Abstract
Experiments are described in which the [Ca]o-dependent component of 22Na efflux is monitored under conditions of membrane potential control by voltage clamp. The apparent affinity of the efflux system for external Ca is very low in choline sea water (apparent KD approximately 50 mM); but increases dramatically when choline is replaced isosmotically by Li or K (apparent KD approximately 1-2 mM). Ca influx changes in a parallel fashion. Tris behaves much like choline and guanidinium is about two-thirds as effective as Li. Replacement of Li by K has little effect on the apparent affinity for external Ca but brings about a small (30-40%) increase in the maximal flux. The increase in maximum flux can be removed by electrical hyperpolarization to the potential before application of K and, in the absence of K, can be mimicked by electrical depolarization. These experiments suggest that the stimulatory effect of K on the Ca-dependent Na efflux into Li sea water is electrical in origin. Partial replacement of choline by K stimulates the Ca-dependent Na efflux; but only part of this stimulation can be removed by electrical hyperpolarization and, in the absence of K, electrical depolarization only brings about a relatively small stimulation. This is because only part of the stimulation that follows addition of K to choline sea waters is electrical in origin: the rest reflects an increase in the apparent affinity for external Ca that is brought about by K acting chemically. The maximum efflux into K is about 40% higher than that into choline. That this may reflect an electrical effect is supported by the observation that electrical depolarization increases the flux into choline sea water containing 110 mM-Ca where the Ca-binding site is close to saturation. The voltage clamp was used to determine the voltage dependence of the Ca-dependent Na efflux into Li sea water, choline sea water and choline sea water containing 100 mM-Na. In all three cases the flux increased with depolarization and was still rising at +70 mV. The dependence on potential was not very steep, an e-fold increase occurred over approximately 50 mV.
Full text
PDF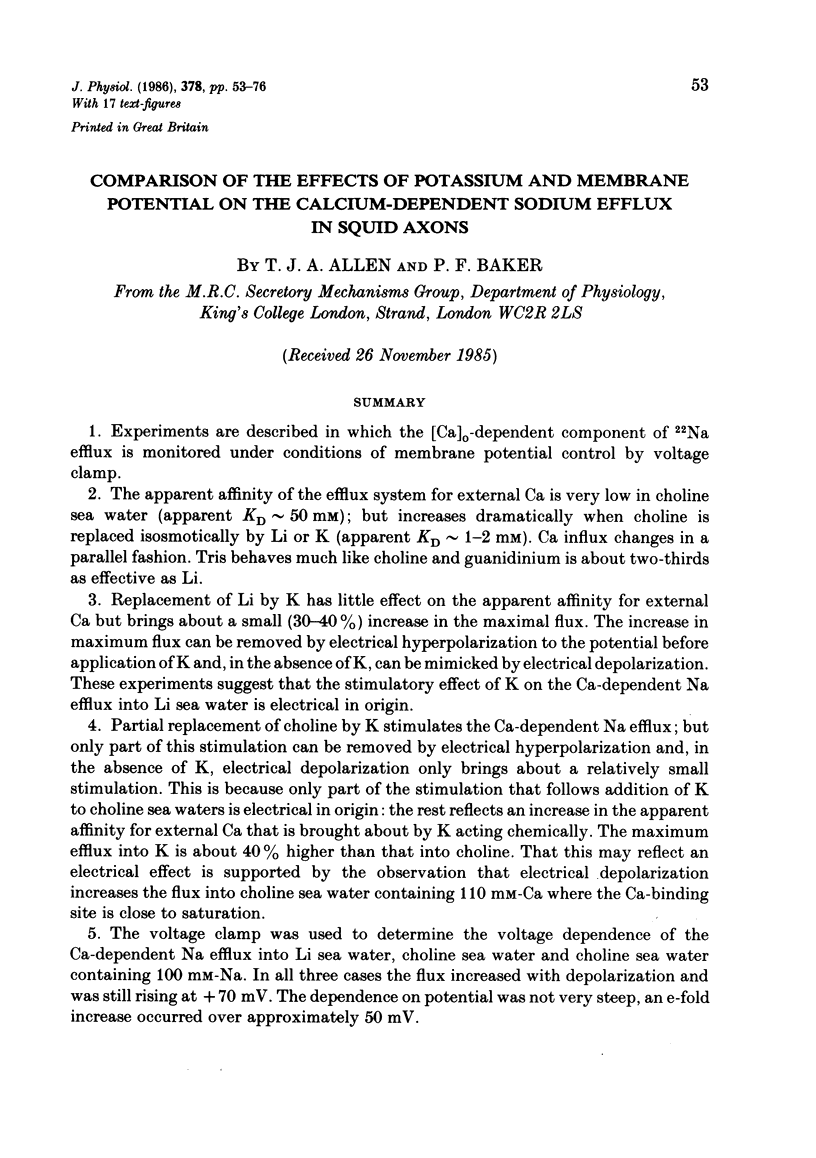
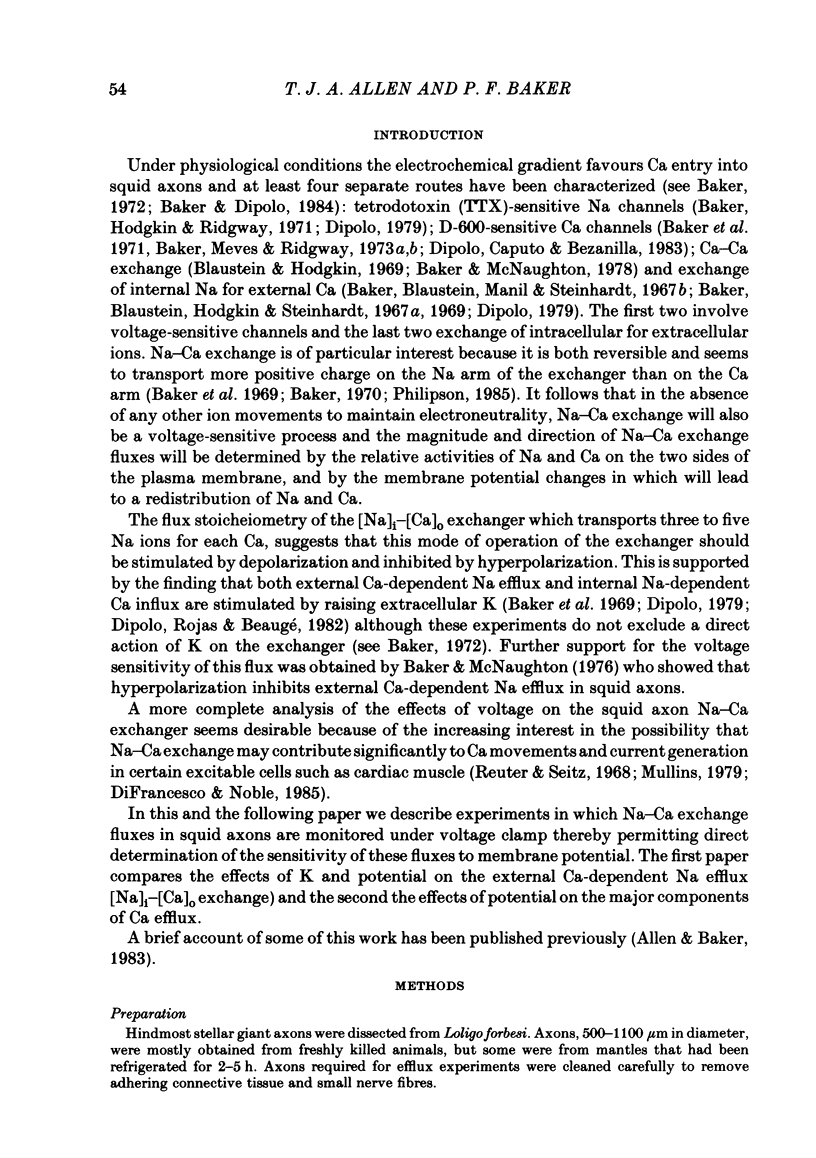
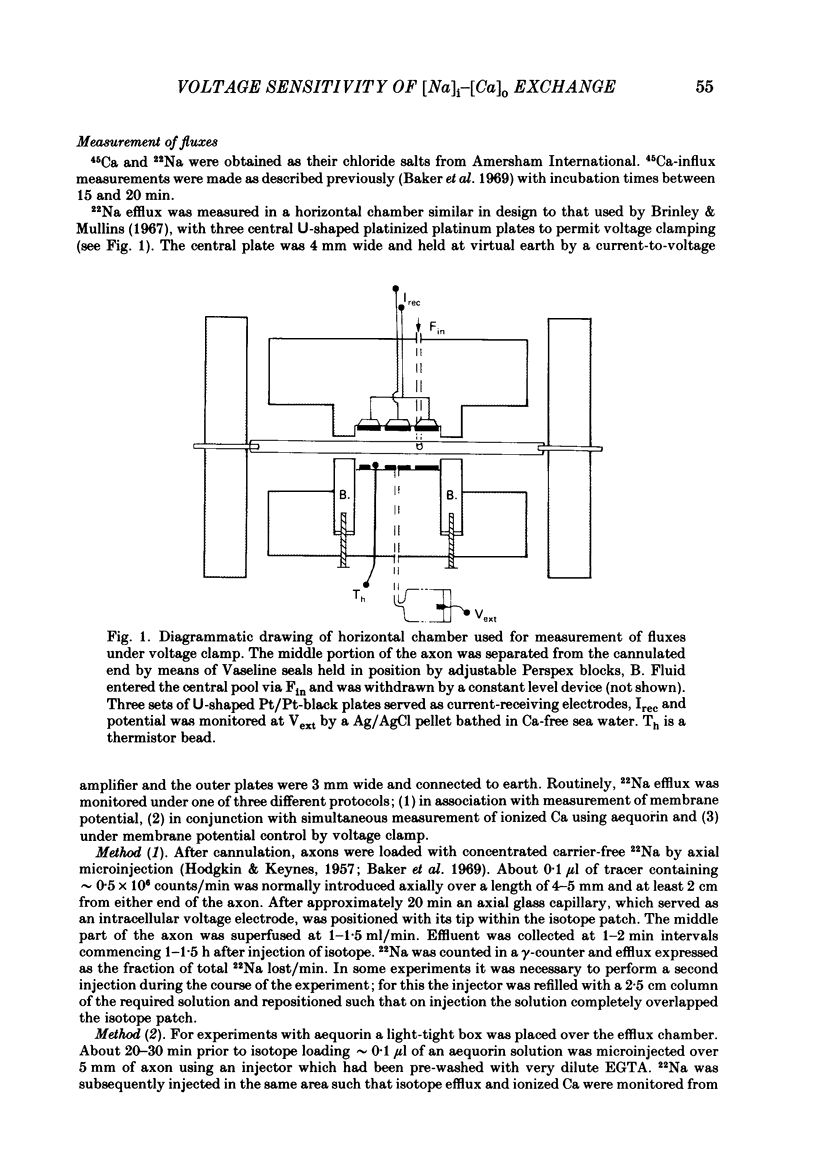
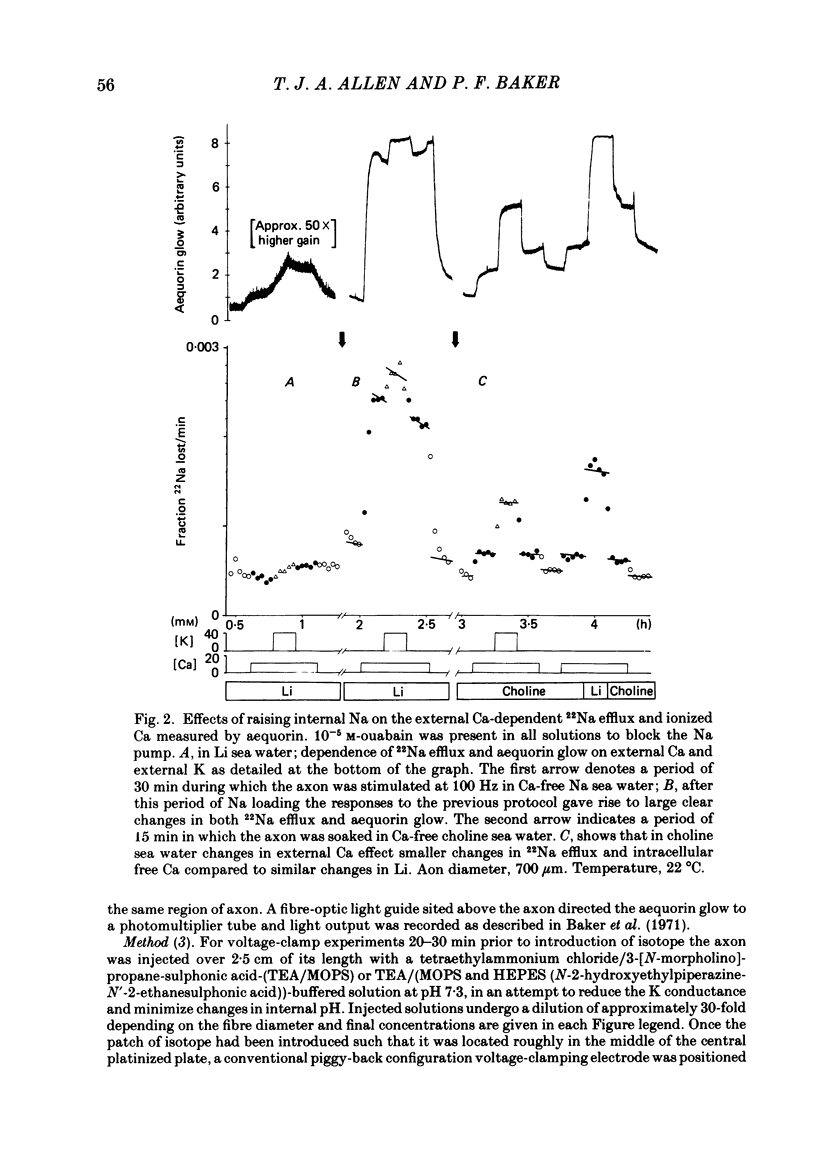
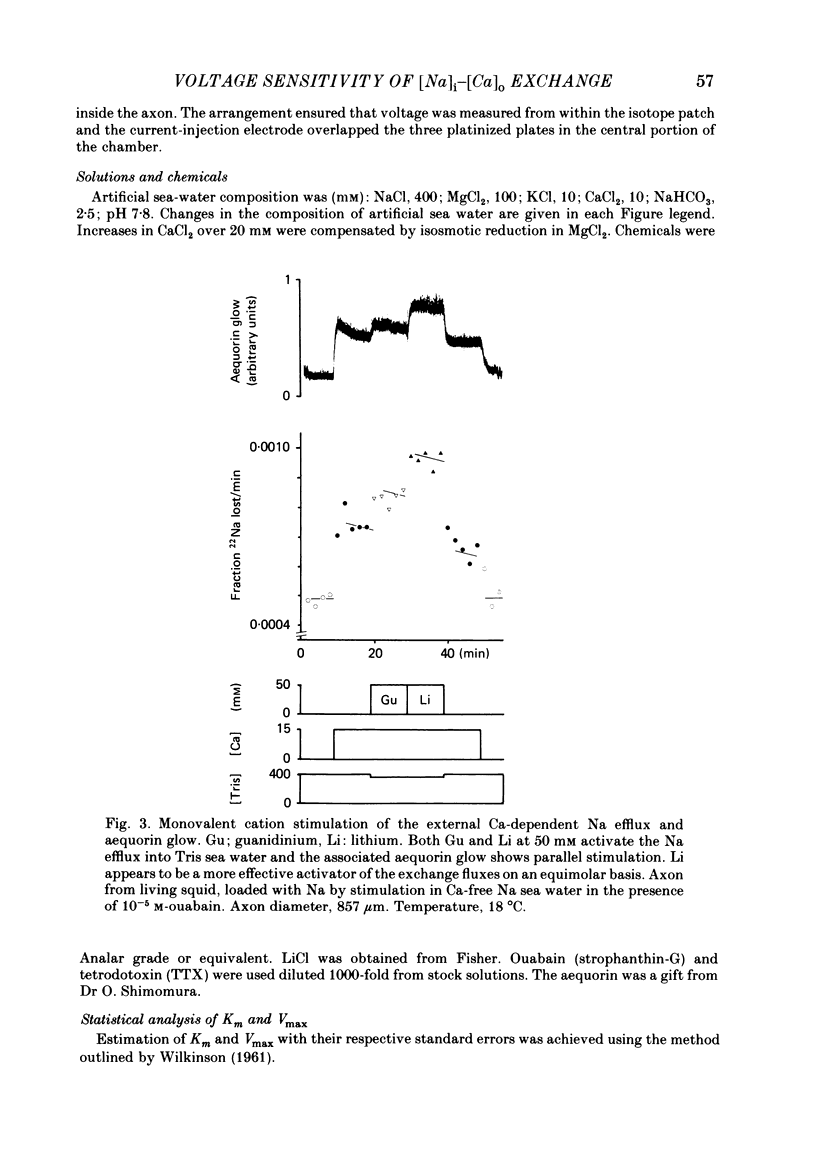
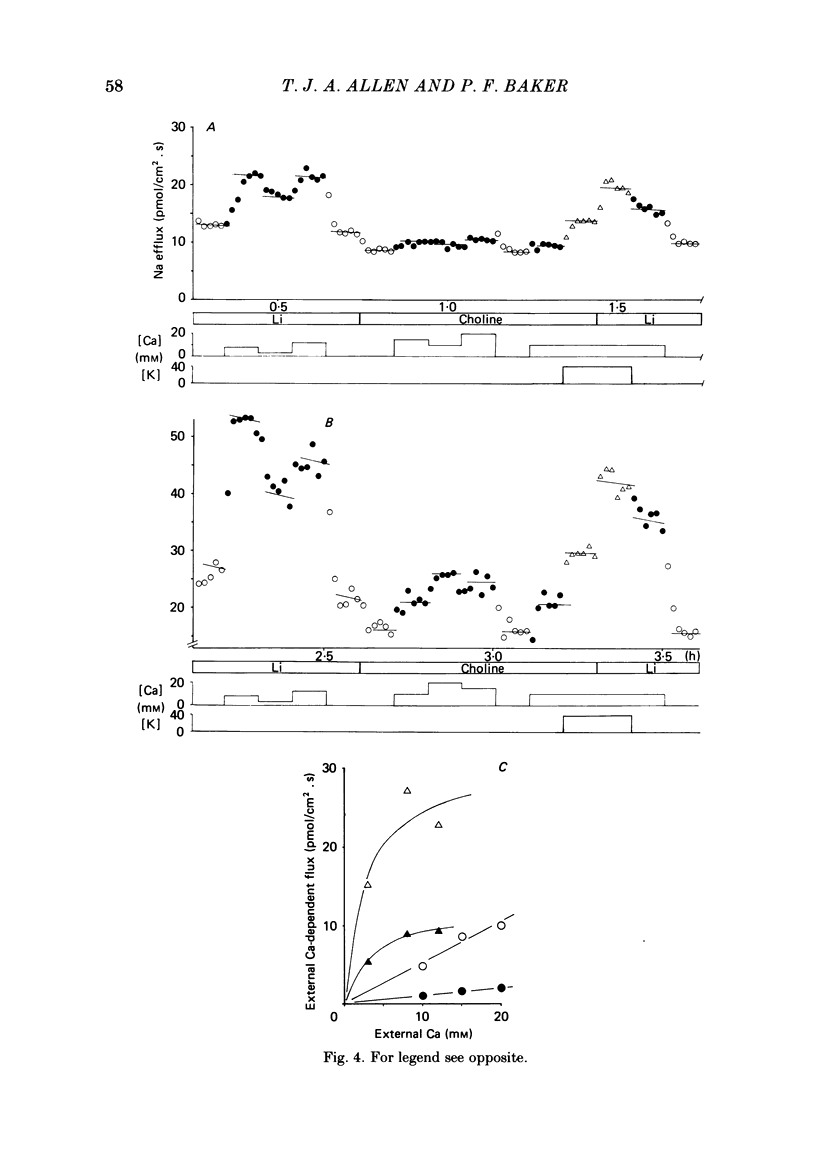
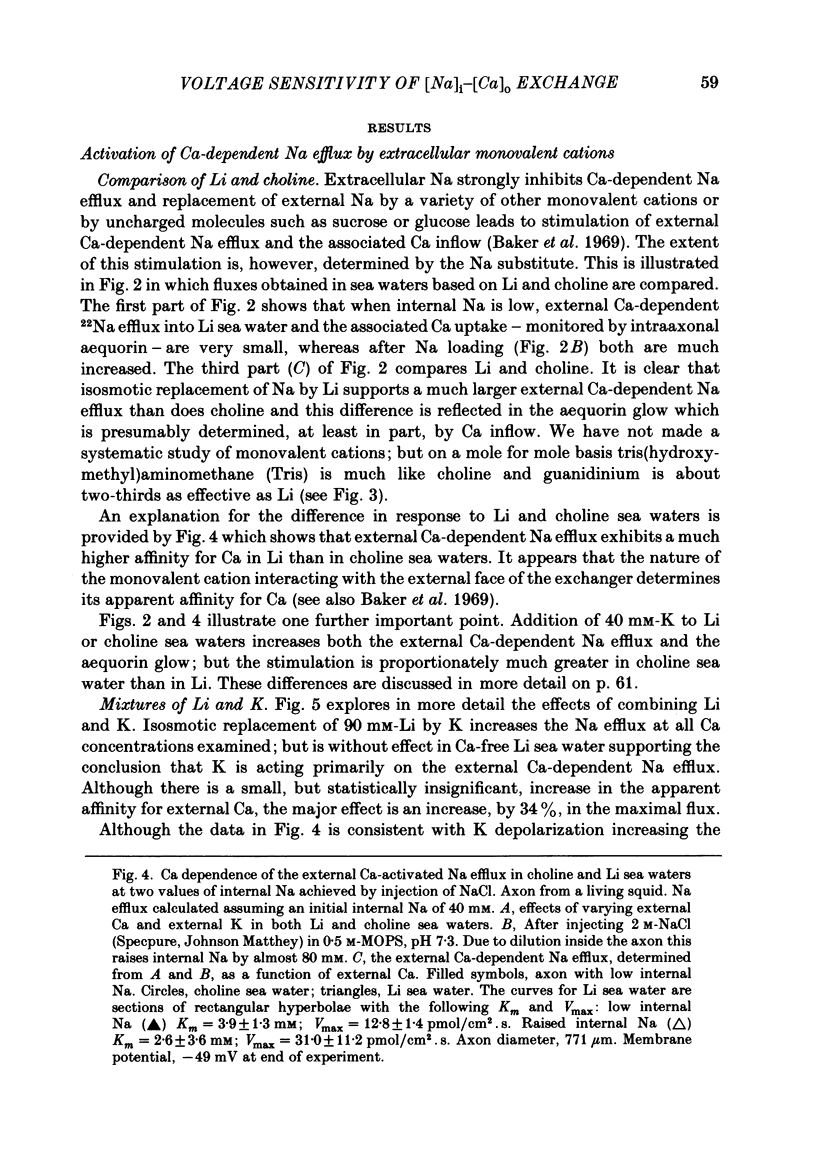
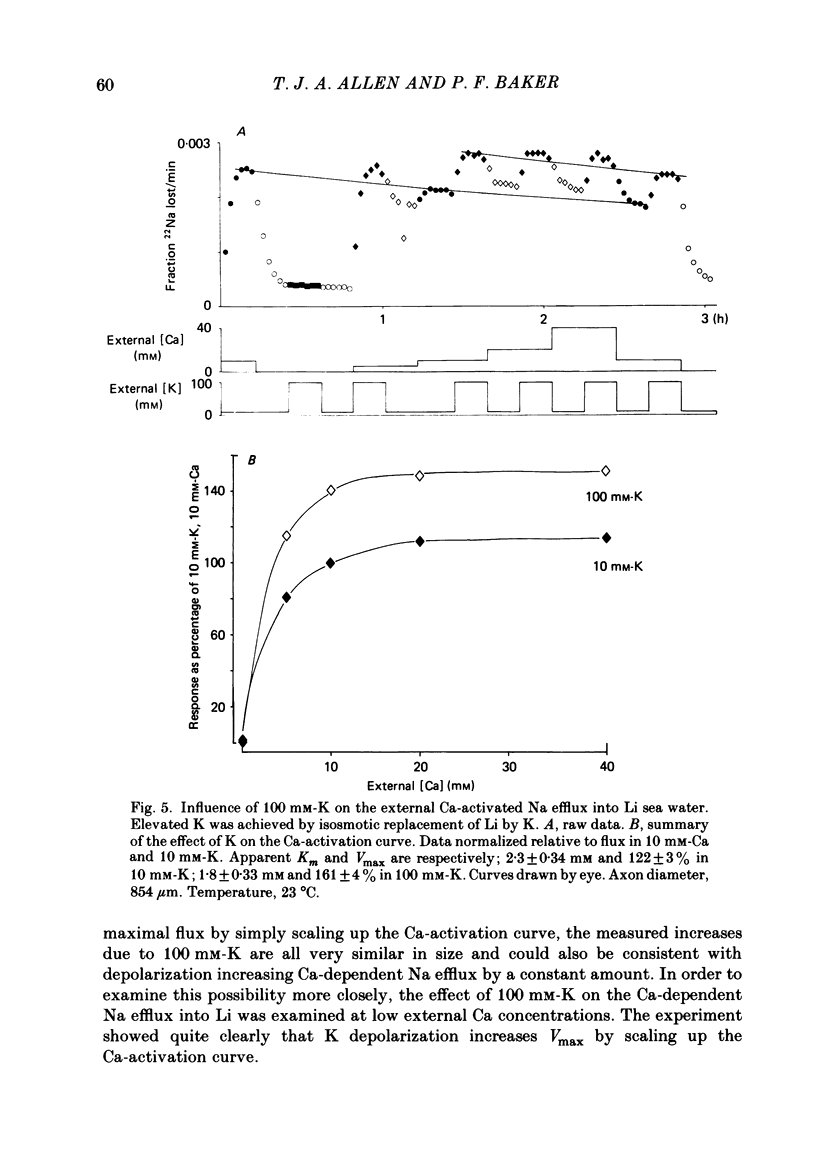
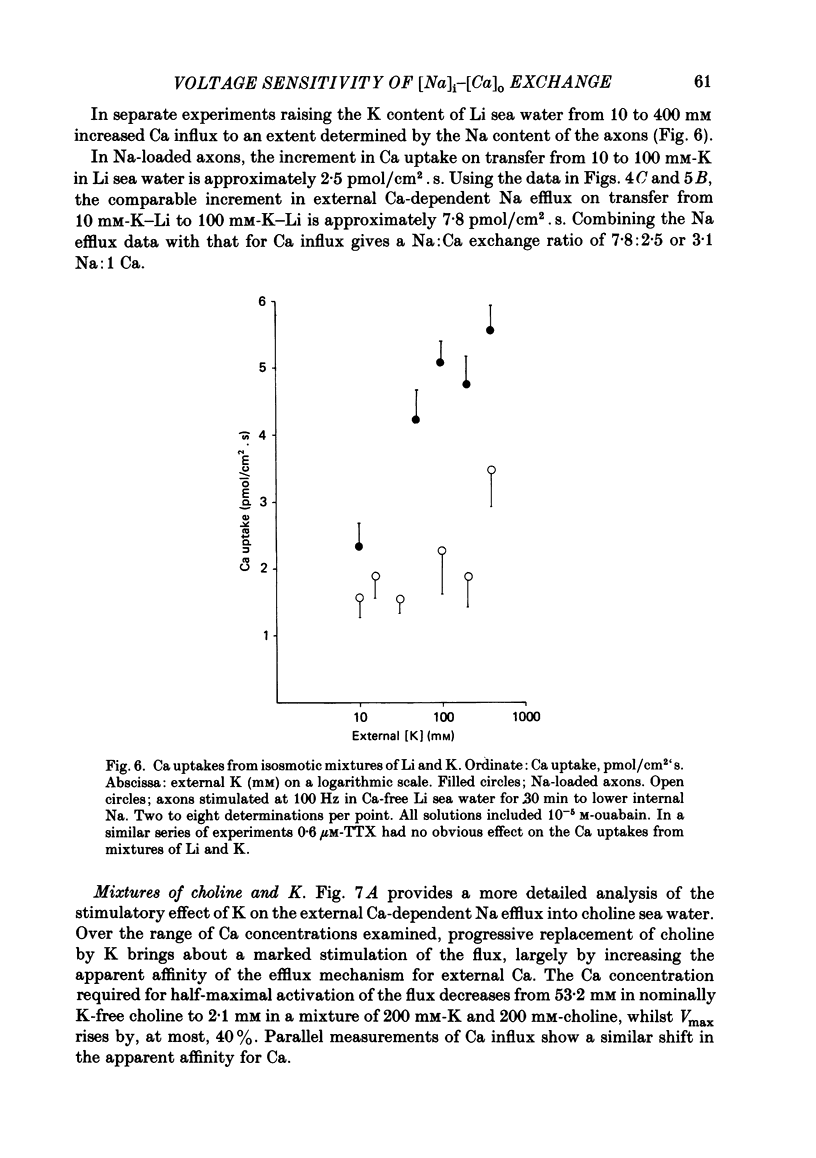
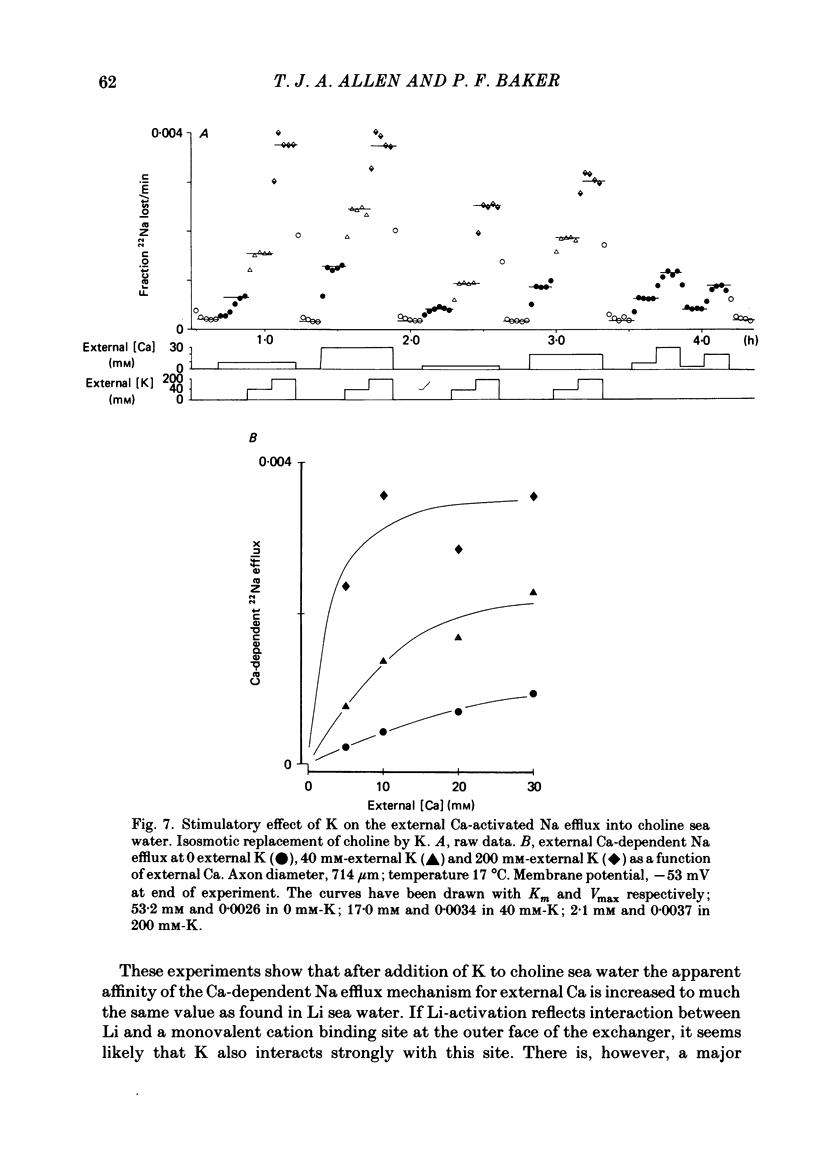
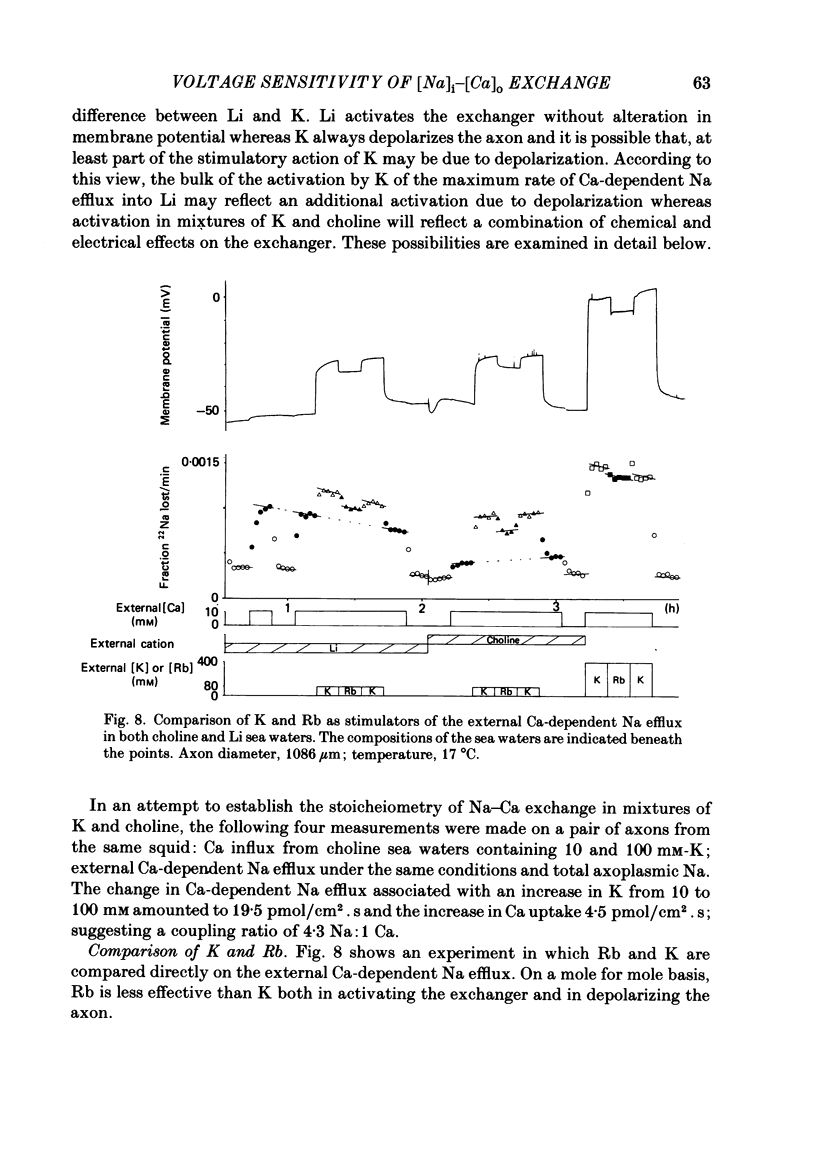
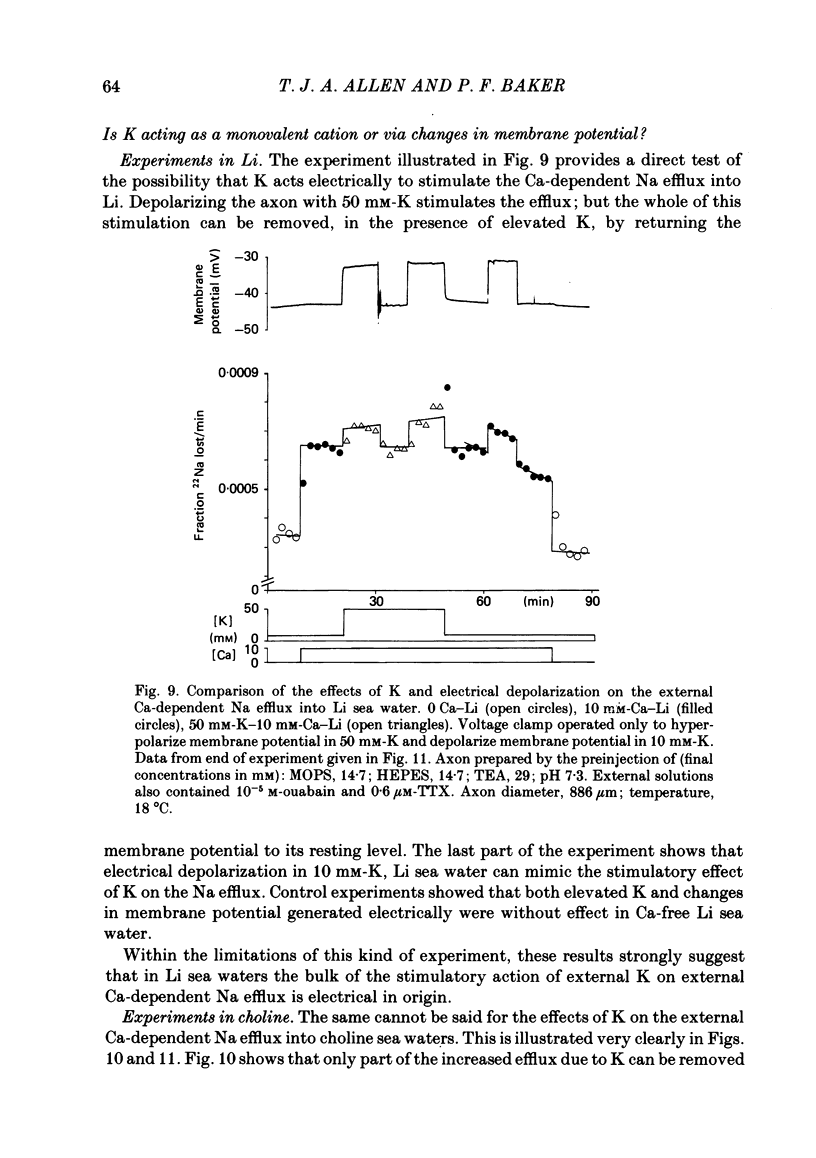
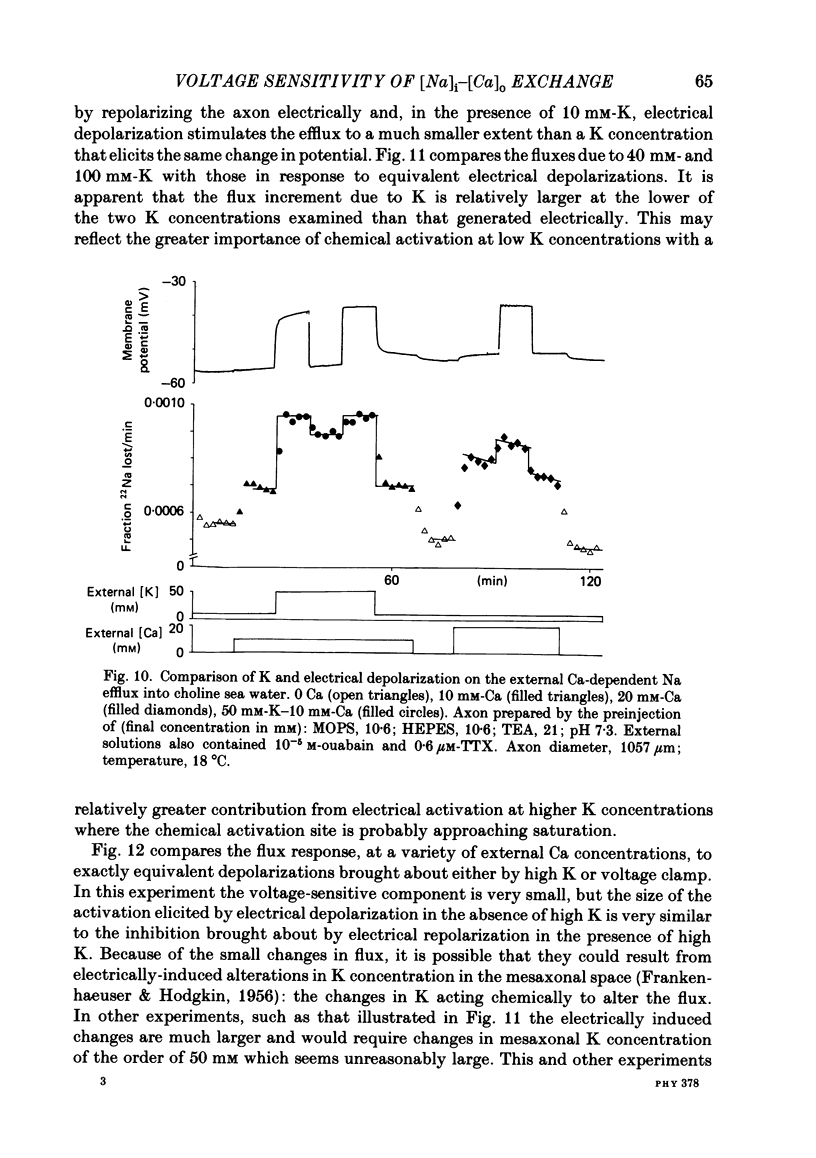
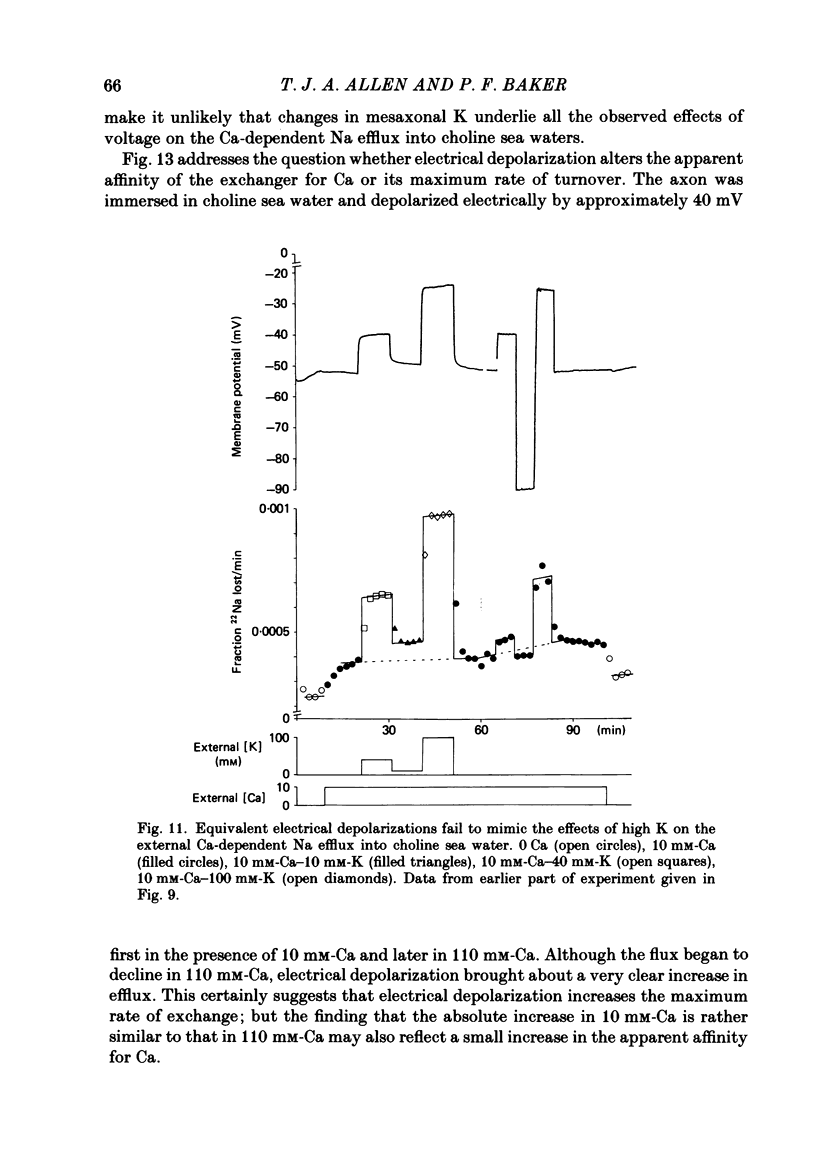
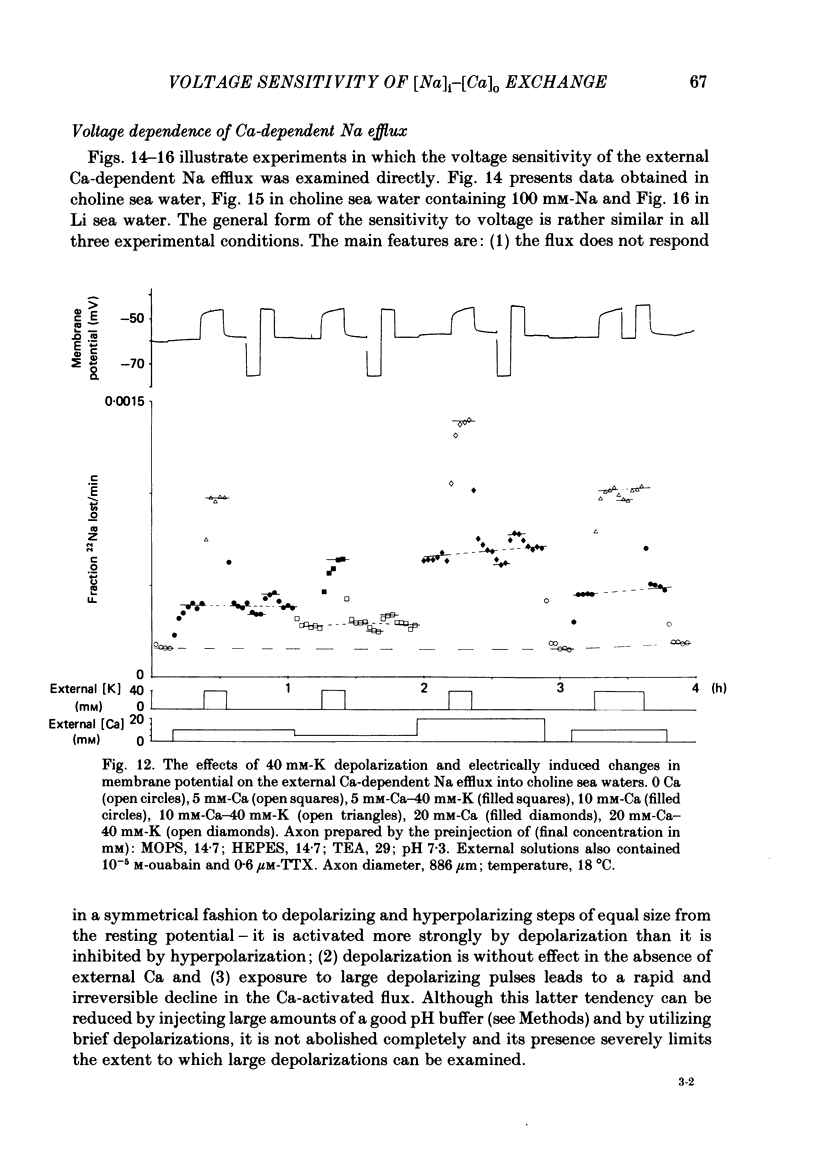
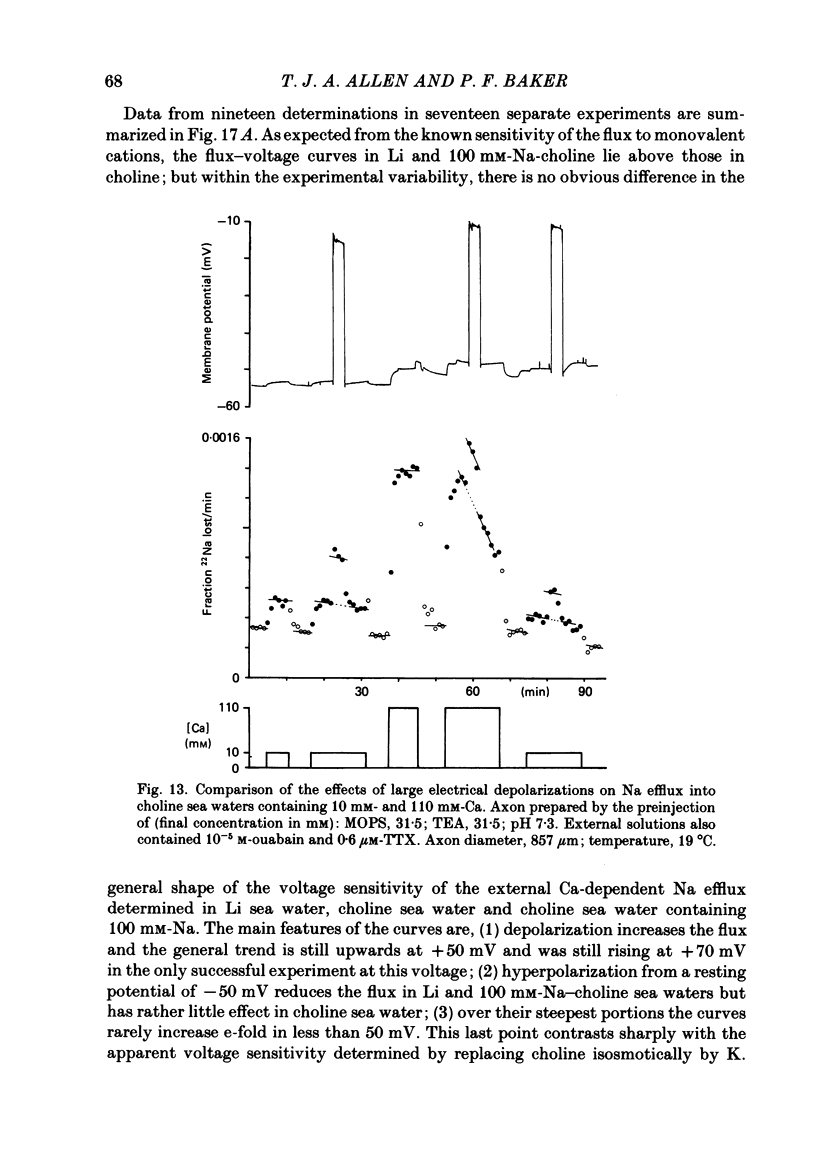
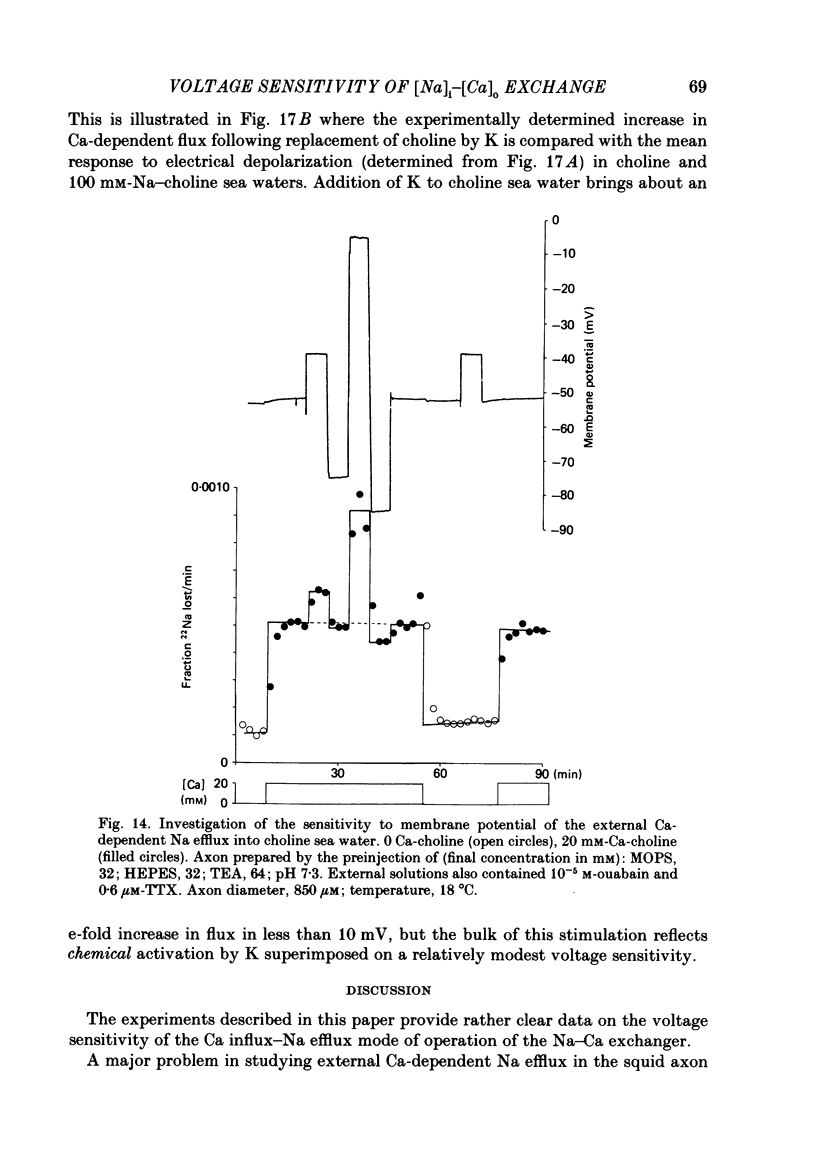
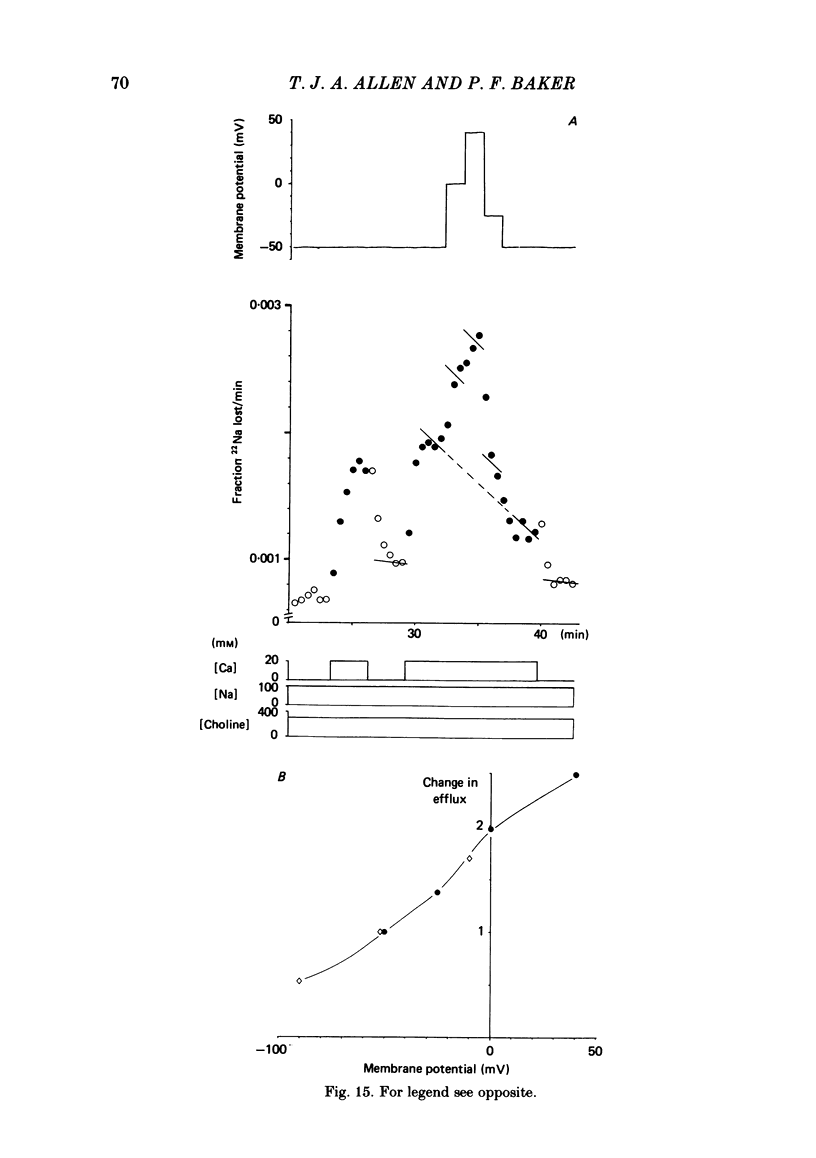
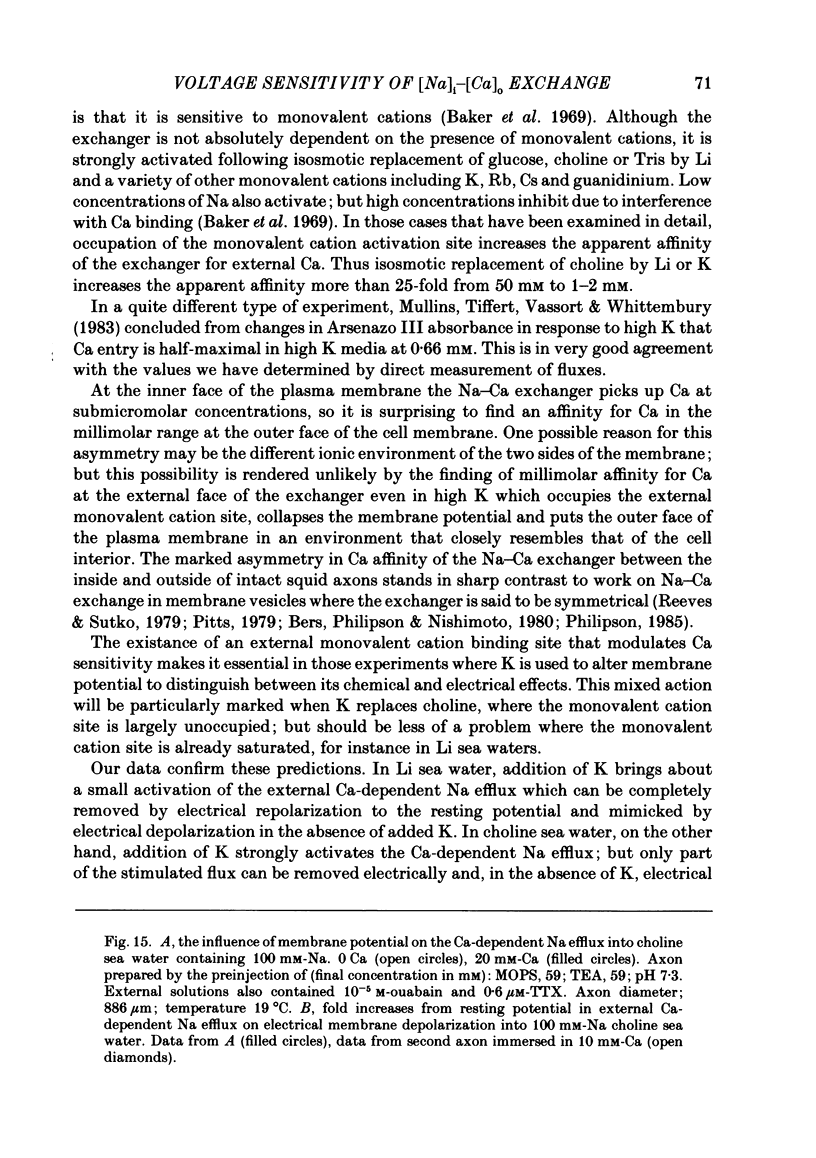
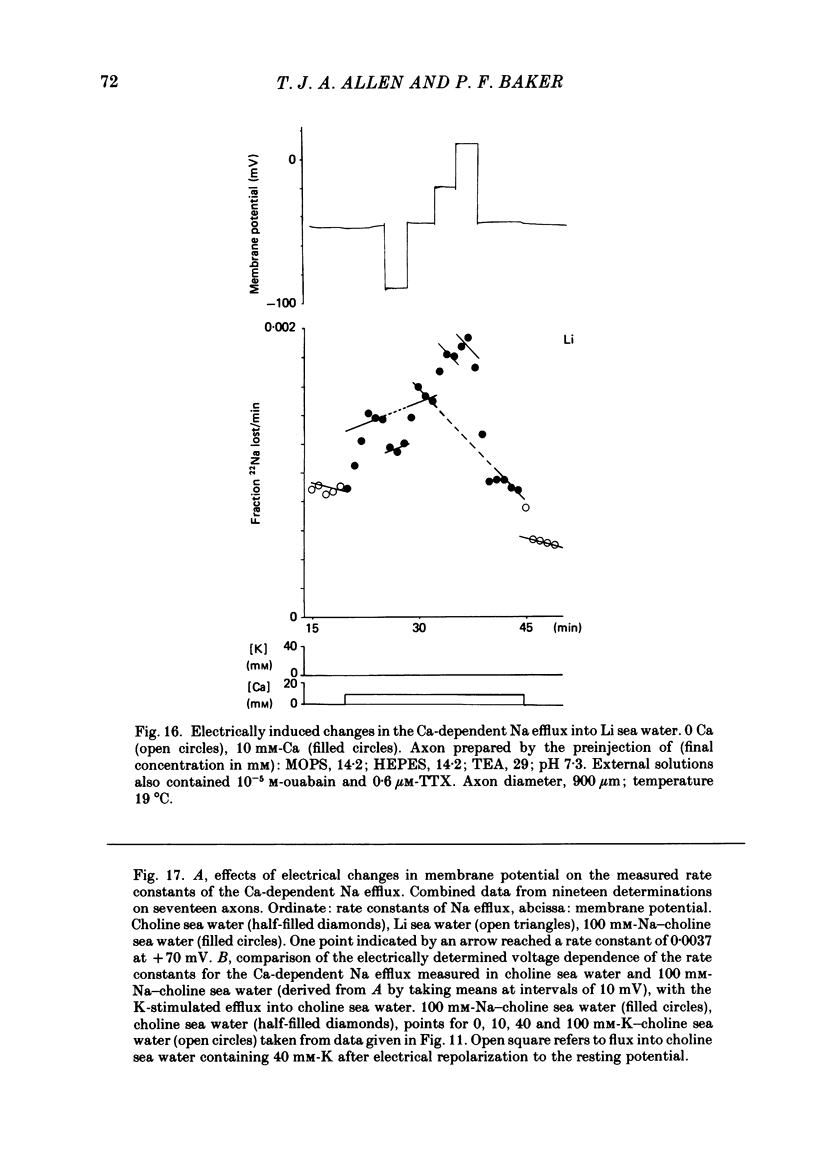
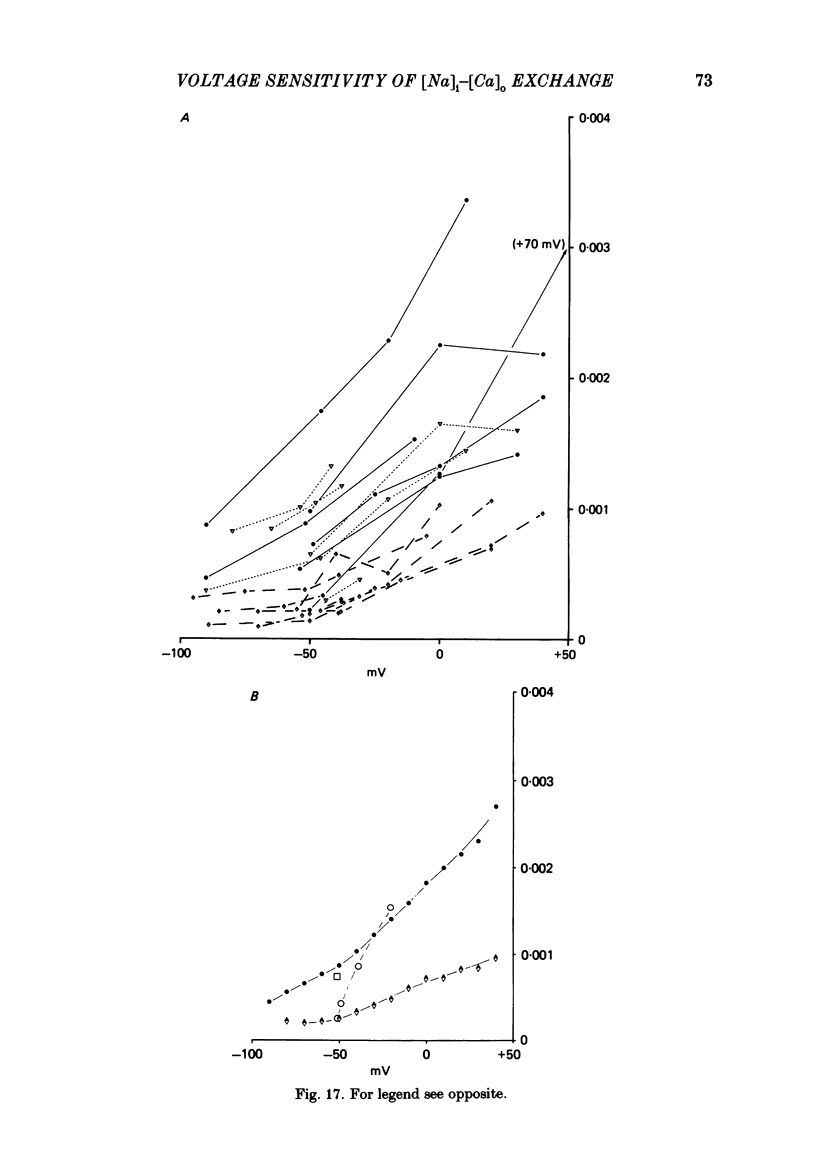
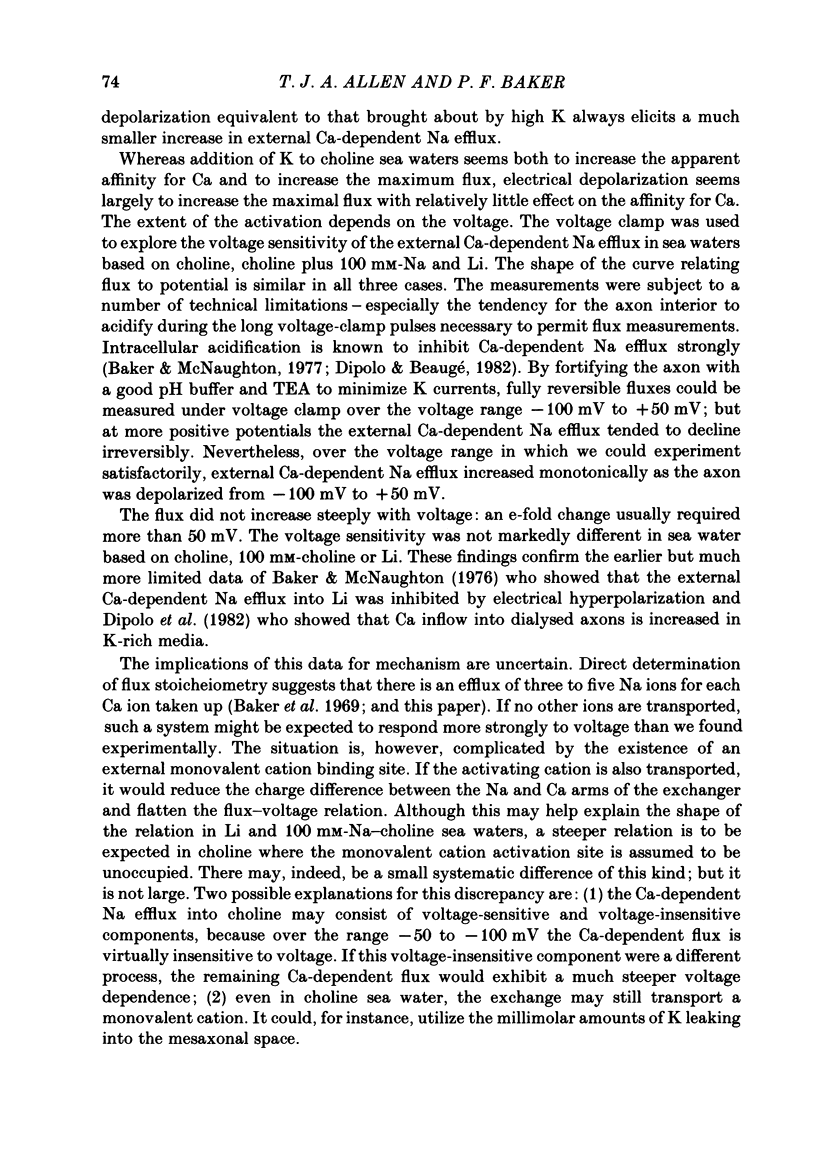
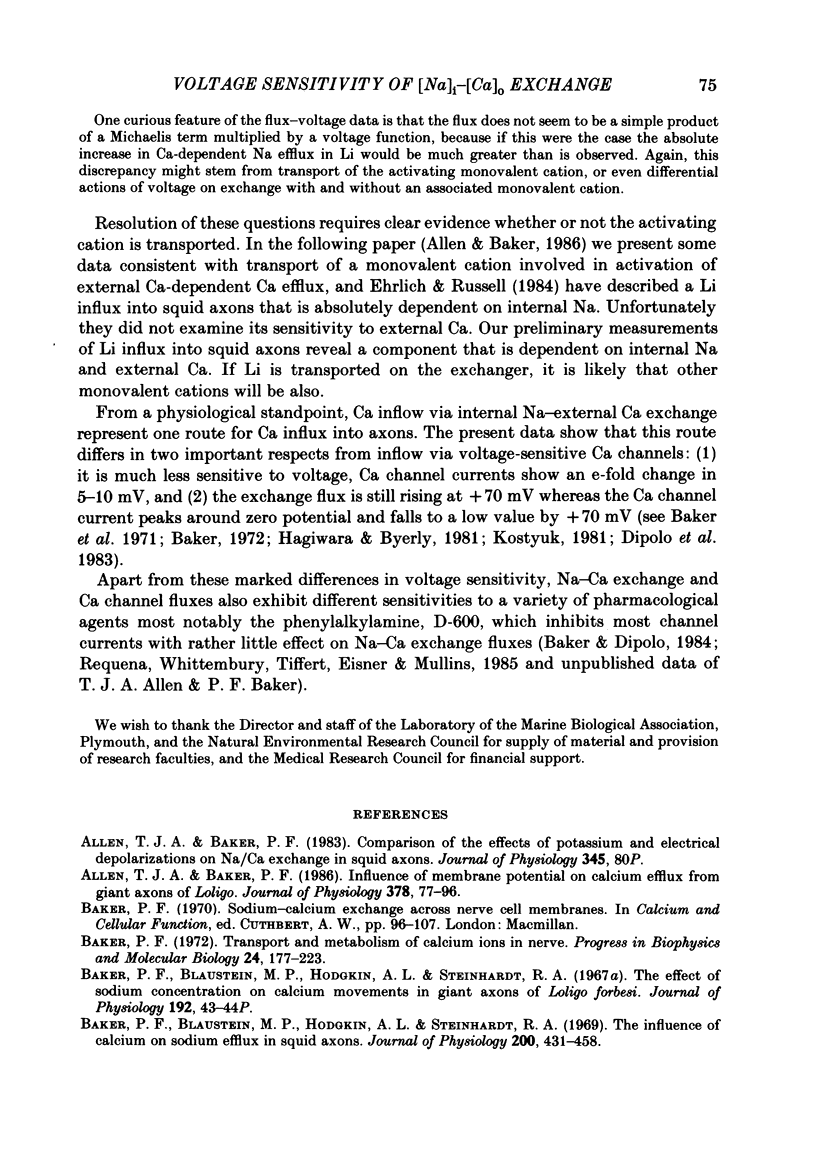
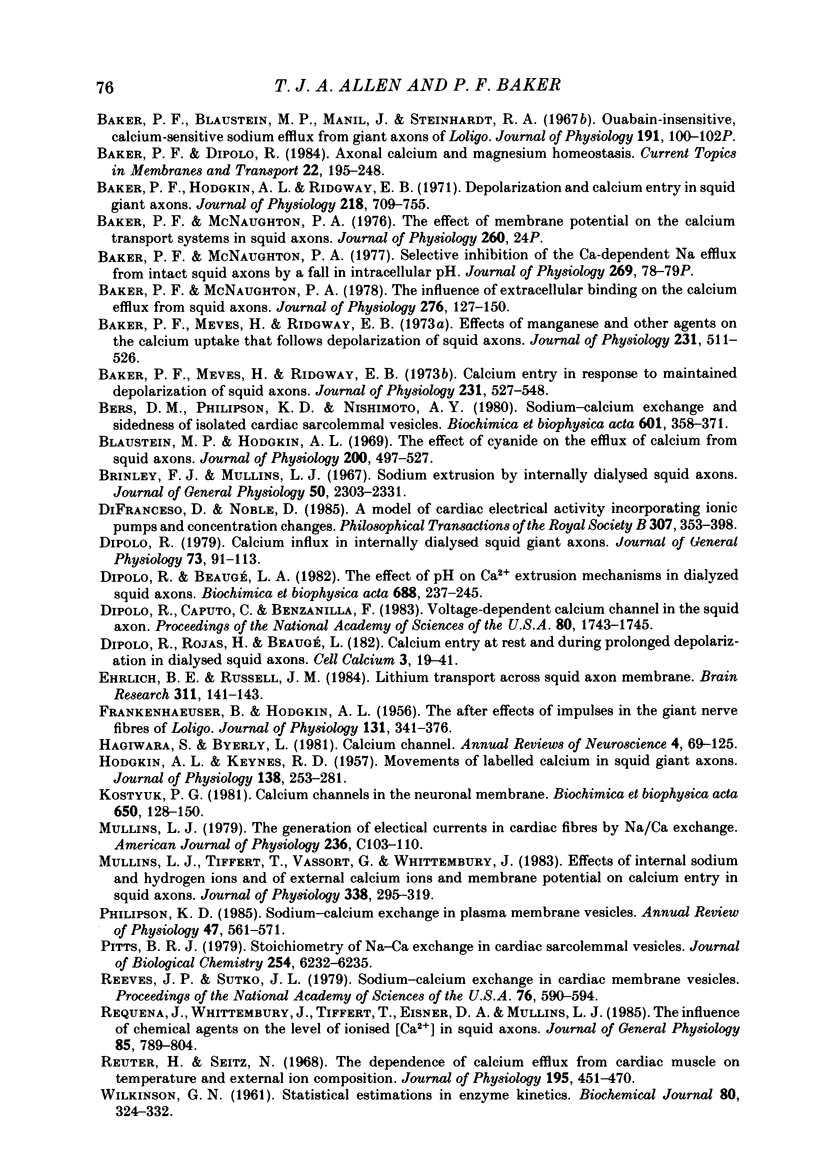
Selected References
These references are in PubMed. This may not be the complete list of references from this article.
- Allen T. J., Baker P. F. Influence of membrane potential on calcium efflux from giant axons of Loligo. J Physiol. 1986 Sep;378:77–96. doi: 10.1113/jphysiol.1986.sp016208. [DOI] [PMC free article] [PubMed] [Google Scholar]
- Baker P. F., Blaustein M. P., Hodgkin A. L., Steinhardt R. A. The influence of calcium on sodium efflux in squid axons. J Physiol. 1969 Feb;200(2):431–458. doi: 10.1113/jphysiol.1969.sp008702. [DOI] [PMC free article] [PubMed] [Google Scholar]
- Baker P. F., Blaustein M. P., Manil J., Steinhardt R. A. A ouabain-insensitive, calcium-sensitive sodium efflux from giant axons of Loligo. J Physiol. 1967 Jul;191(2):100P–102P. [PMC free article] [PubMed] [Google Scholar]
- Baker P. F., Hodgkin A. L., Ridgway E. B. Depolarization and calcium entry in squid giant axons. J Physiol. 1971 Nov;218(3):709–755. doi: 10.1113/jphysiol.1971.sp009641. [DOI] [PMC free article] [PubMed] [Google Scholar]
- Baker P. F., McNaughton P. A. Selective inhibition of the Ca-dependent Na efflux from intact squid axons by a fall in intracellular pH [proceedings]. J Physiol. 1977 Jul;269(1):78P–79P. [PubMed] [Google Scholar]
- Baker P. F., McNaughton P. A. The effect of membrane potential on the calcium transport systems in squid axons [proceedings]. J Physiol. 1976 Sep;260(2):24P–25P. [PubMed] [Google Scholar]
- Baker P. F., McNaughton P. A. The influence of extracellular calcium binding on the calcium efflux from squid axons. J Physiol. 1978 Mar;276:127–150. doi: 10.1113/jphysiol.1978.sp012223. [DOI] [PMC free article] [PubMed] [Google Scholar]
- Baker P. F., Meves H., Ridgway E. B. Calcium entry in response to maintained depolarization of squid axons. J Physiol. 1973 Jun;231(3):527–548. doi: 10.1113/jphysiol.1973.sp010247. [DOI] [PMC free article] [PubMed] [Google Scholar]
- Baker P. F., Meves H., Ridgway E. B. Effects of manganese and other agents on the calcium uptake that follows depolarization of squid axons. J Physiol. 1973 Jun;231(3):511–526. doi: 10.1113/jphysiol.1973.sp010246. [DOI] [PMC free article] [PubMed] [Google Scholar]
- Baker P. F. Transport and metabolism of calcium ions in nerve. Prog Biophys Mol Biol. 1972;24:177–223. doi: 10.1016/0079-6107(72)90007-7. [DOI] [PubMed] [Google Scholar]
- Bers D. M., Philipson K. D., Nishimoto A. Y. Sodium-calcium exchange and sidedness of isolated cardiac sarcolemmal vesicles. Biochim Biophys Acta. 1980 Sep 18;601(2):358–371. doi: 10.1016/0005-2736(80)90540-4. [DOI] [PubMed] [Google Scholar]
- Blaustein M. P., Hodgkin A. L. The effect of cyanide on the efflux of calcium from squid axons. J Physiol. 1969 Feb;200(2):497–527. doi: 10.1113/jphysiol.1969.sp008704. [DOI] [PMC free article] [PubMed] [Google Scholar]
- Brinley F. J., Jr, Mullins L. J. Sodium extrusion by internally dialyzed squid axons. J Gen Physiol. 1967 Nov;50(10):2303–2331. doi: 10.1085/jgp.50.10.2303. [DOI] [PMC free article] [PubMed] [Google Scholar]
- DiFrancesco D., Noble D. A model of cardiac electrical activity incorporating ionic pumps and concentration changes. Philos Trans R Soc Lond B Biol Sci. 1985 Jan 10;307(1133):353–398. doi: 10.1098/rstb.1985.0001. [DOI] [PubMed] [Google Scholar]
- DiPolo R. Calcium influx in internally dialyzed squid giant axons. J Gen Physiol. 1979 Jan;73(1):91–113. doi: 10.1085/jgp.73.1.91. [DOI] [PMC free article] [PubMed] [Google Scholar]
- DiPolo R., Caputo C., Bezanilla F. Voltage-dependent calcium channel in the squid axon. Proc Natl Acad Sci U S A. 1983 Mar;80(6):1743–1745. doi: 10.1073/pnas.80.6.1743. [DOI] [PMC free article] [PubMed] [Google Scholar]
- Dipolo R., Beaugé L. The effect of pH on Ca2+ extrusion mechanisms in dialyzed squid axons. Biochim Biophys Acta. 1982 May 21;688(1):237–245. doi: 10.1016/0005-2736(82)90599-5. [DOI] [PubMed] [Google Scholar]
- Ehrlich B. E., Russell J. M. Lithium transport across squid axon membrane. Brain Res. 1984 Oct 8;311(1):141–143. doi: 10.1016/0006-8993(84)91406-9. [DOI] [PubMed] [Google Scholar]
- FRANKENHAEUSER B., HODGKIN A. L. The after-effects of impulses in the giant nerve fibres of Loligo. J Physiol. 1956 Feb 28;131(2):341–376. doi: 10.1113/jphysiol.1956.sp005467. [DOI] [PMC free article] [PubMed] [Google Scholar]
- HODGKIN A. L., KEYNES R. D. Movements of labelled calcium in squid giant axons. J Physiol. 1957 Sep 30;138(2):253–281. doi: 10.1113/jphysiol.1957.sp005850. [DOI] [PMC free article] [PubMed] [Google Scholar]
- Hagiwara S., Byerly L. Calcium channel. Annu Rev Neurosci. 1981;4:69–125. doi: 10.1146/annurev.ne.04.030181.000441. [DOI] [PubMed] [Google Scholar]
- Kostyuk P. G. Calcium channels in the neuronal membrane. Biochim Biophys Acta. 1981 Dec;650(2-3):128–150. doi: 10.1016/0304-4157(81)90003-4. [DOI] [PubMed] [Google Scholar]
- Lloyd S., Pickford M. The effect of oxytocin and adrenaline on blood flow in the hind limb of the dog following chronic lumbar sympathectomy. J Physiol. 1967 Sep;192(1):43–52. doi: 10.1113/jphysiol.1967.sp008286. [DOI] [PMC free article] [PubMed] [Google Scholar]
- Mullins L. J. The generation of electric currents in cardiac fibers by Na/Ca exchange. Am J Physiol. 1979 Mar;236(3):C103–C110. doi: 10.1152/ajpcell.1979.236.3.C103. [DOI] [PubMed] [Google Scholar]
- Mullins L. J., Tiffert T., Vassort G., Whittembury J. Effects of internal sodium and hydrogen ions and of external calcium ions and membrane potential on calcium entry in squid axons. J Physiol. 1983 May;338:295–319. doi: 10.1113/jphysiol.1983.sp014674. [DOI] [PMC free article] [PubMed] [Google Scholar]
- Philipson K. D. Sodium-calcium exchange in plasma membrane vesicles. Annu Rev Physiol. 1985;47:561–571. doi: 10.1146/annurev.ph.47.030185.003021. [DOI] [PubMed] [Google Scholar]
- Pitts B. J. Stoichiometry of sodium-calcium exchange in cardiac sarcolemmal vesicles. Coupling to the sodium pump. J Biol Chem. 1979 Jul 25;254(14):6232–6235. [PubMed] [Google Scholar]
- Reeves J. P., Sutko J. L. Sodium-calcium ion exchange in cardiac membrane vesicles. Proc Natl Acad Sci U S A. 1979 Feb;76(2):590–594. doi: 10.1073/pnas.76.2.590. [DOI] [PMC free article] [PubMed] [Google Scholar]
- Requena J., Whittembury J., Tiffert T., Eisner D. A., Mullins L. J. The influence of chemical agents on the level of ionized [Ca2+] in squid axons. J Gen Physiol. 1985 Jun;85(6):789–804. doi: 10.1085/jgp.85.6.789. [DOI] [PMC free article] [PubMed] [Google Scholar]
- Reuter H., Seitz N. The dependence of calcium efflux from cardiac muscle on temperature and external ion composition. J Physiol. 1968 Mar;195(2):451–470. doi: 10.1113/jphysiol.1968.sp008467. [DOI] [PMC free article] [PubMed] [Google Scholar]
- WILKINSON G. N. Statistical estimations in enzyme kinetics. Biochem J. 1961 Aug;80:324–332. doi: 10.1042/bj0800324. [DOI] [PMC free article] [PubMed] [Google Scholar]