Abstract
1. Synaptically connected neurones were identified in the granule cell layer of slices of 17- to 21-day-old rat hippocampus. Whole-cell current recording using the patch-clamp technique revealed synaptic currents ranging from less than 10 to 200 pA in symmetrical Cl- conditions, at a holding potential of -50 mV. These currents were blocked by 2 microM-bicuculline, indicating that they result from the activation of postsynaptic gamma-aminobutyric acid receptor (GABAA-receptor) channels. 2. Addition of tetrodotoxin (TTX, 1 microM) resulted in the loss of most currents of more than 40 pA in amplitude. Currents which disappeared after TTX treatment were assumed to be the result of spontaneous presynaptic action potentials. The currents seen in the absence of TTX are referred to as spontaneously occurring inhibitory postsynaptic currents (IPSCs); those remaining in the presence of TTX were defined as miniature IPSCs. 3. Similar currents were observed when recording in the whole-cell configuration while extracellular stimulation was applied to a nearby neurone. These currents were also completely blocked by 2 microM-bicuculline and by 0.5 microM-TTX. They were thus defined as stimulus-evoked IPSCs. 4. The half rise time of both miniature and stimulus-evoked IPSCs was fast (less than 1 ms). The time course of decay of both miniature IPSCs and stimulus-evoked IPSCs could be well fitted with the sum of two exponentials. At a membrane potential of -50 mV, the mean decay time constants of the two components were 2.0 +/- 0.38 and 54.4 +/- 18 ms (mean +/- S.D.) for miniature IPSCs (six cells) and 2.2 +/- 1.3 and 66 +/- 20 ms (three cells) for stimulus-evoked IPSCs. 5. Stimulus-evoked IPSCs varied in amplitude from less than ten to hundreds of picoamperes. In eight of eleven cells histograms of IPSC amplitudes showed several clear peaks which, when fitted with the sum of Gaussian curves, were found to be equidistant. This is consistent with the view that stimulus-evoked IPSC amplitudes vary in a quantal fashion. The quantal size varied between 7 and 20 pA, at a membrane potential of -50 mV. 6. Decreasing the Ca2+ and increasing the Mg2+ concentration in the extracellular solution decreased the number of peaks in the IPSC amplitude histogram but did not affect the size of the quantal event.(ABSTRACT TRUNCATED AT 400 WORDS)
Full text
PDF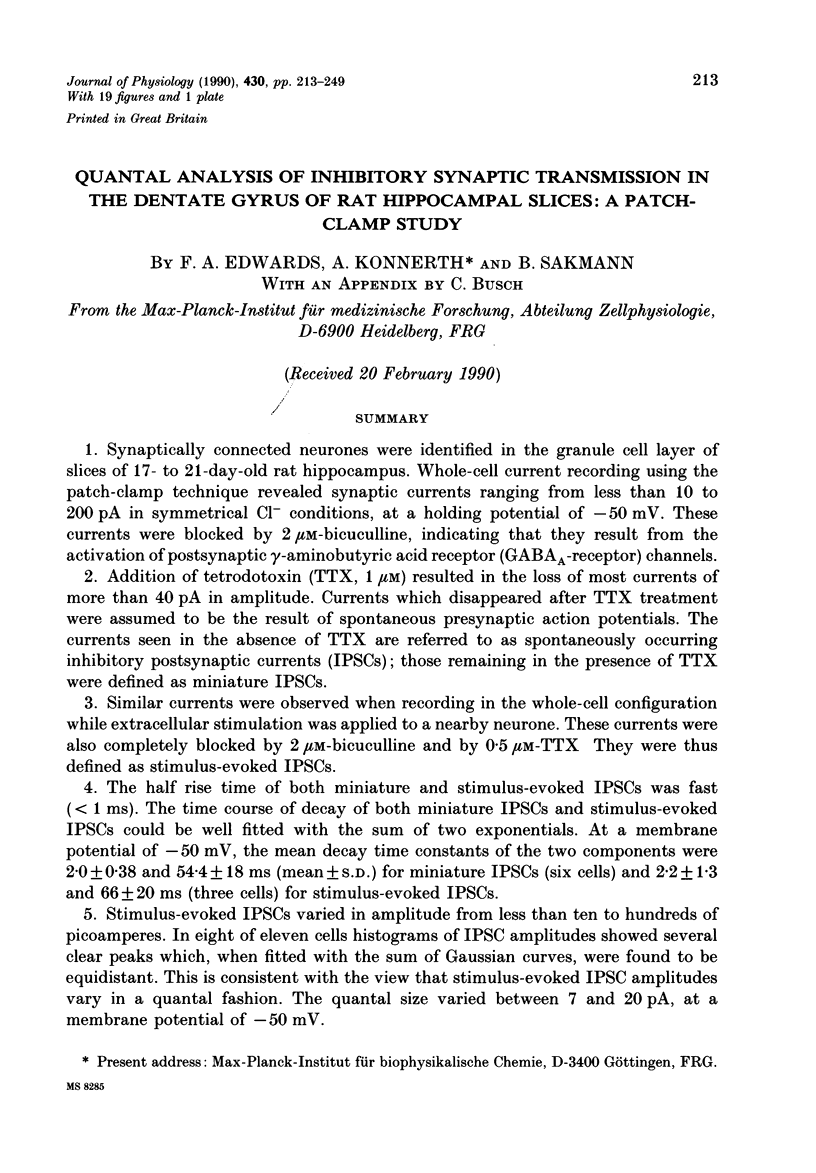
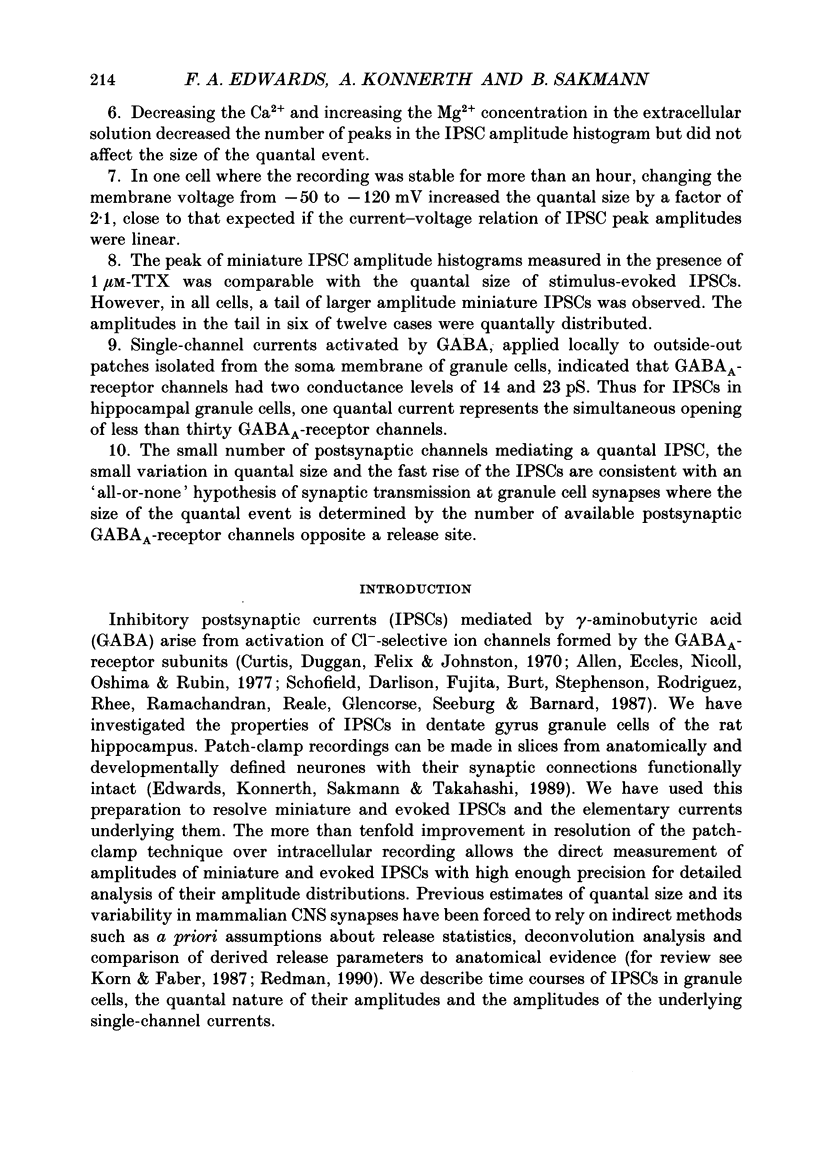
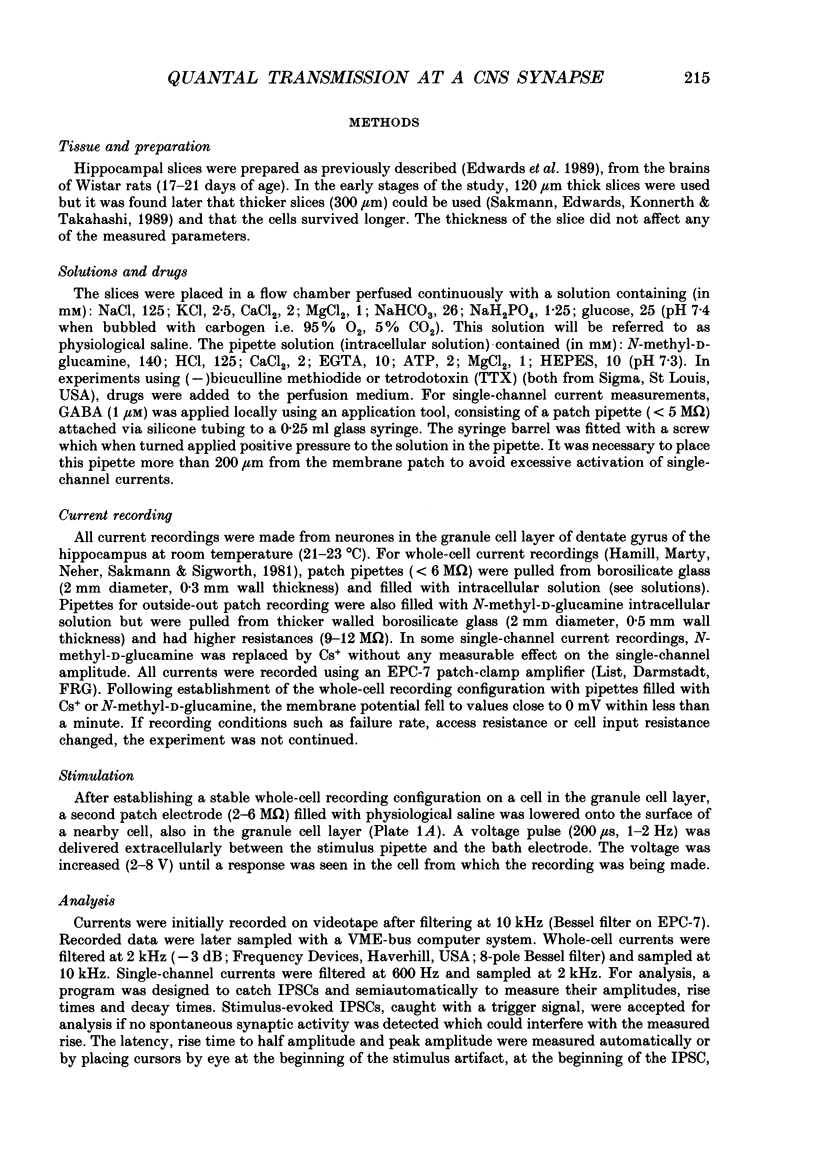
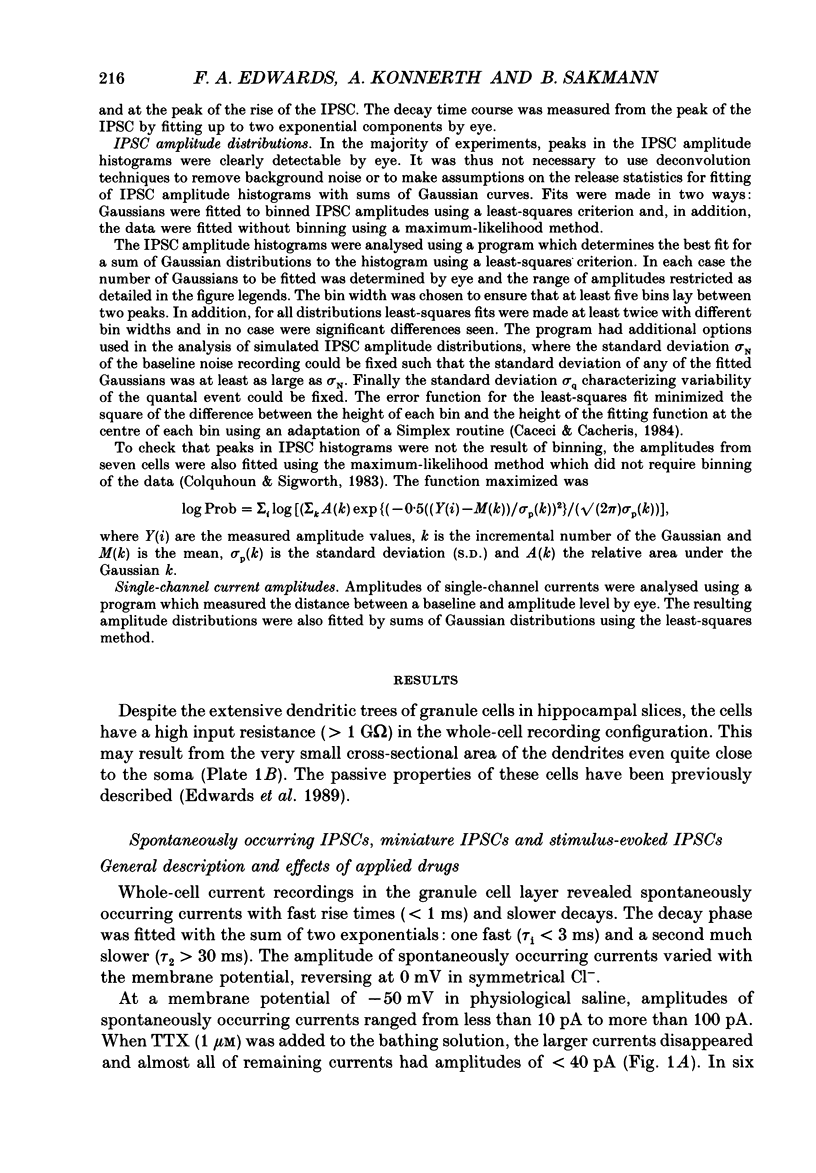
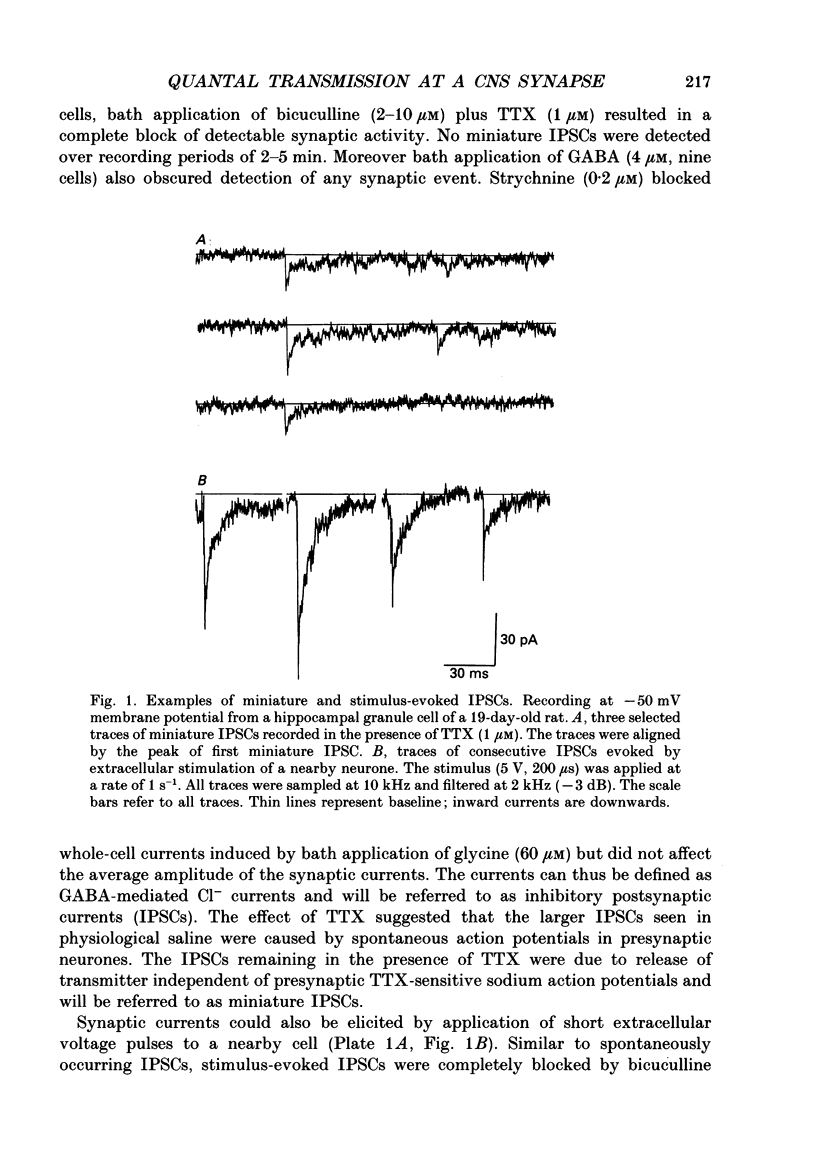
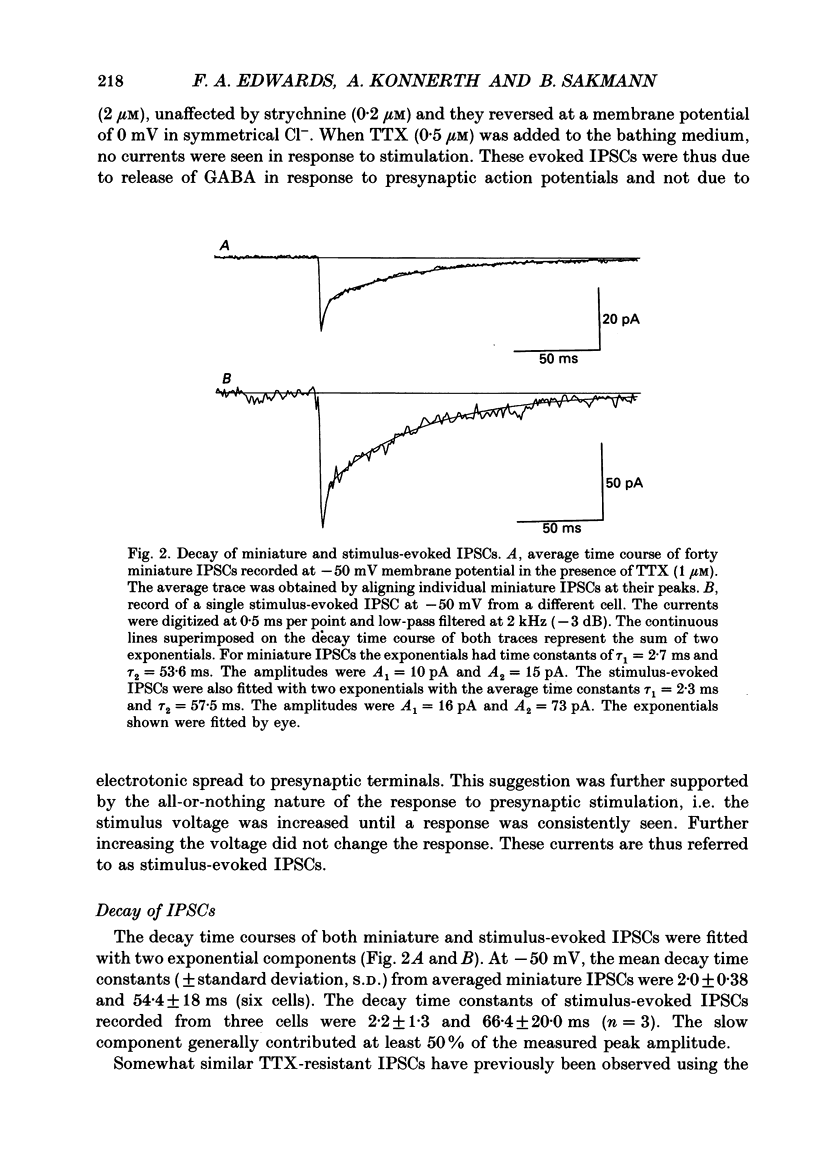
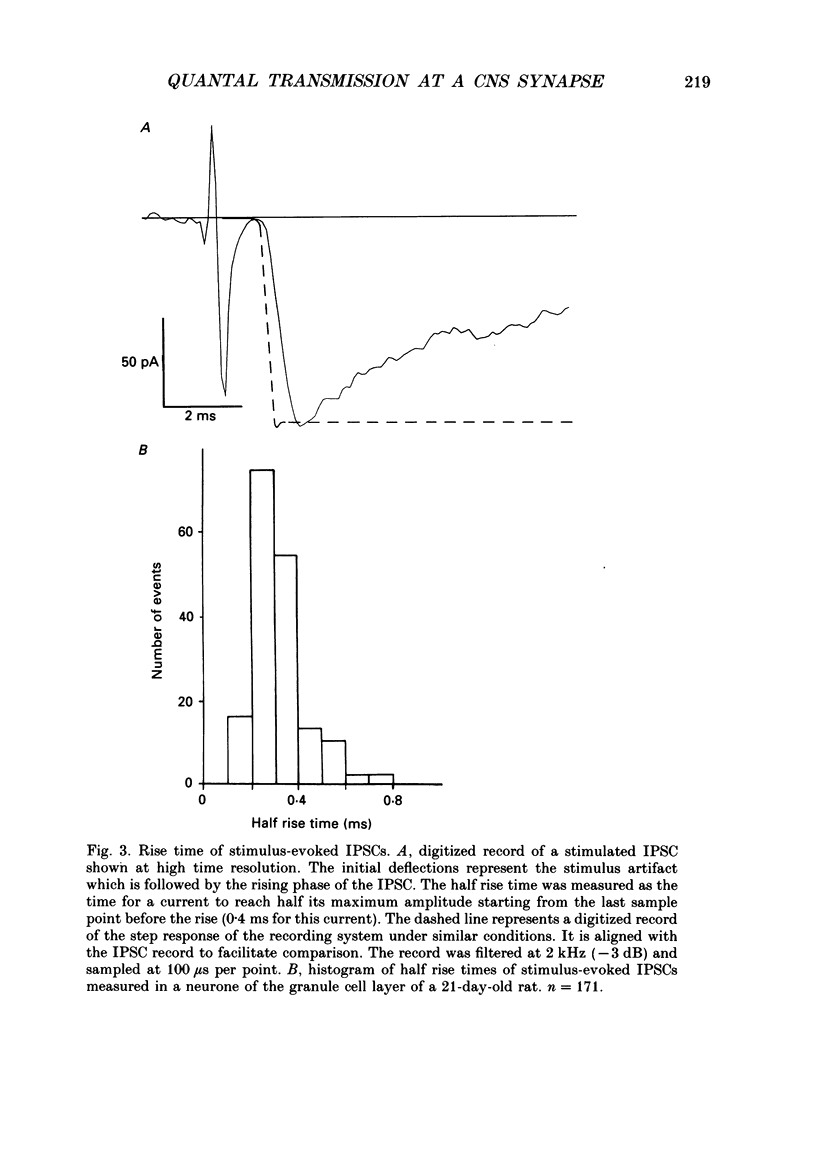
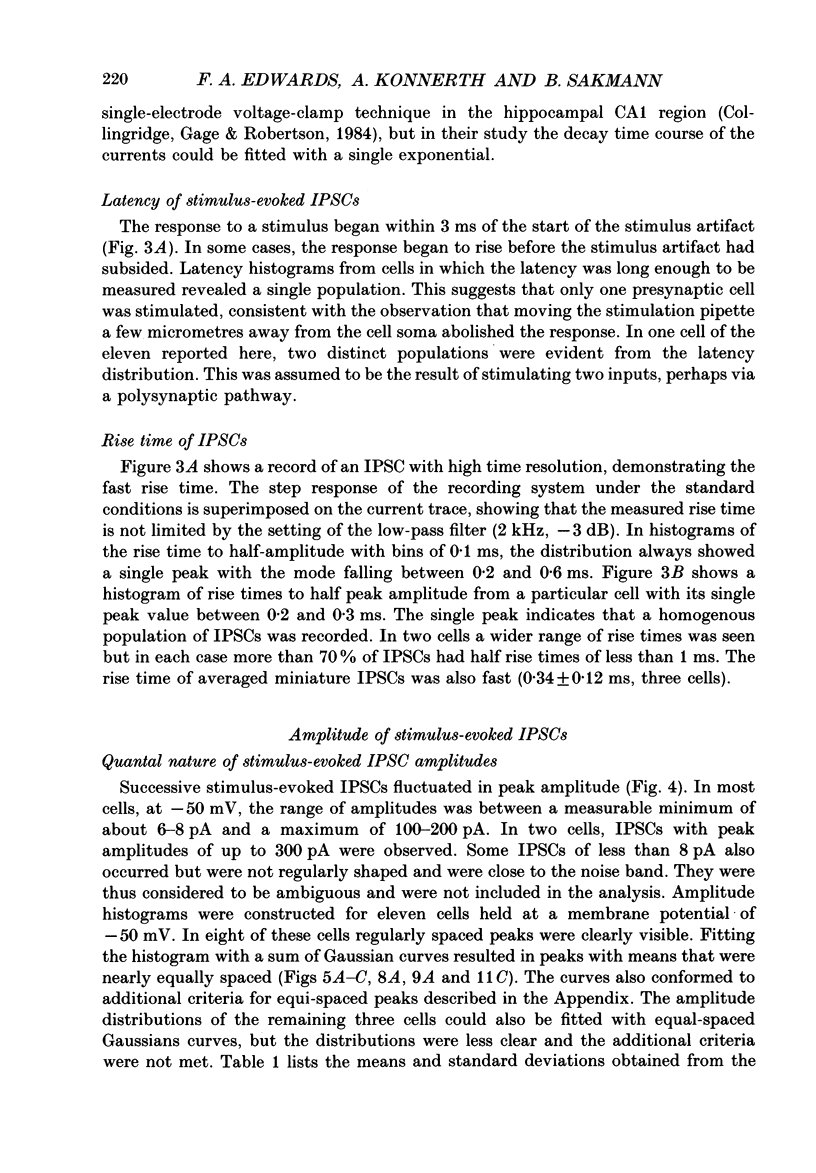
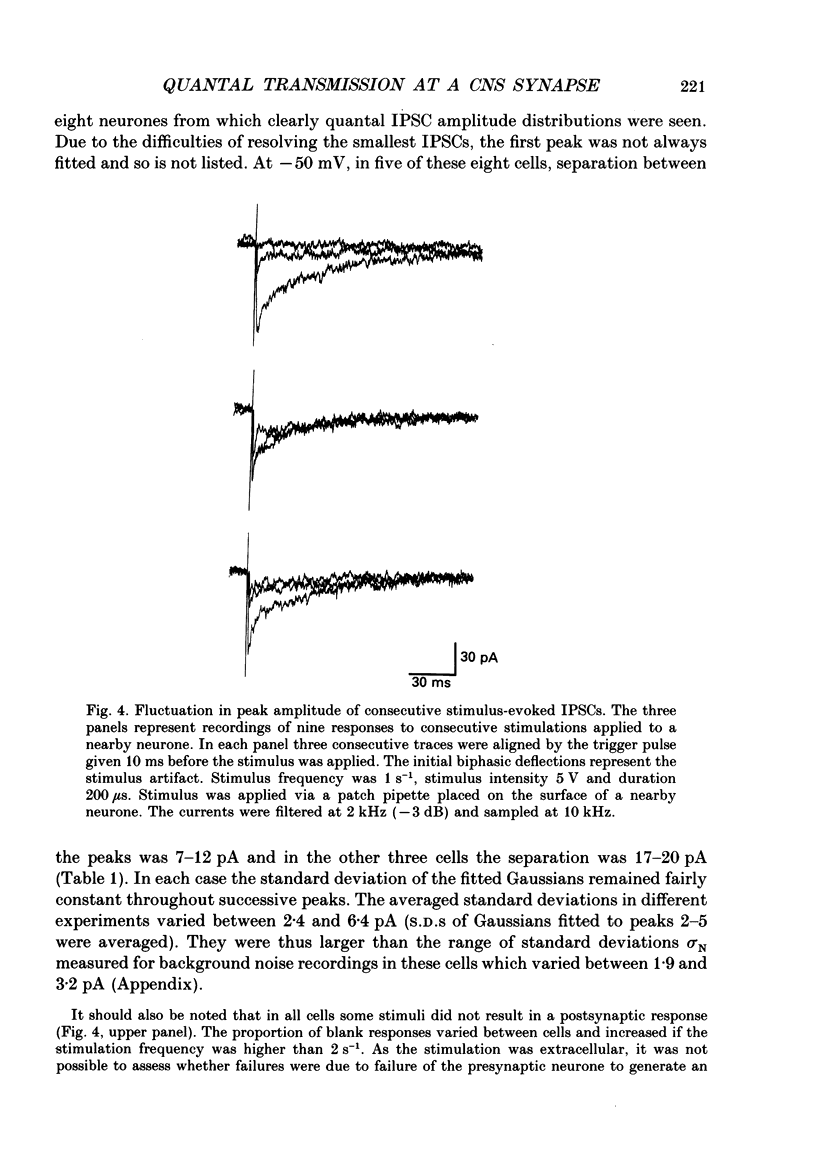
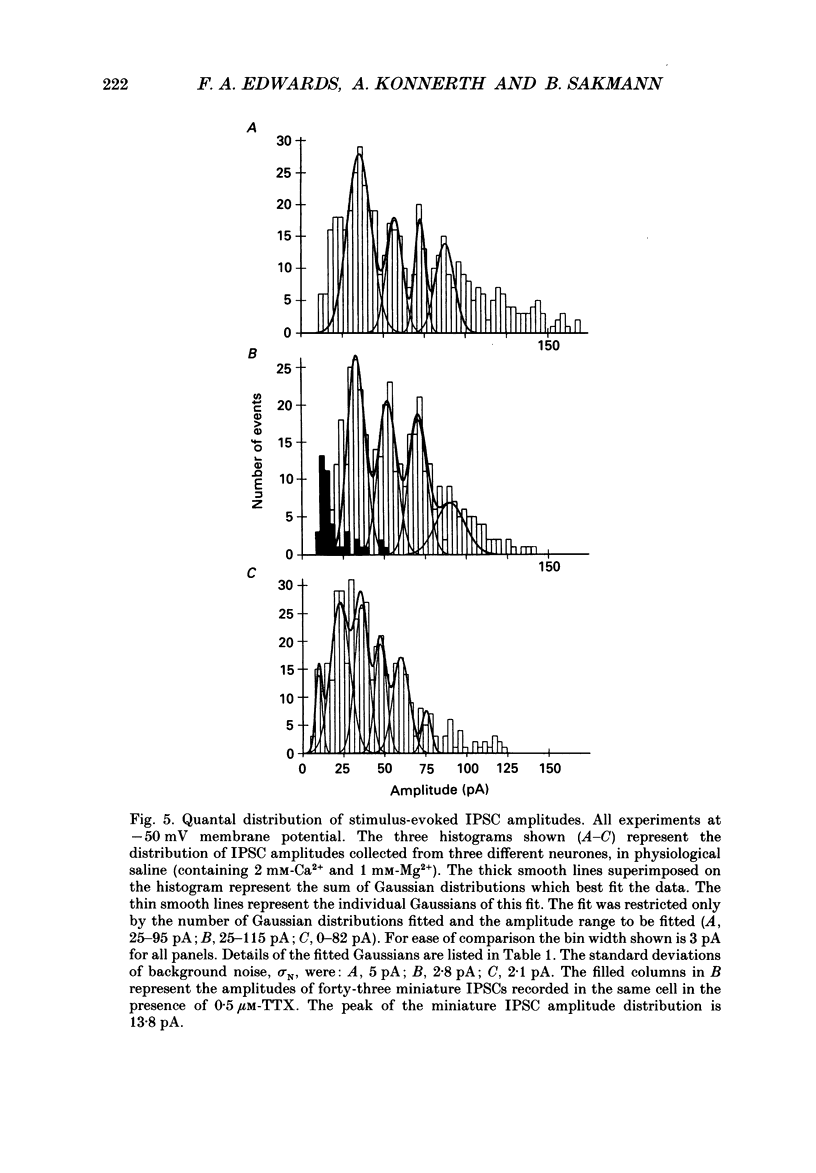
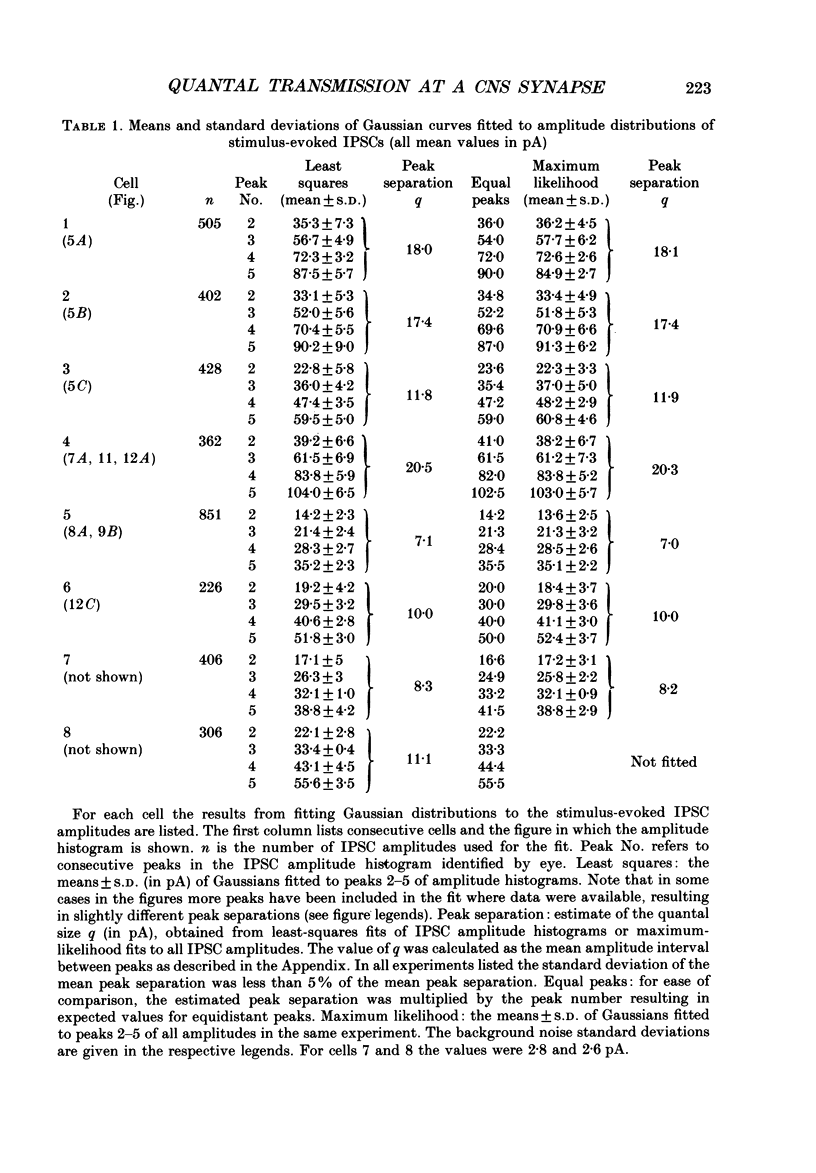
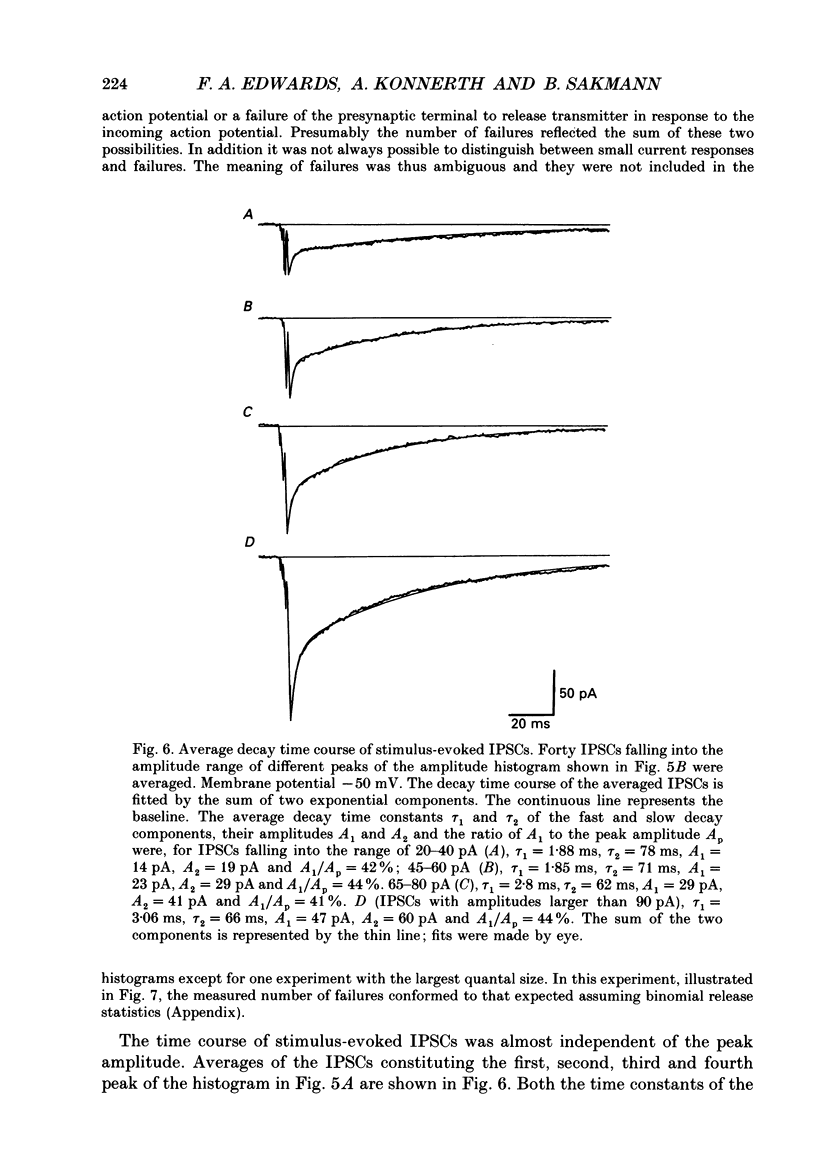
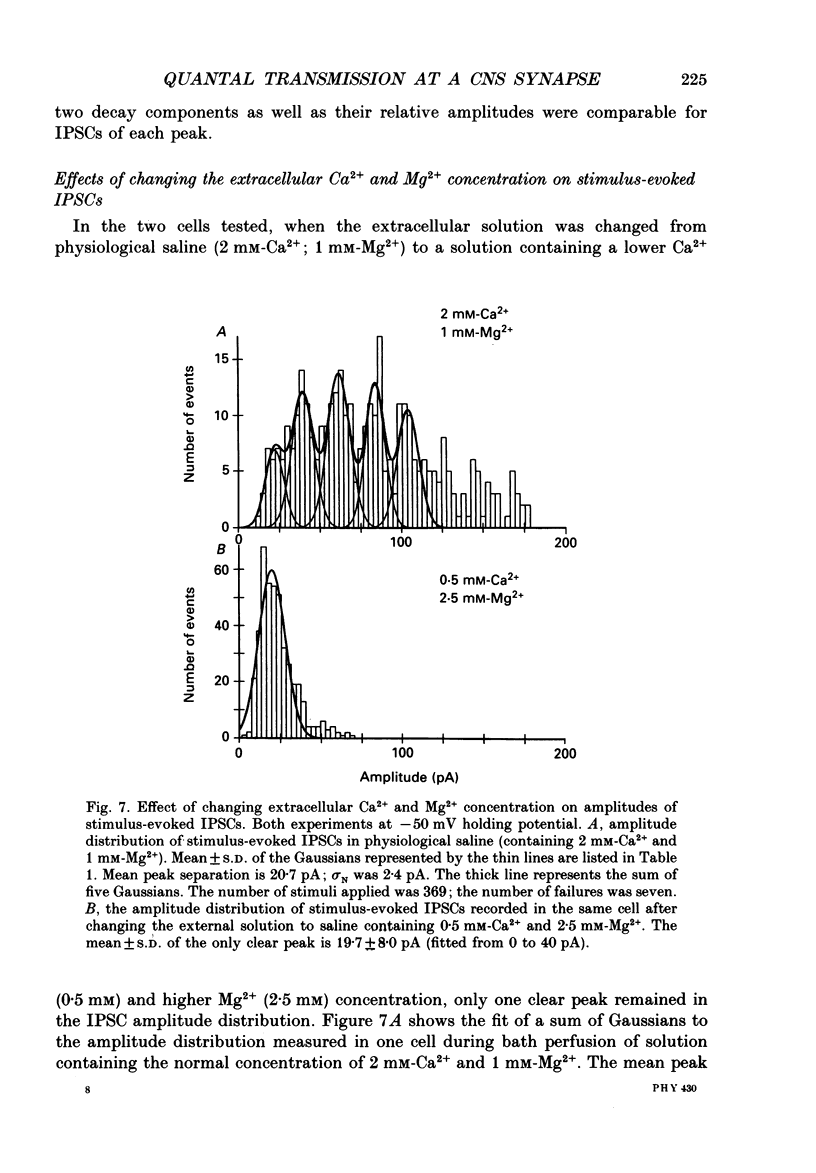
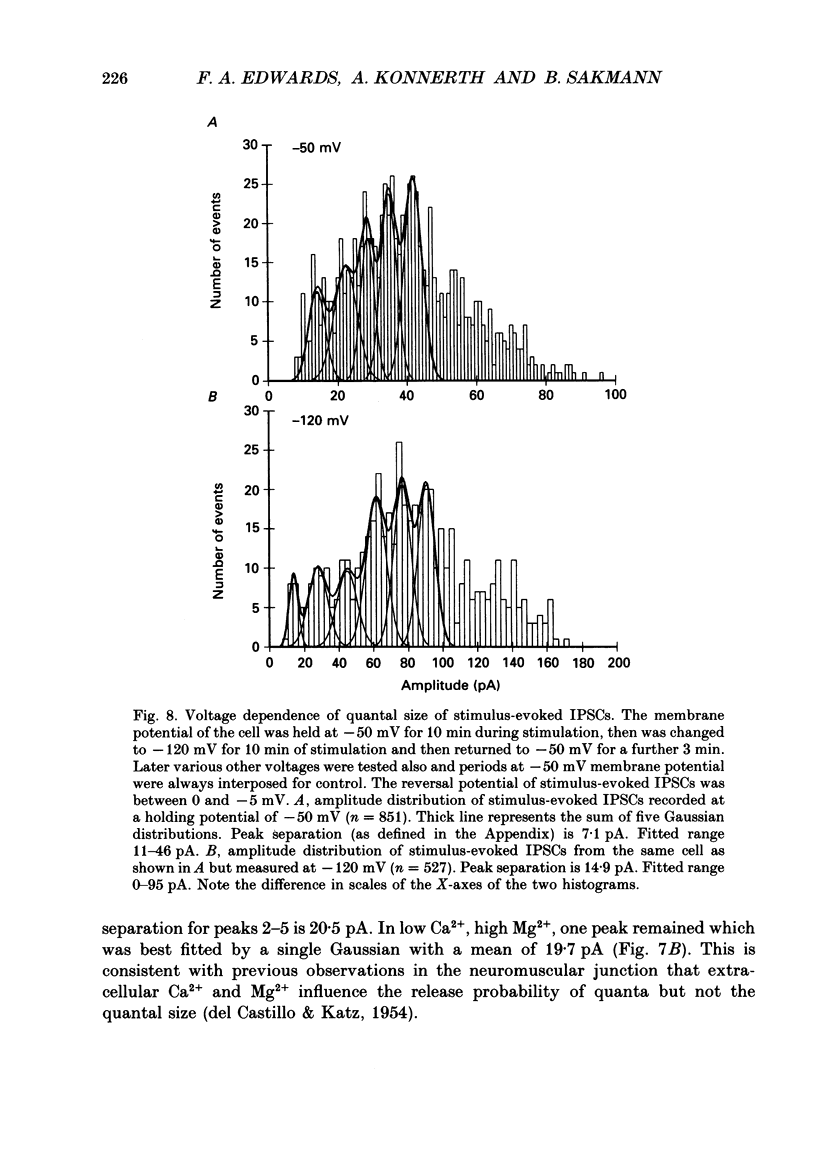
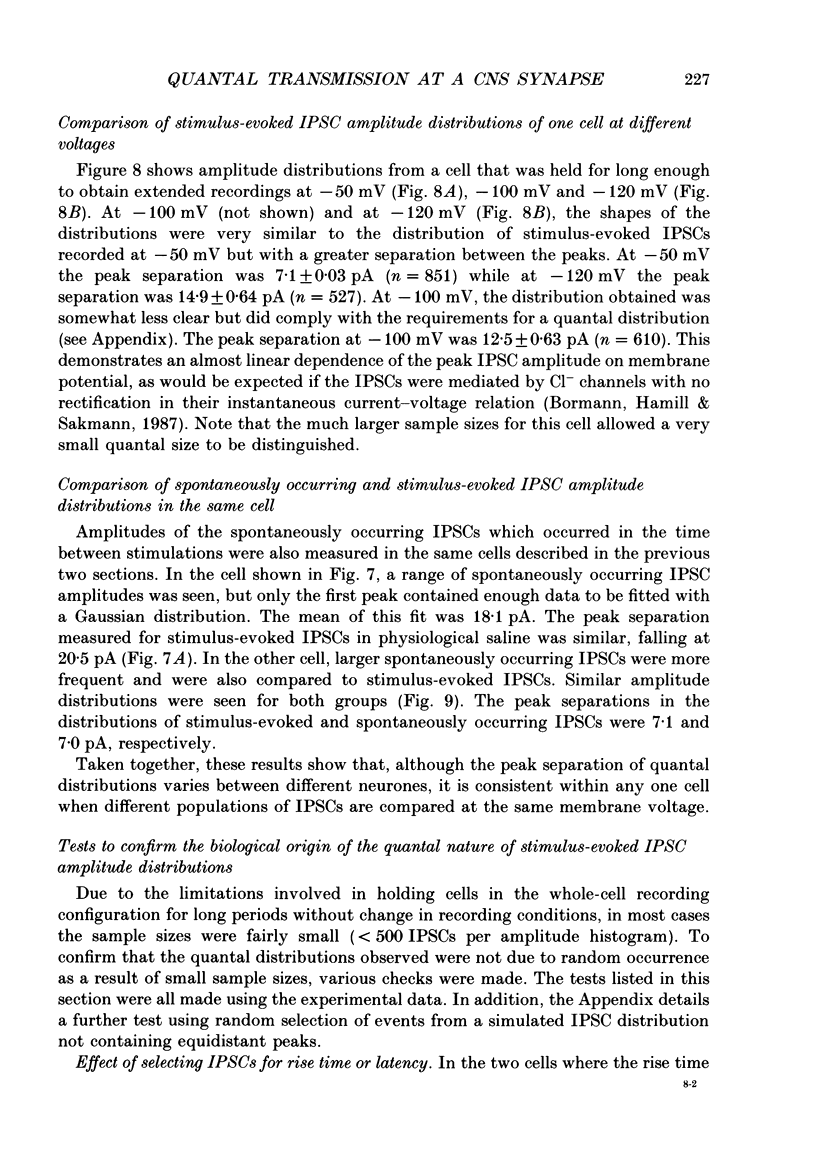
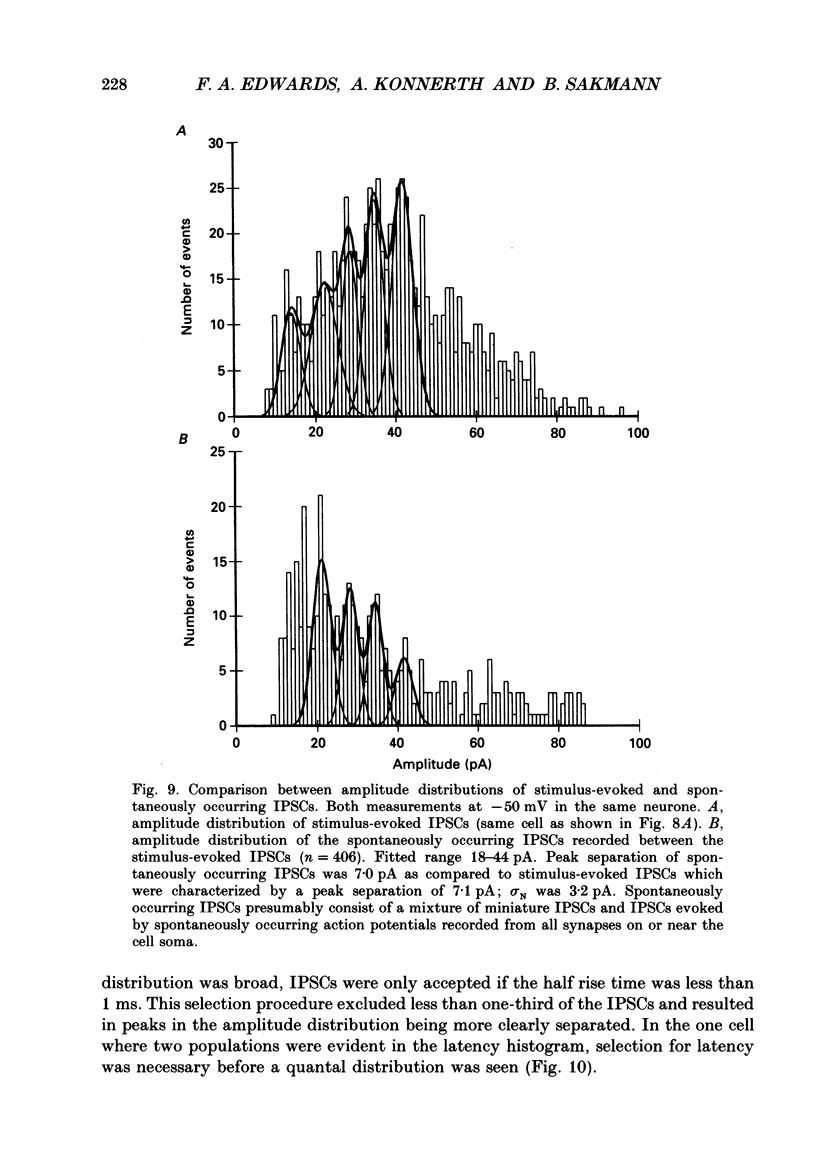
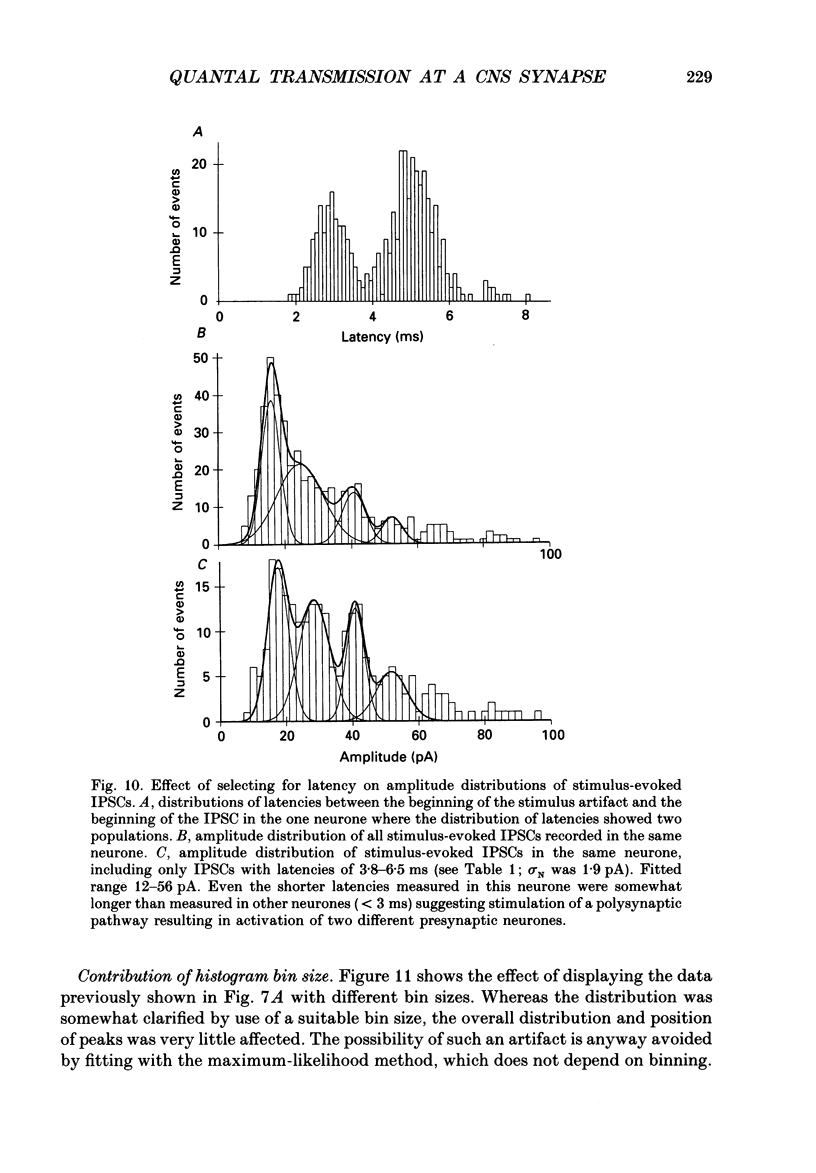
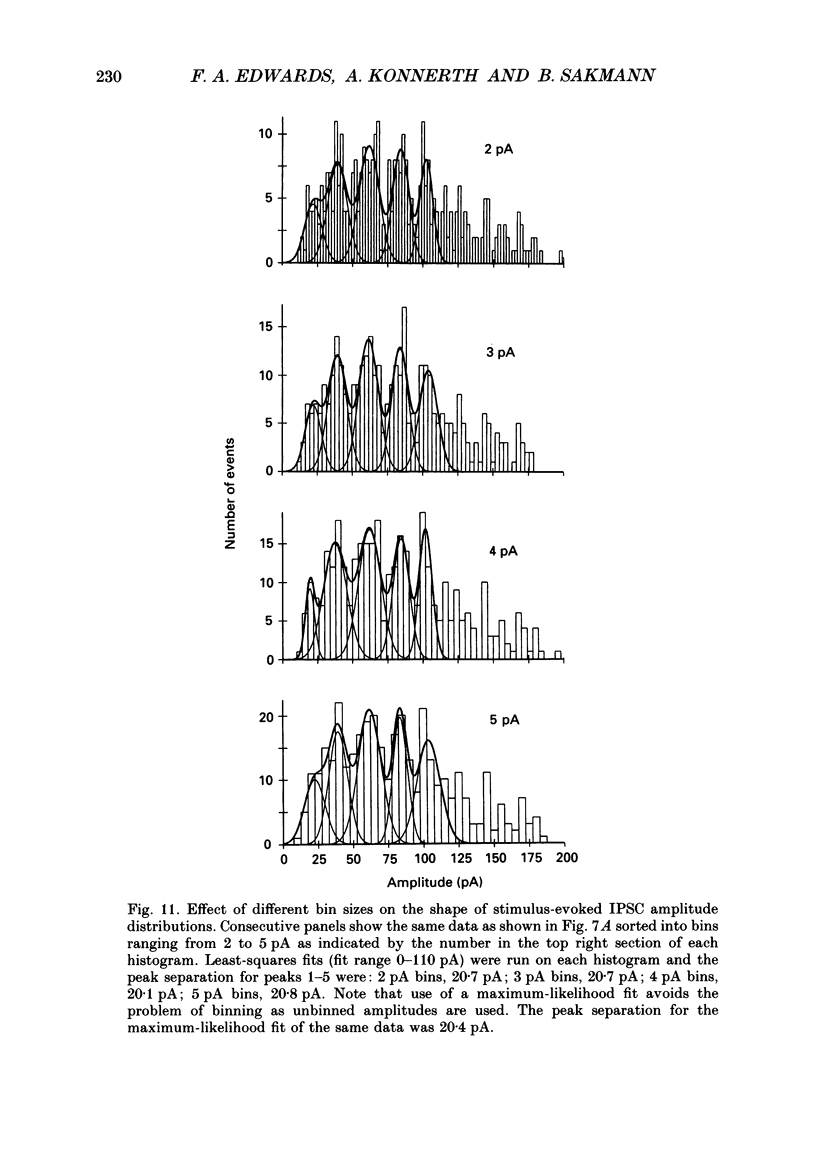
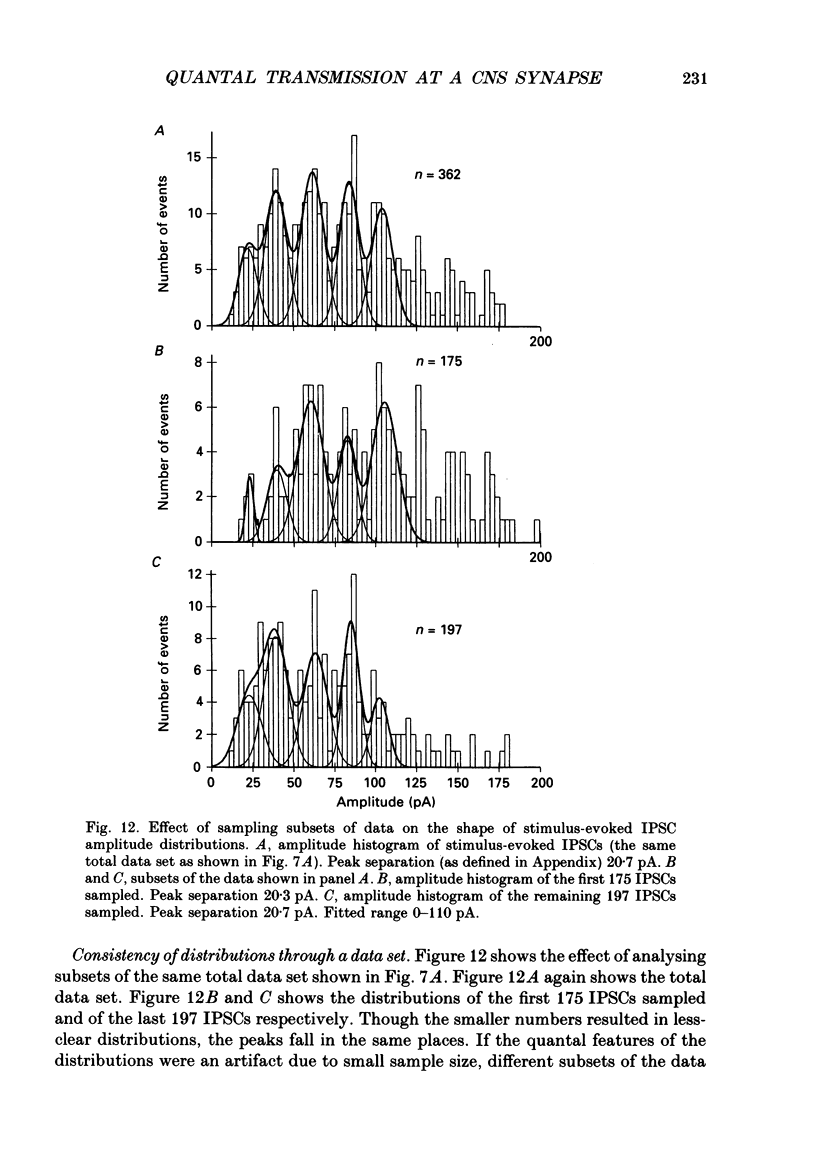
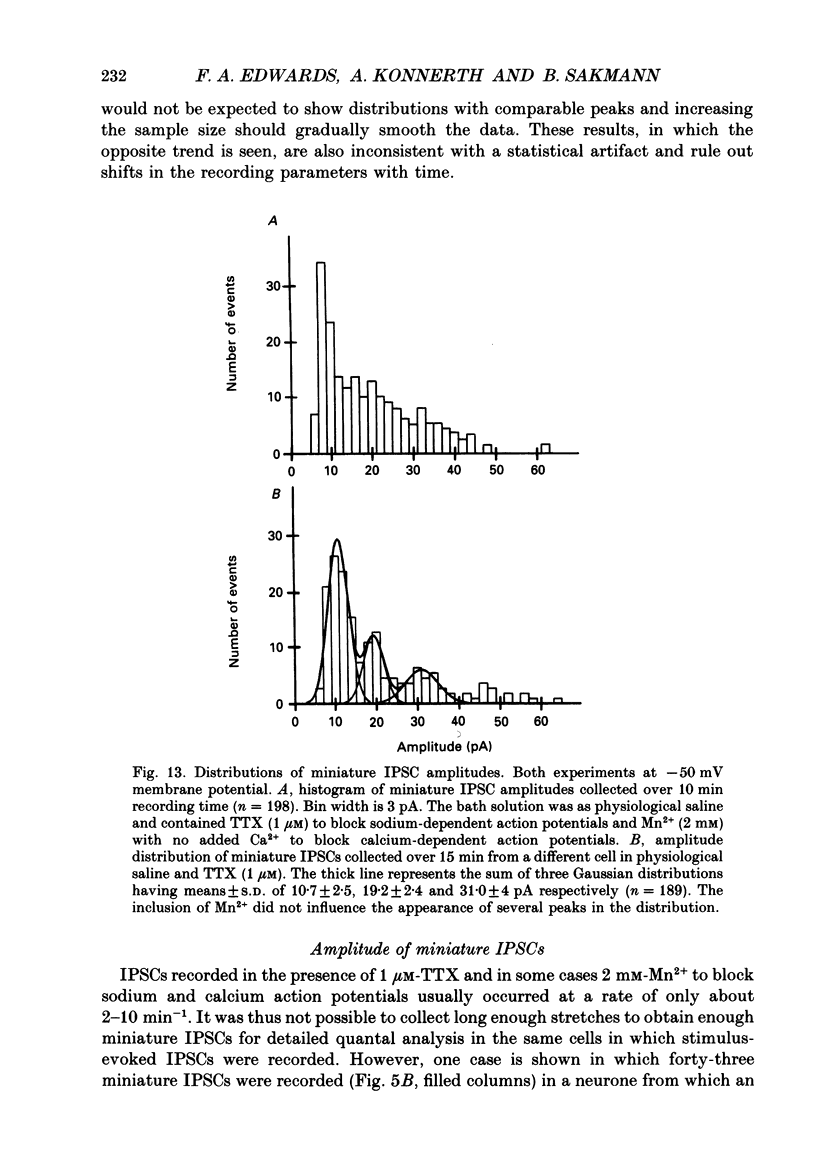
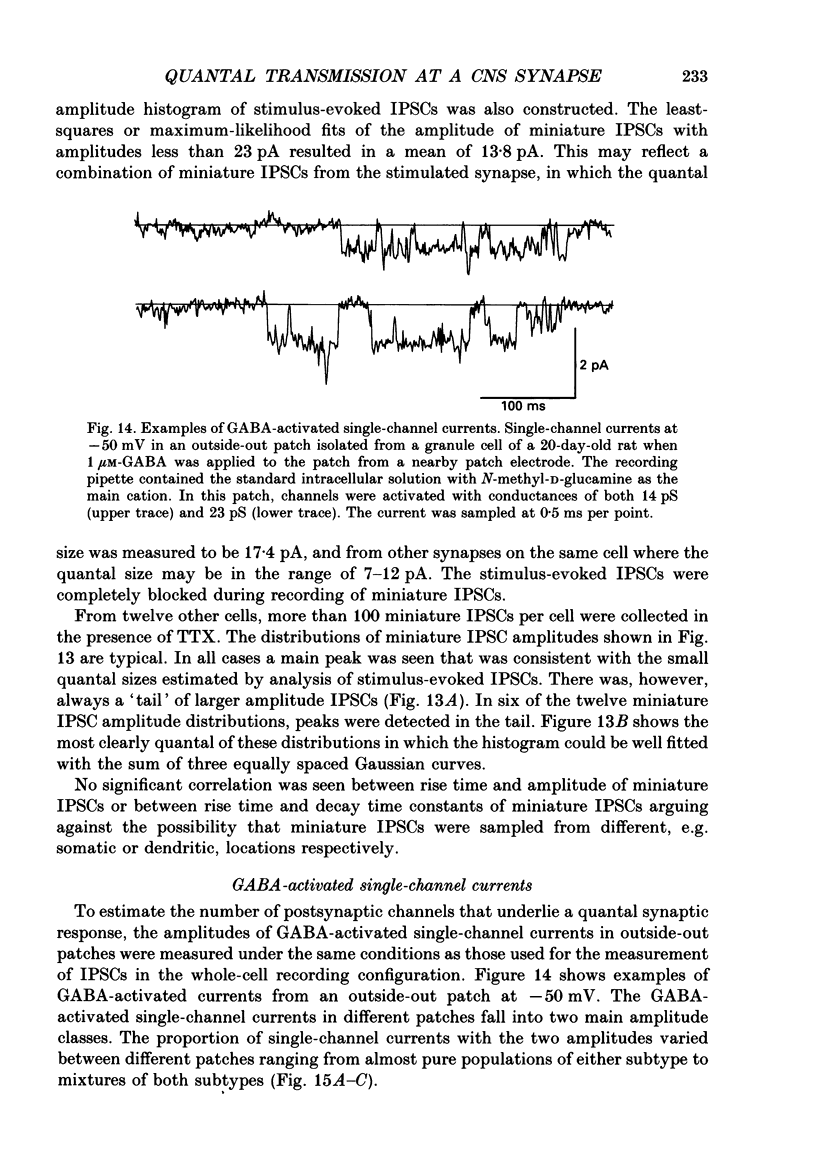
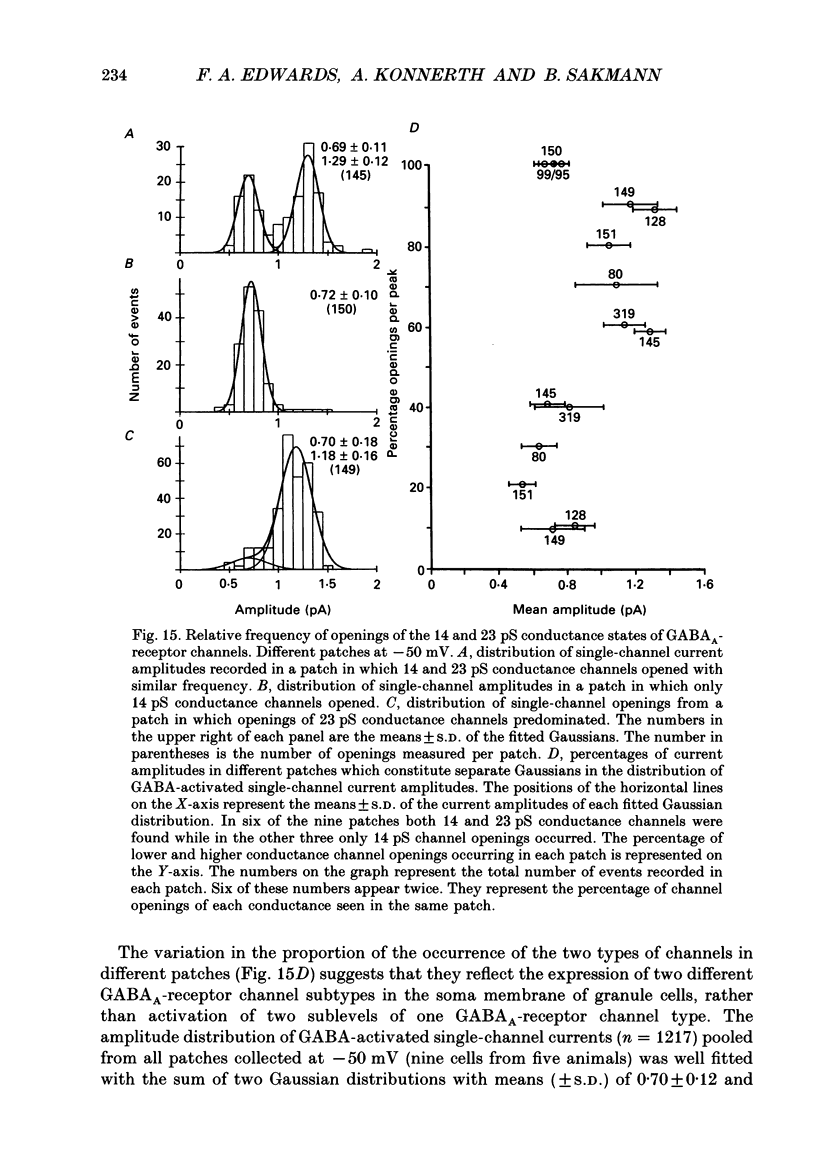
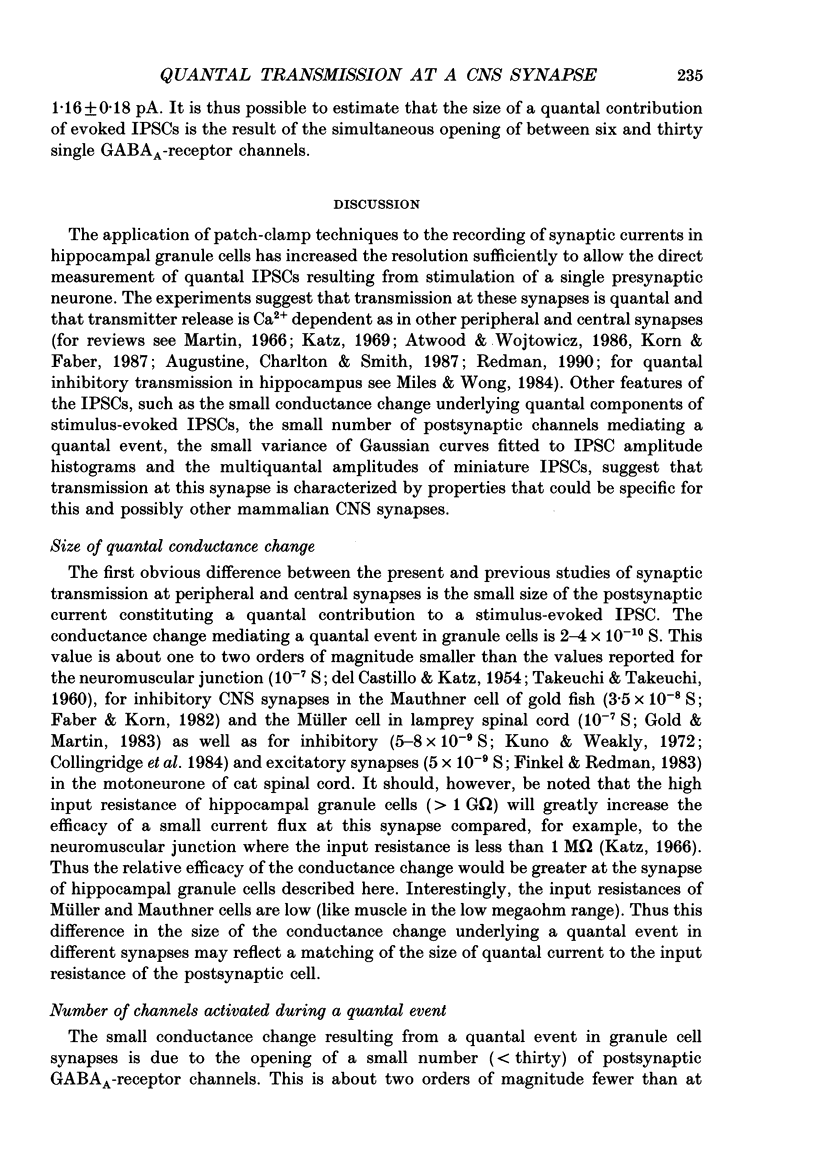
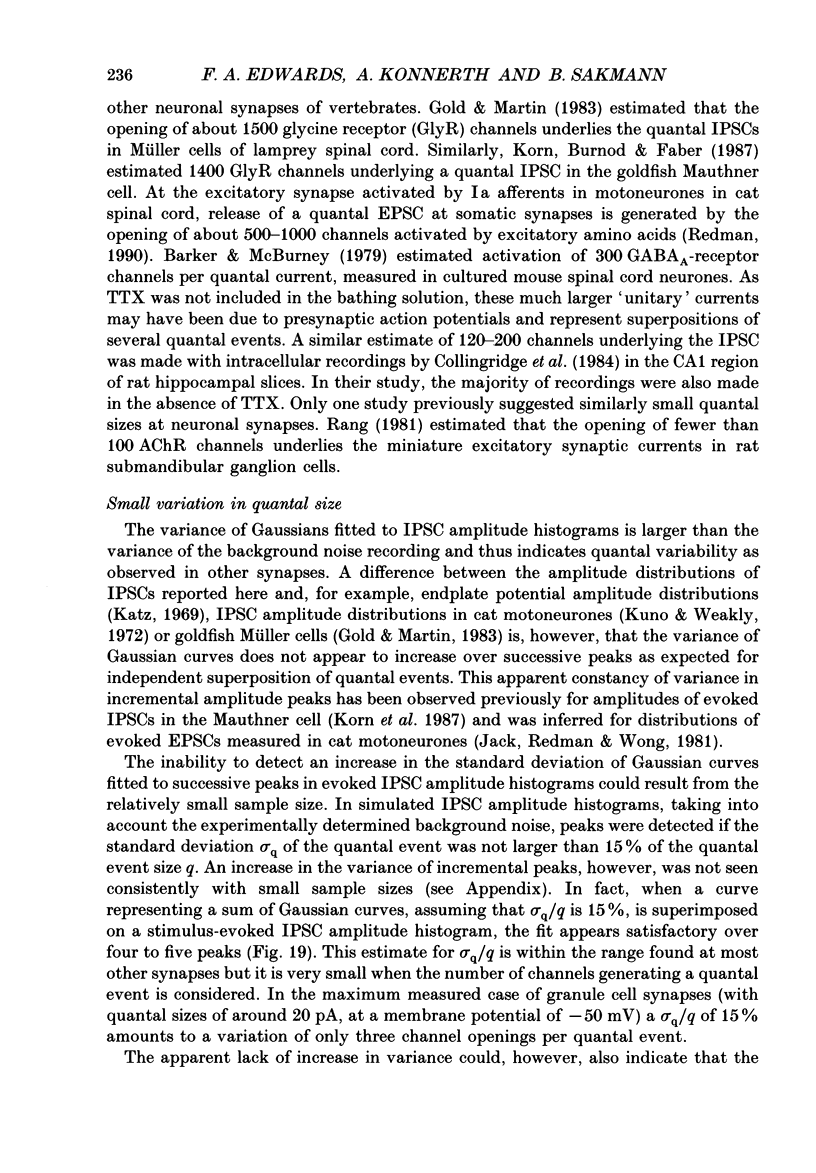
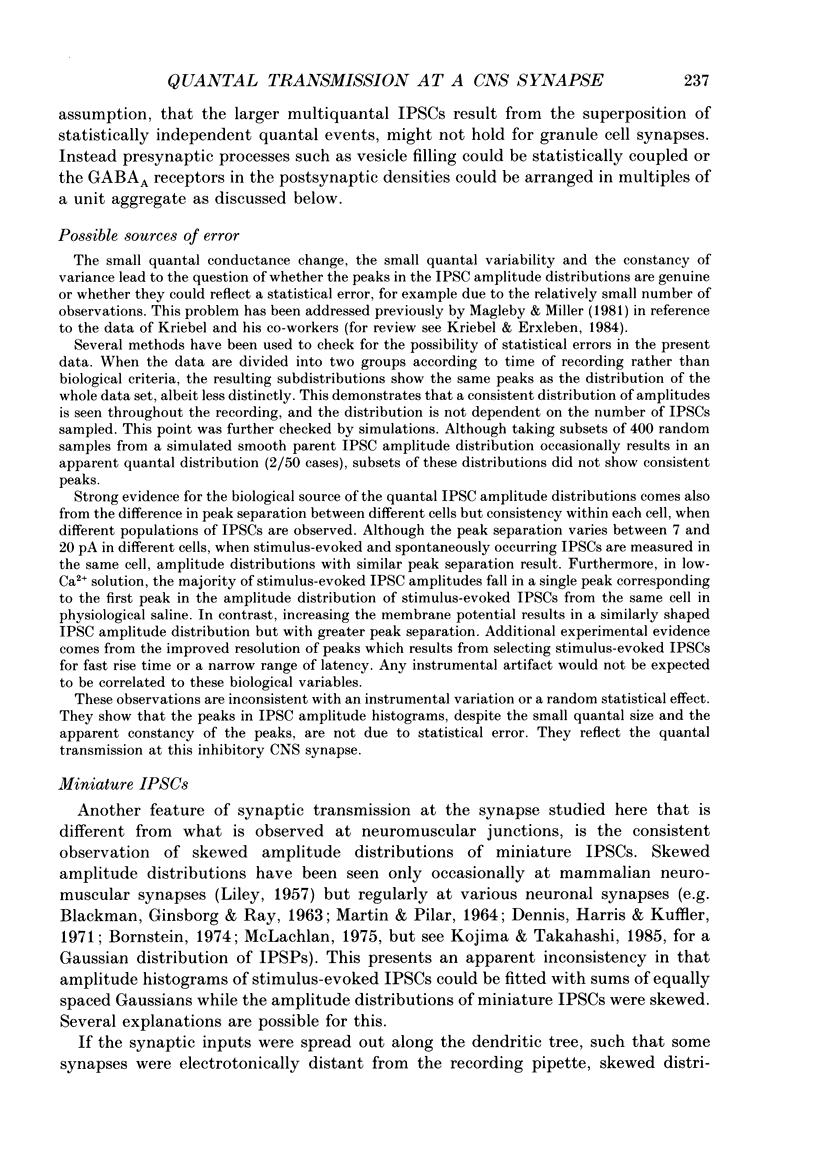
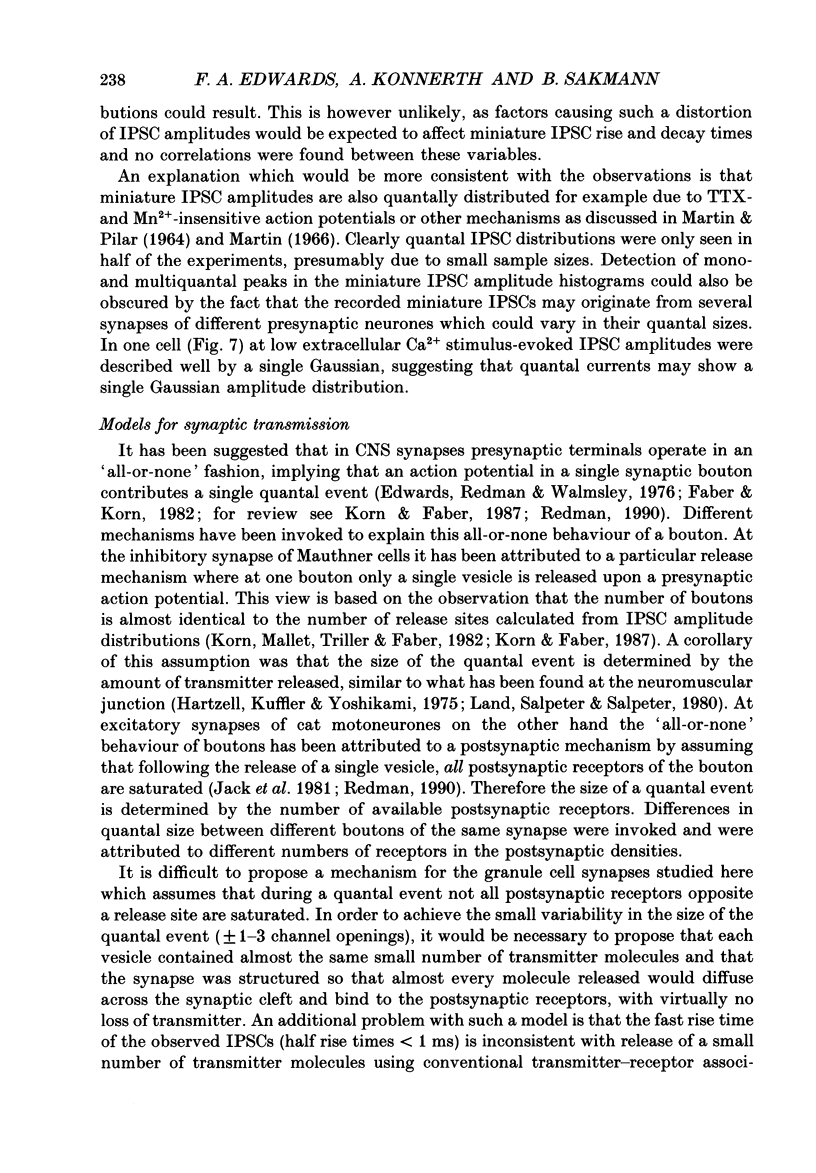
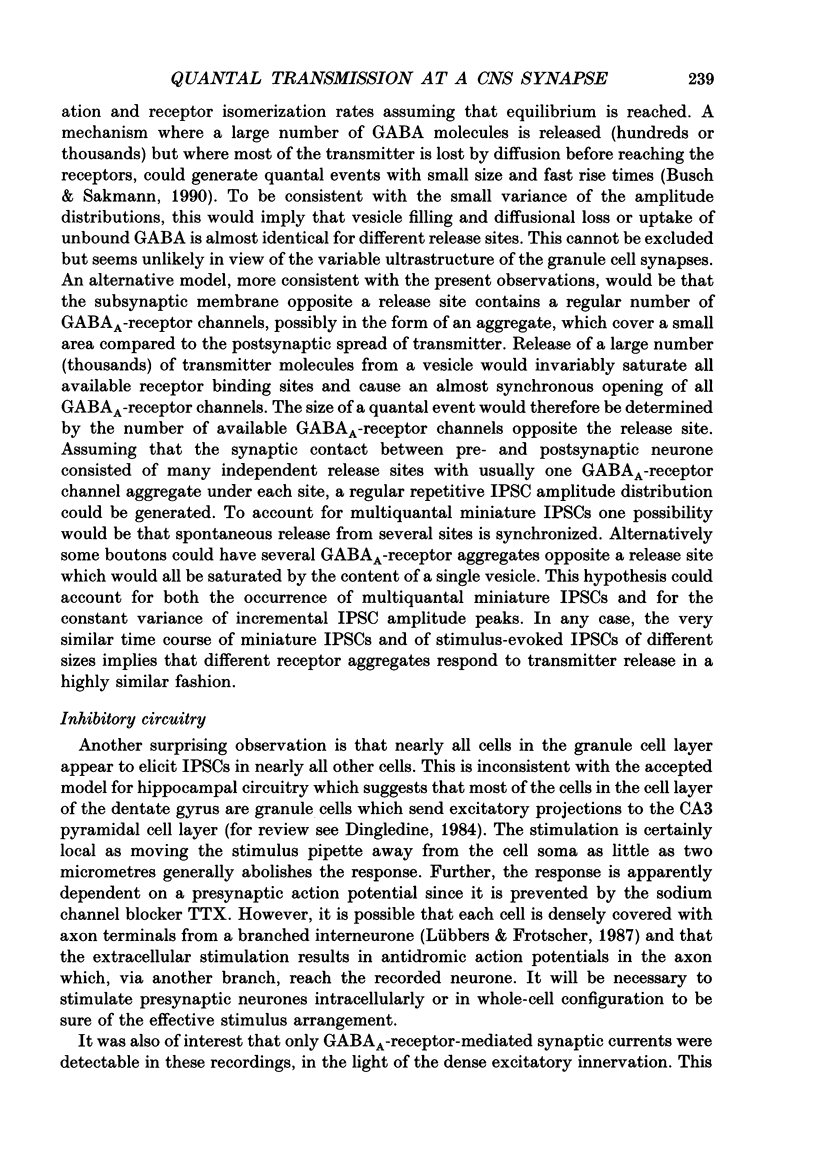
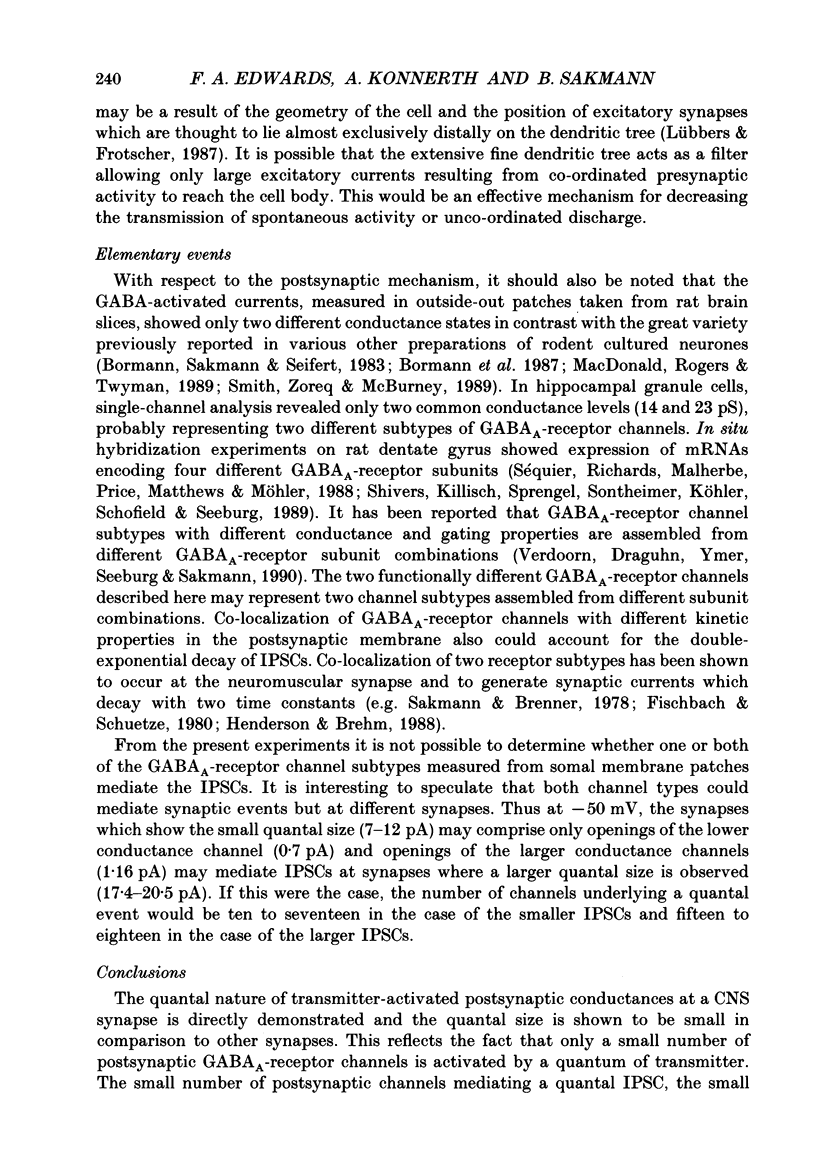
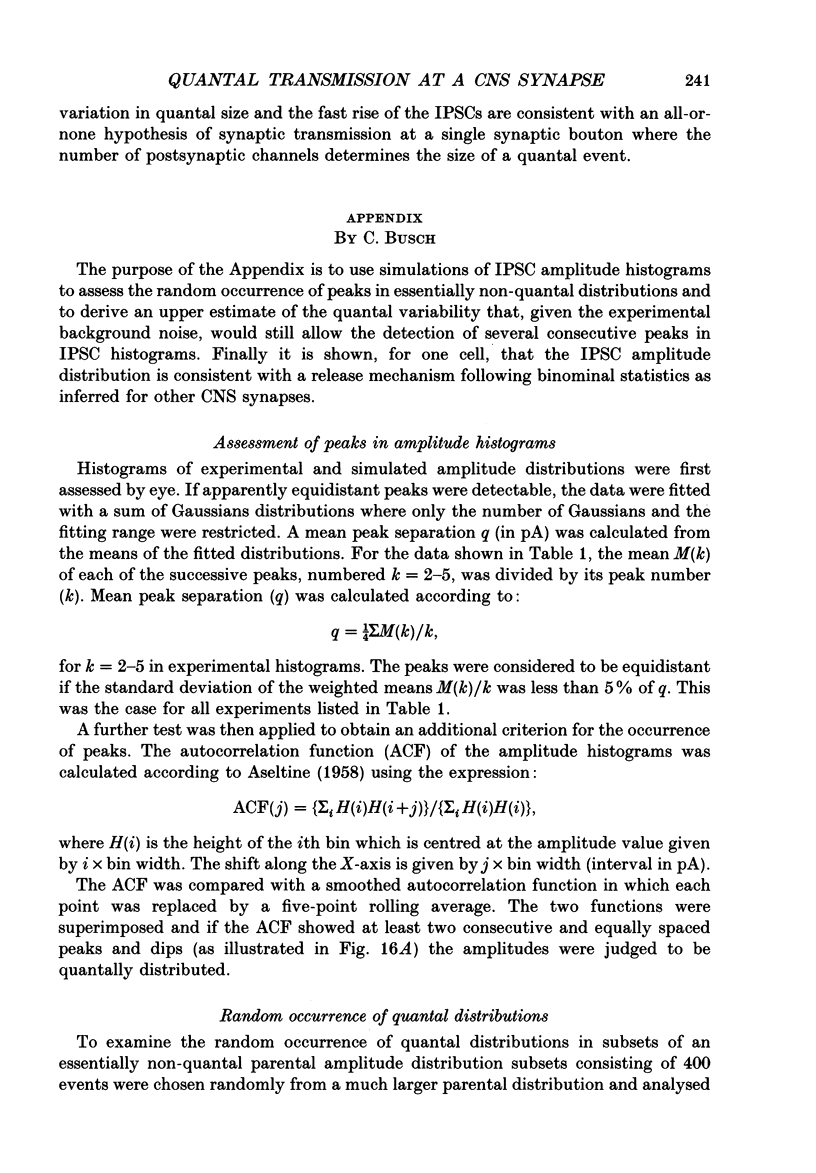
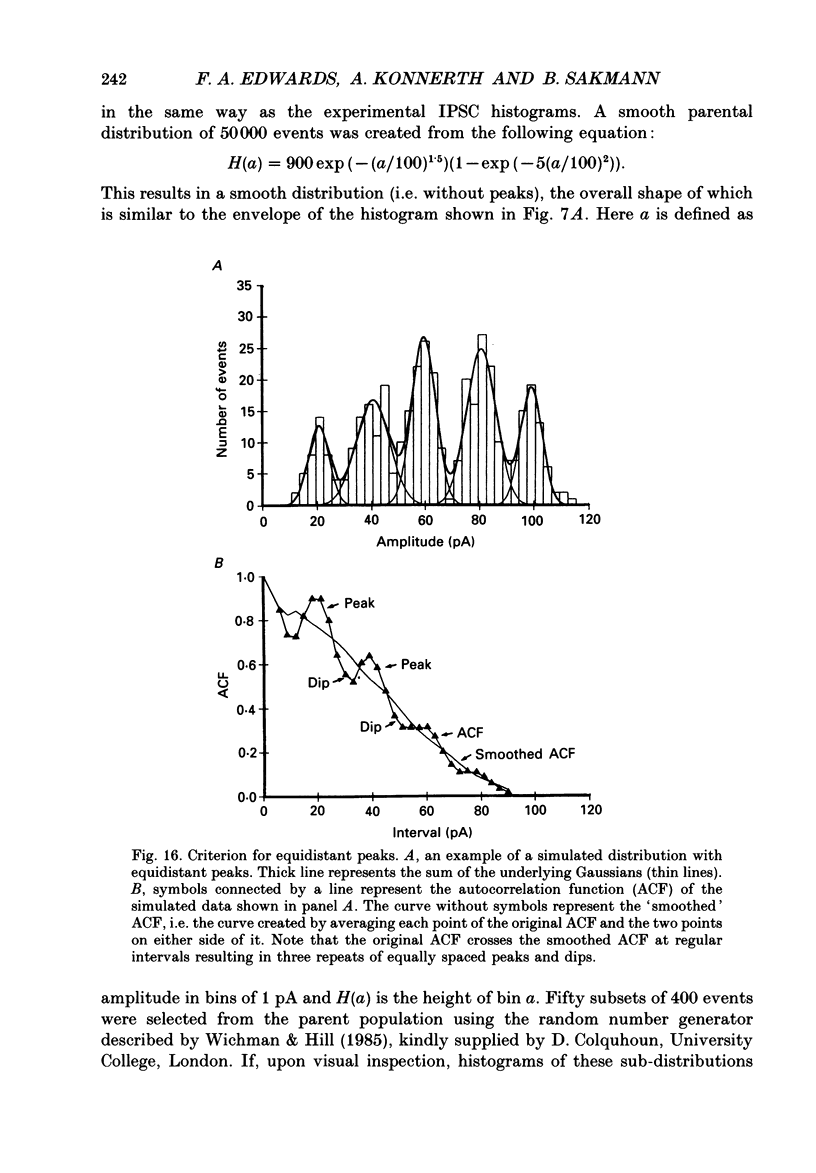
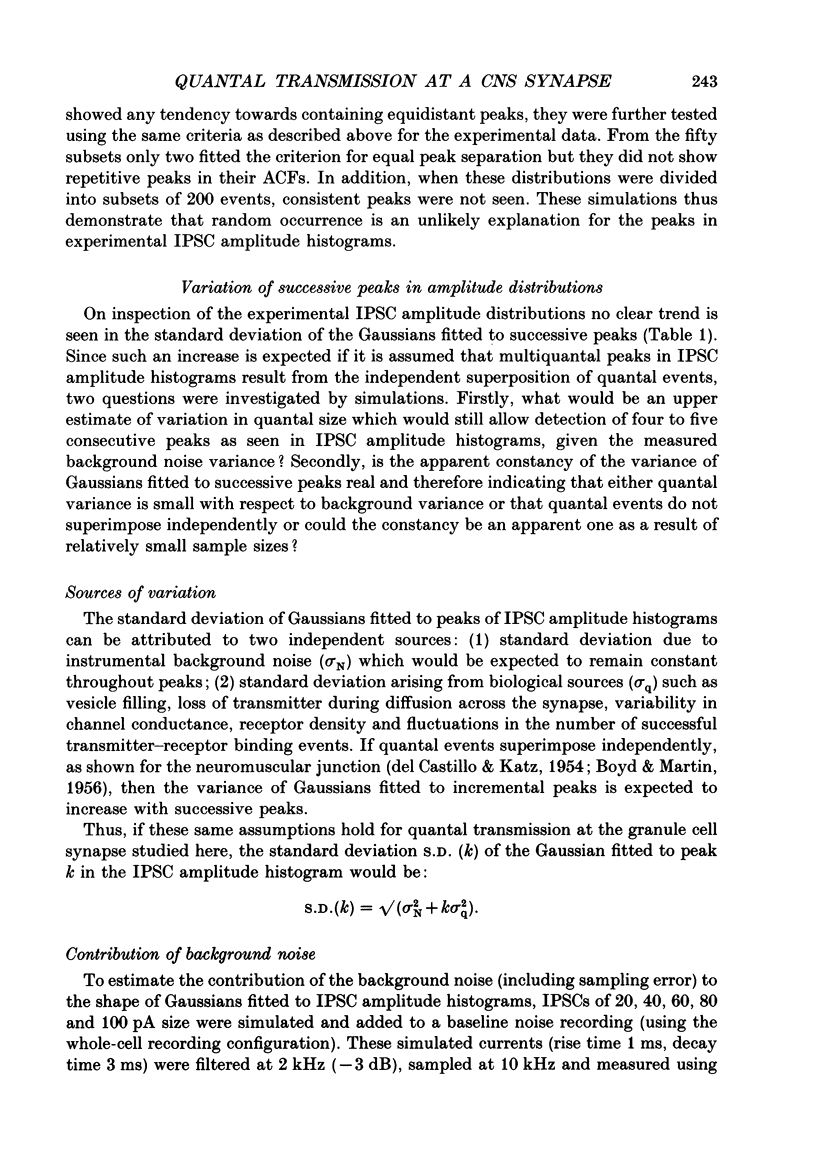
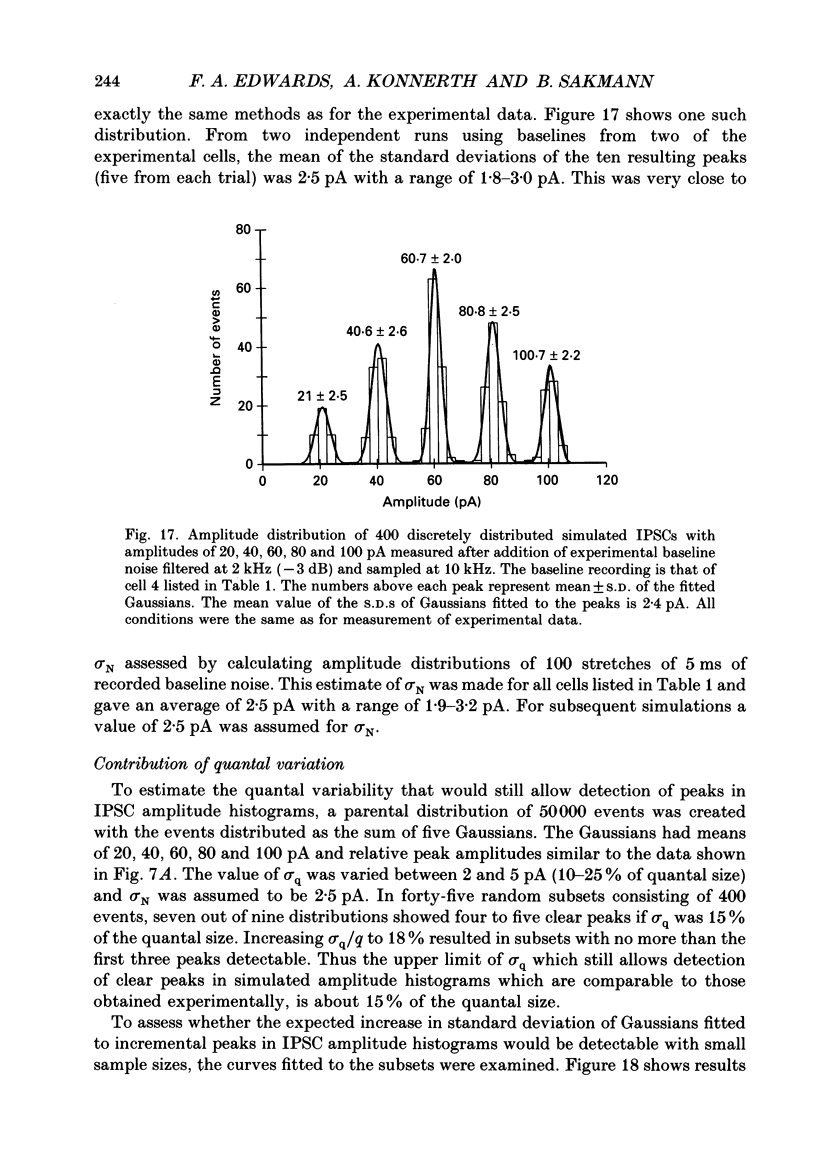
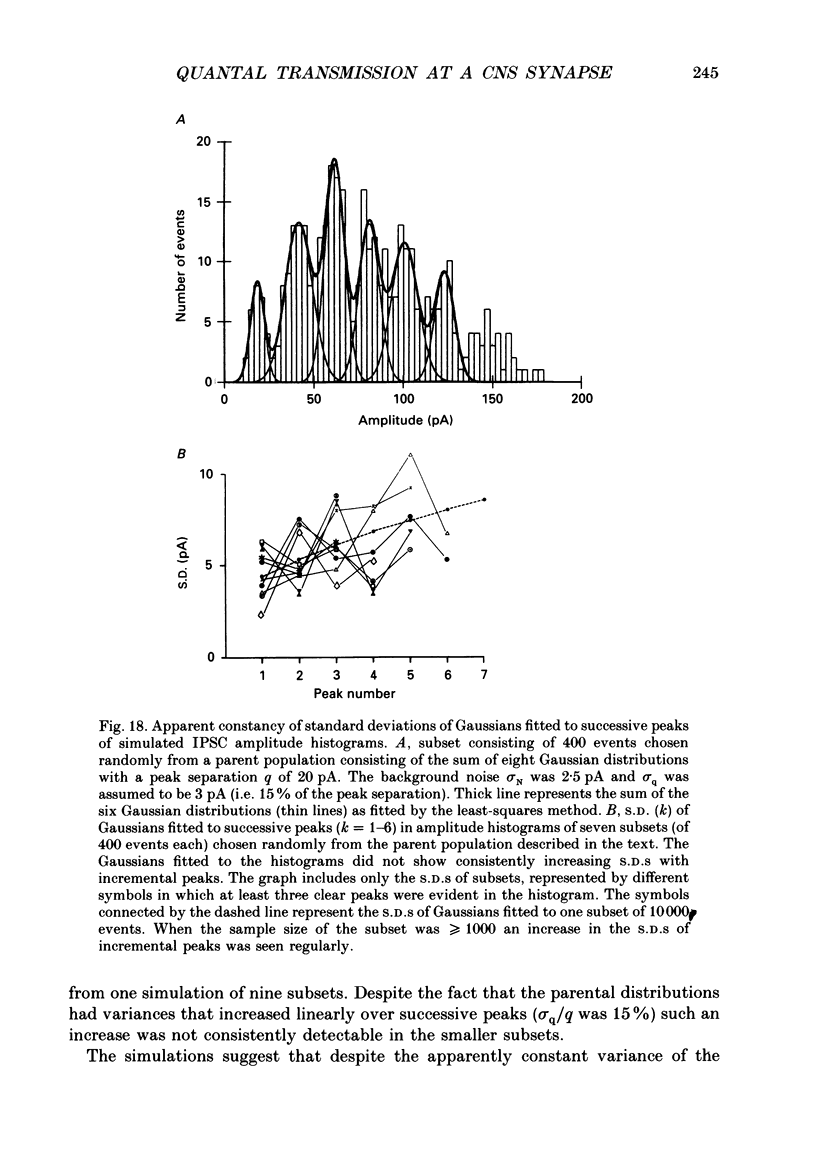
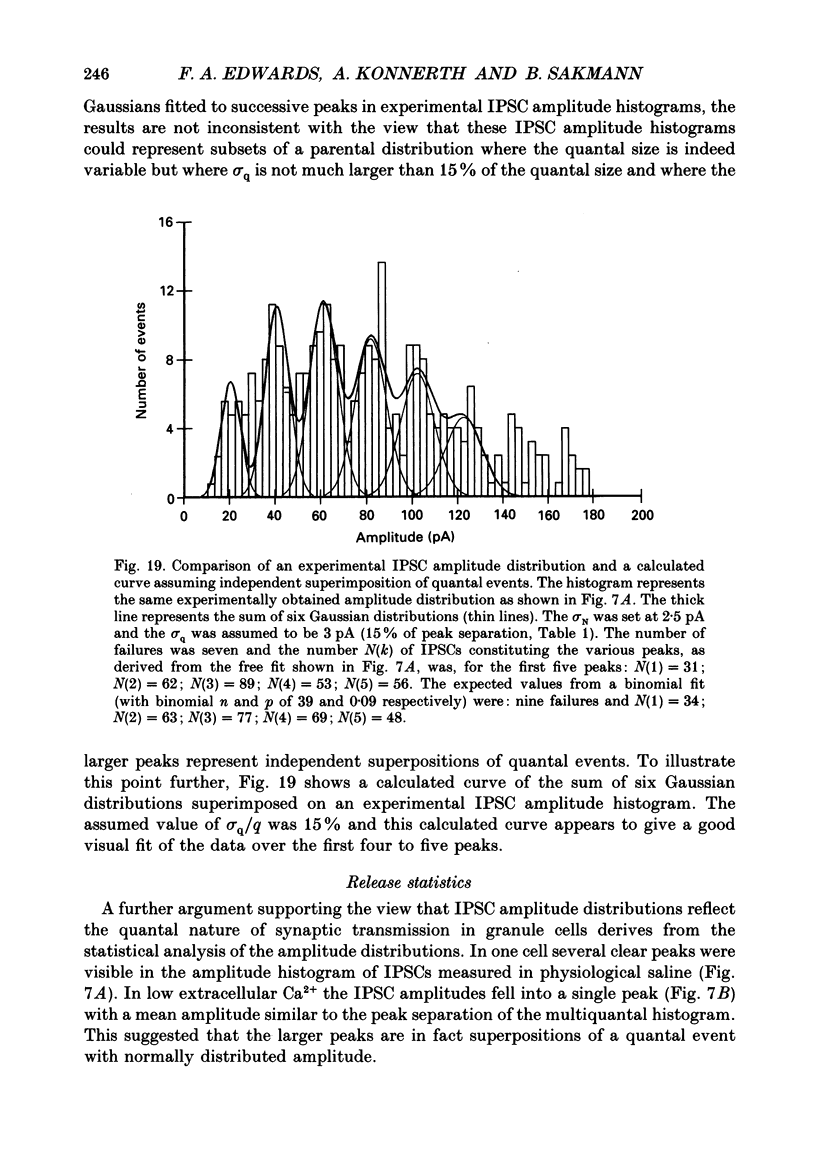
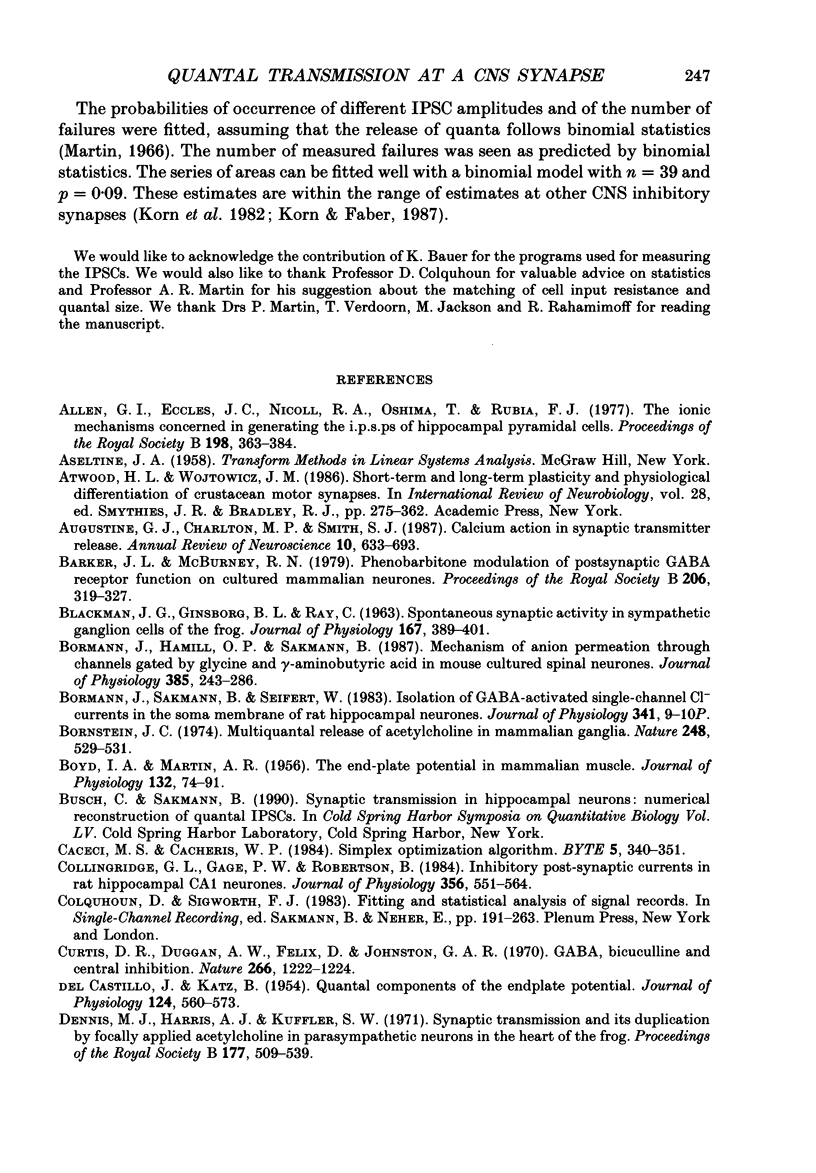
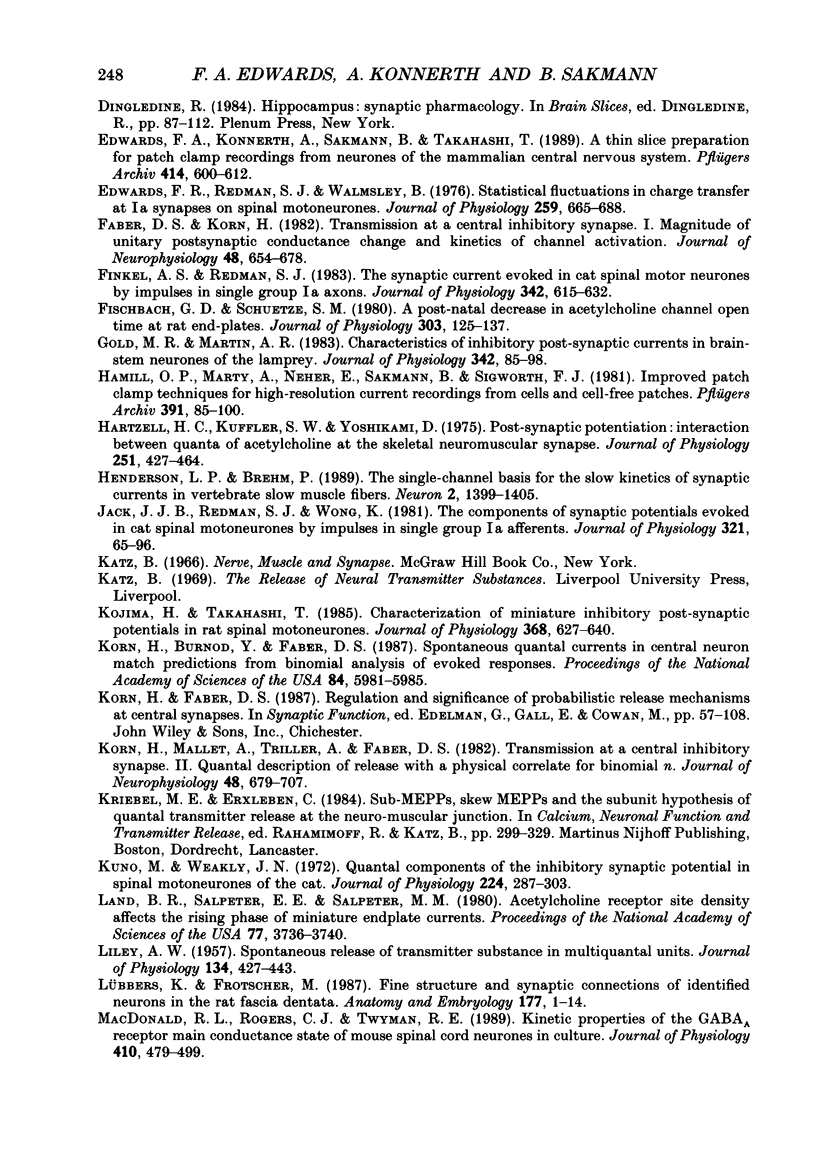
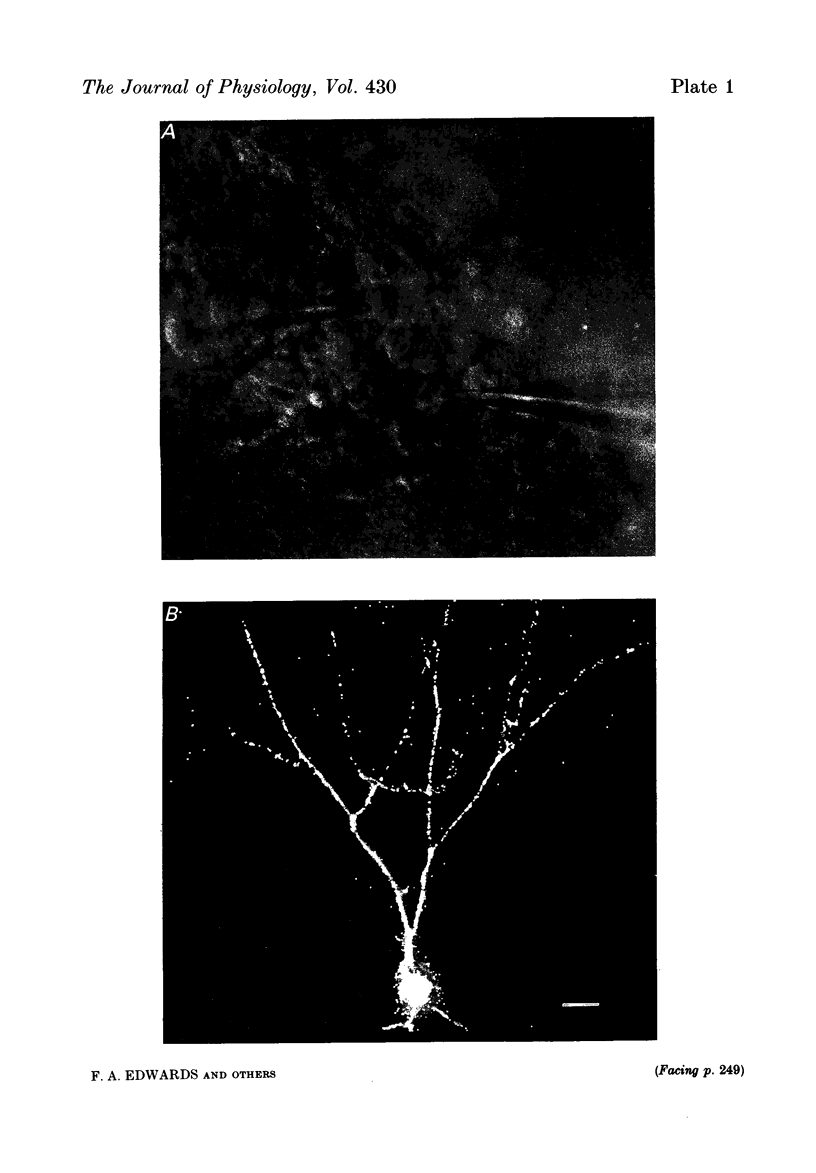
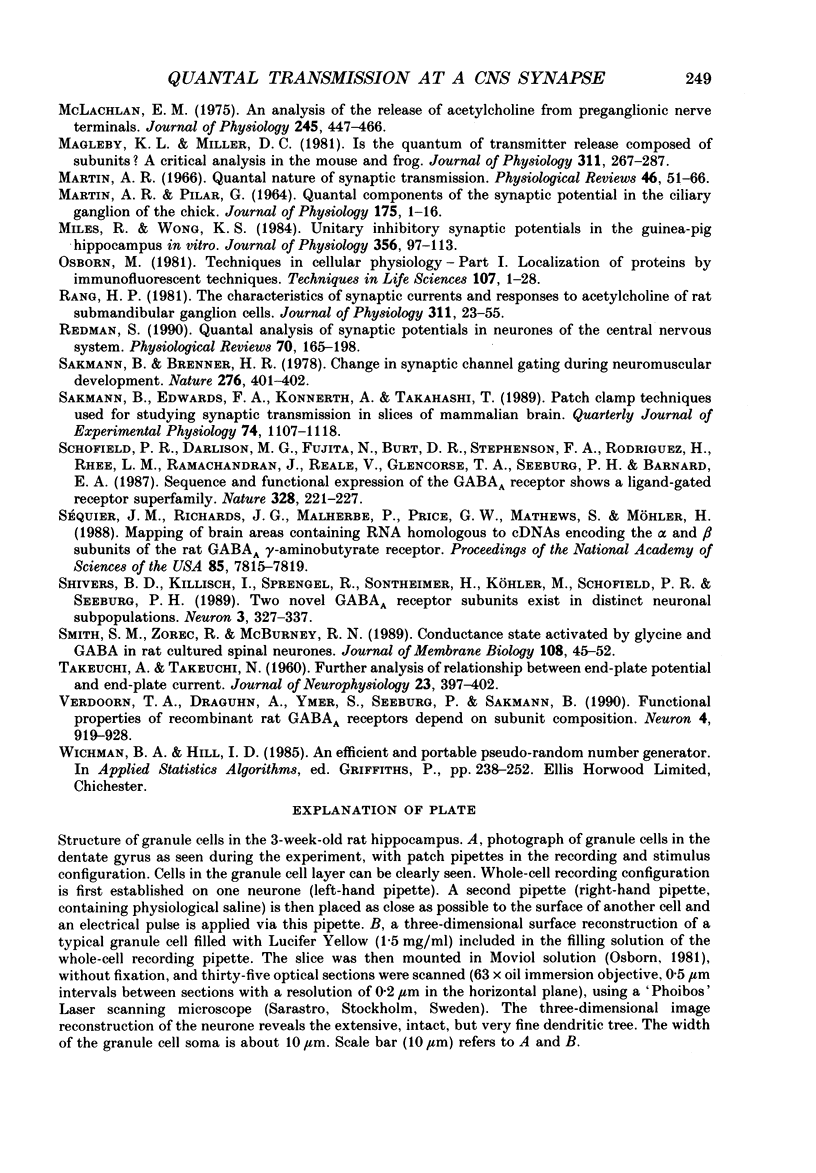
Images in this article
Selected References
These references are in PubMed. This may not be the complete list of references from this article.
- Allen G. I., Eccles J., Nicoll R. A., Oshima T., Rubia F. J. The ionic mechanisms concerned in generating the i.p.s.ps of hippocampal pyramidal cells. Proc R Soc Lond B Biol Sci. 1977 Sep 19;198(1133):363–384. doi: 10.1098/rspb.1977.0103. [DOI] [PubMed] [Google Scholar]
- Atwood H. L., Wojtowicz J. M. Short-term and long-term plasticity and physiological differentiation of crustacean motor synapses. Int Rev Neurobiol. 1986;28:275–362. doi: 10.1016/s0074-7742(08)60111-7. [DOI] [PubMed] [Google Scholar]
- Augustine G. J., Charlton M. P., Smith S. J. Calcium action in synaptic transmitter release. Annu Rev Neurosci. 1987;10:633–693. doi: 10.1146/annurev.ne.10.030187.003221. [DOI] [PubMed] [Google Scholar]
- BLACKMAN J. G., GINSBORG B. L., RAY C. Spontaneous synaptic activity in sympathetic ganglion cells of the frog. J Physiol. 1963 Jul;167:389–401. doi: 10.1113/jphysiol.1963.sp007157. [DOI] [PMC free article] [PubMed] [Google Scholar]
- BOYD I. A., MARTIN A. R. The end-plate potential in mammalian muscle. J Physiol. 1956 Apr 27;132(1):74–91. doi: 10.1113/jphysiol.1956.sp005503. [DOI] [PMC free article] [PubMed] [Google Scholar]
- Barker J. L., McBurney R. N. Phenobarbitone modulation of postsynaptic GABA receptor function on cultured mammalian neurons. Proc R Soc Lond B Biol Sci. 1979 Dec 31;206(1164):319–327. doi: 10.1098/rspb.1979.0108. [DOI] [PubMed] [Google Scholar]
- Bormann J., Hamill O. P., Sakmann B. Mechanism of anion permeation through channels gated by glycine and gamma-aminobutyric acid in mouse cultured spinal neurones. J Physiol. 1987 Apr;385:243–286. doi: 10.1113/jphysiol.1987.sp016493. [DOI] [PMC free article] [PubMed] [Google Scholar]
- Bornstein J. C. Multiquantal release of acetylcholine in mammalian ganglia. Nature. 1974 Apr 5;248(448):529–531. doi: 10.1038/248529a0. [DOI] [PubMed] [Google Scholar]
- Collingridge G. L., Gage P. W., Robertson B. Inhibitory post-synaptic currents in rat hippocampal CA1 neurones. J Physiol. 1984 Nov;356:551–564. doi: 10.1113/jphysiol.1984.sp015482. [DOI] [PMC free article] [PubMed] [Google Scholar]
- Curtis D. R., Duggan A. W., Felix D., Johnston G. A. GABA, bicuculline and central inhibition. Nature. 1970 Jun 27;226(5252):1222–1224. doi: 10.1038/2261222a0. [DOI] [PubMed] [Google Scholar]
- DEL CASTILLO J., KATZ B. Quantal components of the end-plate potential. J Physiol. 1954 Jun 28;124(3):560–573. doi: 10.1113/jphysiol.1954.sp005129. [DOI] [PMC free article] [PubMed] [Google Scholar]
- Dennis M. J., Harris A. J., Kuffler S. W. Synaptic transmission and its duplication by focally applied acetylcholine in parasympathetic neurons in the heart of the frog. Proc R Soc Lond B Biol Sci. 1971 Apr 27;177(1049):509–539. doi: 10.1098/rspb.1971.0045. [DOI] [PubMed] [Google Scholar]
- Edwards F. A., Konnerth A., Sakmann B., Takahashi T. A thin slice preparation for patch clamp recordings from neurones of the mammalian central nervous system. Pflugers Arch. 1989 Sep;414(5):600–612. doi: 10.1007/BF00580998. [DOI] [PubMed] [Google Scholar]
- Edwards F. R., Redman S. J., Walmsley B. Statistical fluctuations in charge transfer at Ia synapses on spinal motoneurones. J Physiol. 1976 Aug;259(3):665–688. doi: 10.1113/jphysiol.1976.sp011488. [DOI] [PMC free article] [PubMed] [Google Scholar]
- Faber D. S., Korn H. Transmission at a central inhibitory synapse. I. Magnitude of unitary postsynaptic conductance change and kinetics of channel activation. J Neurophysiol. 1982 Sep;48(3):654–678. doi: 10.1152/jn.1982.48.3.654. [DOI] [PubMed] [Google Scholar]
- Finkel A. S., Redman S. J. The synaptic current evoked in cat spinal motoneurones by impulses in single group 1a axons. J Physiol. 1983 Sep;342:615–632. doi: 10.1113/jphysiol.1983.sp014872. [DOI] [PMC free article] [PubMed] [Google Scholar]
- Fischbach G. D., Schuetze S. M. A post-natal decrease in acetylcholine channel open time at rat end-plates. J Physiol. 1980 Jun;303:125–137. doi: 10.1113/jphysiol.1980.sp013275. [DOI] [PMC free article] [PubMed] [Google Scholar]
- Gold M. R., Martin A. R. Characteristics of inhibitory post-synaptic currents in brain-stem neurones of the lamprey. J Physiol. 1983 Sep;342:85–98. doi: 10.1113/jphysiol.1983.sp014841. [DOI] [PMC free article] [PubMed] [Google Scholar]
- Hamill O. P., Marty A., Neher E., Sakmann B., Sigworth F. J. Improved patch-clamp techniques for high-resolution current recording from cells and cell-free membrane patches. Pflugers Arch. 1981 Aug;391(2):85–100. doi: 10.1007/BF00656997. [DOI] [PubMed] [Google Scholar]
- Hartzell H. C., Kuffler S. W., Yoshikami D. Post-synaptic potentiation: interaction between quanta of acetylcholine at the skeletal neuromuscular synapse. J Physiol. 1975 Oct;251(2):427–463. doi: 10.1113/jphysiol.1975.sp011102. [DOI] [PMC free article] [PubMed] [Google Scholar]
- Henderson L. P., Brehm P. The single-channel basis for the slow kinetics of synaptic currents in vertebrate slow muscle fibers. Neuron. 1989 Apr;2(4):1399–1405. doi: 10.1016/0896-6273(89)90078-0. [DOI] [PubMed] [Google Scholar]
- Jack J. J., Redman S. J., Wong K. The components of synaptic potentials evoked in cat spinal motoneurones by impulses in single group Ia afferents. J Physiol. 1981 Dec;321:65–96. doi: 10.1113/jphysiol.1981.sp013972. [DOI] [PMC free article] [PubMed] [Google Scholar]
- Kojima H., Takahashi T. Characterization of miniature inhibitory post-synaptic potentials in rat spinal motoneurones. J Physiol. 1985 Nov;368:627–640. doi: 10.1113/jphysiol.1985.sp015880. [DOI] [PMC free article] [PubMed] [Google Scholar]
- Korn H., Burnod Y., Faber D. S. Spontaneous quantal currents in a central neuron match predictions from binomial analysis of evoked responses. Proc Natl Acad Sci U S A. 1987 Aug;84(16):5981–5985. doi: 10.1073/pnas.84.16.5981. [DOI] [PMC free article] [PubMed] [Google Scholar]
- Korn H., Mallet A., Triller A., Faber D. S. Transmission at a central inhibitory synapse. II. Quantal description of release, with a physical correlate for binomial n. J Neurophysiol. 1982 Sep;48(3):679–707. doi: 10.1152/jn.1982.48.3.679. [DOI] [PubMed] [Google Scholar]
- Kuno M., Weakly J. N. Quantal components of the inhibitory synaptic potential in spinal mononeurones of the cat. J Physiol. 1972 Jul;224(2):287–303. doi: 10.1113/jphysiol.1972.sp009895. [DOI] [PMC free article] [PubMed] [Google Scholar]
- LILEY A. W. The effects of presynaptic polarization on the spontaneous activity at the mammalian neuromuscular junction. J Physiol. 1956 Nov 28;134(2):427–443. doi: 10.1113/jphysiol.1956.sp005655. [DOI] [PMC free article] [PubMed] [Google Scholar]
- Land B. R., Salpeter E. E., Salpeter M. M. Acetylcholine receptor site density affects the rising phase of miniature endplate currents. Proc Natl Acad Sci U S A. 1980 Jun;77(6):3736–3740. doi: 10.1073/pnas.77.6.3736. [DOI] [PMC free article] [PubMed] [Google Scholar]
- Lübbers K., Frotscher M. Fine structure and synaptic connections of identified neurons in the rat fascia dentata. Anat Embryol (Berl) 1987;177(1):1–14. doi: 10.1007/BF00325285. [DOI] [PubMed] [Google Scholar]
- MARTIN A. R., PILAR G. QUANTAL COMPONENTS OF THE SYNAPTIC POTENTIAL IN THE CILIARY GANGLION OF THE CHICK. J Physiol. 1964 Dec;175:1–16. doi: 10.1113/jphysiol.1964.sp007499. [DOI] [PMC free article] [PubMed] [Google Scholar]
- Macdonald R. L., Rogers C. J., Twyman R. E. Kinetic properties of the GABAA receptor main conductance state of mouse spinal cord neurones in culture. J Physiol. 1989 Mar;410:479–499. doi: 10.1113/jphysiol.1989.sp017545. [DOI] [PMC free article] [PubMed] [Google Scholar]
- Magleby K. L., Miller D. C. Is the quantum of transmitter release composed of subunits? A critical analysis in the mouse and frog. J Physiol. 1981 Feb;311:267–287. doi: 10.1113/jphysiol.1981.sp013584. [DOI] [PMC free article] [PubMed] [Google Scholar]
- McLachlan E. M. An analysis of the release of acetylcholine from preganglionic nerve terminals. J Physiol. 1975 Feb;245(2):447–466. doi: 10.1113/jphysiol.1975.sp010855. [DOI] [PMC free article] [PubMed] [Google Scholar]
- Miles R., Wong R. K. Unitary inhibitory synaptic potentials in the guinea-pig hippocampus in vitro. J Physiol. 1984 Nov;356:97–113. doi: 10.1113/jphysiol.1984.sp015455. [DOI] [PMC free article] [PubMed] [Google Scholar]
- Rang H. P. The characteristics of synaptic currents and responses to acetylcholine of rat submandibular ganglion cells. J Physiol. 1981 Feb;311:23–55. doi: 10.1113/jphysiol.1981.sp013571. [DOI] [PMC free article] [PubMed] [Google Scholar]
- Redman S. Quantal analysis of synaptic potentials in neurons of the central nervous system. Physiol Rev. 1990 Jan;70(1):165–198. doi: 10.1152/physrev.1990.70.1.165. [DOI] [PubMed] [Google Scholar]
- Sakmann B., Brenner H. R. Change in synaptic channel gating during neuromuscular development. Nature. 1978 Nov 23;276(5686):401–402. doi: 10.1038/276401a0. [DOI] [PubMed] [Google Scholar]
- Sakmann B., Edwards F., Konnerth A., Takahashi T. Patch clamp techniques used for studying synaptic transmission in slices of mammalian brain. Q J Exp Physiol. 1989 Dec;74(7):1107–1118. doi: 10.1113/expphysiol.1989.sp003336. [DOI] [PubMed] [Google Scholar]
- Schofield P. R., Darlison M. G., Fujita N., Burt D. R., Stephenson F. A., Rodriguez H., Rhee L. M., Ramachandran J., Reale V., Glencorse T. A. Sequence and functional expression of the GABA A receptor shows a ligand-gated receptor super-family. Nature. 1987 Jul 16;328(6127):221–227. doi: 10.1038/328221a0. [DOI] [PubMed] [Google Scholar]
- Shivers B. D., Killisch I., Sprengel R., Sontheimer H., Köhler M., Schofield P. R., Seeburg P. H. Two novel GABAA receptor subunits exist in distinct neuronal subpopulations. Neuron. 1989 Sep;3(3):327–337. doi: 10.1016/0896-6273(89)90257-2. [DOI] [PubMed] [Google Scholar]
- Smith S. M., Zorec R., McBurney R. N. Conductance states activated by glycine and GABA in rat cultured spinal neurones. J Membr Biol. 1989 Apr;108(1):45–52. doi: 10.1007/BF01870424. [DOI] [PubMed] [Google Scholar]
- Séquier J. M., Richards J. G., Malherbe P., Price G. W., Mathews S., Möhler H. Mapping of brain areas containing RNA homologous to cDNAs encoding the alpha and beta subunits of the rat GABAA gamma-aminobutyrate receptor. Proc Natl Acad Sci U S A. 1988 Oct;85(20):7815–7819. doi: 10.1073/pnas.85.20.7815. [DOI] [PMC free article] [PubMed] [Google Scholar]
- TAKEUCHI A., TAKEUCHI N. Further analysis of relationship between end-plate potential and end-plate current. J Neurophysiol. 1960 Jul;23:397–402. doi: 10.1152/jn.1960.23.4.397. [DOI] [PubMed] [Google Scholar]
- Verdoorn T. A., Draguhn A., Ymer S., Seeburg P. H., Sakmann B. Functional properties of recombinant rat GABAA receptors depend upon subunit composition. Neuron. 1990 Jun;4(6):919–928. doi: 10.1016/0896-6273(90)90145-6. [DOI] [PubMed] [Google Scholar]