Abstract
1. Intracellular recordings were obtained from taste receptor cells and surface epithelial cells of isolated mudpuppy lingual epithelium. 2. Surface epithelial cells had a mean resting potential of -40.2 +/- 8.9 mV, a mean input resistance of 40.3 +/- 11.3 M omega, and a linear current-voltage (I-V) relationship. Taste receptor cells had a mean resting potential of -61.7 +/- 15 mV, a mean input resistance of 380.3 +/- 177.2 M omega, and the I-V relationship showed pronounced outward rectification; the outward rectification persisted in high-K+ saline, but was abolished by tetraethylammonium bromide (TEA). 3. Surface epithelial cells responded to depolarizing current injection with only passive membrane potential changes. Taste receptor cells responded to brief pulses of depolarizing current injection with regenerative action potentials characterized by an abrupt rising phase, an inflexion on the falling phase, and a prolonged after-potential. 4. The abrupt rising phase of the action potential was blocked by tetrodotoxin (TTX), suggesting that voltage-gated Na+ currents are responsible for the rising phase. 5. Long-duration action potentials were elicited from cells treated with TEA to block outward K+ currents and with TTX to block Na+ currents, and from cells bathed in isotonic CaCl2. These results suggest that the active membrane response contains a significant Ca2+ component. 6. The after-potential was blocked or greatly reduced by the addition of Ca2+ channel blockers to the bathing medium. In contrast, addition of TEA to the bathing medium greatly enhanced the after-potential. These data suggest that a significant portion of the after-potential is Ca2+ mediated. 7. The mean reversal potential for the after-potential (-76.8 +/- 6.0 mV) was significantly different from the mean reversal potential for the undershoot of the action potential (-86 +/- 5.6 mV). Superfusion with TEA reduced the reversal potential of the after-potential to -42.3 +/- 8.2 mV and abolished the undershoot. These results suggest that the after-potential results from at least two conductances, one which is blocked by TEA and the other which is Ca2+ dependent and involves ions other than, or in addition to K+. 8. Our data suggest that taste receptor cells, unlike surface epithelial cells, possess voltage-gated Na+, Ca2+, and K+ channels, as well as Ca2+-mediated channels. The role of the Ca2+ channels may be in part to regulate release of transmitter onto nerve terminals. The role of the other conductances in taste transduction is unknown.
Full text
PDF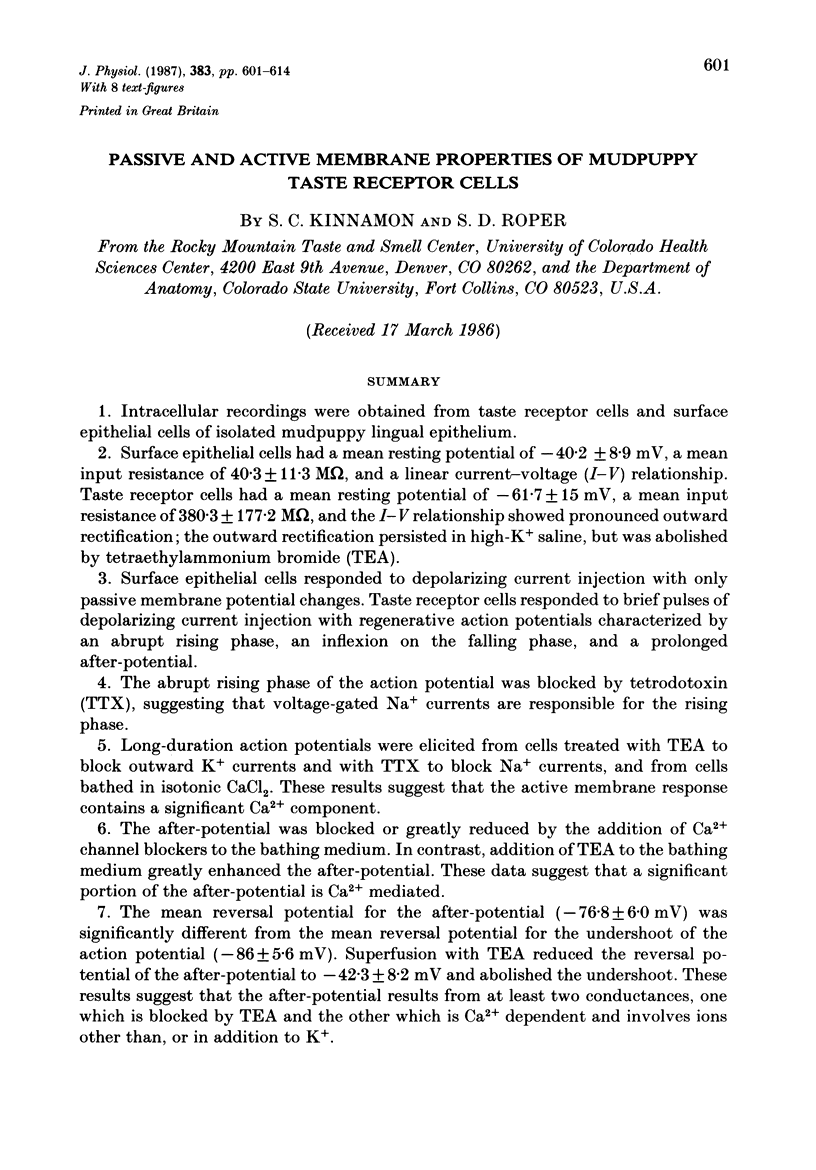
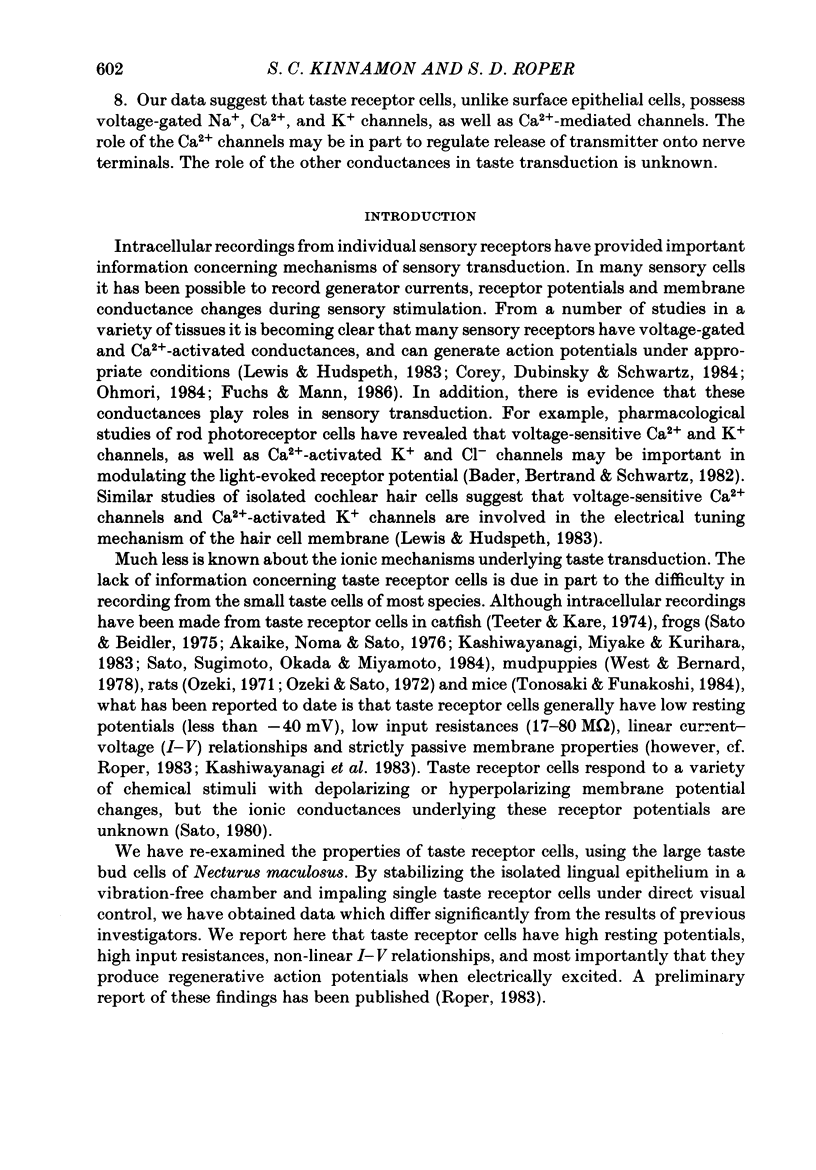
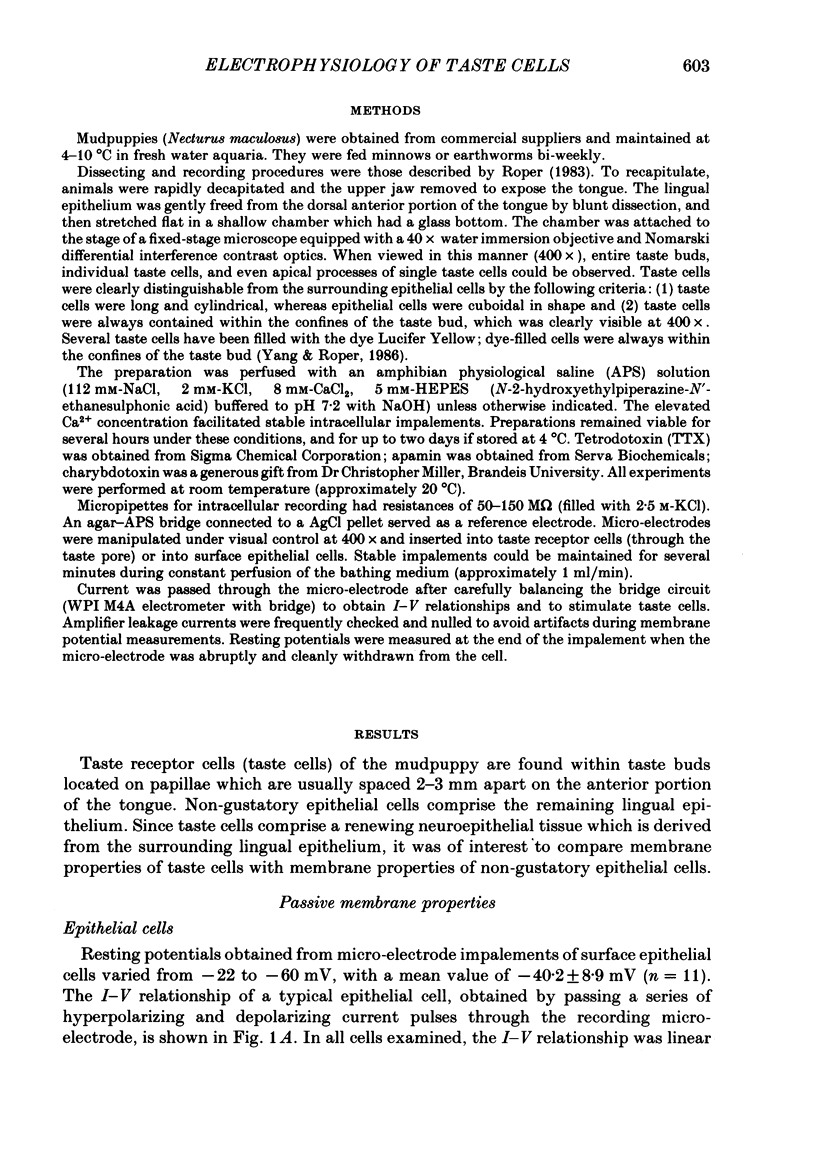
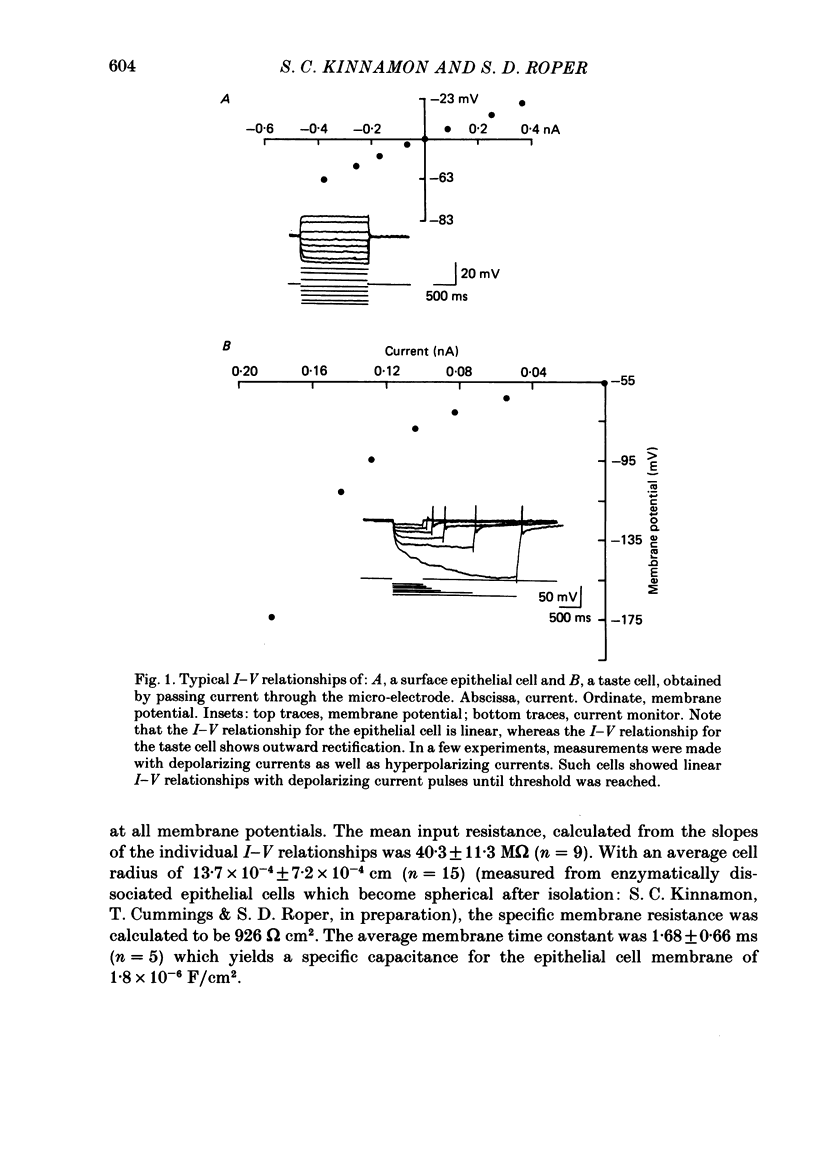
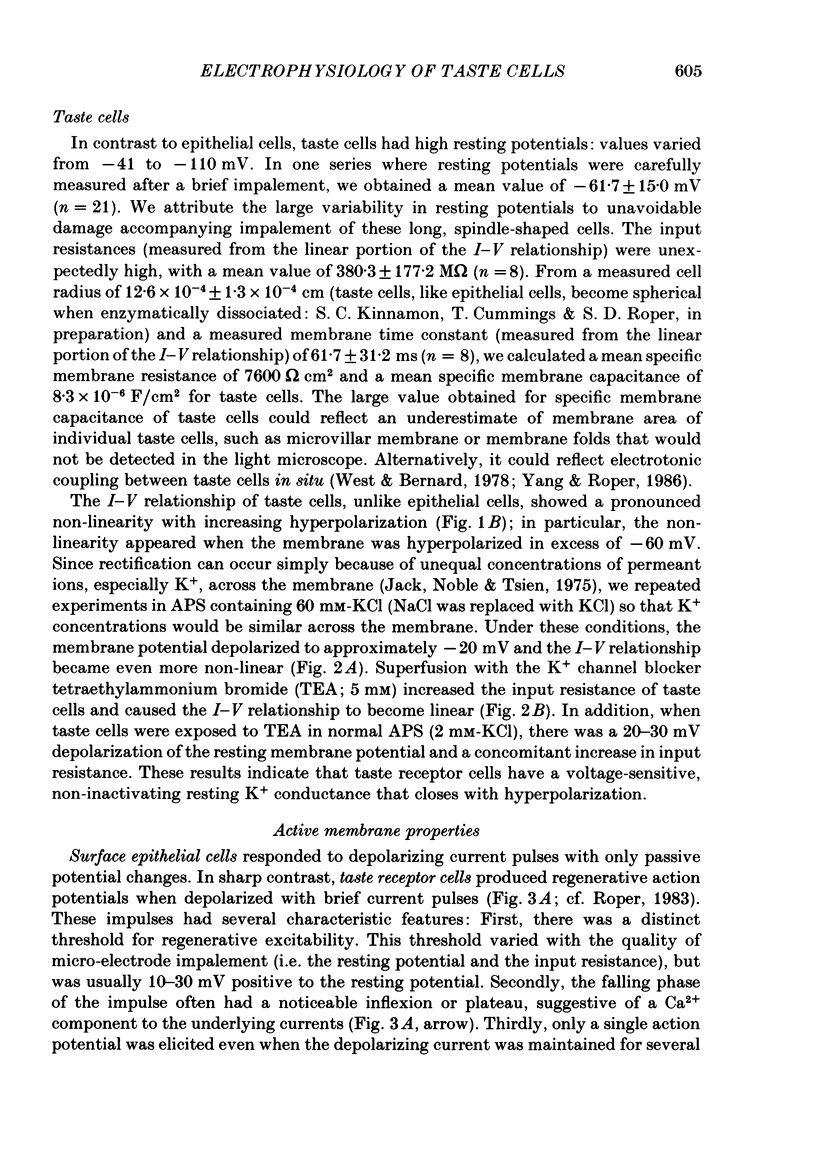
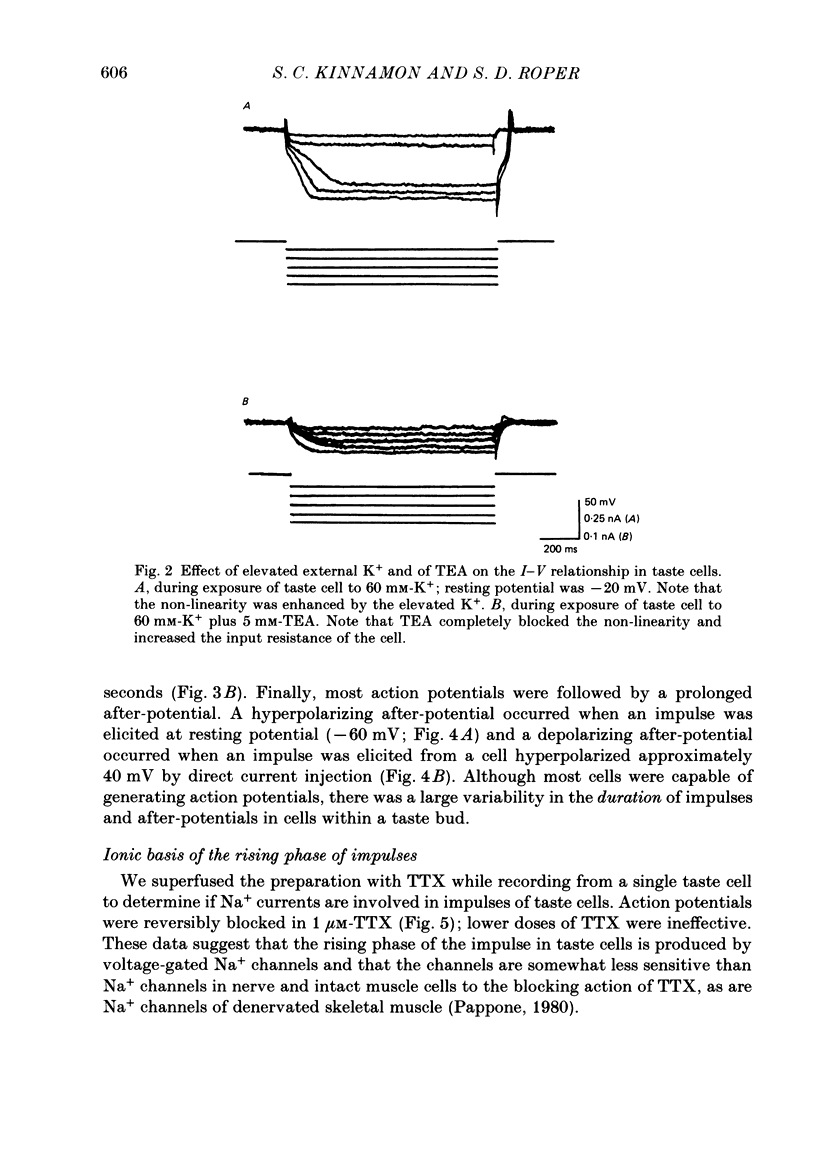
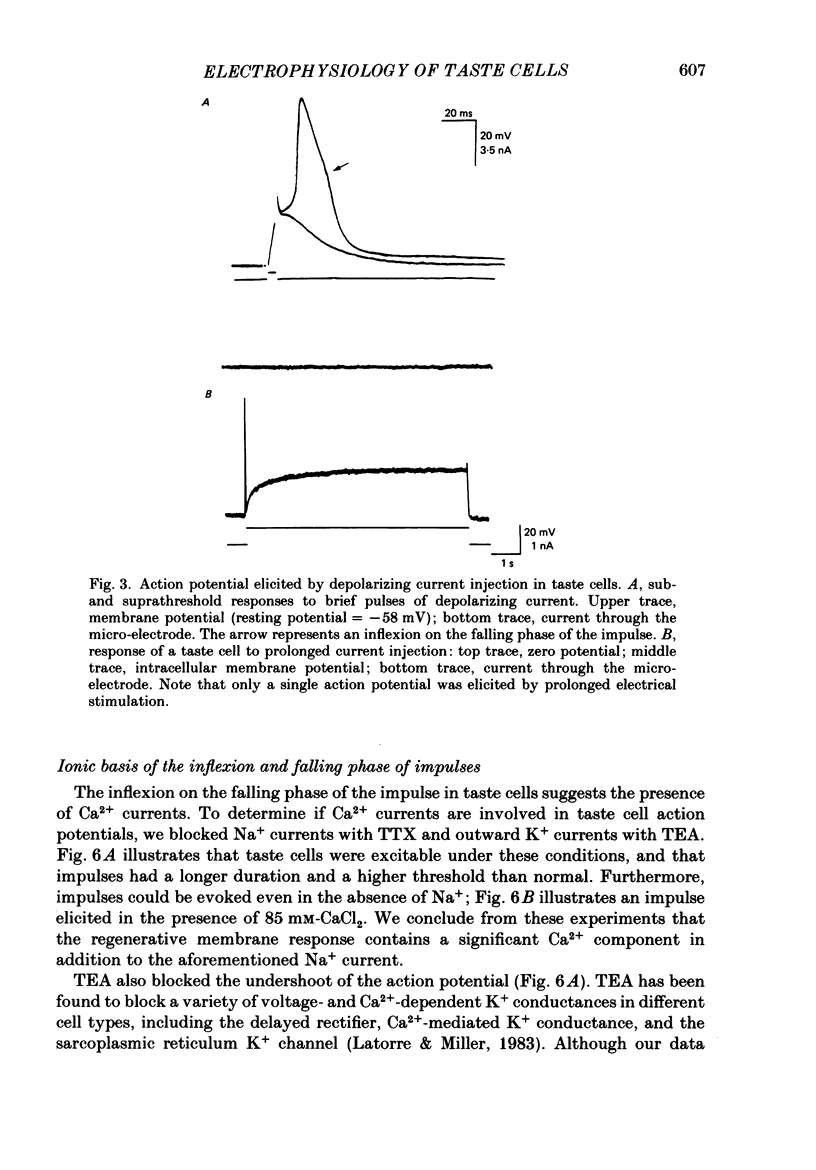
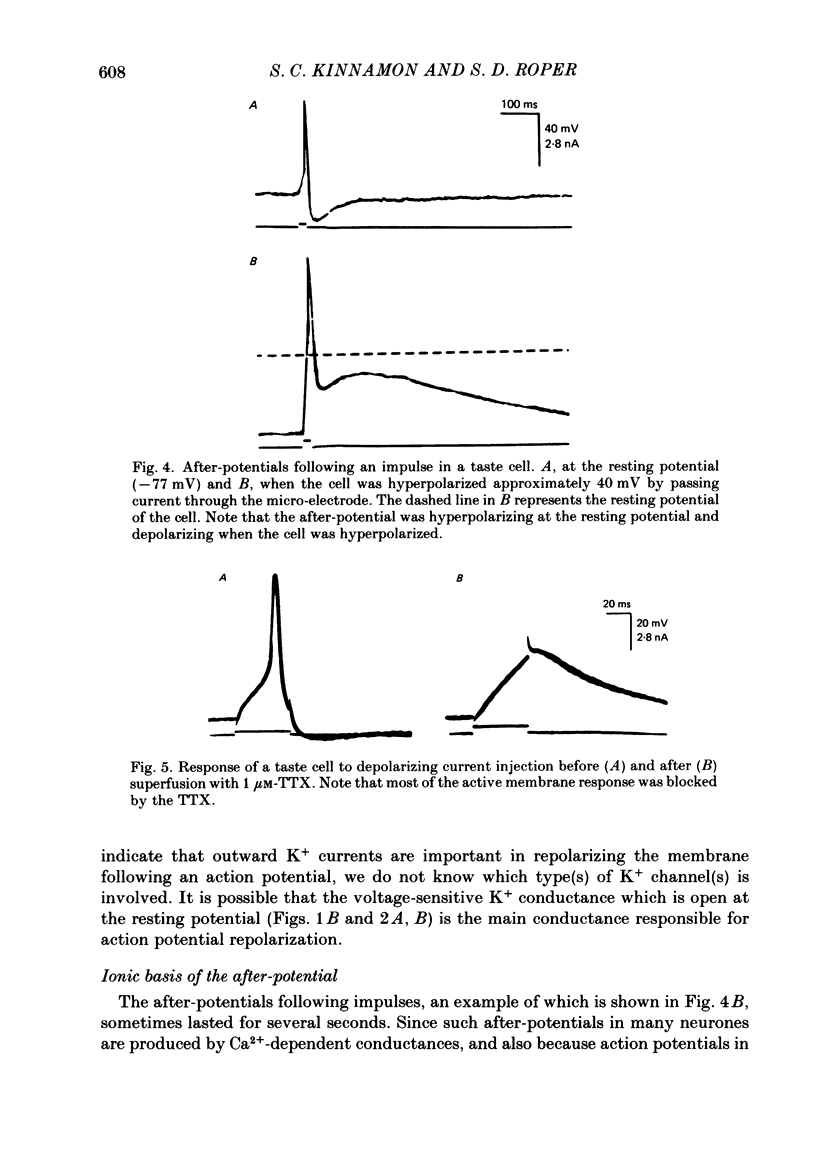
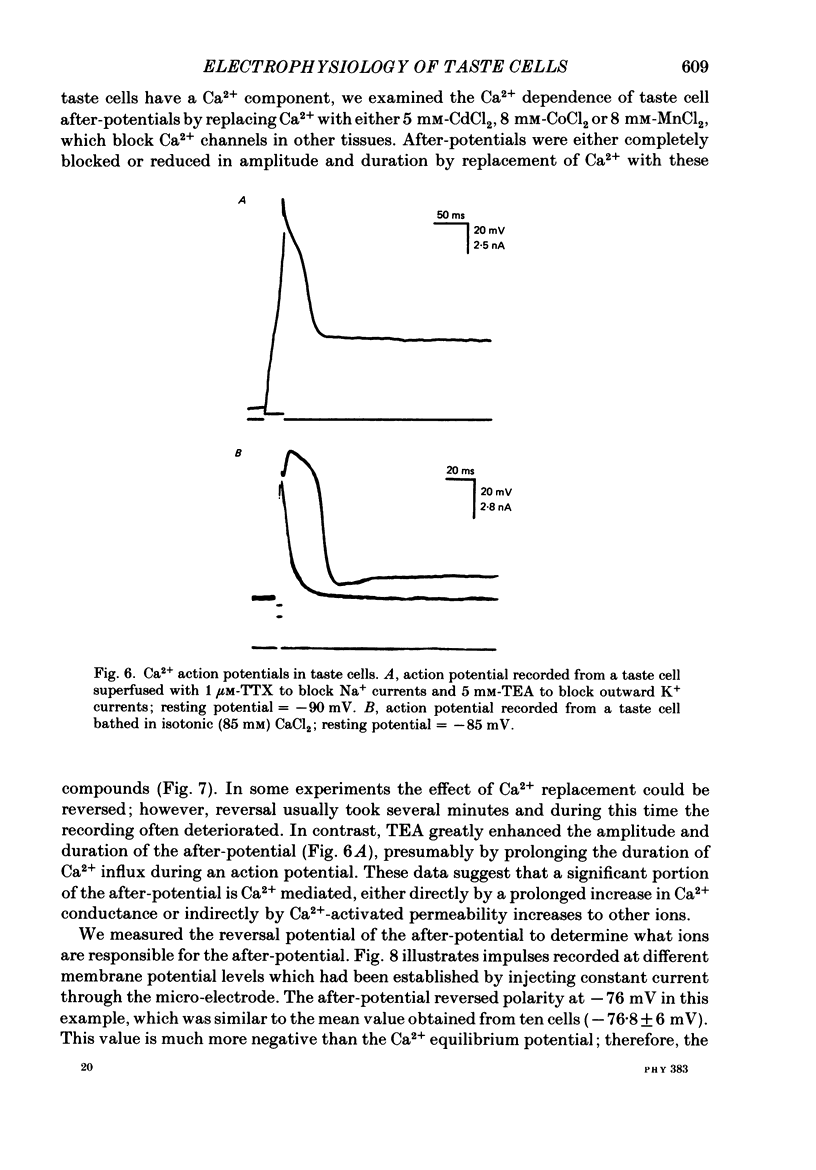
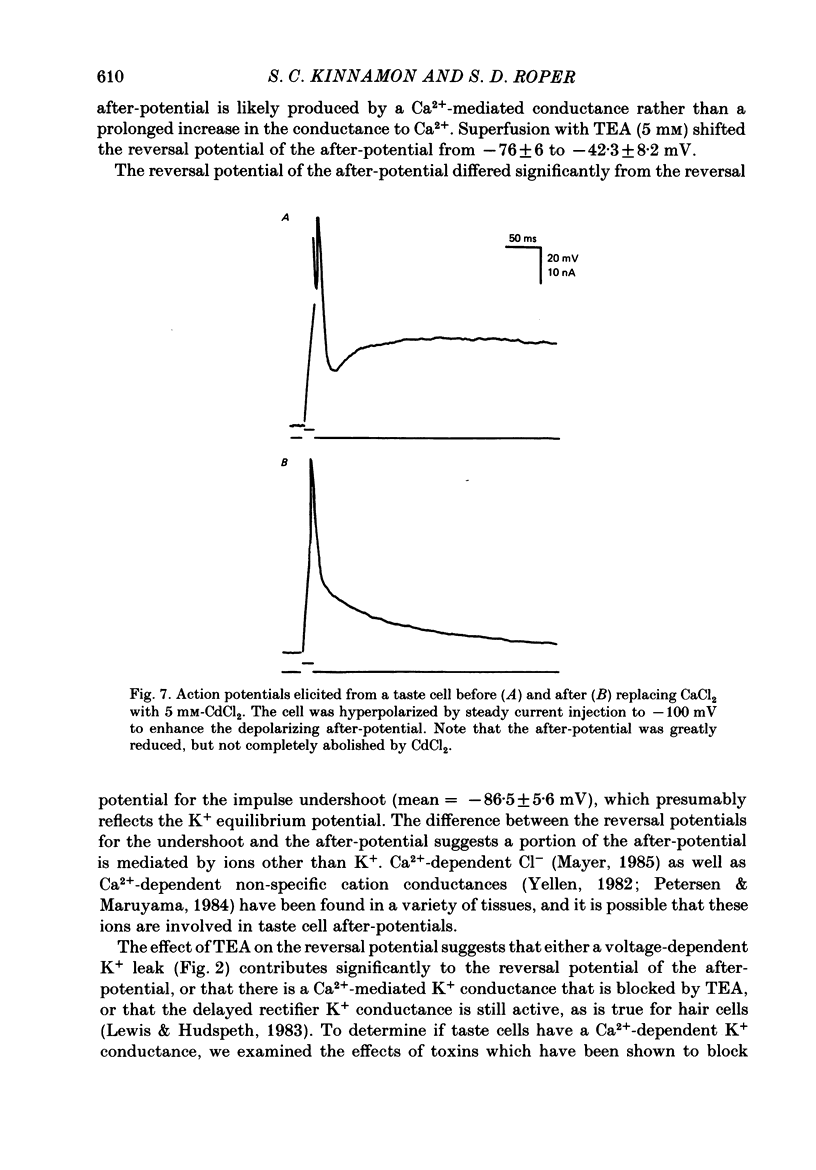
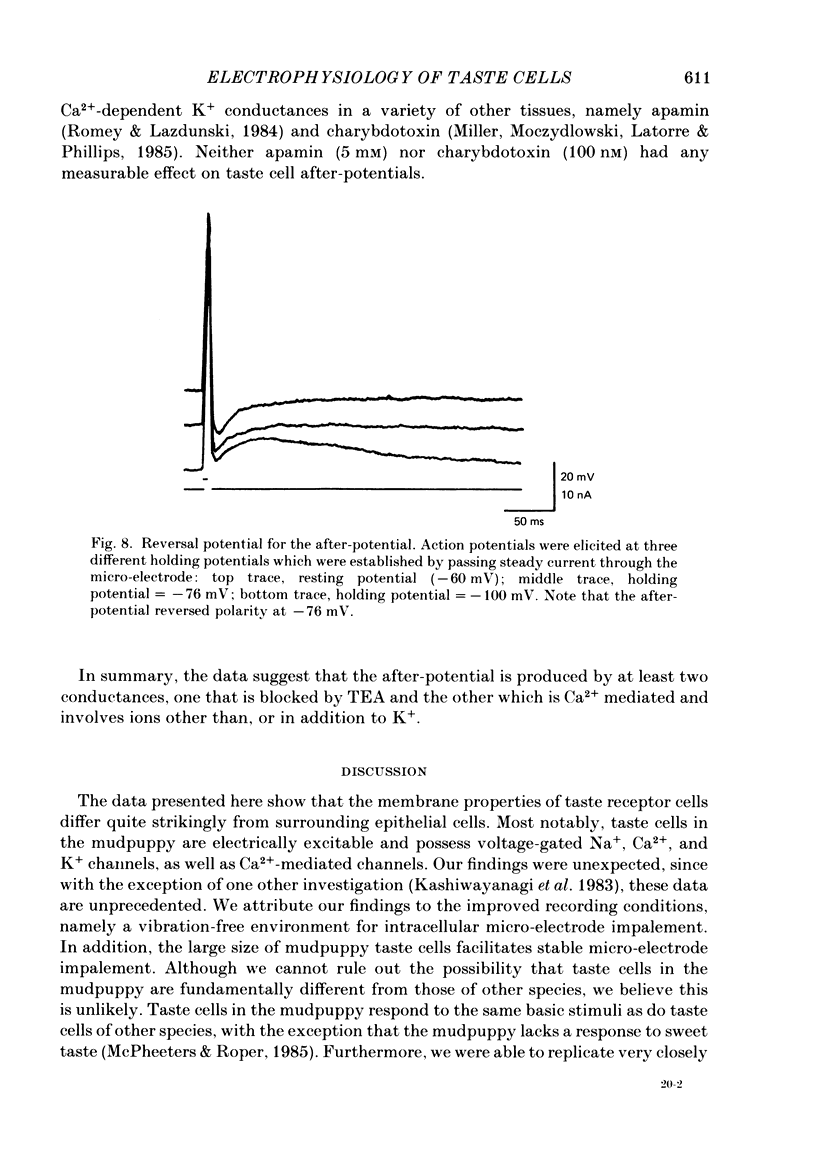
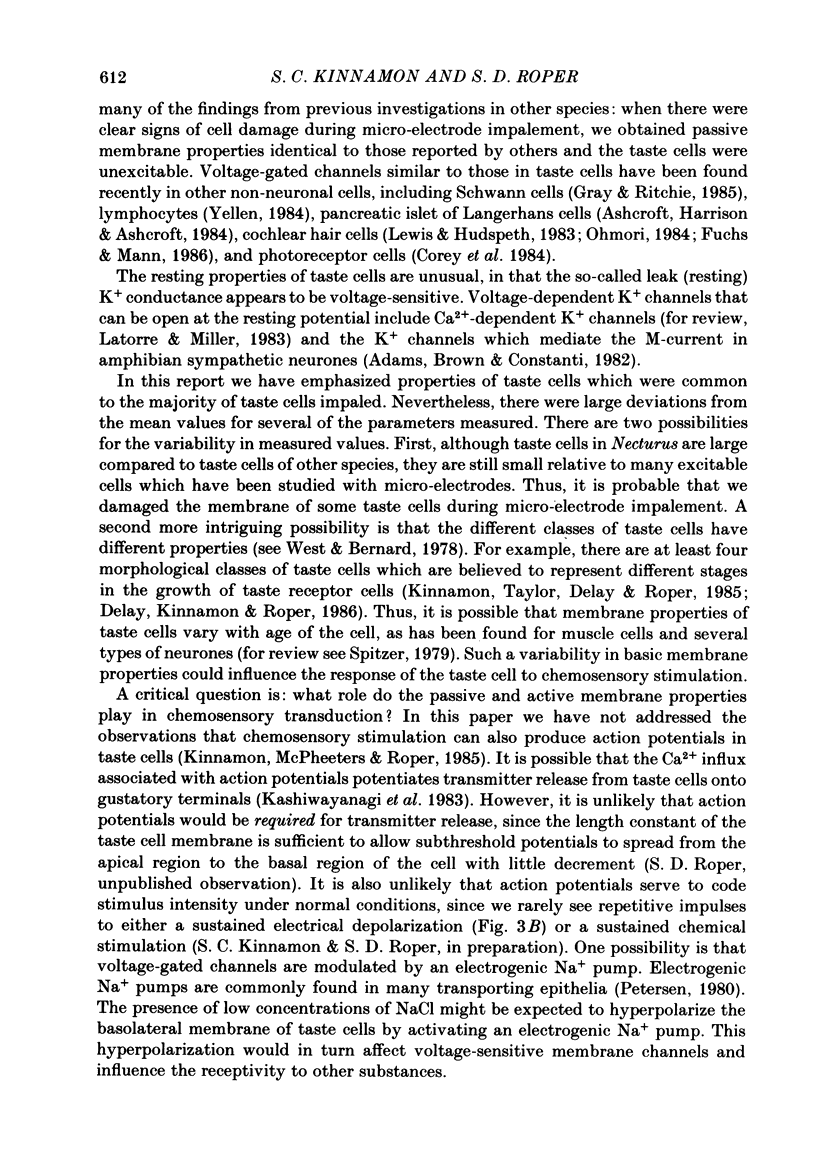
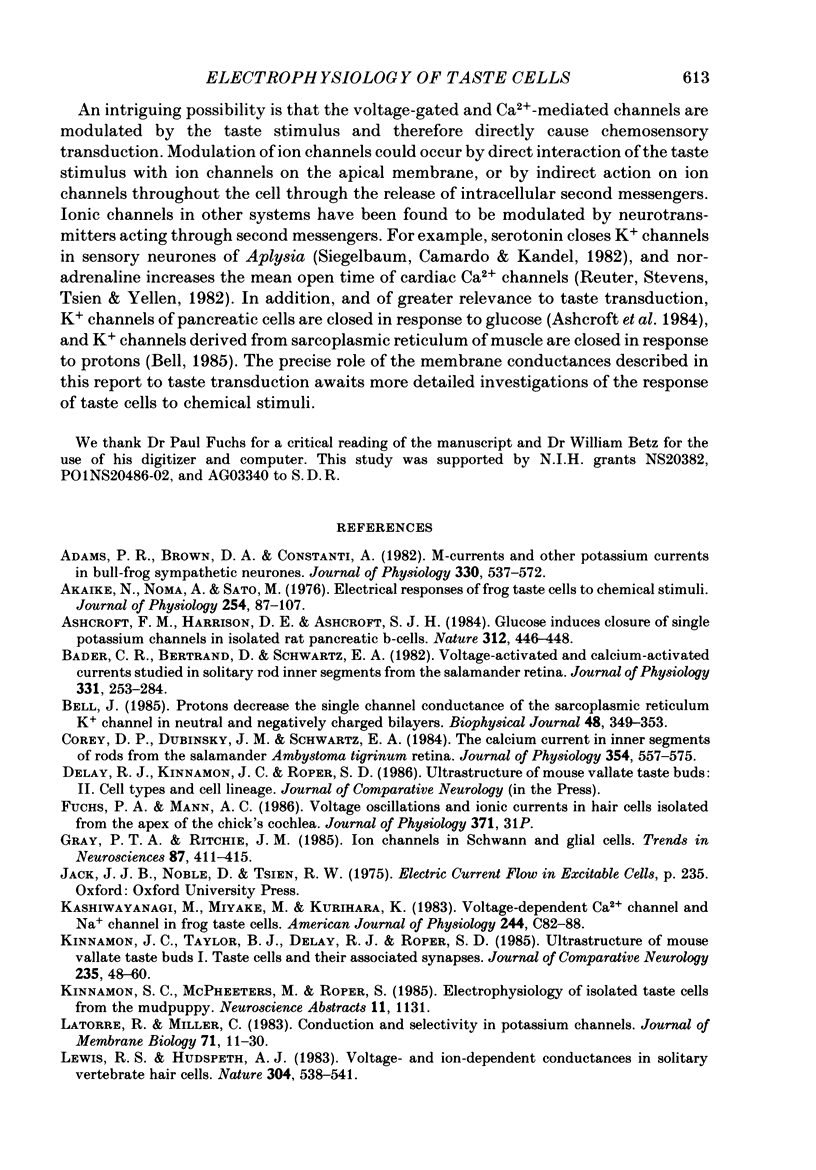
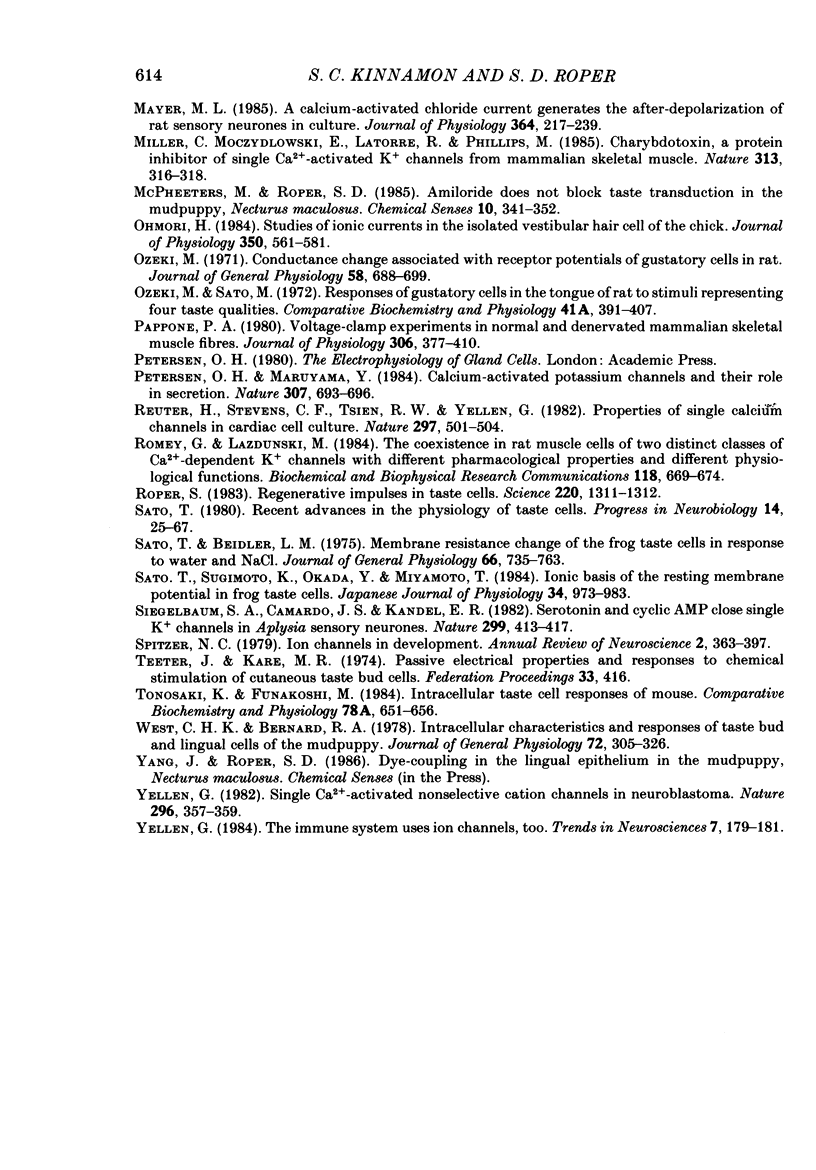
Selected References
These references are in PubMed. This may not be the complete list of references from this article.
- Adams P. R., Brown D. A., Constanti A. M-currents and other potassium currents in bullfrog sympathetic neurones. J Physiol. 1982 Sep;330:537–572. doi: 10.1113/jphysiol.1982.sp014357. [DOI] [PMC free article] [PubMed] [Google Scholar]
- Akaike N., Noma A., Sato M. Electrical responses to frog taste cells to chemical stimuli. J Physiol. 1976 Jan;254(1):87–107. doi: 10.1113/jphysiol.1976.sp011223. [DOI] [PMC free article] [PubMed] [Google Scholar]
- Ashcroft F. M., Harrison D. E., Ashcroft S. J. Glucose induces closure of single potassium channels in isolated rat pancreatic beta-cells. 1984 Nov 29-Dec 5Nature. 312(5993):446–448. doi: 10.1038/312446a0. [DOI] [PubMed] [Google Scholar]
- Bader C. R., Bertrand D., Schwartz E. A. Voltage-activated and calcium-activated currents studied in solitary rod inner segments from the salamander retina. J Physiol. 1982 Oct;331:253–284. doi: 10.1113/jphysiol.1982.sp014372. [DOI] [PMC free article] [PubMed] [Google Scholar]
- Bell J. Protons decrease the single channel conductance of the sarcoplasmic reticulum K+ channel in neutral and negatively charged bilayers. Biophys J. 1985 Aug;48(2):349–353. doi: 10.1016/S0006-3495(85)83790-5. [DOI] [PMC free article] [PubMed] [Google Scholar]
- Corey D. P., Dubinsky J. M., Schwartz E. A. The calcium current in inner segments of rods from the salamander (Ambystoma tigrinum) retina. J Physiol. 1984 Sep;354:557–575. doi: 10.1113/jphysiol.1984.sp015393. [DOI] [PMC free article] [PubMed] [Google Scholar]
- Kashiwayanagi M., Miyake M., Kurihara K. Voltage-dependent Ca2+ channel and Na+ channel in frog taste cells. Am J Physiol. 1983 Jan;244(1):C82–C88. doi: 10.1152/ajpcell.1983.244.1.C82. [DOI] [PubMed] [Google Scholar]
- Kinnamon J. C., Taylor B. J., Delay R. J., Roper S. D. Ultrastructure of mouse vallate taste buds. I. Taste cells and their associated synapses. J Comp Neurol. 1985 May 1;235(1):48–60. doi: 10.1002/cne.902350105. [DOI] [PubMed] [Google Scholar]
- Latorre R., Miller C. Conduction and selectivity in potassium channels. J Membr Biol. 1983;71(1-2):11–30. doi: 10.1007/BF01870671. [DOI] [PubMed] [Google Scholar]
- Lewis R. S., Hudspeth A. J. Voltage- and ion-dependent conductances in solitary vertebrate hair cells. Nature. 1983 Aug 11;304(5926):538–541. doi: 10.1038/304538a0. [DOI] [PubMed] [Google Scholar]
- Mayer M. L. A calcium-activated chloride current generates the after-depolarization of rat sensory neurones in culture. J Physiol. 1985 Jul;364:217–239. doi: 10.1113/jphysiol.1985.sp015740. [DOI] [PMC free article] [PubMed] [Google Scholar]
- Miller C., Moczydlowski E., Latorre R., Phillips M. Charybdotoxin, a protein inhibitor of single Ca2+-activated K+ channels from mammalian skeletal muscle. Nature. 1985 Jan 24;313(6000):316–318. doi: 10.1038/313316a0. [DOI] [PubMed] [Google Scholar]
- Ohmori H. Studies of ionic currents in the isolated vestibular hair cell of the chick. J Physiol. 1984 May;350:561–581. doi: 10.1113/jphysiol.1984.sp015218. [DOI] [PMC free article] [PubMed] [Google Scholar]
- Ozeki M. Conductance change associated with receptor potentials of gustatory cells in rat. J Gen Physiol. 1971 Dec;58(6):688–699. doi: 10.1085/jgp.58.6.688. [DOI] [PMC free article] [PubMed] [Google Scholar]
- Ozeki M., Sato M. Responses of gustatory cells in the tongue of rat to stimuli representing four taste qualities. Comp Biochem Physiol A Comp Physiol. 1972 Feb 1;41(2):391–407. doi: 10.1016/0300-9629(72)90070-9. [DOI] [PubMed] [Google Scholar]
- Pappone P. A. Voltage-clamp experiments in normal and denervated mammalian skeletal muscle fibres. J Physiol. 1980 Sep;306:377–410. doi: 10.1113/jphysiol.1980.sp013403. [DOI] [PMC free article] [PubMed] [Google Scholar]
- Petersen O. H., Maruyama Y. Calcium-activated potassium channels and their role in secretion. Nature. 1984 Feb 23;307(5953):693–696. doi: 10.1038/307693a0. [DOI] [PubMed] [Google Scholar]
- Reuter H., Stevens C. F., Tsien R. W., Yellen G. Properties of single calcium channels in cardiac cell culture. Nature. 1982 Jun 10;297(5866):501–504. doi: 10.1038/297501a0. [DOI] [PubMed] [Google Scholar]
- Romey G., Lazdunski M. The coexistence in rat muscle cells of two distinct classes of Ca2+-dependent K+ channels with different pharmacological properties and different physiological functions. Biochem Biophys Res Commun. 1984 Jan 30;118(2):669–674. doi: 10.1016/0006-291x(84)91355-x. [DOI] [PubMed] [Google Scholar]
- Roper S. Regenerative impulses in taste cells. Science. 1983 Jun 17;220(4603):1311–1312. doi: 10.1126/science.6857254. [DOI] [PubMed] [Google Scholar]
- Sato T., Beidler L. M. Membrane resistance change of the frog taste cells in response to water and Nacl. J Gen Physiol. 1975 Dec;66(6):735–763. doi: 10.1085/jgp.66.6.735. [DOI] [PMC free article] [PubMed] [Google Scholar]
- Sato T. Recent advances in the physiology of taste cells. Prog Neurobiol. 1980;14(1):25–67. doi: 10.1016/0301-0082(80)90003-9. [DOI] [PubMed] [Google Scholar]
- Sato T., Sugimoto K., Okada Y., Miyamoto T. Ionic basis of resting membrane potential in frog taste cells. Jpn J Physiol. 1984;34(6):973–983. doi: 10.2170/jjphysiol.34.973. [DOI] [PubMed] [Google Scholar]
- Siegelbaum S. A., Camardo J. S., Kandel E. R. Serotonin and cyclic AMP close single K+ channels in Aplysia sensory neurones. Nature. 1982 Sep 30;299(5882):413–417. doi: 10.1038/299413a0. [DOI] [PubMed] [Google Scholar]
- Tonosaki K., Funakoshi M. Intracellular taste cell responses of mouse. Comp Biochem Physiol A Comp Physiol. 1984;78(4):651–656. doi: 10.1016/0300-9629(84)90611-x. [DOI] [PubMed] [Google Scholar]
- West C. H., Bernard R. A. Intracellular characteristics and responses of taste bud and lingual cells of the mudpuppy. J Gen Physiol. 1978 Sep;72(3):305–326. doi: 10.1085/jgp.72.3.305. [DOI] [PMC free article] [PubMed] [Google Scholar]
- Yellen G. Single Ca2+-activated nonselective cation channels in neuroblastoma. Nature. 1982 Mar 25;296(5855):357–359. doi: 10.1038/296357a0. [DOI] [PubMed] [Google Scholar]