Abstract
1. In order to study the neuronal response to hypoxia with maturation, hypoglossal neurons from adult and neonatal rat (3-7, 14-16, 21 and 28 days) brain stem slices were subjected to O2 deprivation. All neurons depolarized and showed no evidence of hyperpolarization at any time during the hypoxic period. 2. The magnitude of depolarization was about three-fold larger in adult hypoglossal neurons (mean = 32.0 mV) than in young neonatal neurons (mean = 10.4-11.2 mV) during hypoxic exposure (15-20 Torr) of 5 min. During longer periods of hypoxia of 15-30 min, neonatal cells showed an increase in the magnitude of depolarization reaching a level close to 80% of that in the adult. 3. In the early phase of hypoxia, adult neurons increased peak and steady-state spike frequency to induced current injections. Later, both spike frequencies decreased and, in 1/2 of adult neurons, there was a depolarization block. Input resistance (RN) of most adult neurons increased during hypoxia (RN = 180% of control after 5 min). Though neonatal neurons increased firing frequency, none had depolarization block and there was no increase in RN. 4. Tetrodotoxin (TTX), tetraethylammonium (TEA), apamin, high Mg2+/low Ca2+ solutions and intracellular ethyleneglycol-bis-(beta-aminoethylether)N,N,N',N'-tetrac etic acid (EGTA) did not reduce the magnitude of depolarization in hypoglossal neurons of 4-week-old and adult rats. Strophanthidin application depolarized hypoglossal neurons but decreased RN. 5. Ion-selective electrodes used to measure K+ concentrations in the extracellular fluid (Ko+) revealed a major increase in Ko+ (mean = 3.2 mM) in the adult hypoglossal area but not in the newborn tissue (mean = 0.65 mM). This probably reflects a difference in the amount of K+ efflux between neonatal and adult hypoglossal neurons. Shrinkage in the extracellular compartment in the adult may also account for some of the difference. 6. These results suggest that neonatal neuronal tissue is more tolerant to hypoxia than the adult, with the inherent cellular properties being maintained in newborn but not in adult neurons. The difference in membrane depolarization during hypoxia between the neonate and the adult can be attributed, in part, to differences in Ko+. The reason(s) for the difference in Ko+ is not known but could be due, to some extent, to different rates of intracellular ATP depletion and failure of the Na(+)-K+ pump.
Full text
PDF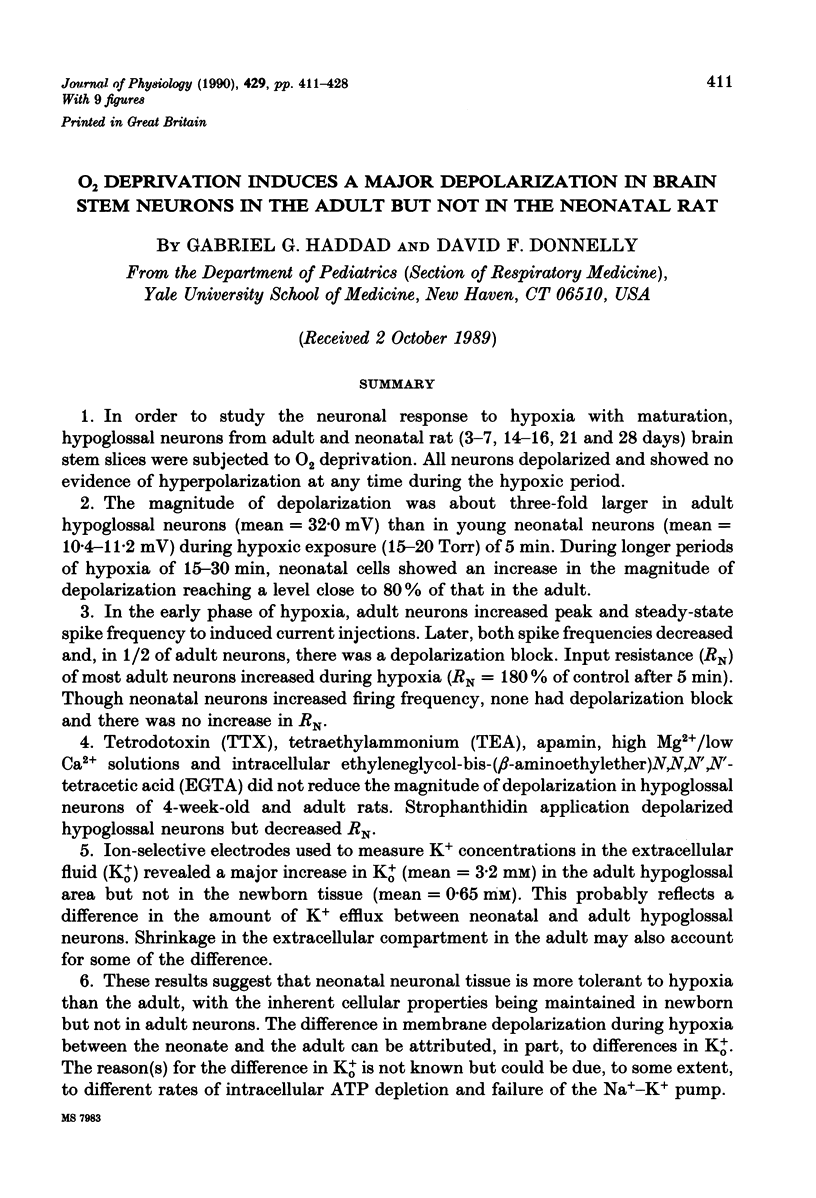
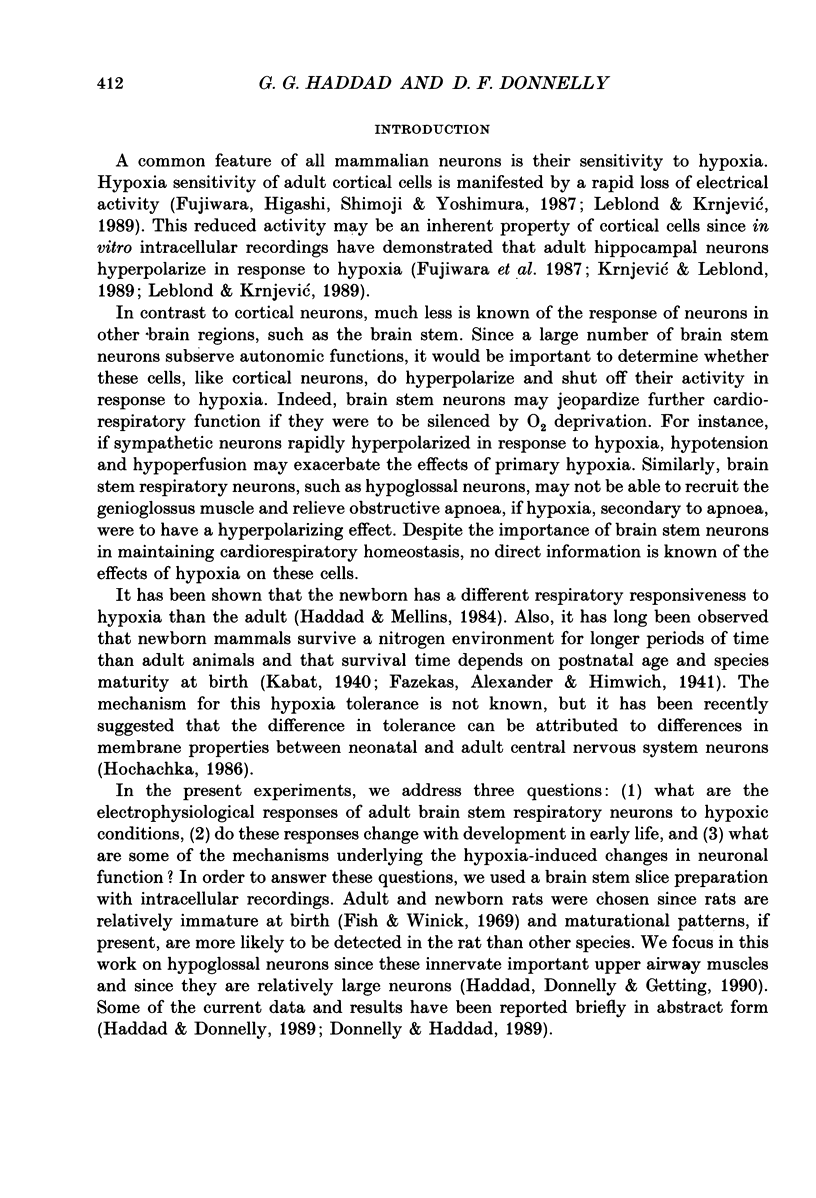
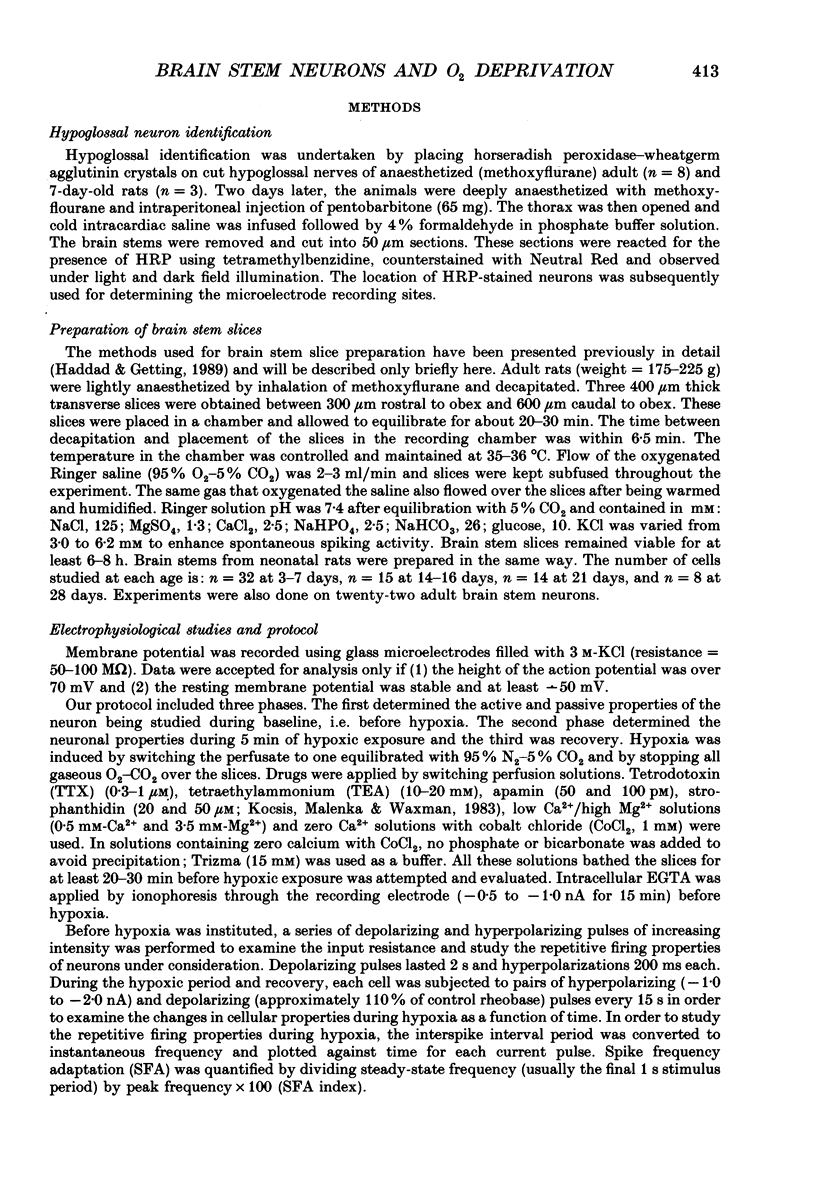
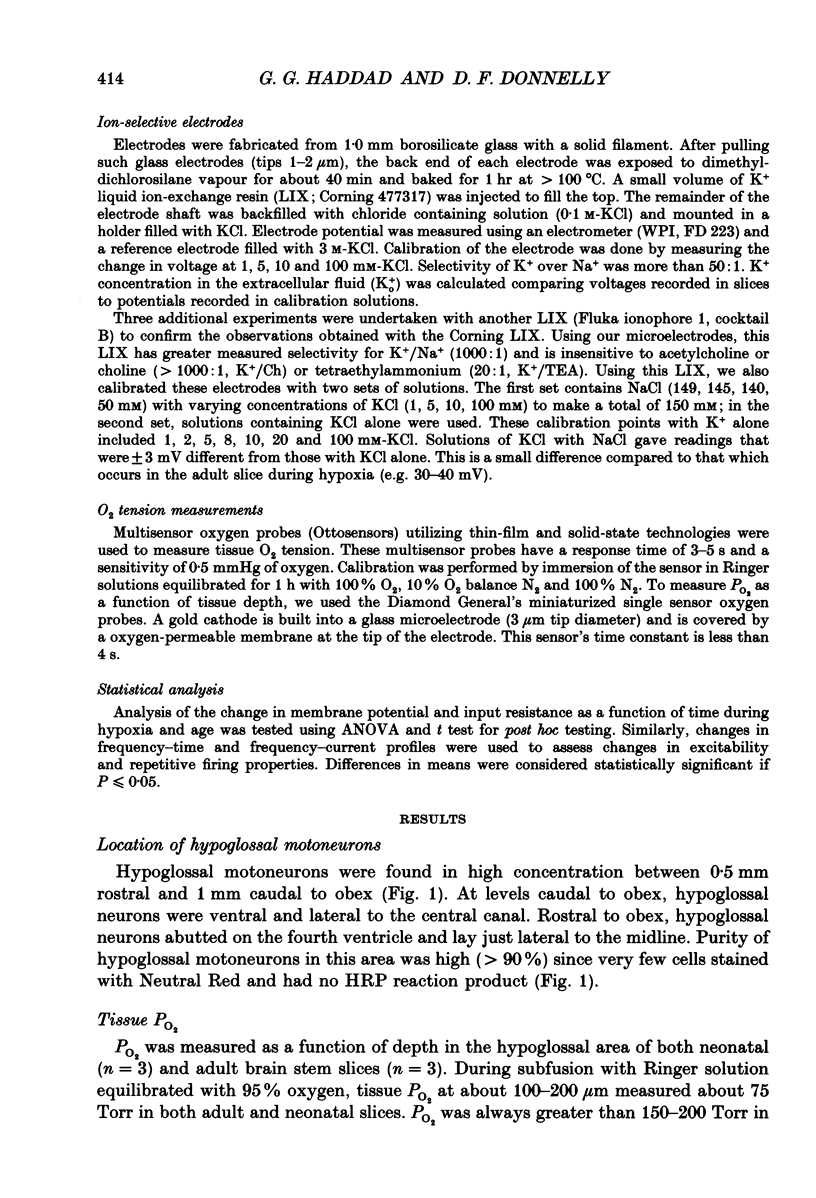
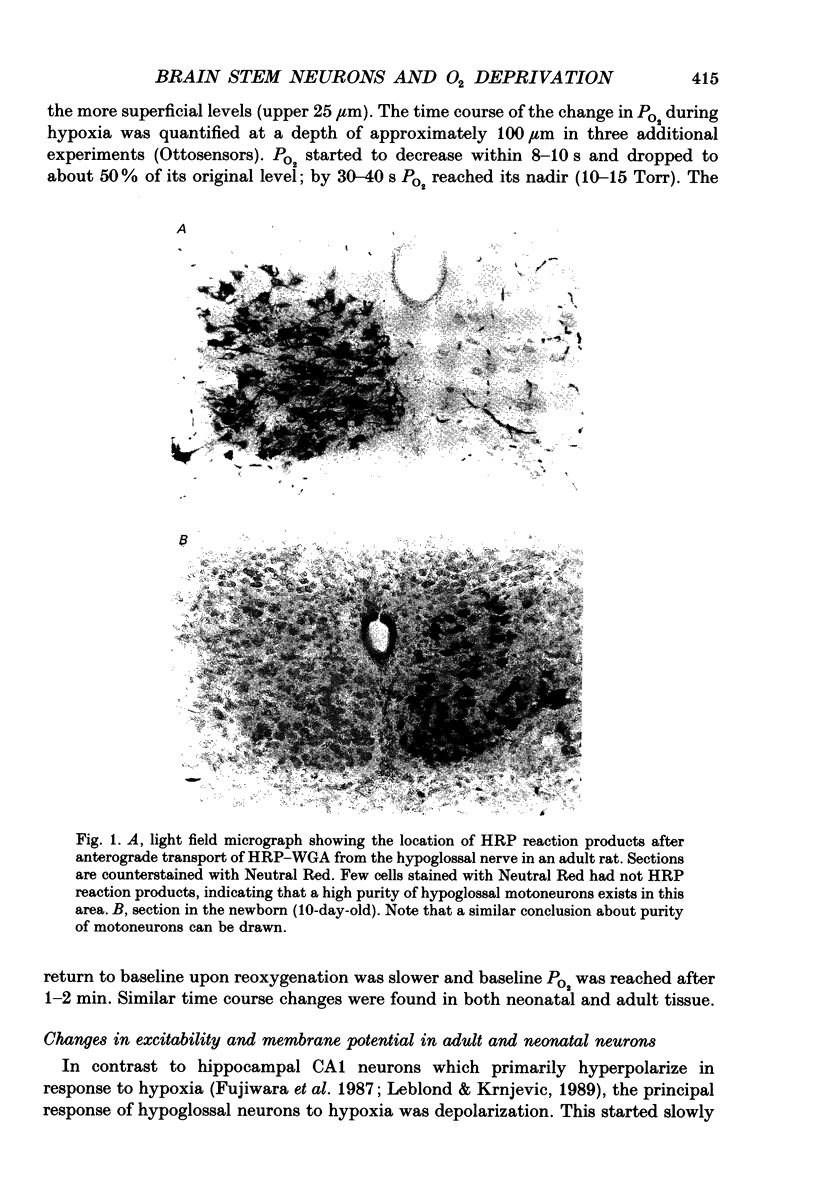
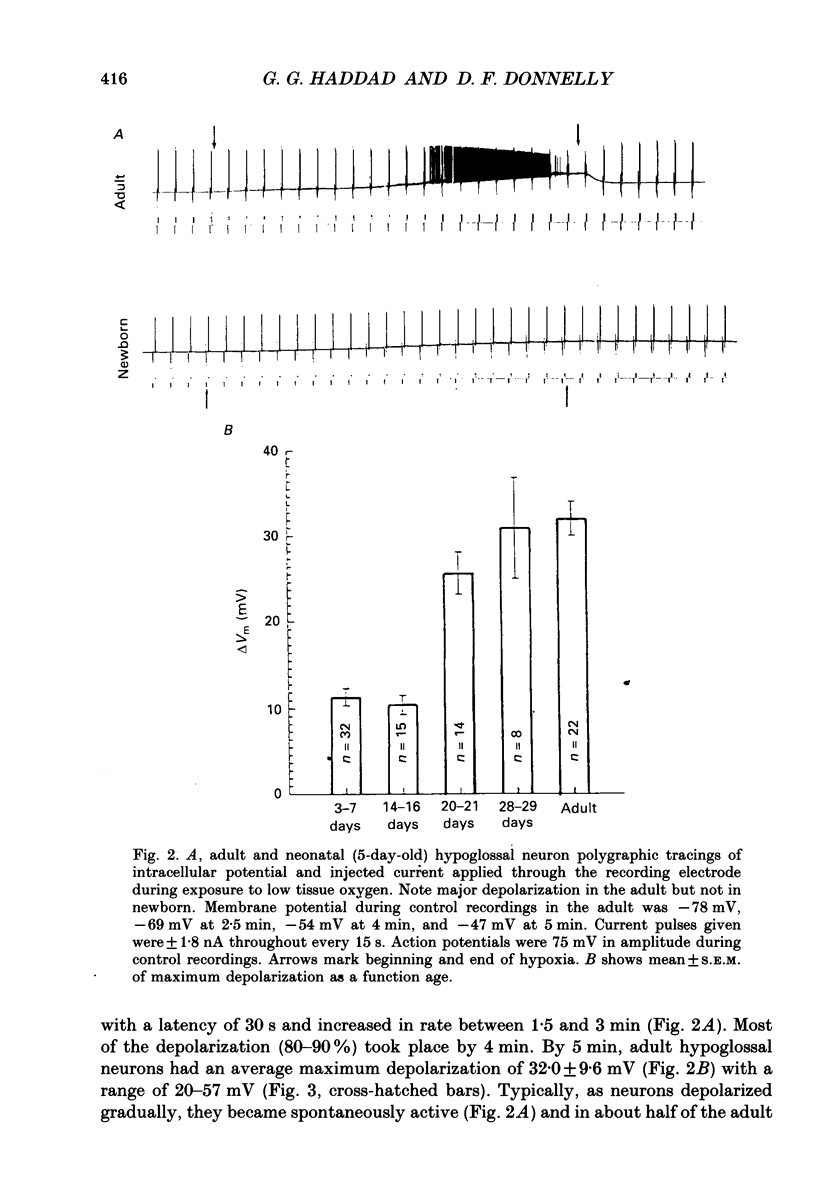
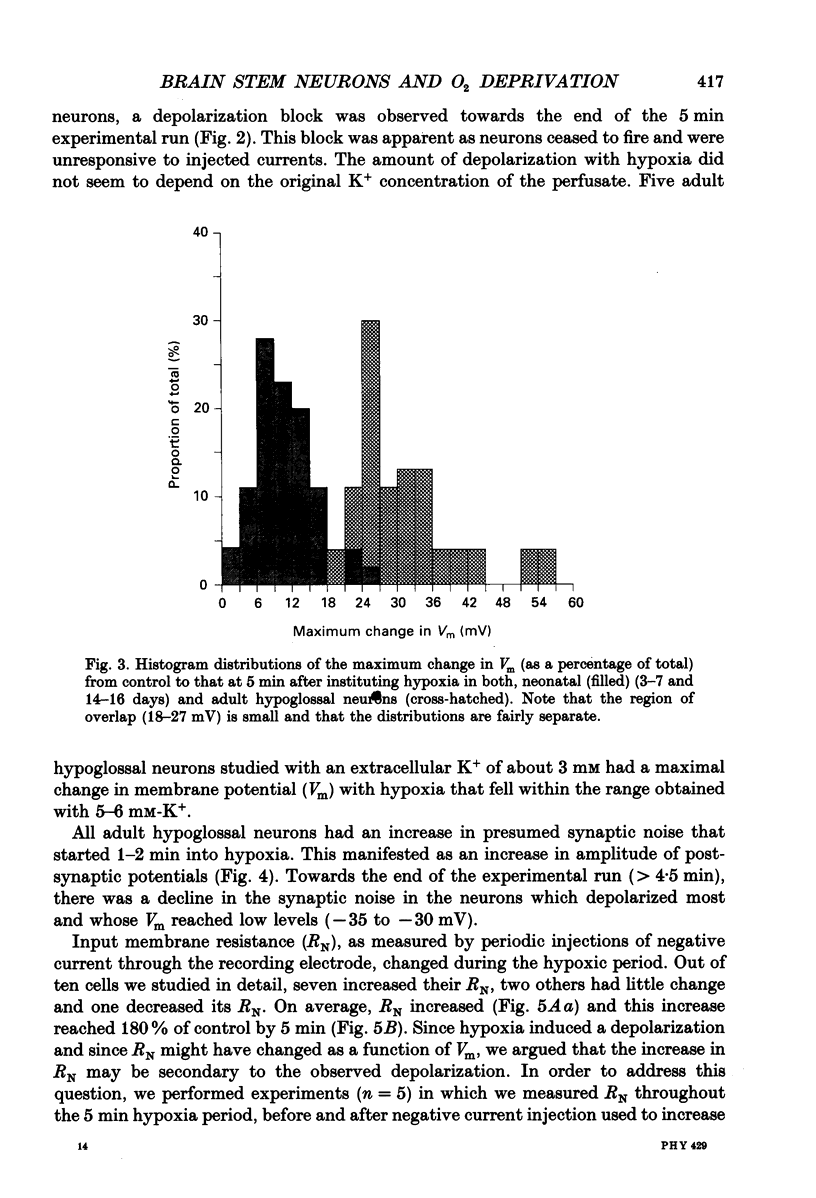
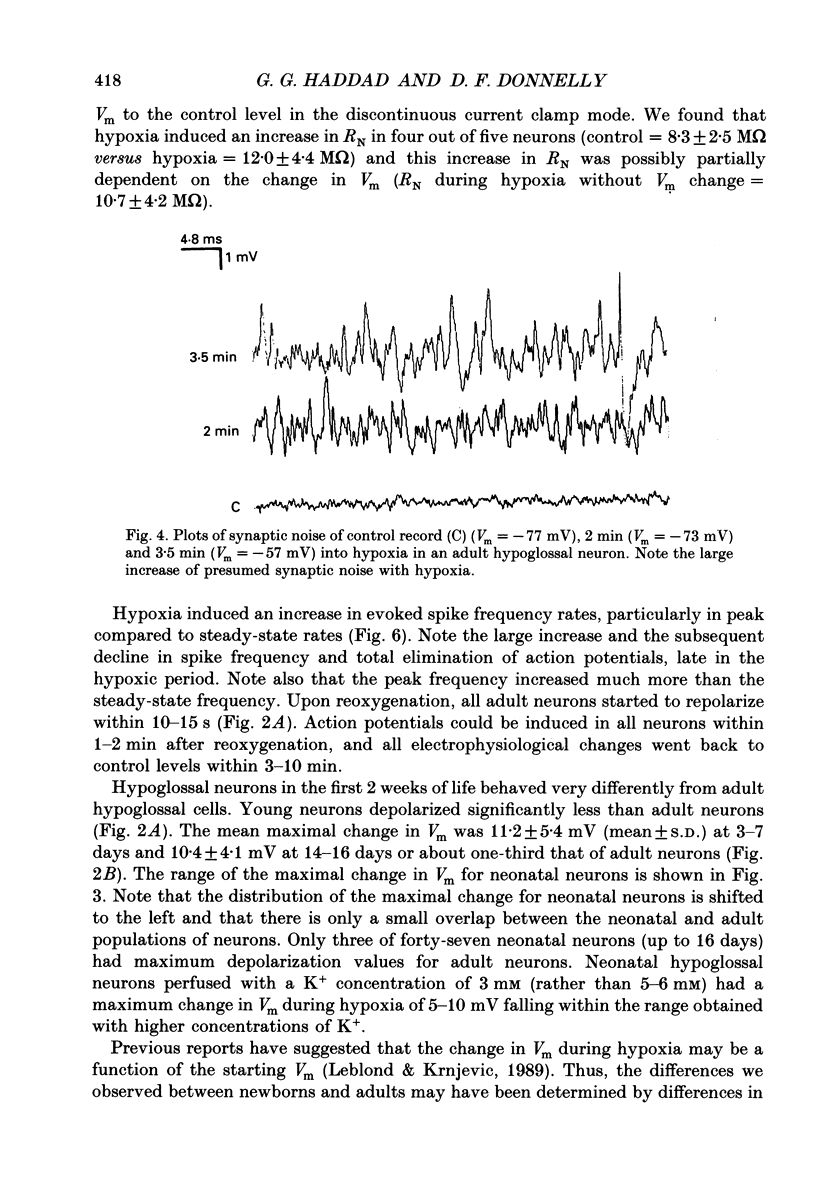
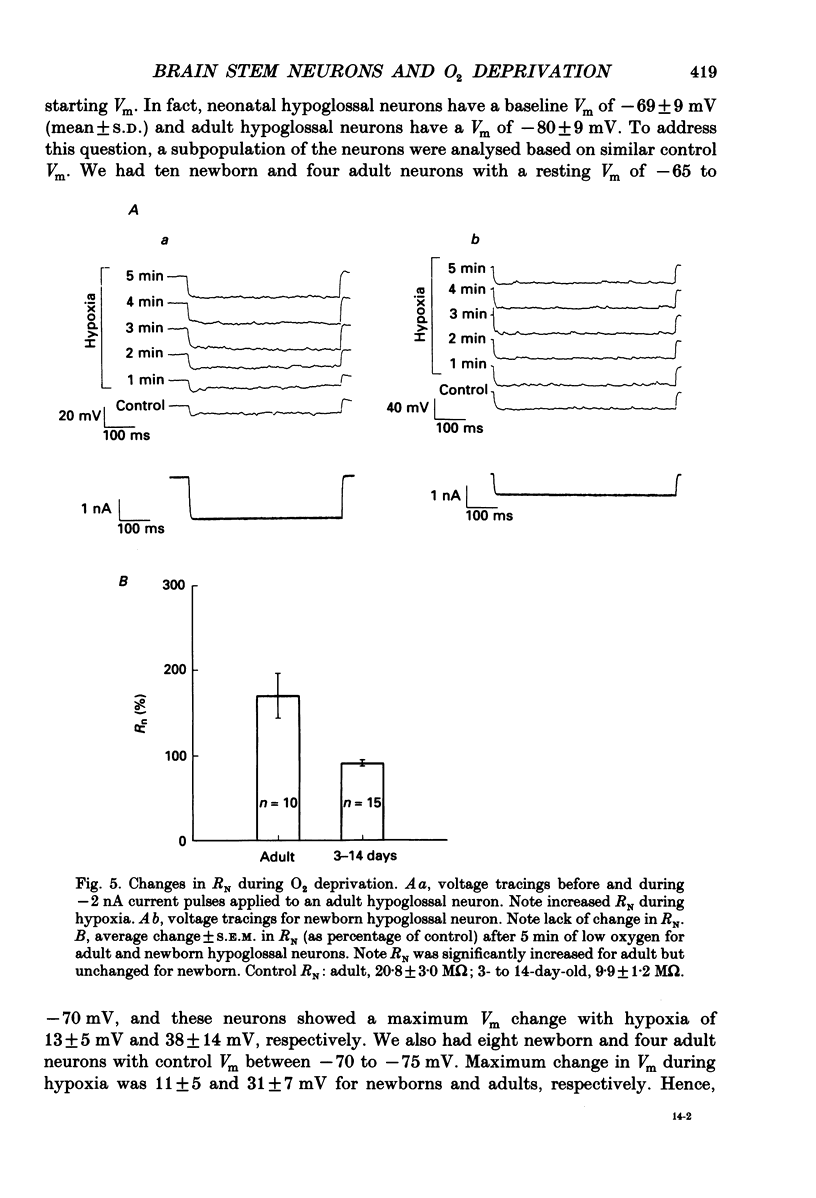
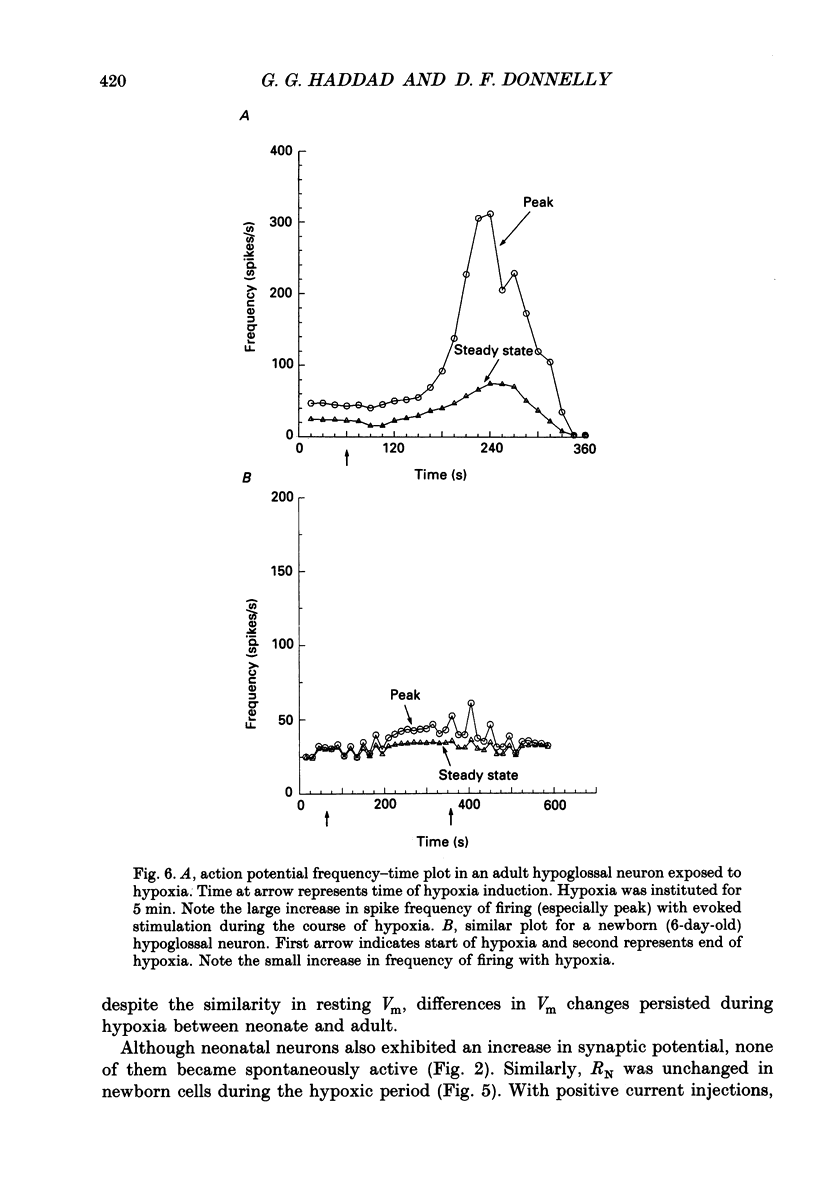
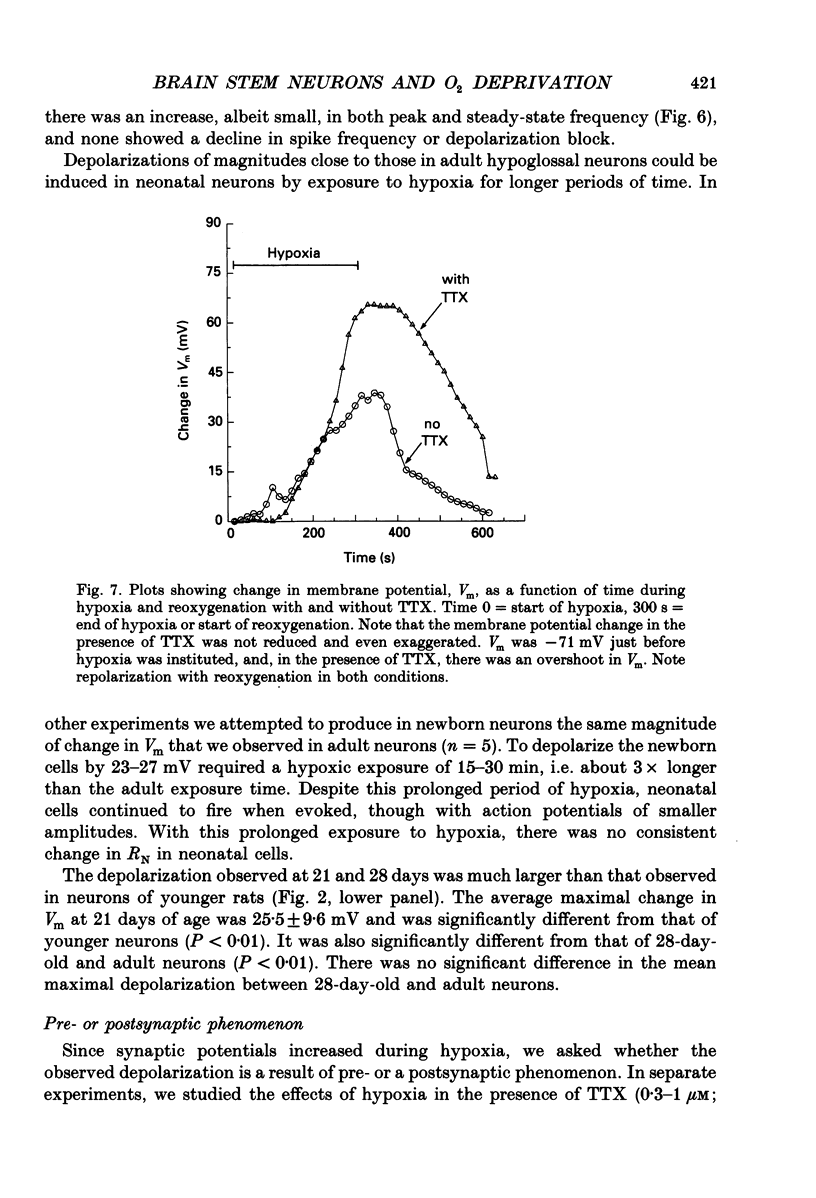
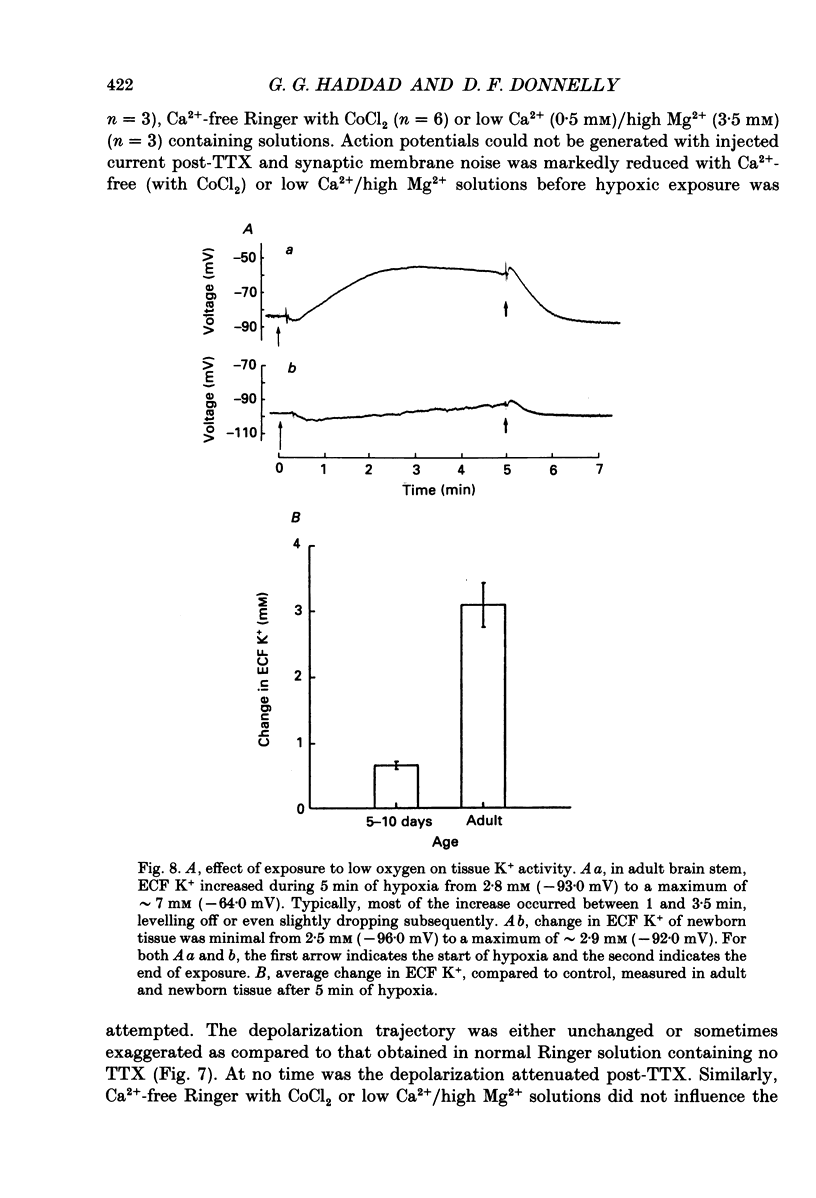
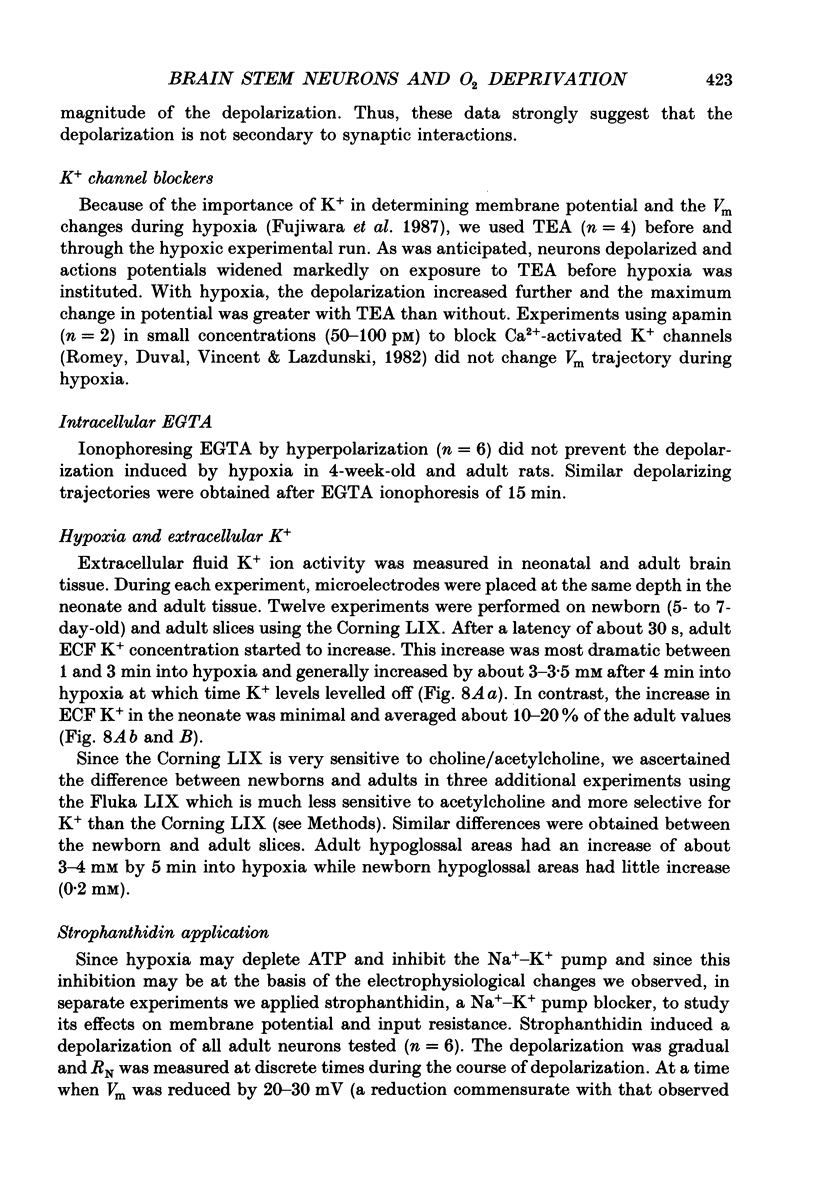
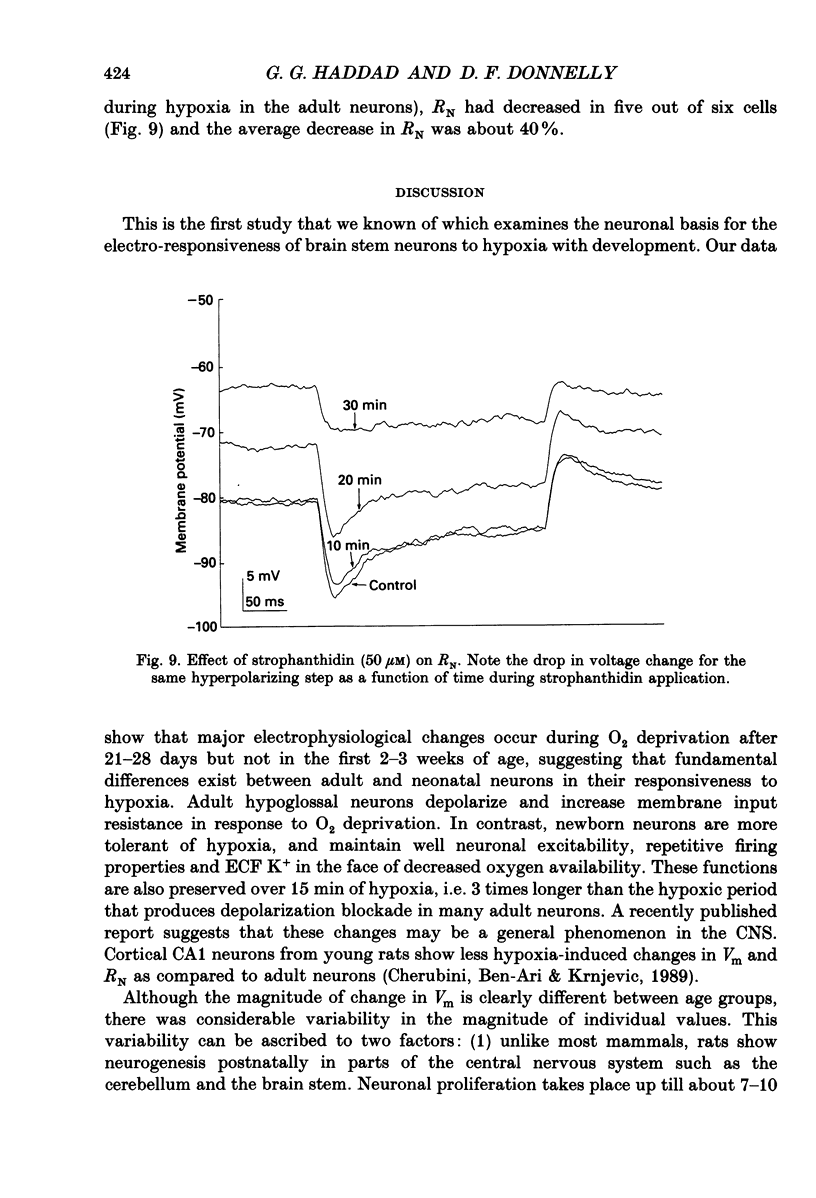
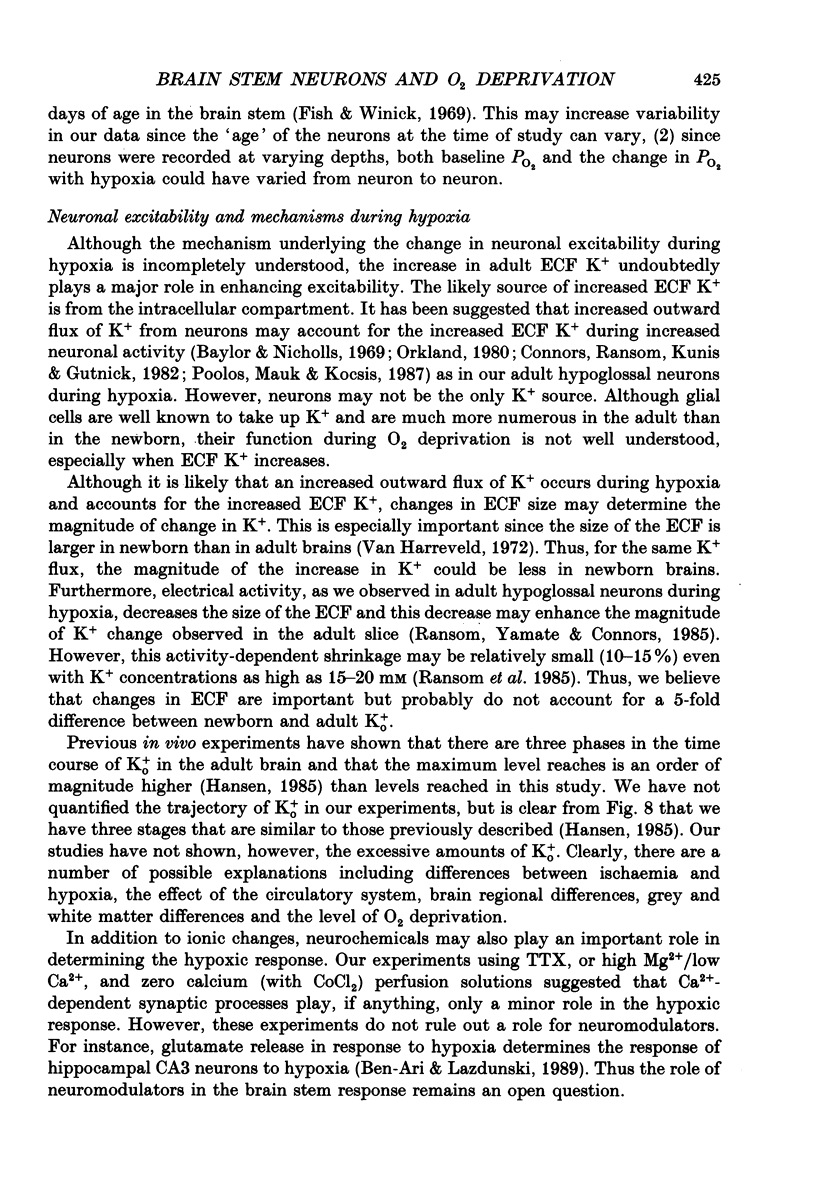
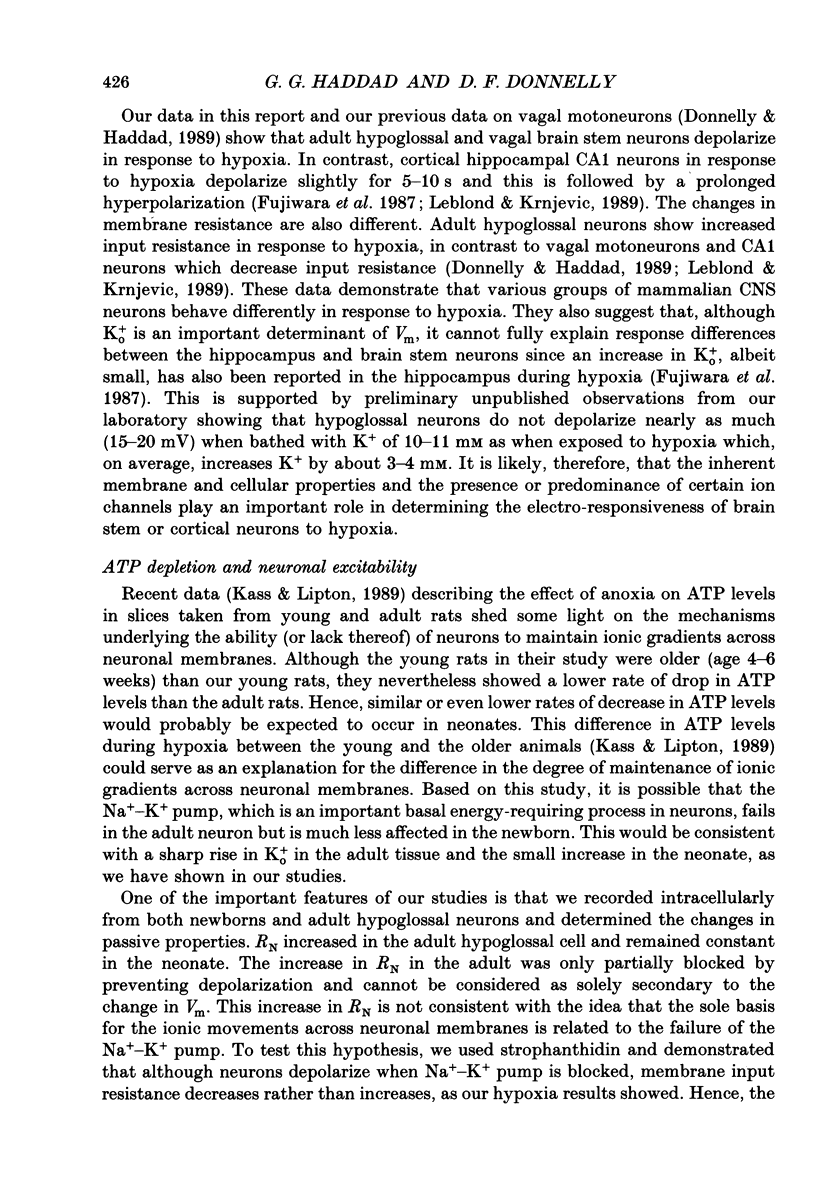
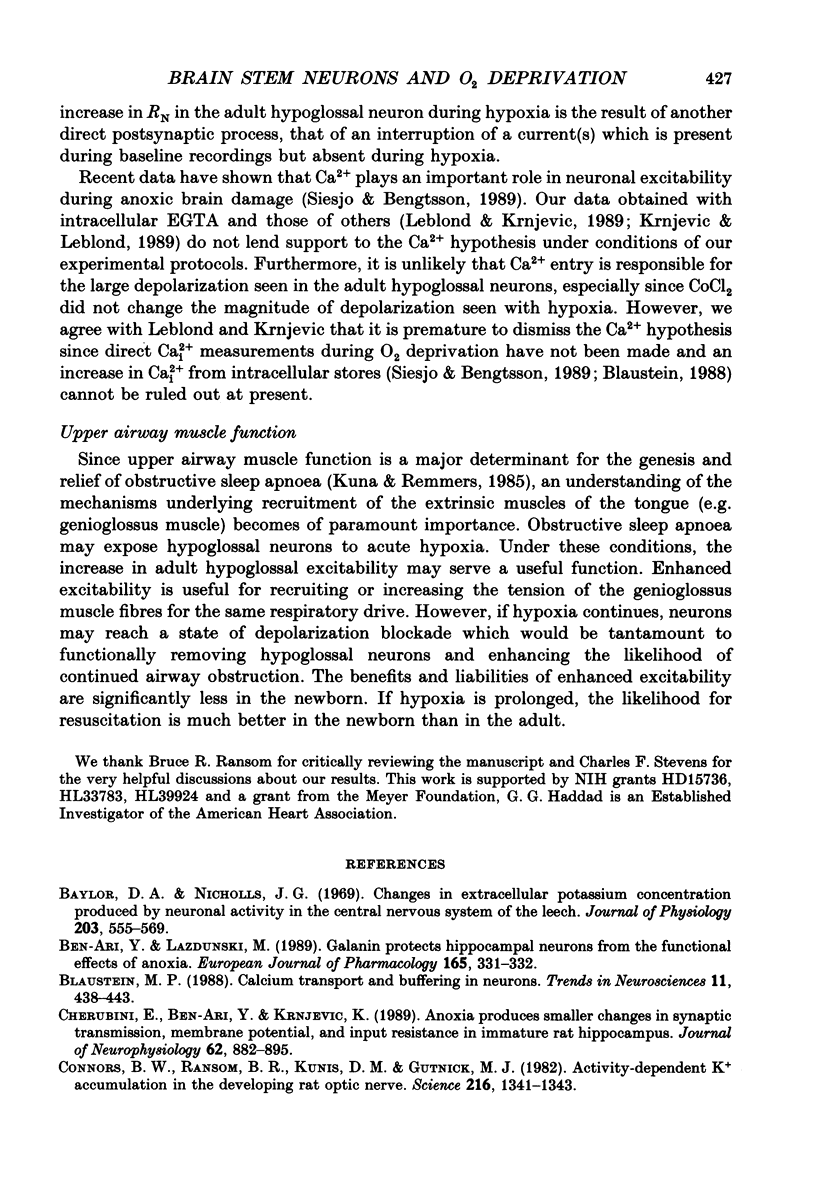
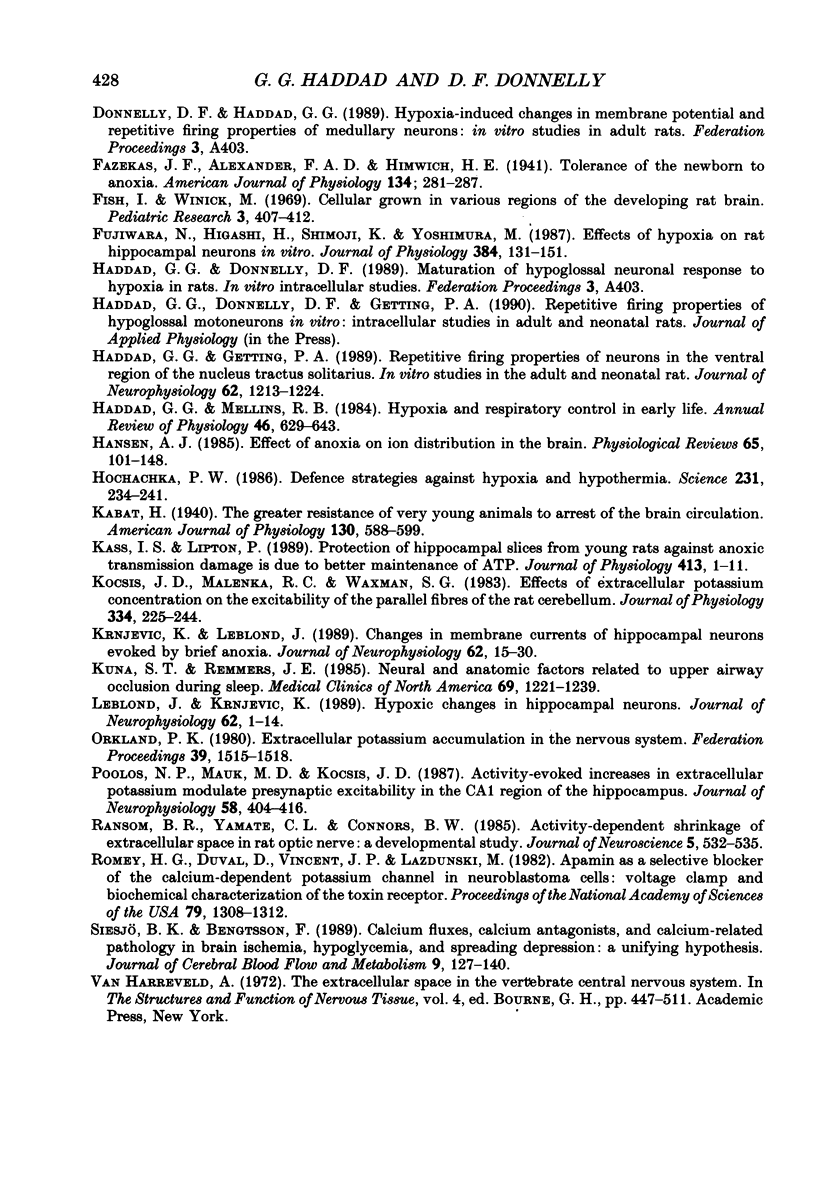
Images in this article
Selected References
These references are in PubMed. This may not be the complete list of references from this article.
- Ari Y. B., Lazdunski M. Galanin protects hippocampal neurons from the functional effects of anoxia. Eur J Pharmacol. 1989 Jun 20;165(2-3):331–332. doi: 10.1016/0014-2999(89)90732-2. [DOI] [PubMed] [Google Scholar]
- Baylor D. A., Nicholls J. G. Changes in extracellular potassium concentration produced by neuronal activity in the central nervous system of the leech. J Physiol. 1969 Aug;203(3):555–569. doi: 10.1113/jphysiol.1969.sp008879. [DOI] [PMC free article] [PubMed] [Google Scholar]
- Blaustein M. P. Calcium transport and buffering in neurons. Trends Neurosci. 1988 Oct;11(10):438–443. doi: 10.1016/0166-2236(88)90195-6. [DOI] [PubMed] [Google Scholar]
- Cherubini E., Ben-Ari Y., Krnjević K. Anoxia produces smaller changes in synaptic transmission, membrane potential, and input resistance in immature rat hippocampus. J Neurophysiol. 1989 Oct;62(4):882–895. doi: 10.1152/jn.1989.62.4.882. [DOI] [PubMed] [Google Scholar]
- Connors B. W., Ransom B. R., Kunis D. M., Gutnick M. J. Activity-dependent K+ accumulation in the developing rat optic nerve. Science. 1982 Jun 18;216(4552):1341–1343. doi: 10.1126/science.7079771. [DOI] [PubMed] [Google Scholar]
- Fish I., Winick M. Cellular growth in various regions of the developing rat brain. Pediatr Res. 1969 Sep;3(5):407–412. doi: 10.1203/00006450-196909000-00003. [DOI] [PubMed] [Google Scholar]
- Fujiwara N., Higashi H., Shimoji K., Yoshimura M. Effects of hypoxia on rat hippocampal neurones in vitro. J Physiol. 1987 Mar;384:131–151. doi: 10.1113/jphysiol.1987.sp016447. [DOI] [PMC free article] [PubMed] [Google Scholar]
- Haddad G. G., Getting P. A. Repetitive firing properties of neurons in the ventral region of nucleus tractus solitarius. In vitro studies in adult and neonatal rat. J Neurophysiol. 1989 Dec;62(6):1213–1224. doi: 10.1152/jn.1989.62.6.1213. [DOI] [PubMed] [Google Scholar]
- Haddad G. G., Mellins R. B. Hypoxia and respiratory control in early life. Annu Rev Physiol. 1984;46:629–643. doi: 10.1146/annurev.ph.46.030184.003213. [DOI] [PubMed] [Google Scholar]
- Hansen A. J. Effect of anoxia on ion distribution in the brain. Physiol Rev. 1985 Jan;65(1):101–148. doi: 10.1152/physrev.1985.65.1.101. [DOI] [PubMed] [Google Scholar]
- Hochachka P. W. Defense strategies against hypoxia and hypothermia. Science. 1986 Jan 17;231(4735):234–241. doi: 10.1126/science.2417316. [DOI] [PubMed] [Google Scholar]
- Hugues M., Romey G., Duval D., Vincent J. P., Lazdunski M. Apamin as a selective blocker of the calcium-dependent potassium channel in neuroblastoma cells: voltage-clamp and biochemical characterization of the toxin receptor. Proc Natl Acad Sci U S A. 1982 Feb;79(4):1308–1312. doi: 10.1073/pnas.79.4.1308. [DOI] [PMC free article] [PubMed] [Google Scholar]
- Kass I. S., Lipton P. Protection of hippocampal slices from young rats against anoxic transmission damage is due to better maintenance of ATP. J Physiol. 1989 Jun;413:1–11. doi: 10.1113/jphysiol.1989.sp017638. [DOI] [PMC free article] [PubMed] [Google Scholar]
- Kocsis J. D., Malenka R. C., Waxman S. G. Effects of extracellular potassium concentration on the excitability of the parallel fibres of the rat cerebellum. J Physiol. 1983 Jan;334:225–244. doi: 10.1113/jphysiol.1983.sp014491. [DOI] [PMC free article] [PubMed] [Google Scholar]
- Krnjević K., Leblond J. Changes in membrane currents of hippocampal neurons evoked by brief anoxia. J Neurophysiol. 1989 Jul;62(1):15–30. doi: 10.1152/jn.1989.62.1.15. [DOI] [PubMed] [Google Scholar]
- Kuna S. T., Remmers J. E. Neural and anatomic factors related to upper airway occlusion during sleep. Med Clin North Am. 1985 Nov;69(6):1221–1242. doi: 10.1016/s0025-7125(16)30984-1. [DOI] [PubMed] [Google Scholar]
- Leblond J., Krnjevic K. Hypoxic changes in hippocampal neurons. J Neurophysiol. 1989 Jul;62(1):1–14. doi: 10.1152/jn.1989.62.1.1. [DOI] [PubMed] [Google Scholar]
- Orkand R. K. Extracellular potassium accumulation in the nervous system. Fed Proc. 1980 Apr;39(5):1515–1518. [PubMed] [Google Scholar]
- Poolos N. P., Mauk M. D., Kocsis J. D. Activity-evoked increases in extracellular potassium modulate presynaptic excitability in the CA1 region of the hippocampus. J Neurophysiol. 1987 Aug;58(2):404–416. doi: 10.1152/jn.1987.58.2.404. [DOI] [PubMed] [Google Scholar]
- Ransom B. R., Yamate C. L., Connors B. W. Activity-dependent shrinkage of extracellular space in rat optic nerve: a developmental study. J Neurosci. 1985 Feb;5(2):532–535. doi: 10.1523/JNEUROSCI.05-02-00532.1985. [DOI] [PMC free article] [PubMed] [Google Scholar]
- Siesjö B. K., Bengtsson F. Calcium fluxes, calcium antagonists, and calcium-related pathology in brain ischemia, hypoglycemia, and spreading depression: a unifying hypothesis. J Cereb Blood Flow Metab. 1989 Apr;9(2):127–140. doi: 10.1038/jcbfm.1989.20. [DOI] [PubMed] [Google Scholar]