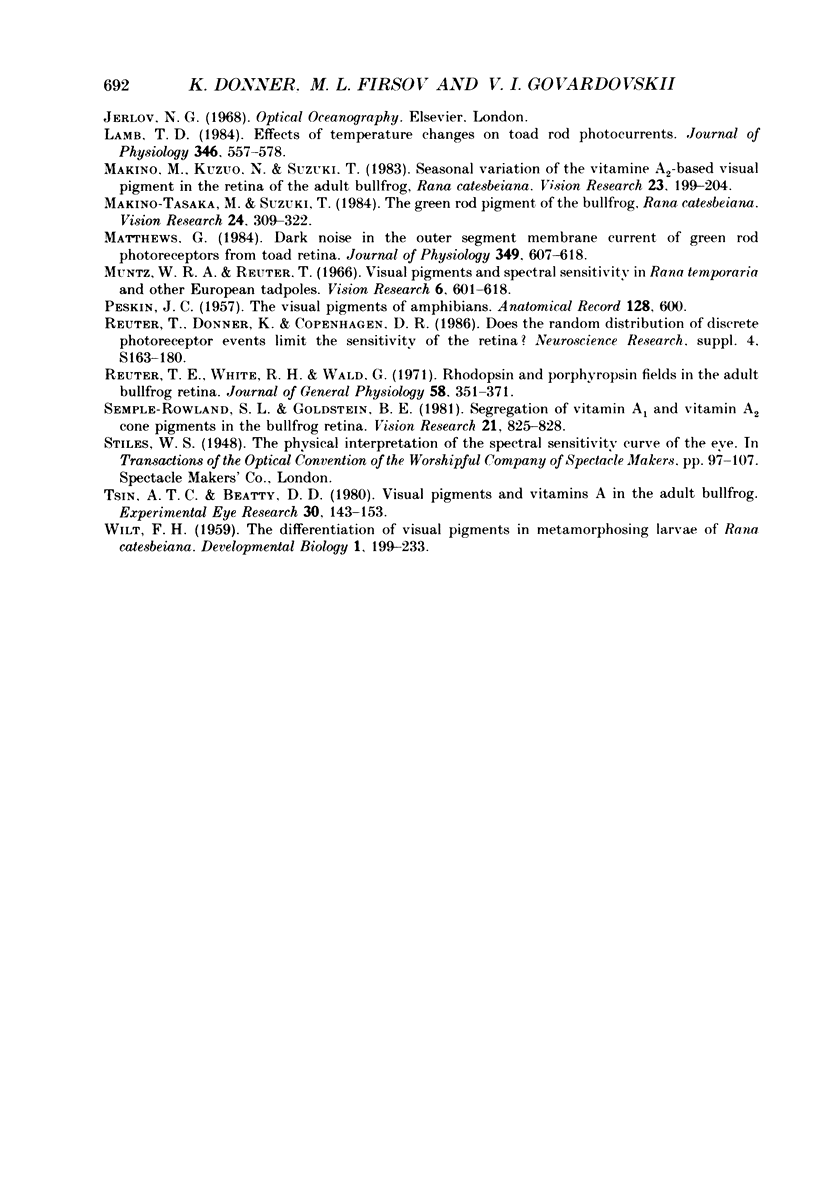
Selected References
These references are in PubMed. This may not be the complete list of references from this article.
- Aho A. C., Donner K., Hydén C., Larsen L. O., Reuter T. Low retinal noise in animals with low body temperature allows high visual sensitivity. Nature. 1988 Jul 28;334(6180):348–350. doi: 10.1038/334348a0. [DOI] [PubMed] [Google Scholar]
- Ashmore J. F., Falk G. An analysis of voltage noise in rod bipolar cells of the dogfish retina. J Physiol. 1982 Nov;332:273–297. doi: 10.1113/jphysiol.1982.sp014413. [DOI] [PMC free article] [PubMed] [Google Scholar]
- Ashmore J. F., Falk G. Dark noise in retinal bipolar cells and stability of rhodopsin in rods. Nature. 1977 Nov 3;270(5632):69–71. doi: 10.1038/270069a0. [DOI] [PubMed] [Google Scholar]
- BARLOW H. B. Purkinje shift and retinal noise. Nature. 1957 Feb 2;179(4553):255–256. doi: 10.1038/179255b0. [DOI] [PubMed] [Google Scholar]
- BARLOW H. B. Retinal noise and absolute threshold. J Opt Soc Am. 1956 Aug;46(8):634–639. doi: 10.1364/josa.46.000634. [DOI] [PubMed] [Google Scholar]
- Barlow R. B., Jr, Kaplan E., Renninger G. H., Saito T. Circadian rhythms in Limulus photoreceptors. I. Intracellular studies. J Gen Physiol. 1987 Mar;89(3):353–378. doi: 10.1085/jgp.89.3.353. [DOI] [PMC free article] [PubMed] [Google Scholar]
- Baylor D. A., Hodgkin A. L., Lamb T. D. The electrical response of turtle cones to flashes and steps of light. J Physiol. 1974 Nov;242(3):685–727. doi: 10.1113/jphysiol.1974.sp010731. [DOI] [PMC free article] [PubMed] [Google Scholar]
- Baylor D. A., Lamb T. D., Yau K. W. The membrane current of single rod outer segments. J Physiol. 1979 Mar;288:589–611. [PMC free article] [PubMed] [Google Scholar]
- Baylor D. A., Matthews G., Yau K. W. Two components of electrical dark noise in toad retinal rod outer segments. J Physiol. 1980 Dec;309:591–621. doi: 10.1113/jphysiol.1980.sp013529. [DOI] [PMC free article] [PubMed] [Google Scholar]
- Baylor D. A., Nunn B. J., Schnapf J. L. The photocurrent, noise and spectral sensitivity of rods of the monkey Macaca fascicularis. J Physiol. 1984 Dec;357:575–607. doi: 10.1113/jphysiol.1984.sp015518. [DOI] [PMC free article] [PubMed] [Google Scholar]
- Bowmaker J. K., Loew E. R., Liebman P. A. Variation in the lambdamax of rhodopsin from individual frogs. Vision Res. 1975 Aug-Sep;15:997–1003. doi: 10.1016/0042-6989(75)90242-4. [DOI] [PubMed] [Google Scholar]
- Bowmaker J. K., Loew E. R. The action of hydroxylamine on visual pigments in the intact retina of the frog (Rana temporaria). Vision Res. 1976;16(8):811–818. doi: 10.1016/0042-6989(76)90140-1. [DOI] [PubMed] [Google Scholar]
- Bridges C. D. Spectroscopic properties of porphyropsins. Vision Res. 1967 May;7(5):349–369. doi: 10.1016/0042-6989(67)90044-2. [DOI] [PubMed] [Google Scholar]
- CRESCITELLI F. The natural history of visual pigments. Ann N Y Acad Sci. 1959 Nov 12;74(2):230–255. doi: 10.1111/j.1749-6632.1958.tb39548.x. [DOI] [PubMed] [Google Scholar]
- Capovilla M., Cervetto L., Torre V. The effect of phosphodiesterase inhibitors on the electrical activity of toad rods. J Physiol. 1983 Oct;343:277–294. doi: 10.1113/jphysiol.1983.sp014892. [DOI] [PMC free article] [PubMed] [Google Scholar]
- Copenhagen D. R., Donner K., Reuter T. Ganglion cell performance at absolute threshold in toad retina: effects of dark events in rods. J Physiol. 1987 Dec;393:667–680. doi: 10.1113/jphysiol.1987.sp016847. [DOI] [PMC free article] [PubMed] [Google Scholar]
- Corson D. W., Cornwall M. C., MacNichol E. F., Mani V., Crouch R. K. Transduction noise induced by 4-hydroxy retinals in rod photoreceptors. Biophys J. 1990 Jan;57(1):109–115. doi: 10.1016/S0006-3495(90)82511-X. [DOI] [PMC free article] [PubMed] [Google Scholar]
- DARTNALL H. J. A. The interpretation of spectral sensitivity curves. Br Med Bull. 1953;9(1):24–30. doi: 10.1093/oxfordjournals.bmb.a074302. [DOI] [PubMed] [Google Scholar]
- DE VRIES H. Der Einfluss der Temperatur des Auges auf die spektrale Empfindlichkeitskurve. Experientia. 1948 Sep 15;4(9):357–357. doi: 10.1007/BF02164493. [DOI] [PubMed] [Google Scholar]
- Dawis S. M. Polynomial expressions of pigment nomograms. Vision Res. 1981;21(9):1427–1430. doi: 10.1016/0042-6989(81)90250-9. [DOI] [PubMed] [Google Scholar]
- FUORTES M. G., HODGKIN A. L. CHANGES IN TIME SCALE AND SENSITIVITY IN THE OMMATIDIA OF LIMULUS. J Physiol. 1964 Aug;172:239–263. doi: 10.1113/jphysiol.1964.sp007415. [DOI] [PMC free article] [PubMed] [Google Scholar]
- Govardovskii V. I., Zueva L. V. Prostoi vysokochuvstvitel'nyi registriruiushchii mikrospektrofotometr. Tsitologiia. 1988 Apr;30(4):499–502. [PubMed] [Google Scholar]
- Hárosi F. I. Absorption spectra and linear dichroism of some amphibian photoreceptors. J Gen Physiol. 1975 Sep;66(3):357–382. doi: 10.1085/jgp.66.3.357. [DOI] [PMC free article] [PubMed] [Google Scholar]
- Lamb T. D. Effects of temperature changes on toad rod photocurrents. J Physiol. 1984 Jan;346:557–578. doi: 10.1113/jphysiol.1984.sp015041. [DOI] [PMC free article] [PubMed] [Google Scholar]
- Makino-Tasaka M., Suzuki T. The green rod pigment of the bullfrog, Rana catesbeiana. Vision Res. 1984;24(4):309–322. doi: 10.1016/0042-6989(84)90056-7. [DOI] [PubMed] [Google Scholar]
- Makino M., Nagai K., Suzuki T. Seasonal variation of the vitamin A2-based visual pigment in the retina of adult bullfrog, Rana catesbeiana. Vision Res. 1983;23(2):199–204. doi: 10.1016/0042-6989(83)90143-8. [DOI] [PubMed] [Google Scholar]
- Matthews G. Dark noise in the outer segment membrane current of green rod photoreceptors from toad retina. J Physiol. 1984 Apr;349:607–618. doi: 10.1113/jphysiol.1984.sp015176. [DOI] [PMC free article] [PubMed] [Google Scholar]
- Muntz W. R., Reuter T. Visual pigments and spectral sensitivity in Rana temporaria and other European tadpoles. Vision Res. 1966 Dec;6(12):601–618. doi: 10.1016/0042-6989(66)90072-1. [DOI] [PubMed] [Google Scholar]
- Reuter T. E., White R. H., Wald G. Rhodopsin and porphyropsin fields in the adult bullfrog retina. J Gen Physiol. 1971 Oct;58(4):351–371. doi: 10.1085/jgp.58.4.351. [DOI] [PMC free article] [PubMed] [Google Scholar]
- Semple-Rowland S. L., Goldstein E. B. Segregation of vitamin A1 and vitamin A2 cone pigments in the bullfrog retina. Vision Res. 1981;21(6):825–828. doi: 10.1016/0042-6989(81)90181-4. [DOI] [PubMed] [Google Scholar]
- Tsin A. T., Beatty D. D. Visual pigments and vitamins A in the adult bullfrog. Exp Eye Res. 1980 Feb;30(2):143–153. doi: 10.1016/0014-4835(80)90108-6. [DOI] [PubMed] [Google Scholar]