Abstract
The mechanism of long-term anoxic damage in brain tissue is investigated using the rat hippocampal slice as a model system. The effects of short durations of anoxia on subsequent transmission through two neural pathways are studied. 10 min of anoxia irreversibly abolishes transmission between the perforant path and the dentate granule cells while only 7 min of anoxia irreversibly abolishes transmission between the Schaeffer collaterals and the CA1 pyramidal cells. We examine the involvement of Ca2+ in this irreversible transmission damage and, also, the differential sensitivities of the dentate gyrus and CA1 regions. Substitution of a buffer containing 0 Ca2+ and 10 mM-Mg2+ during the anoxic period substantially improves the recovery of synaptic transmission in both regions of the slice. Dentate gyrus transmission recovers completely after 20 min of anoxia and CA1 transmission survives 10 min of anoxia. These results suggest that Ca2+ influx during anoxia may be an important cause of the long-term damage. The uptake of 45Ca2+ into the intracellular space of the slice is increased during anoxia. This effect is approximately twice as large in CA1 as in the dentate gyrus. Thus, in the dentate gyrus the calculated exchangeable pool of Ca2+ is increased 30% by anoxia and in the CA1 it is increased by 70%. Two incubating conditions which decrease the amount of 45Ca2+ uptake during anoxia protect transmission against long-term damage. (a) Pre-incubation of the slices with 25 mM-creatine elevates tissue phosphocreatine and attenuates the fall in adenosine 5'-triphosphate (ATP) during anoxia. This is associated with partial protection against transmission damage and an approximate 50% attenuation of the anoxic uptake of 45Ca2+. (b) Inclusion of 2 mM-cobalt in the buffer reduces the normoxic uptake of 45Ca2+ so that the uptake during anoxia is no greater than normoxic uptake in the absence of cobalt. This is associated with a complete protection against long-term transmission damage following 10 min of anoxia in the dentate gyrus. A kinetic analysis of the 45Ca2+ uptake shows that the anoxic uptake results primarily from inhibition of the unidirectional efflux of Ca2+ from the cells; there is no calculable increase in the undirectional influx. This suggests that anoxia increases Ca2+ uptake by inhibiting one or more Ca2+-extrusion processes and not by opening depolarization-sensitive Ca2+ channels.(ABSTRACT TRUNCATED AT 400 WORDS)
Full text
PDF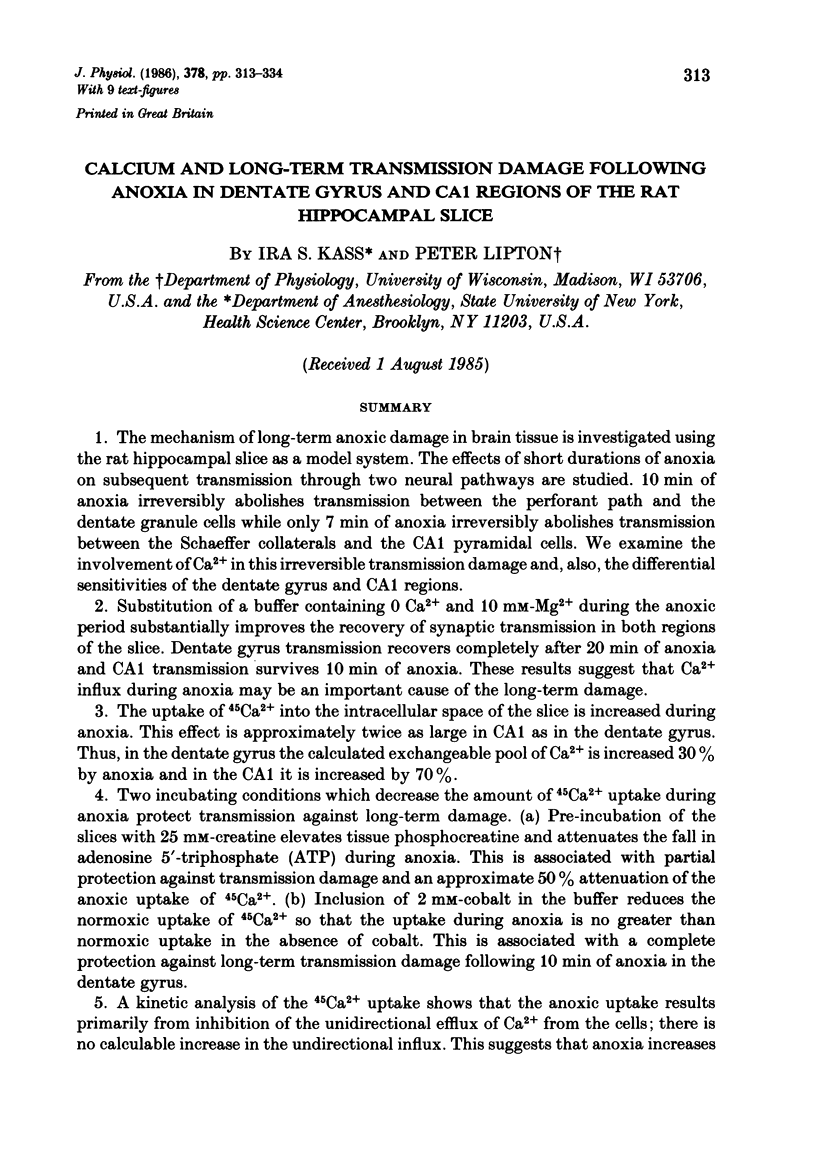
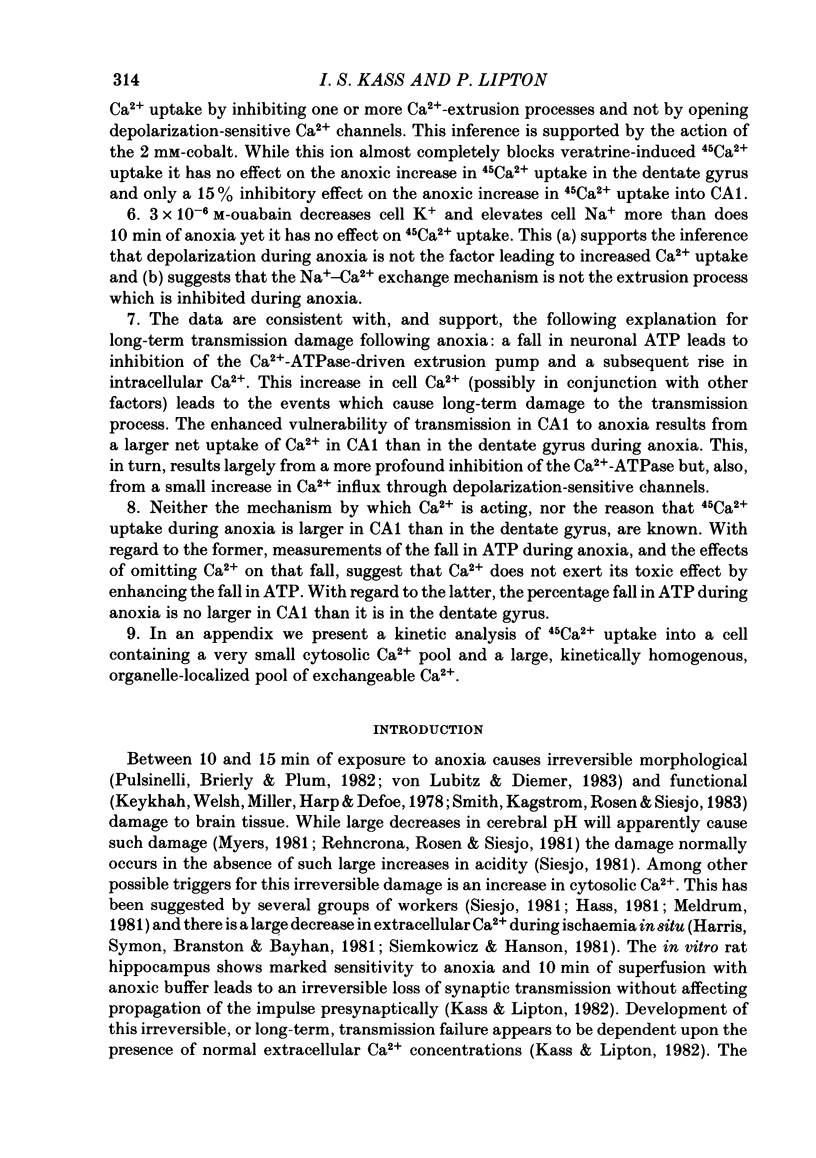
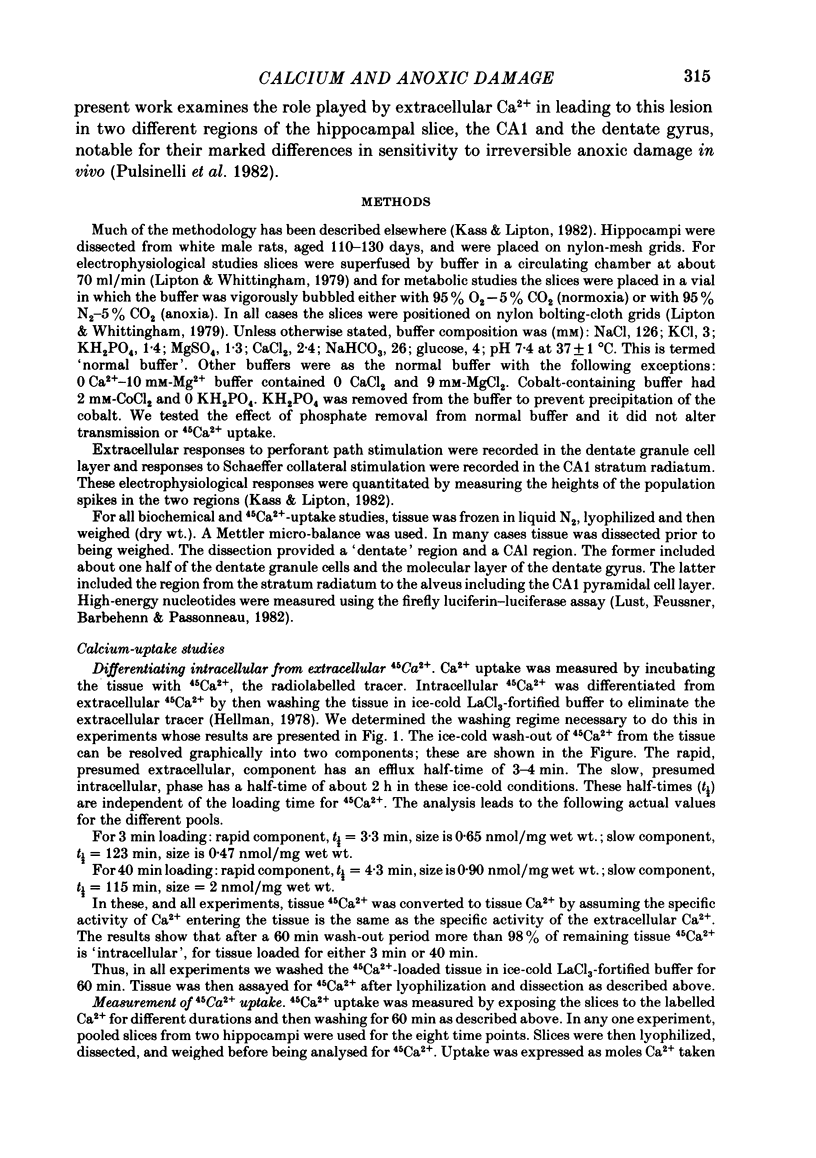
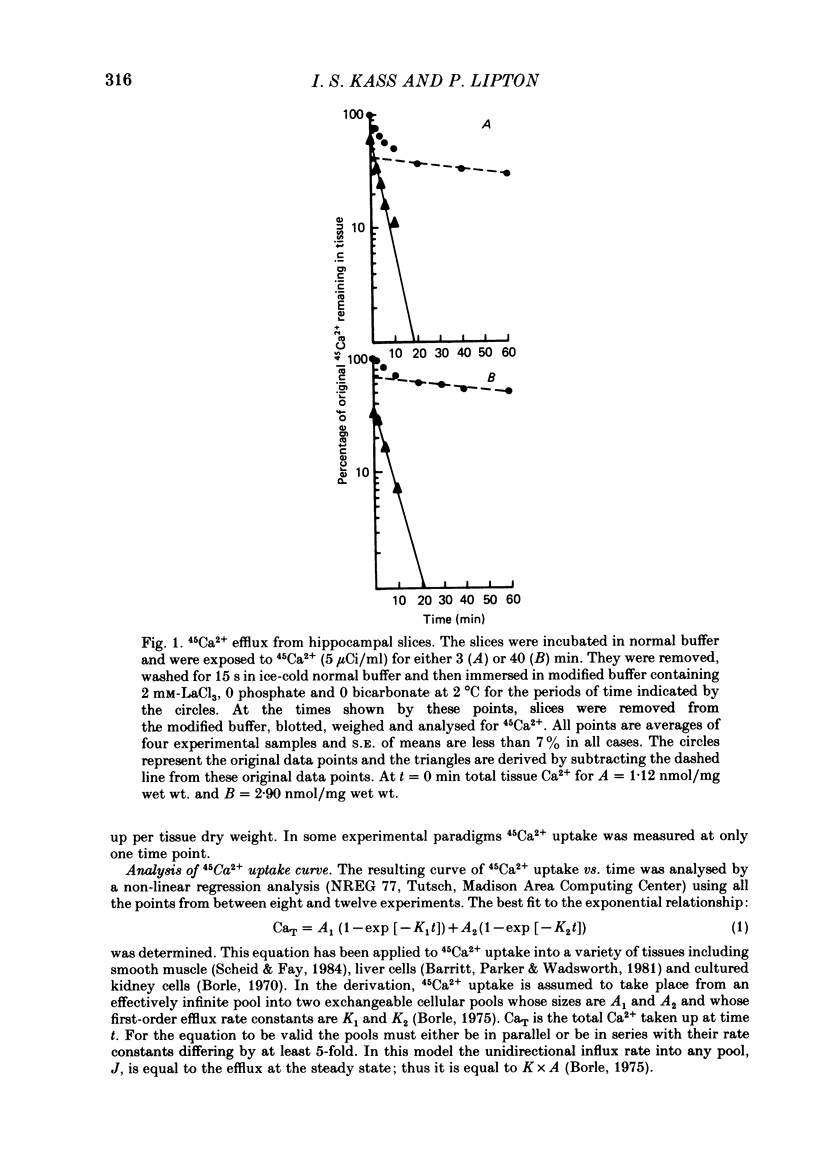
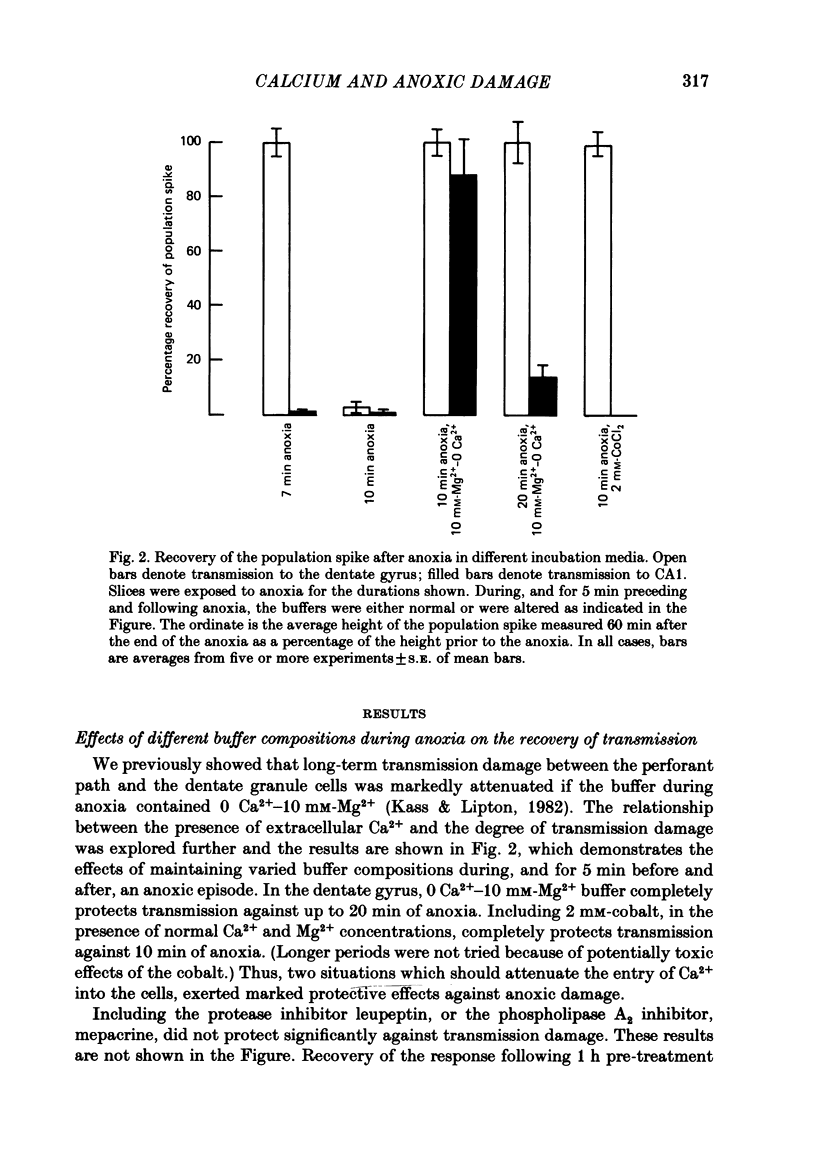
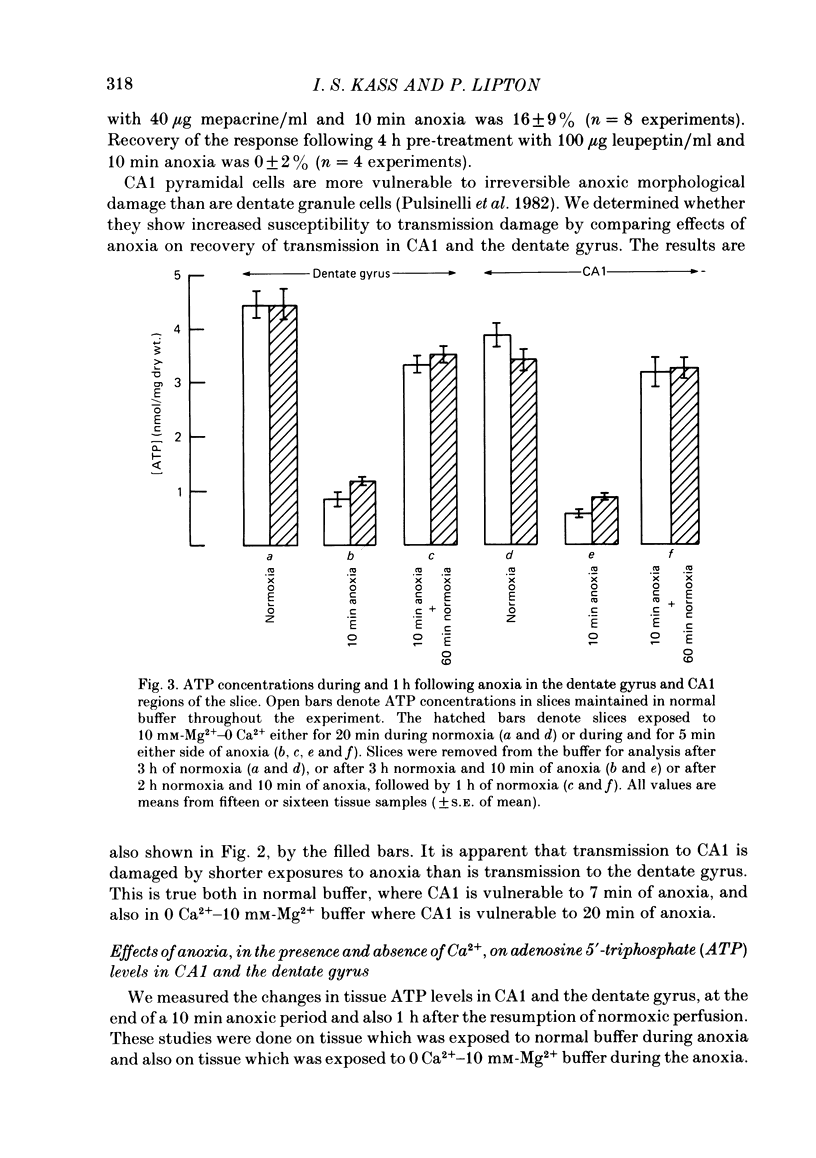
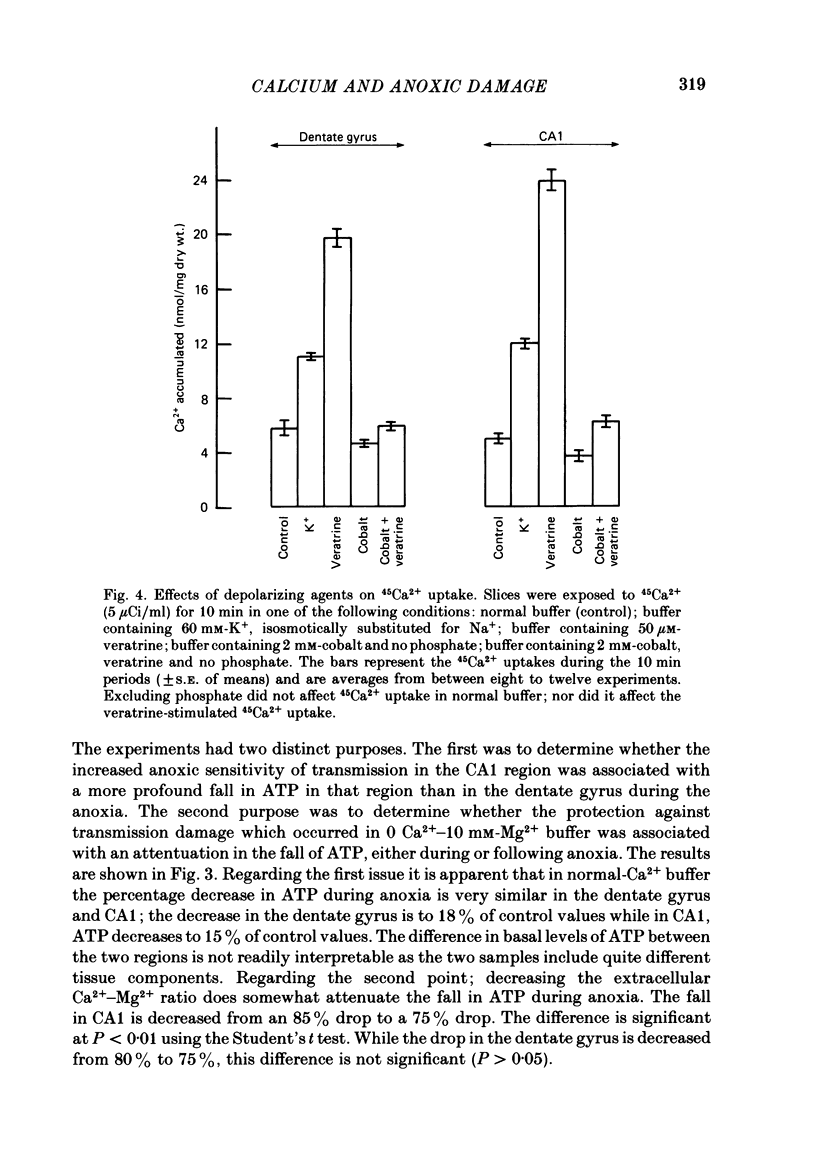
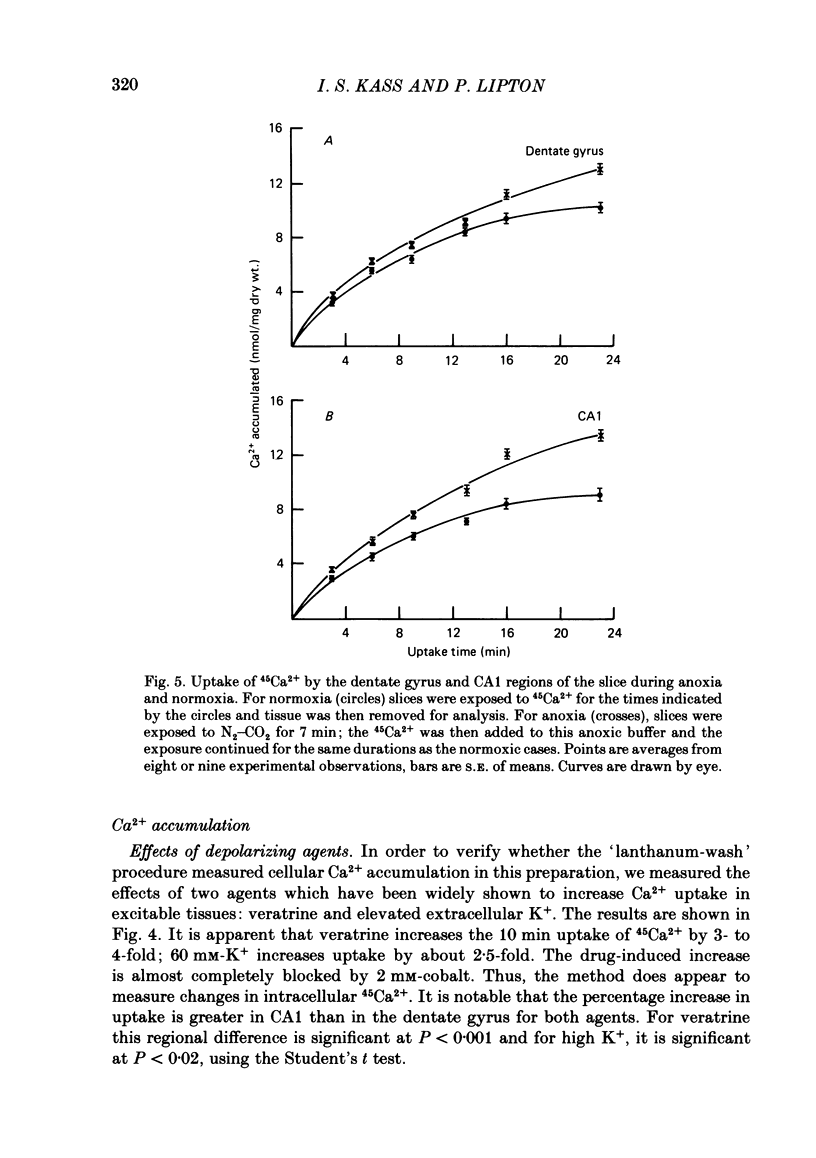
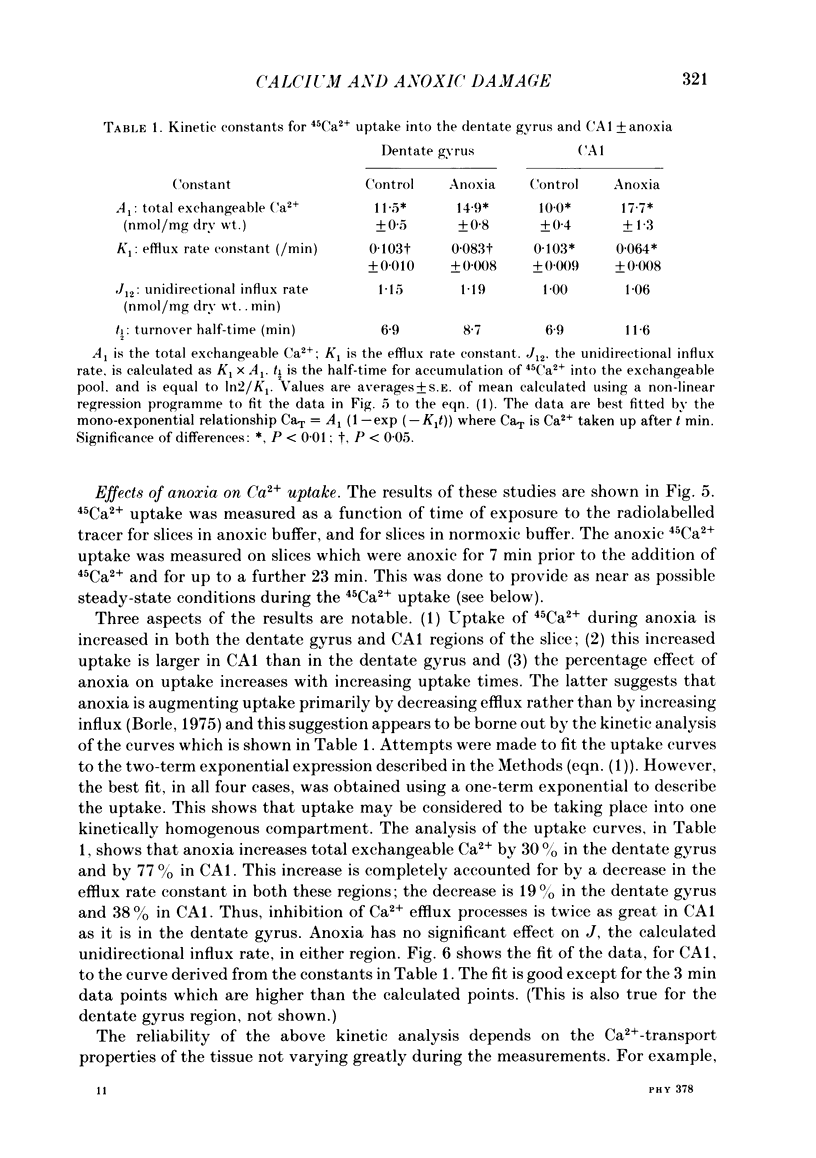
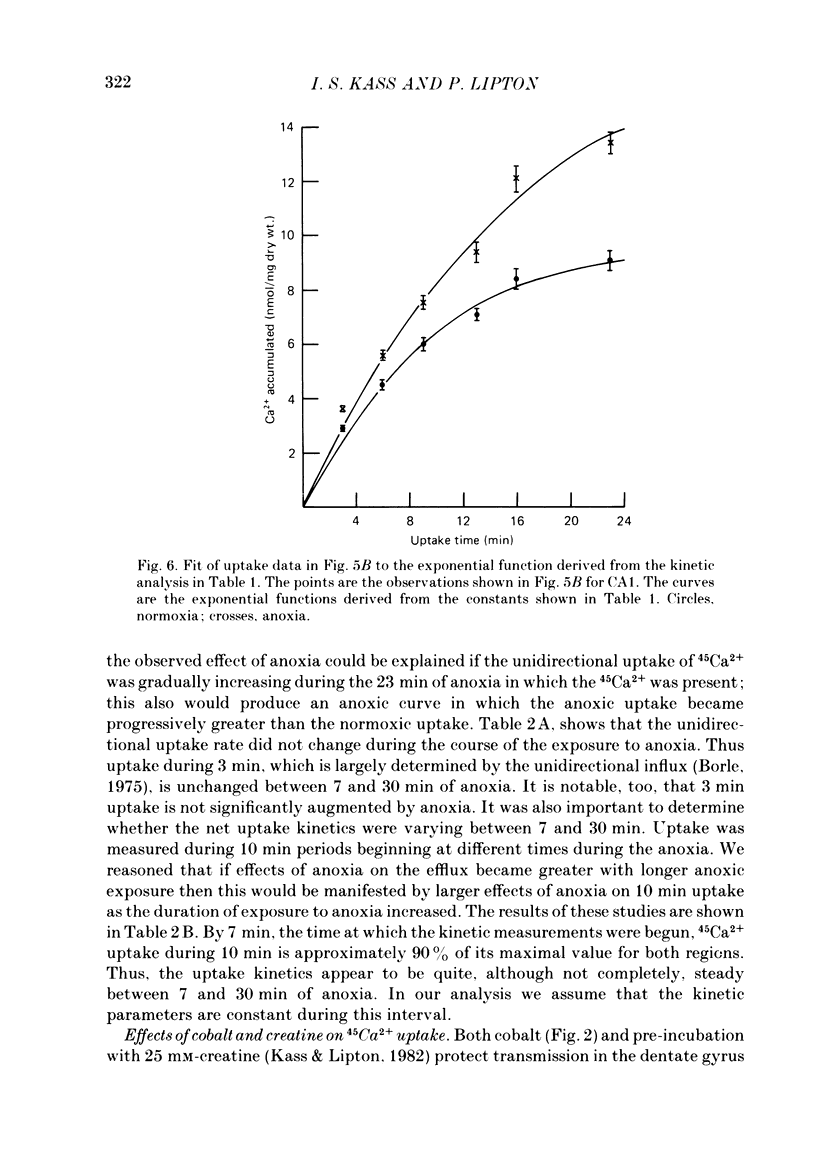
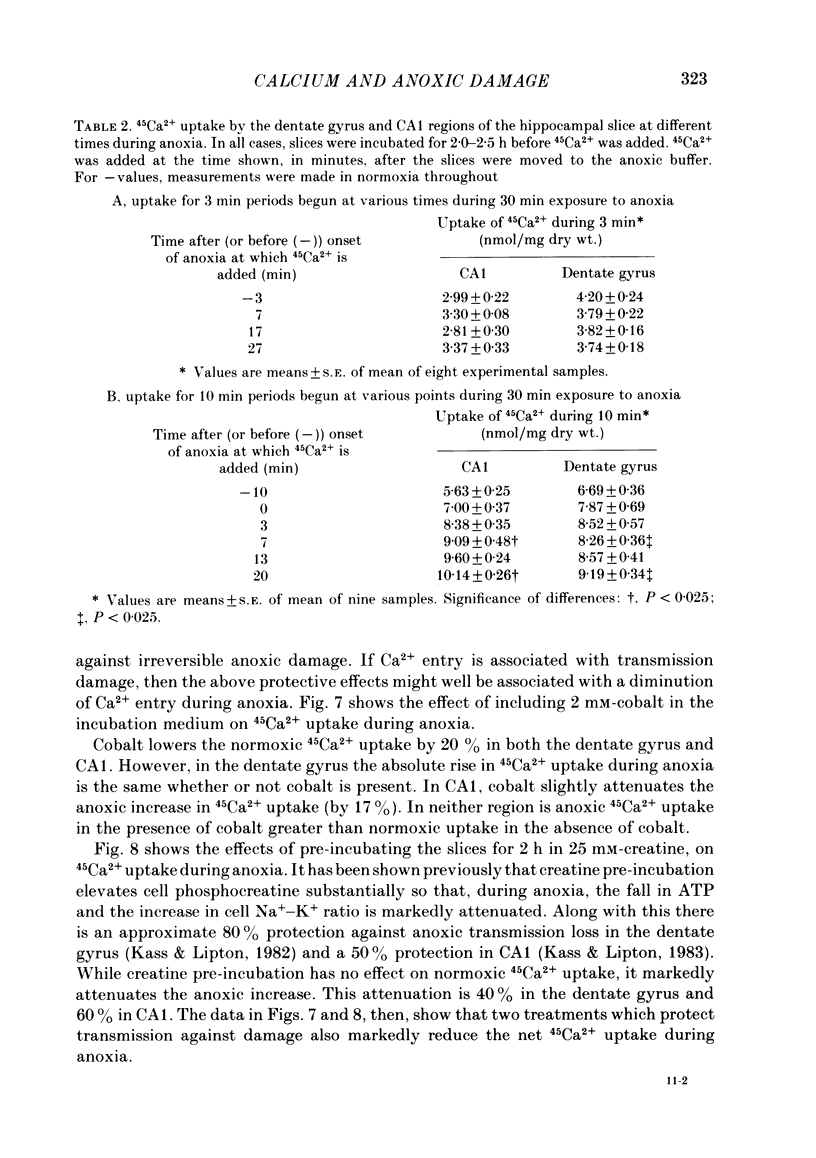
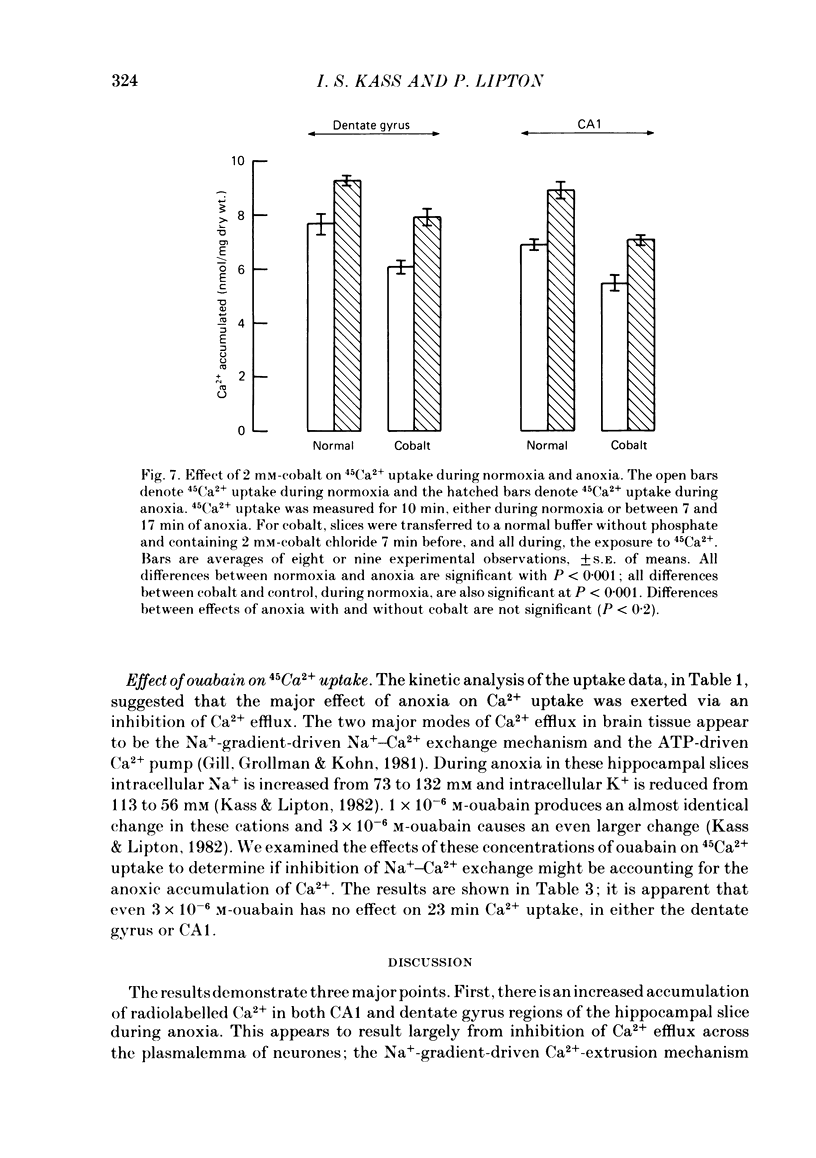
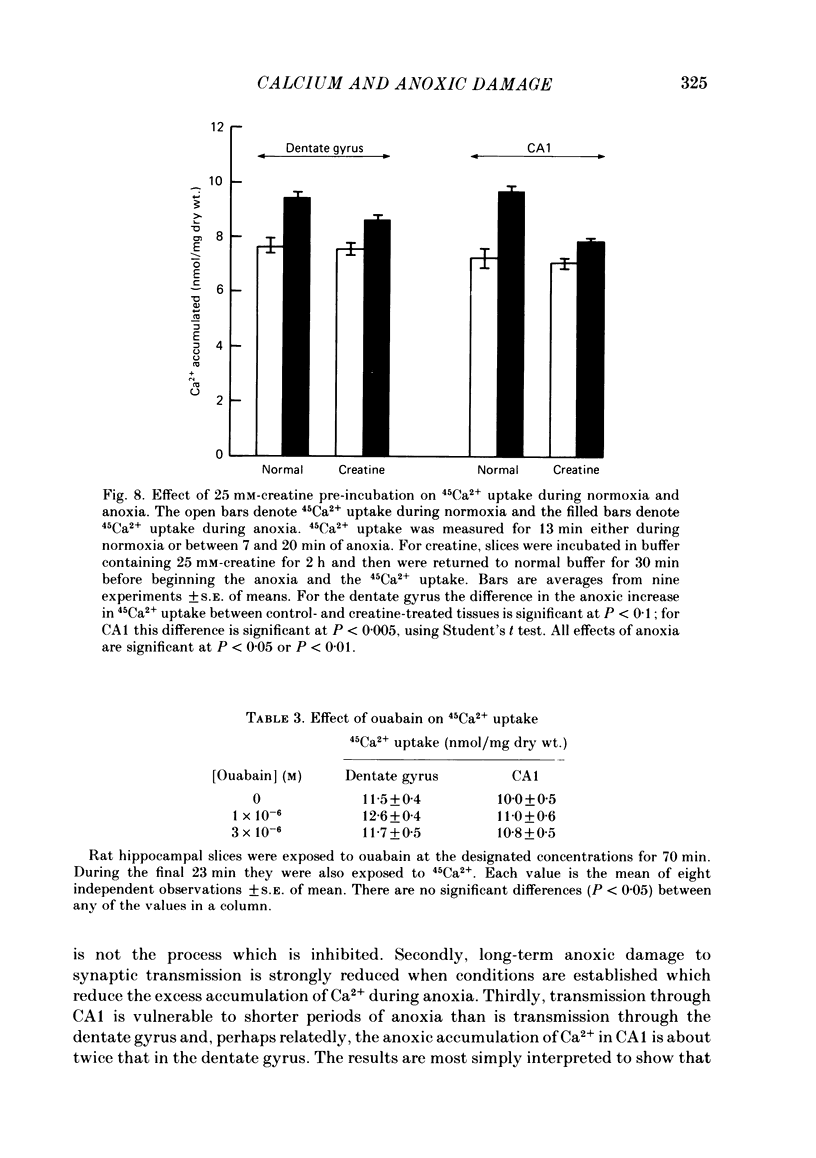
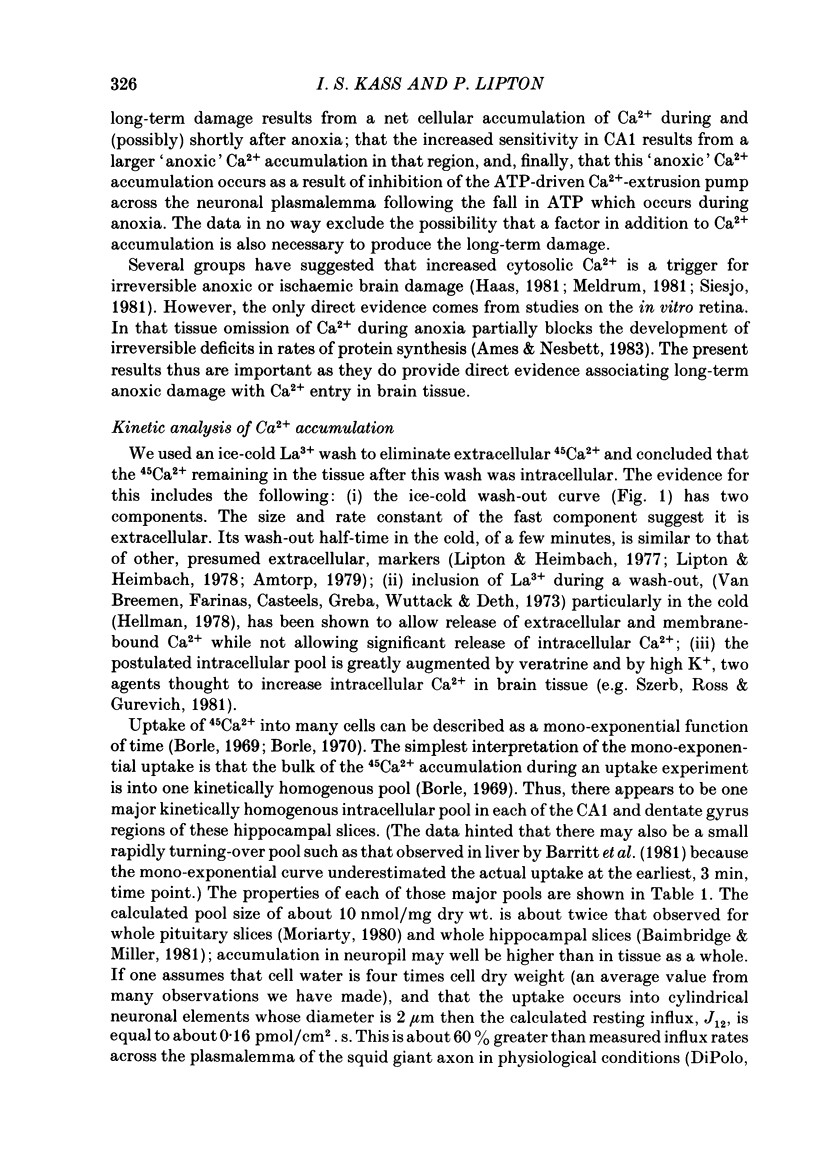
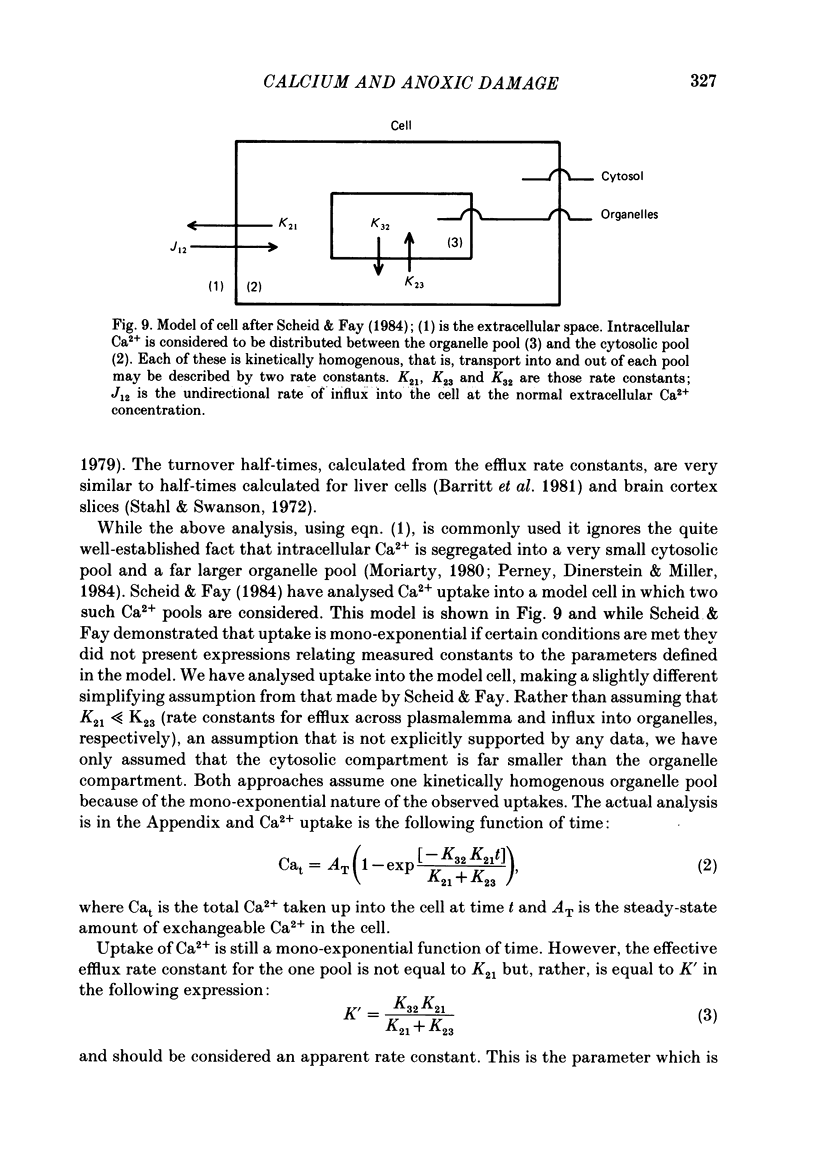
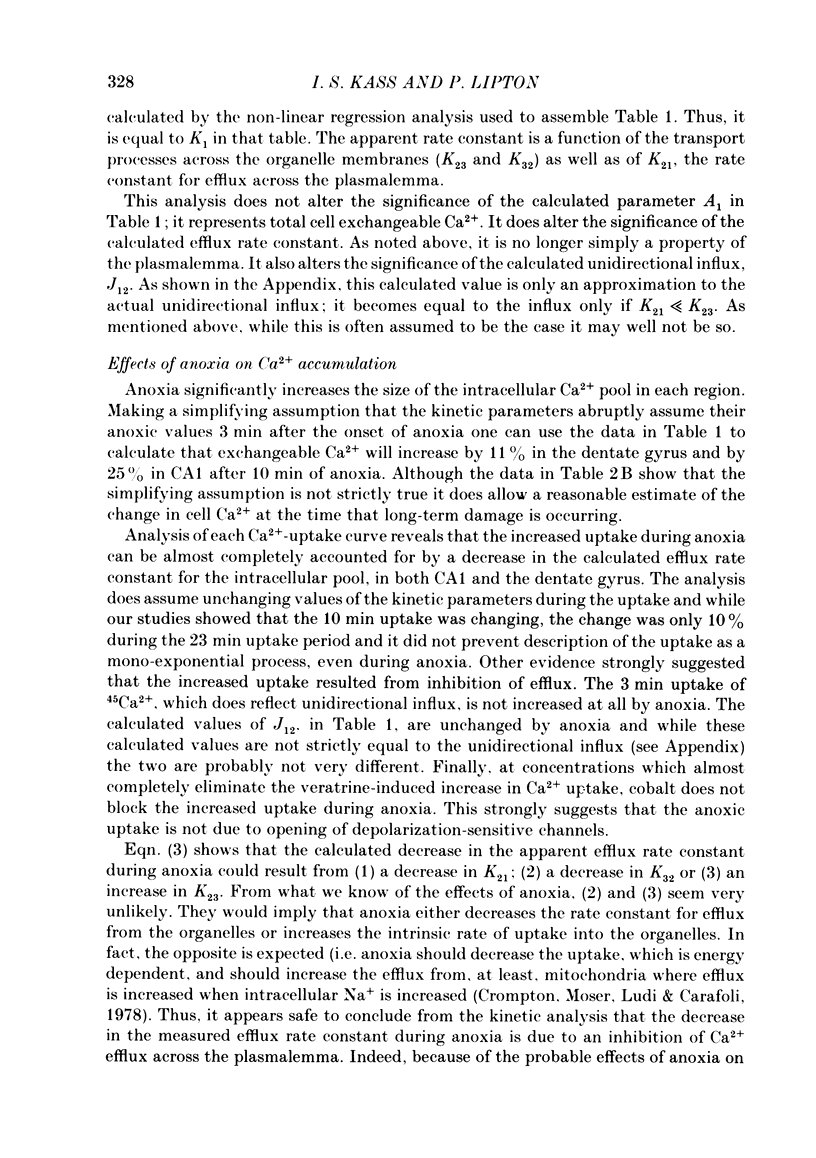
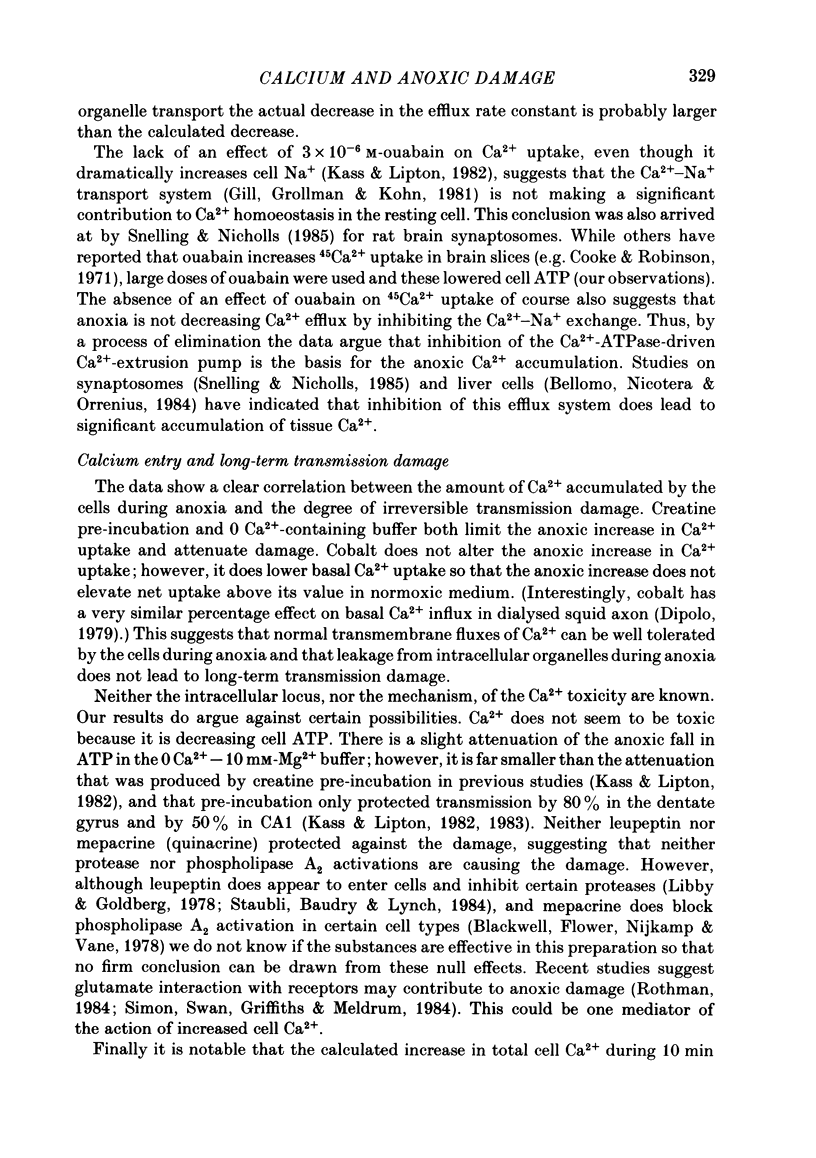
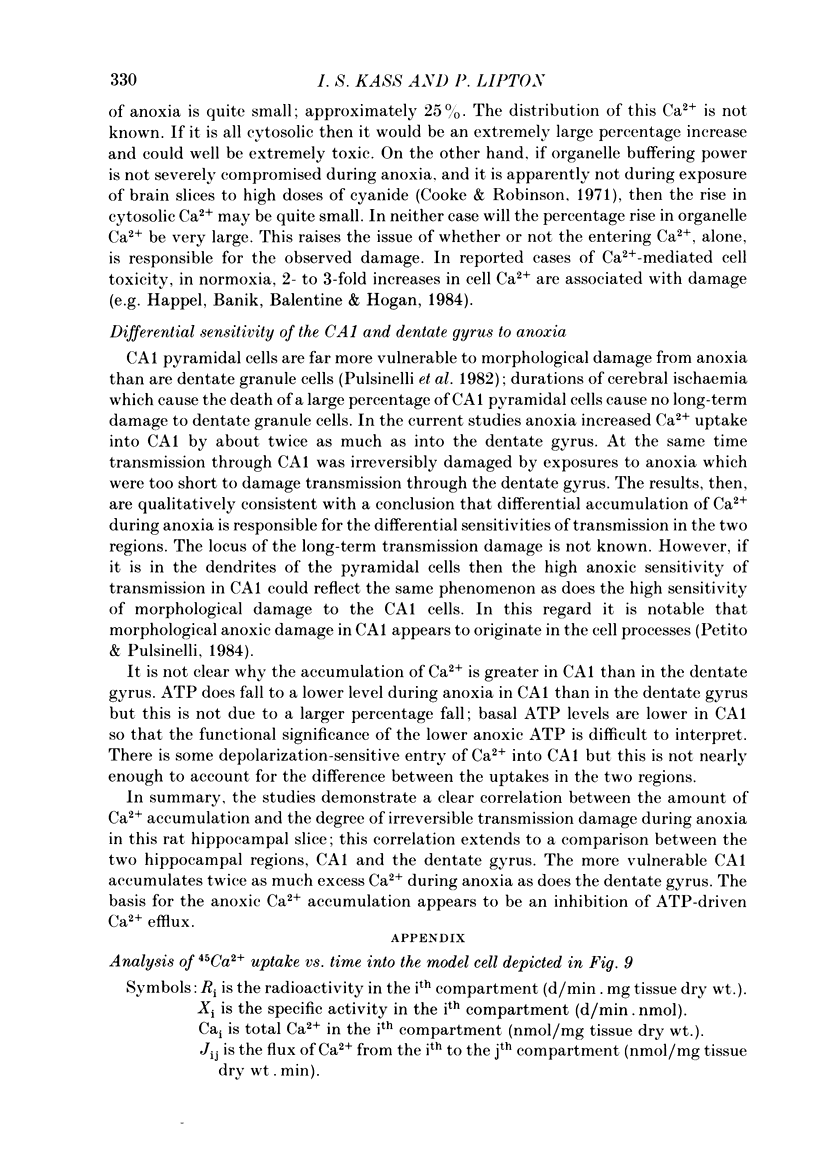
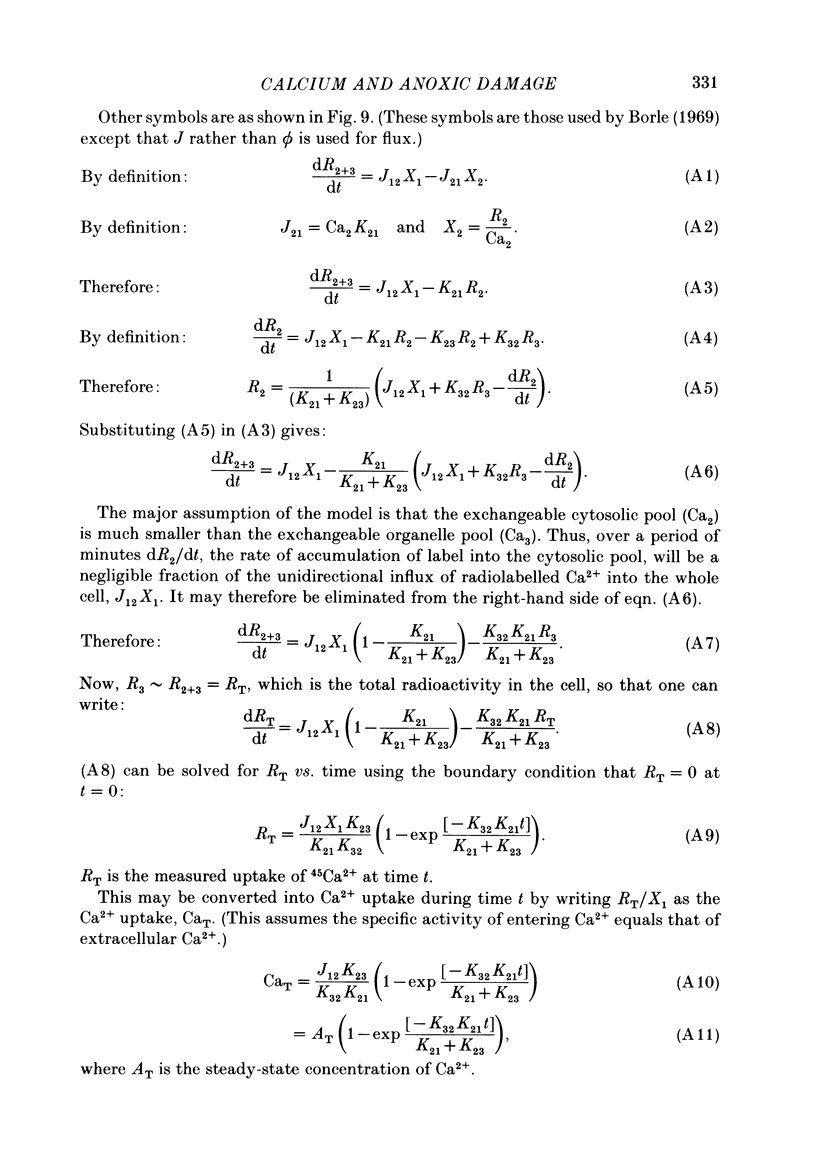
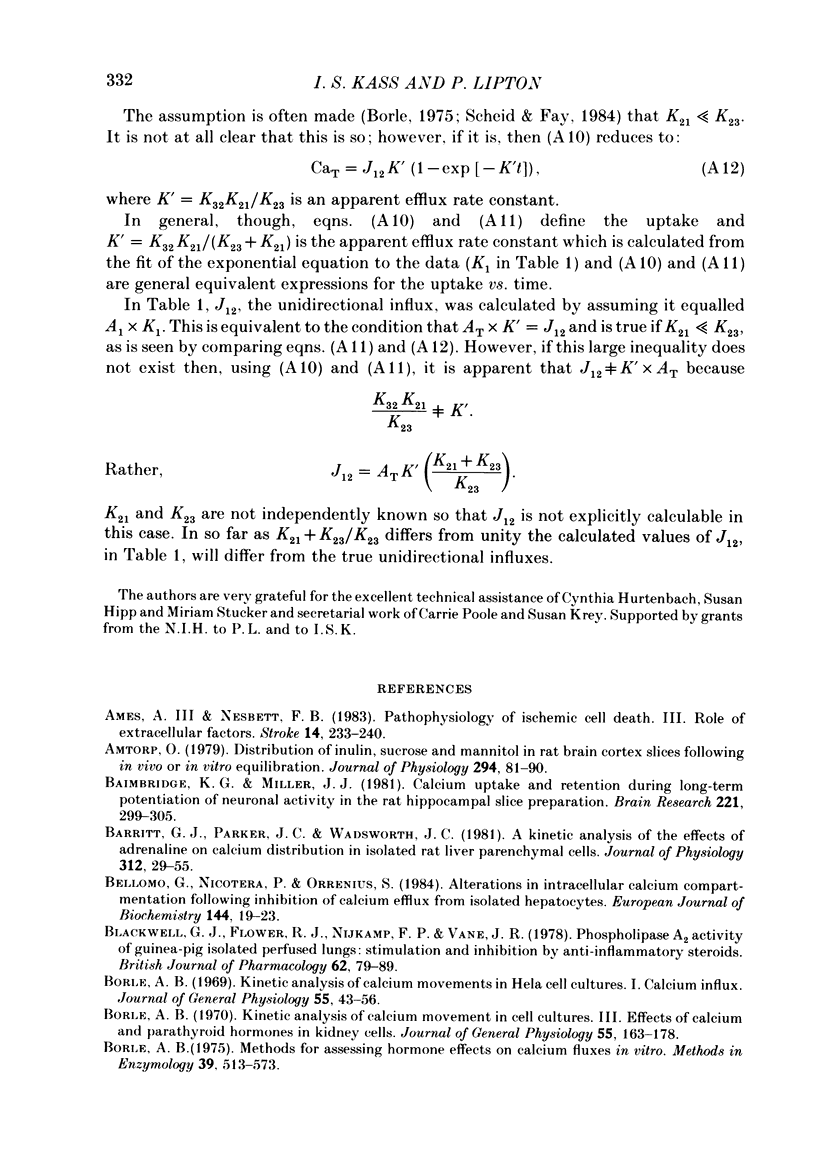
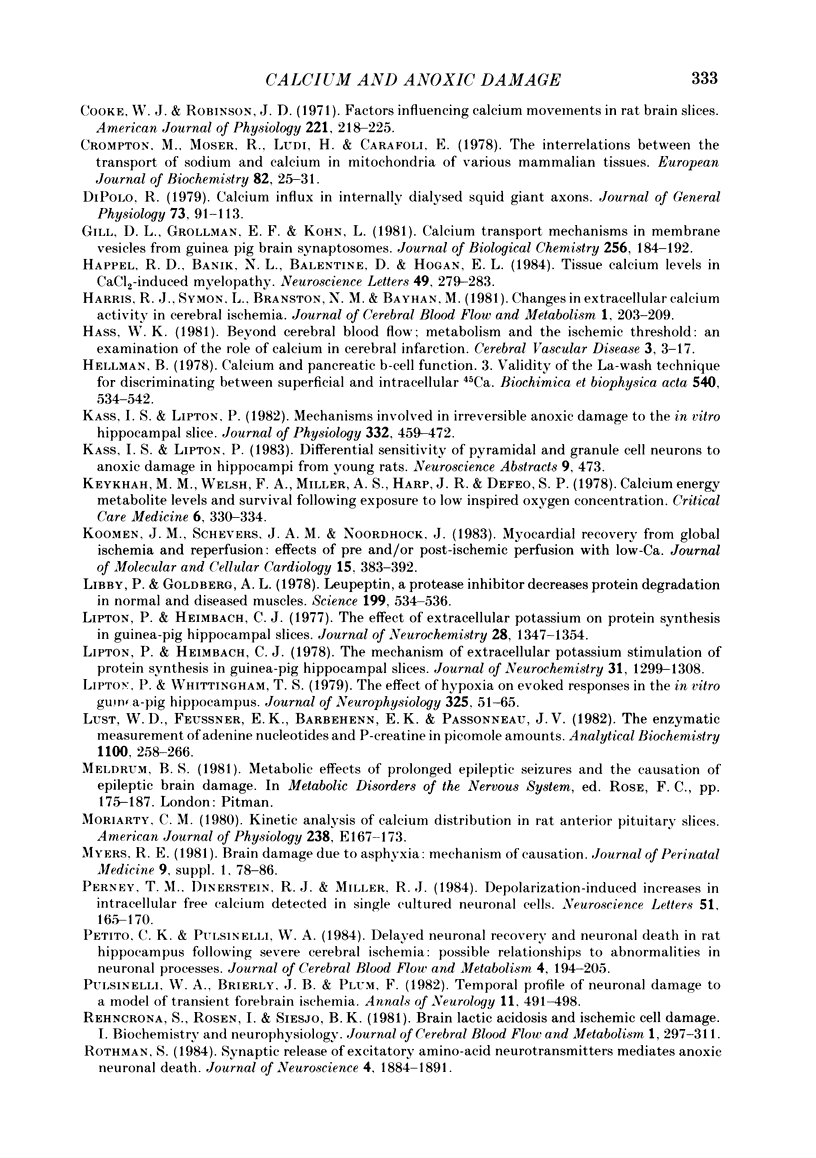
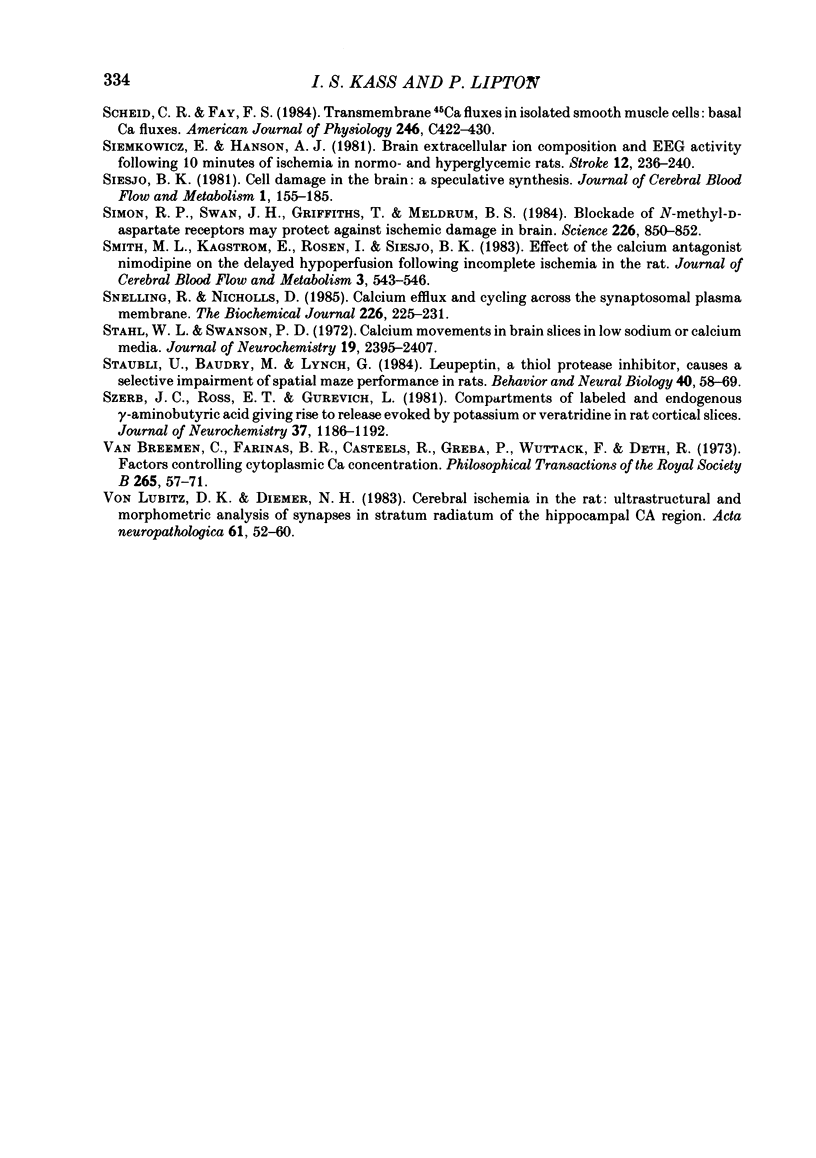
Selected References
These references are in PubMed. This may not be the complete list of references from this article.
- Ames A., 3rd, Nesbett F. B. Pathophysiology of ischemic cell death: III. Role of extracellular factors. Stroke. 1983 Mar-Apr;14(2):233–240. doi: 10.1161/01.str.14.2.233. [DOI] [PubMed] [Google Scholar]
- Amtorp O. Distribution of inulin, sucrose and mannitol in rat brain cortex slices following in vivo or in vitro equilibration. J Physiol. 1979 Sep;294:81–90. doi: 10.1113/jphysiol.1979.sp012916. [DOI] [PMC free article] [PubMed] [Google Scholar]
- Baimbridge K. G., Miller J. J. Calcium uptake and retention during long-term potentiation of neuronal activity in the rat hippocampal slice preparation. Brain Res. 1981 Sep 28;221(2):299–305. doi: 10.1016/0006-8993(81)90779-4. [DOI] [PubMed] [Google Scholar]
- Barritt G. J., Parker J. C., Wadsworth J. C. A kinetic analysis of the effects of adrenaline on calcium distribution in isolated rat liver parenchymal cells. J Physiol. 1981 Mar;312:29–55. doi: 10.1113/jphysiol.1981.sp013614. [DOI] [PMC free article] [PubMed] [Google Scholar]
- Bellomo G., Nicotera P., Orrenius S. Alterations in intracellular calcium compartmentation following inhibition of calcium efflux from isolated hepatocytes. Eur J Biochem. 1984 Oct 1;144(1):19–23. doi: 10.1111/j.1432-1033.1984.tb08425.x. [DOI] [PubMed] [Google Scholar]
- Blackwell G. J., Flower R. J., Nijkamp F. P., Vane J. R. Phospholipase A2 activity of guinea-pig isolated perfused lungs: stimulation, and inhibition by anti-inflammatory steroids. Br J Pharmacol. 1978 Jan;62(1):79–89. doi: 10.1111/j.1476-5381.1978.tb07009.x. [DOI] [PMC free article] [PubMed] [Google Scholar]
- Borle A. B. Kinetic analyses of calcium movements in cell cultures. 3. Effects of calcium and parathyroid hormone in kidney cells. J Gen Physiol. 1970 Feb;55(2):163–186. doi: 10.1085/jgp.55.2.163. [DOI] [PMC free article] [PubMed] [Google Scholar]
- Borle A. B. Methods for assessing hormone effects on calcium fluxes in vitro. Methods Enzymol. 1975;39:513–573. doi: 10.1016/s0076-6879(75)39046-0. [DOI] [PubMed] [Google Scholar]
- Cooke W. J., Robinson J. D. Factors influencing calcium movements in rat brain slices. Am J Physiol. 1971 Jul;221(1):218–225. doi: 10.1152/ajplegacy.1971.221.1.218. [DOI] [PubMed] [Google Scholar]
- Crompton M., Moser R., Lüdi H., Carafoli E. The interrelations between the transport of sodium and calcium in mitochondria of various mammalian tissues. Eur J Biochem. 1978 Jan 2;82(1):25–31. doi: 10.1111/j.1432-1033.1978.tb11993.x. [DOI] [PubMed] [Google Scholar]
- DiPolo R. Calcium influx in internally dialyzed squid giant axons. J Gen Physiol. 1979 Jan;73(1):91–113. doi: 10.1085/jgp.73.1.91. [DOI] [PMC free article] [PubMed] [Google Scholar]
- Gill D. L., Grollman E. F., Kohn L. D. Calcium transport mechanisms in membrane vesicles from guinea pig brain synaptosomes. J Biol Chem. 1981 Jan 10;256(1):184–192. [PubMed] [Google Scholar]
- Happel R. D., Banik N. L., Balentine J. D., Hogan E. L. Tissue calcium levels in CaCl2-induced myelopathy. Neurosci Lett. 1984 Aug 31;49(3):279–283. doi: 10.1016/0304-3940(84)90302-1. [DOI] [PubMed] [Google Scholar]
- Harris R. J., Symon L., Branston N. M., Bayhan M. Changes in extracellular calcium activity in cerebral ischaemia. J Cereb Blood Flow Metab. 1981;1(2):203–209. doi: 10.1038/jcbfm.1981.21. [DOI] [PubMed] [Google Scholar]
- Kass I. S., Lipton P. Mechanisms involved in irreversible anoxic damage to the in vitro rat hippocampal slice. J Physiol. 1982 Nov;332:459–472. doi: 10.1113/jphysiol.1982.sp014424. [DOI] [PMC free article] [PubMed] [Google Scholar]
- Keykhah M. M., Welsh F. A., Miller A. S., Harp J. R., DeFeo S. P. Cerebral energy metabolite levels and survival following exposure to low inspired oxygen concentration. Crit Care Med. 1978 Sep-Oct;6(5):330–334. doi: 10.1097/00003246-197809000-00007. [DOI] [PubMed] [Google Scholar]
- Koomen J. M., Schevers J. A., Noordhoek J. Myocardial recovery from global ischemia and reperfusion: effects of pre- and/or post-ischemic perfusion with low-Ca2+. J Mol Cell Cardiol. 1983 Jun;15(6):383–392. doi: 10.1016/0022-2828(83)90322-x. [DOI] [PubMed] [Google Scholar]
- Libby P., Goldberg A. L. Leupeptin, a protease inhibitor, decreases protein degradation in normal and diseased muscles. Science. 1978 Feb 3;199(4328):534–536. doi: 10.1126/science.622552. [DOI] [PubMed] [Google Scholar]
- Lipton P., Heimbach C. J. Mechanism of extracellular potassium stimulation of protein synthesis in the in vitro hippocampus. J Neurochem. 1978 Nov;31(5):1299–1307. doi: 10.1111/j.1471-4159.1978.tb06255.x. [DOI] [PubMed] [Google Scholar]
- Lipton P., Heimbach C. J. The effect of extracellular potassium concentration on protein synthesis in guinea-pig hippocampal slices. J Neurochem. 1977 Jun;28(6):1347–1354. doi: 10.1111/j.1471-4159.1977.tb12330.x. [DOI] [PubMed] [Google Scholar]
- Lipton P., Whittingham T. S. Reduced ATP concentration as a basis for synaptic transmission failure during hypoxia in the in vitro guinea-pig hippocampus. J Physiol. 1982 Apr;325:51–65. doi: 10.1113/jphysiol.1982.sp014135. [DOI] [PMC free article] [PubMed] [Google Scholar]
- Moriarty C. M. Kinetic analysis of calcium distribution in rat anterior pituitary slices. Am J Physiol. 1980 Feb;238(2):E167–E173. doi: 10.1152/ajpendo.1980.238.2.E167. [DOI] [PubMed] [Google Scholar]
- Perney T. M., Dinerstein R. J., Miller R. J. Depolarization-induced increases in intracellular free calcium detected in single cultured neuronal cells. Neurosci Lett. 1984 Oct 12;51(2):165–170. doi: 10.1016/0304-3940(84)90545-7. [DOI] [PubMed] [Google Scholar]
- Petito C. K., Pulsinelli W. A. Delayed neuronal recovery and neuronal death in rat hippocampus following severe cerebral ischemia: possible relationship to abnormalities in neuronal processes. J Cereb Blood Flow Metab. 1984 Jun;4(2):194–205. doi: 10.1038/jcbfm.1984.28. [DOI] [PubMed] [Google Scholar]
- Pulsinelli W. A., Brierley J. B., Plum F. Temporal profile of neuronal damage in a model of transient forebrain ischemia. Ann Neurol. 1982 May;11(5):491–498. doi: 10.1002/ana.410110509. [DOI] [PubMed] [Google Scholar]
- Rehncrona S., Rosén I., Siesjö B. K. Brain lactic acidosis and ischemic cell damage: 1. Biochemistry and neurophysiology. J Cereb Blood Flow Metab. 1981;1(3):297–311. doi: 10.1038/jcbfm.1981.34. [DOI] [PubMed] [Google Scholar]
- Rothman S. Synaptic release of excitatory amino acid neurotransmitter mediates anoxic neuronal death. J Neurosci. 1984 Jul;4(7):1884–1891. doi: 10.1523/JNEUROSCI.04-07-01884.1984. [DOI] [PMC free article] [PubMed] [Google Scholar]
- Scheid C. R., Fay F. S. Transmembrane 45Ca fluxes in isolated smooth muscle cells: basal Ca2+ fluxes. Am J Physiol. 1984 May;246(5 Pt 1):C422–C430. doi: 10.1152/ajpcell.1984.246.5.C422. [DOI] [PubMed] [Google Scholar]
- Siemkowicz E., Hansen A. J. Brain extracellular ion composition and EEG activity following 10 minutes ischemia in normo- and hyperglycemic rats. Stroke. 1981 Mar-Apr;12(2):236–240. doi: 10.1161/01.str.12.2.236. [DOI] [PubMed] [Google Scholar]
- Siesjö B. K. Cell damage in the brain: a speculative synthesis. J Cereb Blood Flow Metab. 1981;1(2):155–185. doi: 10.1038/jcbfm.1981.18. [DOI] [PubMed] [Google Scholar]
- Simon R. P., Swan J. H., Griffiths T., Meldrum B. S. Blockade of N-methyl-D-aspartate receptors may protect against ischemic damage in the brain. Science. 1984 Nov 16;226(4676):850–852. doi: 10.1126/science.6093256. [DOI] [PubMed] [Google Scholar]
- Smith M. L., Kågström E., Rosén I., Siesjö B. K. Effect of the calcium antagonist nimodipine on the delayed hypoperfusion following incomplete ischemia in the rat. J Cereb Blood Flow Metab. 1983 Dec;3(4):543–546. doi: 10.1038/jcbfm.1983.83. [DOI] [PubMed] [Google Scholar]
- Snelling R., Nicholls D. Calcium efflux and cycling across the synaptosomal plasma membrane. Biochem J. 1985 Feb 15;226(1):225–231. doi: 10.1042/bj2260225. [DOI] [PMC free article] [PubMed] [Google Scholar]
- Stahl W. L., Swanson P. D. Calcium movements in brain slices in low sodium or calcium media. J Neurochem. 1972 Oct;19(10):2395–2407. doi: 10.1111/j.1471-4159.1972.tb01294.x. [DOI] [PubMed] [Google Scholar]
- Stäubli U., Baudry M., Lynch G. Leupeptin, a thiol proteinase inhibitor, causes a selective impairment of spatial maze performance in rats. Behav Neural Biol. 1984 Jan;40(1):58–69. doi: 10.1016/s0163-1047(84)90170-5. [DOI] [PubMed] [Google Scholar]
- Szerb J. C., Ross T. E., Gurevich L. Compartments of labeled and endogenous gamma-aminobutyric acid giving rise to release evoked by potassium or veratridine in rat cortical slices. J Neurochem. 1981 Nov;37(5):1186–1192. doi: 10.1111/j.1471-4159.1981.tb04669.x. [DOI] [PubMed] [Google Scholar]
- van Breemen C., Farinas B. R., Casteels R., Gerba P., Wuytack F., Deth R. Factors controlling cytoplasmic Ca 2+ concentration. Philos Trans R Soc Lond B Biol Sci. 1973 Mar 15;265(867):57–71. doi: 10.1098/rstb.1973.0009. [DOI] [PubMed] [Google Scholar]
- von Lubitz D. K., Diemer N. H. Cerebral ischemia in the rat: ultrastructural and morphometric analysis of synapses in stratum radiatum of the hippocampal CA-1 region. Acta Neuropathol. 1983;61(1):52–60. doi: 10.1007/BF00688386. [DOI] [PubMed] [Google Scholar]