Abstract
1. When Triton-filled lysosomes from rat liver are incubated for up to 50min at 37°C, pH7.4, in 0.25m-sucrose, no loss of latency of N-acetyl-β-glucosaminidase or p-nitrophenyl phosphatase occurs unless the incubated lysosomes are cooled to approx. 15°C. 2. It is suggested that a phase change takes place in the incubated lysosomal membranes on cooling; it starts at approx. 15°C and probably is not complete at 0°C. 3. Incubation of the lysosomes causes an increased potential for loss of latency of the lysosomal enzymes. This potential is not fully expressed at elevated temperature (e.g. 37°C), but is expressed on cooling. 4. The increase at elevated temperature in potential for loss of latency exhibits biphasic kinetics, with an initial rapid phase followed by a slower phase, which is linear with respect to time. The extra loss of latency resulting from the rapid phase in proportional to the temperature of the incubation. 5. Arrhenius plots of the increase is potential for loss of latency during the slow phase for N-acetyl-β-glucosaminidase and p-nitrophenyl phosphatase exhibit marked deviations from linearity beginning at approx. 15°C. This suggests that the increase in potential for loss of latency is affected by a phase change that occurs around this temperature. 6. Activation energies for the increase in potential for loss of latency at and above 22°C are 53.1±5.4kJ/mol (12.7±1.3kcal/mol) for N-acetyl-β-glucosaminidase and 45.2±7.5kJ/mol (10.8±1.8kcal/mol) for p-nitrophenyl phosphatase. It is postulated that these energies reflect enzymic action, the products of which cause loss of latency to occur on cooling.
Full text
PDF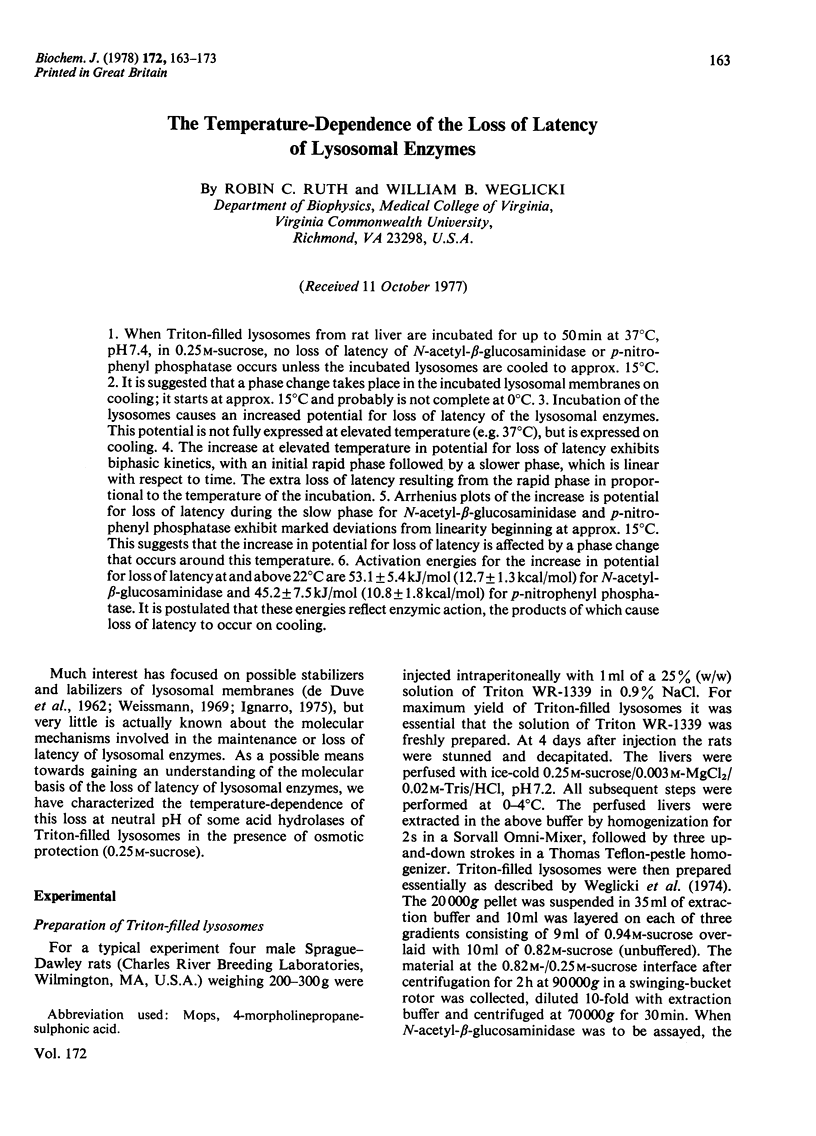
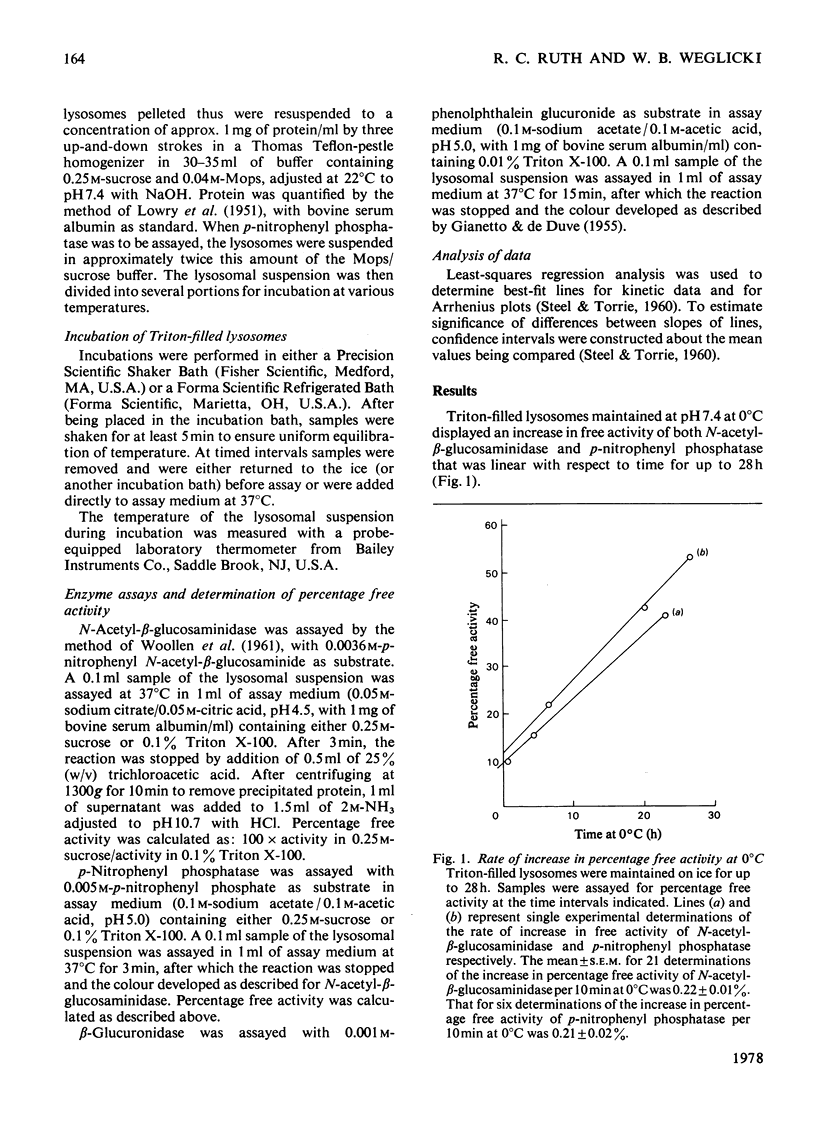
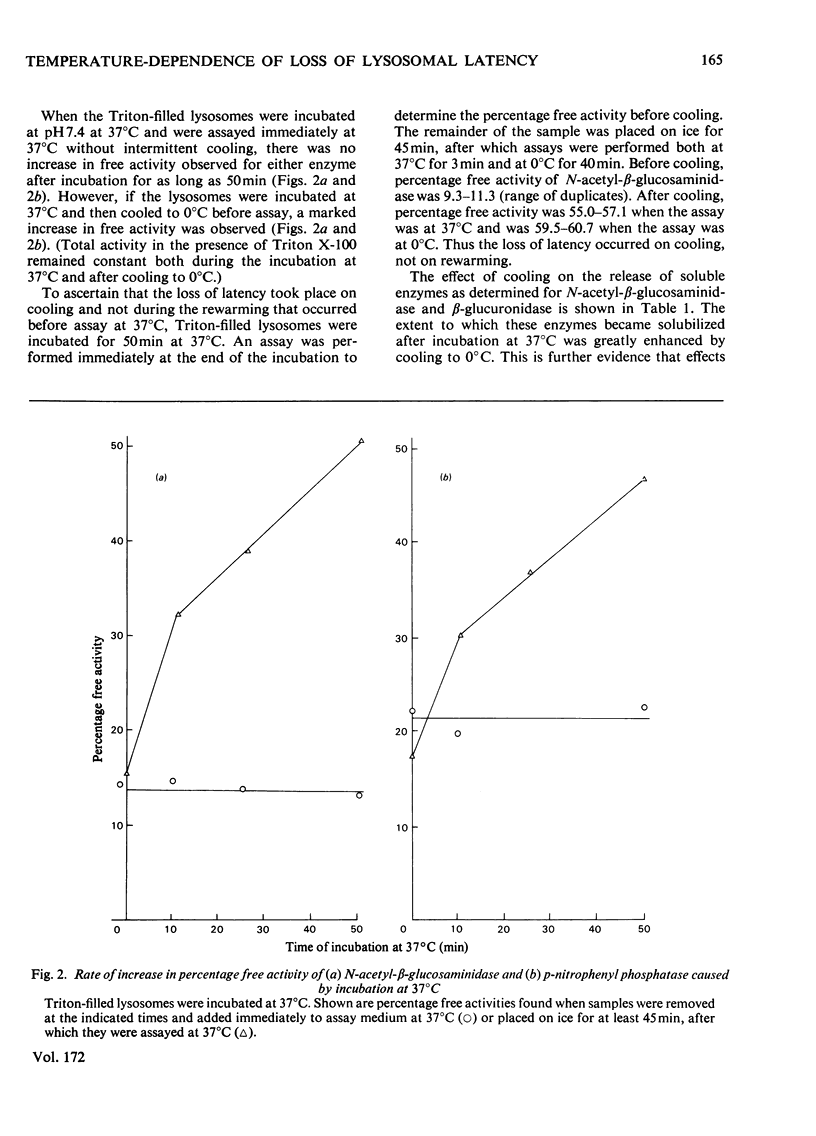
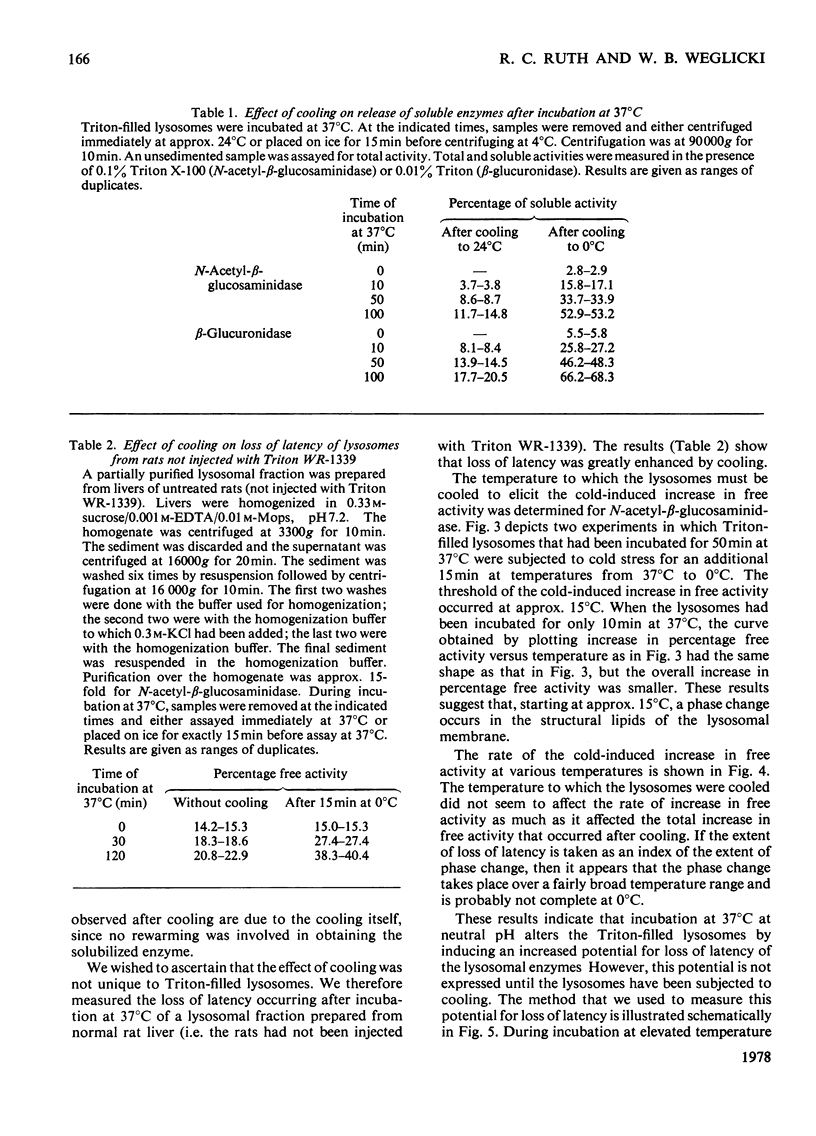
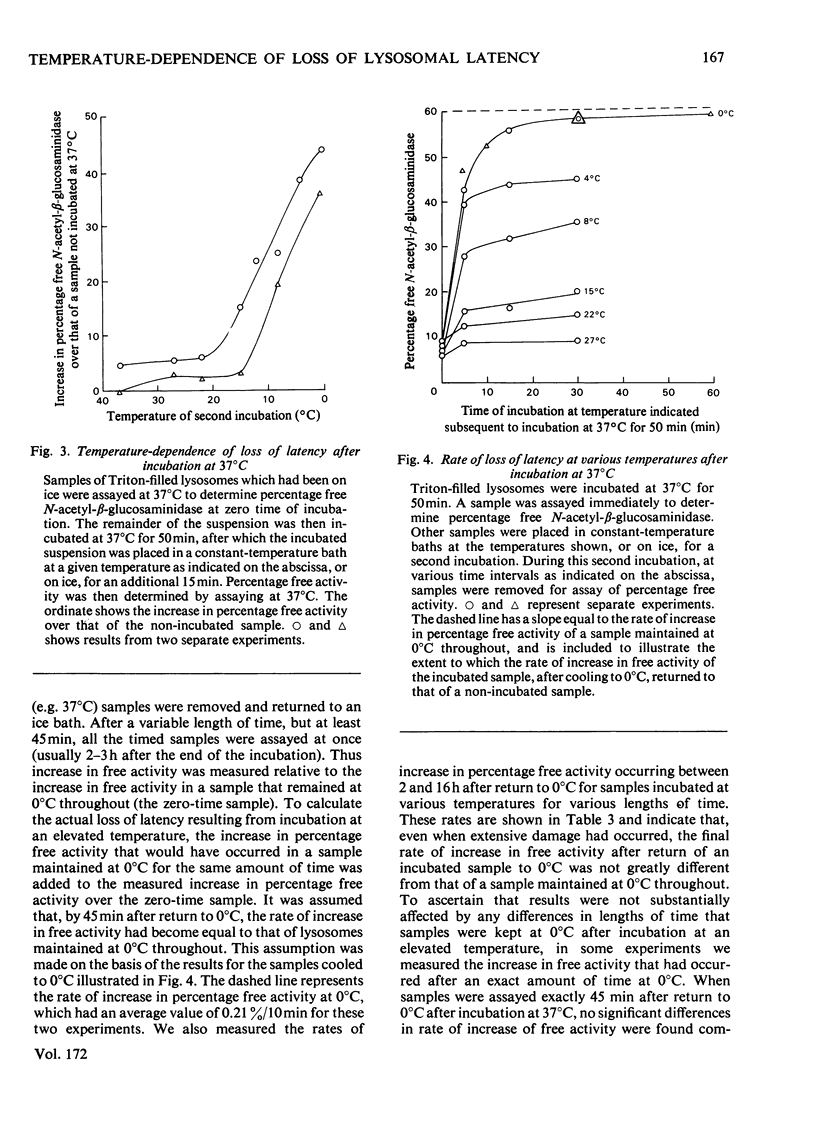
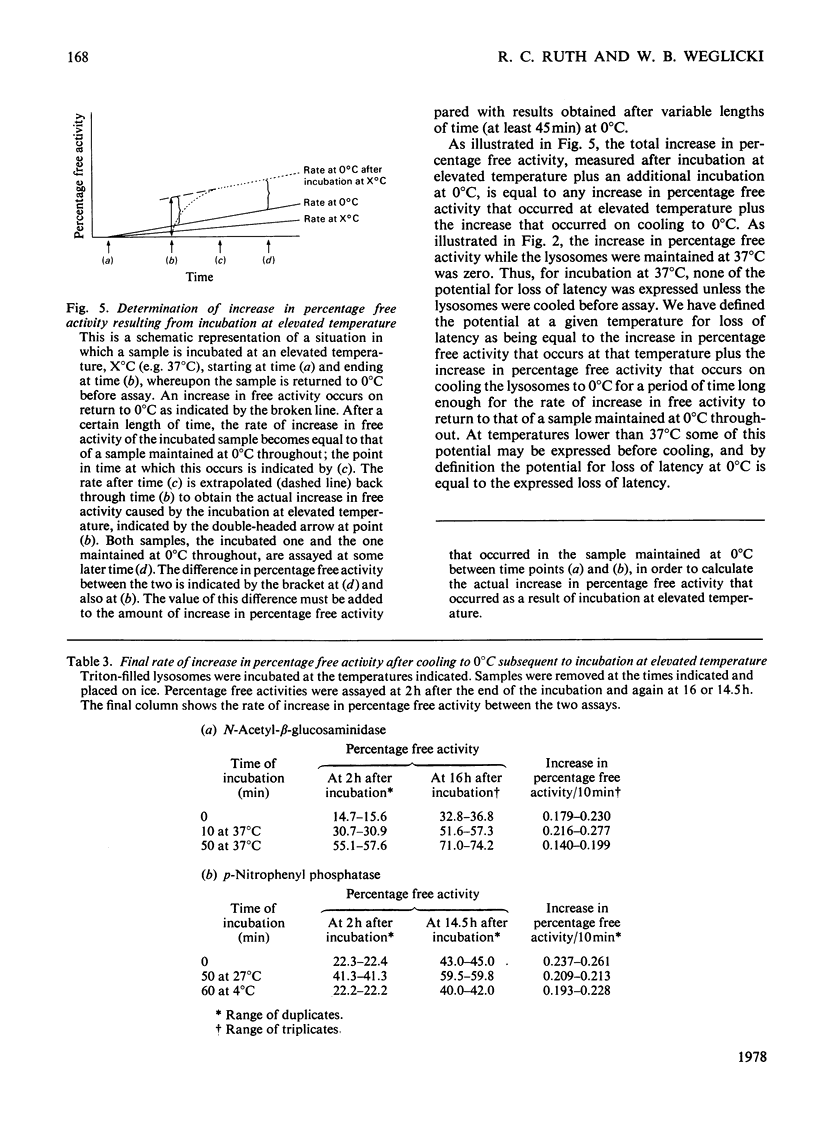
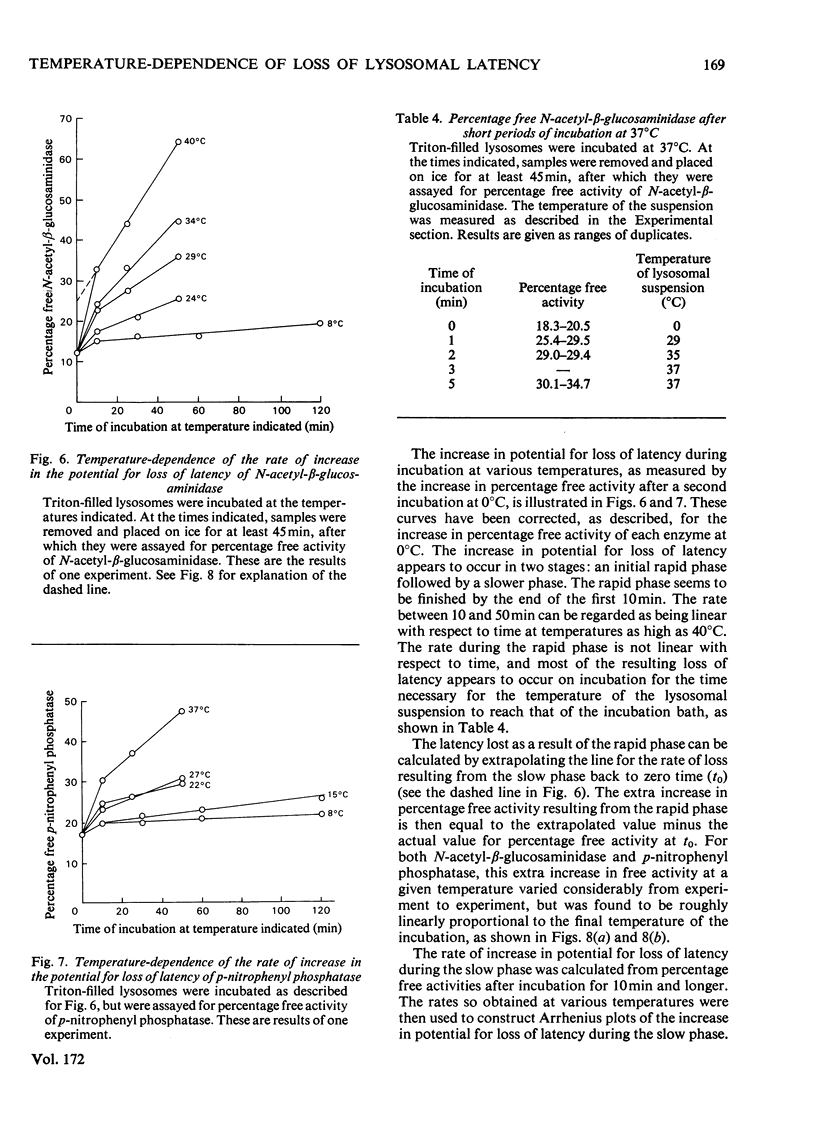
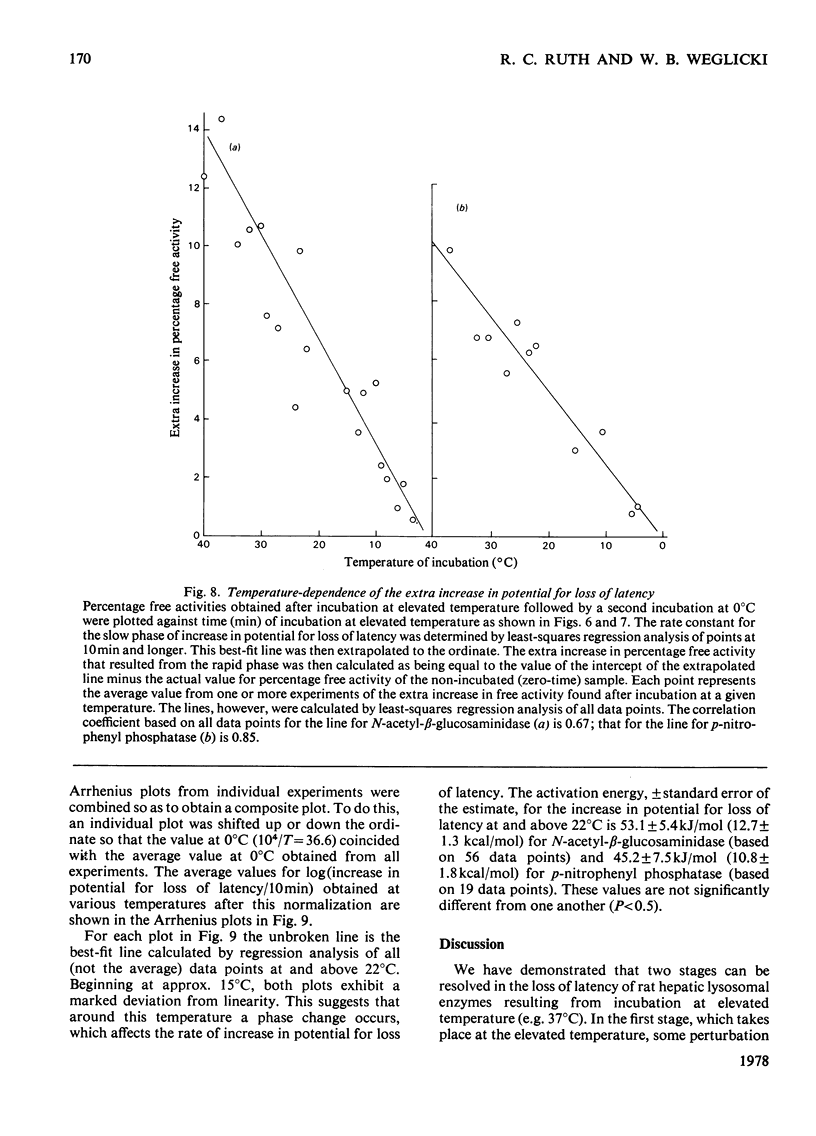
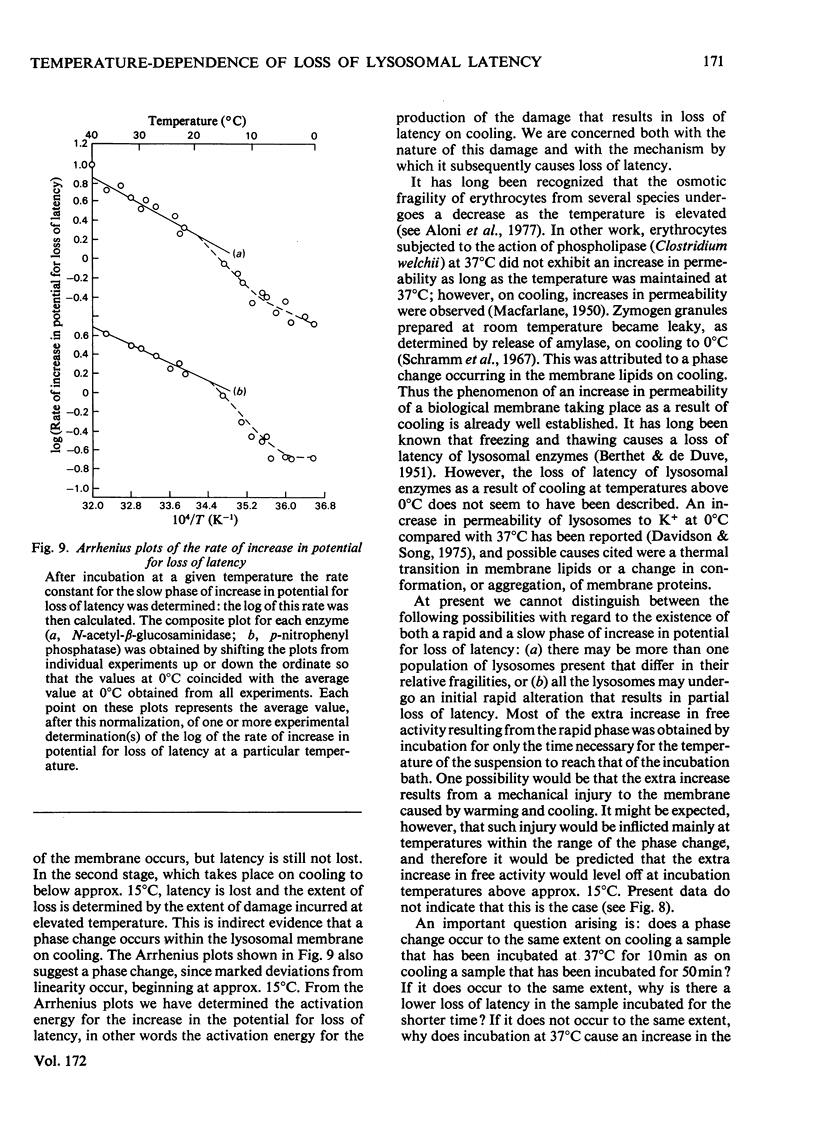
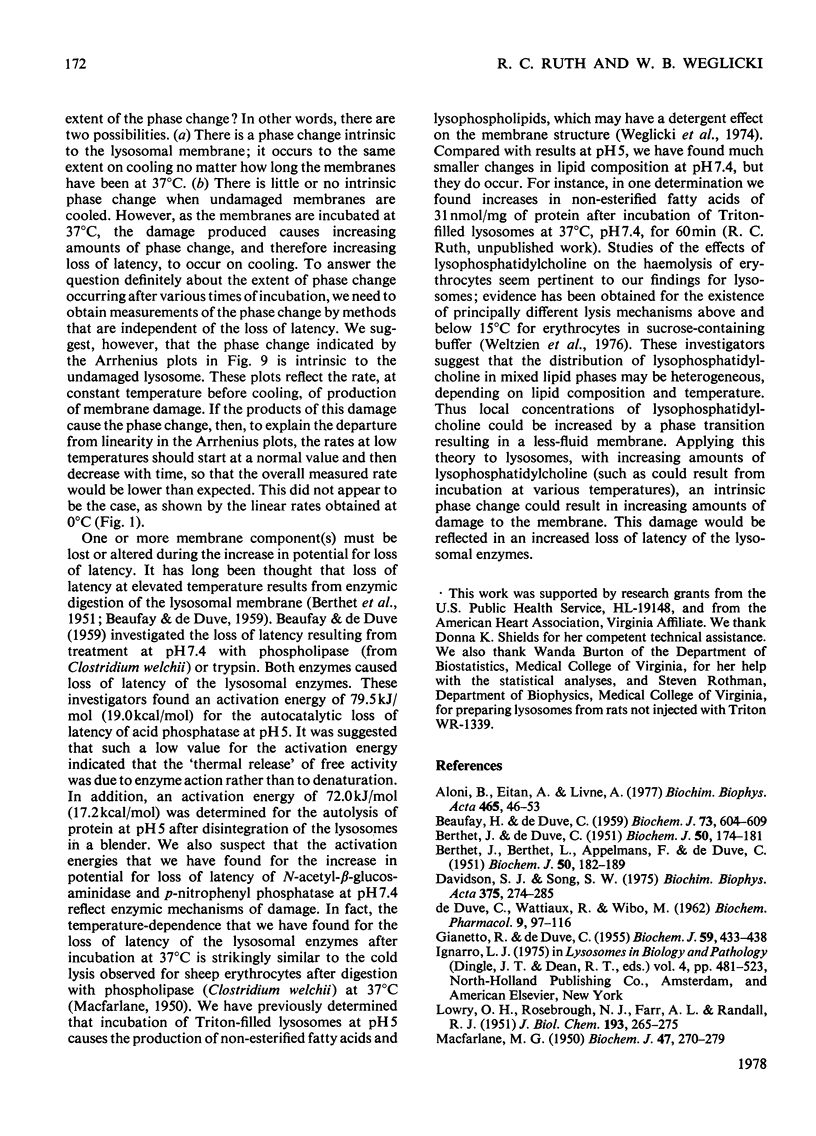
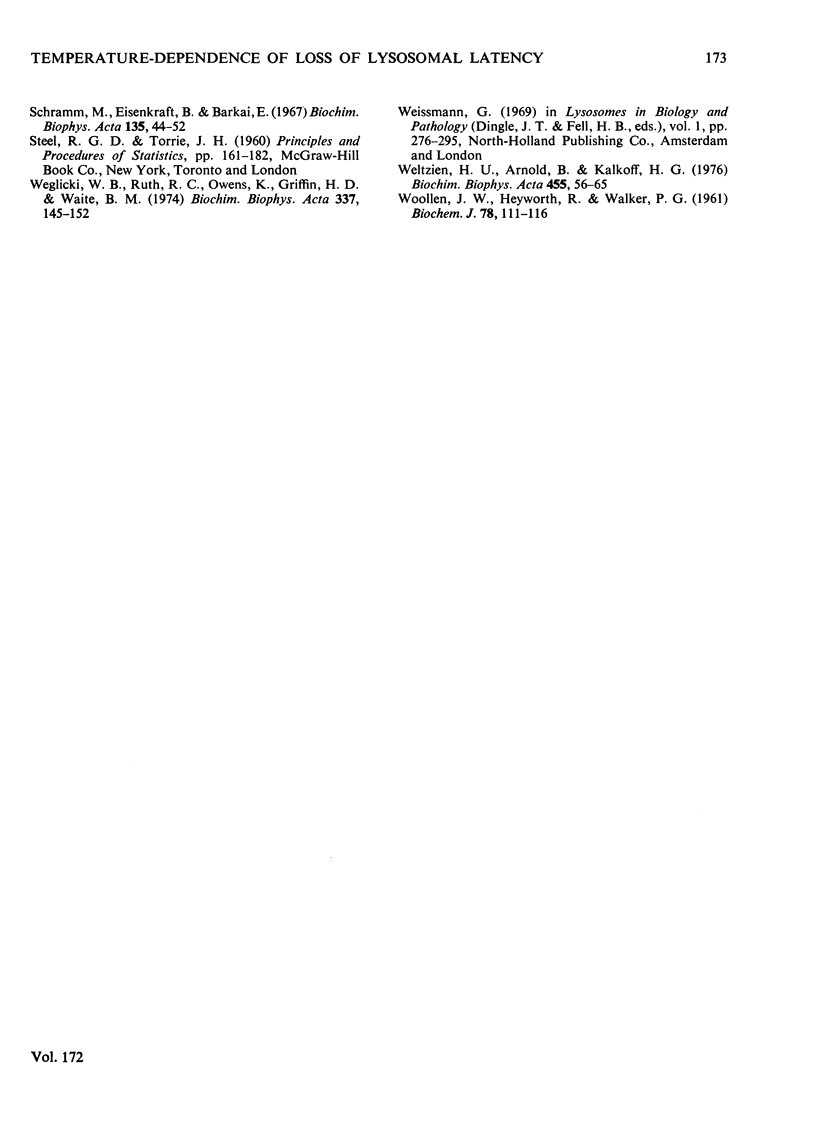
Selected References
These references are in PubMed. This may not be the complete list of references from this article.
- Aloni B., Eitan A., Livne A. The erythrocyte membrane site for the effect of temperature on osmotic fragility. Biochim Biophys Acta. 1977 Feb 14;465(1):46–53. doi: 10.1016/0005-2736(77)90354-6. [DOI] [PubMed] [Google Scholar]
- BEAUFAY H., DE DUVE C. Tissue fractionation studies. 9. Enzymic release of bound hydrolases. Biochem J. 1959 Dec;73:604–609. doi: 10.1042/bj0730604. [DOI] [PMC free article] [PubMed] [Google Scholar]
- BERTHET J., BERTHET L., APPELMANS F., DE DUVE C. Tissue fractionation studies. II. The nature of the linkage between acid phosphatase and mitochondria in rat-liver tissue. Biochem J. 1951 Dec;50(2):182–189. doi: 10.1042/bj0500182. [DOI] [PMC free article] [PubMed] [Google Scholar]
- BERTHET J., DE DUVE C. Tissue fractionation studies. I. The existence of a mitochondria-linked, enzymically inactive form of acid phosphatase in rat-liver tissue. Biochem J. 1951 Dec;50(2):174–181. doi: 10.1042/bj0500174. [DOI] [PMC free article] [PubMed] [Google Scholar]
- DE DUVE C., WATTIAUX R., WIBO M. Effects of fat-soluble compounds on lysosomes in vitro. Biochem Pharmacol. 1962 Aug;9:97–116. doi: 10.1016/0006-2952(62)90015-1. [DOI] [PubMed] [Google Scholar]
- Davidson S. J., Song S. W. A thermally induced alteration in lysosome membranes: salt permeability at 0 and 37 degrees C. Biochim Biophys Acta. 1975 Jan 28;375(2):274–285. doi: 10.1016/0005-2736(75)90195-9. [DOI] [PubMed] [Google Scholar]
- GIANETTO R., DE DUVE C. Tissue fractionation studies. 4. Comparative study of the binding of acid phosphatase, beta-glucuronidase and cathepsin by rat-liver particles. Biochem J. 1955 Mar;59(3):433–438. doi: 10.1042/bj0590433. [DOI] [PMC free article] [PubMed] [Google Scholar]
- LOWRY O. H., ROSEBROUGH N. J., FARR A. L., RANDALL R. J. Protein measurement with the Folin phenol reagent. J Biol Chem. 1951 Nov;193(1):265–275. [PubMed] [Google Scholar]
- MACFARLANE M. G. The biochemistry of bacterial toxins; variation in haemolytic activity of immunologically distinct lecithinases towards erythrocytes from different species. Biochem J. 1950 Sep;47(3):270–279. doi: 10.1042/bj0470270. [DOI] [PMC free article] [PubMed] [Google Scholar]
- Schramm M., Eisenkraft B., Barkai E. Cold-induced leakage of amylase from the zymogen granule and sealing of its membrane by specific lipids. Biochim Biophys Acta. 1967 Feb 1;135(1):44–52. doi: 10.1016/0005-2736(67)90006-5. [DOI] [PubMed] [Google Scholar]
- WOOLLEN J. W., HEYWORTH R., WALKER P. G. Studies on glucosaminidase. 3. Testicular N-acetyl-beta-glucosaminidase and N-acetyl-beta-galactosaminidase. Biochem J. 1961 Jan;78:111–116. doi: 10.1042/bj0780111. [DOI] [PMC free article] [PubMed] [Google Scholar]
- Weglicki W. B., Ruth R. C., Owens K., Griffin H. D., Waite B. M. Changes in lipid composition of triton-filled lysosomes during lysis. Association with activation of acid-active lipases and phospholipases. Biochim Biophys Acta. 1974 Jan 23;337(1):145–152. doi: 10.1016/0005-2760(74)90049-6. [DOI] [PubMed] [Google Scholar]
- Weltzien H. U., Arnold B., Kalkoff H. G. Quantitative studies on lysolecithin mediated hemolysis. Benzylated lysolecithin as a probe to study effects of temperature and red cell species on the hemolytic reaction. Biochim Biophys Acta. 1976 Nov 11;455(1):56–65. doi: 10.1016/0005-2736(76)90153-x. [DOI] [PubMed] [Google Scholar]