Abstract
Discharges of mossy fibres were recorded from the cerebellar flocculus of monkeys trained to fixate a small visual target and to track the target when it moved slowly. The experimental paradigms used were designed to study neural responses to retinal-slip velocity, eye velocity, or head velocity, individually or in combination. Among 485 mossy-fibre units recorded from the flocculus, sixty-four units (or 13%) responded to movement of the visual stimulus in the horizontal plane. Two distinct groups of visual mossy fibres were found: they were designated 'visual units' (thirty-nine/sixty-four units or 61%) and 'visuomotor units' (twenty-five/sixty-four units or 39%). The visual units responded exclusively to the retinal-slip velocity. Stationary fixation was necessary for clear cyclic modulation of activity. Their responses declined when the retinal-slip velocity was reduced by eye movements in the same direction. The responses of the visual units were directionally selective and lagged behind the occurrence of 'turnabouts' (changes in direction of stimulus movement) and their peak discharges also lagged the occurrence of peak velocity. Each visual unit had a limited range of velocity sensitivity; in some units the range covered the velocity range of smooth-pursuit eye movements. The visuomotor units had visual receptive fields in the peripheral retina (outside of the central 10 deg); they received also oculomotor and vestibular signals. When the head was stationary, the visuomotor units responded to the target velocity (or visual stimulus velocity) which is the algebraic sum of the retinal-slip velocity and the eye velocity. Their responses reflected the retinal-slip velocity during stationary fixation and the eye velocity during smooth-pursuit eye movements. The responses to stimulus movements were, therefore, almost identical regardless of whether the eyes remained stationary or moved with the stimulus. In response to sinusoidal stimulus movements, the responses of the visuomotor units frequently preceded the stimulus velocity, and the phase lead relative to the velocity curve increased when the frequency of sinusoidal movements was increased. This reflected a relatively constant lead of neural discharges (circa 125 ms) during various frequencies. When the head was moved, the responses of the visuomotor units were dominated by the head velocity, and discharges in response either to the retinal-slip velocity or to the eye velocity (both in the direction opposite to the head velocity) were occluded.(ABSTRACT TRUNCATED AT 400 WORDS)
Full text
PDF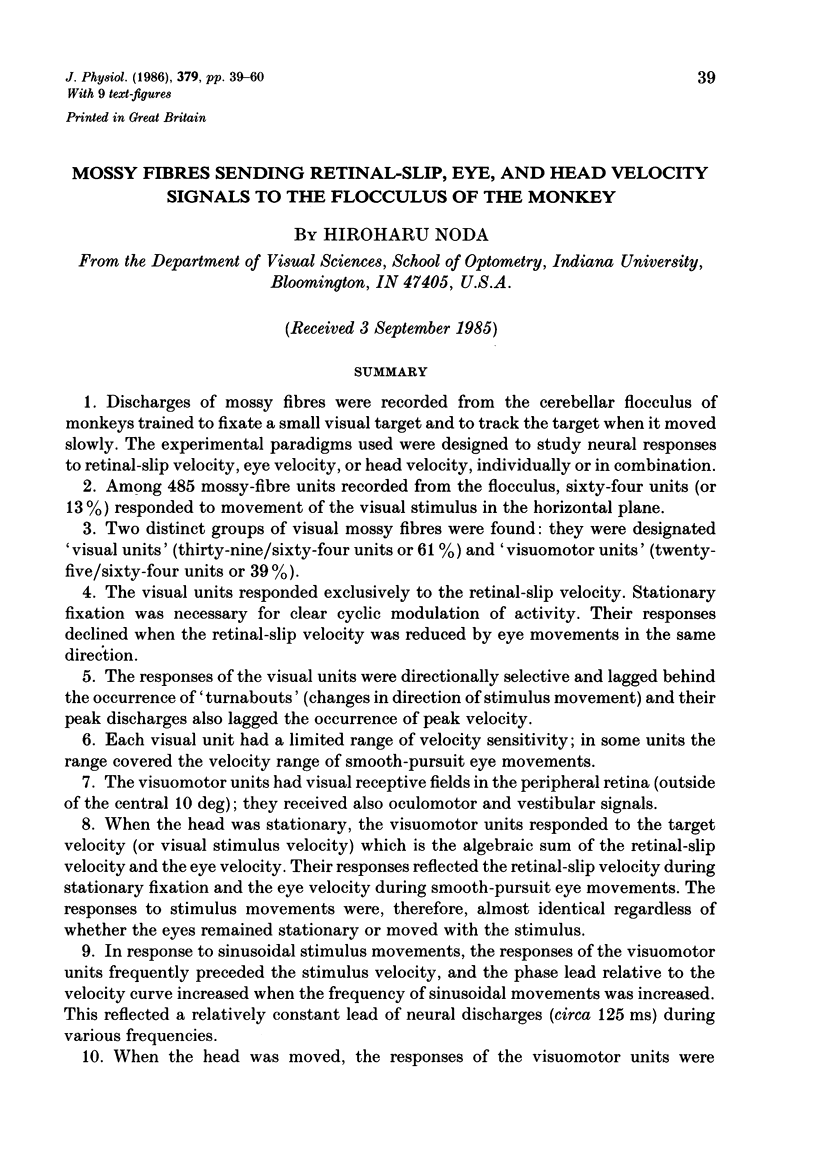
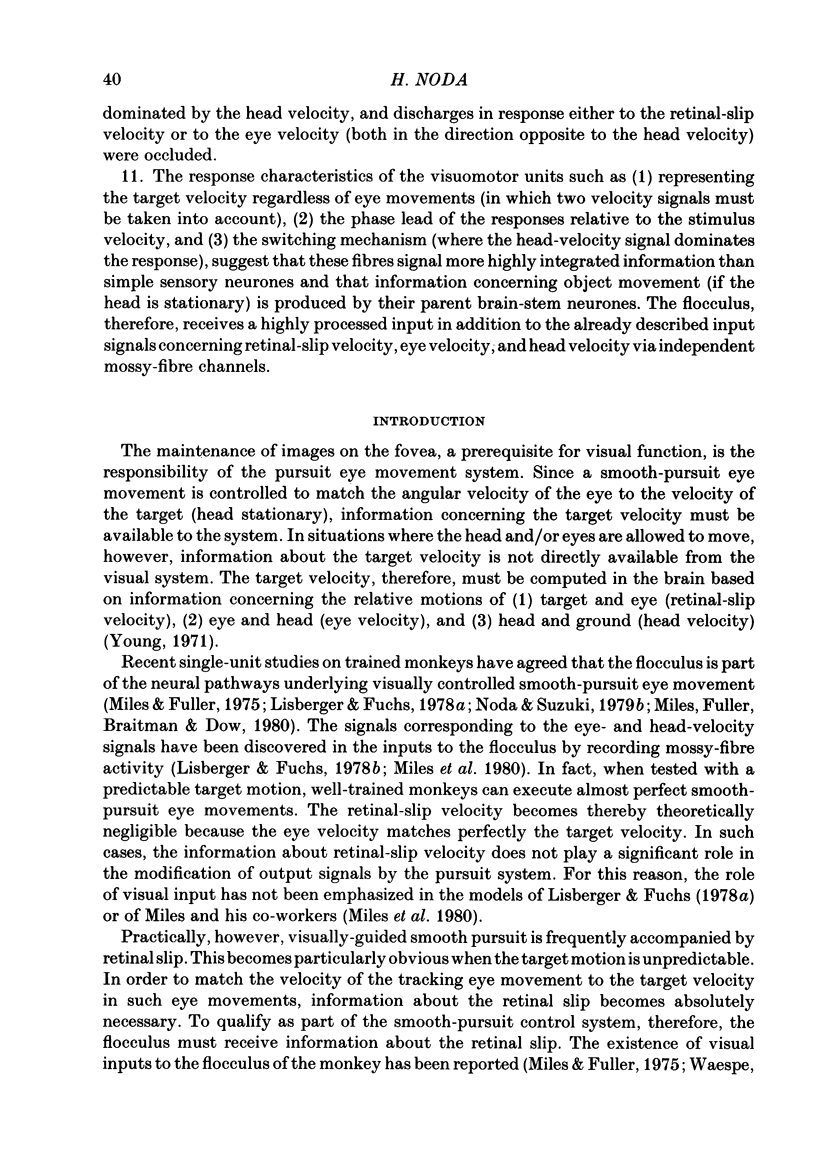
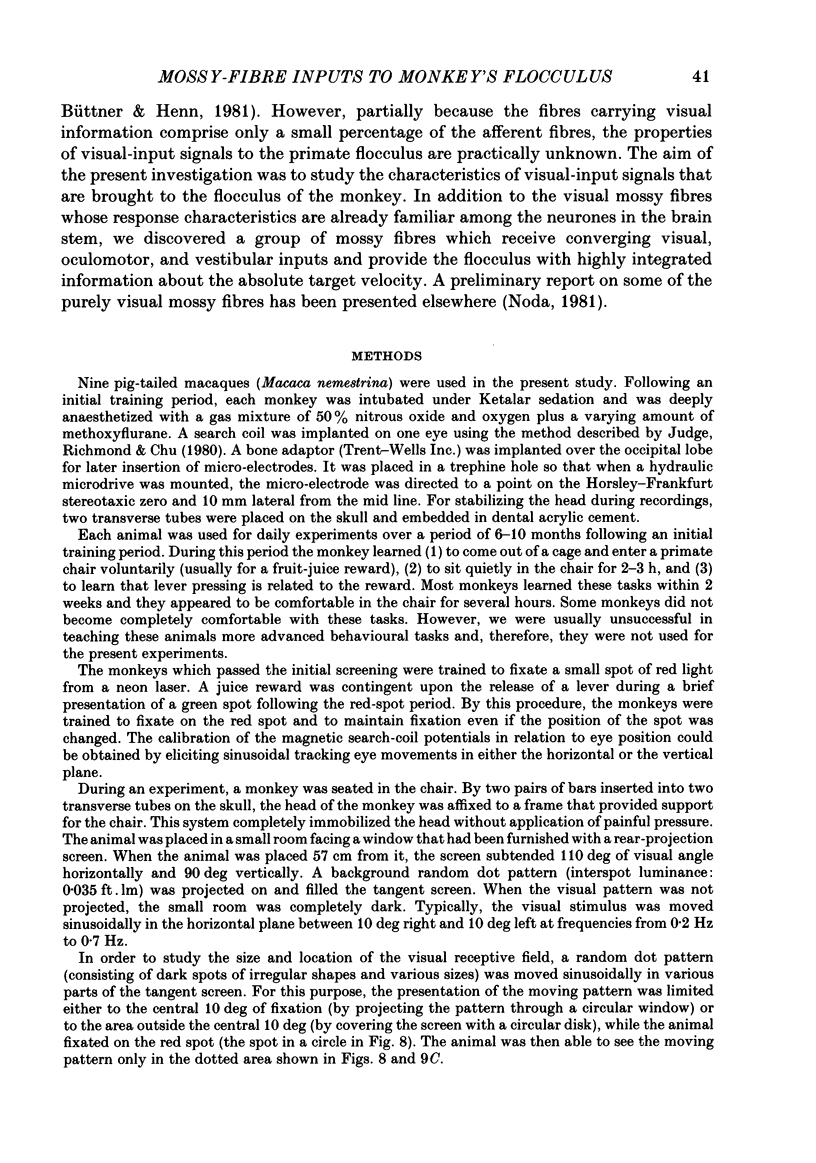
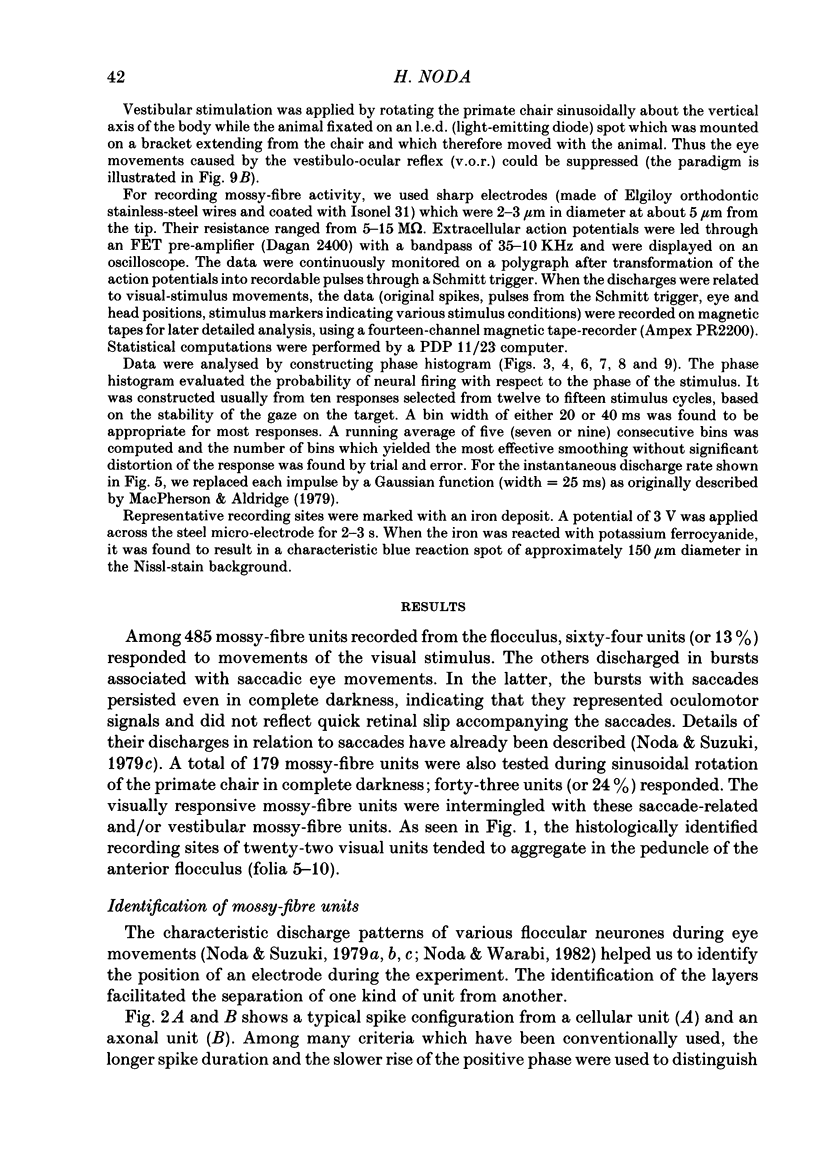
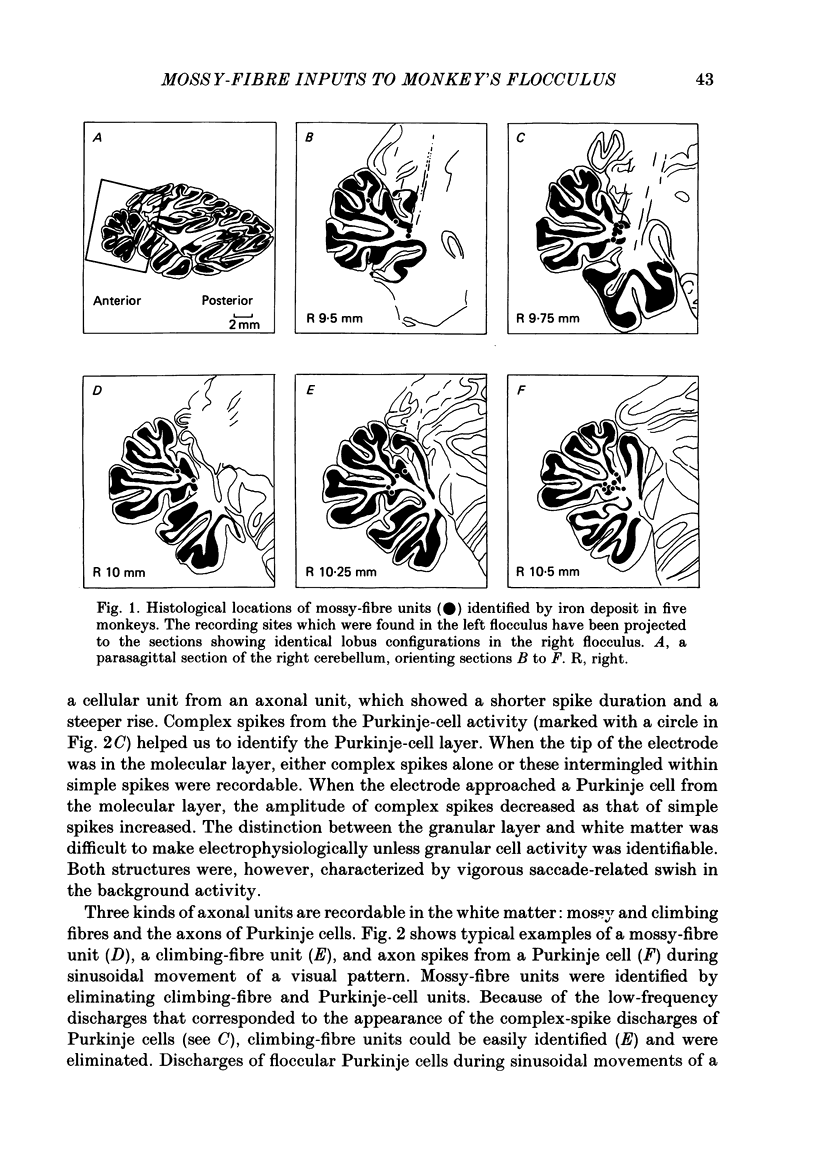
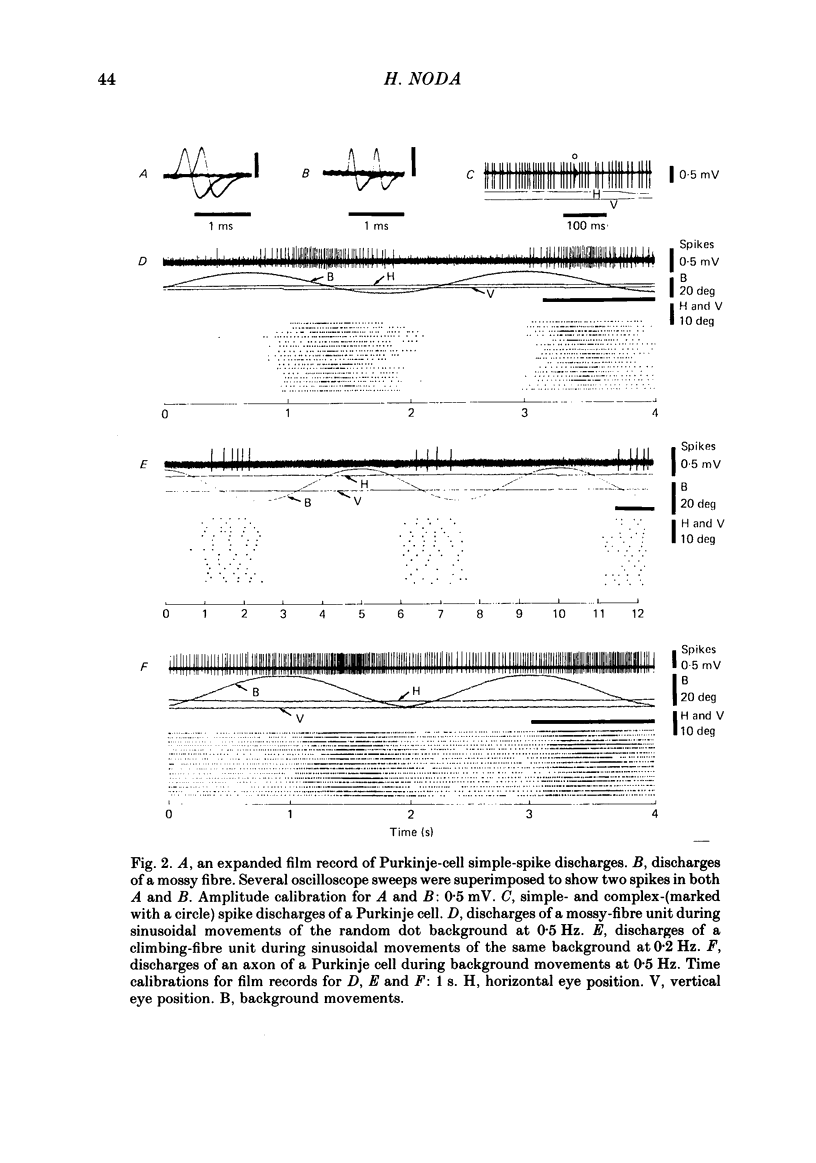
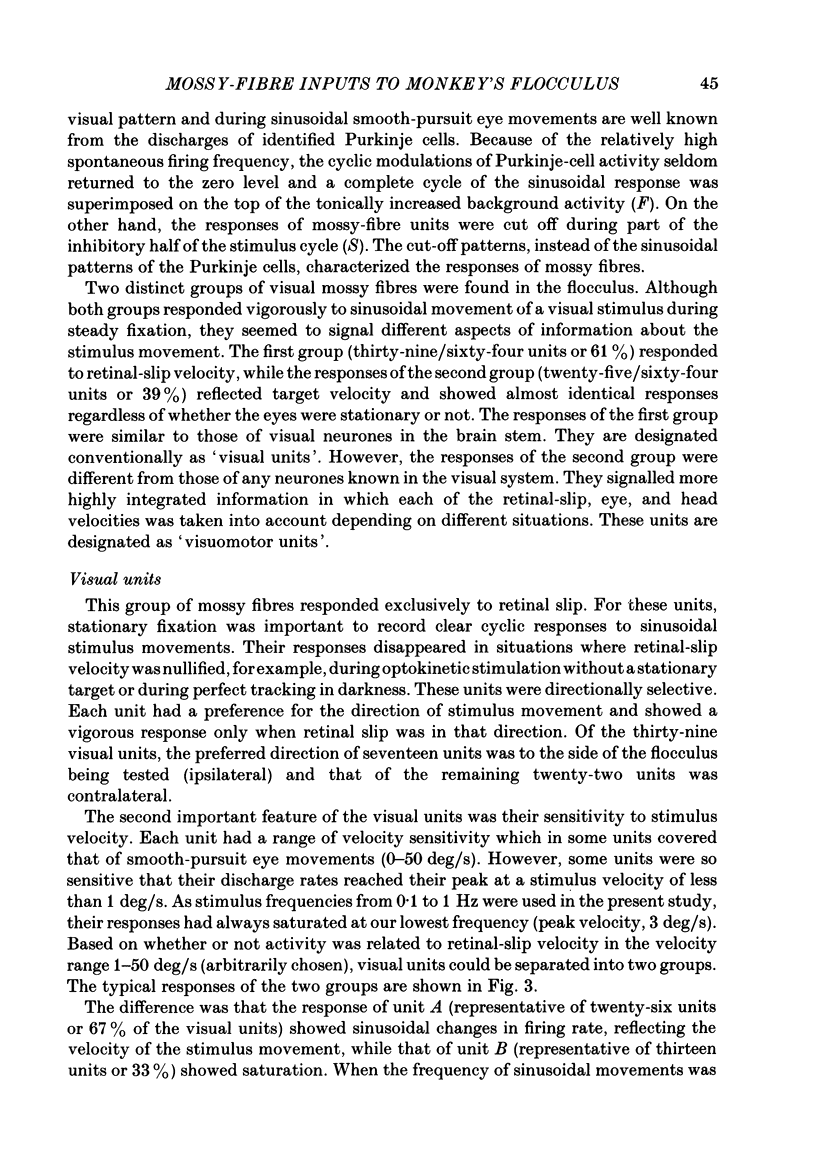
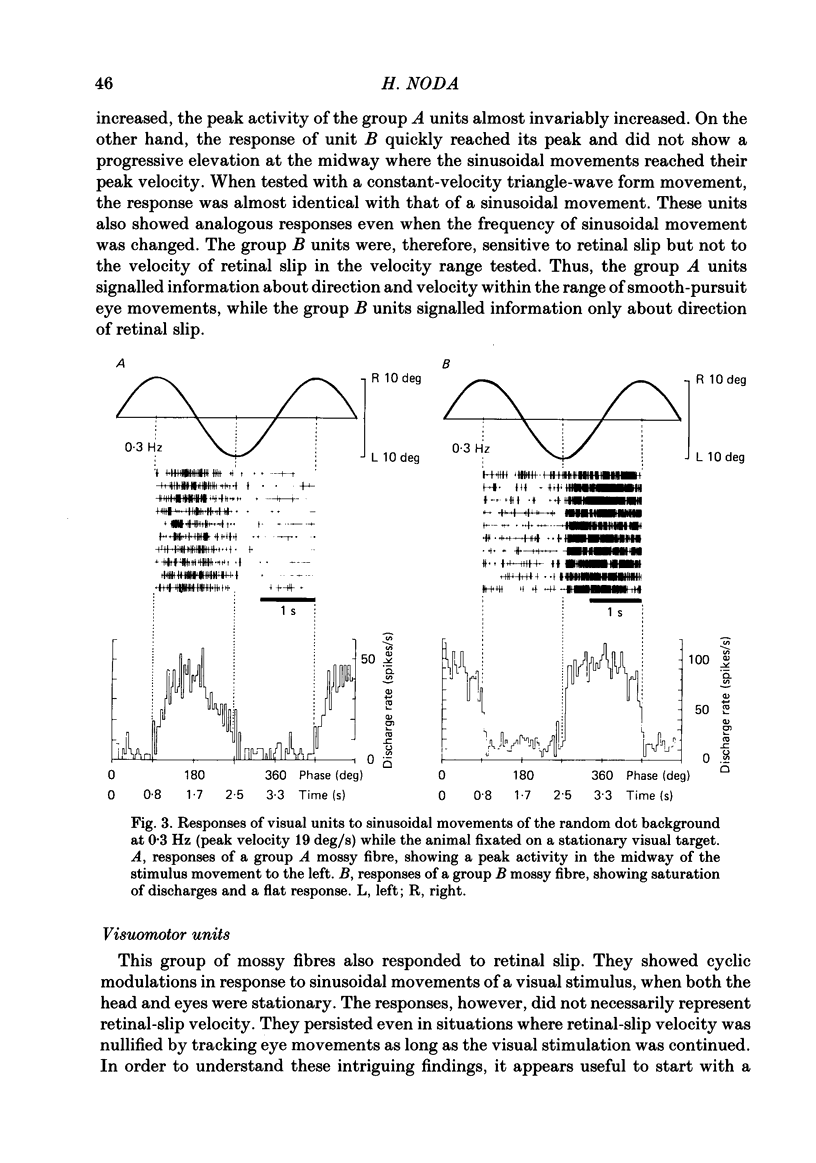
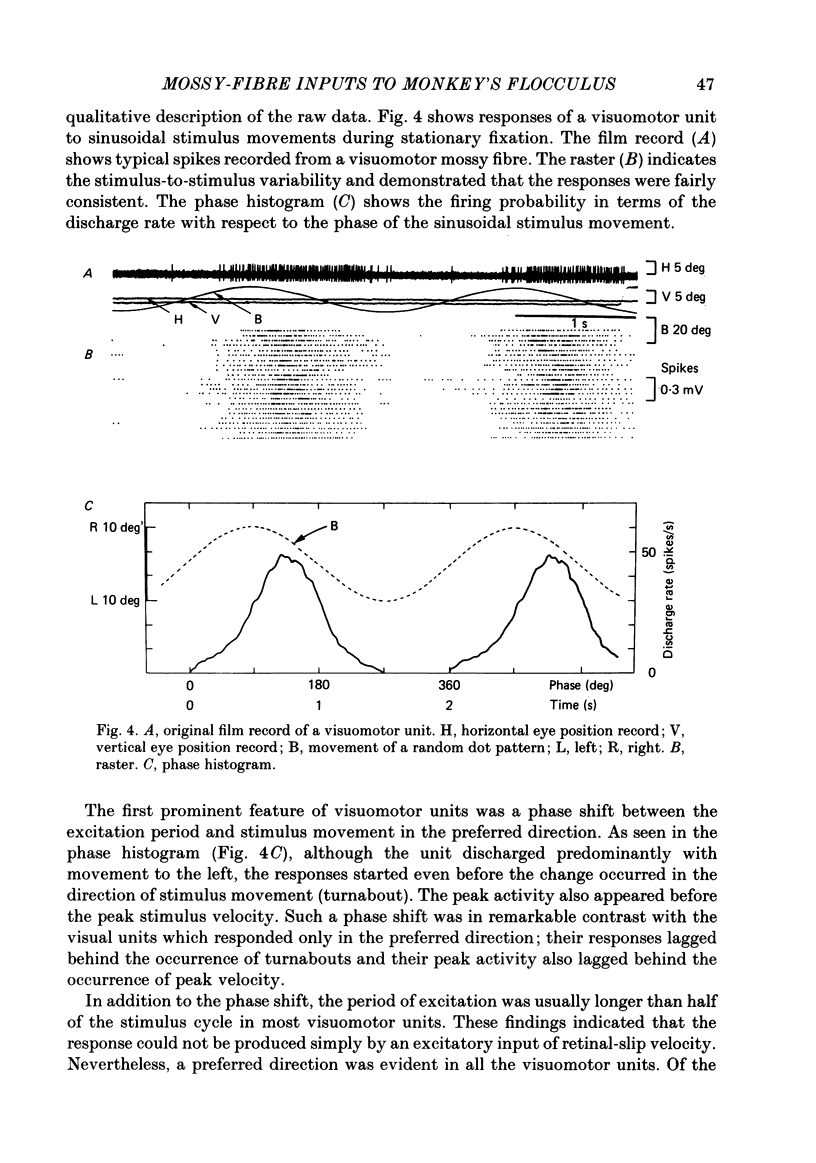
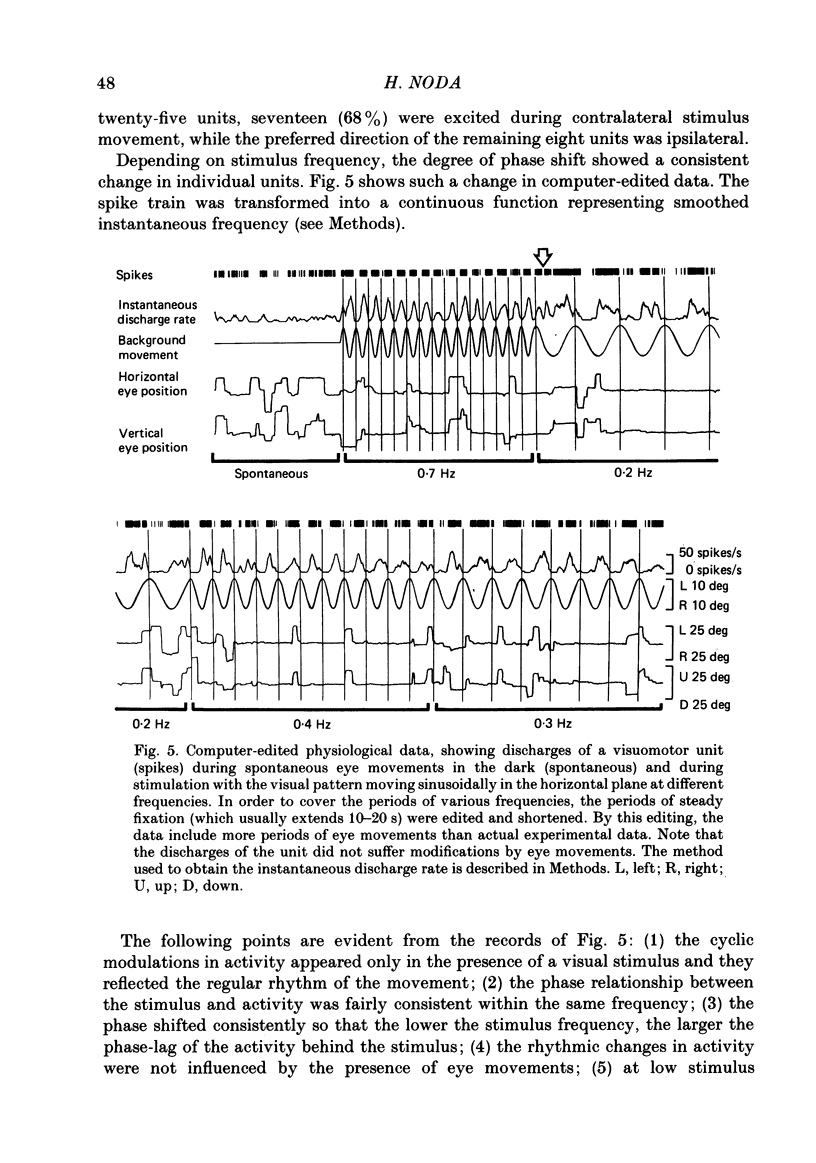
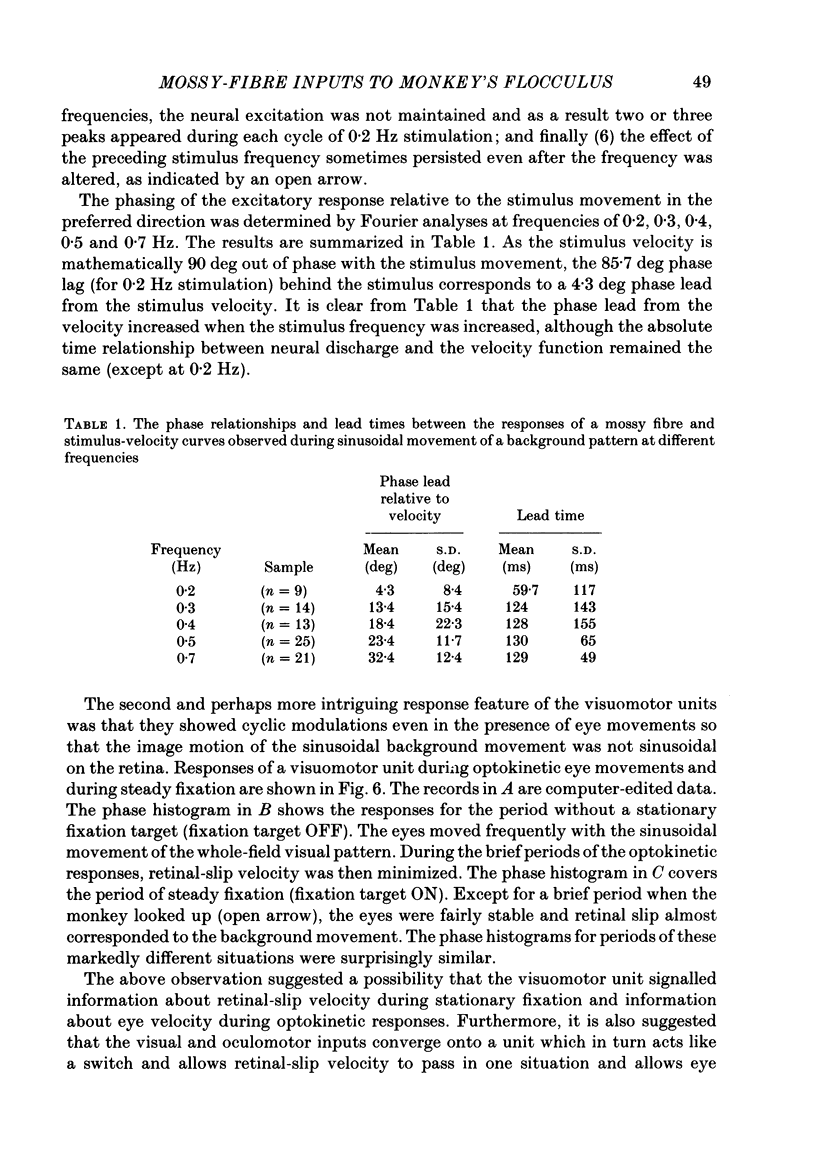
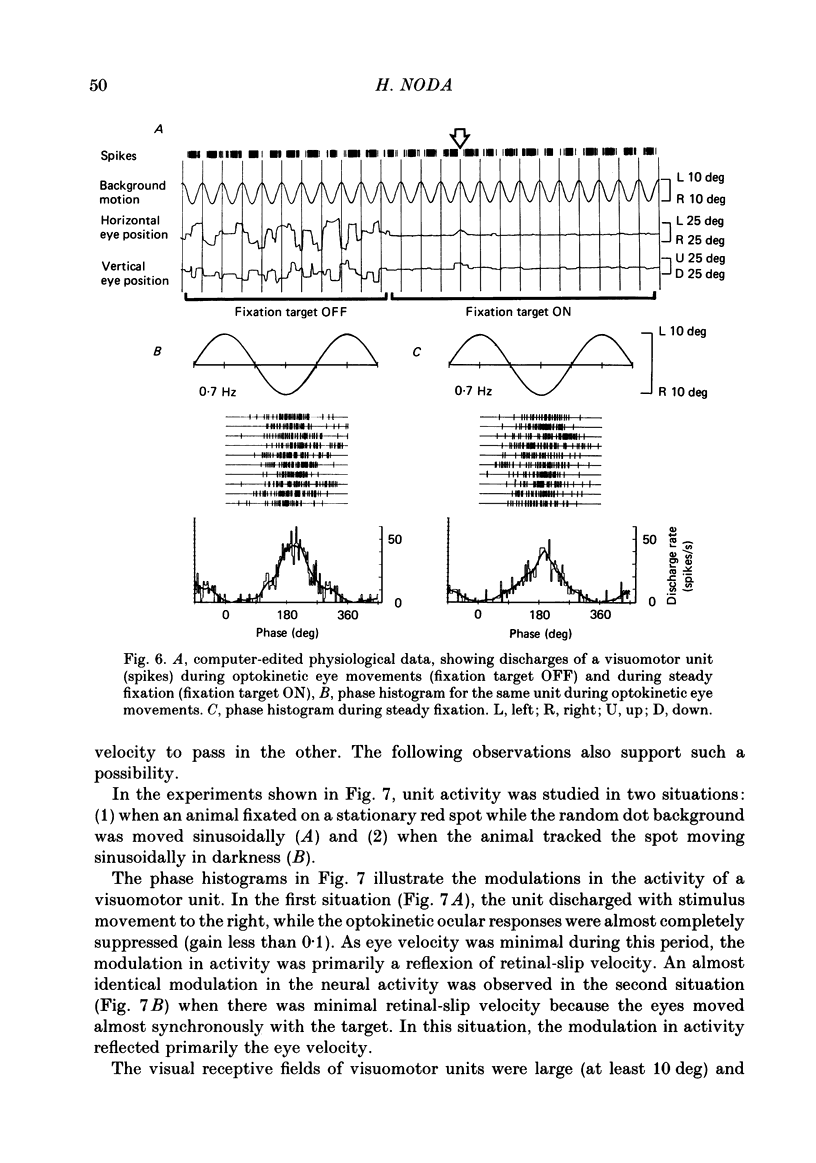
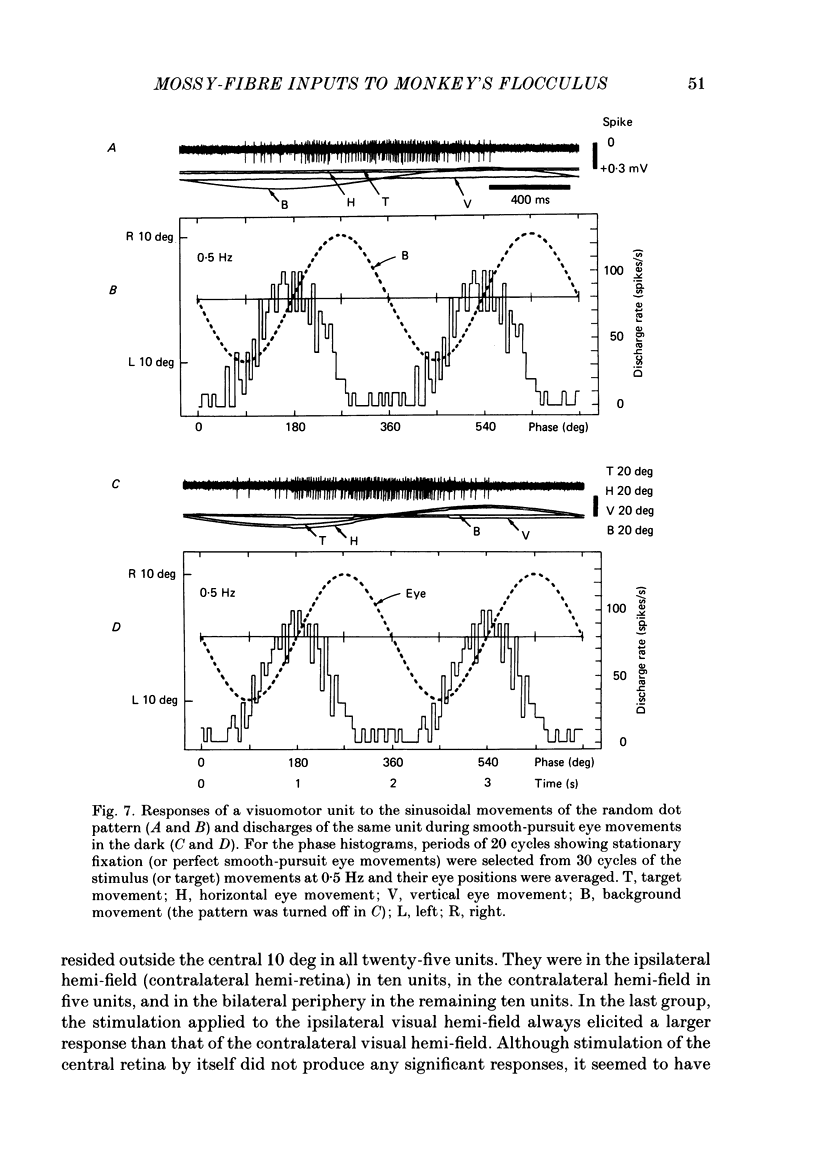
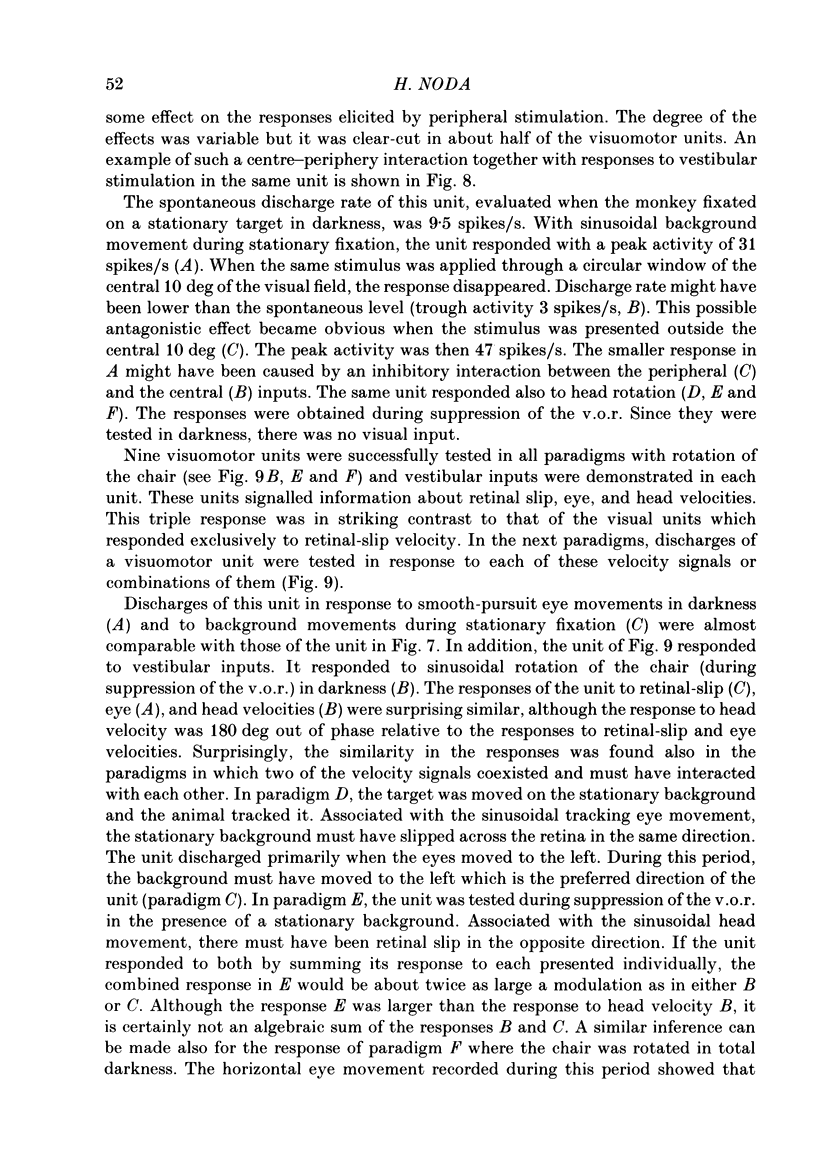
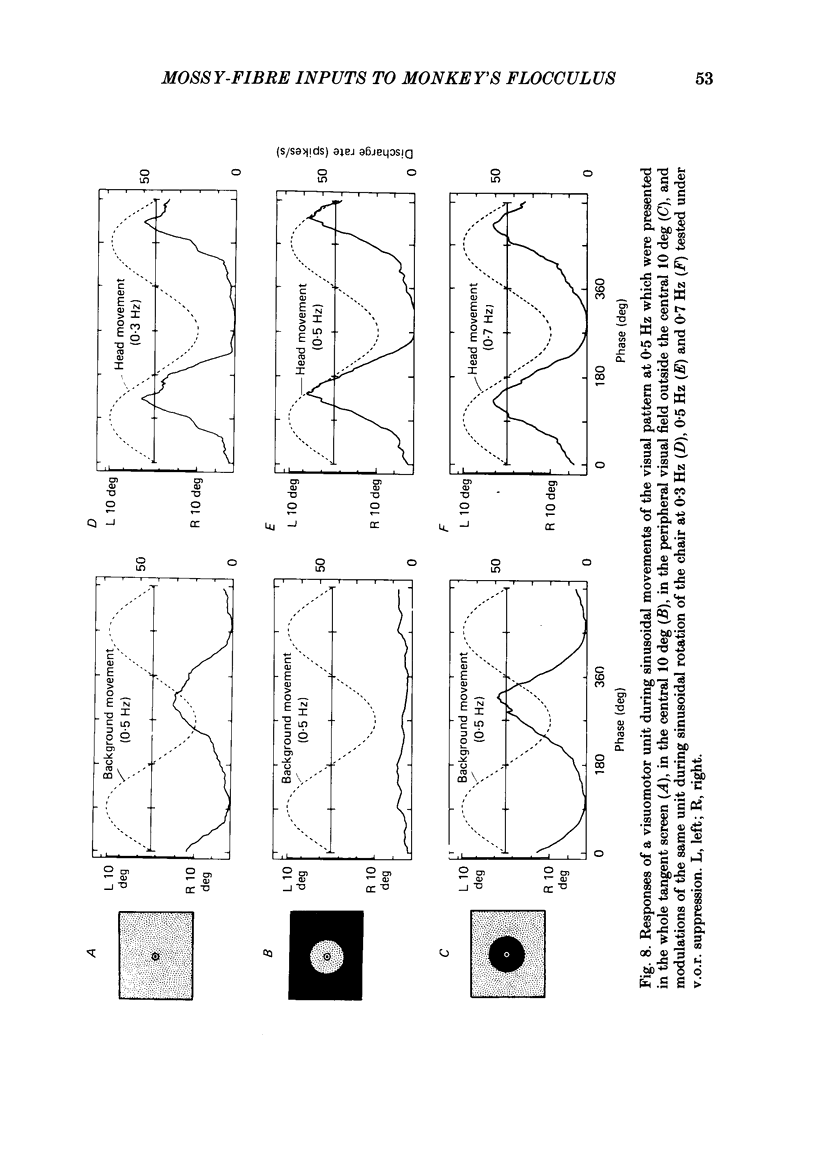
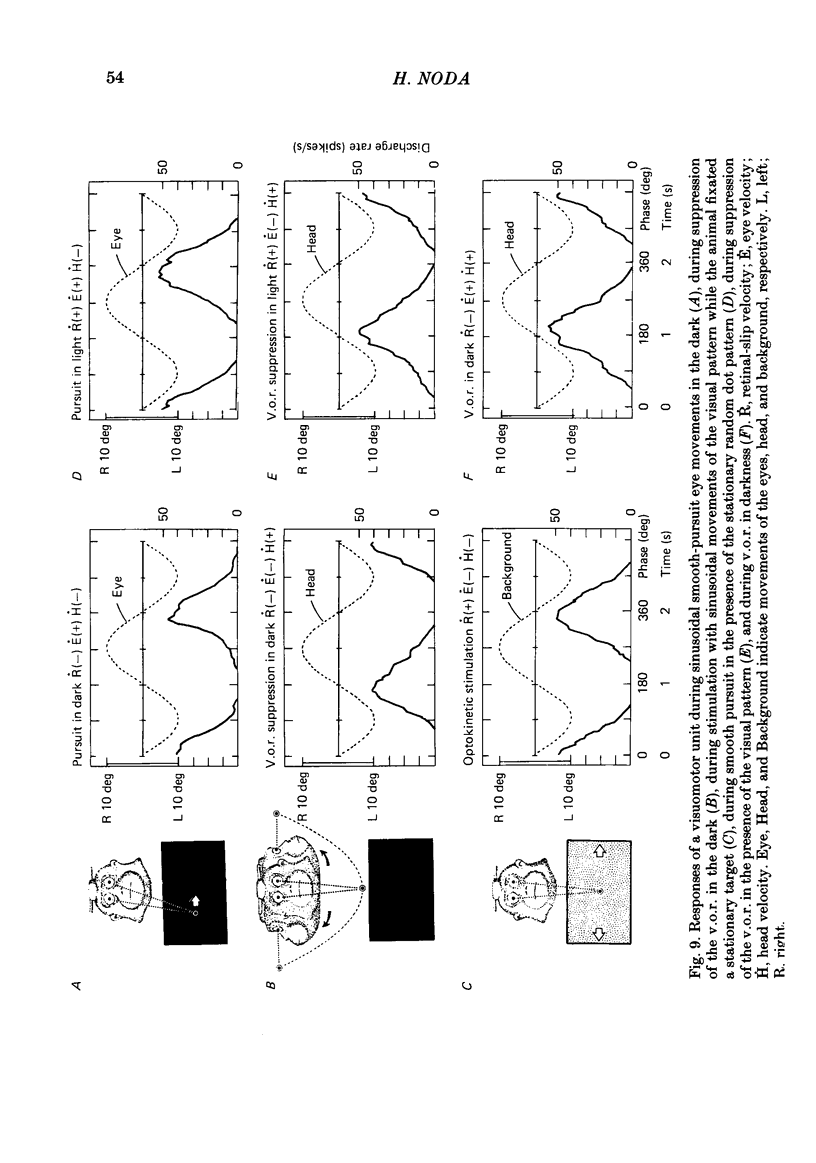
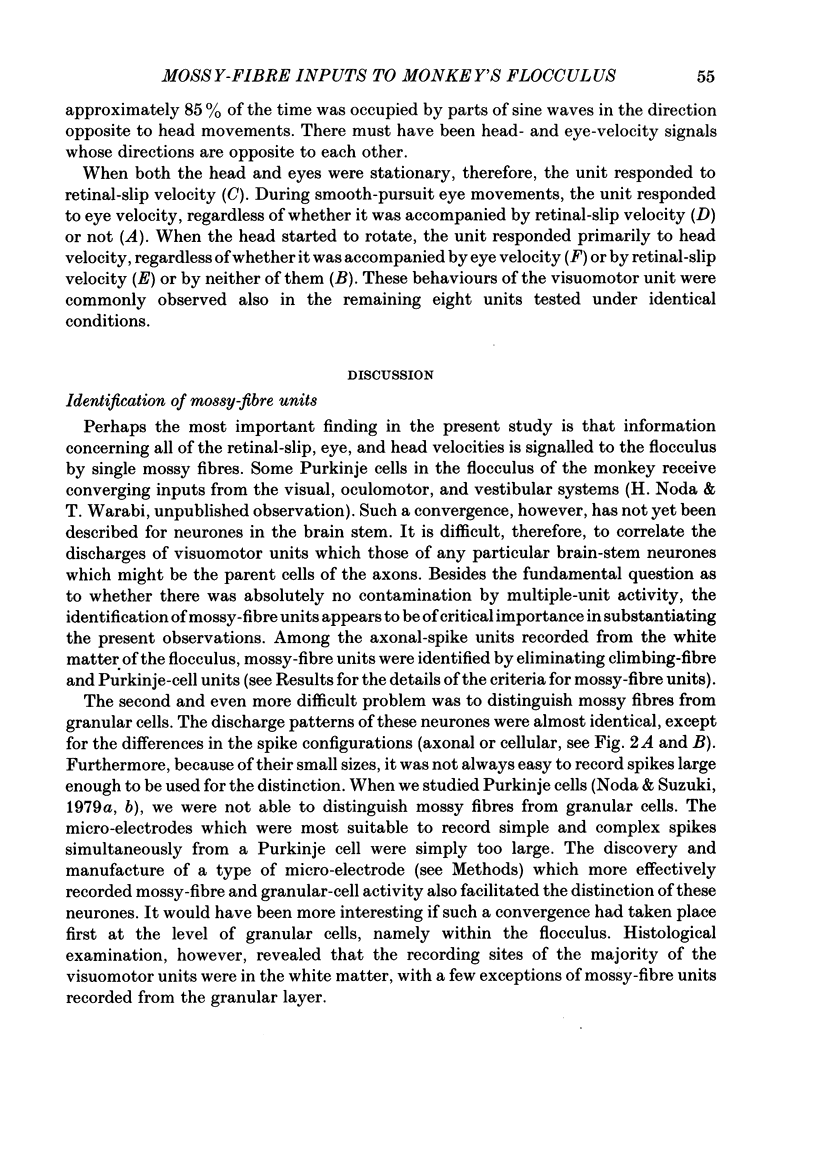
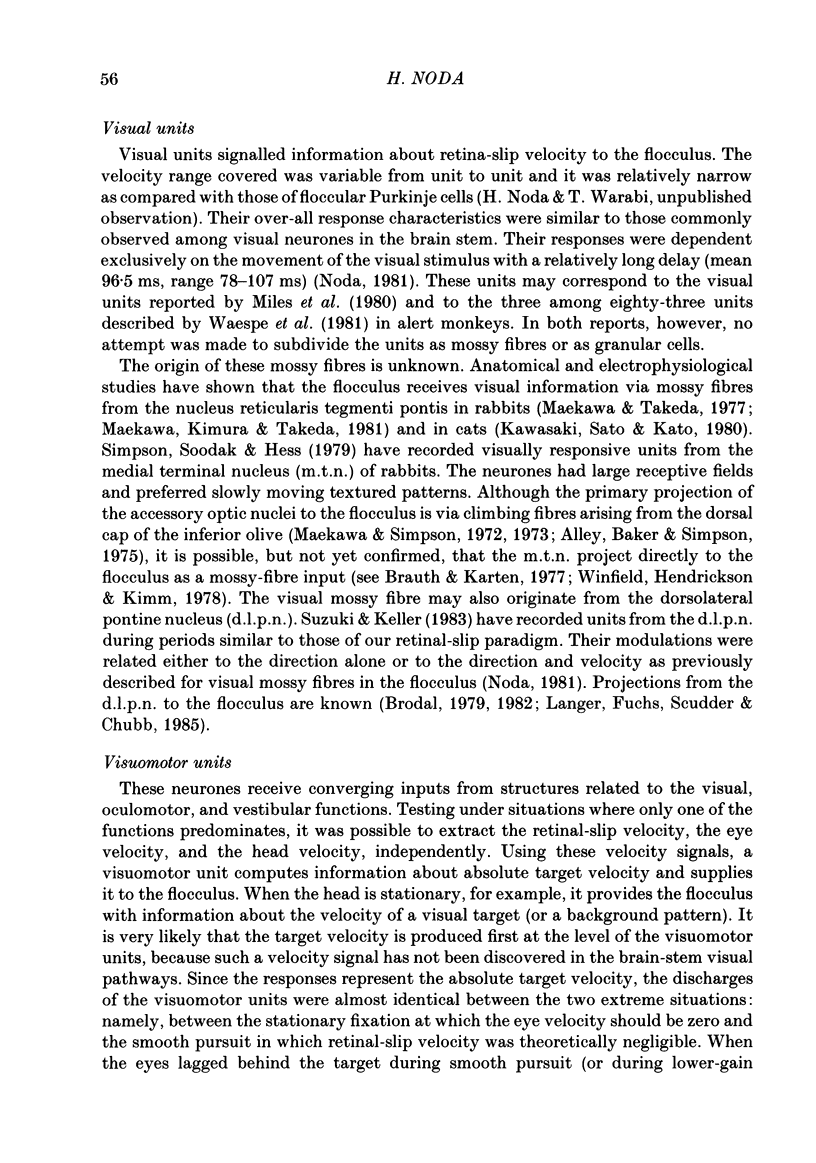
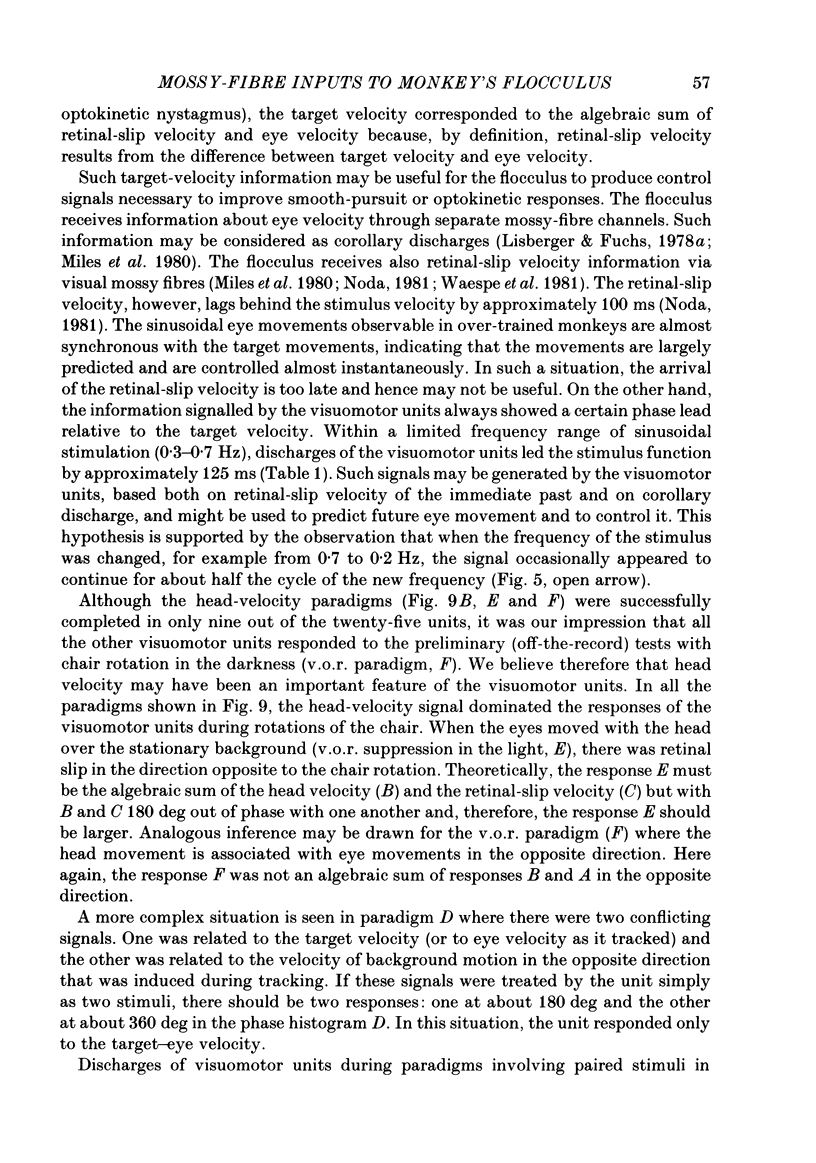
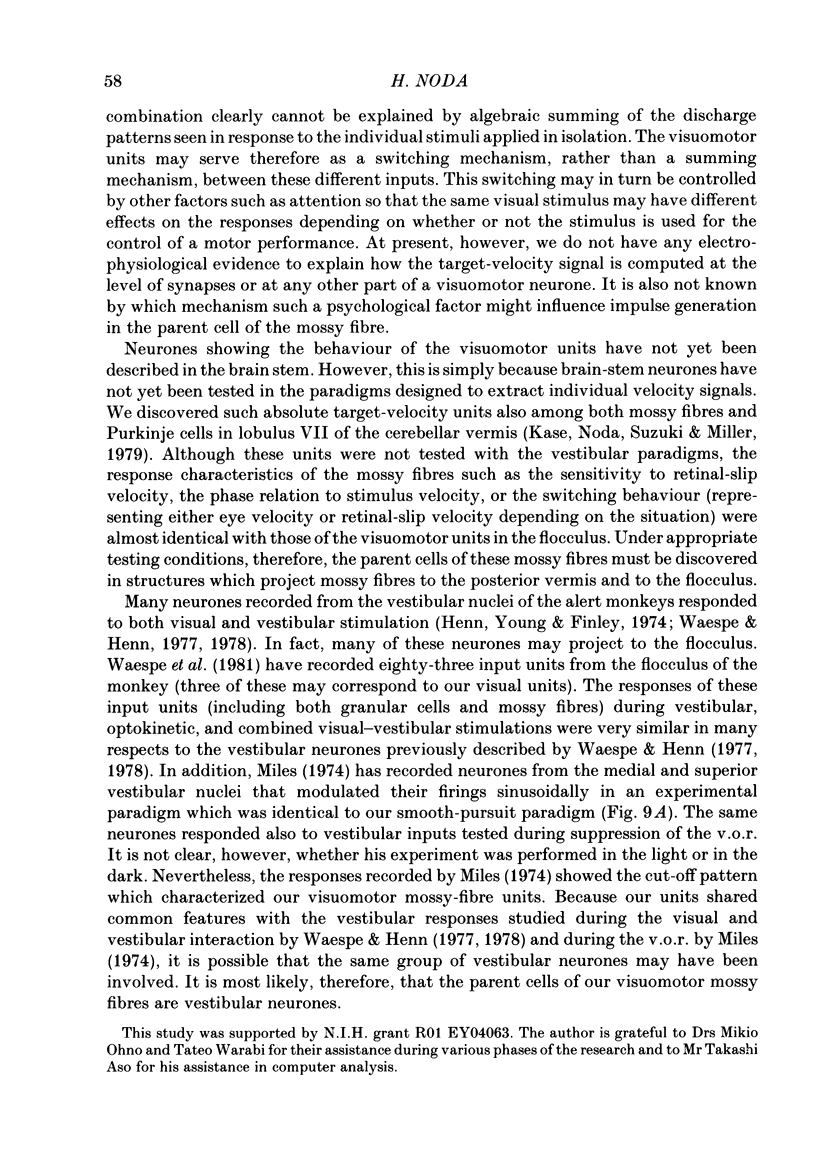
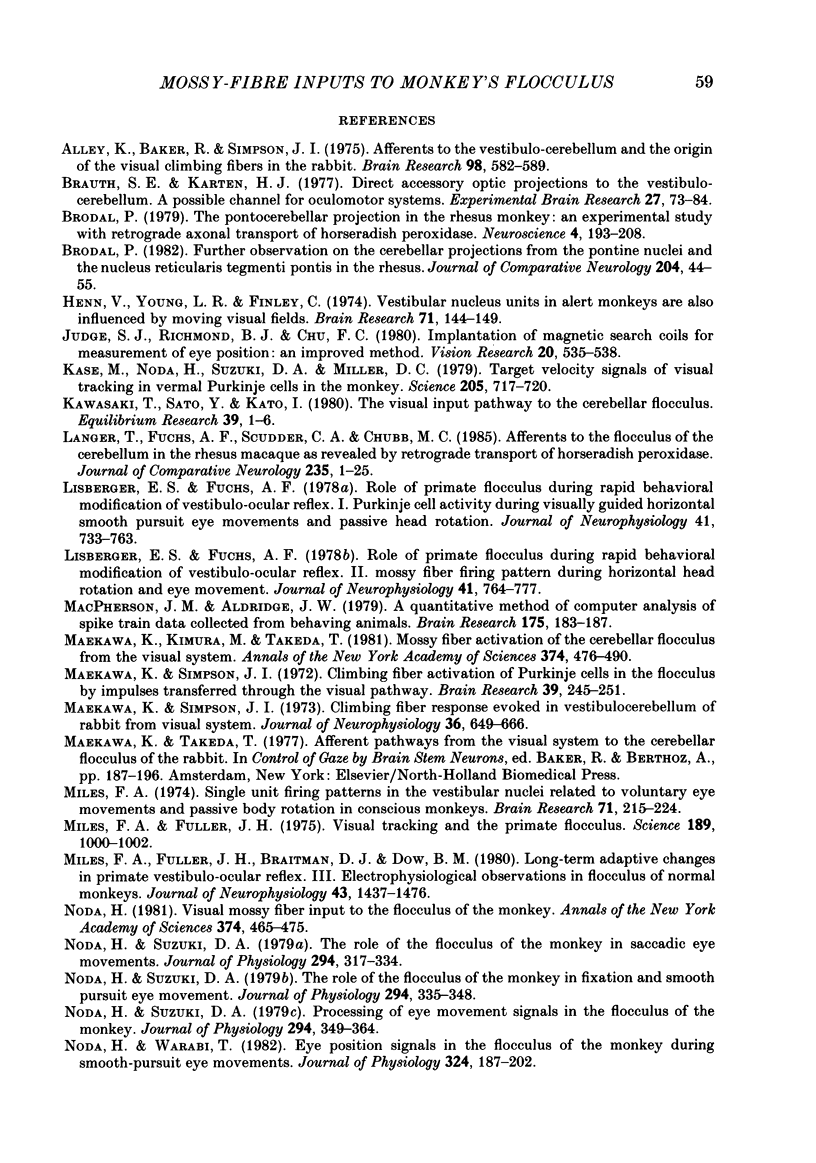
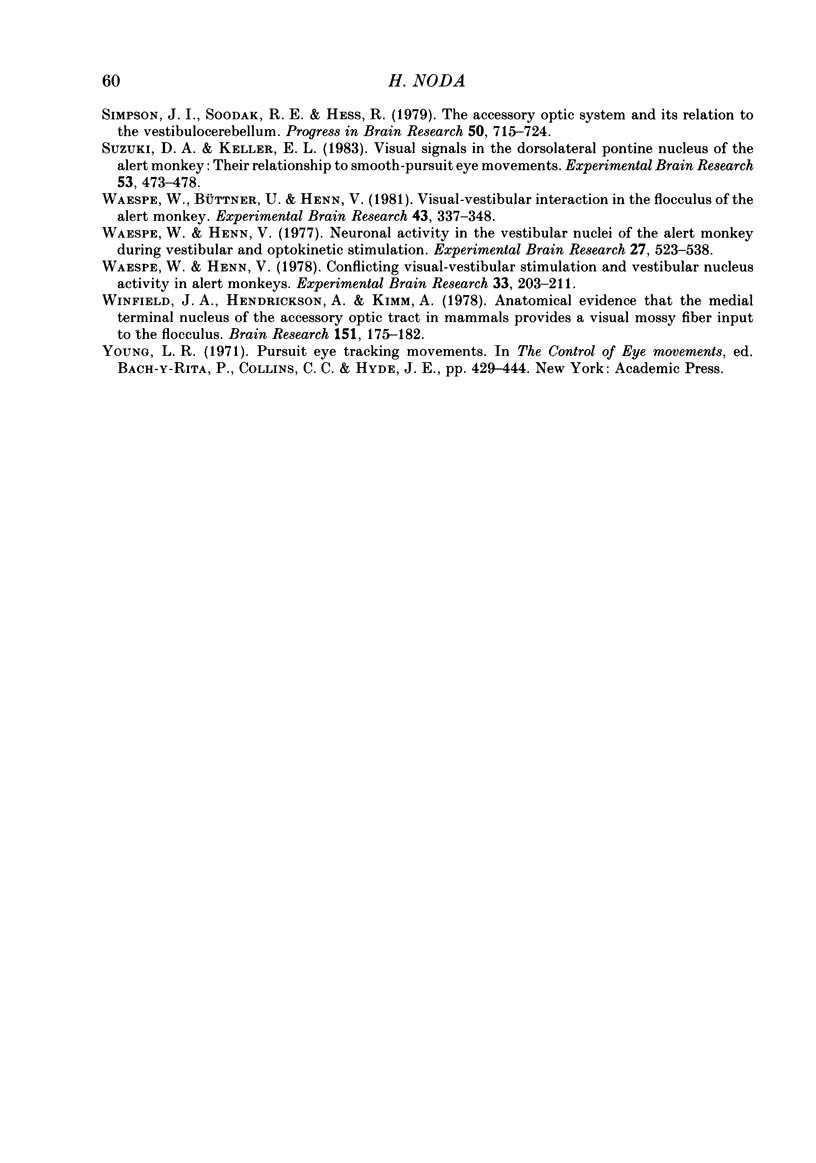
Selected References
These references are in PubMed. This may not be the complete list of references from this article.
- Alley K., Baker R., Simpson J. I. Afferents to the vestibulo-cerebellum and the origin of the visual climbing fibers in the rabbit. Brain Res. 1975 Nov 21;98(3):582–589. doi: 10.1016/0006-8993(75)90375-3. [DOI] [PubMed] [Google Scholar]
- Brauth S. E. Direct accessory optic projections to the vestibulo-cerebellum: a possible channel for oculomotor control systems. Exp Brain Res. 1977 May 23;28(1-2):73–84. doi: 10.1007/BF00237087. [DOI] [PubMed] [Google Scholar]
- Brodal P. Further observations on the cerebellar projections from the pontine nuclei and the nucleus reticularis tegmenti pontis in the rhesus monkey. J Comp Neurol. 1982 Jan 1;204(1):44–55. doi: 10.1002/cne.902040106. [DOI] [PubMed] [Google Scholar]
- Brodal P. The pontocerebellar projection in the rhesus monkey: an experimental study with retrograde axonal transport of horseradish peroxidase. Neuroscience. 1979;4(2):193–208. doi: 10.1016/0306-4522(79)90082-4. [DOI] [PubMed] [Google Scholar]
- Henn V., Young L. R., Finley C. Vestibular nucleus units in alert monkeys are also influenced by moving visual fields. Brain Res. 1974 May 10;71(1):144–149. doi: 10.1016/0006-8993(74)90198-x. [DOI] [PubMed] [Google Scholar]
- Judge S. J., Richmond B. J., Chu F. C. Implantation of magnetic search coils for measurement of eye position: an improved method. Vision Res. 1980;20(6):535–538. doi: 10.1016/0042-6989(80)90128-5. [DOI] [PubMed] [Google Scholar]
- Kase M., Noda H., Suzuki D. A., Miller D. C. Target velocity signals of visual tracking in vermal Purkinje cells of the monkey. Science. 1979 Aug 17;205(4407):717–720. doi: 10.1126/science.111350. [DOI] [PubMed] [Google Scholar]
- Langer T., Fuchs A. F., Scudder C. A., Chubb M. C. Afferents to the flocculus of the cerebellum in the rhesus macaque as revealed by retrograde transport of horseradish peroxidase. J Comp Neurol. 1985 May 1;235(1):1–25. doi: 10.1002/cne.902350102. [DOI] [PubMed] [Google Scholar]
- Lisberger S. G., Fuchs A. F. Role of primate flocculus during rapid behavioral modification of vestibuloocular reflex. I. Purkinje cell activity during visually guided horizontal smooth-pursuit eye movements and passive head rotation. J Neurophysiol. 1978 May;41(3):733–763. doi: 10.1152/jn.1978.41.3.733. [DOI] [PubMed] [Google Scholar]
- Lisberger S. G., Fuchs A. F. Role of primate flocculus during rapid behavioral modification of vestibuloocular reflex. II. Mossy fiber firing patterns during horizontal head rotation and eye movement. J Neurophysiol. 1978 May;41(3):764–777. doi: 10.1152/jn.1978.41.3.764. [DOI] [PubMed] [Google Scholar]
- MacPherson J. M., Aldridge J. W. A quantitative method of computer analysis of spike train data collected from behaving animals. Brain Res. 1979 Oct 12;175(1):183–187. doi: 10.1016/0006-8993(79)90530-4. [DOI] [PubMed] [Google Scholar]
- Maekawa K., Kimura M., Takeda T. Mossy fiber activation of the cerebellar flocculus from the visual system. Ann N Y Acad Sci. 1981;374:476–490. doi: 10.1111/j.1749-6632.1981.tb30893.x. [DOI] [PubMed] [Google Scholar]
- Maekawa K., Simpson J. I. Climbing fiber activation of Purkinje cells in the flocculus by impulses transferred through the visual pathway. Brain Res. 1972 Apr 14;39(1):245–251. doi: 10.1016/0006-8993(72)90800-1. [DOI] [PubMed] [Google Scholar]
- Maekawa K., Simpson J. I. Climbing fiber responses evoked in vestibulocerebellum of rabbit from visual system. J Neurophysiol. 1973 Jul;36(4):649–666. doi: 10.1152/jn.1973.36.4.649. [DOI] [PubMed] [Google Scholar]
- Miles F. A., Fuller J. H., Braitman D. J., Dow B. M. Long-term adaptive changes in primate vestibuloocular reflex. III. Electrophysiological observations in flocculus of normal monkeys. J Neurophysiol. 1980 May;43(5):1437–1476. doi: 10.1152/jn.1980.43.5.1437. [DOI] [PubMed] [Google Scholar]
- Miles F. A., Fuller J. H. Visual tracking and the primate flocculus. Science. 1975 Sep 19;189(4207):1000–1002. doi: 10.1126/science.1083068. [DOI] [PubMed] [Google Scholar]
- Miles F. A. Single unit firing patterns in the vestibular nuclei related to voluntary eye movements and passive body rotation in conscious monkeys. Brain Res. 1974 May 17;71(2-3):215–224. doi: 10.1016/0006-8993(74)90963-9. [DOI] [PubMed] [Google Scholar]
- Noda H., Suzuki D. A. Processing of eye movement signals in the flocculus of the monkey. J Physiol. 1979 Sep;294:349–364. doi: 10.1113/jphysiol.1979.sp012934. [DOI] [PMC free article] [PubMed] [Google Scholar]
- Noda H., Suzuki D. A. The role of the flocculus of the monkey in fixation and smooth pursuit eye movements. J Physiol. 1979 Sep;294:335–348. doi: 10.1113/jphysiol.1979.sp012933. [DOI] [PMC free article] [PubMed] [Google Scholar]
- Noda H., Suzuki D. A. The role of the flocculus of the monkey in saccadic eye movements. J Physiol. 1979 Sep;294:317–334. doi: 10.1113/jphysiol.1979.sp012932. [DOI] [PMC free article] [PubMed] [Google Scholar]
- Noda H. Visual mossy fiber inputs to the flocculus of the monkey. Ann N Y Acad Sci. 1981;374:465–475. doi: 10.1111/j.1749-6632.1981.tb30892.x. [DOI] [PubMed] [Google Scholar]
- Noda H., Warabi T. Eye position signals in the flocculus of the monkey during smooth-pursuit eye movements. J Physiol. 1982 Mar;324:187–202. doi: 10.1113/jphysiol.1982.sp014106. [DOI] [PMC free article] [PubMed] [Google Scholar]
- Simpson J. I., Soodak R. E., Hess R. The accessory optic system and its relation to the vestibulocerebellum. Prog Brain Res. 1979;50:715–724. doi: 10.1016/S0079-6123(08)60868-7. [DOI] [PubMed] [Google Scholar]
- Suzuki D. A., Keller E. L. Visual signals in the dorsolateral pontine nucleus of the alert monkey: their relationship to smooth-pursuit eye movements. Exp Brain Res. 1984;53(2):473–478. doi: 10.1007/BF00238178. [DOI] [PubMed] [Google Scholar]
- Waespe W., Büttner U., Henn V. Visual-vestibular interaction in the flocculus of the alert monkey. I. Input activity. Exp Brain Res. 1981;43(3-4):337–348. doi: 10.1007/BF00238376. [DOI] [PubMed] [Google Scholar]
- Waespe W., Henn V. Conflicting visual-vestibular stimulation and vestibular nucleus activity in alert monkeys. Exp Brain Res. 1978 Oct 13;33(2):203–211. doi: 10.1007/BF00238060. [DOI] [PubMed] [Google Scholar]
- Waespe W., Henn V. Neuronal activity in the vestibular nuclei of the alert monkey during vestibular and optokinetic stimulation. Exp Brain Res. 1977 Apr 21;27(5):523–538. doi: 10.1007/BF00239041. [DOI] [PubMed] [Google Scholar]
- Winfield J. A., Hendrickson A., Kimm J. Anatomical evidence that the medial terminal nucleus of the accessory optic tract in mammals provides a visual mossy fiber input to the flocculus. Brain Res. 1978 Jul 28;151(1):175–182. doi: 10.1016/0006-8993(78)90961-7. [DOI] [PubMed] [Google Scholar]