Abstract
1. Modulation of the hyperpolarization-activated cation current, Ih, by noradrenaline (NA) and serotonin (5-HT) was examined in guinea-pig and cat medial and lateral geniculate relay neurones using the in vitro slice technique. 2. In the absence of pharmacological antagonists, local application of NA resulted in a slow depolarization and decrease in apparent input conductance, a response which was blocked by local or bath application of the alpha 1-adrenoceptor antagonist prazosin. Application of NA after pharmacological block of alpha 1- and alpha 2-adrenoceptors, or application of 5-HT in all conditions, induced a 1-3 mV slow depolarization which was associated with a pronounced increase in apparent input conductance. This response to NA and 5-HT persisted during blocked synaptic transmission and was present in both the guinea-pig and cat medial and lateral geniculate nuclei. 3. The increase in membrane conductance elicited by NA was mimicked by the beta-specific agonist isoprenaline and blocked by the beta-antagonists propranolol and atenolol, indicating that it is mediated by beta-adrenoceptors. The response to 5-HT was blocked by the 5-HT1 and 5-HT2 antagonist methysergide, but not by the 5-HT2 antagonist ritanserin. Applications of either the 5-HT1A agonist ipsapirone or the partial agonist 8-hydroxy-dipropylaminotetralin (8-OHDPAT) were without effect. 4. Current versus voltage relationships obtained under voltage clamp revealed NA and 5-HT to cause a voltage-dependent inward shift at membrane potentials negative to approximately -60 mV. This response appeared to be shared by NA and 5-HT since maximal application of 5-HT greatly reduced or abolished the response to NA. 5. Application of NA and/or 5-HT during hyperpolarizing voltage steps in voltage clamp resulted in a marked increase in amplitude of the hyperpolarization-activated cation current, Ih. In addition, the rate of activation of Ih was strongly increased during activation of beta-adrenoceptors. 6. The activation curve of the conductance underlying Ih (Gh) was shifted by 4-6 mV on the voltage axis with NA and/or 5-HT. The positive shift of Gh activation in the voltage domain resulted in an increase in the amplitude of Gh which is active at resting, and more hyperpolarized, membrane potentials. The subsequent increase in resting membrane conductance decreased the responsiveness of thalamic neurones to hyperpolarizations of all durations. 7. Local or bath application of caesium blocked both Ih and the increase in membrane conductance in response to NA and 5-HT. By contrast, barium blocked neither Ih nor the responses to NA and 5-HT.(ABSTRACT TRUNCATED AT 400 WORDS)
Full text
PDF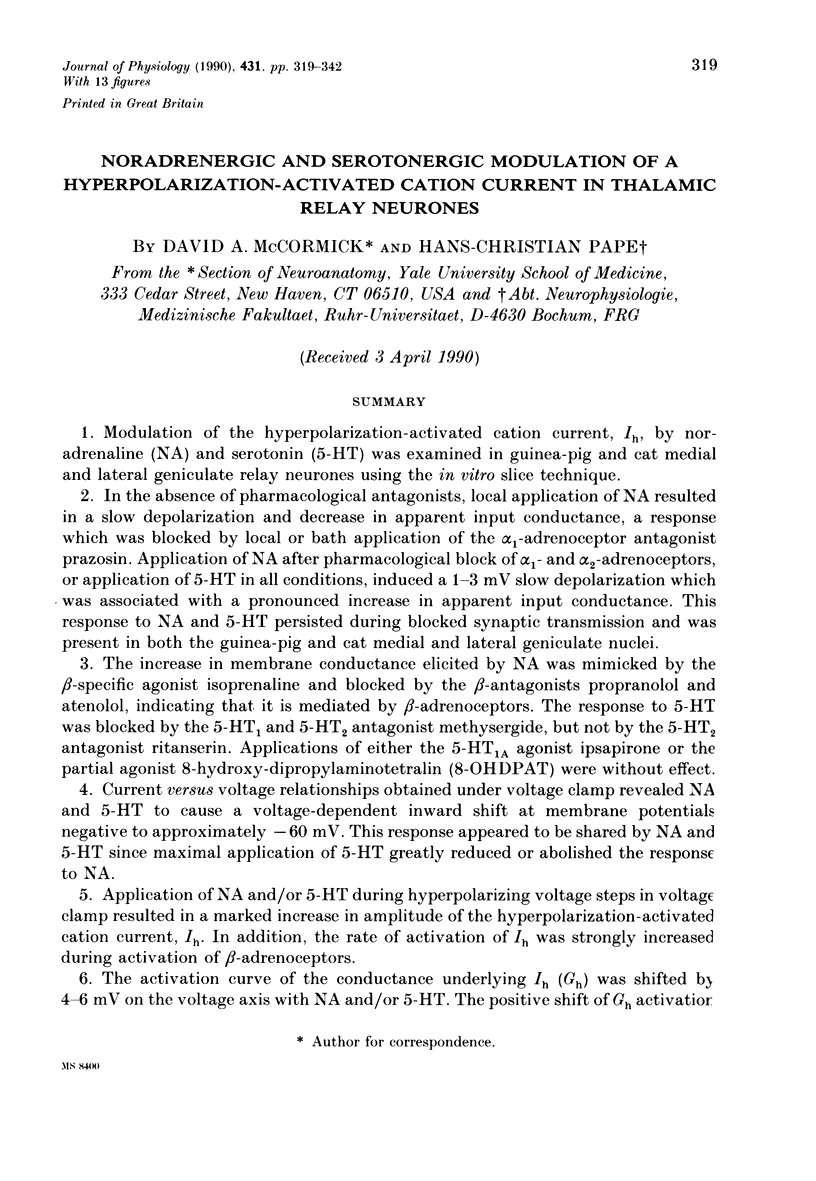
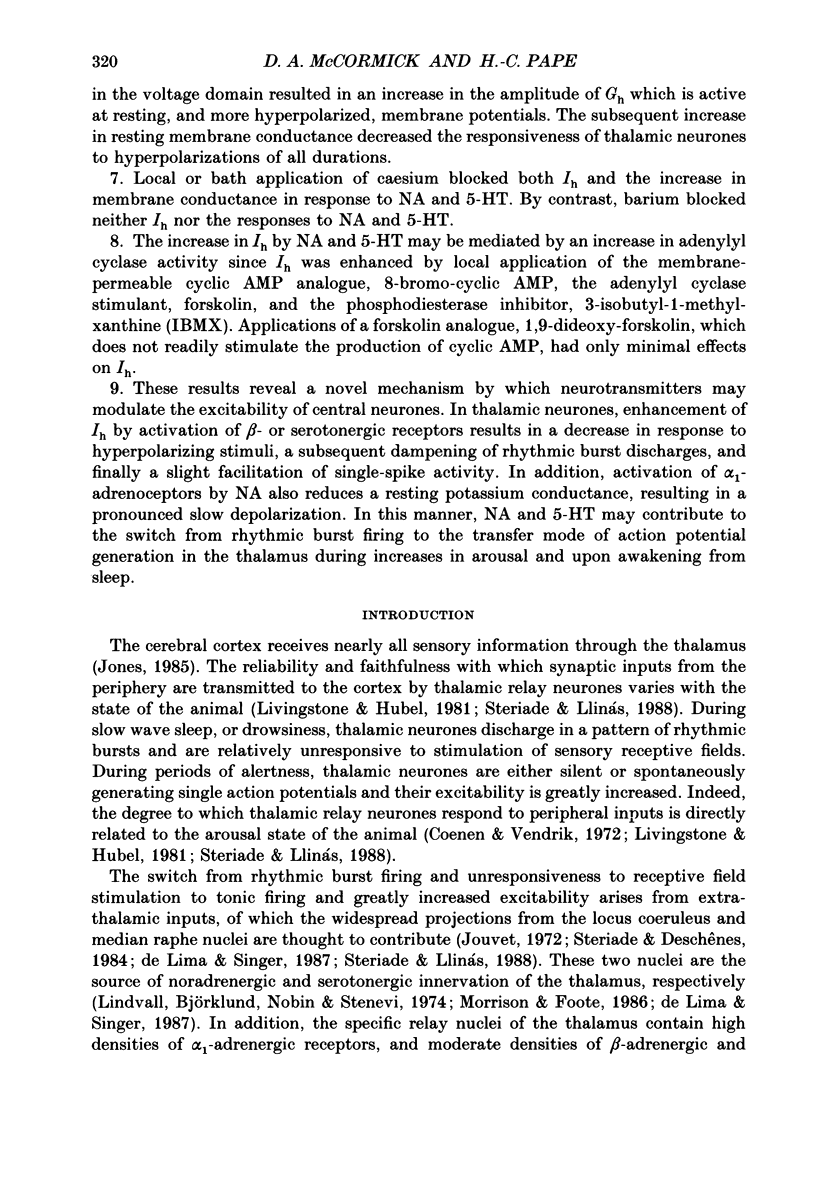
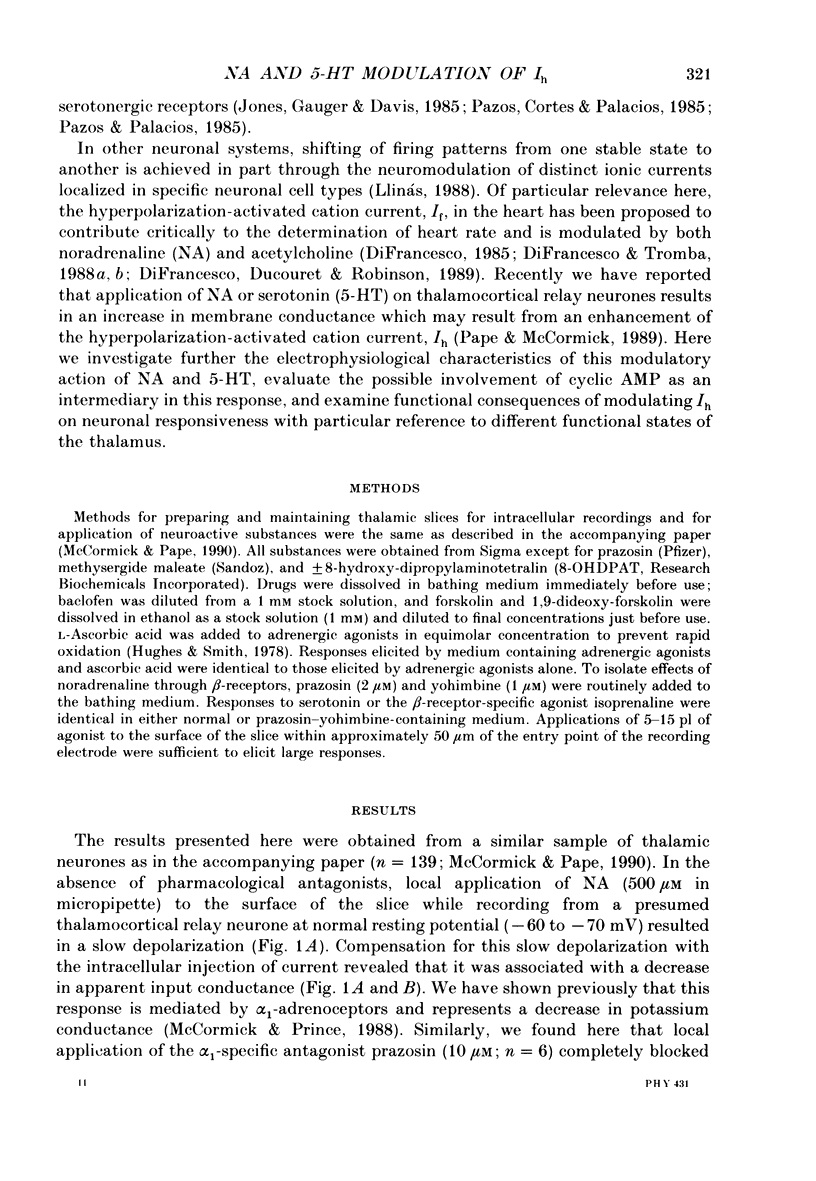
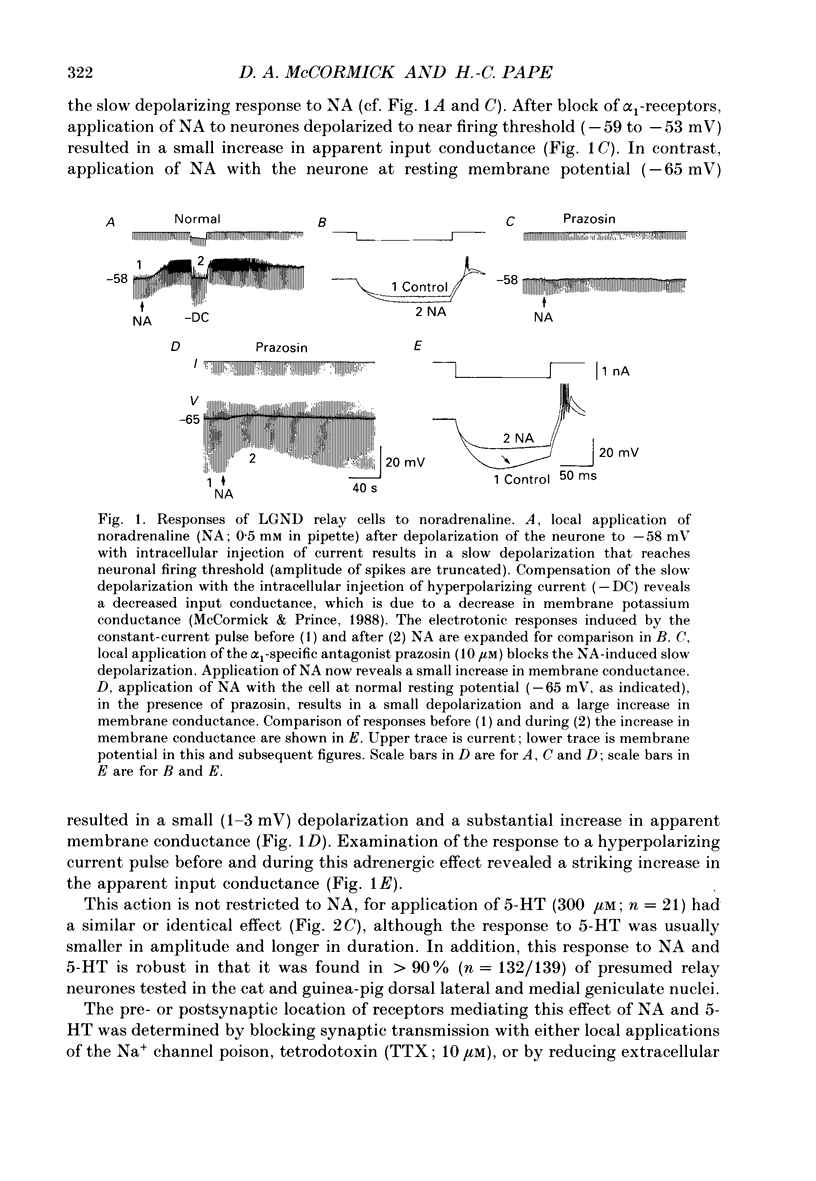
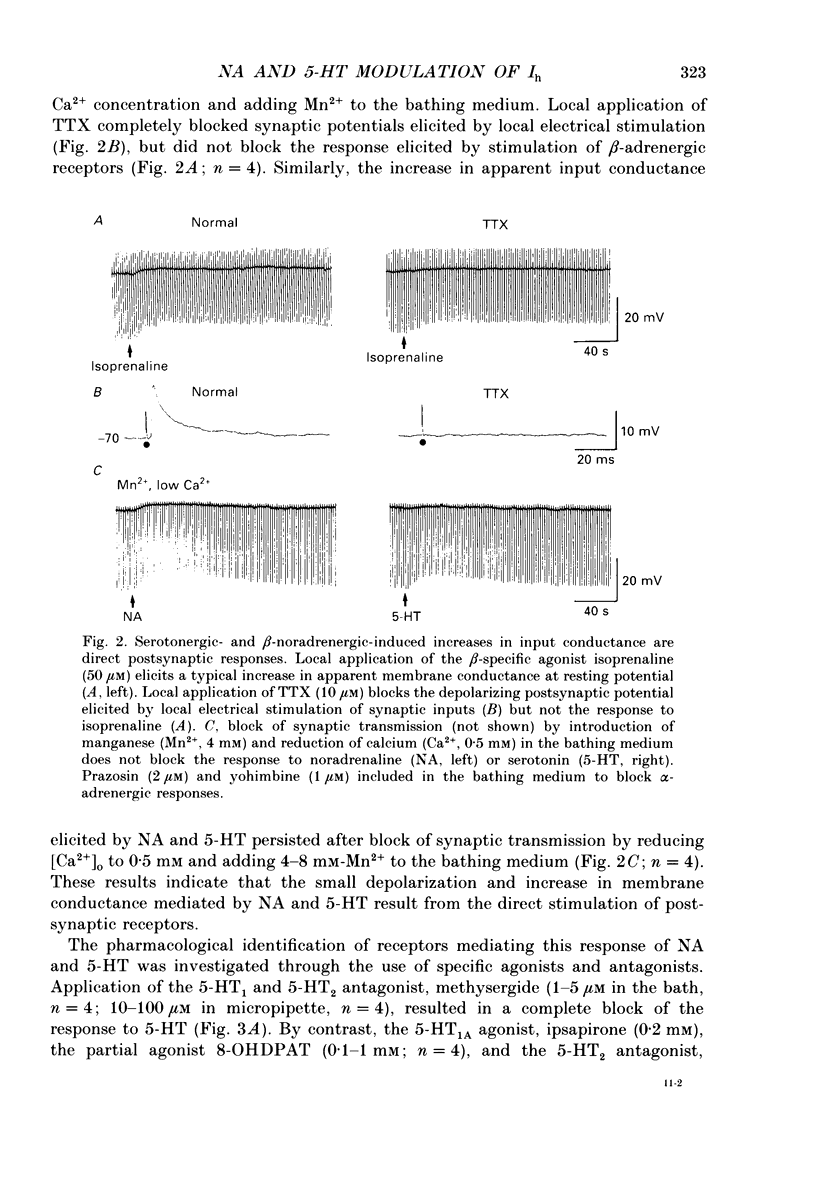
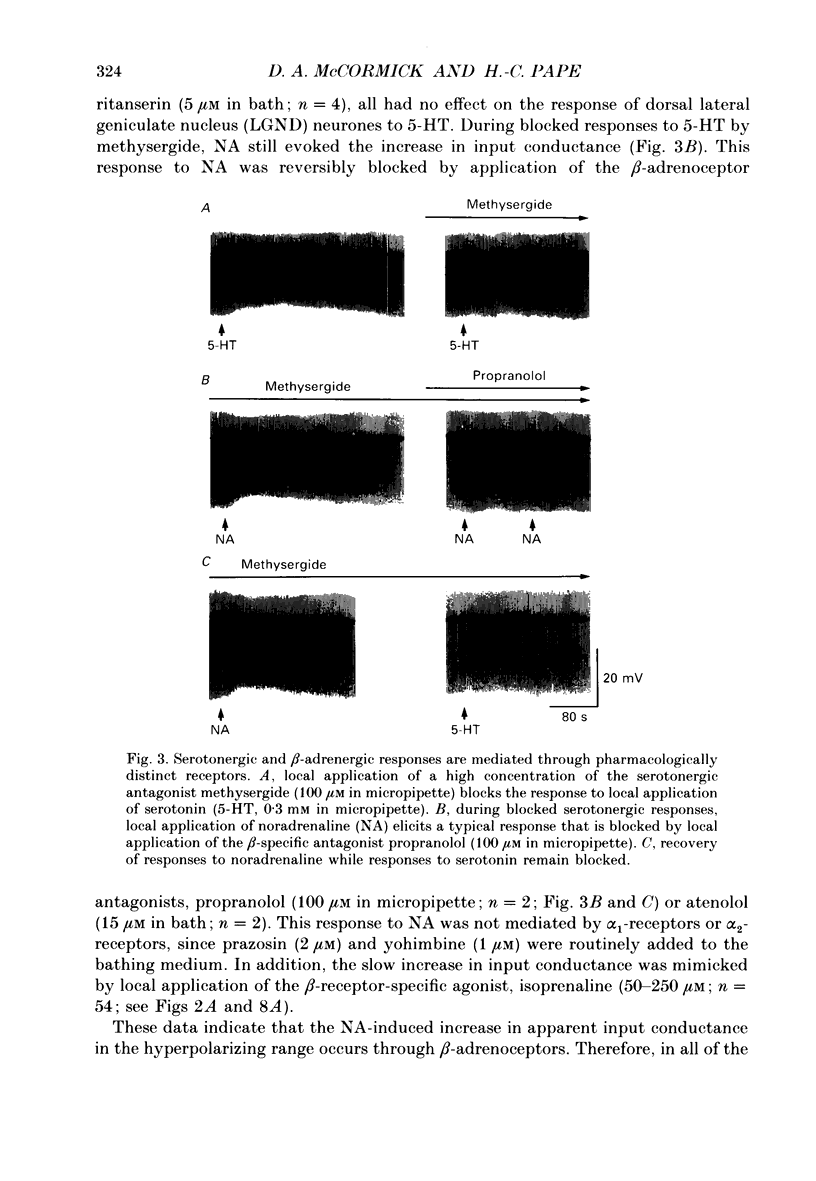
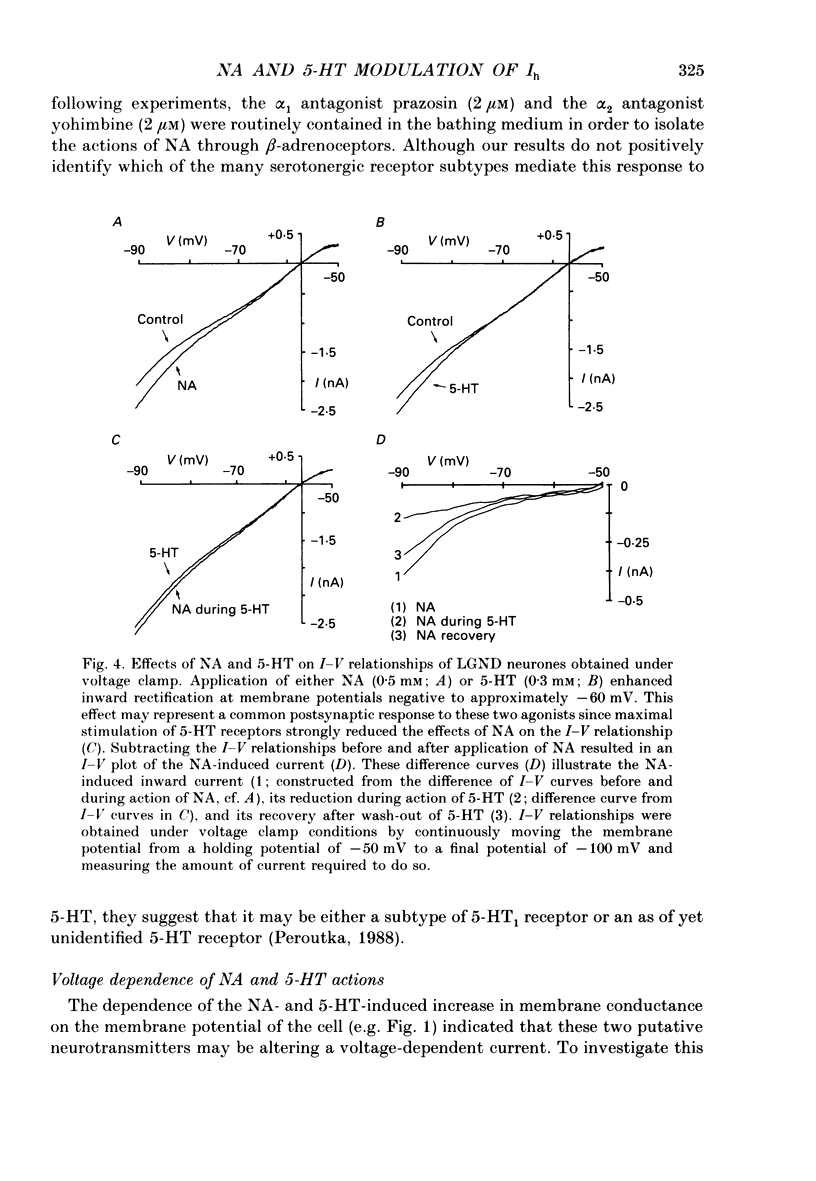
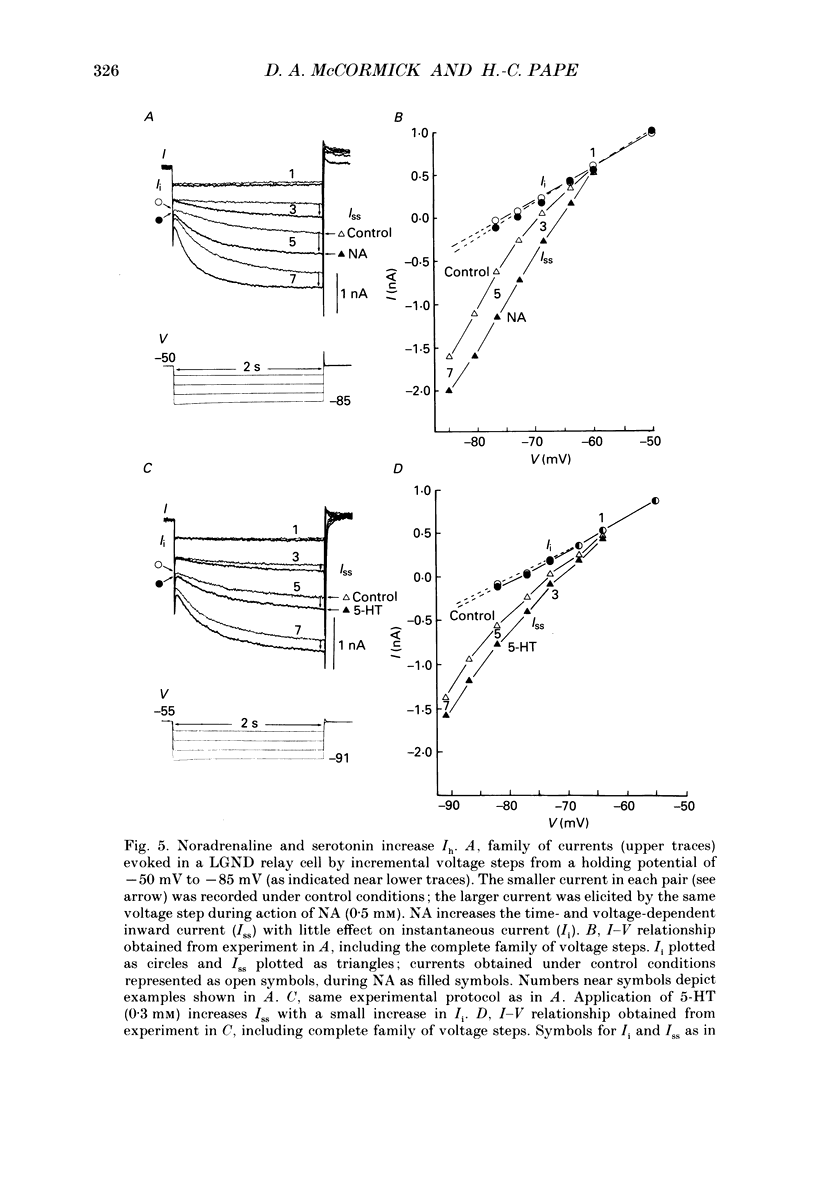
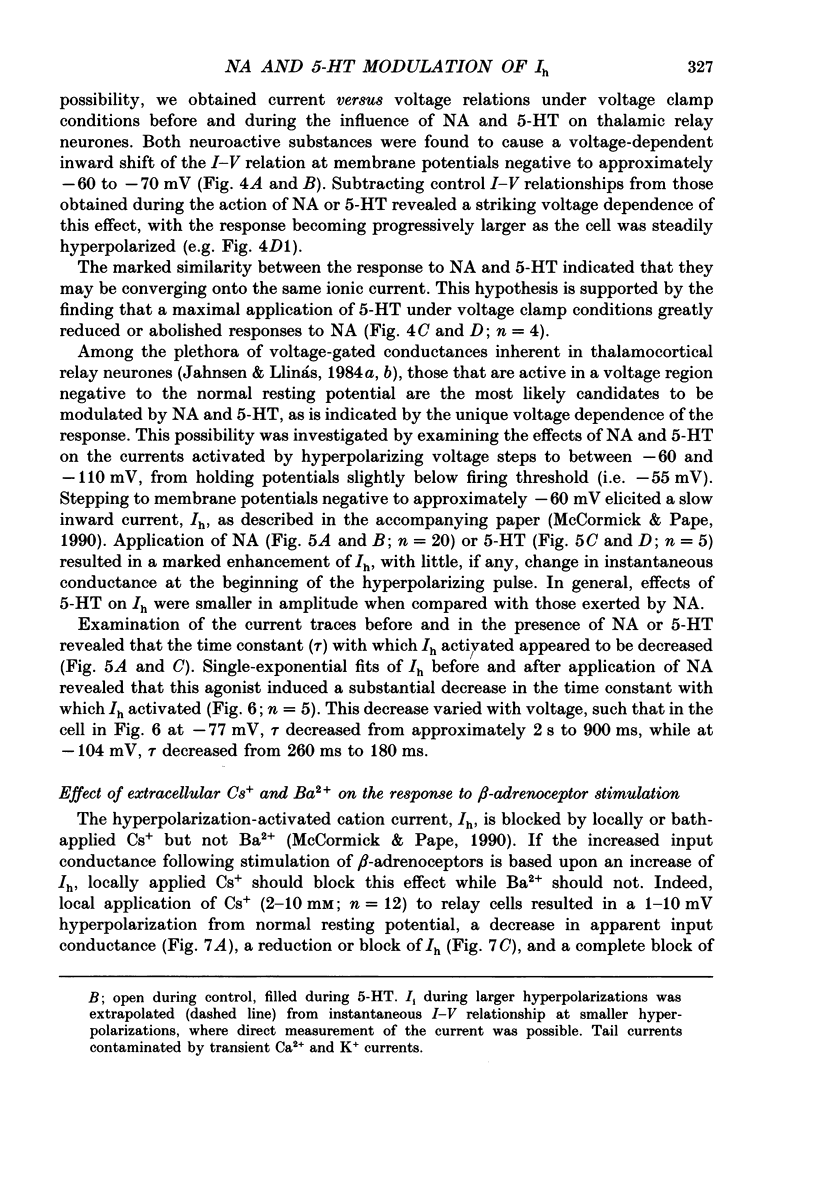
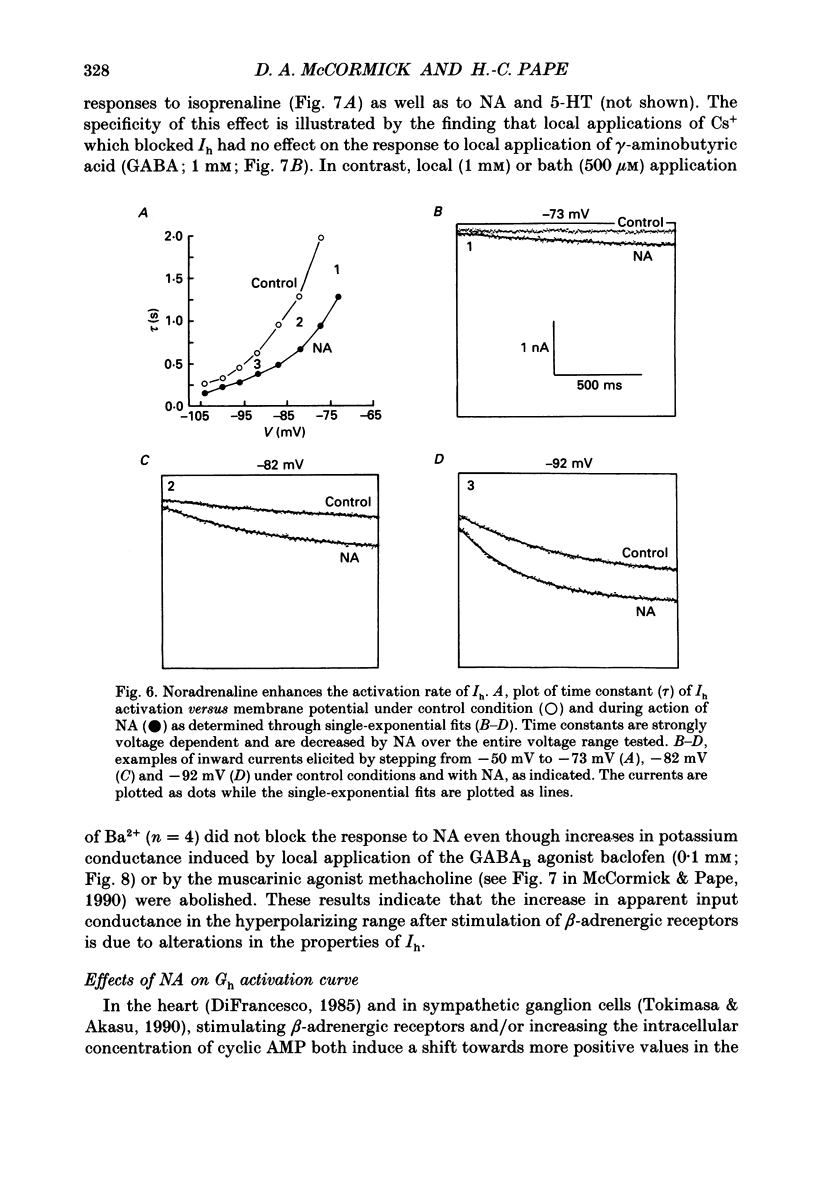
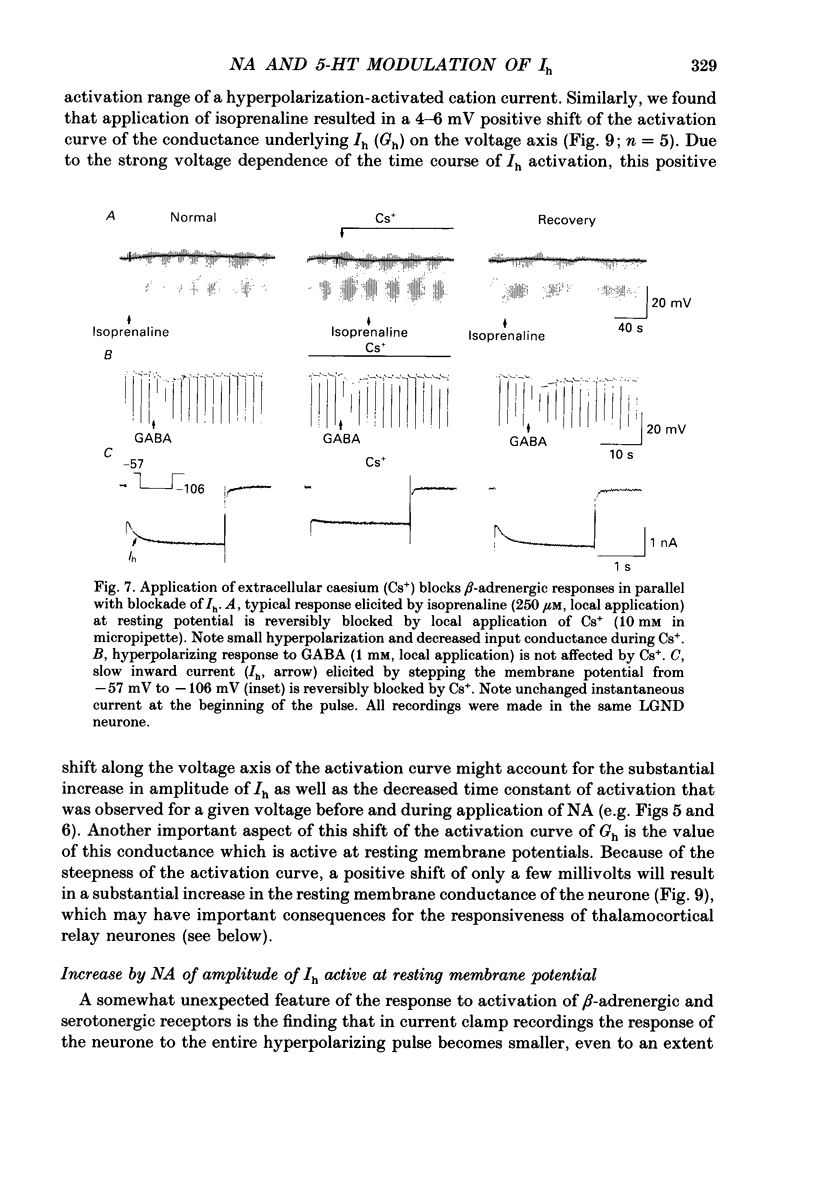
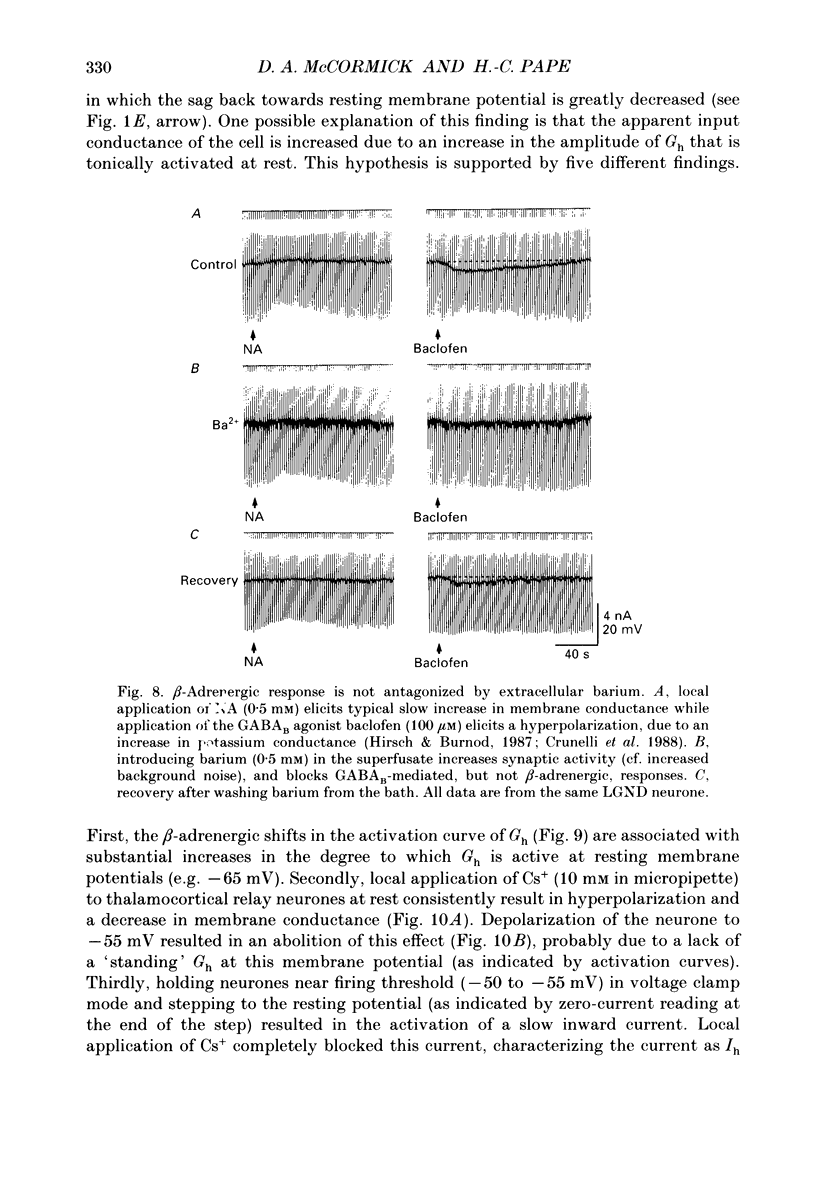
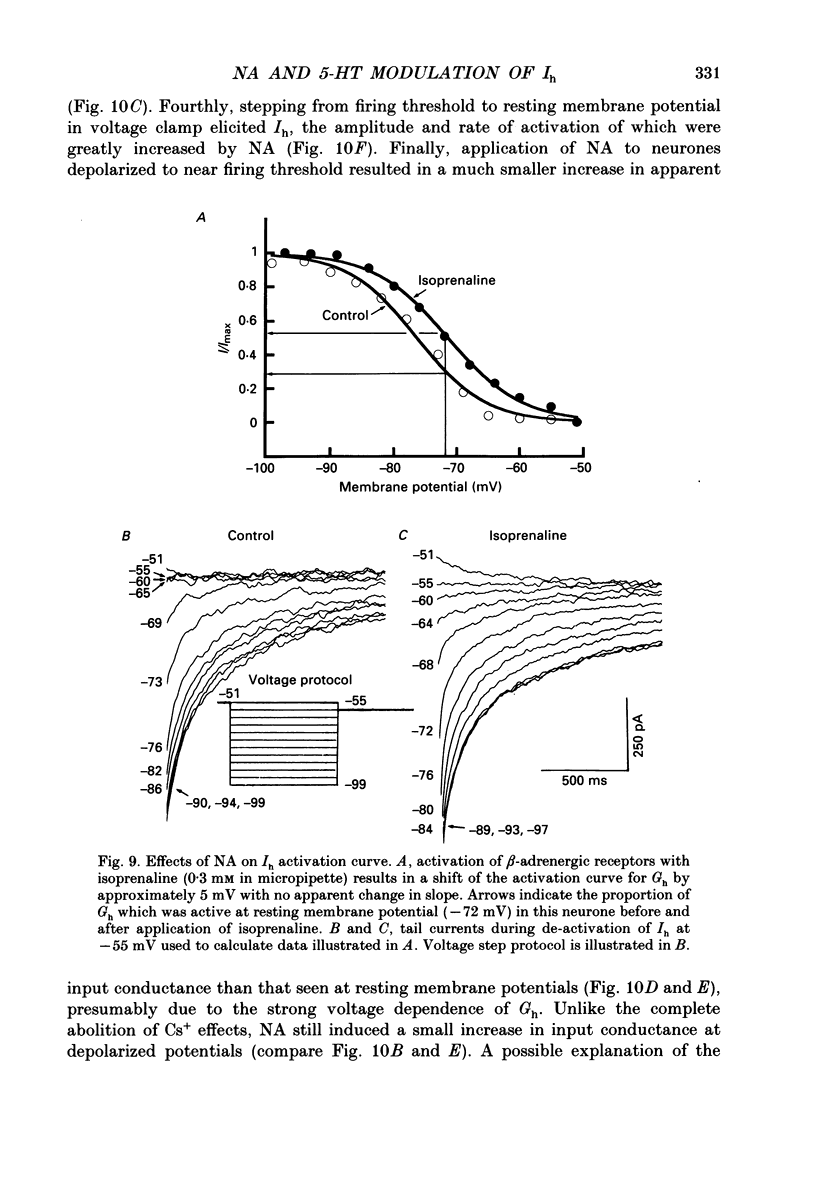
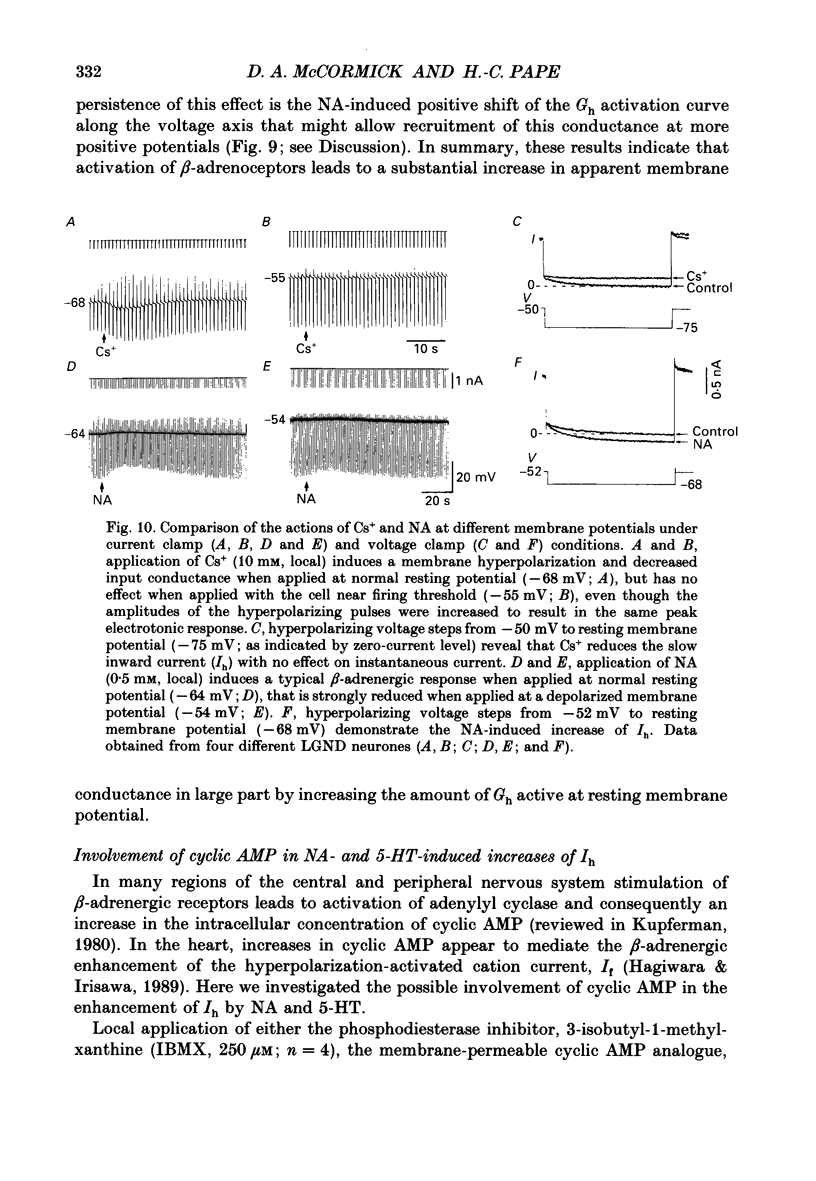
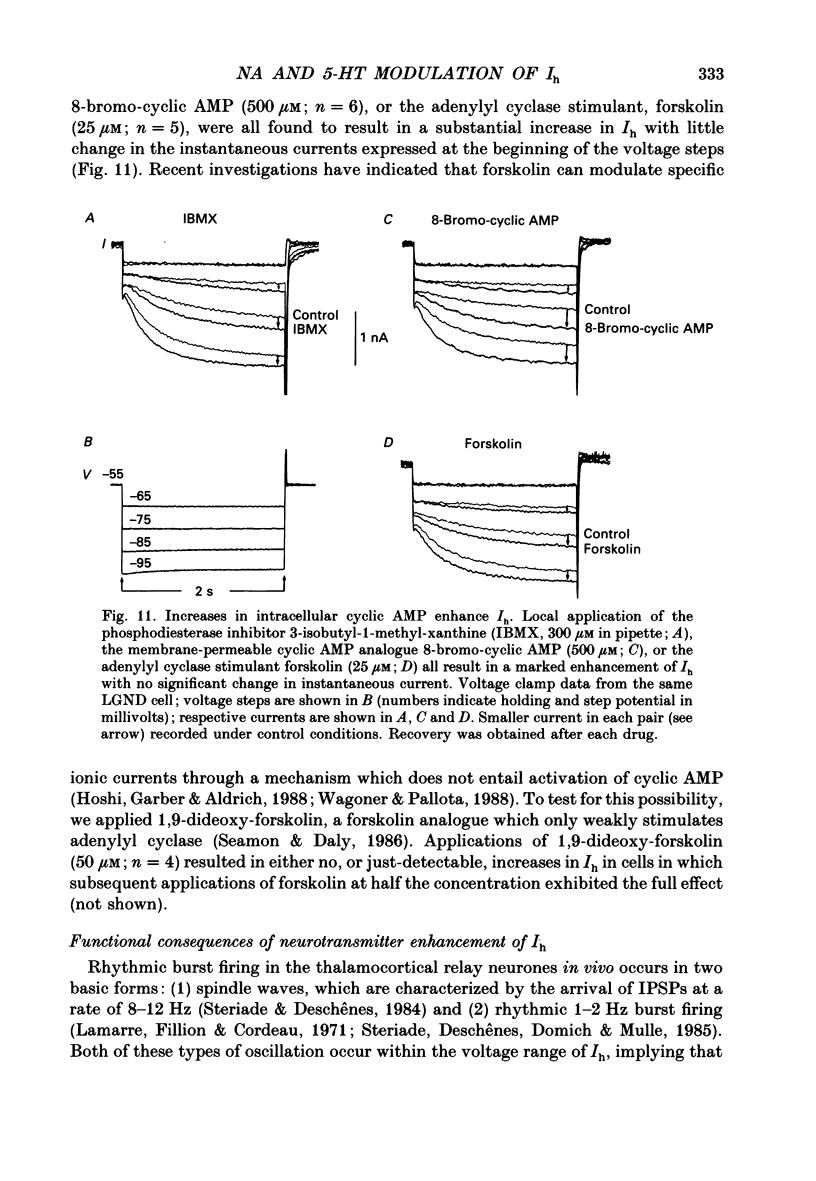
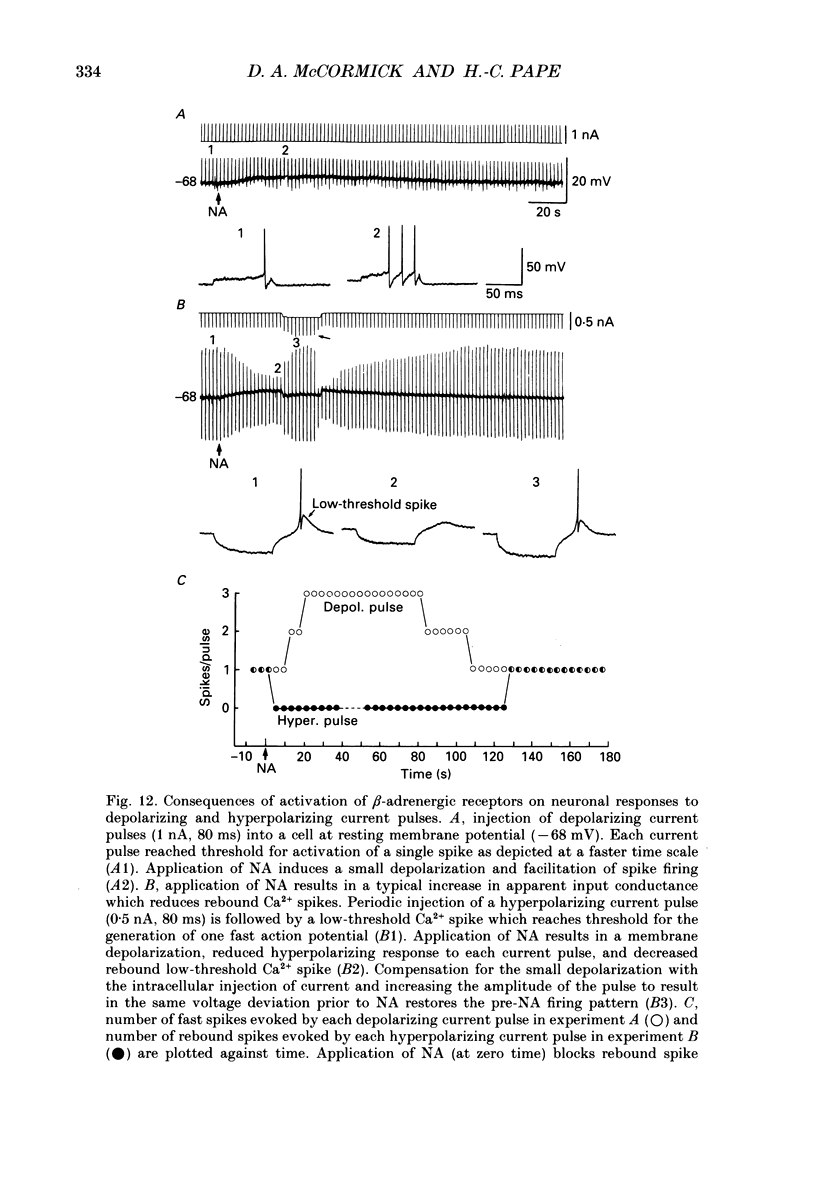
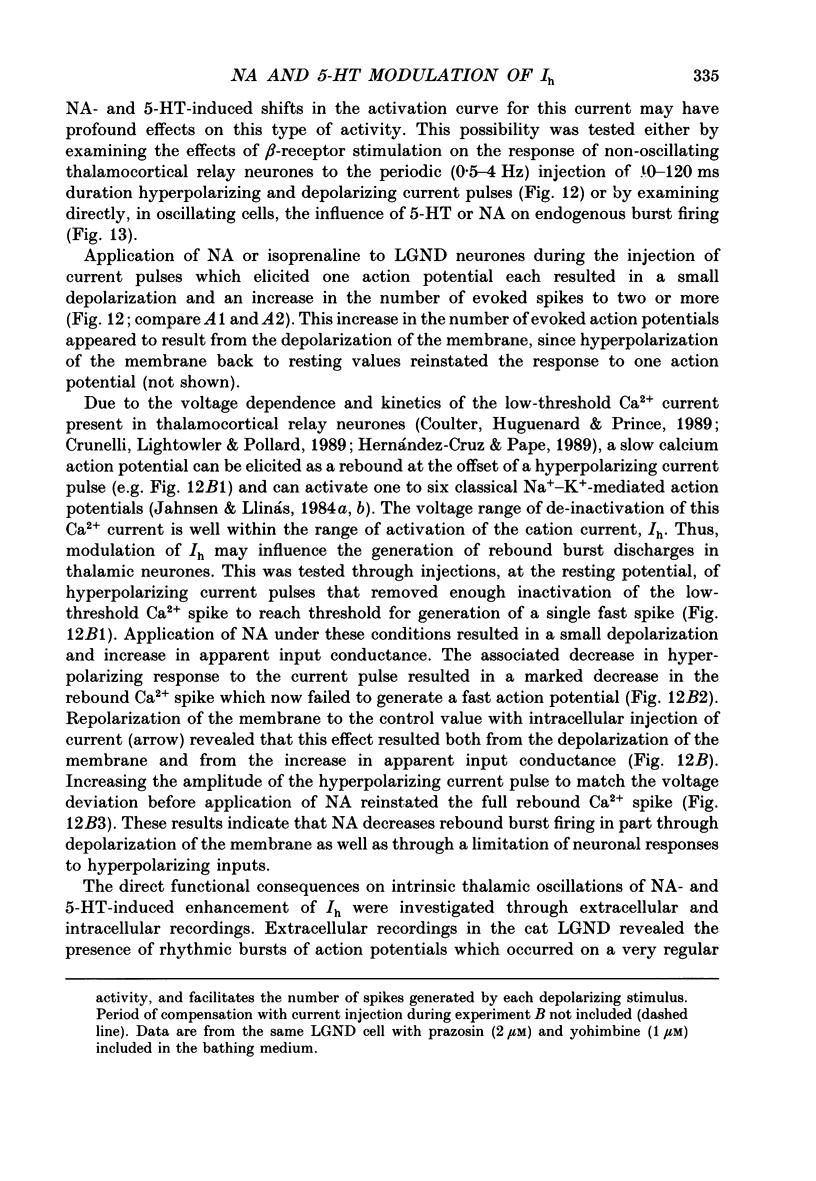
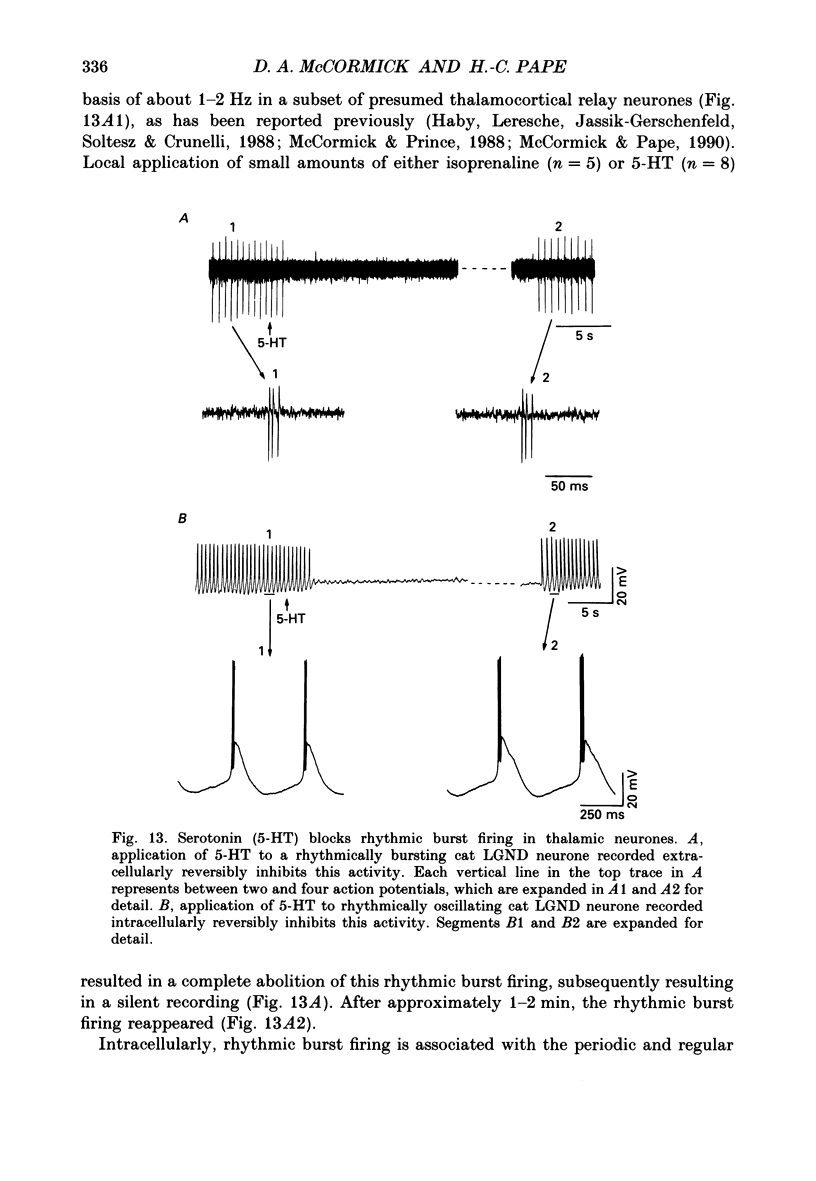
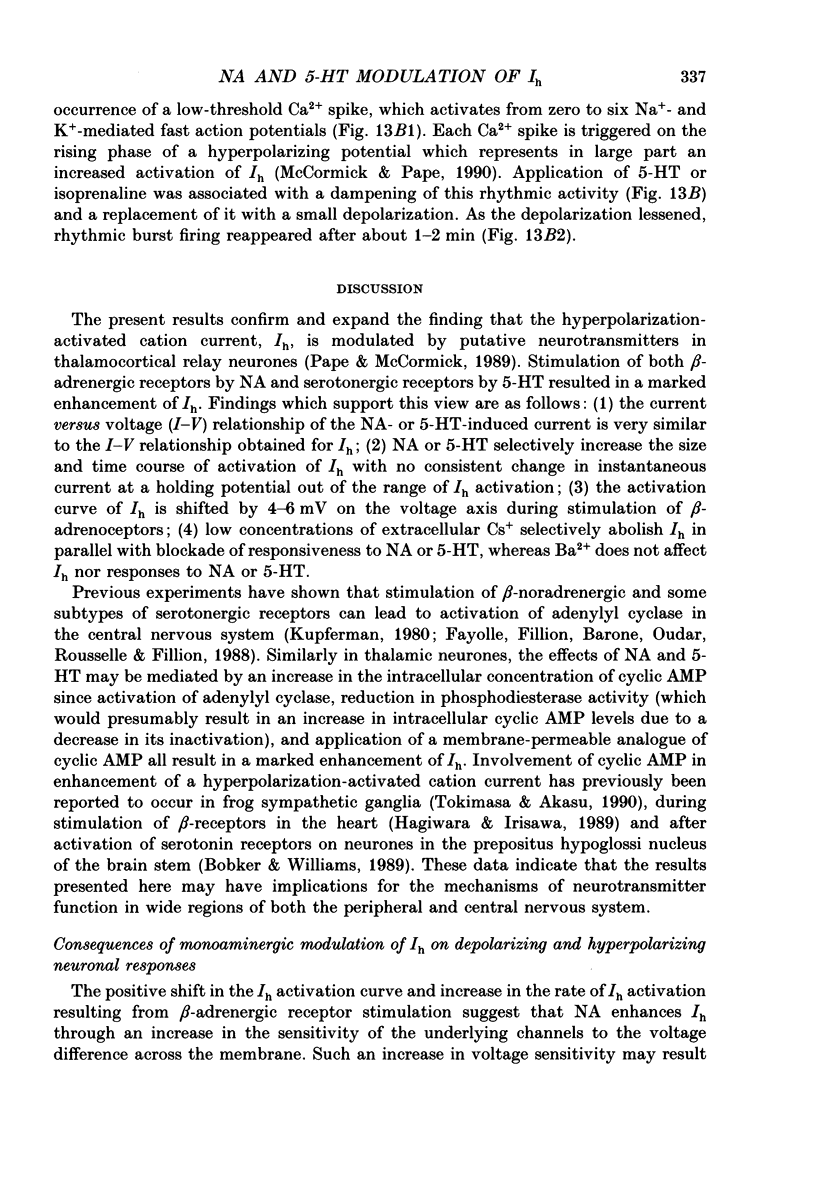
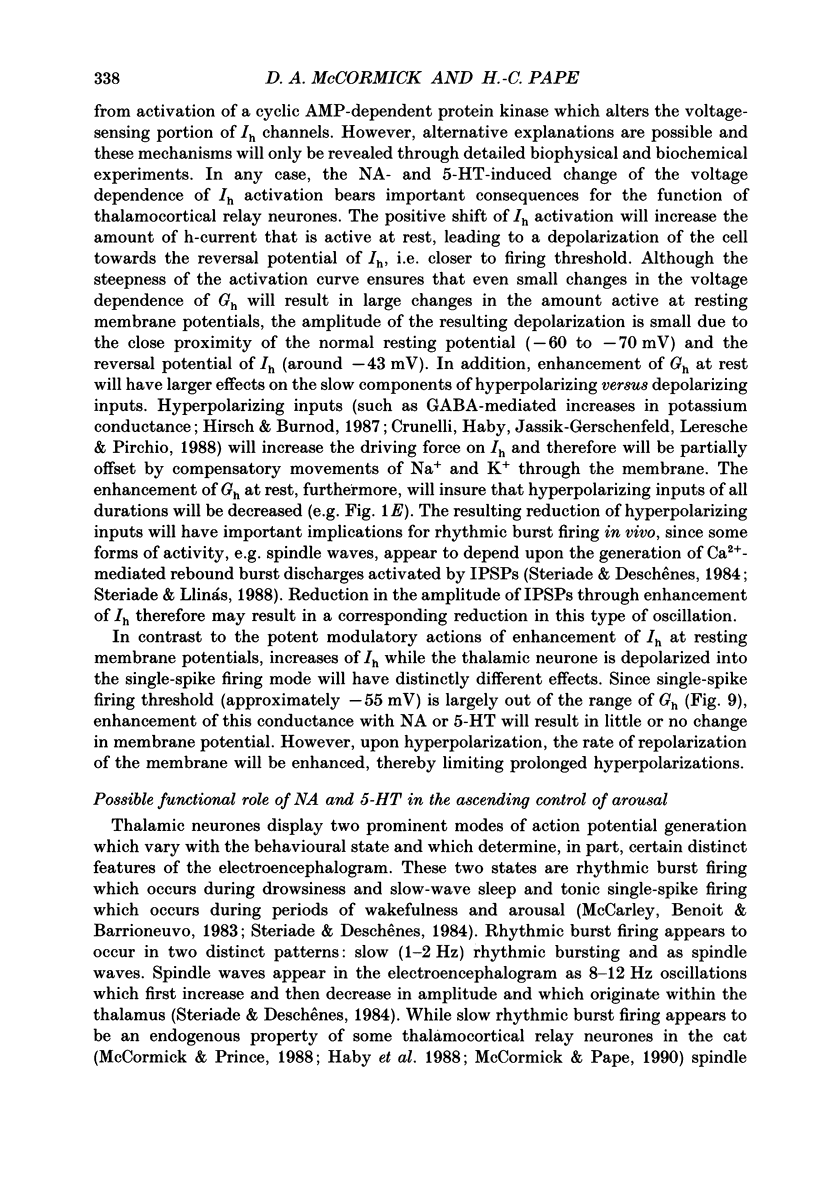
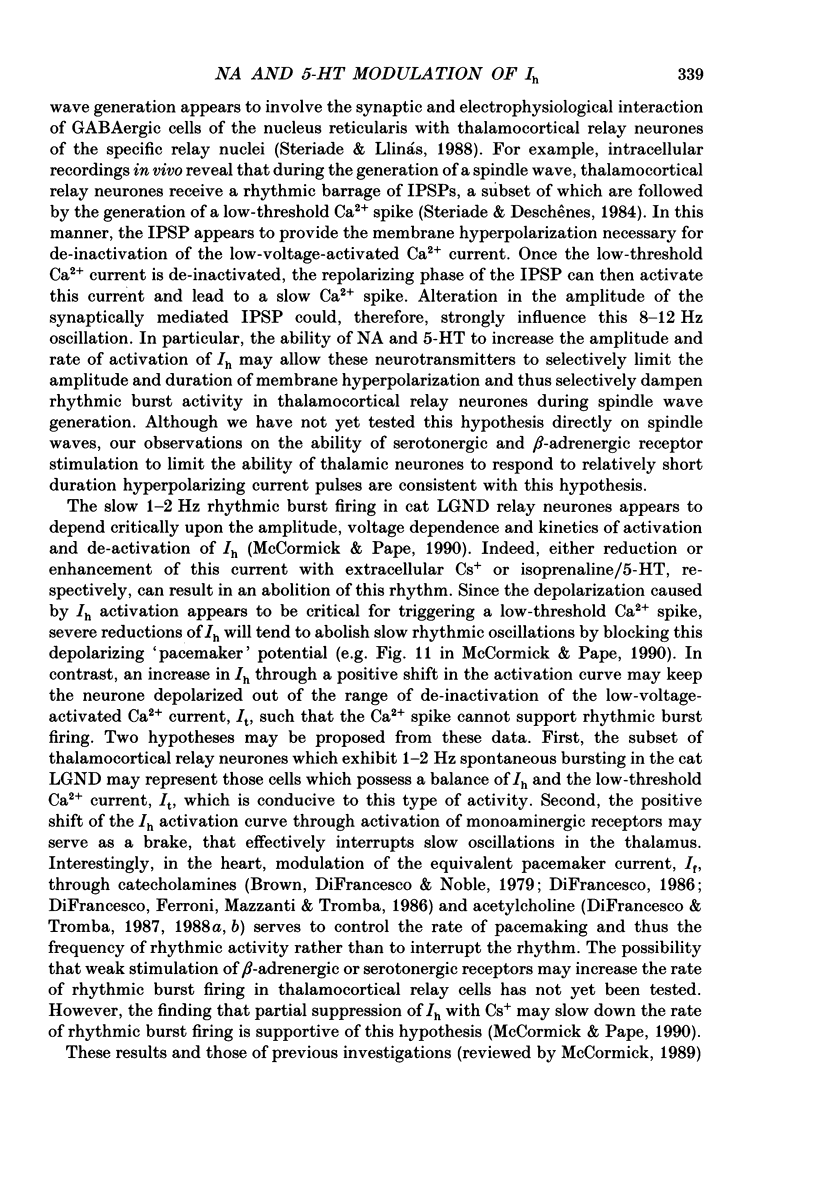
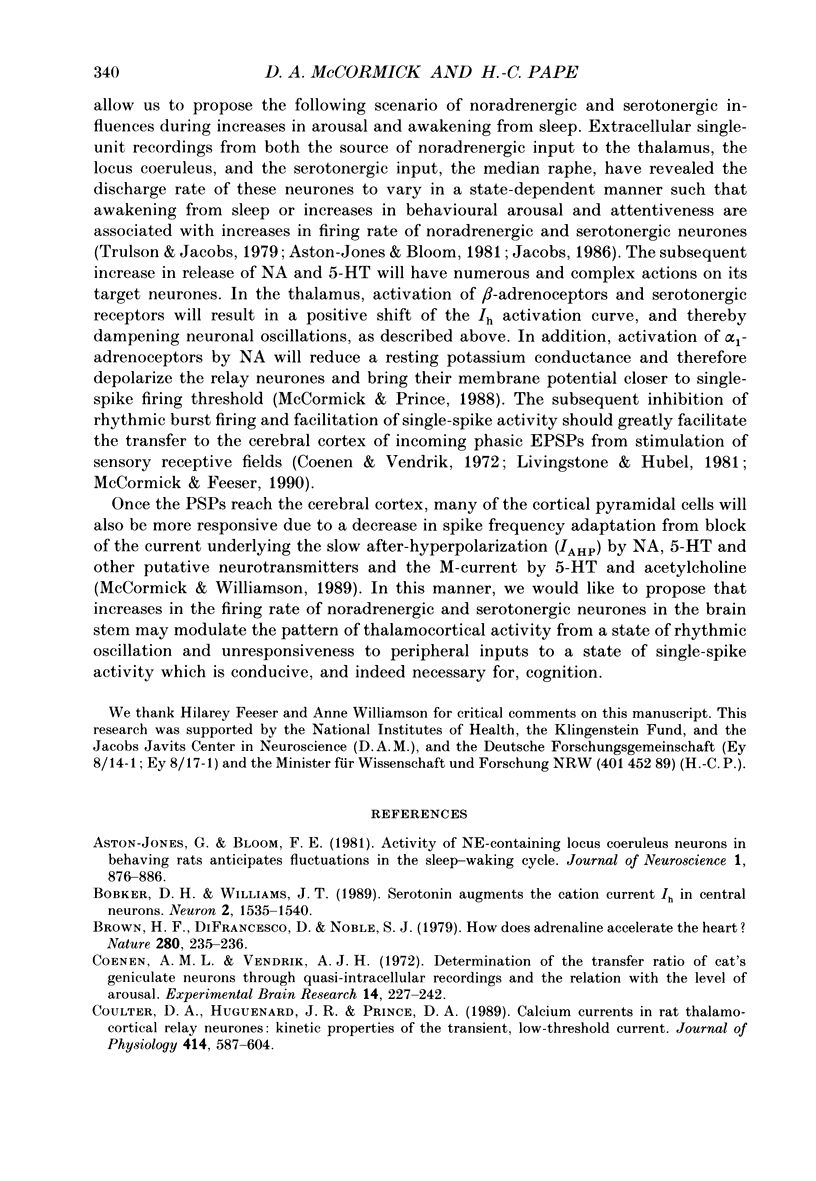
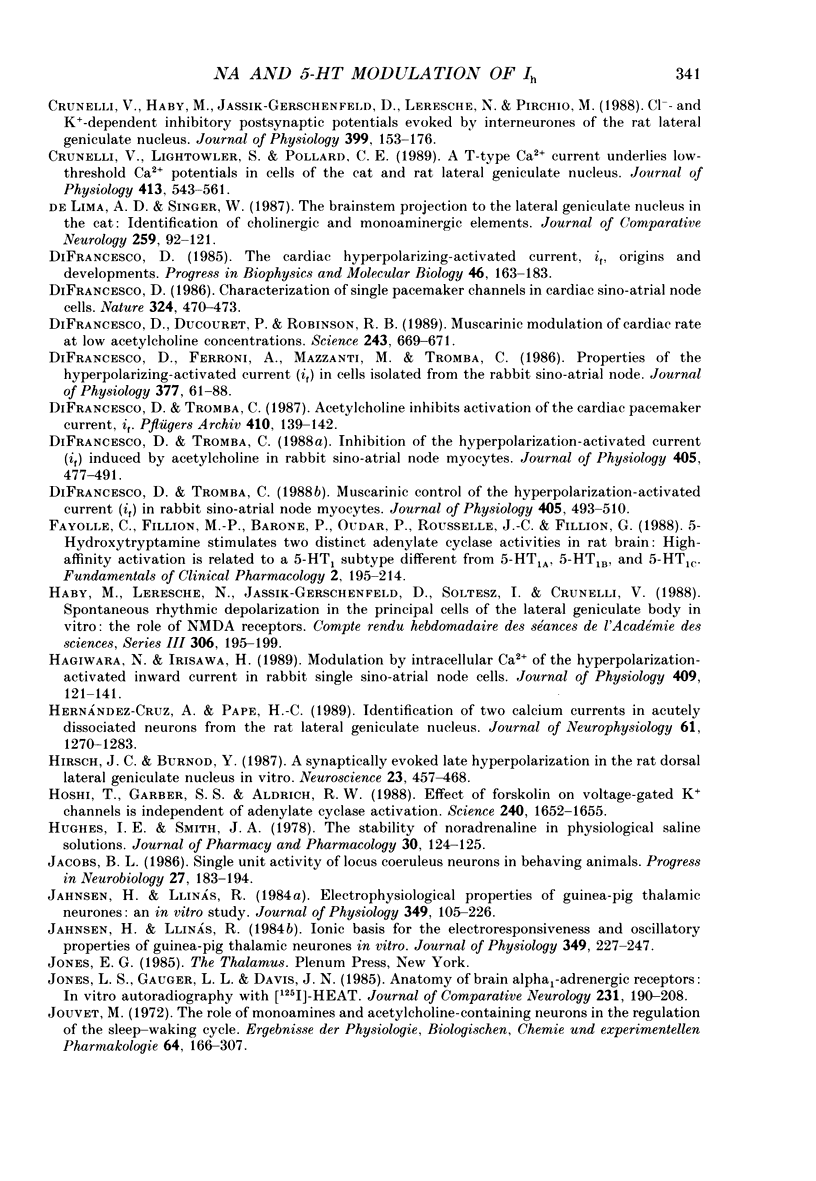
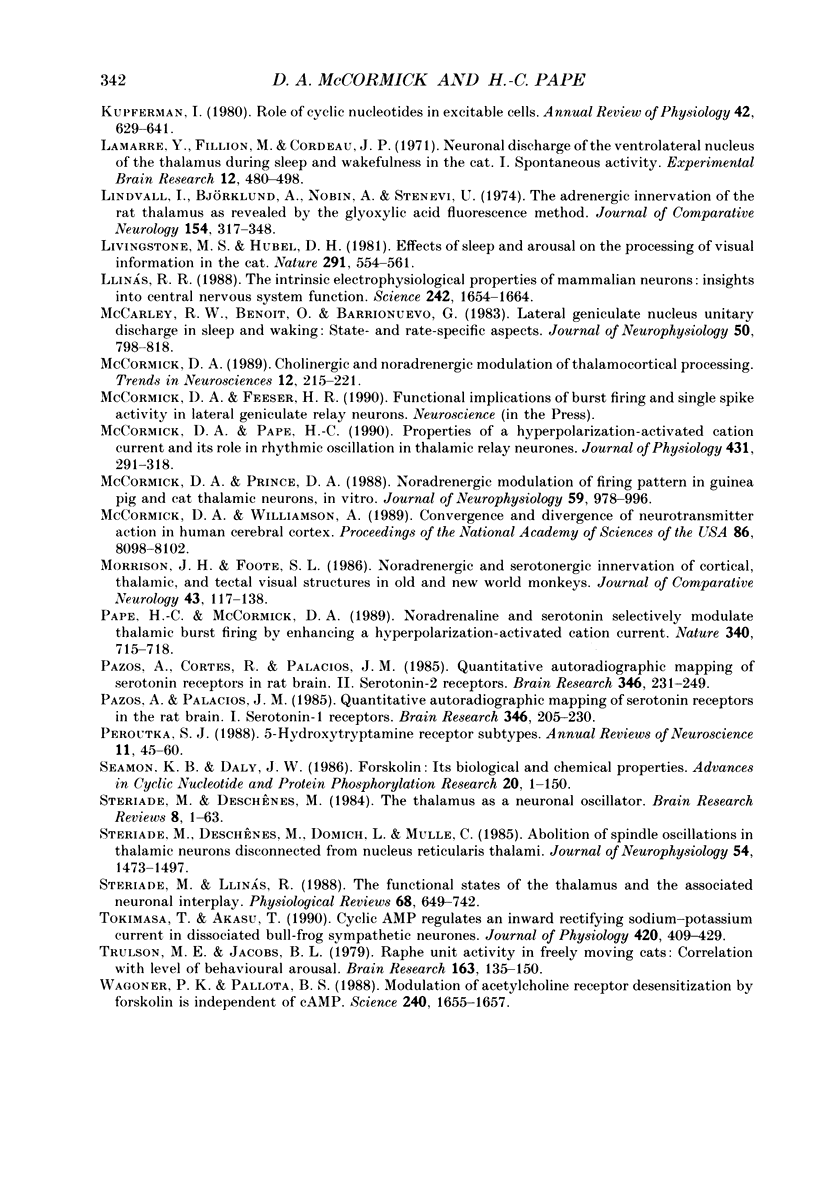
Images in this article
Selected References
These references are in PubMed. This may not be the complete list of references from this article.
- Aston-Jones G., Bloom F. E. Activity of norepinephrine-containing locus coeruleus neurons in behaving rats anticipates fluctuations in the sleep-waking cycle. J Neurosci. 1981 Aug;1(8):876–886. doi: 10.1523/JNEUROSCI.01-08-00876.1981. [DOI] [PMC free article] [PubMed] [Google Scholar]
- Bobker D. H., Williams J. T. Serotonin augments the cationic current Ih in central neurons. Neuron. 1989 Jun;2(6):1535–1540. doi: 10.1016/0896-6273(89)90041-x. [DOI] [PubMed] [Google Scholar]
- Coenen A. M., Vendrik A. J. Determination of the transfer ratio of cat's geniculate neurons through quasi-intracellular recordings and the relation with the level of alertness. Exp Brain Res. 1972;14(3):227–242. doi: 10.1007/BF00816160. [DOI] [PubMed] [Google Scholar]
- Coulter D. A., Huguenard J. R., Prince D. A. Calcium currents in rat thalamocortical relay neurones: kinetic properties of the transient, low-threshold current. J Physiol. 1989 Jul;414:587–604. doi: 10.1113/jphysiol.1989.sp017705. [DOI] [PMC free article] [PubMed] [Google Scholar]
- Crunelli V., Haby M., Jassik-Gerschenfeld D., Leresche N., Pirchio M. Cl- - and K+-dependent inhibitory postsynaptic potentials evoked by interneurones of the rat lateral geniculate nucleus. J Physiol. 1988 May;399:153–176. doi: 10.1113/jphysiol.1988.sp017073. [DOI] [PMC free article] [PubMed] [Google Scholar]
- Crunelli V., Lightowler S., Pollard C. E. A T-type Ca2+ current underlies low-threshold Ca2+ potentials in cells of the cat and rat lateral geniculate nucleus. J Physiol. 1989 Jun;413:543–561. doi: 10.1113/jphysiol.1989.sp017668. [DOI] [PMC free article] [PubMed] [Google Scholar]
- De Lima A. D., Singer W. The brainstem projection to the lateral geniculate nucleus in the cat: identification of cholinergic and monoaminergic elements. J Comp Neurol. 1987 May 1;259(1):92–121. doi: 10.1002/cne.902590107. [DOI] [PubMed] [Google Scholar]
- DiFrancesco D. Characterization of single pacemaker channels in cardiac sino-atrial node cells. Nature. 1986 Dec 4;324(6096):470–473. doi: 10.1038/324470a0. [DOI] [PubMed] [Google Scholar]
- DiFrancesco D., Ducouret P., Robinson R. B. Muscarinic modulation of cardiac rate at low acetylcholine concentrations. Science. 1989 Feb 3;243(4891):669–671. doi: 10.1126/science.2916119. [DOI] [PubMed] [Google Scholar]
- DiFrancesco D., Ferroni A., Mazzanti M., Tromba C. Properties of the hyperpolarizing-activated current (if) in cells isolated from the rabbit sino-atrial node. J Physiol. 1986 Aug;377:61–88. doi: 10.1113/jphysiol.1986.sp016177. [DOI] [PMC free article] [PubMed] [Google Scholar]
- DiFrancesco D. The cardiac hyperpolarizing-activated current, if. Origins and developments. Prog Biophys Mol Biol. 1985;46(3):163–183. doi: 10.1016/0079-6107(85)90008-2. [DOI] [PubMed] [Google Scholar]
- DiFrancesco D., Tromba C. Acetylcholine inhibits activation of the cardiac hyperpolarizing-activated current, if. Pflugers Arch. 1987 Sep;410(1-2):139–142. doi: 10.1007/BF00581906. [DOI] [PubMed] [Google Scholar]
- DiFrancesco D., Tromba C. Inhibition of the hyperpolarization-activated current (if) induced by acetylcholine in rabbit sino-atrial node myocytes. J Physiol. 1988 Nov;405:477–491. doi: 10.1113/jphysiol.1988.sp017343. [DOI] [PMC free article] [PubMed] [Google Scholar]
- DiFrancesco D., Tromba C. Muscarinic control of the hyperpolarization-activated current (if) in rabbit sino-atrial node myocytes. J Physiol. 1988 Nov;405:493–510. doi: 10.1113/jphysiol.1988.sp017344. [DOI] [PMC free article] [PubMed] [Google Scholar]
- Fayolle C., Fillion M. P., Barone P., Oudar P., Rousselle J. C., Fillion G. 5-Hydroxytryptamine stimulates two distinct adenylate cyclase activities in rat brain: high-affinity activation is related to a 5-HT1 subtype different from 5-HT1A, 5-HT1B, and 5-HT1C. Fundam Clin Pharmacol. 1988;2(3):195–214. doi: 10.1111/j.1472-8206.1988.tb00632.x. [DOI] [PubMed] [Google Scholar]
- Hagiwara N., Irisawa H. Modulation by intracellular Ca2+ of the hyperpolarization-activated inward current in rabbit single sino-atrial node cells. J Physiol. 1989 Feb;409:121–141. doi: 10.1113/jphysiol.1989.sp017488. [DOI] [PMC free article] [PubMed] [Google Scholar]
- Hernández-Cruz A., Pape H. C. Identification of two calcium currents in acutely dissociated neurons from the rat lateral geniculate nucleus. J Neurophysiol. 1989 Jun;61(6):1270–1283. doi: 10.1152/jn.1989.61.6.1270. [DOI] [PubMed] [Google Scholar]
- Hirsch J. C., Burnod Y. A synaptically evoked late hyperpolarization in the rat dorsolateral geniculate neurons in vitro. Neuroscience. 1987 Nov;23(2):457–468. doi: 10.1016/0306-4522(87)90069-8. [DOI] [PubMed] [Google Scholar]
- Hoshi T., Garber S. S., Aldrich R. W. Effect of forskolin on voltage-gated K+ channels is independent of adenylate cyclase activation. Science. 1988 Jun 17;240(4859):1652–1655. doi: 10.1126/science.2454506. [DOI] [PubMed] [Google Scholar]
- Hughes I. E., Smith J. A. The stability of noradrenaline in physiological saline solutions. J Pharm Pharmacol. 1978 Feb;30(2):124–126. doi: 10.1111/j.2042-7158.1978.tb13179.x. [DOI] [PubMed] [Google Scholar]
- Jacobs B. L. Single unit activity of locus coeruleus neurons in behaving animals. Prog Neurobiol. 1986;27(2):183–194. doi: 10.1016/0301-0082(86)90008-0. [DOI] [PubMed] [Google Scholar]
- Jahnsen H., Llinás R. Electrophysiological properties of guinea-pig thalamic neurones: an in vitro study. J Physiol. 1984 Apr;349:205–226. doi: 10.1113/jphysiol.1984.sp015153. [DOI] [PMC free article] [PubMed] [Google Scholar]
- Jahnsen H., Llinás R. Ionic basis for the electro-responsiveness and oscillatory properties of guinea-pig thalamic neurones in vitro. J Physiol. 1984 Apr;349:227–247. doi: 10.1113/jphysiol.1984.sp015154. [DOI] [PMC free article] [PubMed] [Google Scholar]
- Jones L. S., Gauger L. L., Davis J. N. Anatomy of brain alpha 1-adrenergic receptors: in vitro autoradiography with [125I]-heat. J Comp Neurol. 1985 Jan 8;231(2):190–208. doi: 10.1002/cne.902310207. [DOI] [PubMed] [Google Scholar]
- Jouvet M. The role of monoamines and acetylcholine-containing neurons in the regulation of the sleep-waking cycle. Ergeb Physiol. 1972;64:166–307. doi: 10.1007/3-540-05462-6_2. [DOI] [PubMed] [Google Scholar]
- Lamarre Y., Filion M., Cordeau J. P. Neuronal discharges of the ventrolateral nucleus of the thalamus during sleep and wakefulness in the cat. I. Spontaneous activity. Exp Brain Res. 1971 Jun 29;12(5):480–498. doi: 10.1007/BF00234244. [DOI] [PubMed] [Google Scholar]
- Lindvall O., Björklund A., Nobin A., Stenevi U. The adrenergic innervation of the rat thalamus as revealed by the glyoxylic acid fluorescence method. J Comp Neurol. 1974 Apr 1;154(3):317–347. doi: 10.1002/cne.901540307. [DOI] [PubMed] [Google Scholar]
- Livingstone M. S., Hubel D. H. Effects of sleep and arousal on the processing of visual information in the cat. Nature. 1981 Jun 18;291(5816):554–561. doi: 10.1038/291554a0. [DOI] [PubMed] [Google Scholar]
- McCarley R. W., Benoit O., Barrionuevo G. Lateral geniculate nucleus unitary discharge in sleep and waking: state- and rate-specific aspects. J Neurophysiol. 1983 Oct;50(4):798–818. doi: 10.1152/jn.1983.50.4.798. [DOI] [PubMed] [Google Scholar]
- McCormick D. A. Cholinergic and noradrenergic modulation of thalamocortical processing. Trends Neurosci. 1989 Jun;12(6):215–221. doi: 10.1016/0166-2236(89)90125-2. [DOI] [PubMed] [Google Scholar]
- McCormick D. A., Pape H. C. Properties of a hyperpolarization-activated cation current and its role in rhythmic oscillation in thalamic relay neurones. J Physiol. 1990 Dec;431:291–318. doi: 10.1113/jphysiol.1990.sp018331. [DOI] [PMC free article] [PubMed] [Google Scholar]
- McCormick D. A., Prince D. A. Noradrenergic modulation of firing pattern in guinea pig and cat thalamic neurons, in vitro. J Neurophysiol. 1988 Mar;59(3):978–996. doi: 10.1152/jn.1988.59.3.978. [DOI] [PubMed] [Google Scholar]
- McCormick D. A., Williamson A. Convergence and divergence of neurotransmitter action in human cerebral cortex. Proc Natl Acad Sci U S A. 1989 Oct;86(20):8098–8102. doi: 10.1073/pnas.86.20.8098. [DOI] [PMC free article] [PubMed] [Google Scholar]
- Morrison J. H., Foote S. L. Noradrenergic and serotoninergic innervation of cortical, thalamic, and tectal visual structures in Old and New World monkeys. J Comp Neurol. 1986 Jan 1;243(1):117–138. doi: 10.1002/cne.902430110. [DOI] [PubMed] [Google Scholar]
- Pape H. C., McCormick D. A. Noradrenaline and serotonin selectively modulate thalamic burst firing by enhancing a hyperpolarization-activated cation current. Nature. 1989 Aug 31;340(6236):715–718. doi: 10.1038/340715a0. [DOI] [PubMed] [Google Scholar]
- Pazos A., Cortés R., Palacios J. M. Quantitative autoradiographic mapping of serotonin receptors in the rat brain. II. Serotonin-2 receptors. Brain Res. 1985 Nov 4;346(2):231–249. doi: 10.1016/0006-8993(85)90857-1. [DOI] [PubMed] [Google Scholar]
- Pazos A., Palacios J. M. Quantitative autoradiographic mapping of serotonin receptors in the rat brain. I. Serotonin-1 receptors. Brain Res. 1985 Nov 4;346(2):205–230. doi: 10.1016/0006-8993(85)90856-x. [DOI] [PubMed] [Google Scholar]
- Peroutka S. J. 5-Hydroxytryptamine receptor subtypes. Annu Rev Neurosci. 1988;11:45–60. doi: 10.1146/annurev.ne.11.030188.000401. [DOI] [PubMed] [Google Scholar]
- Seamon K. B., Daly J. W. Forskolin: its biological and chemical properties. Adv Cyclic Nucleotide Protein Phosphorylation Res. 1986;20:1–150. [PubMed] [Google Scholar]
- Steriade M., Deschenes M. The thalamus as a neuronal oscillator. Brain Res. 1984 Nov;320(1):1–63. doi: 10.1016/0165-0173(84)90017-1. [DOI] [PubMed] [Google Scholar]
- Steriade M., Deschênes M., Domich L., Mulle C. Abolition of spindle oscillations in thalamic neurons disconnected from nucleus reticularis thalami. J Neurophysiol. 1985 Dec;54(6):1473–1497. doi: 10.1152/jn.1985.54.6.1473. [DOI] [PubMed] [Google Scholar]
- Steriade M., Llinás R. R. The functional states of the thalamus and the associated neuronal interplay. Physiol Rev. 1988 Jul;68(3):649–742. doi: 10.1152/physrev.1988.68.3.649. [DOI] [PubMed] [Google Scholar]
- Tokimasa T., Akasu T. Cyclic AMP regulates an inward rectifying sodium-potassium current in dissociated bull-frog sympathetic neurones. J Physiol. 1990 Jan;420:409–429. doi: 10.1113/jphysiol.1990.sp017920. [DOI] [PMC free article] [PubMed] [Google Scholar]
- Trulson M. E., Jacobs B. L. Raphe unit activity in freely moving cats: correlation with level of behavioral arousal. Brain Res. 1979 Mar 9;163(1):135–150. doi: 10.1016/0006-8993(79)90157-4. [DOI] [PubMed] [Google Scholar]
- Wagoner P. K., Pallotta B. S. Modulation of acetylcholine receptor desensitization by forskolin is independent of cAMP. Science. 1988 Jun 17;240(4859):1655–1657. doi: 10.1126/science.2454507. [DOI] [PubMed] [Google Scholar]