Abstract
1. The interrelationship of metabolism of pyruvate or 3-hydroxybutyrate and glutamate transamination in rat brain mitochondria was studied. 2. If brain mitochondria are incubated in the presence of equimolar concentrations of pyruvate and glutamate and the K+ concentration is increased from 1 to 20mm, the rate of pyruvate utilization is increased 3-fold, but the rate of production of aspartate and 2-oxoglutarate is decreased by half. 3. Brain mitochondria incubated in the presence of a fixed concentration of glutamate (0.87 or 8.7mm) but different concentrations of pyruvate (0 to 1mm) produce aspartate at rates that decrease as the pyruvate concentration is increased. At 1mm-pyruvate, the rate of aspartate production is decreased to 40% of that when zero pyruvate was present. 4. Brain mitochondria incubated in the presence of glutamate and malate alone produce 2-oxoglutarate at rates stoicheiometric with the rate of aspartate production. Both the 2-oxoglutarate and aspartate accumulate extramitochondrially. 5. Externally added 2-oxoglutarate has little inhibitory effect (Ki approx. 31mm) on the production of aspartate from glutamate by rat brain mitochondria. 6. It is concluded that the inhibitory effect of increased C2 flux into the tricarboxylic acid cycle on glutamate transamination is caused by competition for oxaloacetate between the transaminase and citrate synthase. 7. Evidence is provided from a reconstituted malate–aspartate (or Borst) cycle with brain mitochondria that increased C2 flux into the tricarboxylic acid cycle from pyruvate may inhibit the reoxidation of exogenous NADH. These results are discussed in the light of the relationship between glycolysis and reoxidation of cytosolic NADH by the Borst cycle and the requirement of the brain for a continuous supply of energy.
Full text
PDF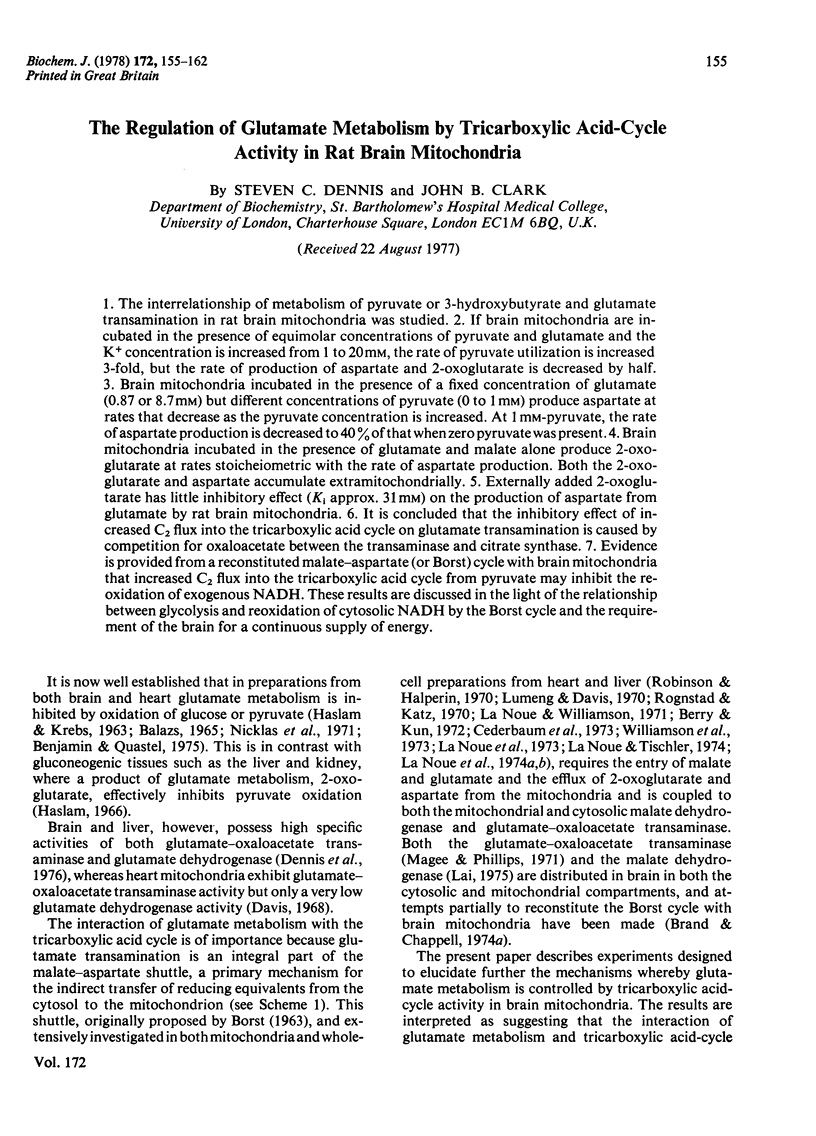
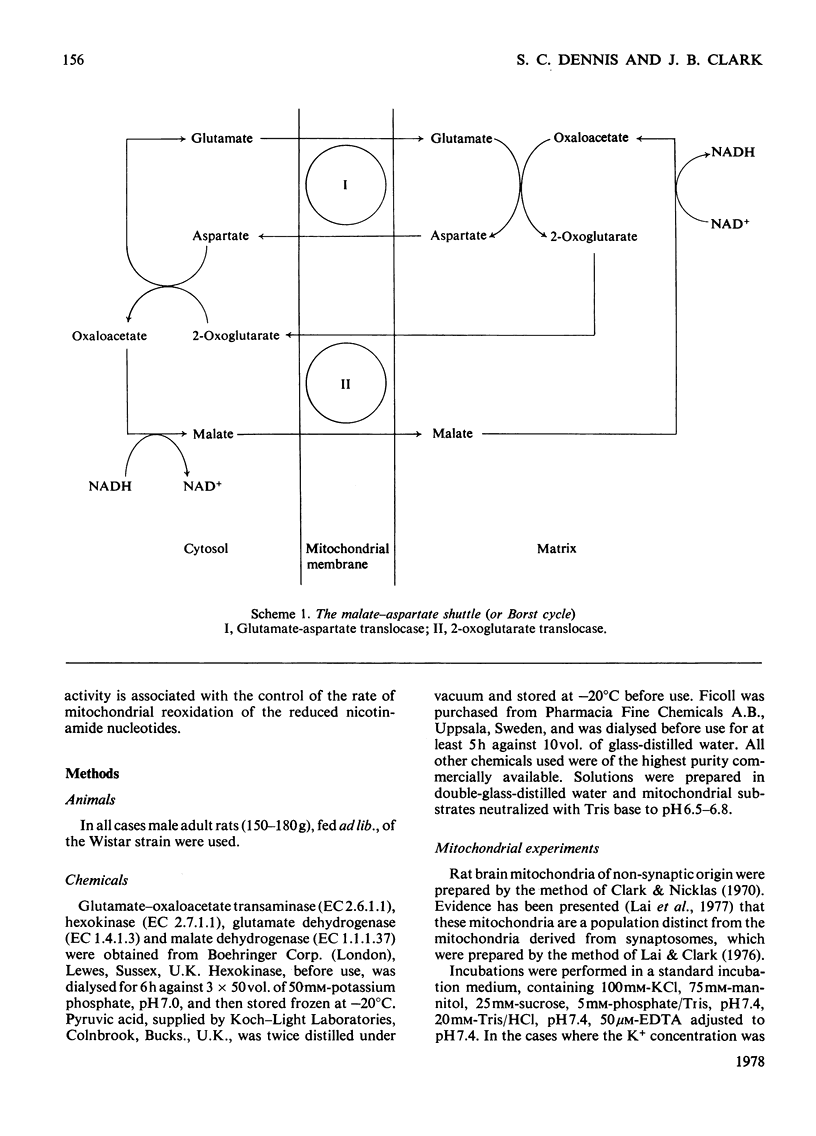
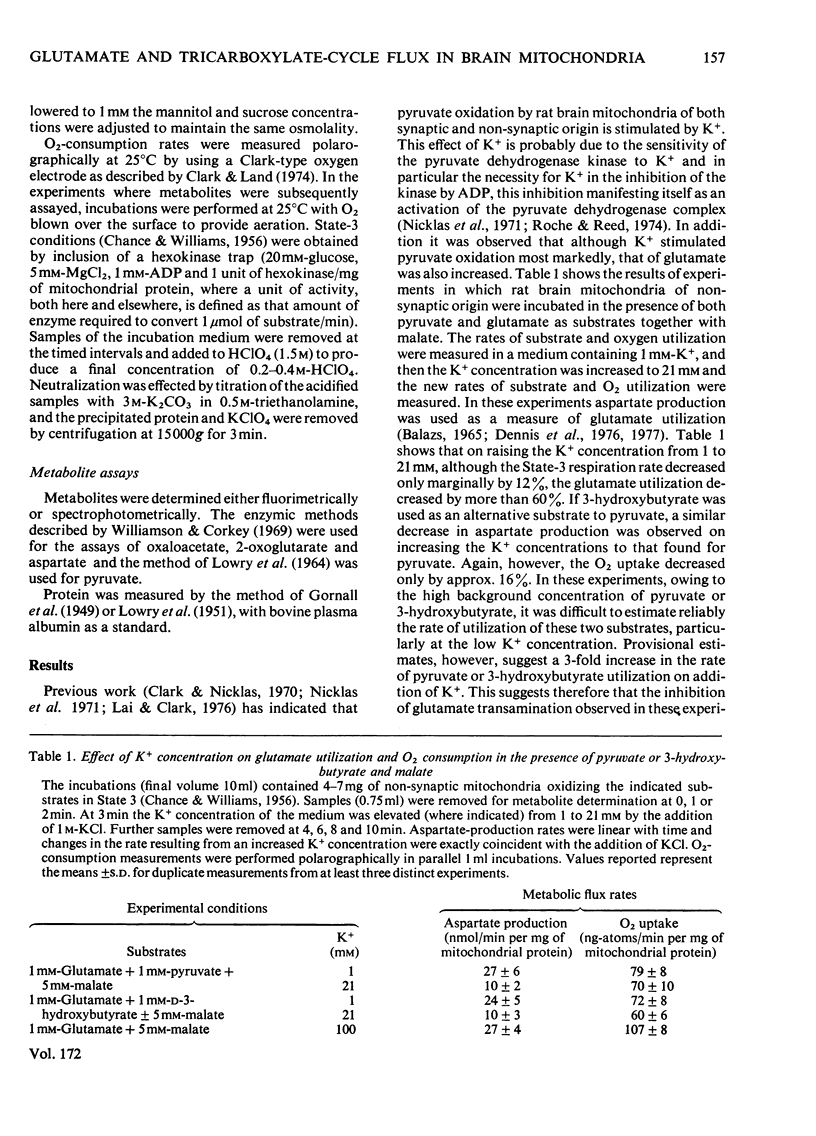
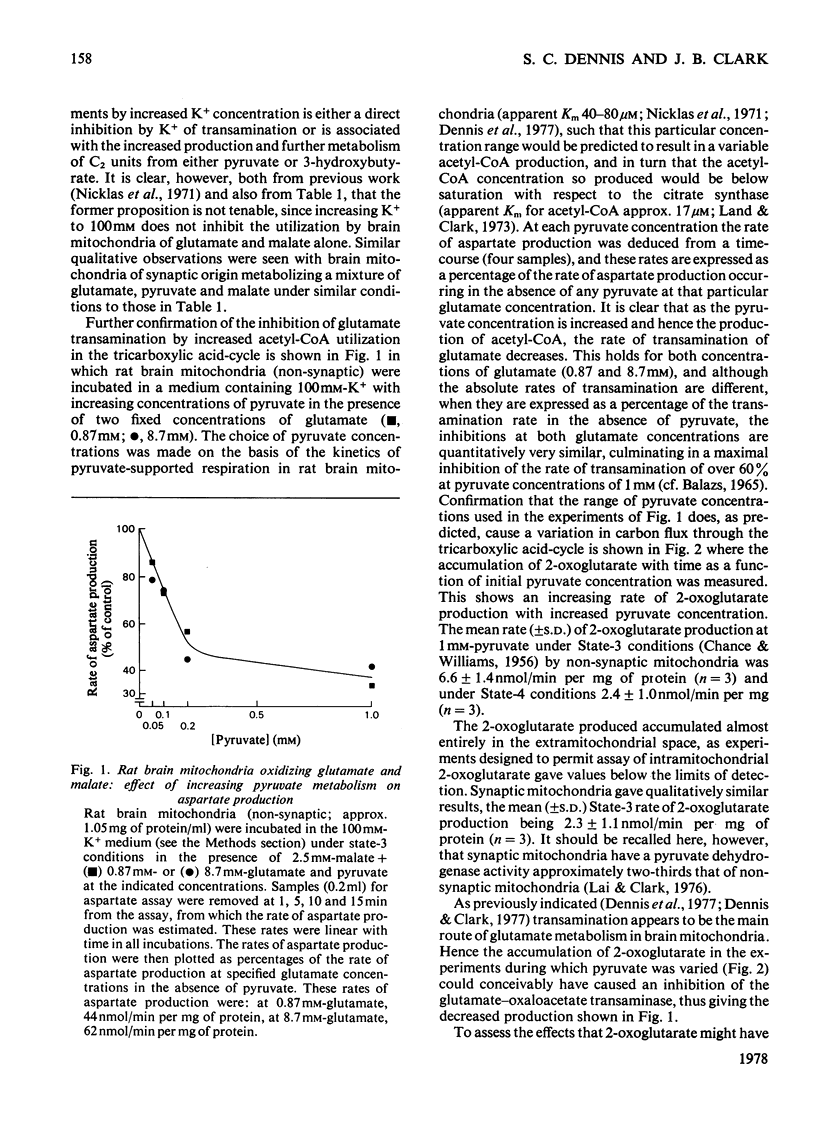
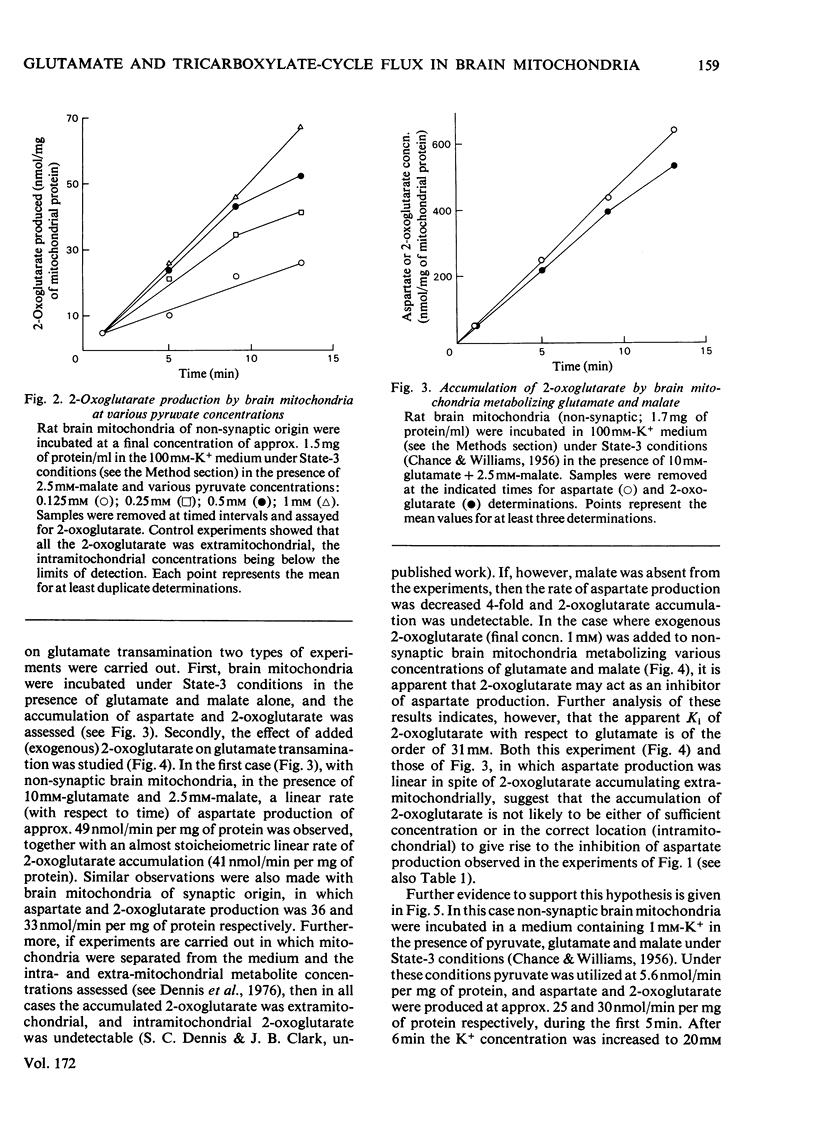
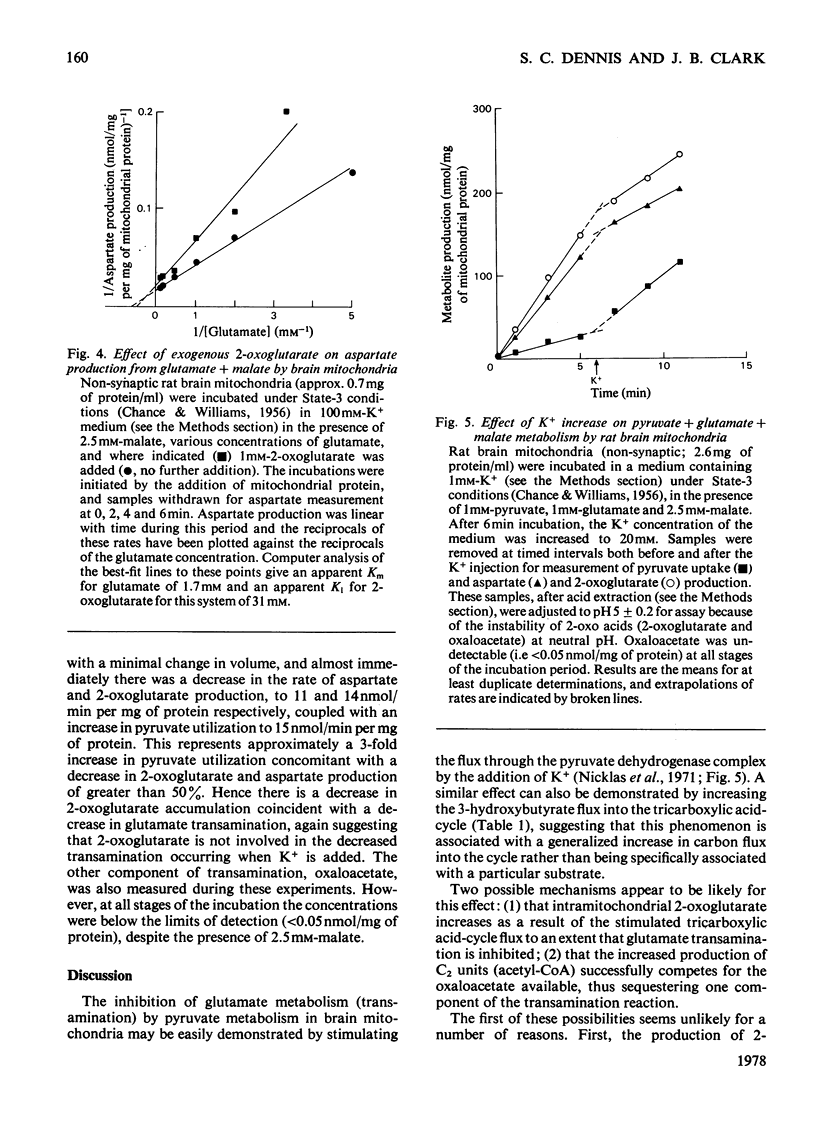
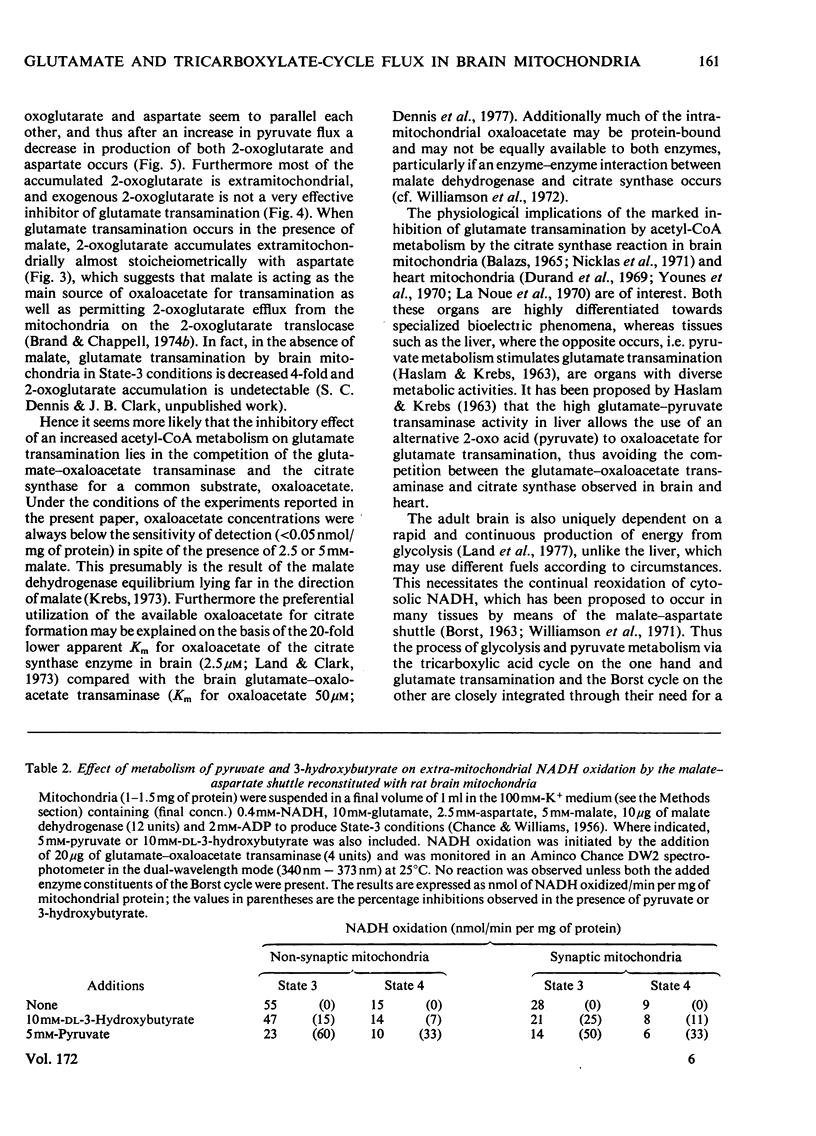
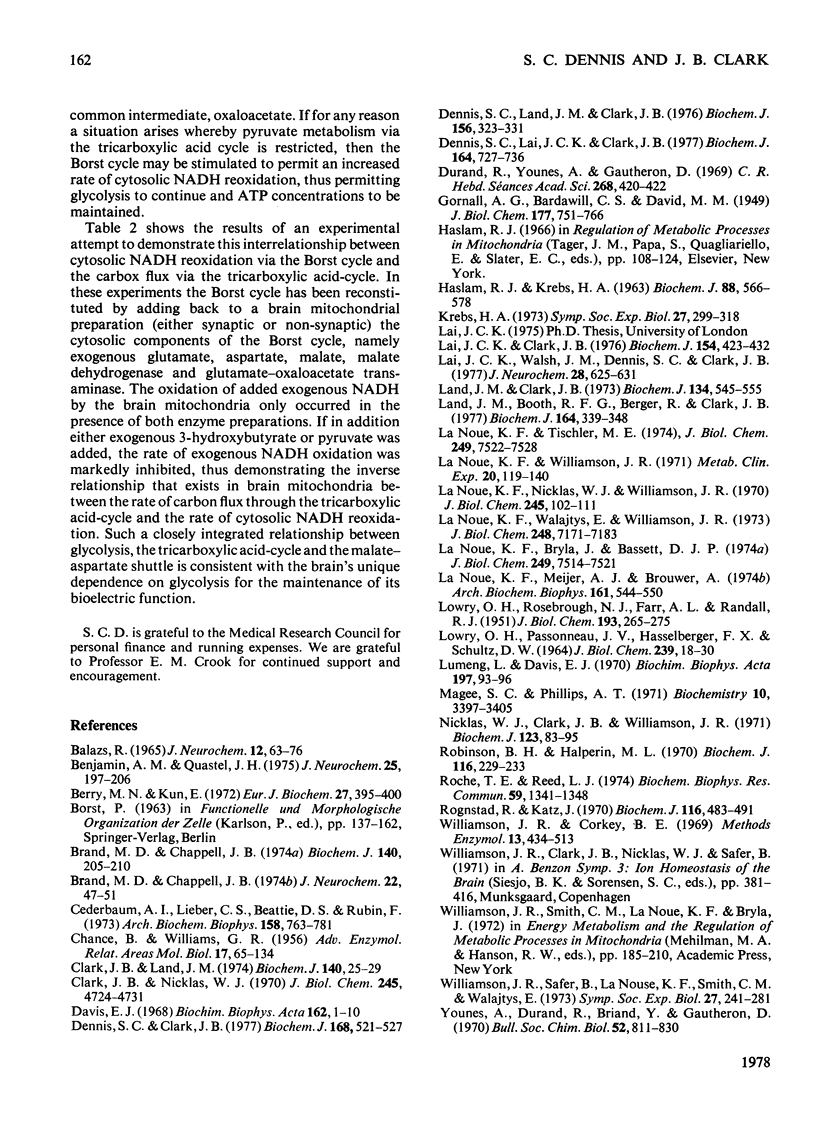
Selected References
These references are in PubMed. This may not be the complete list of references from this article.
- BALAZS R. CONTROL OF GLUTAMATE METABOLISM. THE EFFECT OF PYRUVATE. J Neurochem. 1965 Feb;12:63–76. doi: 10.1111/j.1471-4159.1965.tb11940.x. [DOI] [PubMed] [Google Scholar]
- Benjamin A. M., Quastel J. H. Metabolism of amino acids and ammonia in rat brain cortex slices in vitro: a possible role of ammonia in brain function. J Neurochem. 1975 Sep;25(3):197–206. doi: 10.1111/j.1471-4159.1975.tb06953.x. [DOI] [PubMed] [Google Scholar]
- Berry M. N., Kun E. Rate-limiting steps of gluconeogenesis in liver cells as determined with the aid of fluoro-dicarboxylic acids. Eur J Biochem. 1972 May 23;27(2):395–400. doi: 10.1111/j.1432-1033.1972.tb01850.x. [DOI] [PubMed] [Google Scholar]
- Brand M. D., Chappell J. B. Glutamate and aspartate transport in rat brain mitochondria. Biochem J. 1974 May;140(2):205–210. doi: 10.1042/bj1400205. [DOI] [PMC free article] [PubMed] [Google Scholar]
- Brand M. D., Chappell J. B. Permeability of mitochondria from rat brain and rat liver to GABA. J Neurochem. 1974 Jan;22(1):47–51. doi: 10.1111/j.1471-4159.1974.tb12177.x. [DOI] [PubMed] [Google Scholar]
- CHANCE B., WILLIAMS G. R. The respiratory chain and oxidative phosphorylation. Adv Enzymol Relat Subj Biochem. 1956;17:65–134. doi: 10.1002/9780470122624.ch2. [DOI] [PubMed] [Google Scholar]
- Cederbaum A. I., Lieber C. S., Beattie D. S., Rubin E. Characterization of shuttle mechanisms for the transport of reducing equivalents into mitochondria. Arch Biochem Biophys. 1973 Oct;158(2):763–781. doi: 10.1016/0003-9861(73)90571-7. [DOI] [PubMed] [Google Scholar]
- Clark J. B., Land J. M. Differential effects of 2-oxo acids on pyruvate utilization and fatty acid synthesis in rat brain. Biochem J. 1974 Apr;140(1):25–29. doi: 10.1042/bj1400025. [DOI] [PMC free article] [PubMed] [Google Scholar]
- Clark J. B., Nicklas W. J. The metabolism of rat brain mitochondria. Preparation and characterization. J Biol Chem. 1970 Sep 25;245(18):4724–4731. [PubMed] [Google Scholar]
- Davis E. J. On the nature of malonate-insensitive oxidation of pyruvate and glutamate by heart sarcosomes. Biochim Biophys Acta. 1968 Jul 16;162(1):1–10. doi: 10.1016/0005-2728(68)90208-9. [DOI] [PubMed] [Google Scholar]
- Dennis S. C., Clark J. B. The pathway of glutamate metabolism in rat brain mitochondria. Biochem J. 1977 Dec 15;168(3):521–527. doi: 10.1042/bj1680521. [DOI] [PMC free article] [PubMed] [Google Scholar]
- Dennis S. C., Lai J. C., Clark J. B. Comparative studies on glutamate metabolism in synpatic and non-synaptic rat brain mitochondria. Biochem J. 1977 Jun 15;164(3):727–736. doi: 10.1042/bj1640727. [DOI] [PMC free article] [PubMed] [Google Scholar]
- Dennis S. C., Land J. M., Clark J. B. Glutamate metabolism and transport in rat brain mitochondria. Biochem J. 1976 May 15;156(2):323–331. doi: 10.1042/bj1560323. [DOI] [PMC free article] [PubMed] [Google Scholar]
- Durand R., Younes A., Gautheron D. Interaction de l'oxydation du pyruvate et du glutamate au niveau des mitochondries de caeur de porc. C R Acad Sci Hebd Seances Acad Sci D. 1969 Jan 13;268(2):420–422. [PubMed] [Google Scholar]
- HASLAM R. J., KREBS H. A. THE METABOLISM OF GLUTAMATE IN HOMOGENATES AND SLICES OF BRAIN CORTEX. Biochem J. 1963 Sep;88:566–578. doi: 10.1042/bj0880566. [DOI] [PMC free article] [PubMed] [Google Scholar]
- Krebs H. A. Pyridine nucleotides and rate control. Symp Soc Exp Biol. 1973;27:299–318. [PubMed] [Google Scholar]
- LOWRY O. H., PASSONNEAU J. V., HASSELBERGER F. X., SCHULZ D. W. EFFECT OF ISCHEMIA ON KNOWN SUBSTRATES AND COFACTORS OF THE GLYCOLYTIC PATHWAY IN BRAIN. J Biol Chem. 1964 Jan;239:18–30. [PubMed] [Google Scholar]
- LaNoue K. F., Bryla J., Bassett D. J. Energy-driven aspartate efflux from heart and liver mitochondria. J Biol Chem. 1974 Dec 10;249(23):7514–7521. [PubMed] [Google Scholar]
- LaNoue K. F., Meijer A. J., Brouwer A. Evidence for electrogenic aspartate transport in rat liver mitochondria. Arch Biochem Biophys. 1974 Apr 2;161(2):544–550. doi: 10.1016/0003-9861(74)90337-3. [DOI] [PubMed] [Google Scholar]
- LaNoue K. F., Tischler M. E. Electrogenic characteristics of the mitochondrial glutamate-aspartate antiporter. J Biol Chem. 1974 Dec 10;249(23):7522–7528. [PubMed] [Google Scholar]
- LaNoue K. F., Walajtys E. I., Williamson J. R. Regulation of glutamate metabolism and interactions with the citric acid cycle in rat heart mitochondria. J Biol Chem. 1973 Oct 25;248(20):7171–7183. [PubMed] [Google Scholar]
- LaNoue K. F., Williamson J. R. Interrelationships between malate-aspartate shuttle and citric acid cycle in rat heart mitochondria. Metabolism. 1971 Feb;20(2):119–140. doi: 10.1016/0026-0495(71)90087-4. [DOI] [PubMed] [Google Scholar]
- LaNoue K., Nicklas W. J., Williamson J. R. Control of citric acid cycle activity in rat heart mitochondria. J Biol Chem. 1970 Jan 10;245(1):102–111. [PubMed] [Google Scholar]
- Lai J. C., Clark J. B. Preparation and properties of mitochondria derived from synaptosomes. Biochem J. 1976 Feb 15;154(2):423–432. doi: 10.1042/bj1540423. [DOI] [PMC free article] [PubMed] [Google Scholar]
- Lai J. C., Walsh J. M., Dennis S. C., Clark J. B. Synaptic and non-synaptic mitochondria from rat brain: isolation and characterization. J Neurochem. 1977 Mar;28(3):625–631. doi: 10.1111/j.1471-4159.1977.tb10434.x. [DOI] [PubMed] [Google Scholar]
- Land J. M., Booth R. F., Berger R., Clark J. B. Development of mitochondrial energy metabolism in rat brain. Biochem J. 1977 May 15;164(2):339–348. doi: 10.1042/bj1640339. [DOI] [PMC free article] [PubMed] [Google Scholar]
- Land J. M., Clark J. B. Effect of phenylpyruvate on enzymes involved in fatty acid synthesis in rat brain. Biochem J. 1973 Jun;134(2):545–555. doi: 10.1042/bj1340545. [DOI] [PMC free article] [PubMed] [Google Scholar]
- Lumeng L., Davis E. J. Demonstration in vitro of competition between added NADH and glutamate for oxidation by liver mitochondria. Biochim Biophys Acta. 1970 Jan 13;197(1):93–96. doi: 10.1016/0005-2728(70)90014-9. [DOI] [PubMed] [Google Scholar]
- Magee S. C., Phillips A. T. Molecular properties of the multiple aspartate aminotransferases purified from rat brain. Biochemistry. 1971 Aug 31;10(18):3397–3405. doi: 10.1021/bi00794a013. [DOI] [PubMed] [Google Scholar]
- Nicklas W. J., Clark J. B., Williamson J. R. Metabolism of rat brain mitochondria. Studies on the potassium ion-stimulated oxidation of pyruvate. Biochem J. 1971 Jun;123(1):83–95. doi: 10.1042/bj1230083. [DOI] [PMC free article] [PubMed] [Google Scholar]
- Robinson B. H., Halperin M. L. Transport of reduced nicotinamide-adenine dinucleotide into mitochondria of rat white adipose tissue. Biochem J. 1970 Jan;116(2):229–233. doi: 10.1042/bj1160229. [DOI] [PMC free article] [PubMed] [Google Scholar]
- Roche T. E., Reed L. J. Monovalent cation requirement for ADP inhibition of pyruvate dehydrogenase kinase. Biochem Biophys Res Commun. 1974 Aug 19;59(4):1341–1348. doi: 10.1016/0006-291x(74)90461-6. [DOI] [PubMed] [Google Scholar]
- Rognstad R., Katz J. Gluconeogenesis in the kidney cortex. Effects of D-malate and amino-oxyacetate. Biochem J. 1970 Feb;116(3):483–491. doi: 10.1042/bj1160483. [DOI] [PMC free article] [PubMed] [Google Scholar]
- Williamson J. R., Safer B., LaNoue K. F., Smith C. M., Walajtys E. Mitochondrial-cytosolic interactions in cardiac tissue: role of the malate-aspartate cycle in the removal of glycolytic NADH from the cytosol. Symp Soc Exp Biol. 1973;27:241–281. [PubMed] [Google Scholar]
- Younes A., Durand R., Briand Y., Gautheron D. Interaction des oxydations du pyruvate et du glutamate au niveau des mitochondries de coeur de porc. Bull Soc Chim Biol (Paris) 1970 Sep 15;52(7):811–830. [PubMed] [Google Scholar]