Abstract
Using an in vitro motility assay, we have investigated Ca2+ regulation of individual, regulated thin filaments reconstituted from rabbit fast skeletal actin, troponin, and tropomyosin. Rhodamine-phalloidin labeling was used to visualize the filaments by epifluorescence, and assays were conducted at 30 degrees C and at ionic strengths near the physiological range. Regulated thin filaments exhibited well-regulated behavior when tropomyosin and troponin were added to the motility solutions because there was no directed motion in the absence of Ca2+. Unlike F-actin, the speed increased in a graded manner with increasing [Ca2+], whereas the number of regulated thin filaments moving was more steeply regulated. With increased ionic strength, Ca2+ sensitivity of both the number of filaments moving and their speed was shifted toward higher [Ca2+] and was steepest at the highest ionic strength studied (0.14 M gamma/2). Methylcellulose concentration (0.4% versus 0.7%) had no effect on the Ca2+ dependence of speed or number of filaments moving. These conclusions hold for five different methods used to analyze the data, indicating that the conclusions are robust. The force-pCa relationship (pCa = -log10[Ca2+]) for rabbit psoas skinned fibers taken under similar conditions of temperature and solution composition (0.14 M gamma/2) paralleled the speed-pCa relationship for the regulated filaments in the in vitro motility assay. Comparison of motility results with the force-pCa relationship in fibers suggests that relatively few cross-bridges are needed to make filaments move, but many have to be cycling to make the regulated filament move at maximum speed.
Full text
PDF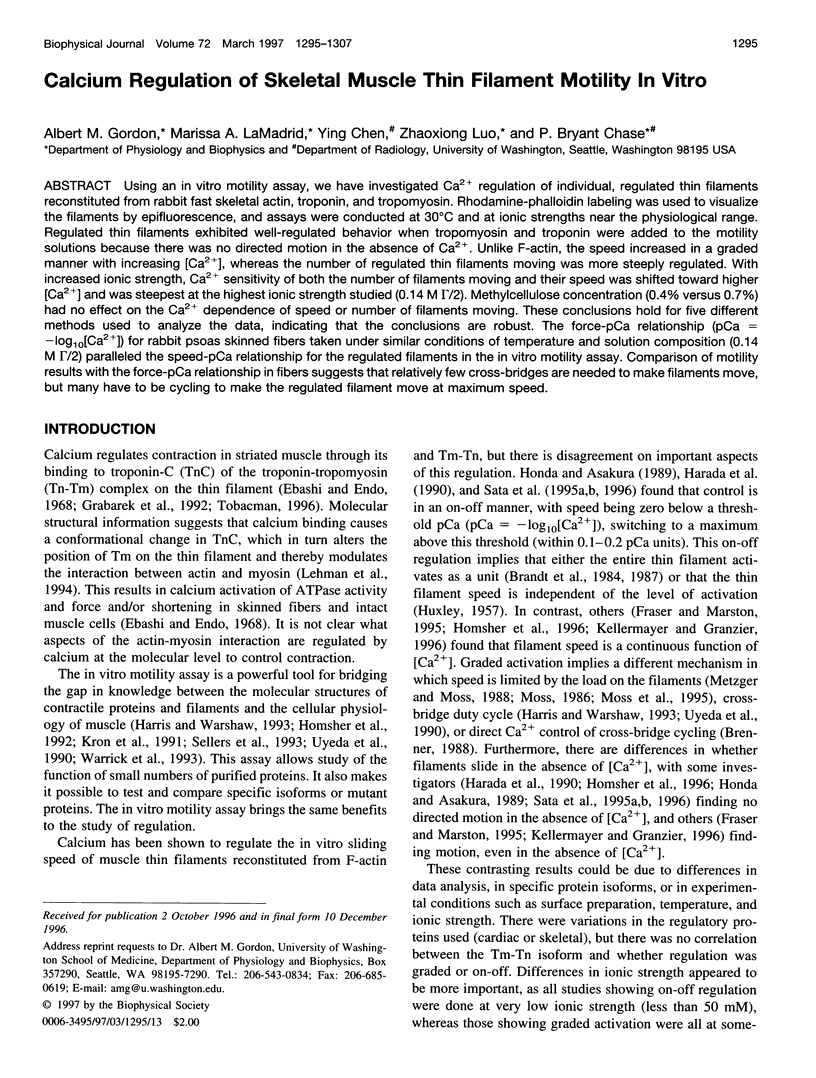
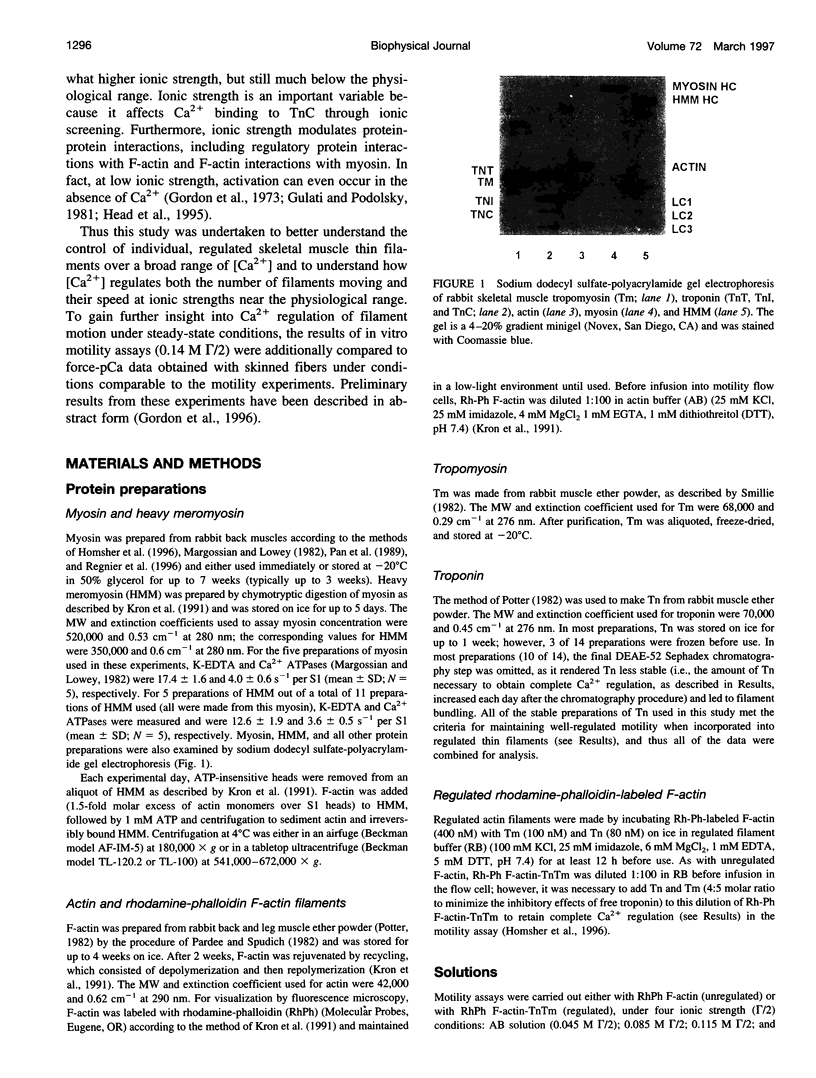
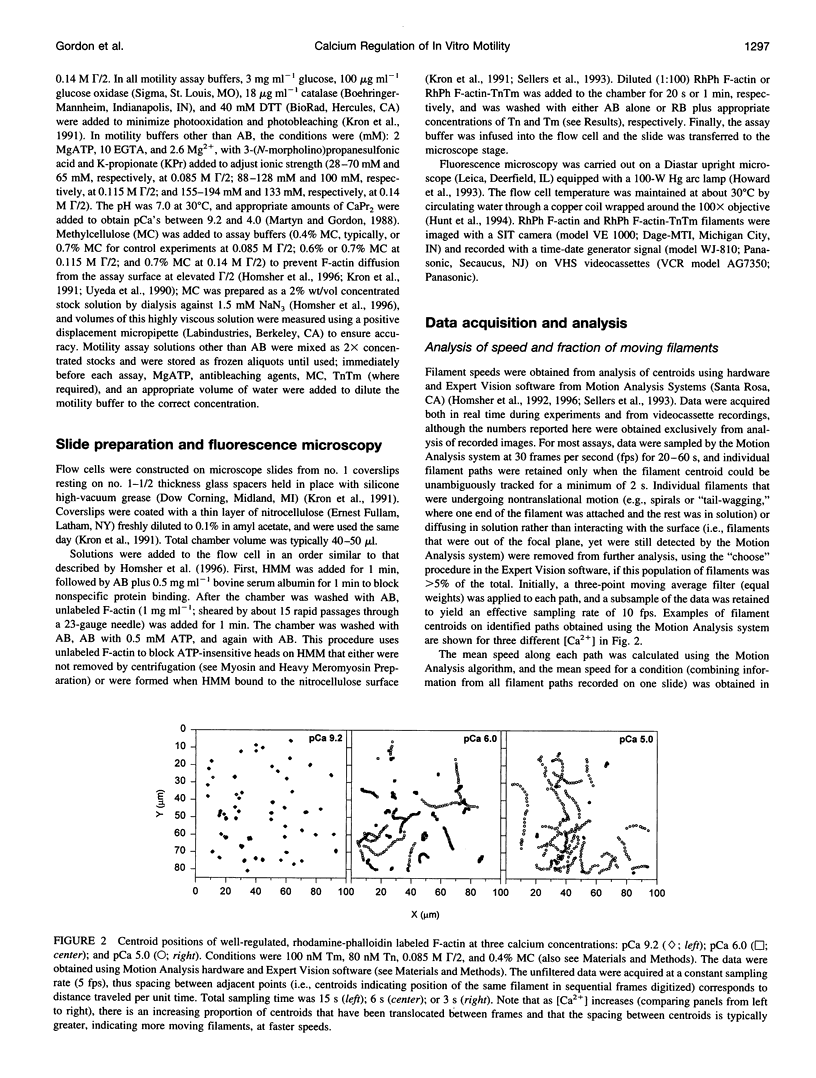
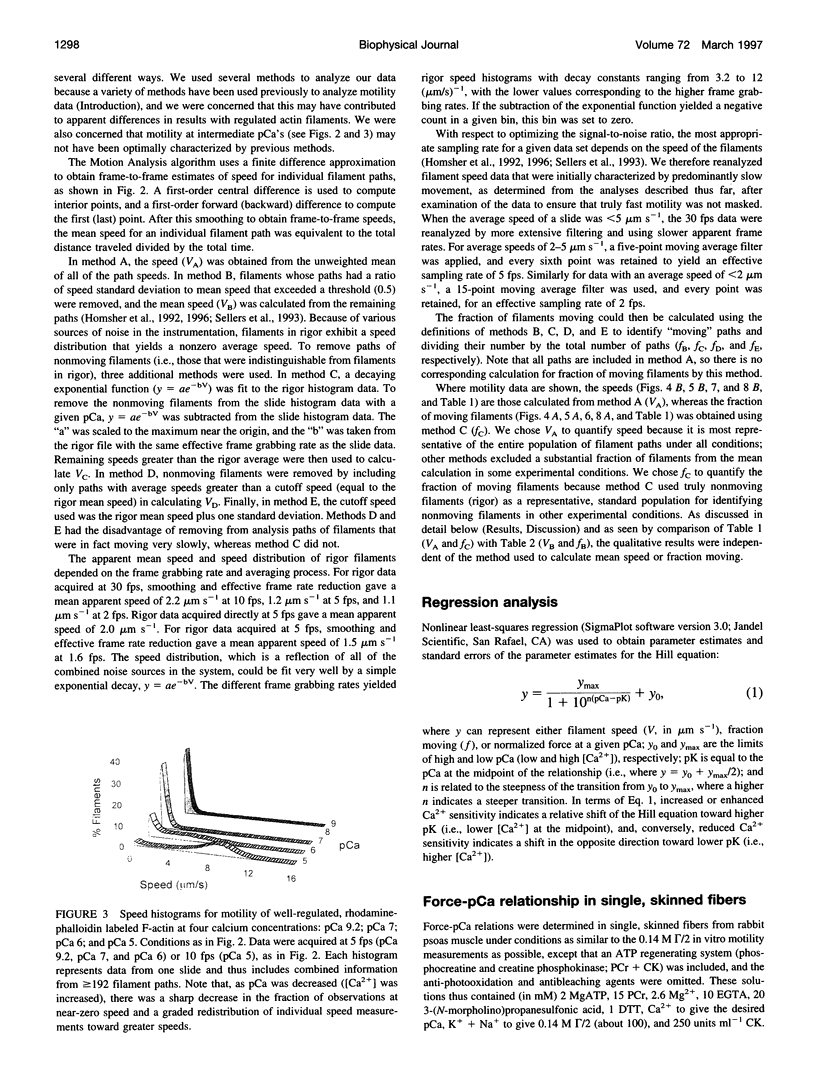
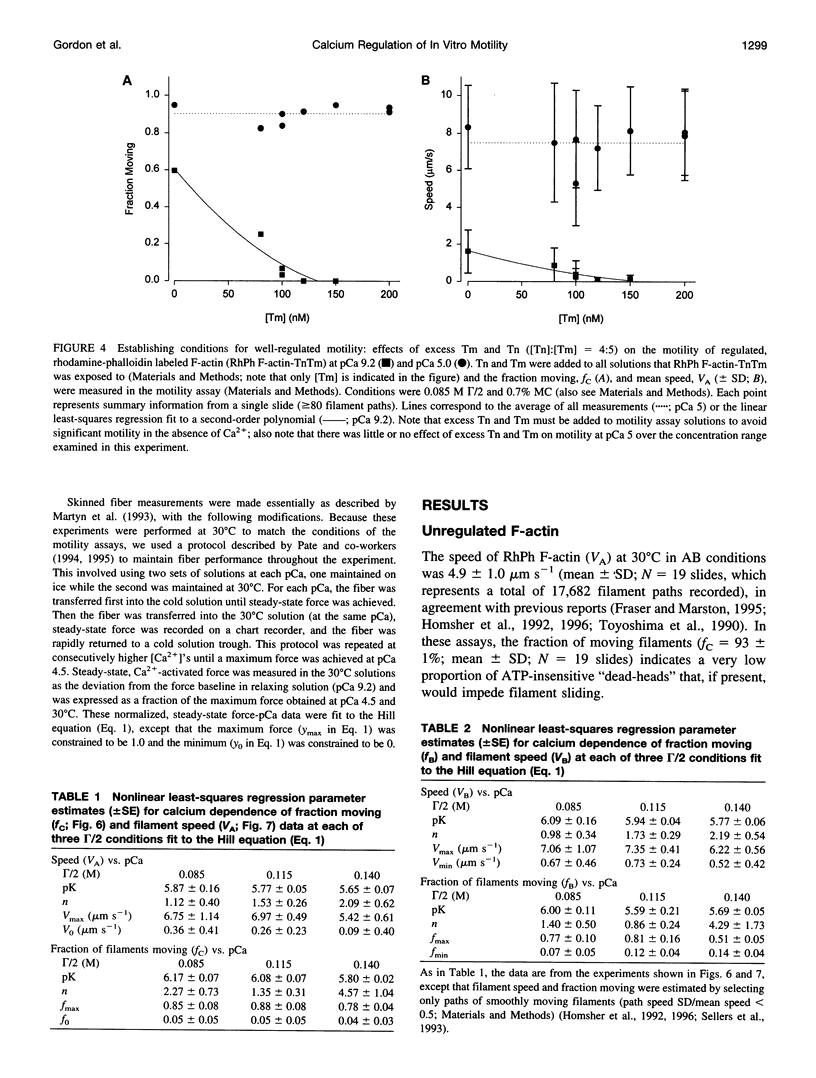
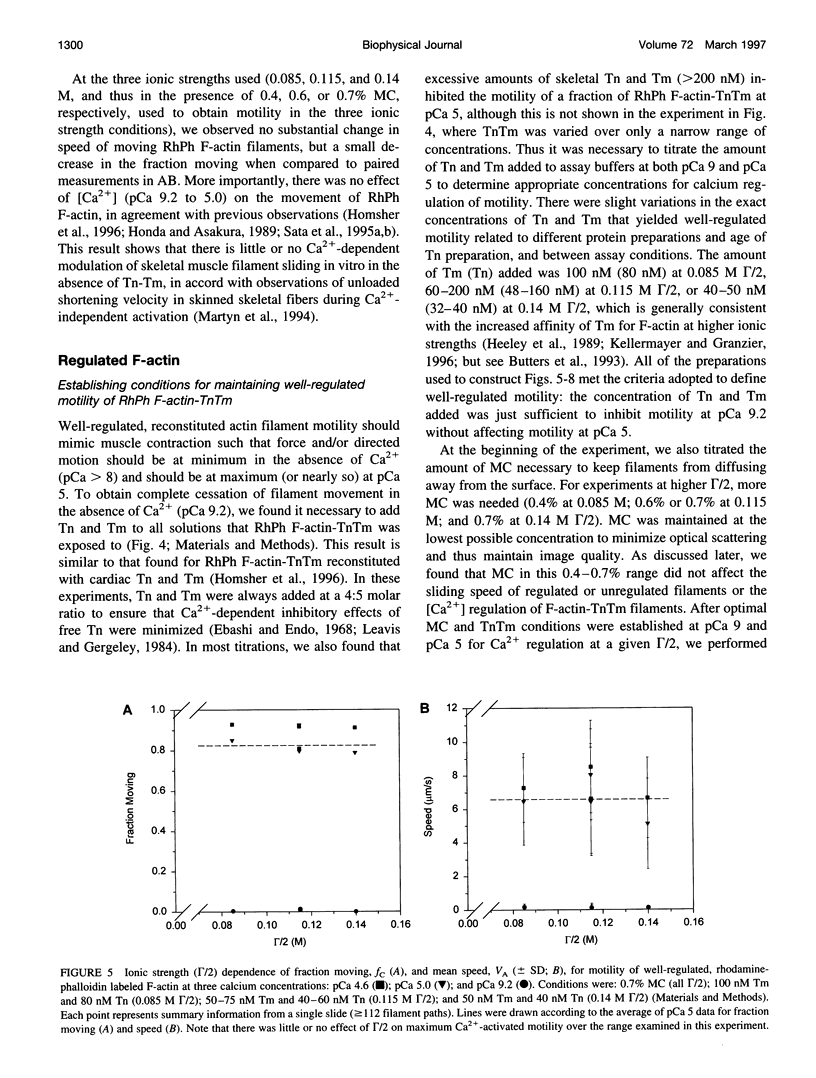
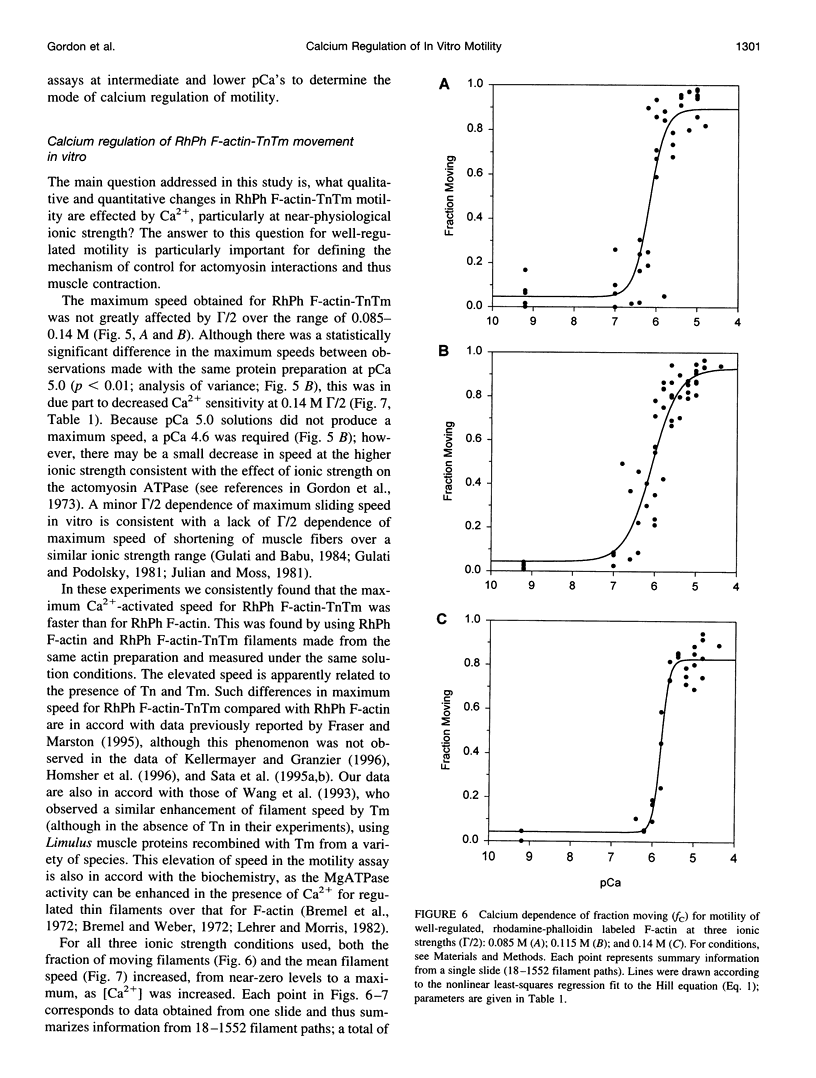
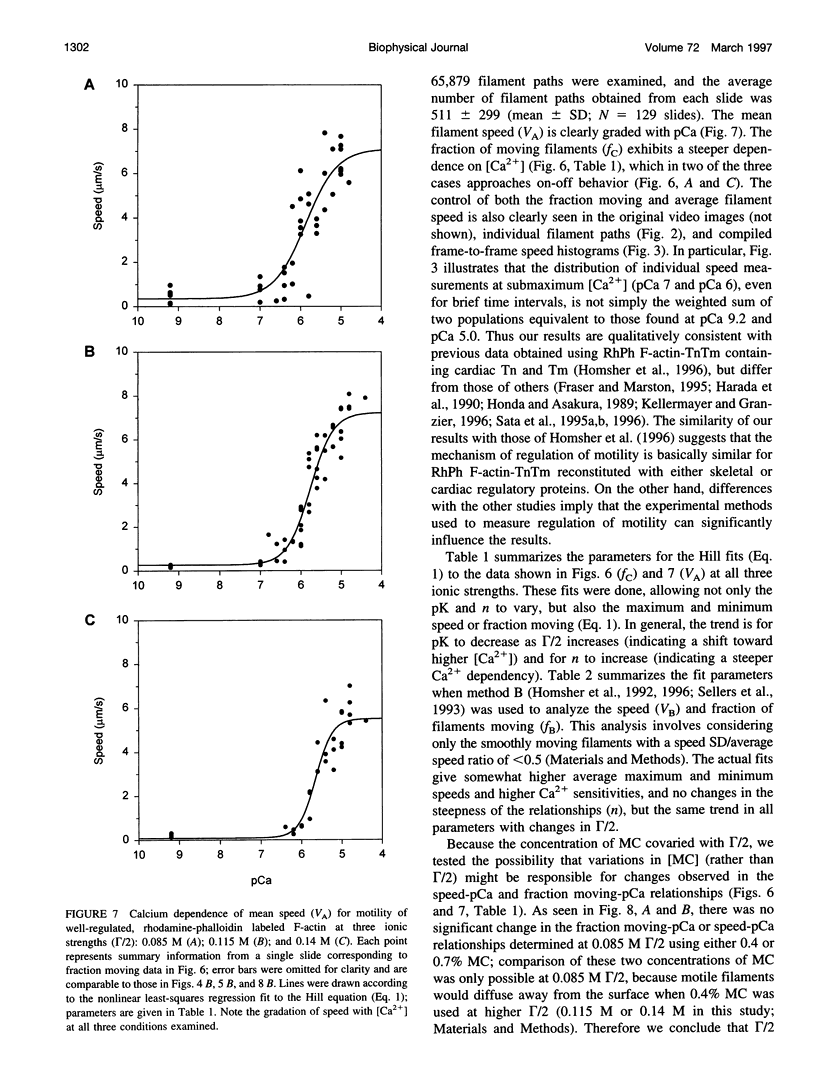
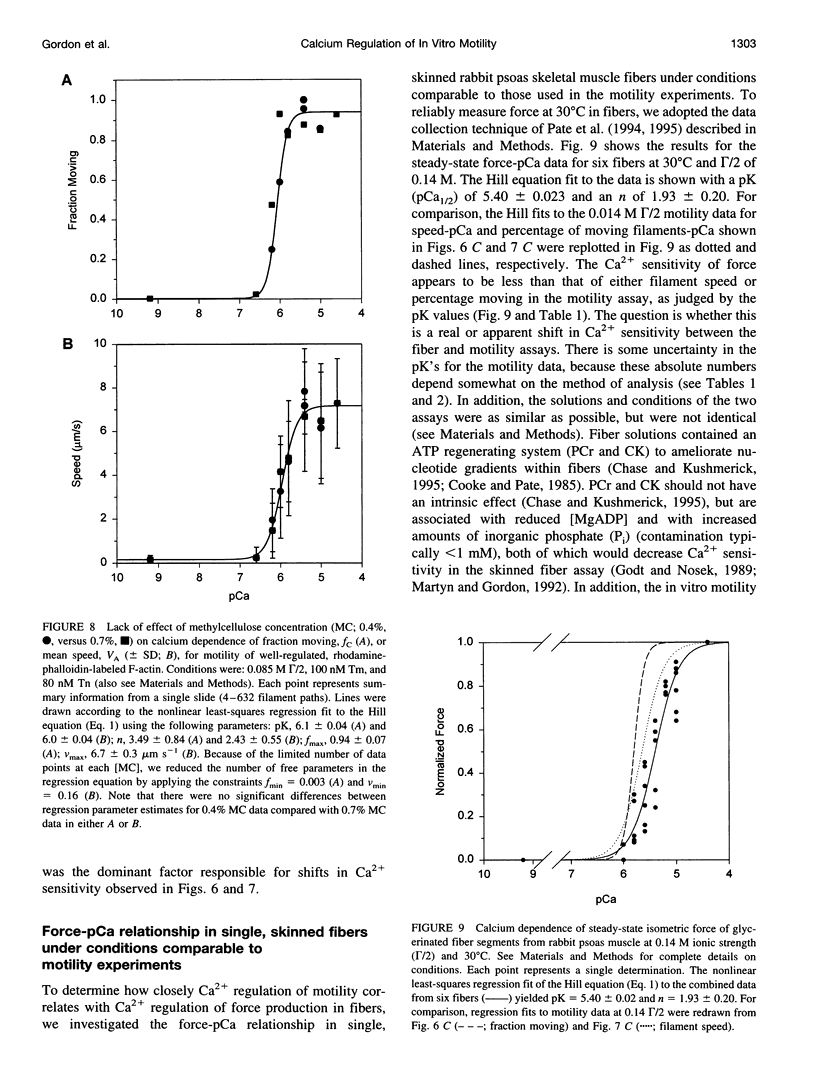
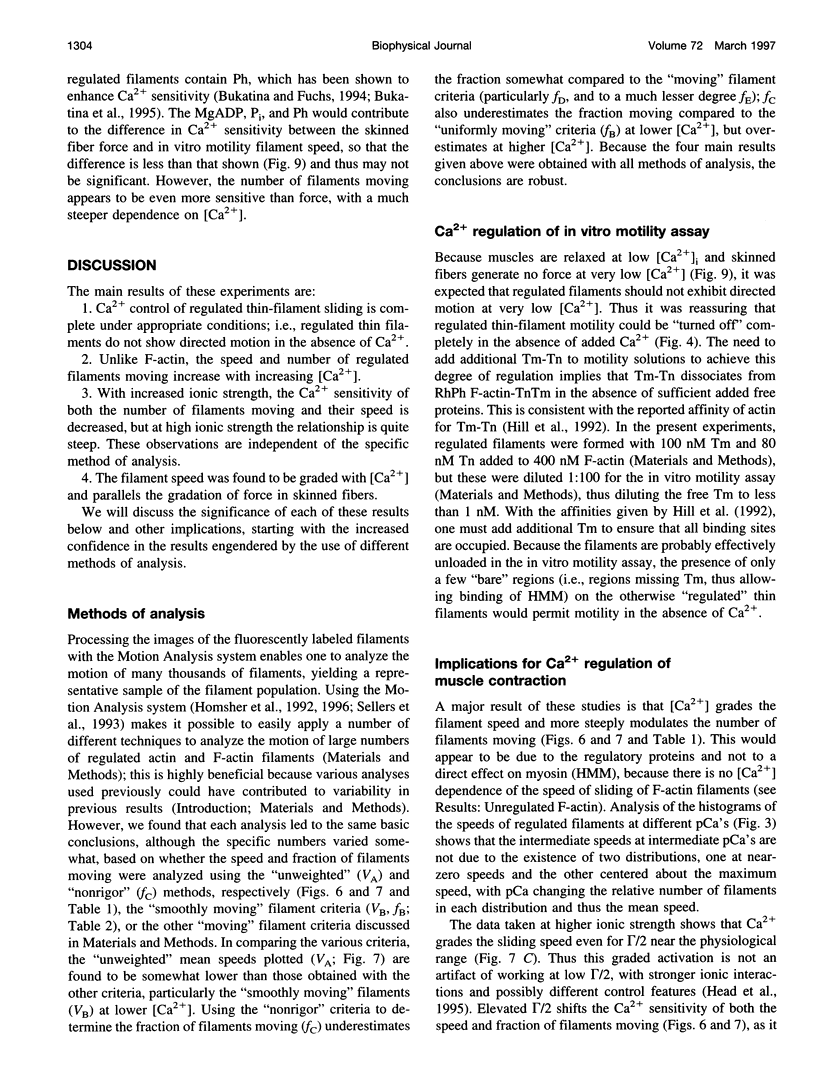
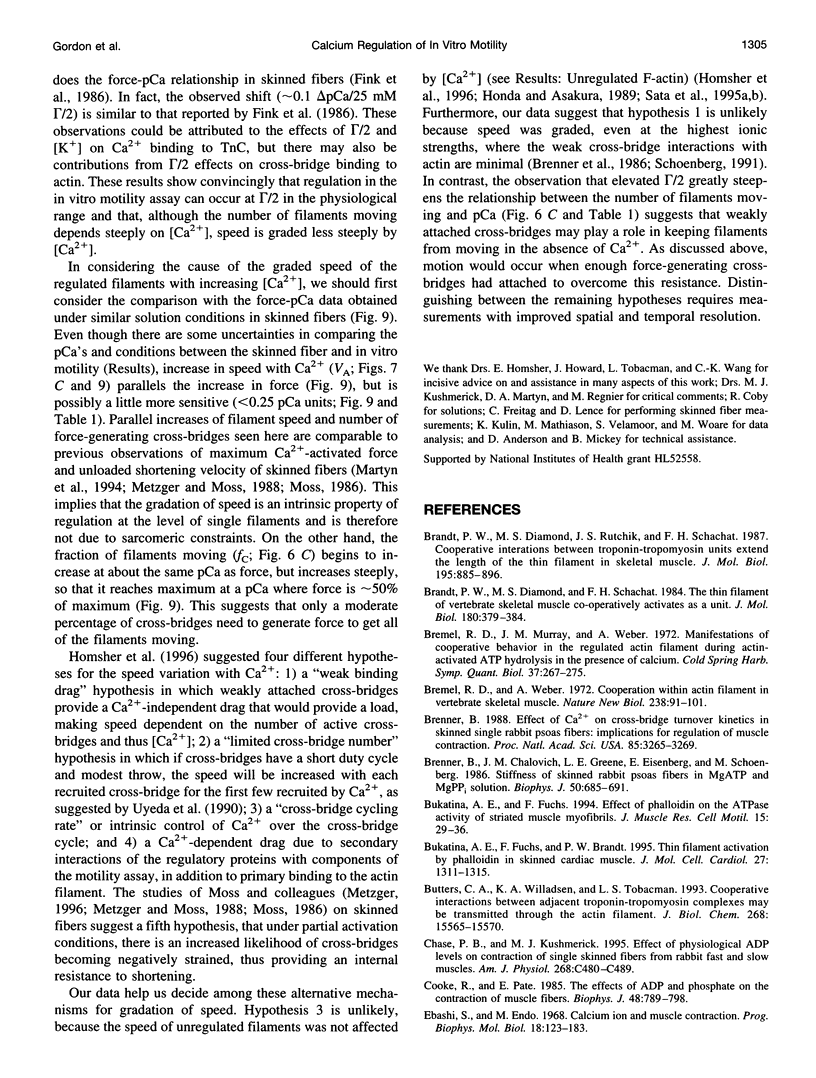
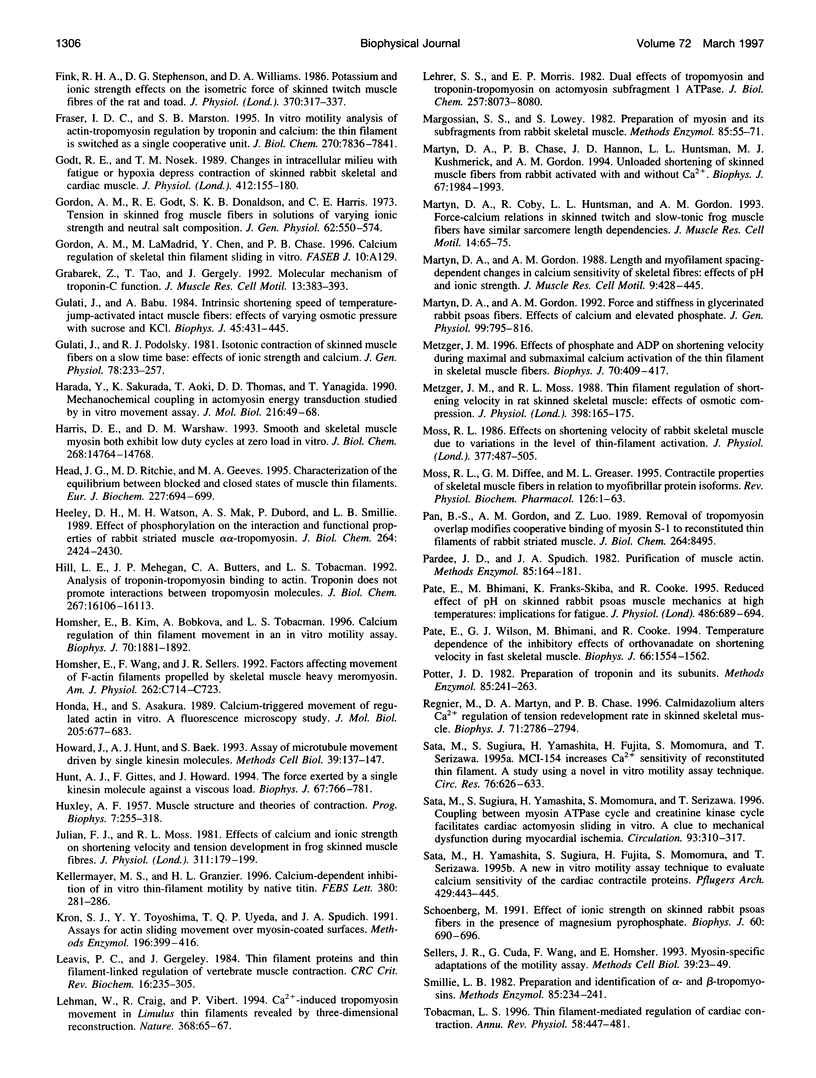
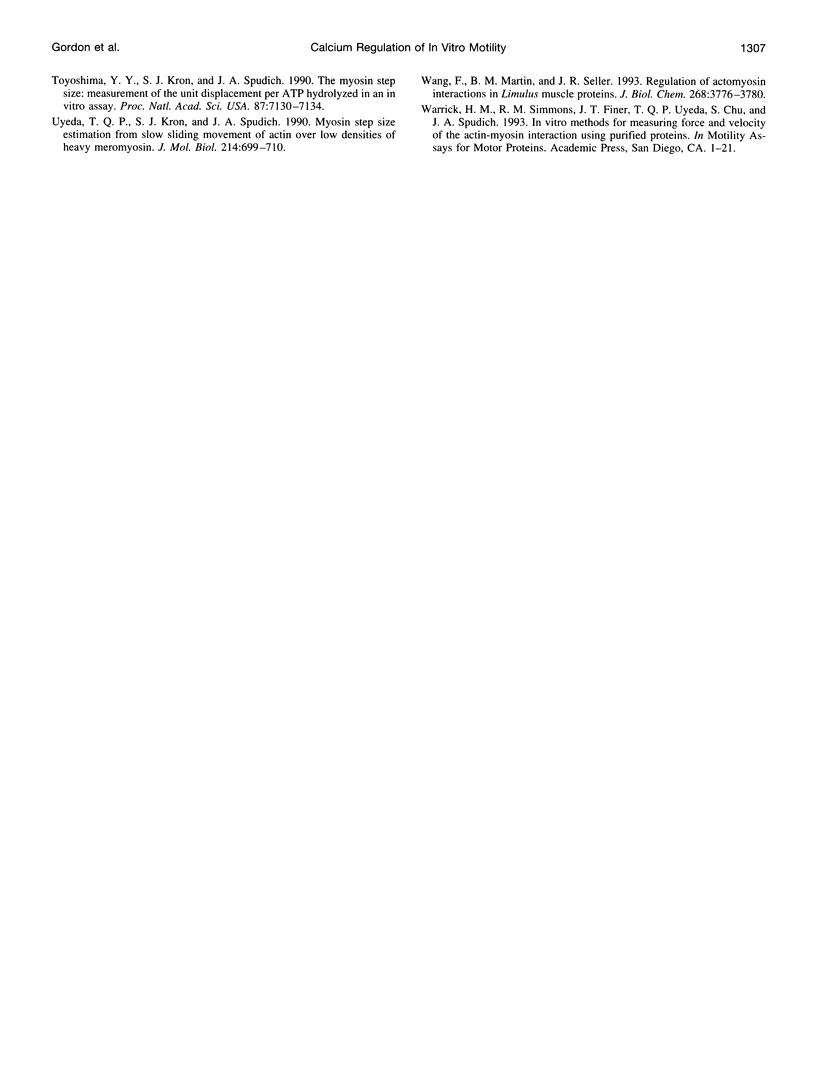
Images in this article
Selected References
These references are in PubMed. This may not be the complete list of references from this article.
- Brandt P. W., Diamond M. S., Rutchik J. S., Schachat F. H. Co-operative interactions between troponin-tropomyosin units extend the length of the thin filament in skeletal muscle. J Mol Biol. 1987 Jun 20;195(4):885–896. doi: 10.1016/0022-2836(87)90492-x. [DOI] [PubMed] [Google Scholar]
- Brandt P. W., Diamond M. S., Schachat F. H. The thin filament of vertebrate skeletal muscle co-operatively activates as a unit. J Mol Biol. 1984 Dec 5;180(2):379–384. doi: 10.1016/s0022-2836(84)80010-8. [DOI] [PubMed] [Google Scholar]
- Bremel R. D., Weber A. Cooperation within actin filament in vertebrate skeletal muscle. Nat New Biol. 1972 Jul 26;238(82):97–101. doi: 10.1038/newbio238097a0. [DOI] [PubMed] [Google Scholar]
- Brenner B., Chalovich J. M., Greene L. E., Eisenberg E., Schoenberg M. Stiffness of skinned rabbit psoas fibers in MgATP and MgPPi solution. Biophys J. 1986 Oct;50(4):685–691. doi: 10.1016/S0006-3495(86)83509-3. [DOI] [PMC free article] [PubMed] [Google Scholar]
- Brenner B. Effect of Ca2+ on cross-bridge turnover kinetics in skinned single rabbit psoas fibers: implications for regulation of muscle contraction. Proc Natl Acad Sci U S A. 1988 May;85(9):3265–3269. doi: 10.1073/pnas.85.9.3265. [DOI] [PMC free article] [PubMed] [Google Scholar]
- Bukatina A. E., Fuchs F., Brandt P. W. Thin filament activation by phalloidin in skinned cardiac muscle. J Mol Cell Cardiol. 1995 Jun;27(6):1311–1315. doi: 10.1016/s0022-2828(05)82393-4. [DOI] [PubMed] [Google Scholar]
- Bukatina A. E., Fuchs F. Effect of phalloidin on the ATPase activity of striated muscle myofibrils. J Muscle Res Cell Motil. 1994 Feb;15(1):29–36. doi: 10.1007/BF00123830. [DOI] [PubMed] [Google Scholar]
- Butters C. A., Willadsen K. A., Tobacman L. S. Cooperative interactions between adjacent troponin-tropomyosin complexes may be transmitted through the actin filament. J Biol Chem. 1993 Jul 25;268(21):15565–15570. [PubMed] [Google Scholar]
- Chase P. B., Kushmerick M. J. Effect of physiological ADP concentrations on contraction of single skinned fibers from rabbit fast and slow muscles. Am J Physiol. 1995 Feb;268(2 Pt 1):C480–C489. doi: 10.1152/ajpcell.1995.268.2.C480. [DOI] [PubMed] [Google Scholar]
- Cooke R., Pate E. The effects of ADP and phosphate on the contraction of muscle fibers. Biophys J. 1985 Nov;48(5):789–798. doi: 10.1016/S0006-3495(85)83837-6. [DOI] [PMC free article] [PubMed] [Google Scholar]
- Ebashi S., Endo M. Calcium ion and muscle contraction. Prog Biophys Mol Biol. 1968;18:123–183. doi: 10.1016/0079-6107(68)90023-0. [DOI] [PubMed] [Google Scholar]
- Fink R. H., Stephenson D. G., Williams D. A. Potassium and ionic strength effects on the isometric force of skinned twitch muscle fibres of the rat and toad. J Physiol. 1986 Jan;370:317–337. doi: 10.1113/jphysiol.1986.sp015937. [DOI] [PMC free article] [PubMed] [Google Scholar]
- Fraser I. D., Marston S. B. In vitro motility analysis of actin-tropomyosin regulation by troponin and calcium. The thin filament is switched as a single cooperative unit. J Biol Chem. 1995 Apr 7;270(14):7836–7841. doi: 10.1074/jbc.270.14.7836. [DOI] [PubMed] [Google Scholar]
- Godt R. E., Nosek T. M. Changes of intracellular milieu with fatigue or hypoxia depress contraction of skinned rabbit skeletal and cardiac muscle. J Physiol. 1989 May;412:155–180. doi: 10.1113/jphysiol.1989.sp017609. [DOI] [PMC free article] [PubMed] [Google Scholar]
- Gordon A. M., Godt R. E., Donaldson S. K., Harris C. E. Tension in skinned frog muscle fibers in solutions of varying ionic strength and neutral salt composition. J Gen Physiol. 1973 Nov;62(5):550–574. doi: 10.1085/jgp.62.5.550. [DOI] [PMC free article] [PubMed] [Google Scholar]
- Grabarek Z., Tao T., Gergely J. Molecular mechanism of troponin-C function. J Muscle Res Cell Motil. 1992 Aug;13(4):383–393. doi: 10.1007/BF01738034. [DOI] [PubMed] [Google Scholar]
- Gulati J., Babu A. Intrinsic shortening speed of temperature-jump-activated intact muscle fibers. Effects of varying osmotic pressure with sucrose and KCl. Biophys J. 1984 Feb;45(2):431–445. doi: 10.1016/S0006-3495(84)84166-1. [DOI] [PMC free article] [PubMed] [Google Scholar]
- Gulati J., Podolsky R. J. Isotonic contraction of skinned muscle fibers on a slow time base: effects of ionic strength and calcium. J Gen Physiol. 1981 Sep;78(3):233–257. doi: 10.1085/jgp.78.3.233. [DOI] [PMC free article] [PubMed] [Google Scholar]
- HUXLEY A. F. Muscle structure and theories of contraction. Prog Biophys Biophys Chem. 1957;7:255–318. [PubMed] [Google Scholar]
- Harada Y., Sakurada K., Aoki T., Thomas D. D., Yanagida T. Mechanochemical coupling in actomyosin energy transduction studied by in vitro movement assay. J Mol Biol. 1990 Nov 5;216(1):49–68. doi: 10.1016/S0022-2836(05)80060-9. [DOI] [PubMed] [Google Scholar]
- Harris D. E., Warshaw D. M. Smooth and skeletal muscle myosin both exhibit low duty cycles at zero load in vitro. J Biol Chem. 1993 Jul 15;268(20):14764–14768. [PubMed] [Google Scholar]
- Head J. G., Ritchie M. D., Geeves M. A. Characterization of the equilibrium between blocked and closed states of muscle thin filaments. Eur J Biochem. 1995 Feb 1;227(3):694–699. doi: 10.1111/j.1432-1033.1995.tb20190.x. [DOI] [PubMed] [Google Scholar]
- Heeley D. H., Watson M. H., Mak A. S., Dubord P., Smillie L. B. Effect of phosphorylation on the interaction and functional properties of rabbit striated muscle alpha alpha-tropomyosin. J Biol Chem. 1989 Feb 15;264(5):2424–2430. [PubMed] [Google Scholar]
- Hill L. E., Mehegan J. P., Butters C. A., Tobacman L. S. Analysis of troponin-tropomyosin binding to actin. Troponin does not promote interactions between tropomyosin molecules. J Biol Chem. 1992 Aug 15;267(23):16106–16113. [PubMed] [Google Scholar]
- Homsher E., Kim B., Bobkova A., Tobacman L. S. Calcium regulation of thin filament movement in an in vitro motility assay. Biophys J. 1996 Apr;70(4):1881–1892. doi: 10.1016/S0006-3495(96)79753-9. [DOI] [PMC free article] [PubMed] [Google Scholar]
- Homsher E., Wang F., Sellers J. R. Factors affecting movement of F-actin filaments propelled by skeletal muscle heavy meromyosin. Am J Physiol. 1992 Mar;262(3 Pt 1):C714–C723. doi: 10.1152/ajpcell.1992.262.3.C714. [DOI] [PubMed] [Google Scholar]
- Honda H., Asakura S. Calcium-triggered movement of regulated actin in vitro. A fluorescence microscopy study. J Mol Biol. 1989 Feb 20;205(4):677–683. doi: 10.1016/0022-2836(89)90313-6. [DOI] [PubMed] [Google Scholar]
- Howard J., Hunt A. J., Baek S. Assay of microtubule movement driven by single kinesin molecules. Methods Cell Biol. 1993;39:137–147. doi: 10.1016/s0091-679x(08)60167-3. [DOI] [PubMed] [Google Scholar]
- Hunt A. J., Gittes F., Howard J. The force exerted by a single kinesin molecule against a viscous load. Biophys J. 1994 Aug;67(2):766–781. doi: 10.1016/S0006-3495(94)80537-5. [DOI] [PMC free article] [PubMed] [Google Scholar]
- Julian F. J., Moss R. L. Effects of calcium and ionic strength on shortening velocity and tension development in frog skinned muscle fibres. J Physiol. 1981 Feb;311:179–199. doi: 10.1113/jphysiol.1981.sp013580. [DOI] [PMC free article] [PubMed] [Google Scholar]
- Kellermayer M. S., Granzier H. L. Calcium-dependent inhibition of in vitro thin-filament motility by native titin. FEBS Lett. 1996 Feb 19;380(3):281–286. doi: 10.1016/0014-5793(96)00055-5. [DOI] [PubMed] [Google Scholar]
- Kron S. J., Toyoshima Y. Y., Uyeda T. Q., Spudich J. A. Assays for actin sliding movement over myosin-coated surfaces. Methods Enzymol. 1991;196:399–416. doi: 10.1016/0076-6879(91)96035-p. [DOI] [PubMed] [Google Scholar]
- Leavis P. C., Gergely J. Thin filament proteins and thin filament-linked regulation of vertebrate muscle contraction. CRC Crit Rev Biochem. 1984;16(3):235–305. doi: 10.3109/10409238409108717. [DOI] [PubMed] [Google Scholar]
- Lehman W., Craig R., Vibert P. Ca(2+)-induced tropomyosin movement in Limulus thin filaments revealed by three-dimensional reconstruction. Nature. 1994 Mar 3;368(6466):65–67. doi: 10.1038/368065a0. [DOI] [PubMed] [Google Scholar]
- Lehrer S. S., Morris E. P. Dual effects of tropomyosin and troponin-tropomyosin on actomyosin subfragment 1 ATPase. J Biol Chem. 1982 Jul 25;257(14):8073–8080. [PubMed] [Google Scholar]
- Margossian S. S., Lowey S. Preparation of myosin and its subfragments from rabbit skeletal muscle. Methods Enzymol. 1982;85(Pt B):55–71. doi: 10.1016/0076-6879(82)85009-x. [DOI] [PubMed] [Google Scholar]
- Martyn D. A., Chase P. B., Hannon J. D., Huntsman L. L., Kushmerick M. J., Gordon A. M. Unloaded shortening of skinned muscle fibers from rabbit activated with and without Ca2+. Biophys J. 1994 Nov;67(5):1984–1993. doi: 10.1016/S0006-3495(94)80681-2. [DOI] [PMC free article] [PubMed] [Google Scholar]
- Martyn D. A., Coby R., Huntsman L. L., Gordon A. M. Force-calcium relations in skinned twitch and slow-tonic frog muscle fibres have similar sarcomere length dependencies. J Muscle Res Cell Motil. 1993 Feb;14(1):65–75. doi: 10.1007/BF00132181. [DOI] [PubMed] [Google Scholar]
- Martyn D. A., Gordon A. M. Force and stiffness in glycerinated rabbit psoas fibers. Effects of calcium and elevated phosphate. J Gen Physiol. 1992 May;99(5):795–816. doi: 10.1085/jgp.99.5.795. [DOI] [PMC free article] [PubMed] [Google Scholar]
- Martyn D. A., Gordon A. M. Length and myofilament spacing-dependent changes in calcium sensitivity of skeletal fibres: effects of pH and ionic strength. J Muscle Res Cell Motil. 1988 Oct;9(5):428–445. doi: 10.1007/BF01774069. [DOI] [PubMed] [Google Scholar]
- Metzger J. M. Effects of phosphate and ADP on shortening velocity during maximal and submaximal calcium activation of the thin filament in skeletal muscle fibers. Biophys J. 1996 Jan;70(1):409–417. doi: 10.1016/S0006-3495(96)79584-X. [DOI] [PMC free article] [PubMed] [Google Scholar]
- Metzger J. M., Moss R. L. Thin filament regulation of shortening velocity in rat skinned skeletal muscle: effects of osmotic compression. J Physiol. 1988 Apr;398:165–175. doi: 10.1113/jphysiol.1988.sp017036. [DOI] [PMC free article] [PubMed] [Google Scholar]
- Moss R. L., Diffee G. M., Greaser M. L. Contractile properties of skeletal muscle fibers in relation to myofibrillar protein isoforms. Rev Physiol Biochem Pharmacol. 1995;126:1–63. doi: 10.1007/BFb0049775. [DOI] [PubMed] [Google Scholar]
- Moss R. L. Effects on shortening velocity of rabbit skeletal muscle due to variations in the level of thin-filament activation. J Physiol. 1986 Aug;377:487–505. doi: 10.1113/jphysiol.1986.sp016199. [DOI] [PMC free article] [PubMed] [Google Scholar]
- Pan B. S., Gordon A. M., Luo Z. X. Removal of tropomyosin overlap modifies cooperative binding of myosin S-1 to reconstituted thin filaments of rabbit striated muscle. J Biol Chem. 1989 May 25;264(15):8495–8498. [PubMed] [Google Scholar]
- Pardee J. D., Spudich J. A. Purification of muscle actin. Methods Enzymol. 1982;85(Pt B):164–181. doi: 10.1016/0076-6879(82)85020-9. [DOI] [PubMed] [Google Scholar]
- Pate E., Bhimani M., Franks-Skiba K., Cooke R. Reduced effect of pH on skinned rabbit psoas muscle mechanics at high temperatures: implications for fatigue. J Physiol. 1995 Aug 1;486(Pt 3):689–694. doi: 10.1113/jphysiol.1995.sp020844. [DOI] [PMC free article] [PubMed] [Google Scholar]
- Pate E., Wilson G. J., Bhimani M., Cooke R. Temperature dependence of the inhibitory effects of orthovanadate on shortening velocity in fast skeletal muscle. Biophys J. 1994 May;66(5):1554–1562. doi: 10.1016/S0006-3495(94)80947-6. [DOI] [PMC free article] [PubMed] [Google Scholar]
- Potter J. D. Preparation of troponin and its subunits. Methods Enzymol. 1982;85(Pt B):241–263. doi: 10.1016/0076-6879(82)85024-6. [DOI] [PubMed] [Google Scholar]
- Regnier M., Martyn D. A., Chase P. B. Calmidazolium alters Ca2+ regulation of tension redevelopment rate in skinned skeletal muscle. Biophys J. 1996 Nov;71(5):2786–2794. doi: 10.1016/S0006-3495(96)79471-7. [DOI] [PMC free article] [PubMed] [Google Scholar]
- Sata M., Sugiura S., Yamashita H., Fujita H., Momomura S., Serizawa T. MCI-154 increases Ca2+ sensitivity of reconstituted thin filament. A study using a novel in vitro motility assay technique. Circ Res. 1995 Apr;76(4):626–633. doi: 10.1161/01.res.76.4.626. [DOI] [PubMed] [Google Scholar]
- Sata M., Sugiura S., Yamashita H., Momomura S., Serizawa T. Coupling between myosin ATPase cycle and creatinine kinase cycle facilitates cardiac actomyosin sliding in vitro. A clue to mechanical dysfunction during myocardial ischemia. Circulation. 1996 Jan 15;93(2):310–317. doi: 10.1161/01.cir.93.2.310. [DOI] [PubMed] [Google Scholar]
- Sata M., Yamashita H., Sugiura S., Fujita H., Momomura S., Serizawa T. A new in vitro motility assay technique to evaluate calcium sensitivity of the cardiac contractile proteins. Pflugers Arch. 1995 Jan;429(3):443–445. doi: 10.1007/BF00374162. [DOI] [PubMed] [Google Scholar]
- Schoenberg M. Effect of ionic strength on skinned rabbit psoas fibers in the presence of magnesium pyrophosphate. Biophys J. 1991 Sep;60(3):690–696. doi: 10.1016/S0006-3495(91)82098-7. [DOI] [PMC free article] [PubMed] [Google Scholar]
- Sellers J. R., Cuda G., Wang F., Homsher E. Myosin-specific adaptations of the motility assay. Methods Cell Biol. 1993;39:23–49. doi: 10.1016/s0091-679x(08)60159-4. [DOI] [PubMed] [Google Scholar]
- Smillie L. B. Preparation and identification of alpha- and beta-tropomyosins. Methods Enzymol. 1982;85(Pt B):234–241. doi: 10.1016/0076-6879(82)85023-4. [DOI] [PubMed] [Google Scholar]
- Tobacman L. S. Thin filament-mediated regulation of cardiac contraction. Annu Rev Physiol. 1996;58:447–481. doi: 10.1146/annurev.ph.58.030196.002311. [DOI] [PubMed] [Google Scholar]
- Toyoshima Y. Y., Kron S. J., Spudich J. A. The myosin step size: measurement of the unit displacement per ATP hydrolyzed in an in vitro assay. Proc Natl Acad Sci U S A. 1990 Sep;87(18):7130–7134. doi: 10.1073/pnas.87.18.7130. [DOI] [PMC free article] [PubMed] [Google Scholar]
- Uyeda T. Q., Kron S. J., Spudich J. A. Myosin step size. Estimation from slow sliding movement of actin over low densities of heavy meromyosin. J Mol Biol. 1990 Aug 5;214(3):699–710. doi: 10.1016/0022-2836(90)90287-V. [DOI] [PubMed] [Google Scholar]
- Wang F., Martin B. M., Sellers J. R. Regulation of actomyosin interactions in Limulus muscle proteins. J Biol Chem. 1993 Feb 15;268(5):3776–3780. [PubMed] [Google Scholar]
- Warrick H. M., Simmons R. M., Finer J. T., Uyeda T. Q., Chu S., Spudich J. A. In vitro methods for measuring force and velocity of the actin-myosin interaction using purified proteins. Methods Cell Biol. 1993;39:1–21. doi: 10.1016/s0091-679x(08)60158-2. [DOI] [PubMed] [Google Scholar]