Abstract
Electrokinetic responses of human breast cancer MDA-MB-231 cells were studied in suspensions of conductivities 18, 56, and 160 mS/m on a microelectrode array consisting of four parallel spiral electrode elements energized with phase-quadrature signals of frequencies between 100 Hz and 100 MHz. At low frequencies cells were levitated and transported toward or away from the center of the spiral array, whereas at high frequencies cells were trapped at electrode edges. The frequencies of transition between these characteristic cell behaviors increased with increasing suspension conductivity. Levitation heights and radial velocities were determined simultaneously for individual cells as a function of the applied field magnitude and frequency. Results were compared with theoretical predictions from generalized dielectrophoresis theory applied in conjunction with cell dielectric parameters and simulated electric field distributions corrected for electrode polarization effects. It was shown that the conventional and traveling-wave dielectrophoretic force components dominated cell levitation and radial motion, respectively. Both theoretical predictions and experimental data showed that the cell radial velocity was very sensitive to the field frequency when the in-phase component of the field-induced polarization was close to zero. Applications of spiral electrode arrays, including the isolation of cells of clinical relevance, are discussed.
Full text
PDF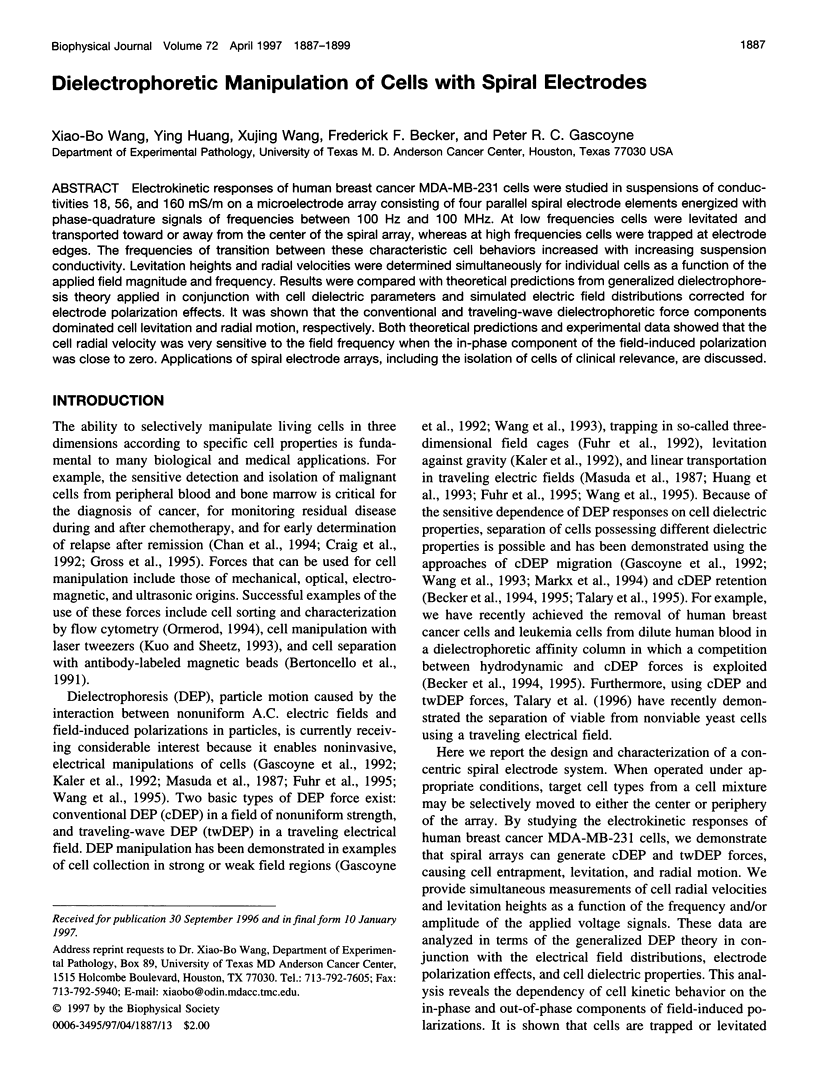
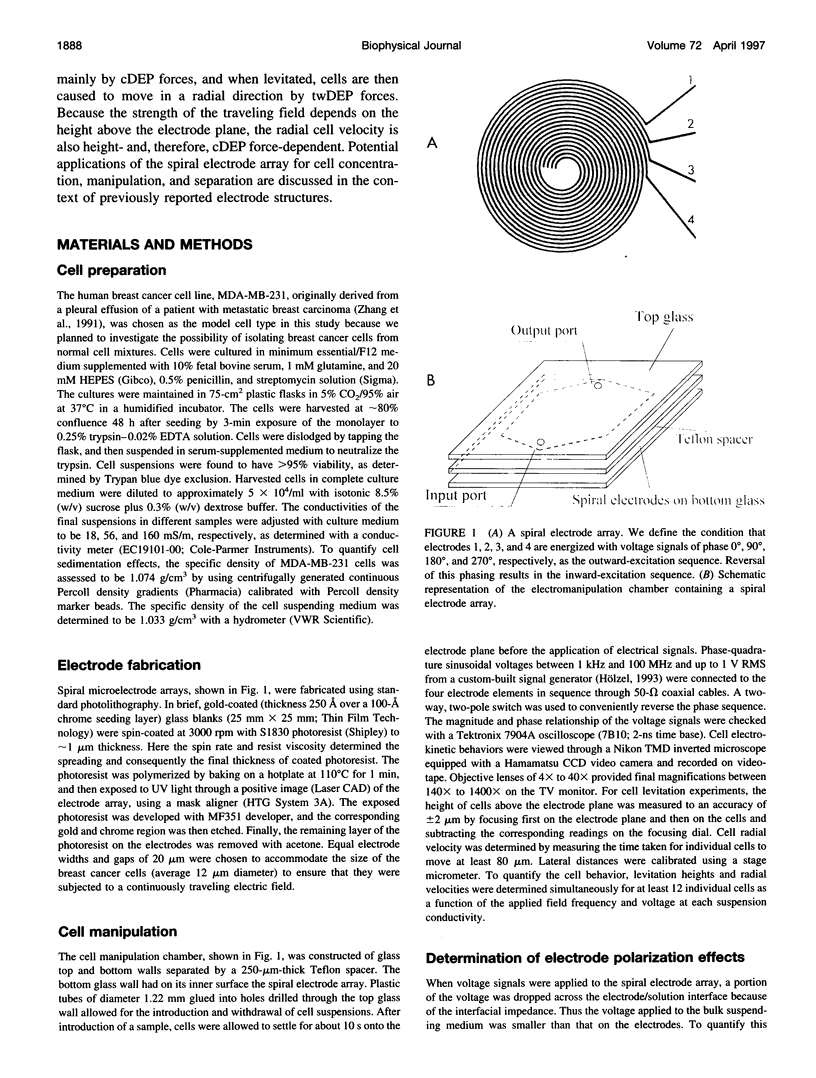
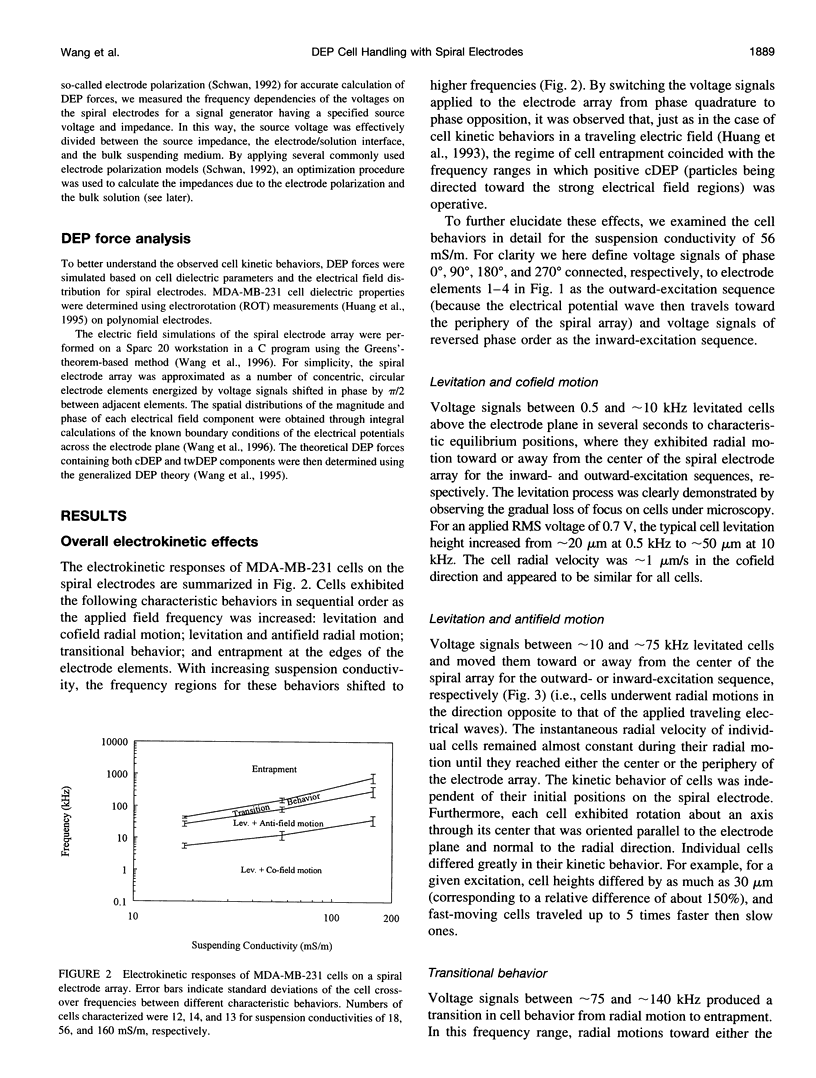
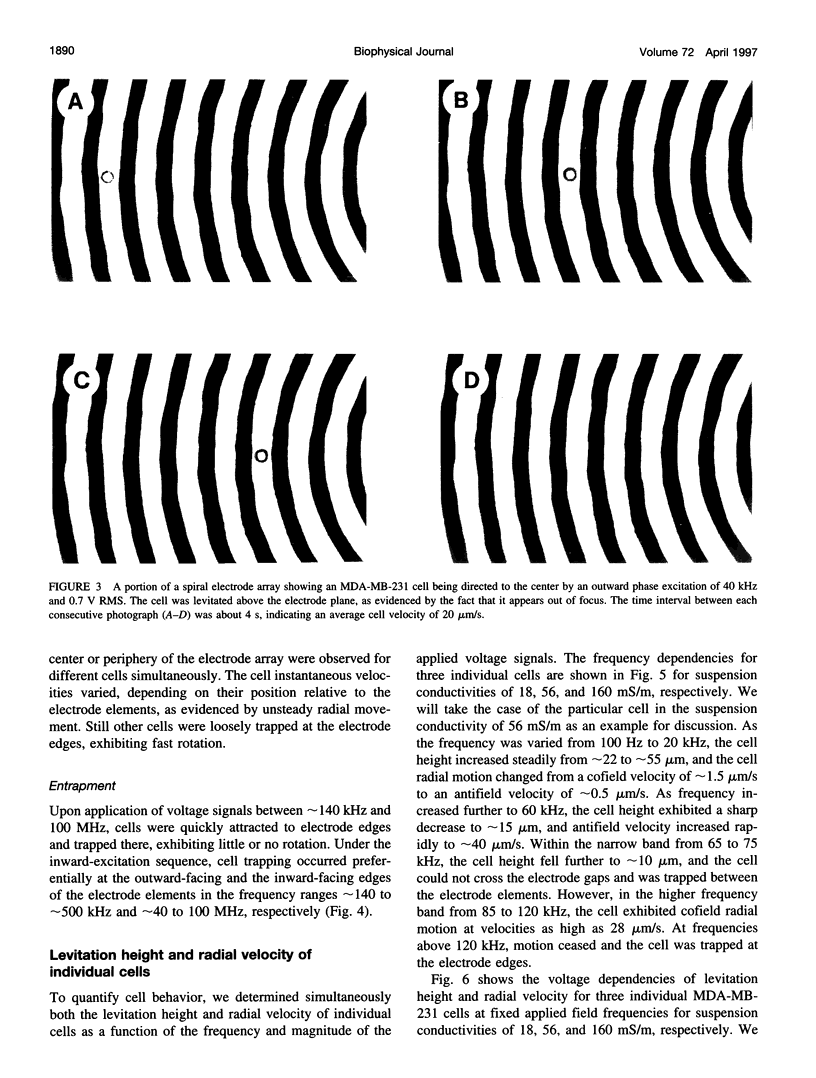
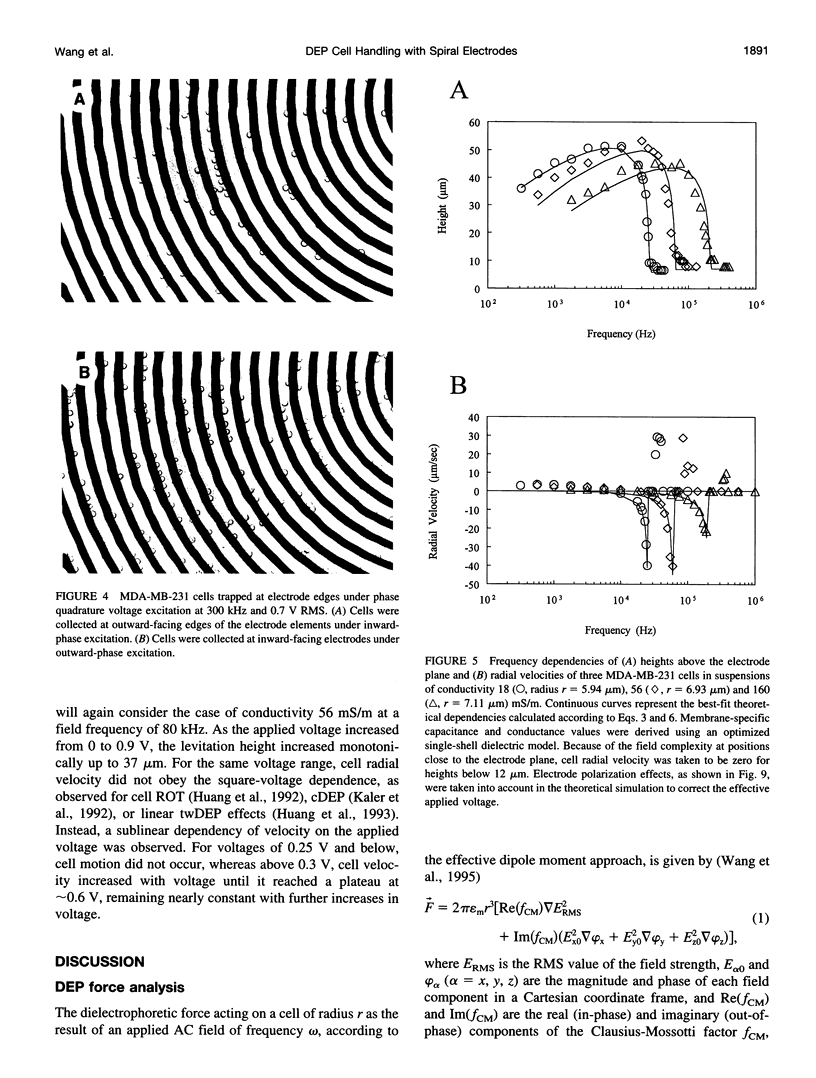
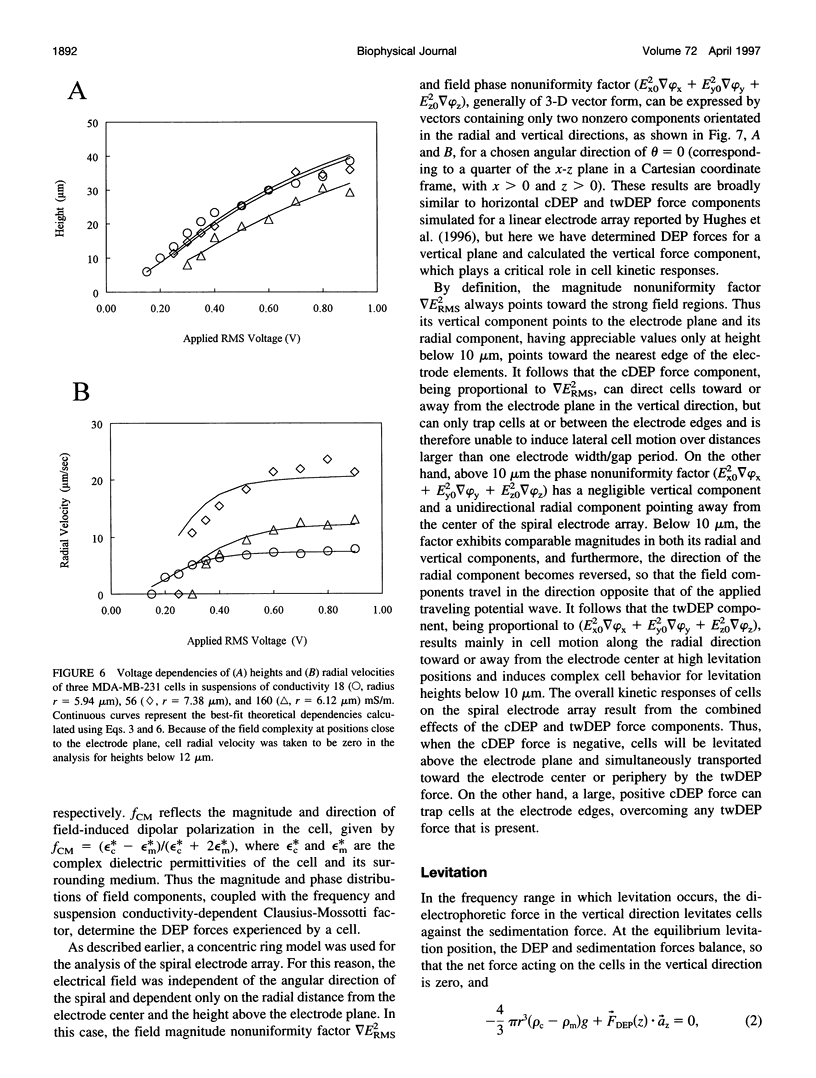
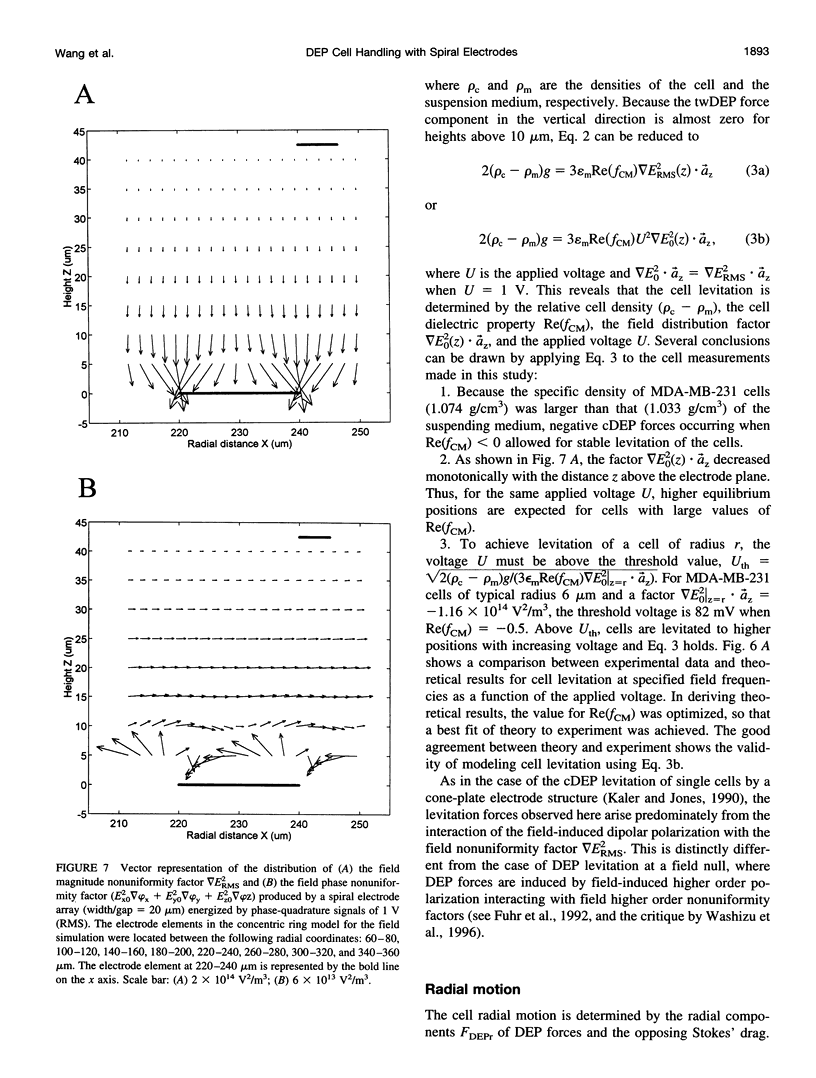
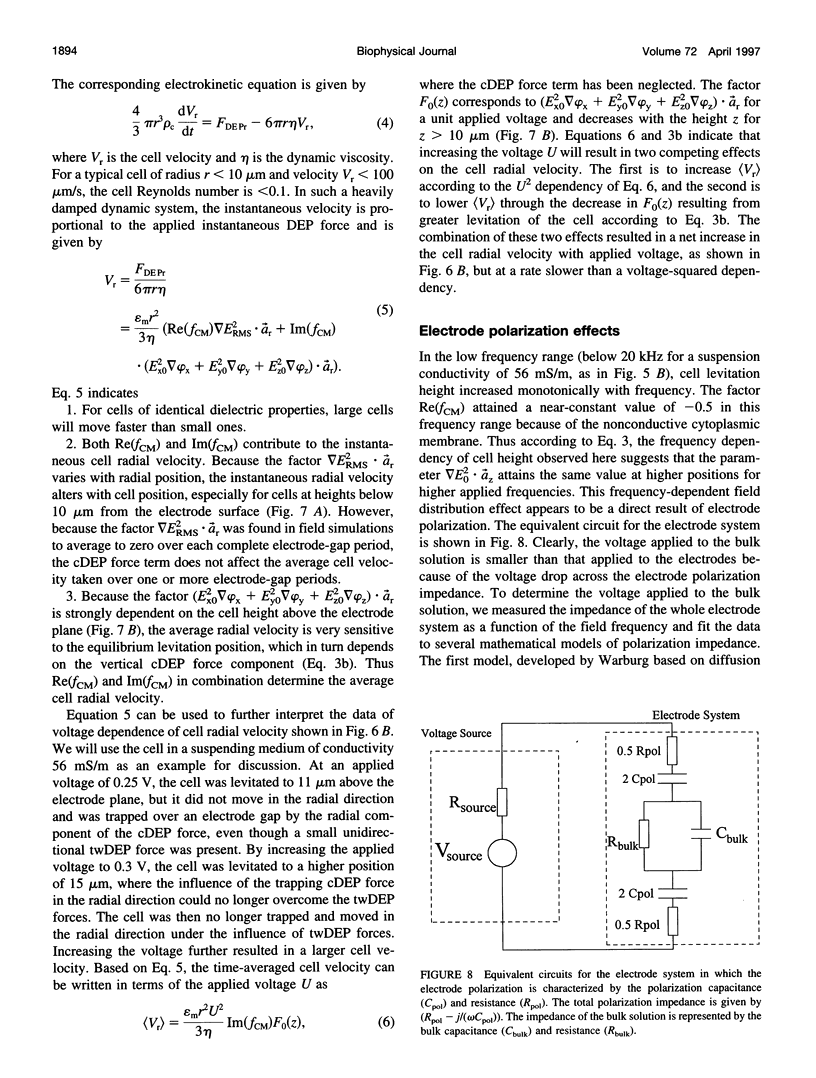
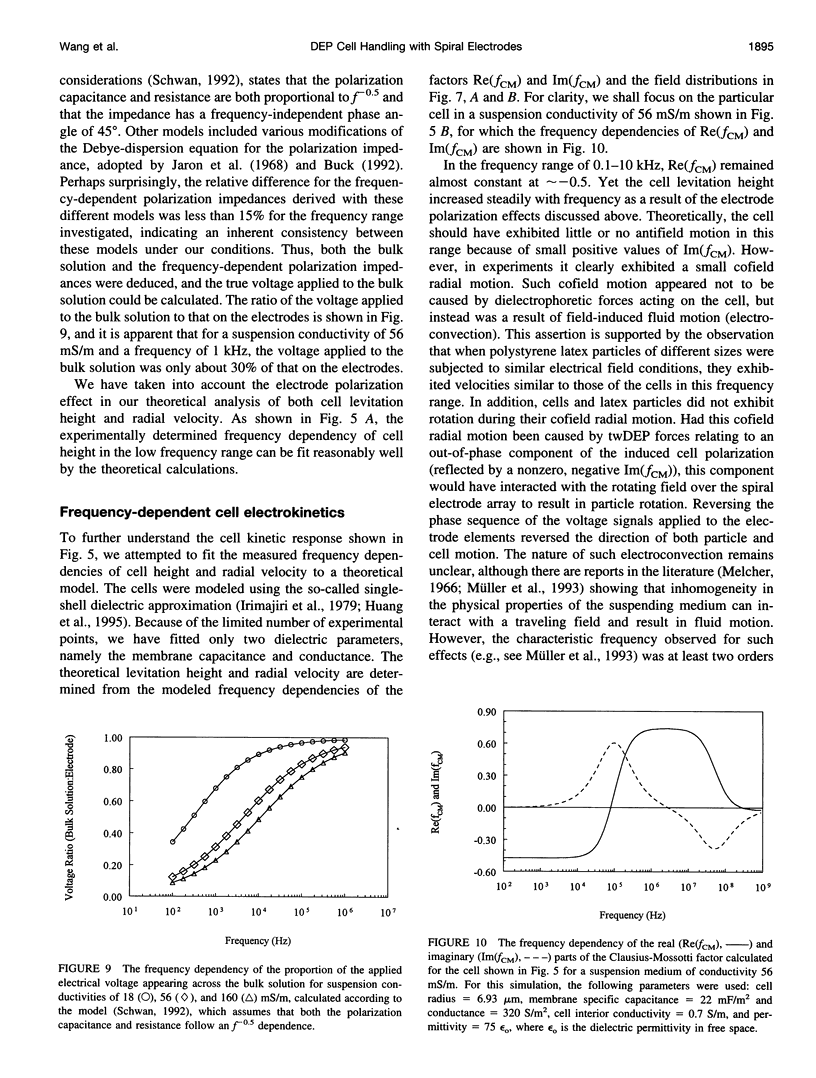
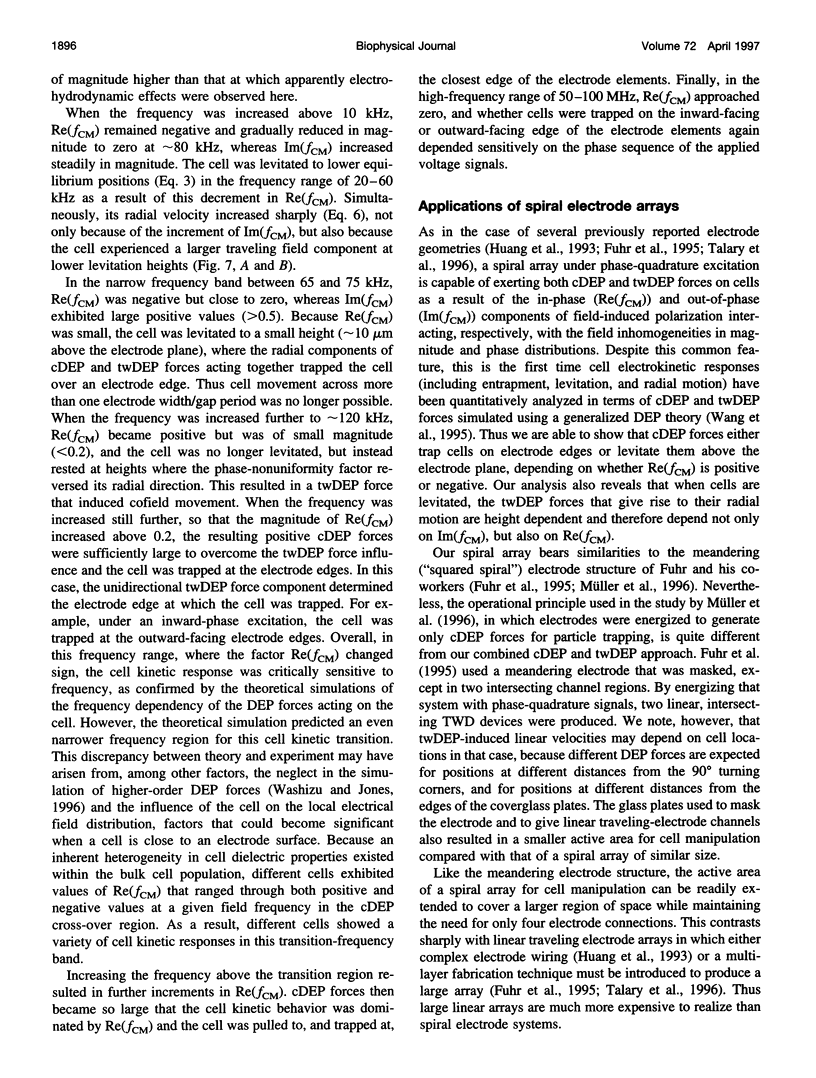
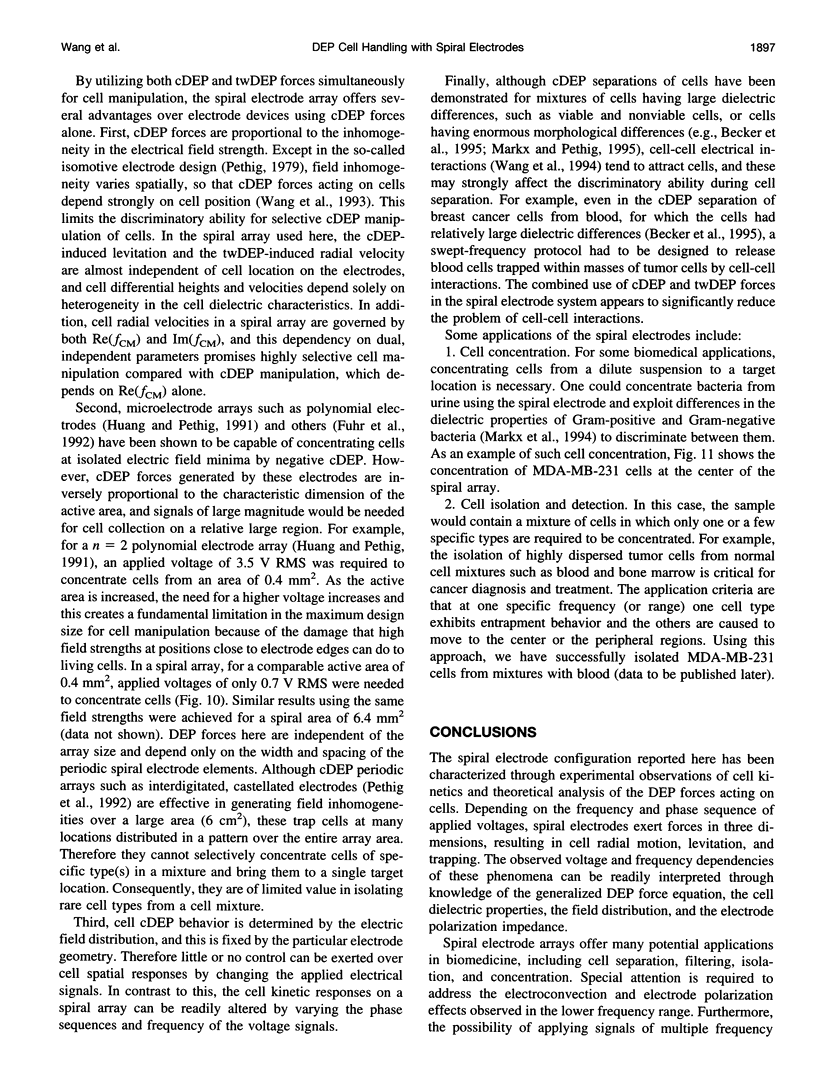
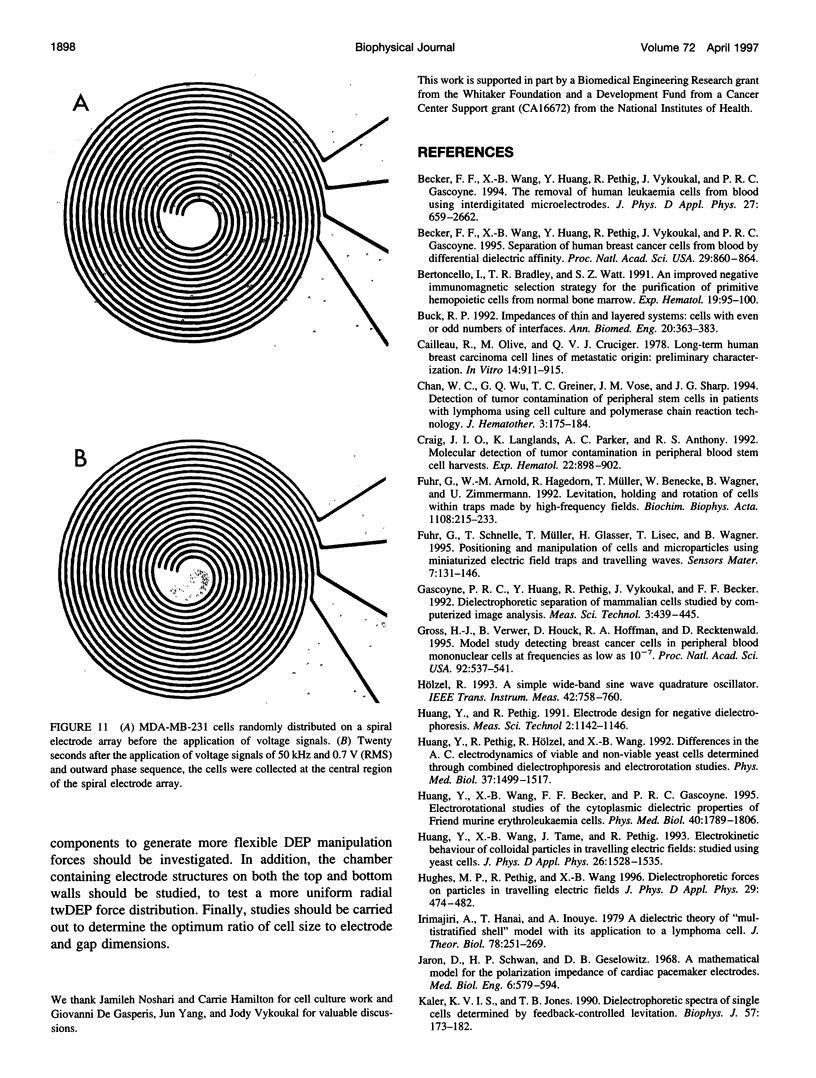
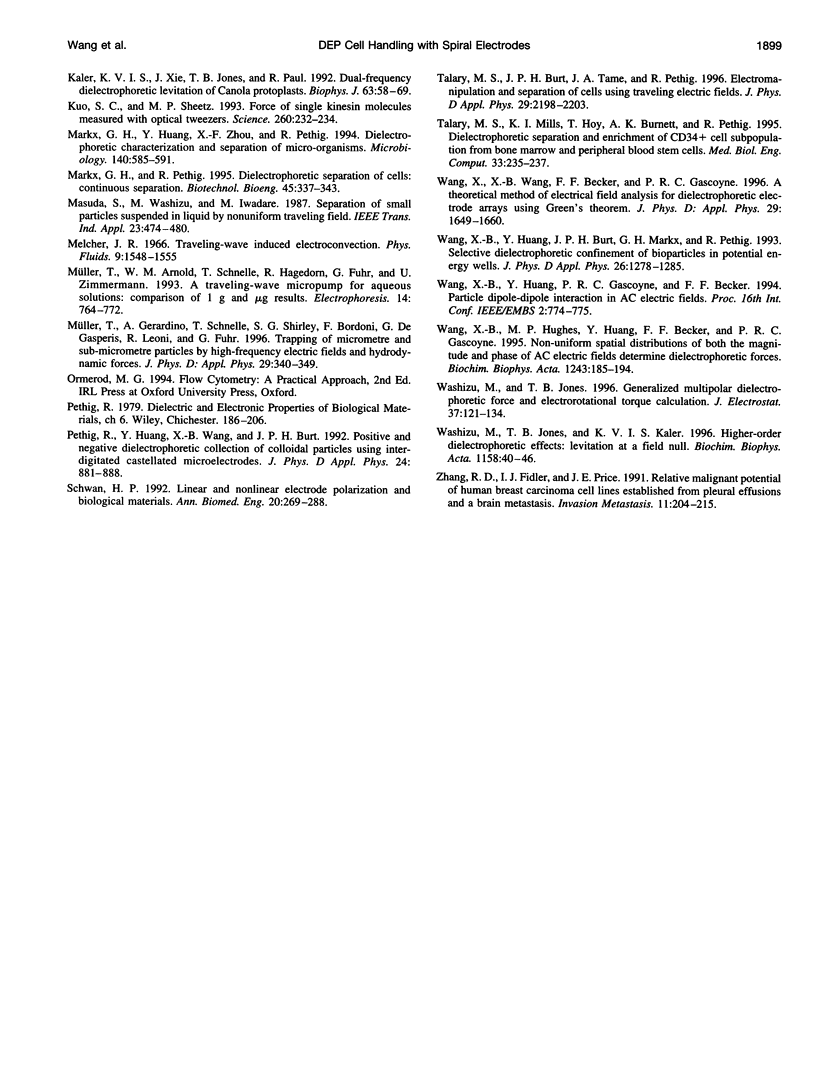
Images in this article
Selected References
These references are in PubMed. This may not be the complete list of references from this article.
- Becker F. F., Wang X. B., Huang Y., Pethig R., Vykoukal J., Gascoyne P. R. Separation of human breast cancer cells from blood by differential dielectric affinity. Proc Natl Acad Sci U S A. 1995 Jan 31;92(3):860–864. doi: 10.1073/pnas.92.3.860. [DOI] [PMC free article] [PubMed] [Google Scholar]
- Bertoncello I., Bradley T. R., Watt S. M. An improved negative immunomagnetic selection strategy for the purification of primitive hemopoietic cells from normal bone marrow. Exp Hematol. 1991 Feb;19(2):95–100. [PubMed] [Google Scholar]
- Buck R. P. Impedances of thin and layered systems: cells with even or odd numbers of interfaces. Ann Biomed Eng. 1992;20(3):363–383. doi: 10.1007/BF02368537. [DOI] [PubMed] [Google Scholar]
- Cailleau R., Olivé M., Cruciger Q. V. Long-term human breast carcinoma cell lines of metastatic origin: preliminary characterization. In Vitro. 1978 Nov;14(11):911–915. doi: 10.1007/BF02616120. [DOI] [PubMed] [Google Scholar]
- Chan W. C., Wu G. Q., Greiner T. C., Vose J. M., Sharp J. G. Detection of tumor contamination of peripheral stem cells in patients with lymphoma using cell culture and polymerase chain reaction technology. J Hematother. 1994 Fall;3(3):175–184. doi: 10.1089/scd.1.1994.3.175. [DOI] [PubMed] [Google Scholar]
- Craig J. I., Langlands K., Parker A. C., Anthony R. S. Molecular detection of tumor contamination in peripheral blood stem cell harvests. Exp Hematol. 1994 Aug;22(9):898–902. [PubMed] [Google Scholar]
- Fuhr G., Arnold W. M., Hagedorn R., Müller T., Benecke W., Wagner B., Zimmermann U. Levitation, holding, and rotation of cells within traps made by high-frequency fields. Biochim Biophys Acta. 1992 Jul 27;1108(2):215–223. doi: 10.1016/0005-2736(92)90028-k. [DOI] [PubMed] [Google Scholar]
- Gross H. J., Verwer B., Houck D., Hoffman R. A., Recktenwald D. Model study detecting breast cancer cells in peripheral blood mononuclear cells at frequencies as low as 10(-7). Proc Natl Acad Sci U S A. 1995 Jan 17;92(2):537–541. doi: 10.1073/pnas.92.2.537. [DOI] [PMC free article] [PubMed] [Google Scholar]
- Hsu S. H., Wang B. T., Huang M. H., Wong W. J., Cross J. H. Growth of Japanese encephalitis virus in Culex tritaeniorhynchus cell cultures. Am J Trop Med Hyg. 1975 Sep;24(5):881–888. doi: 10.4269/ajtmh.1975.24.881. [DOI] [PubMed] [Google Scholar]
- Huang Y., Hölzel R., Pethig R., Wang X. B. Differences in the AC electrodynamics of viable and non-viable yeast cells determined through combined dielectrophoresis and electrorotation studies. Phys Med Biol. 1992 Jul;37(7):1499–1517. doi: 10.1088/0031-9155/37/7/003. [DOI] [PubMed] [Google Scholar]
- Huang Y., Wang X. B., Hölzel R., Becker F. F., Gascoyne P. R. Electrorotational studies of the cytoplasmic dielectric properties of Friend murine erythroleukaemia cells. Phys Med Biol. 1995 Nov;40(11):1789–1806. doi: 10.1088/0031-9155/40/11/002. [DOI] [PubMed] [Google Scholar]
- Irimajiri A., Hanai T., Inouye A. A dielectric theory of "multi-stratified shell" model with its application to a lymphoma cell. J Theor Biol. 1979 May 21;78(2):251–269. doi: 10.1016/0022-5193(79)90268-6. [DOI] [PubMed] [Google Scholar]
- Jaron D., Schwan H. P., Geselowitz D. B. A mathematical model for the polarization impedance of cardiac pacemaker electrodes. Med Biol Eng. 1968 Nov;6(6):579–594. doi: 10.1007/BF02474721. [DOI] [PubMed] [Google Scholar]
- Kaler K. V., Jones T. B. Dielectrophoretic spectra of single cells determined by feedback-controlled levitation. Biophys J. 1990 Feb;57(2):173–182. doi: 10.1016/S0006-3495(90)82520-0. [DOI] [PMC free article] [PubMed] [Google Scholar]
- Kaler K. V., Xie J. P., Jones T. B., Paul R. Dual-frequency dielectrophoretic levitation of Canola protoplasts. Biophys J. 1992 Jul;63(1):58–69. doi: 10.1016/S0006-3495(92)81586-2. [DOI] [PMC free article] [PubMed] [Google Scholar]
- Kuo S. C., Sheetz M. P. Force of single kinesin molecules measured with optical tweezers. Science. 1993 Apr 9;260(5105):232–234. doi: 10.1126/science.8469975. [DOI] [PubMed] [Google Scholar]
- Müller T., Arnold W. M., Schnelle T., Hagedorn R., Fuhr G., Zimmermann U. A traveling-wave micropump for aqueous solutions: comparison of 1 g and microgram results. Electrophoresis. 1993 Aug;14(8):764–772. doi: 10.1002/elps.11501401120. [DOI] [PubMed] [Google Scholar]
- Schwan H. P. Linear and nonlinear electrode polarization and biological materials. Ann Biomed Eng. 1992;20(3):269–288. doi: 10.1007/BF02368531. [DOI] [PubMed] [Google Scholar]
- Talary M. S., Mills K. I., Hoy T., Burnett A. K., Pethig R. Dielectrophoretic separation and enrichment of CD34+ cell subpopulation from bone marrow and peripheral blood stem cells. Med Biol Eng Comput. 1995 Mar;33(2):235–237. doi: 10.1007/BF02523050. [DOI] [PubMed] [Google Scholar]
- Wang X. B., Hughes M. P., Huang Y., Becker F. F., Gascoyne P. R. Non-uniform spatial distributions of both the magnitude and phase of AC electric fields determine dielectrophoretic forces. Biochim Biophys Acta. 1995 Feb 23;1243(2):185–194. doi: 10.1016/0304-4165(94)00146-o. [DOI] [PubMed] [Google Scholar]
- Washizu M., Jones T. B., Kaler K. V. Higher-order dielectrophoretic effects: levitation at a field null. Biochim Biophys Acta. 1993 Aug 20;1158(1):40–46. doi: 10.1016/0304-4165(93)90094-o. [DOI] [PubMed] [Google Scholar]
- Zhang R. D., Fidler I. J., Price J. E. Relative malignant potential of human breast carcinoma cell lines established from pleural effusions and a brain metastasis. Invasion Metastasis. 1991;11(4):204–215. [PubMed] [Google Scholar]