Abstract
1. Double-barrelled ion-sensitive micro-electrodes were used to measure changes in the intracellular activities of K+, Na+ and Cl- (aiK, aiNa, aiCl) in glial cells of slices from guinea-pig olfactory cortex during repetitive stimulation of the lateral olfactory tract. 2. Base-line levels of aiK, aiNa and aiCl were about 66, 25 and 6 mM, respectively, for cells with resting potentials higher than -80 mV. During stimulation, intraglial aiK and aiCl increased, whereas aiNa decreased. Within about 2 min after stimulation the ion activities returned to their base-line levels. 3. The Cl- equilibrium potential was found to be close to the membrane potential (Em). There was also a strong correlation between changes of Em and aiCl. These observations indicate a high Cl- conductance of the glial cell membrane. 4. In the presence of Ba2+, the usual depolarizing response of the glial cells to a rise of the extracellular K+ activity (aeK) reversed into a membrane hyperpolarization. Furthermore, Ba2+ strongly reduced the stimulus-related rise of intraglial aiK. An additional application of ouabain blocked both the membrane hyperpolarization as well as the remaining rise of aiK. 5. In conclusion, our data show that glial cells in guinea-pig olfactory cortex slices possess at least two mechanisms of K+ accumulation. One mechanism is sensitive to the K+ channel blocker Ba2+ and might be a passive KCl influx. The other appears to be the electrogenic Na+/K+ pump, which can be activated by excess extracellular K+.
Full text
PDF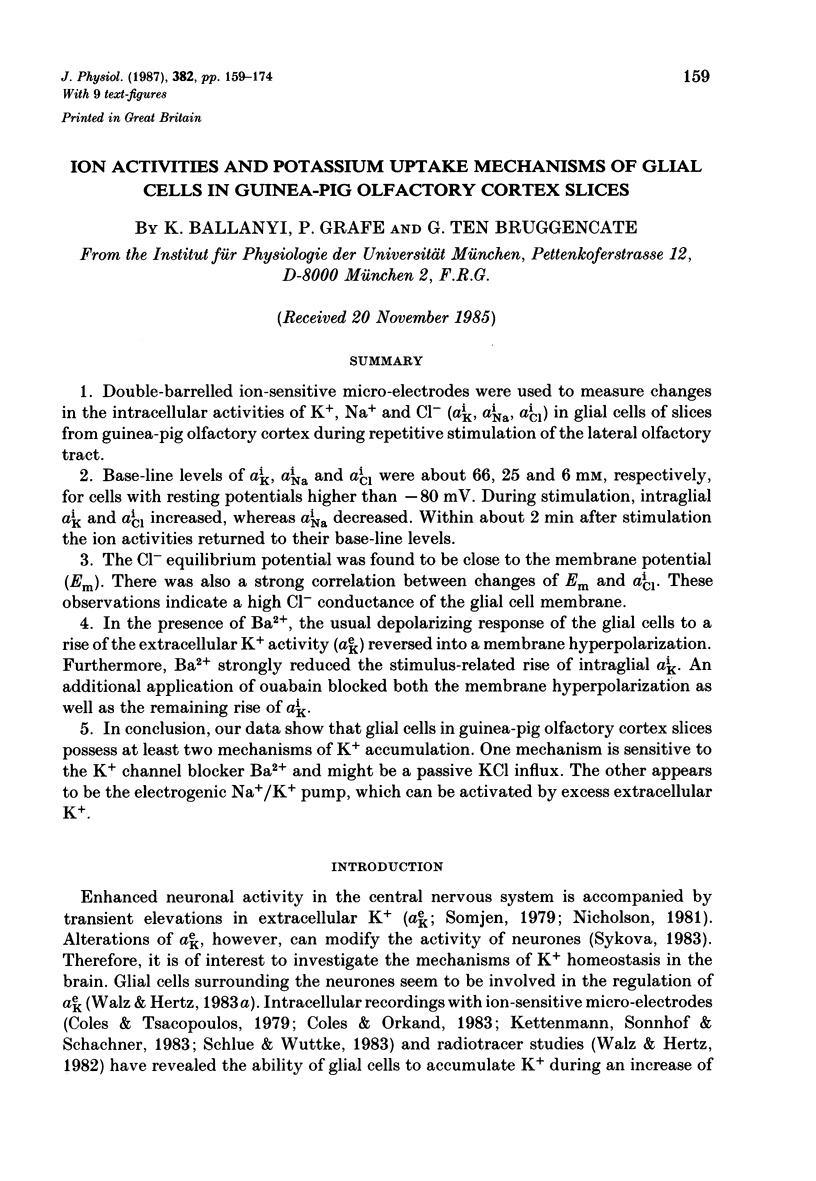
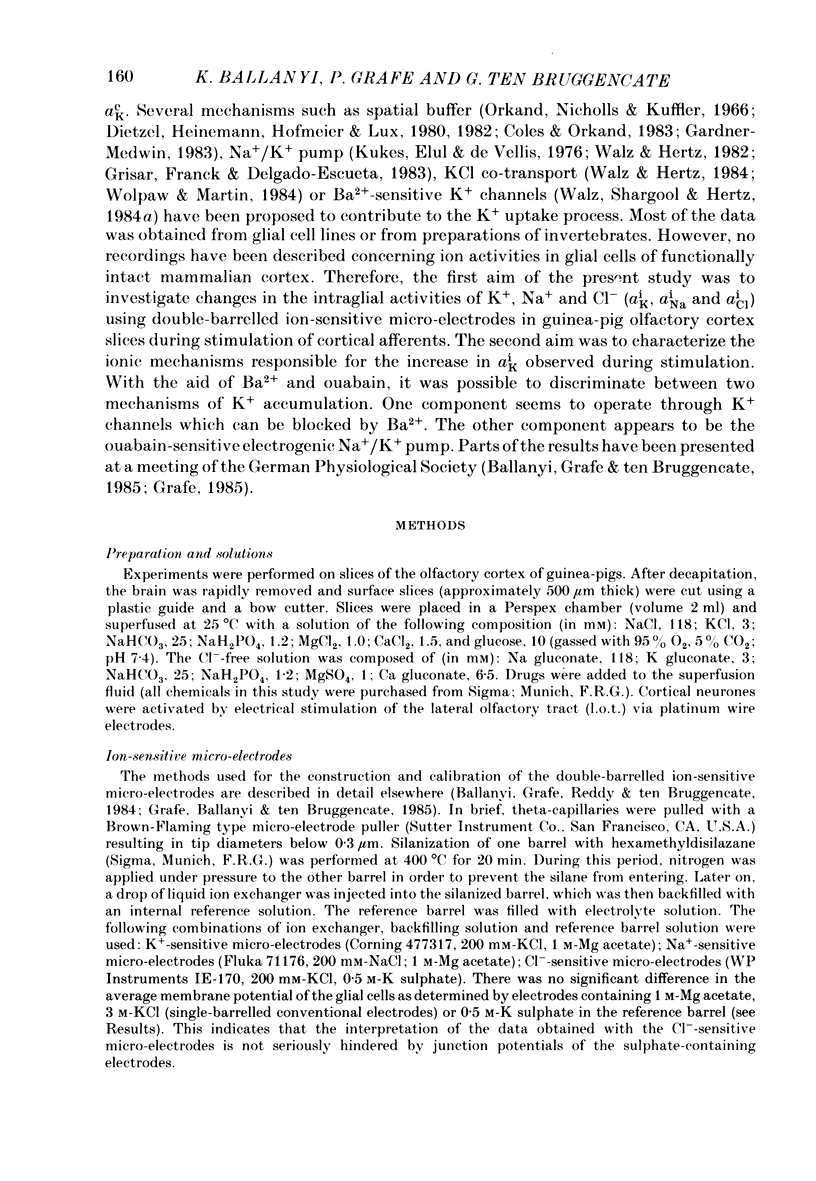
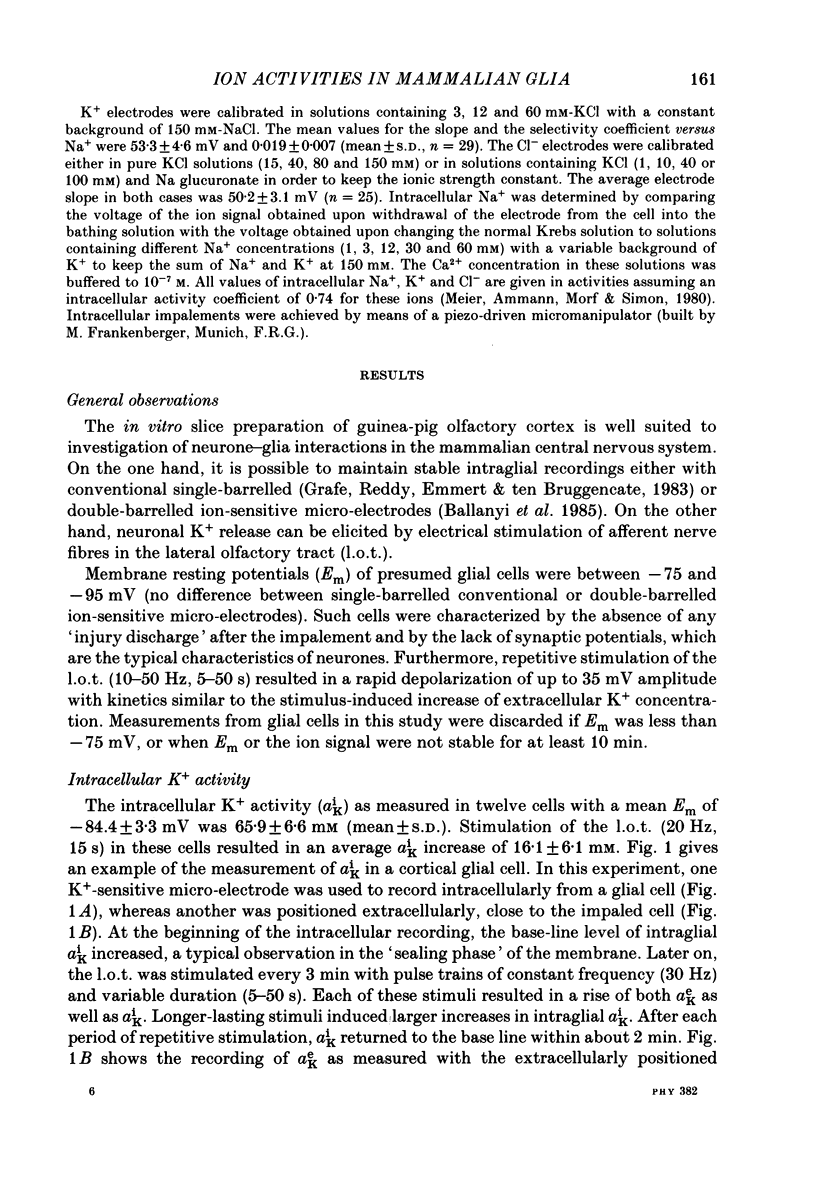
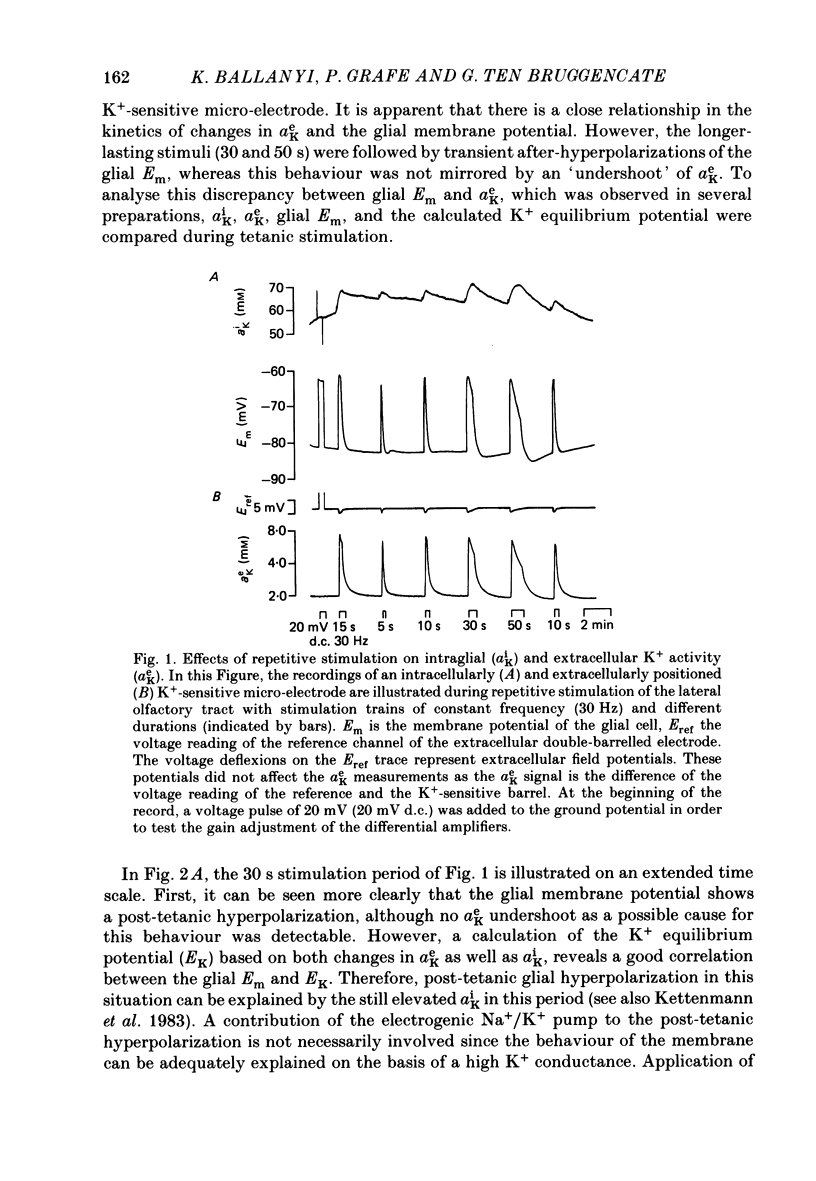
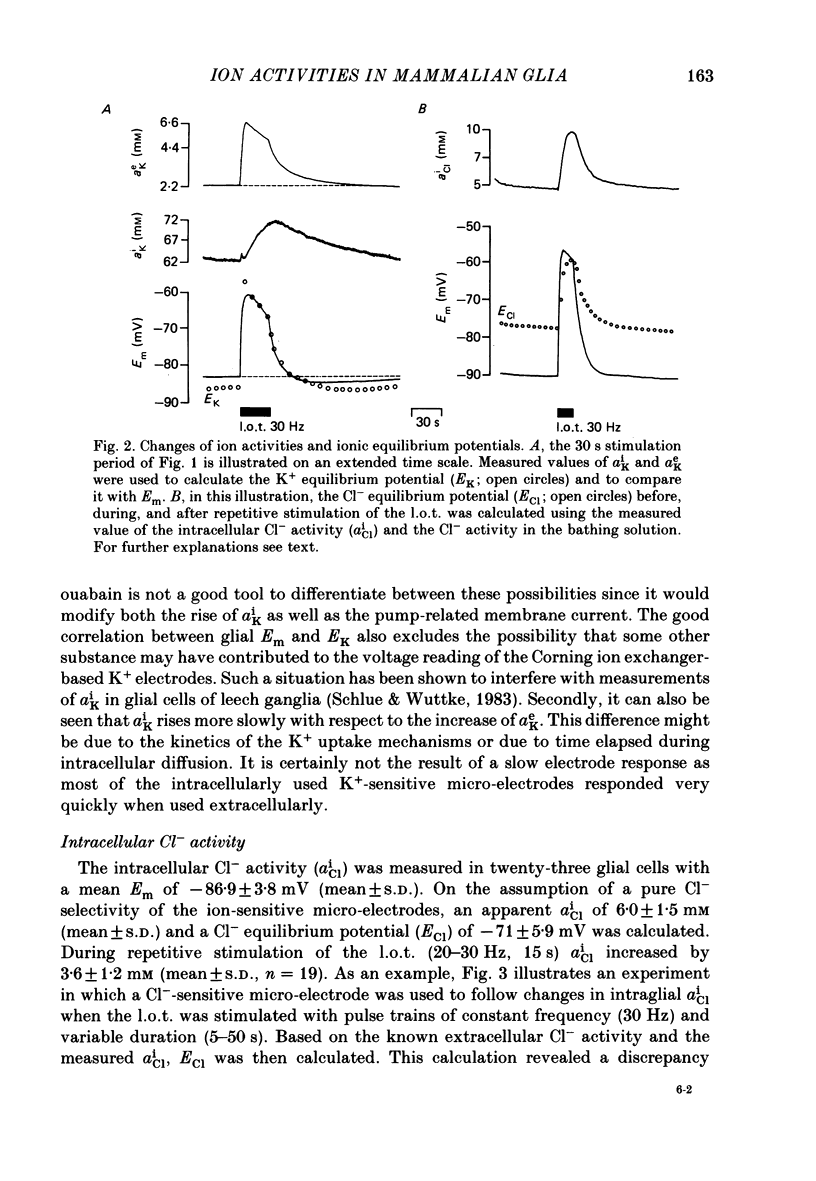
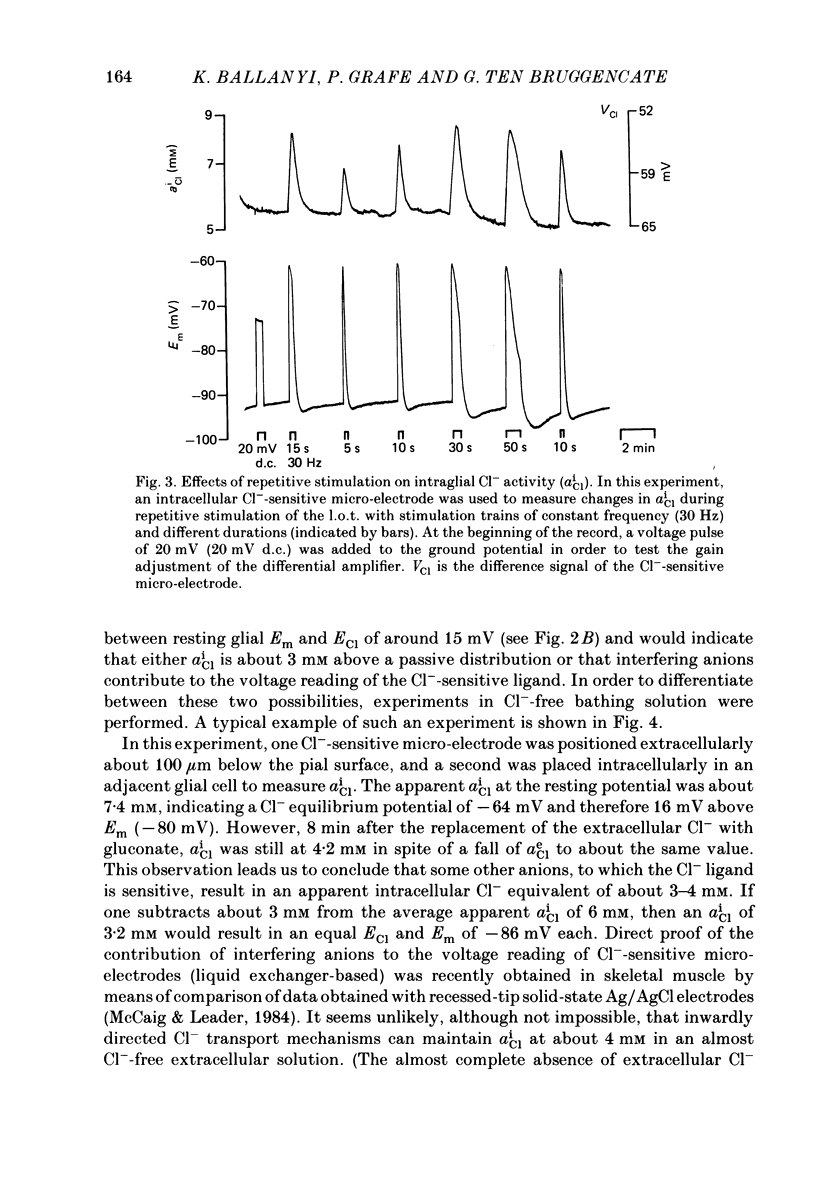
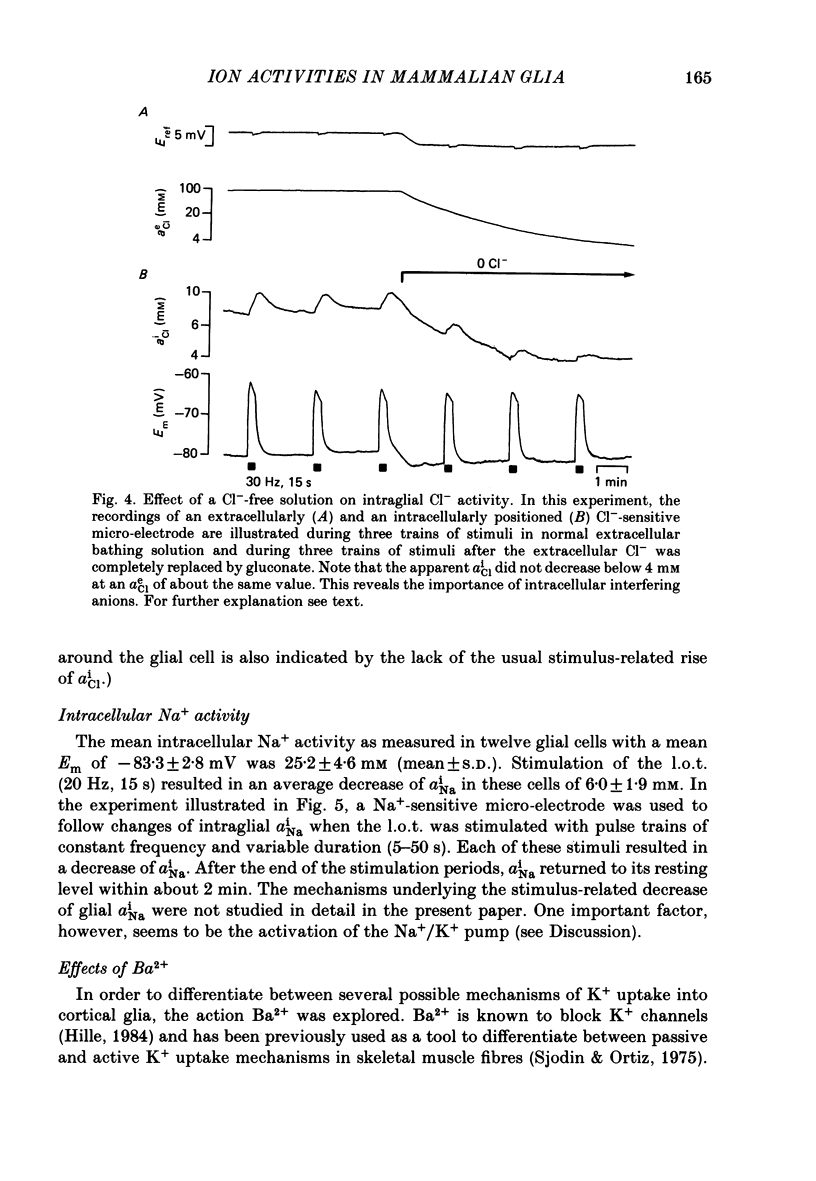
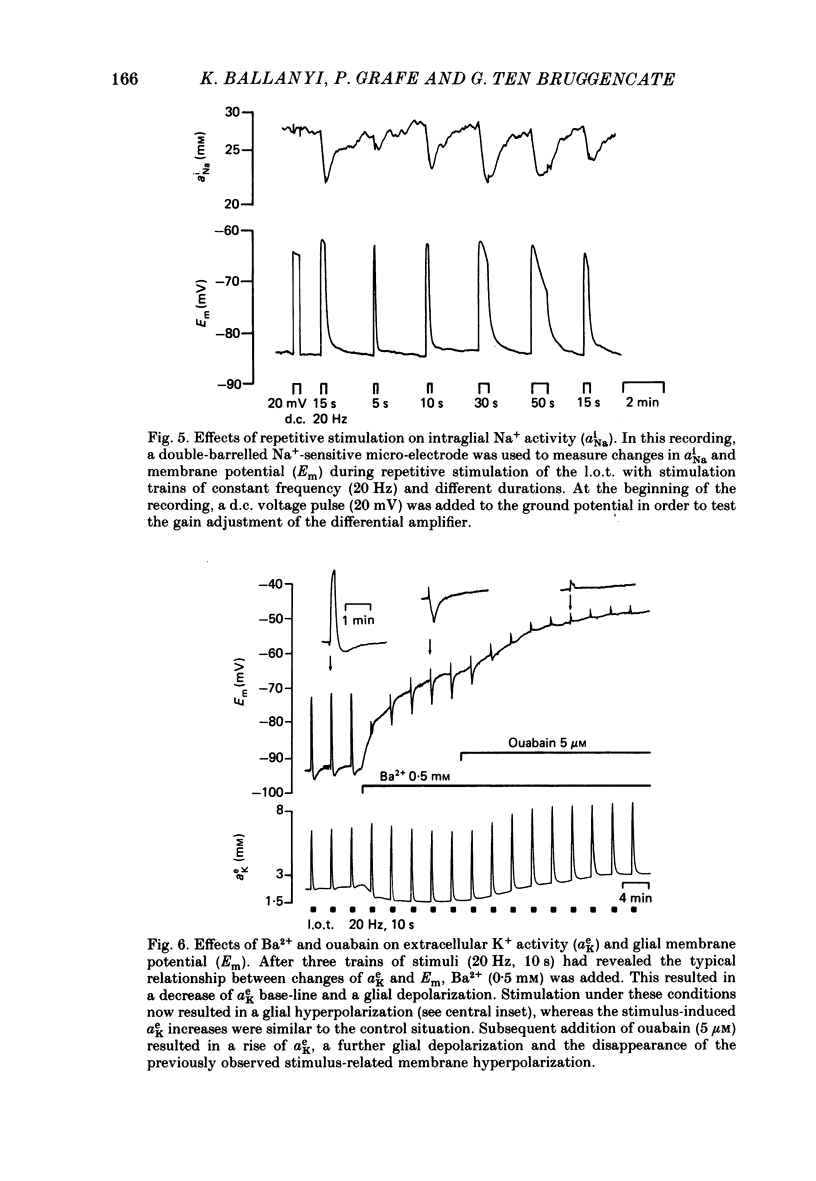
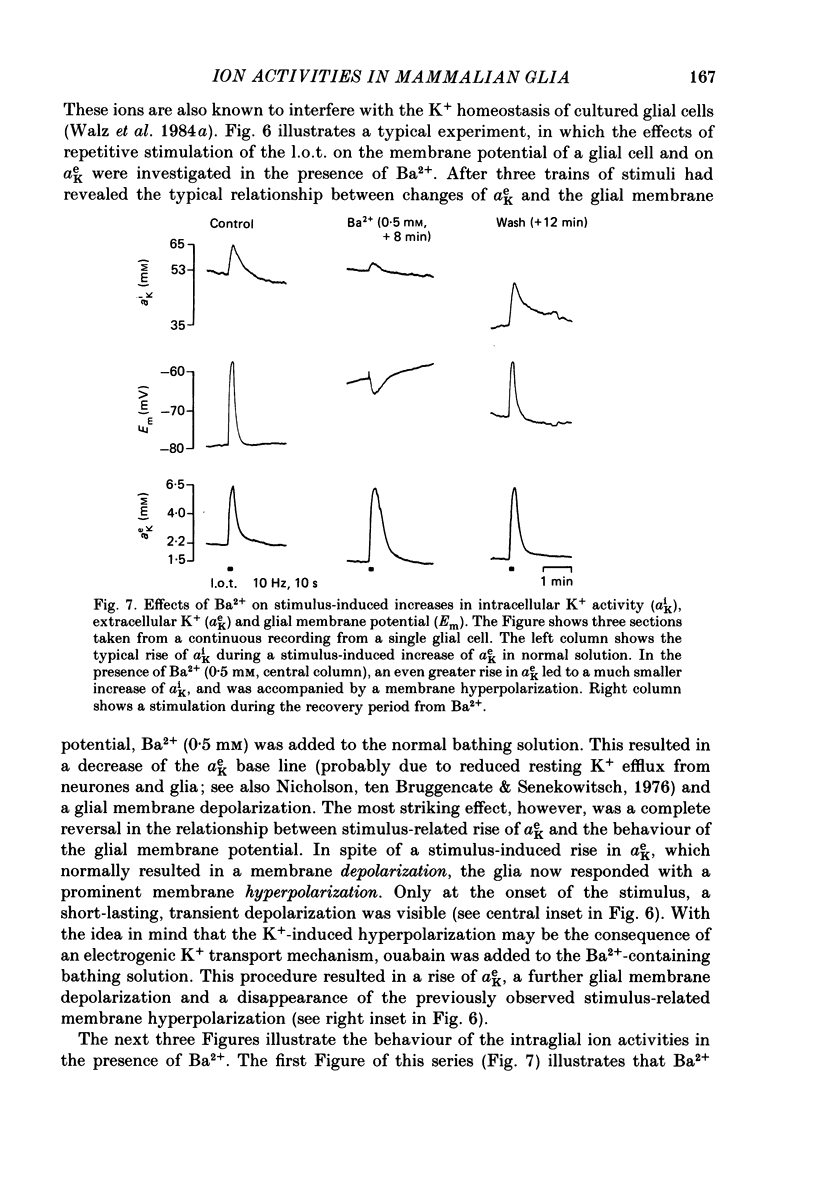
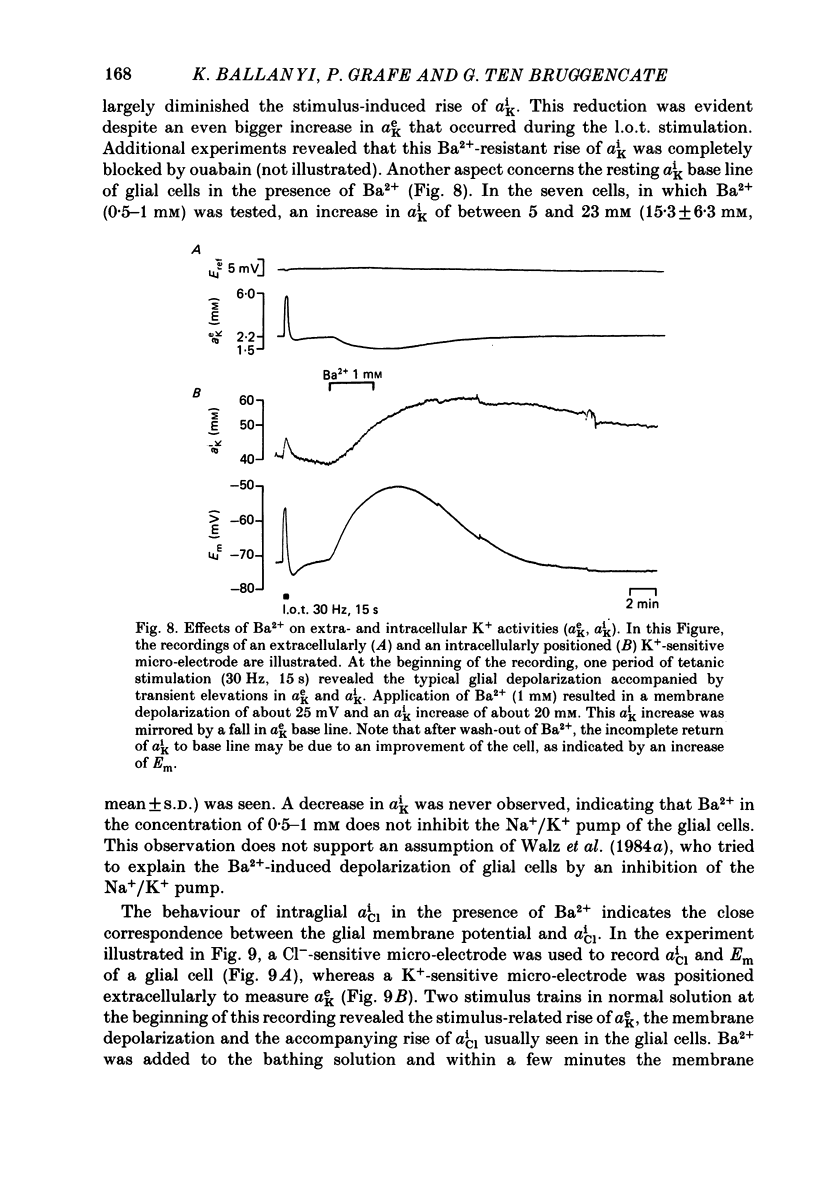
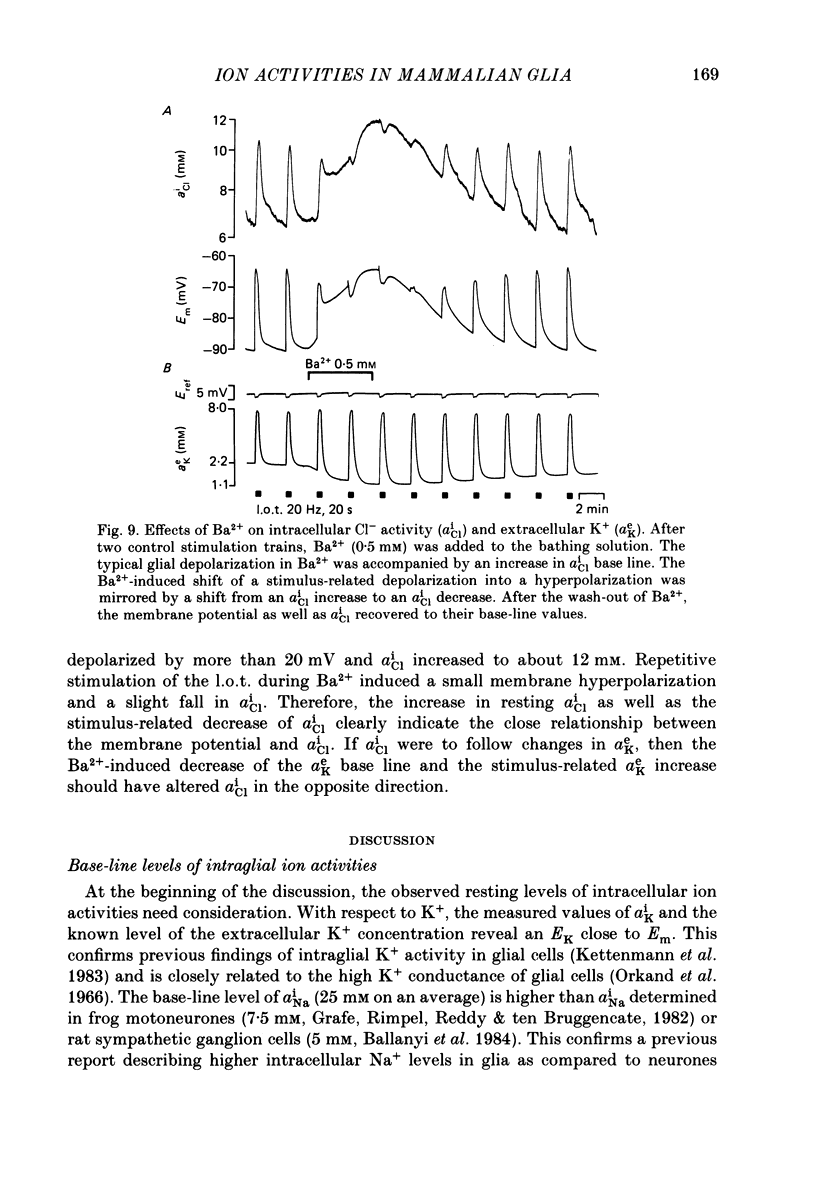
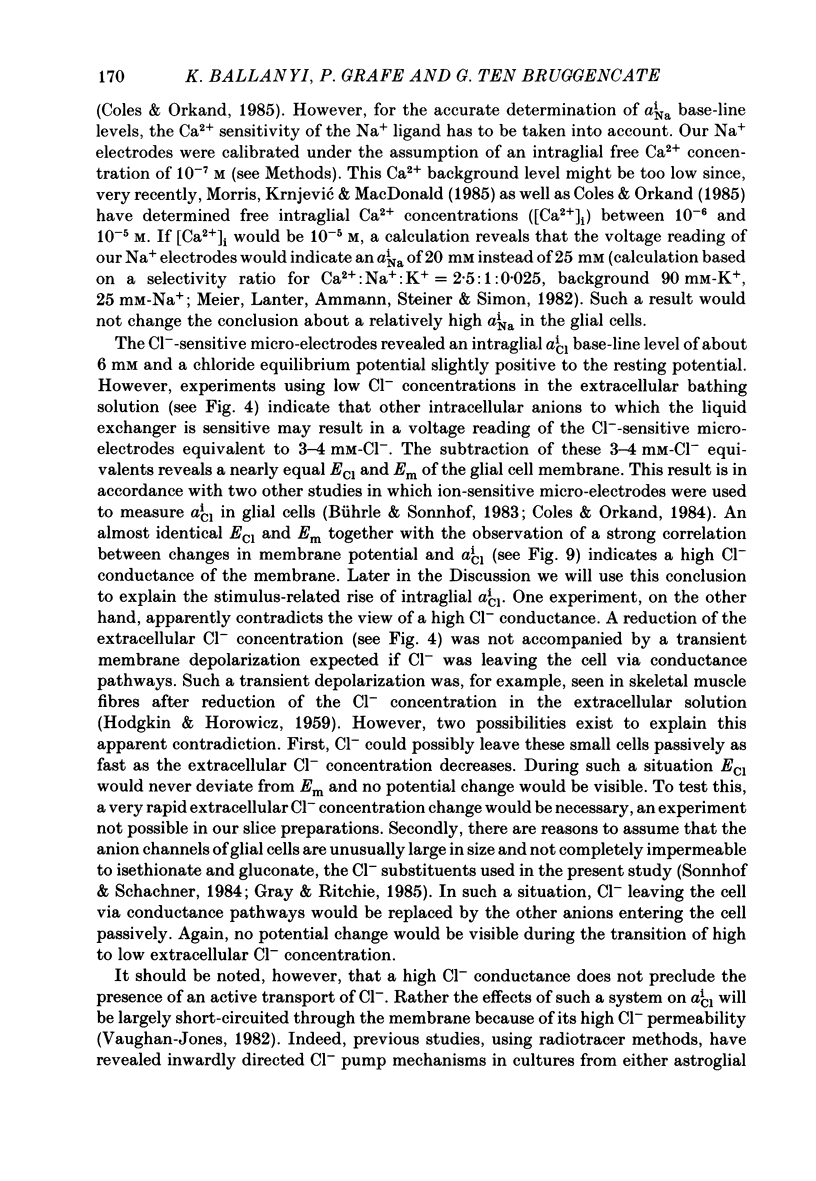
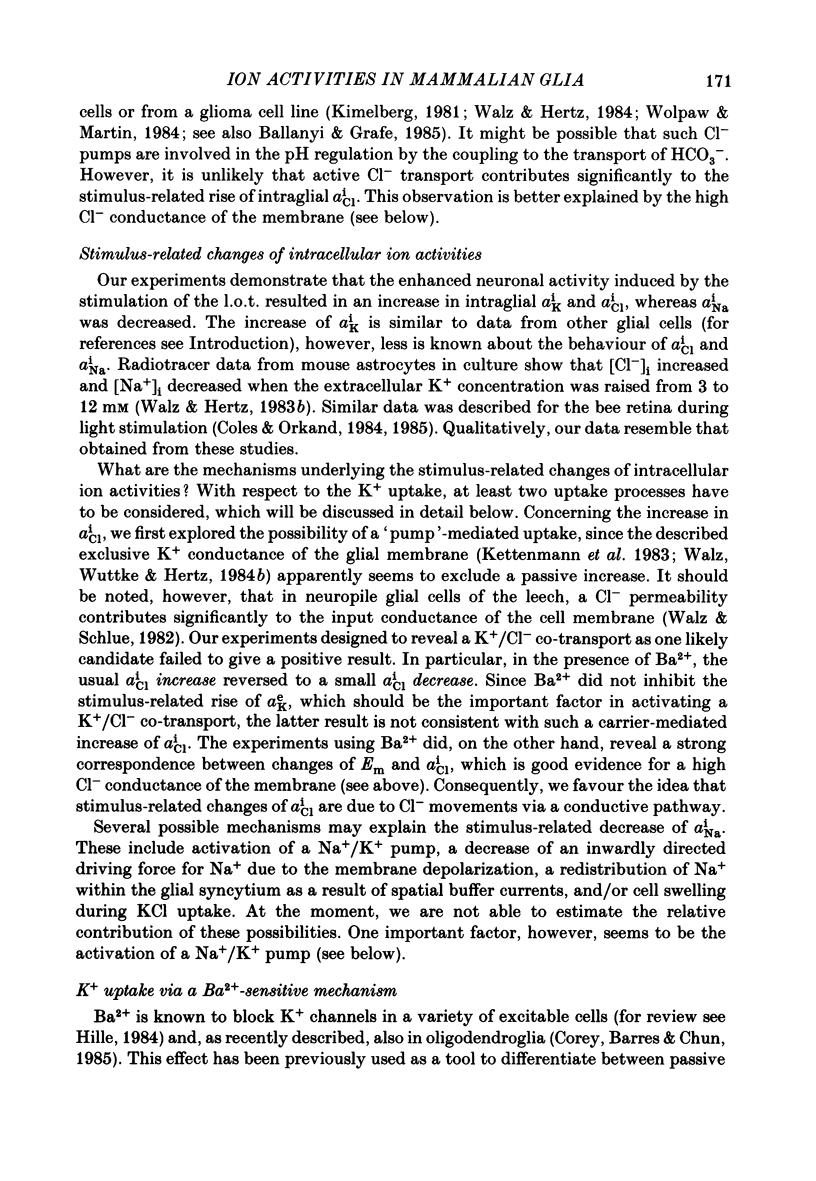
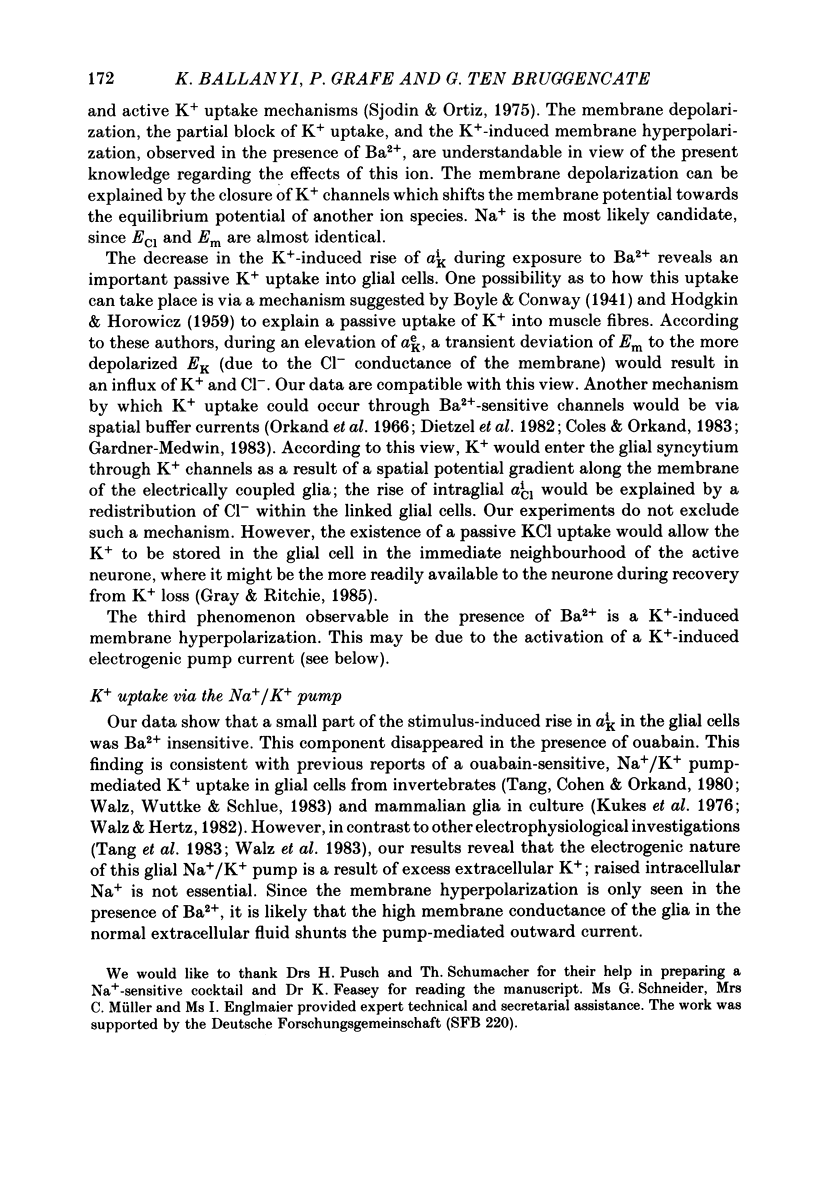
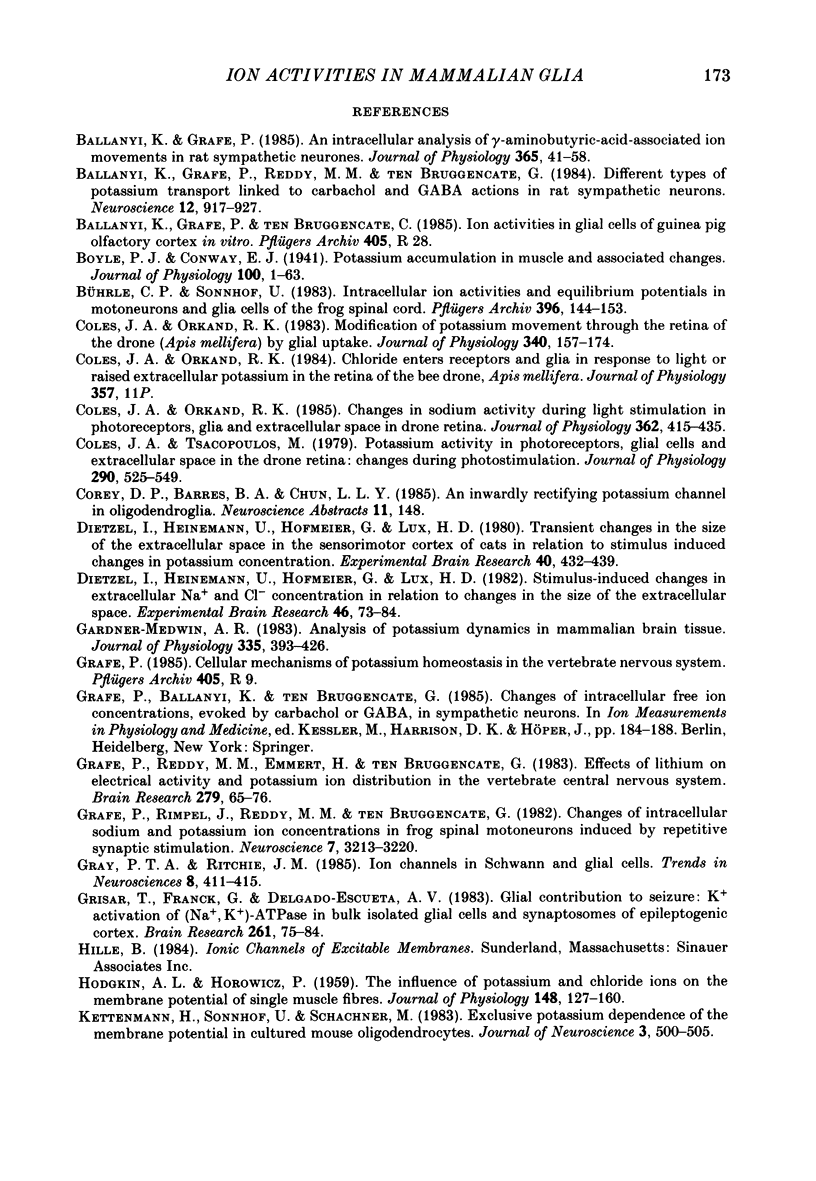
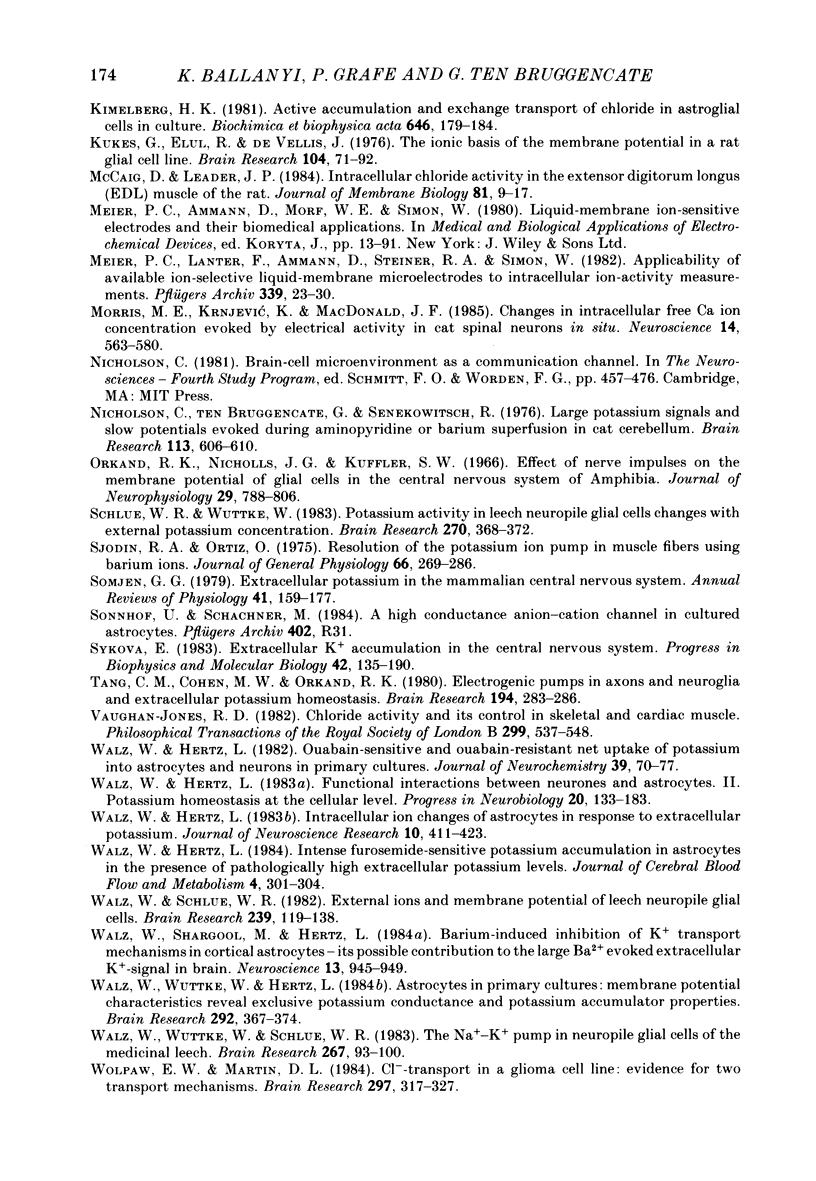
Selected References
These references are in PubMed. This may not be the complete list of references from this article.
- Ballanyi K., Grafe P. An intracellular analysis of gamma-aminobutyric-acid-associated ion movements in rat sympathetic neurones. J Physiol. 1985 Aug;365:41–58. doi: 10.1113/jphysiol.1985.sp015758. [DOI] [PMC free article] [PubMed] [Google Scholar]
- Ballanyi K., Grafe P., Reddy M. M., ten Bruggencate G. Different types of potassium transport linked to carbachol and gamma-aminobutyric acid actions in rat sympathetic neurons. Neuroscience. 1984 Jul;12(3):917–927. doi: 10.1016/0306-4522(84)90179-9. [DOI] [PubMed] [Google Scholar]
- Boyle P. J., Conway E. J. Potassium accumulation in muscle and associated changes. J Physiol. 1941 Aug 11;100(1):1–63. doi: 10.1113/jphysiol.1941.sp003922. [DOI] [PMC free article] [PubMed] [Google Scholar]
- Bührle C. P., Sonnhof U. Intracellular ion activities and equilibrium potentials in motoneurones and glia cells of the frog spinal cord. Pflugers Arch. 1983 Feb;396(2):144–153. doi: 10.1007/BF00615519. [DOI] [PubMed] [Google Scholar]
- Coles J. A., Orkand R. K. Changes in sodium activity during light stimulation in photoreceptors, glia and extracellular space in drone retina. J Physiol. 1985 May;362:415–435. doi: 10.1113/jphysiol.1985.sp015686. [DOI] [PMC free article] [PubMed] [Google Scholar]
- Coles J. A., Orkand R. K. Modification of potassium movement through the retina of the drone (Apis mellifera male) by glial uptake. J Physiol. 1983 Jul;340:157–174. doi: 10.1113/jphysiol.1983.sp014756. [DOI] [PMC free article] [PubMed] [Google Scholar]
- Coles J. A., Tsacopoulos M. Potassium activity in photoreceptors, glial cells and extracellular space in the drone retina: changes during photostimulation. J Physiol. 1979 May;290(2):525–549. doi: 10.1113/jphysiol.1979.sp012788. [DOI] [PMC free article] [PubMed] [Google Scholar]
- Dietzel I., Heinemann U., Hofmeier G., Lux H. D. Stimulus-induced changes in extracellular Na+ and Cl- concentration in relation to changes in the size of the extracellular space. Exp Brain Res. 1982;46(1):73–84. doi: 10.1007/BF00238100. [DOI] [PubMed] [Google Scholar]
- Dietzel I., Heinemann U., Hofmeier G., Lux H. D. Transient changes in the size of the extracellular space in the sensorimotor cortex of cats in relation to stimulus-induced changes in potassium concentration. Exp Brain Res. 1980;40(4):432–439. doi: 10.1007/BF00236151. [DOI] [PubMed] [Google Scholar]
- Dykes R. W., Terzis J. K., Turnbull B. G. Properties of mechanoreceptive fibres serving skin grafts transferred to the hands of adult baboons (Papio anubis). J Physiol. 1984 Dec;357:1–22. doi: 10.1113/jphysiol.1984.sp015485. [DOI] [PMC free article] [PubMed] [Google Scholar]
- Gardner-Medwin A. R. Analysis of potassium dynamics in mammalian brain tissue. J Physiol. 1983 Feb;335:393–426. doi: 10.1113/jphysiol.1983.sp014541. [DOI] [PMC free article] [PubMed] [Google Scholar]
- Grafe P., Reddy M. M., Emmert H., ten Bruggencate G. Effects of lithium on electrical activity and potassium ion distribution in the vertebrate central nervous system. Brain Res. 1983 Nov 21;279(1-2):65–76. doi: 10.1016/0006-8993(83)90163-4. [DOI] [PubMed] [Google Scholar]
- Grafe P., Rimpel J., Reddy M. M., ten Bruggencate G. Changes of intracellular sodium and potassium ion concentrations in frog spinal motoneurons induced by repetitive synaptic stimulation. Neuroscience. 1982;7(12):3213–3220. doi: 10.1016/0306-4522(82)90243-3. [DOI] [PubMed] [Google Scholar]
- Grisar T., Franck G., Delgado-Escueta A. V. Glial contribution to seizure: K+ activation of (Na+, K+)-ATPase in bulk isolated glial cells and synaptosomes of epileptogenic cortex. Brain Res. 1983 Feb 14;261(1):75–84. doi: 10.1016/0006-8993(83)91285-4. [DOI] [PubMed] [Google Scholar]
- HODGKIN A. L., HOROWICZ P. The influence of potassium and chloride ions on the membrane potential of single muscle fibres. J Physiol. 1959 Oct;148:127–160. doi: 10.1113/jphysiol.1959.sp006278. [DOI] [PMC free article] [PubMed] [Google Scholar]
- Kettenmann H., Sonnhof U., Schachner M. Exclusive potassium dependence of the membrane potential in cultured mouse oligodendrocytes. J Neurosci. 1983 Mar;3(3):500–505. doi: 10.1523/JNEUROSCI.03-03-00500.1983. [DOI] [PMC free article] [PubMed] [Google Scholar]
- Kimelberg H. K. Active accumulation and exchange transport of chloride in astroglial cells in culture. Biochim Biophys Acta. 1981 Aug 6;646(1):179–184. doi: 10.1016/0005-2736(81)90285-6. [DOI] [PubMed] [Google Scholar]
- Kukes G., Elul R., De Vellis J. The ionic basis of the membrane potential in a rat glial cell line. Brain Res. 1976 Mar 5;104(1):71–92. doi: 10.1016/0006-8993(76)90648-x. [DOI] [PubMed] [Google Scholar]
- McCaig D., Leader J. P. Intracellular chloride activity in the extensor digitorum longus (EDL) muscle of the rat. J Membr Biol. 1984;81(1):9–17. doi: 10.1007/BF01868805. [DOI] [PubMed] [Google Scholar]
- Meier P. C., Lanter F., Ammann D., Steiner R. A., Simon W. Applicability of available ion-selective liquid-membrane microelectrodes to intracellular ion-activity measurements. Pflugers Arch. 1982 Mar;393(1):23–30. doi: 10.1007/BF00582386. [DOI] [PubMed] [Google Scholar]
- Morris M. E., Krnjević K., MacDonald J. F. Changes in intracellular free Ca ion concentration evoked by electrical activity in cat spinal neurons in situ. Neuroscience. 1985 Feb;14(2):563–580. doi: 10.1016/0306-4522(85)90311-2. [DOI] [PubMed] [Google Scholar]
- Nicholson C., Bruggencate G. T., Senekowitsch R. Large potassium signals and slow potentials evoked during aminopyridine or barium superfusion in cat cerebellum. Brain Res. 1976 Sep 3;113(3):606–610. doi: 10.1016/0006-8993(76)90063-9. [DOI] [PubMed] [Google Scholar]
- Orkand R. K., Nicholls J. G., Kuffler S. W. Effect of nerve impulses on the membrane potential of glial cells in the central nervous system of amphibia. J Neurophysiol. 1966 Jul;29(4):788–806. doi: 10.1152/jn.1966.29.4.788. [DOI] [PubMed] [Google Scholar]
- Schlue W. R., Wuttke W. Potassium activity in leech neuropile glial cells changes with external potassium concentration. Brain Res. 1983 Jul 4;270(2):368–372. doi: 10.1016/0006-8993(83)90616-9. [DOI] [PubMed] [Google Scholar]
- Sjodin R. A., Ortiz O. Resolution of the potassium ion pump in muscle fibers using barium ions. J Gen Physiol. 1975 Sep;66(3):269–286. doi: 10.1085/jgp.66.3.269. [DOI] [PMC free article] [PubMed] [Google Scholar]
- Somjen G. G. Extracellular potassium in the mammalian central nervous system. Annu Rev Physiol. 1979;41:159–177. doi: 10.1146/annurev.ph.41.030179.001111. [DOI] [PubMed] [Google Scholar]
- Syková E. Extracellular K+ accumulation in the central nervous system. Prog Biophys Mol Biol. 1983;42(2-3):135–189. doi: 10.1016/0079-6107(83)90006-8. [DOI] [PubMed] [Google Scholar]
- Tang C. M., Cohen M. W., Orkand R. K. Electrogenic pumps in axons and neuroglia and extracellular potassium homeostasis. Brain Res. 1980 Jul 21;194(1):283–286. doi: 10.1016/0006-8993(80)91345-1. [DOI] [PubMed] [Google Scholar]
- Vaughan-Jones R. D. Chloride activity and its control in skeletal and cardiac muscle. Philos Trans R Soc Lond B Biol Sci. 1982 Dec 1;299(1097):537–548. doi: 10.1098/rstb.1982.0150. [DOI] [PubMed] [Google Scholar]
- Walz W., Hertz L. Functional interactions between neurons and astrocytes. II. Potassium homeostasis at the cellular level. Prog Neurobiol. 1983;20(1-2):133–183. doi: 10.1016/0301-0082(83)90013-8. [DOI] [PubMed] [Google Scholar]
- Walz W., Hertz L. Intense furosemide-sensitive potassium accumulation in astrocytes in the presence of pathologically high extracellular potassium levels. J Cereb Blood Flow Metab. 1984 Jun;4(2):301–304. doi: 10.1038/jcbfm.1984.42. [DOI] [PubMed] [Google Scholar]
- Walz W., Hertz L. Intracellular ion changes of astrocytes in response to extracellular potassium. J Neurosci Res. 1983;10(4):411–423. doi: 10.1002/jnr.490100408. [DOI] [PubMed] [Google Scholar]
- Walz W., Hertz L. Ouabain-sensitive and ouabain-resistant net uptake of potassium into astrocytes and neurons in primary cultures. J Neurochem. 1982 Jul;39(1):70–77. doi: 10.1111/j.1471-4159.1982.tb04702.x. [DOI] [PubMed] [Google Scholar]
- Walz W., Schlue W. R. External ions and membrane potential of leech neuropile glial cells. Brain Res. 1982 May 6;239(1):119–138. doi: 10.1016/0006-8993(82)90837-x. [DOI] [PubMed] [Google Scholar]
- Walz W., Shargool M., Hertz L. Barium-induced inhibition of K+ transport mechanisms in cortical astrocytes--its possible contribution to the large Ba2+-evoked extracellular K+ signal in brain. Neuroscience. 1984 Nov;13(3):945–949. doi: 10.1016/0306-4522(84)90108-8. [DOI] [PubMed] [Google Scholar]
- Walz W., Wuttke W., Hertz L. Astrocytes in primary cultures: membrane potential characteristics reveal exclusive potassium conductance and potassium accumulator properties. Brain Res. 1984 Feb 6;292(2):367–374. doi: 10.1016/0006-8993(84)90772-8. [DOI] [PubMed] [Google Scholar]
- Walz W., Wuttke W., Schlue W. R. The Na+-K+ pump in neuropile glial cells of the medicinal leech. Brain Res. 1983 May 9;267(1):93–100. doi: 10.1016/0006-8993(83)91042-9. [DOI] [PubMed] [Google Scholar]
- Wolpaw E. W., Martin D. L. Cl- transport in a glioma cell line: evidence for two transport mechanisms. Brain Res. 1984 Apr 16;297(2):317–327. doi: 10.1016/0006-8993(84)90573-0. [DOI] [PubMed] [Google Scholar]