Abstract
1. The rate constant of tension redevelopment (ktr) following a rapid release and subsequent re-extension of muscle length has been demonstrated to be Ca2+ sensitive and is thought to reflect the rate-limiting step in the cross-bridge cycle leading to the formation of the strongly bound, force-bearing state. The kinetics of this cross-bridge state transition were investigated at 15 degrees C over a wide range of Ca2+ concentrations while varying pH from 7.00 to 6.20 in rat slow-twitch soleus, rat fast-twitch superficial vastus lateralis (SVL) and rabbit fast-twitch psoas skinned single fibres. 2. At maximal levels of Ca2+ activation, ktr was unaffected by changes in pH from 7.00 to 6.20 while isometric tension was depressed to 0.60 +/- 0.02 P0 (mean +/- S.E.M.) at the low pH in fast-twitch fibres and to 0.78 +/- 0.01 P0 in slow-twitch fibres (P0 is the maximum isometric tension obtained at pH 7.00). 3. At reduced levels of Ca2+ activation, corresponding to pCa (-log [Ca2+]) greater than 5.0, ktr was markedly depressed in all fibre types when pH was lowered. The Ca2+ sensitivity of steady-state isometric tension was also reduced in all fibres at pH 6.20 compared to pH 7.00. 4. The results suggest that pH has a modulatory effect upon an apparent rate constant which is rate limiting in terms of the formation of the strongly bound, force-bearing cross-bridge state. This effect of altered pH may in part account for the reduction in the Ca2+ sensitivity of isometric force at low pH as well as the depression of the rate of rise of tension in living fibres during fatiguing stimulation.
Full text
PDF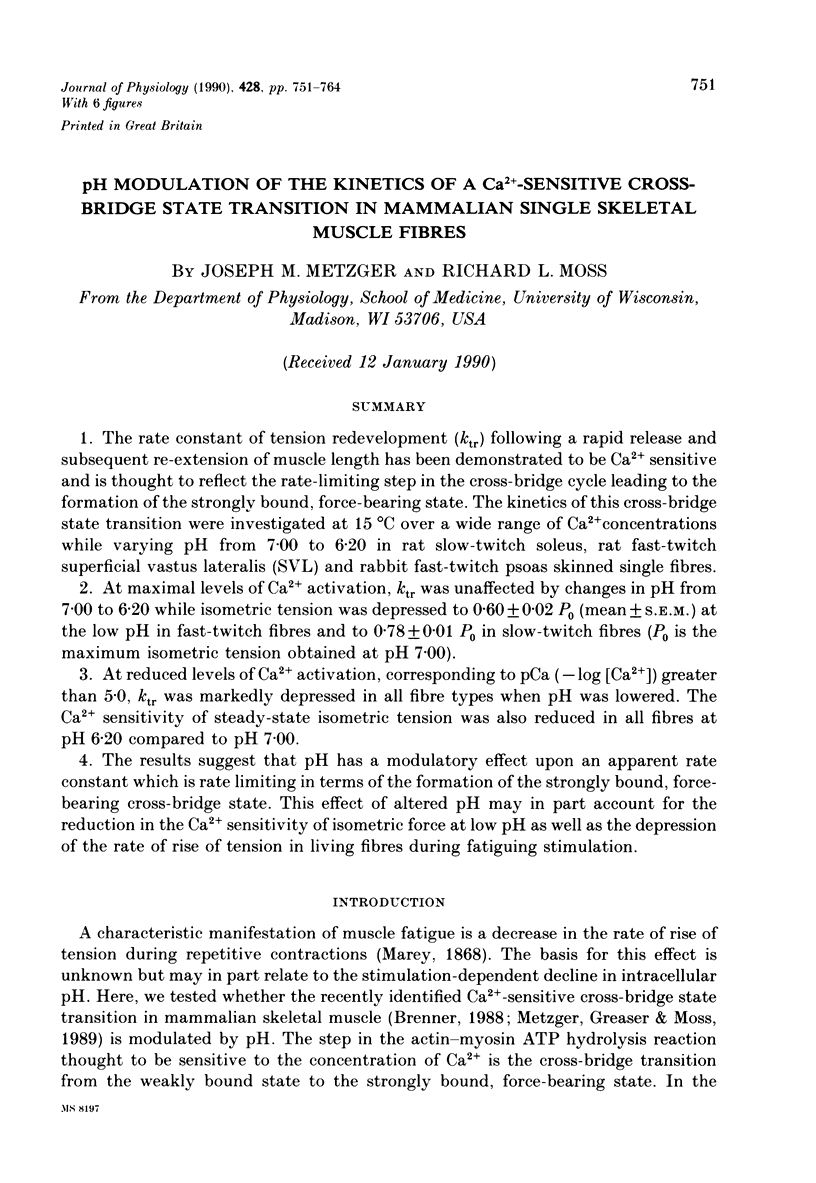
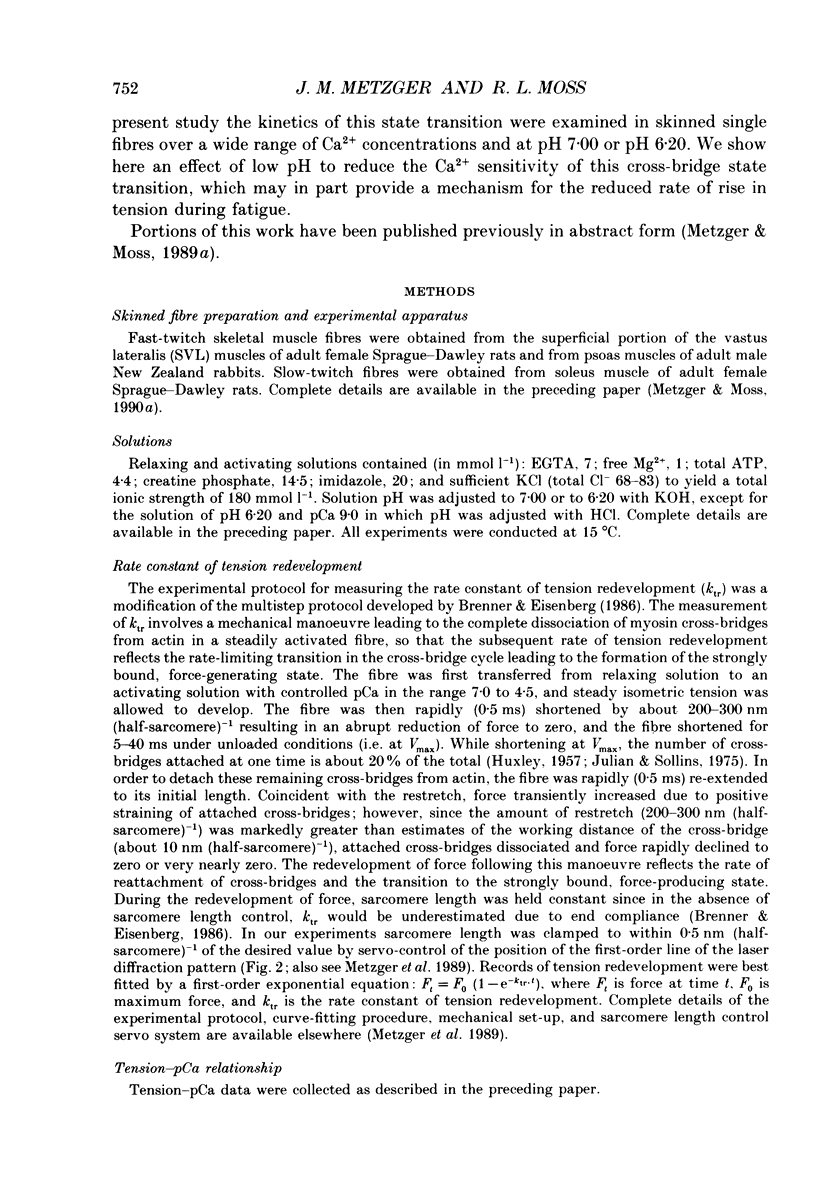
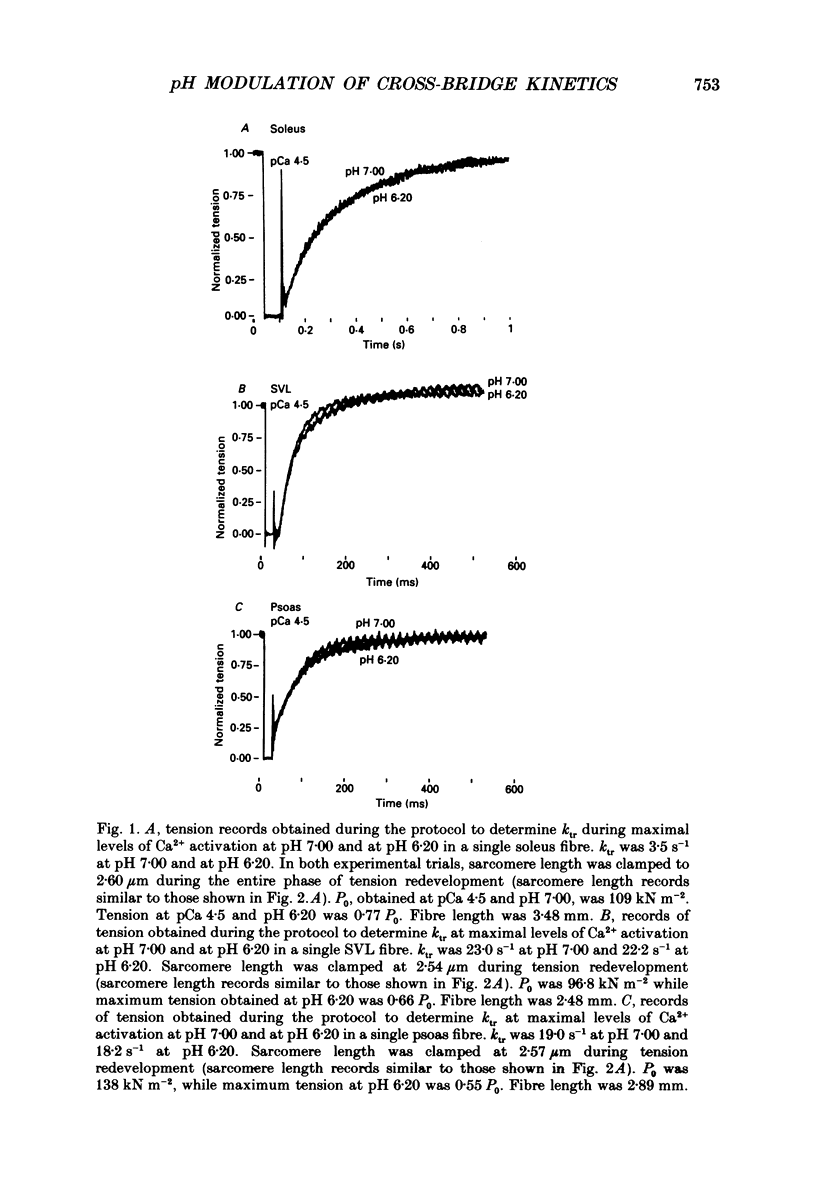
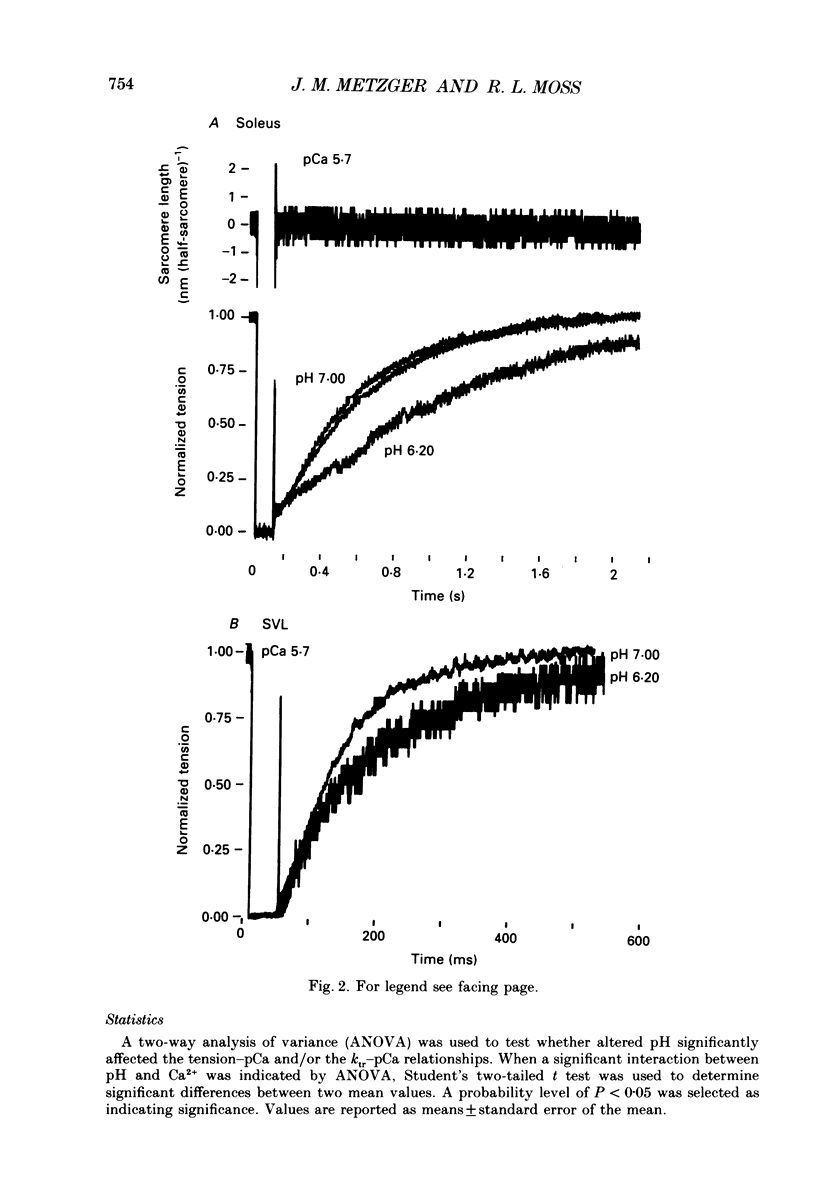
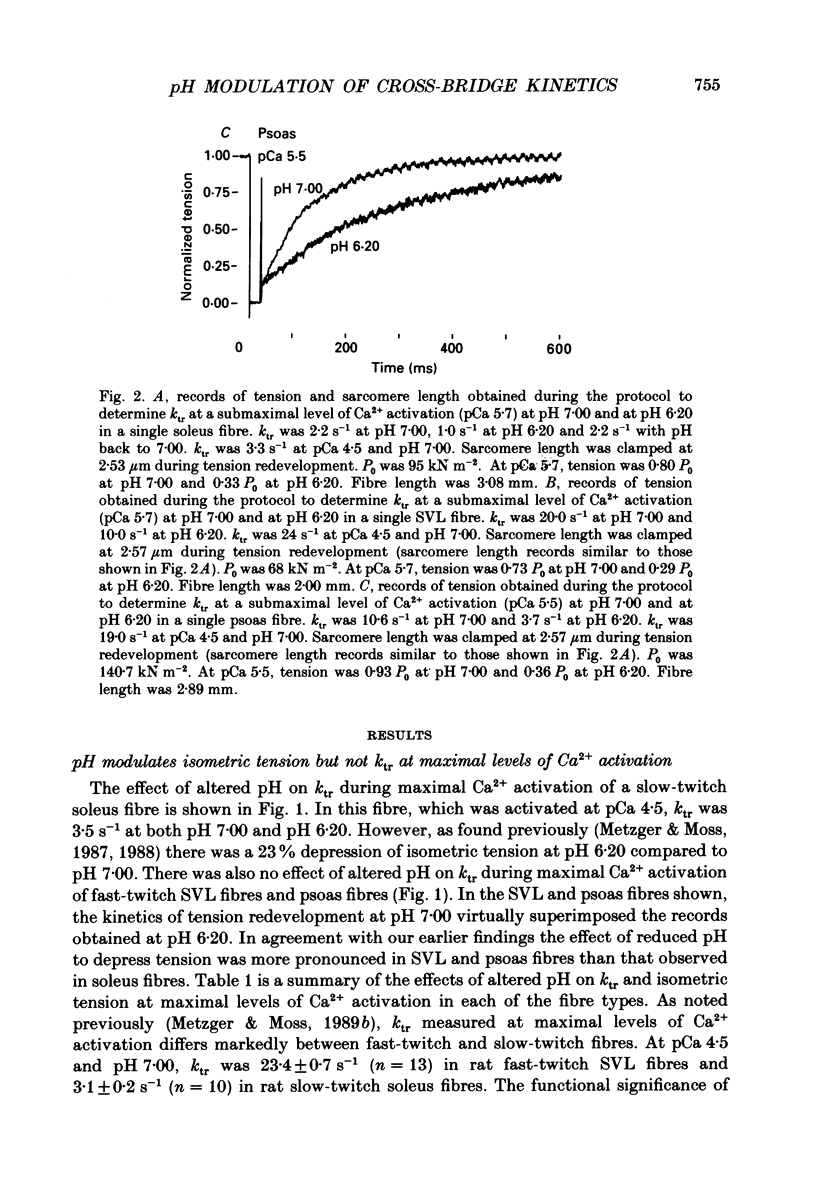
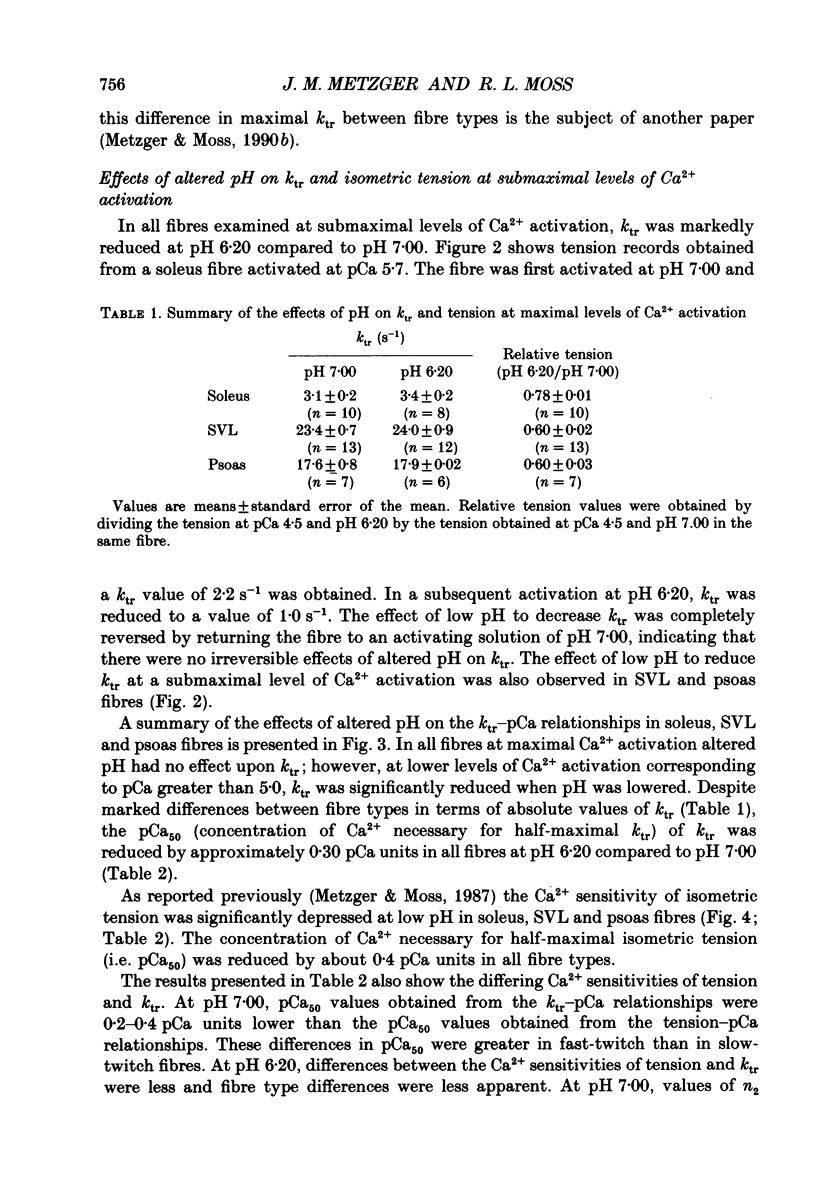
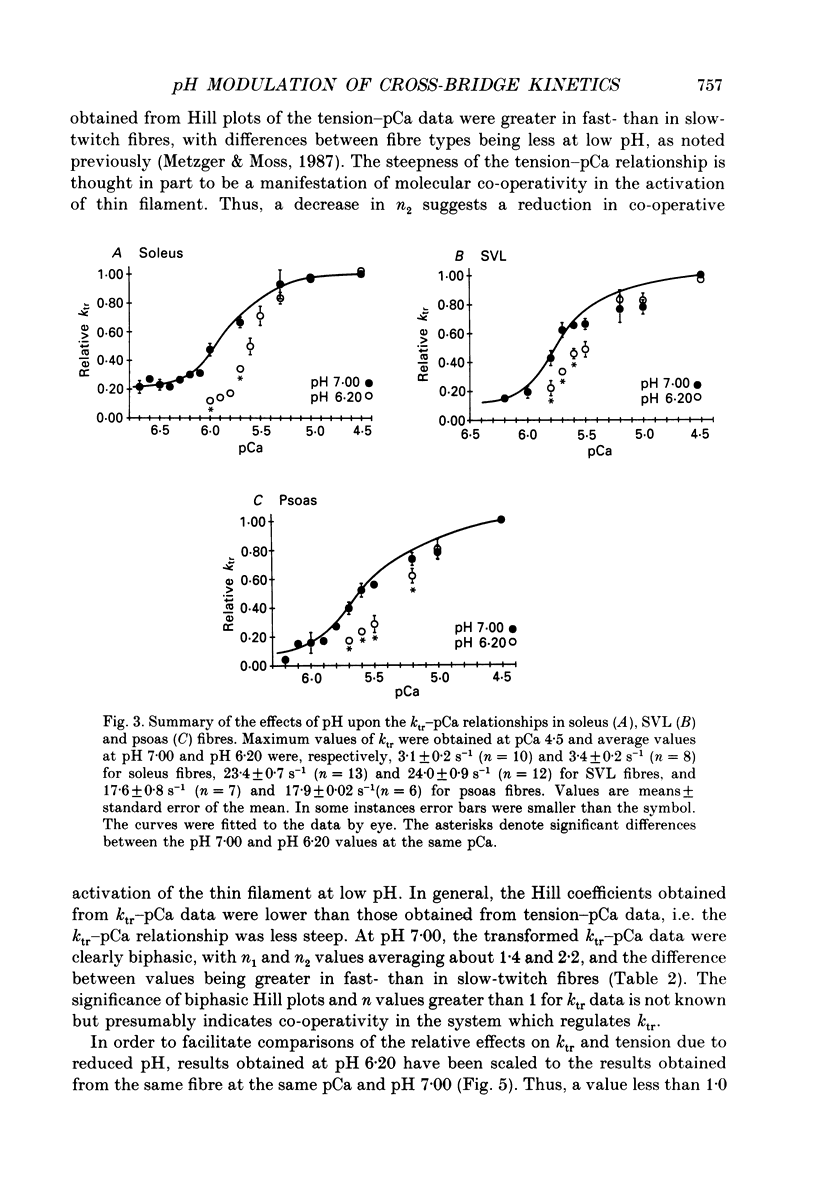
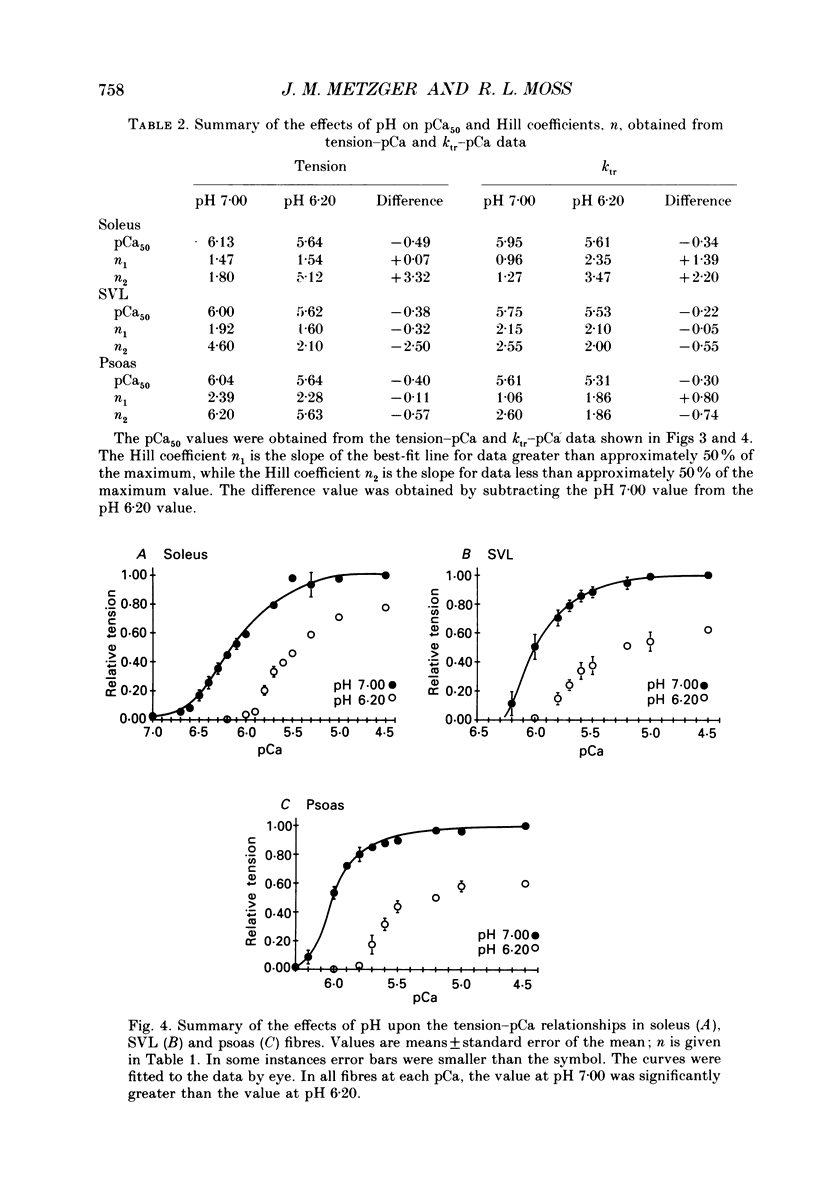
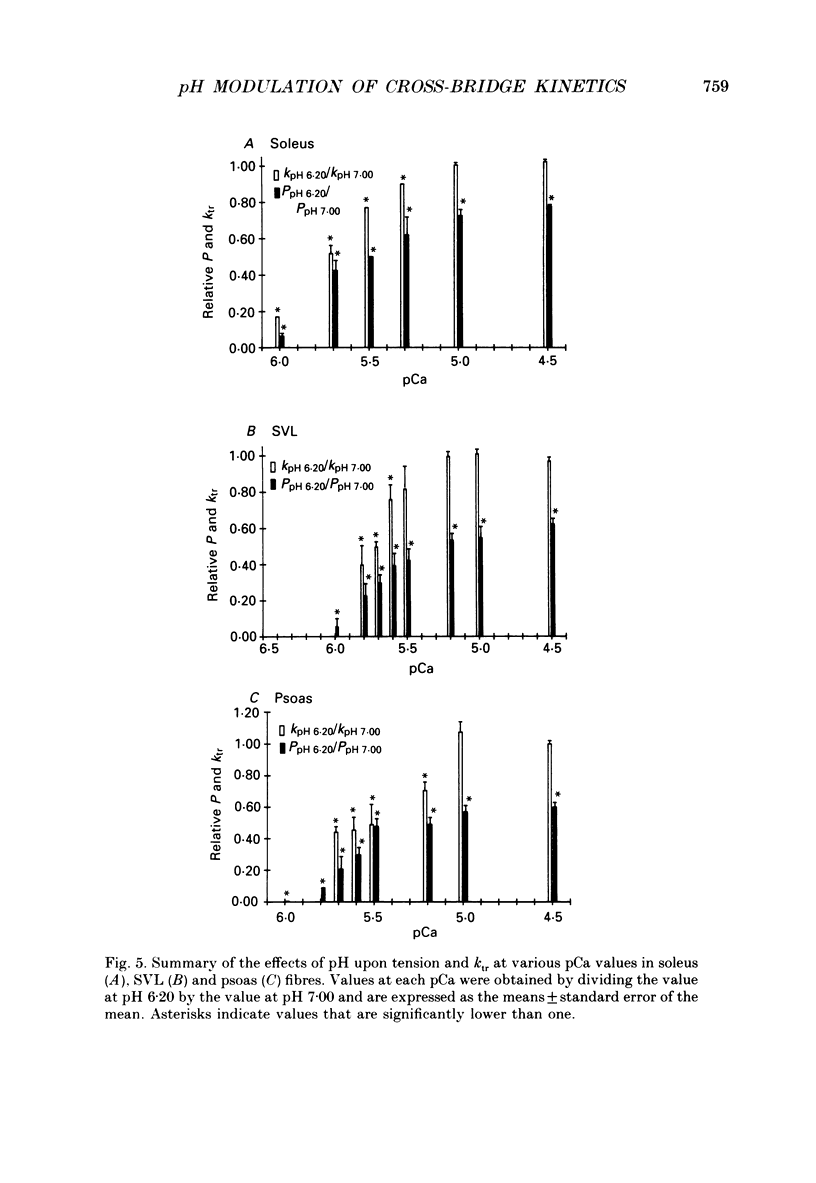
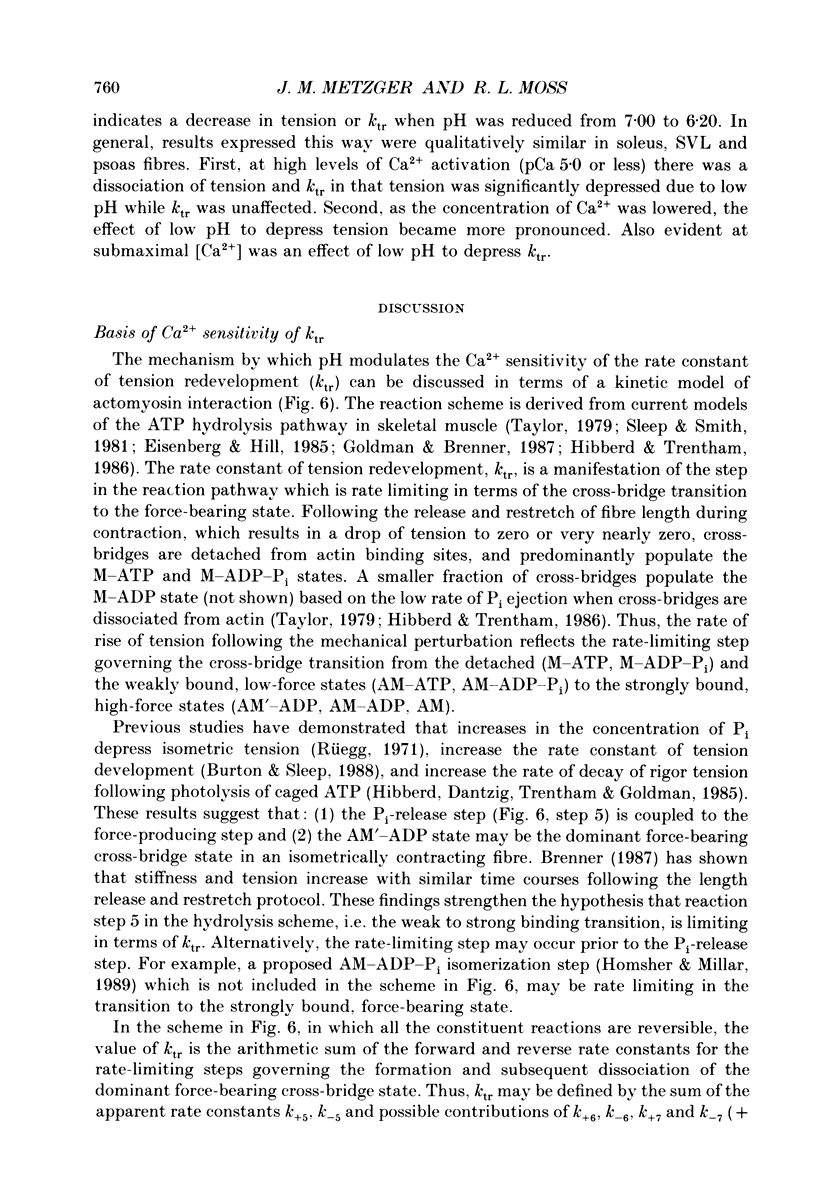
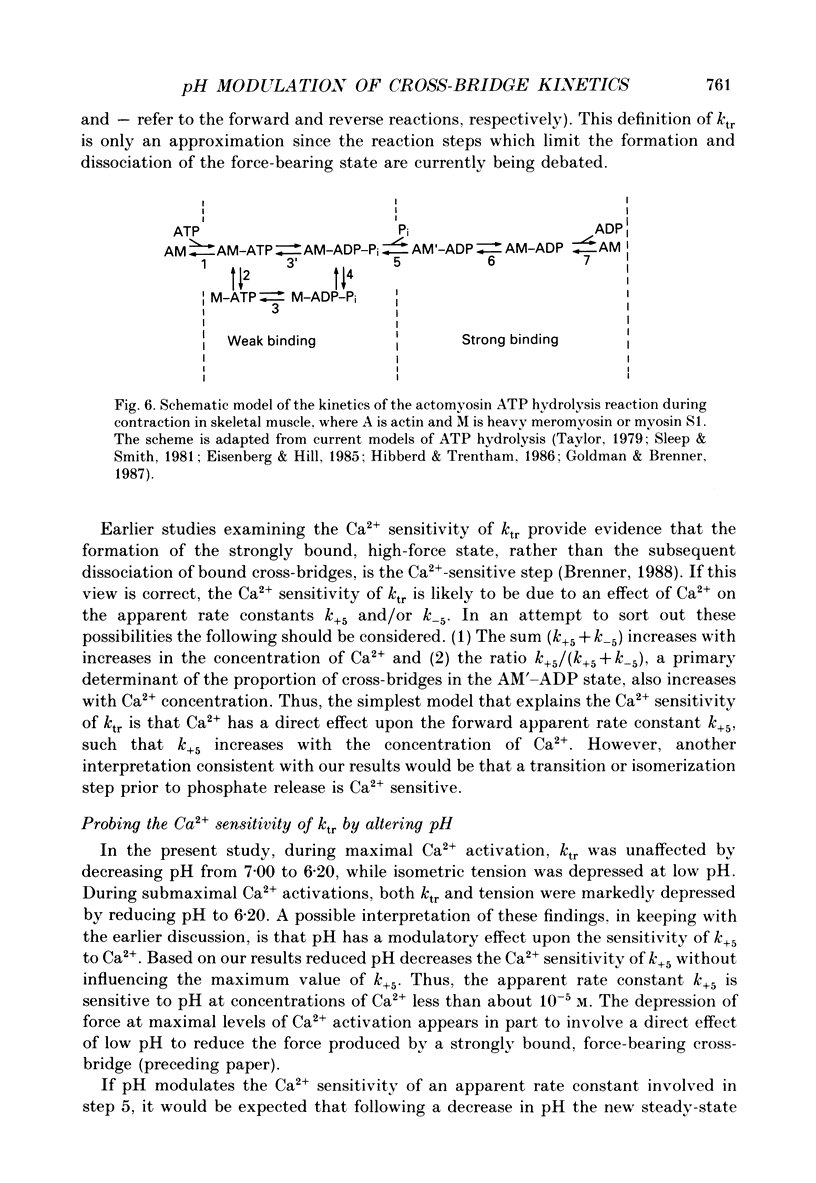
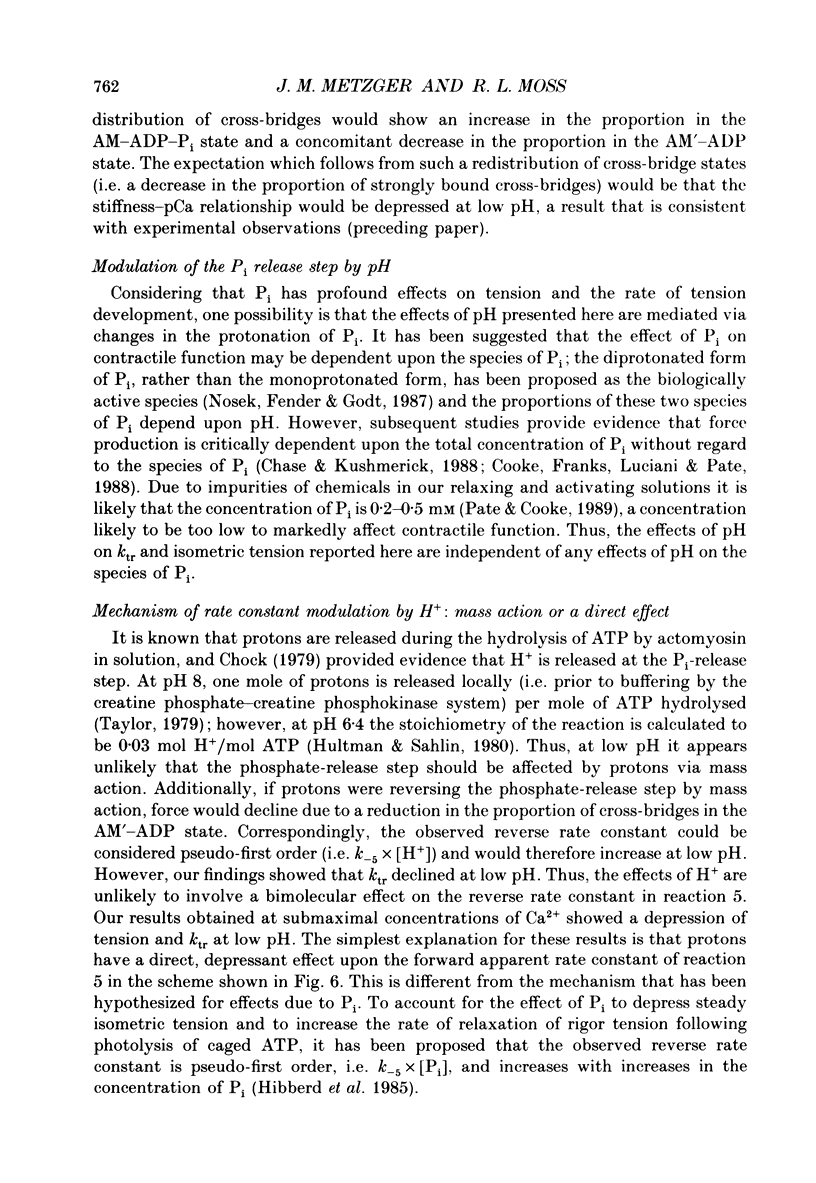
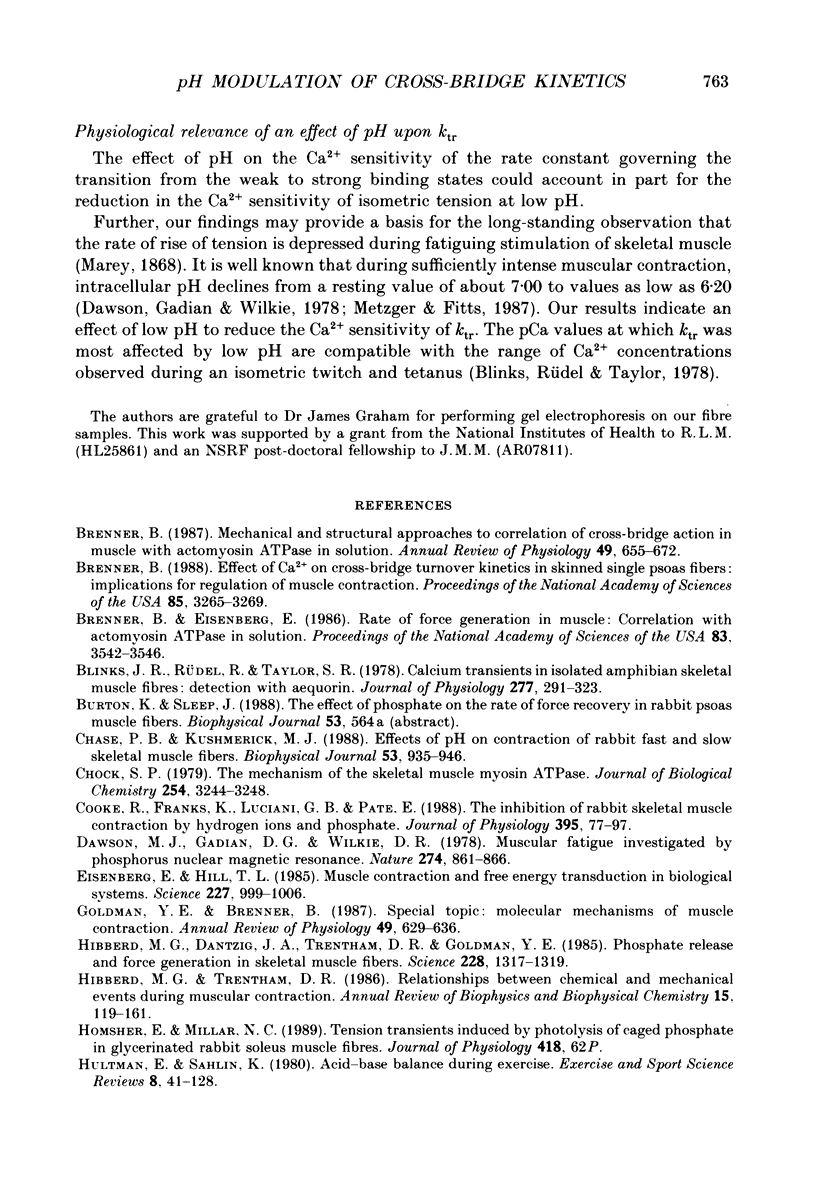
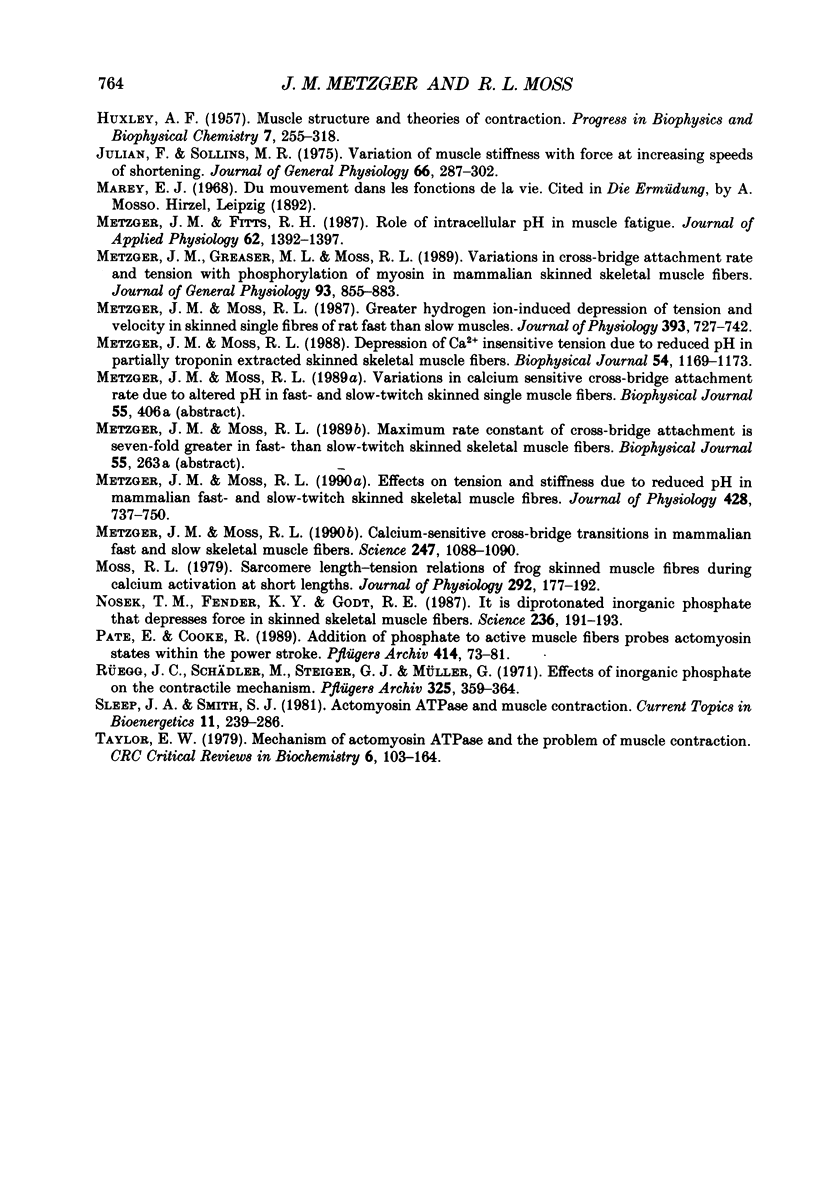
Selected References
These references are in PubMed. This may not be the complete list of references from this article.
- Blinks J. R., Rüdel R., Taylor S. R. Calcium transients in isolated amphibian skeletal muscle fibres: detection with aequorin. J Physiol. 1978 Apr;277:291–323. doi: 10.1113/jphysiol.1978.sp012273. [DOI] [PMC free article] [PubMed] [Google Scholar]
- Brenner B. Effect of Ca2+ on cross-bridge turnover kinetics in skinned single rabbit psoas fibers: implications for regulation of muscle contraction. Proc Natl Acad Sci U S A. 1988 May;85(9):3265–3269. doi: 10.1073/pnas.85.9.3265. [DOI] [PMC free article] [PubMed] [Google Scholar]
- Brenner B., Eisenberg E. Rate of force generation in muscle: correlation with actomyosin ATPase activity in solution. Proc Natl Acad Sci U S A. 1986 May;83(10):3542–3546. doi: 10.1073/pnas.83.10.3542. [DOI] [PMC free article] [PubMed] [Google Scholar]
- Brenner B. Mechanical and structural approaches to correlation of cross-bridge action in muscle with actomyosin ATPase in solution. Annu Rev Physiol. 1987;49:655–672. doi: 10.1146/annurev.ph.49.030187.003255. [DOI] [PubMed] [Google Scholar]
- Chase P. B., Kushmerick M. J. Effects of pH on contraction of rabbit fast and slow skeletal muscle fibers. Biophys J. 1988 Jun;53(6):935–946. doi: 10.1016/S0006-3495(88)83174-6. [DOI] [PMC free article] [PubMed] [Google Scholar]
- Chock S. P. The mechanism of the skeletal muscle myosin ATPase. III. Relationship of the H+ release and the protein absorbance change induced by ATP to the initial Pi burst. J Biol Chem. 1979 May 10;254(9):3244–3248. [PubMed] [Google Scholar]
- Cooke R., Franks K., Luciani G. B., Pate E. The inhibition of rabbit skeletal muscle contraction by hydrogen ions and phosphate. J Physiol. 1988 Jan;395:77–97. doi: 10.1113/jphysiol.1988.sp016909. [DOI] [PMC free article] [PubMed] [Google Scholar]
- Dawson M. J., Gadian D. G., Wilkie D. R. Muscular fatigue investigated by phosphorus nuclear magnetic resonance. Nature. 1978 Aug 31;274(5674):861–866. doi: 10.1038/274861a0. [DOI] [PubMed] [Google Scholar]
- Eisenberg E., Hill T. L. Muscle contraction and free energy transduction in biological systems. Science. 1985 Mar 1;227(4690):999–1006. doi: 10.1126/science.3156404. [DOI] [PubMed] [Google Scholar]
- Goldman Y. E., Brenner B. Special topic: molecular mechanism of muscle contraction. General introduction. Annu Rev Physiol. 1987;49:629–636. doi: 10.1146/annurev.ph.49.030187.003213. [DOI] [PubMed] [Google Scholar]
- HUXLEY A. F. Muscle structure and theories of contraction. Prog Biophys Biophys Chem. 1957;7:255–318. [PubMed] [Google Scholar]
- Hibberd M. G., Dantzig J. A., Trentham D. R., Goldman Y. E. Phosphate release and force generation in skeletal muscle fibers. Science. 1985 Jun 14;228(4705):1317–1319. doi: 10.1126/science.3159090. [DOI] [PubMed] [Google Scholar]
- Hibberd M. G., Trentham D. R. Relationships between chemical and mechanical events during muscular contraction. Annu Rev Biophys Biophys Chem. 1986;15:119–161. doi: 10.1146/annurev.bb.15.060186.001003. [DOI] [PubMed] [Google Scholar]
- Hultman E., Sahlin K. Acid-base balance during exercise. Exerc Sport Sci Rev. 1980;8:41–128. [PubMed] [Google Scholar]
- Julian F. J., Sollins M. R. Variation of muscle stiffness with force at increasing speeds of shortening. J Gen Physiol. 1975 Sep;66(3):287–302. doi: 10.1085/jgp.66.3.287. [DOI] [PMC free article] [PubMed] [Google Scholar]
- Metzger J. M., Fitts R. H. Role of intracellular pH in muscle fatigue. J Appl Physiol (1985) 1987 Apr;62(4):1392–1397. doi: 10.1152/jappl.1987.62.4.1392. [DOI] [PubMed] [Google Scholar]
- Metzger J. M., Greaser M. L., Moss R. L. Variations in cross-bridge attachment rate and tension with phosphorylation of myosin in mammalian skinned skeletal muscle fibers. Implications for twitch potentiation in intact muscle. J Gen Physiol. 1989 May;93(5):855–883. doi: 10.1085/jgp.93.5.855. [DOI] [PMC free article] [PubMed] [Google Scholar]
- Metzger J. M., Moss R. L. Calcium-sensitive cross-bridge transitions in mammalian fast and slow skeletal muscle fibers. Science. 1990 Mar 2;247(4946):1088–1090. doi: 10.1126/science.2309121. [DOI] [PubMed] [Google Scholar]
- Metzger J. M., Moss R. L. Depression of Ca2+ insensitive tension due to reduced pH in partially troponin-extracted skinned skeletal muscle fibers. Biophys J. 1988 Dec;54(6):1169–1173. doi: 10.1016/S0006-3495(88)83052-2. [DOI] [PMC free article] [PubMed] [Google Scholar]
- Metzger J. M., Moss R. L. Effects of tension and stiffness due to reduced pH in mammalian fast- and slow-twitch skinned skeletal muscle fibres. J Physiol. 1990 Sep;428:737–750. doi: 10.1113/jphysiol.1990.sp018238. [DOI] [PMC free article] [PubMed] [Google Scholar]
- Metzger J. M., Moss R. L. Greater hydrogen ion-induced depression of tension and velocity in skinned single fibres of rat fast than slow muscles. J Physiol. 1987 Dec;393:727–742. doi: 10.1113/jphysiol.1987.sp016850. [DOI] [PMC free article] [PubMed] [Google Scholar]
- Moss R. L. Sarcomere length-tension relations of frog skinned muscle fibres during calcium activation at short lengths. J Physiol. 1979 Jul;292:177–192. doi: 10.1113/jphysiol.1979.sp012845. [DOI] [PMC free article] [PubMed] [Google Scholar]
- Nosek T. M., Fender K. Y., Godt R. E. It is diprotonated inorganic phosphate that depresses force in skinned skeletal muscle fibers. Science. 1987 Apr 10;236(4798):191–193. doi: 10.1126/science.3563496. [DOI] [PubMed] [Google Scholar]
- Pate E., Cooke R. Addition of phosphate to active muscle fibers probes actomyosin states within the powerstroke. Pflugers Arch. 1989 May;414(1):73–81. doi: 10.1007/BF00585629. [DOI] [PubMed] [Google Scholar]
- Rüegg J. C., Schädler M., Steiger G. J., Müller G. Effects of inorganic phosphate on the contractile mechanism. Pflugers Arch. 1971;325(4):359–364. doi: 10.1007/BF00592176. [DOI] [PubMed] [Google Scholar]
- Taylor E. W. Mechanism of actomyosin ATPase and the problem of muscle contraction. CRC Crit Rev Biochem. 1979;6(2):103–164. doi: 10.3109/10409237909102562. [DOI] [PubMed] [Google Scholar]