Abstract
1. In kidney-cortex slices from the well-fed rat, glucose (5mm) supplied 25–30% of the respiratory fuel; in the starved state, the corresponding value was 10%. These results are based on measurements of the net uptake of glucose and of the specific radioactivity of labelled carbon dioxide formed in the presence of [U-14C]-glucose. 2. Added acetoacetate (5mm) or butyrate (10mm) provided up to 80%, and added oleate (2mm) up to 50% of the fuel of respiration. The oxidation of endogenous substrates was suppressed correspondingly. 3. More [U-14C]oleate was removed by the tissue than could be oxidized by the amount of oxygen taken up; less than 25% of the oleate removed was converted into respiratory carbon dioxide and about two-thirds was incorporated into the tissue lipids. The rate of oleate incorporation into the neutral-lipid fraction was calculated to be equivalent to the rate of oxidation of endogenous fat, which provided the chief remaining fuel. 4. The contribution of endogenous substrates to the respiration (50%) in the presence of added oleate is taken to reflect either a high turnover rate of the endogenous neutral lipids (approx. half-life 2·5hr.) or a raised rate of lipolysis caused by the experimental conditions in vitro. 5. Added l-α-glycerophosphate (2·5mm) increased oleate incorporation into the neutral-lipid fraction by up to 40% (i.e. caused a net synthesis of triglyceride). 6. Lactate (2·5mm) added as sole substrate supplied 30% of the respiratory fuel, but with added oleate (2mm) lactate was converted quantitatively into glucose. Oleate stimulated the rate of gluconeogenesis from lactate by 45%. 7. The oxidation of both long-chain and short-chain even-numbered fatty acids was accompanied by ketone-body formation. Ketone-body synthesis from oleate, but not from butyrate, increased six- to seven-fold after 48hr. of starvation. The maximum rates of renal ketogenesis (80μmoles/hr./g. dry wt., with butyrate) were about 20% of the maximum rates observed in the liver (on a weight-for-weight basis) and accounted for, at most, 35% of the fatty acid removed. 8. dl-Carnitine (1·0mm) had no effect on the rates of uptake of acetate, butyrate or oleate or on the rate of radioactive carbon dioxide formation from [U-14C]oleate, but increased ketone-body formation from oleate by more than 100%. Ketone-body formation from butyrate was not increased. 9. There is evidence supporting the assumption that there are cells in which gluconeogenesis and ketogenesis occur together, characterized by equal labelling of [U-14C]oleate and the ketone bodies formed, and other cells that oxidize fat and do not form ketone bodies. 10. Inhibitory effects of unlabelled acetoacetate on the oxidation of [1-14C]butyrate and of unlabelled butyrate on [4-14C]acetoacetate oxidation show that fatty acids and ketone bodies compete as fuels on the basis of their relative concentrations. 11. The pathway of ketogenesis in renal cortex must differ from that of the liver, as β-hydroxy-β-methylglutaryl-CoA synthetase is virtually absent from the kidney. In contrast with the liver the kidney possesses 3-oxo acid CoA-transferase (EC 2.8.3.5), and the ready reversibility of this reaction and that of thiolase (EC 2.3.1.9) provide a mechanism for ketone-body formation from acetyl-CoA. This mechanism may apply to extrahepatic tissues generally, with the possible exception of the epithelium of the rumen and intestines.
Full text
PDF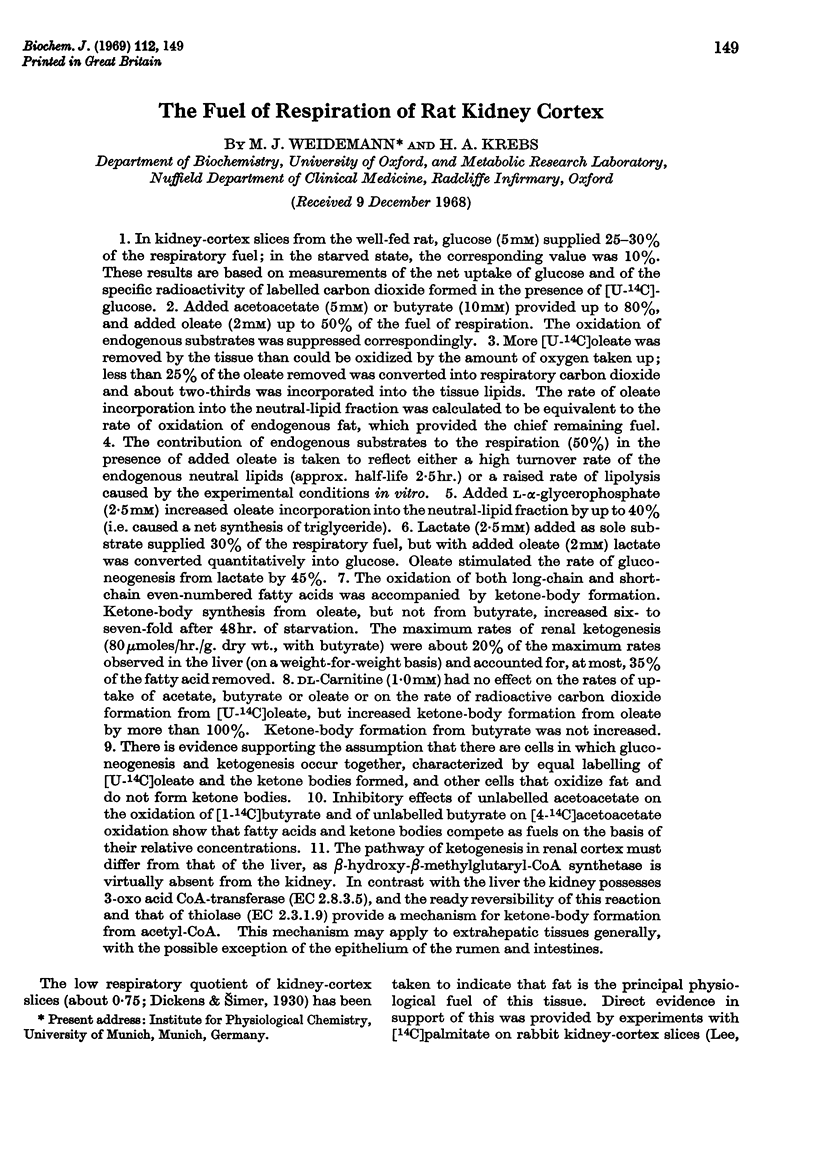
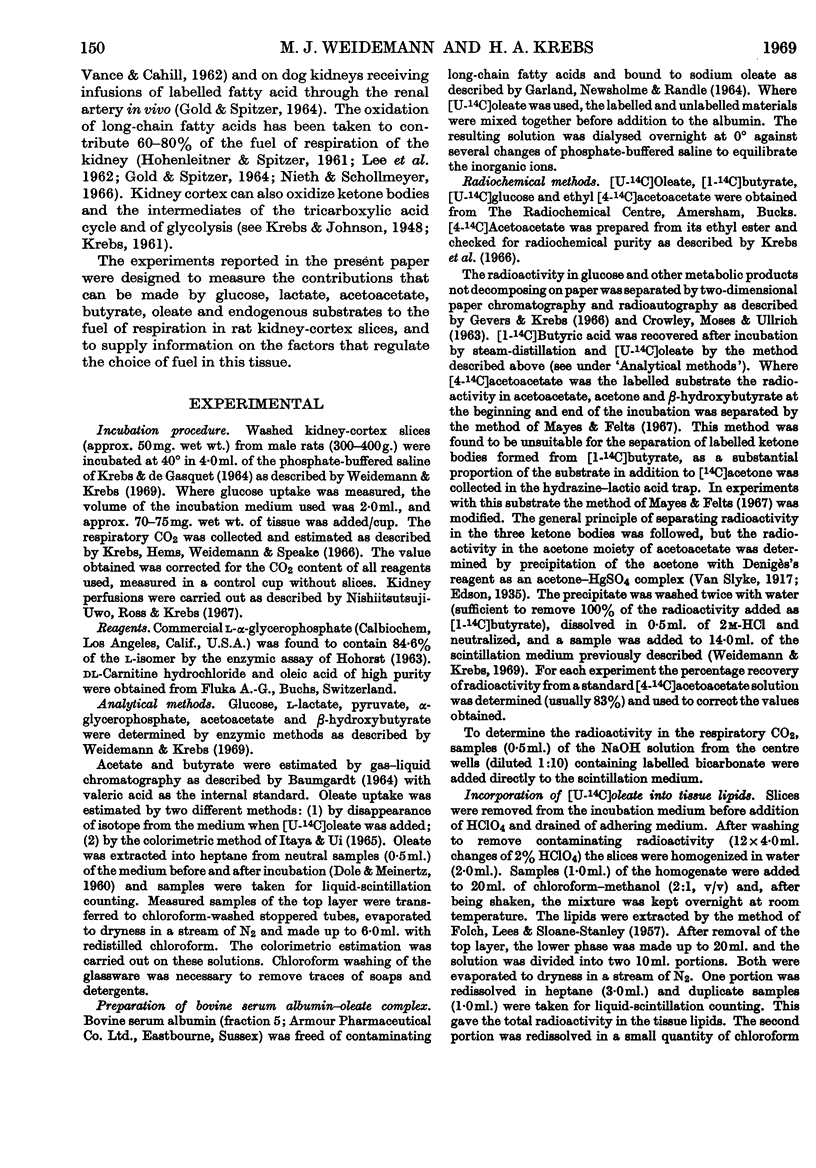
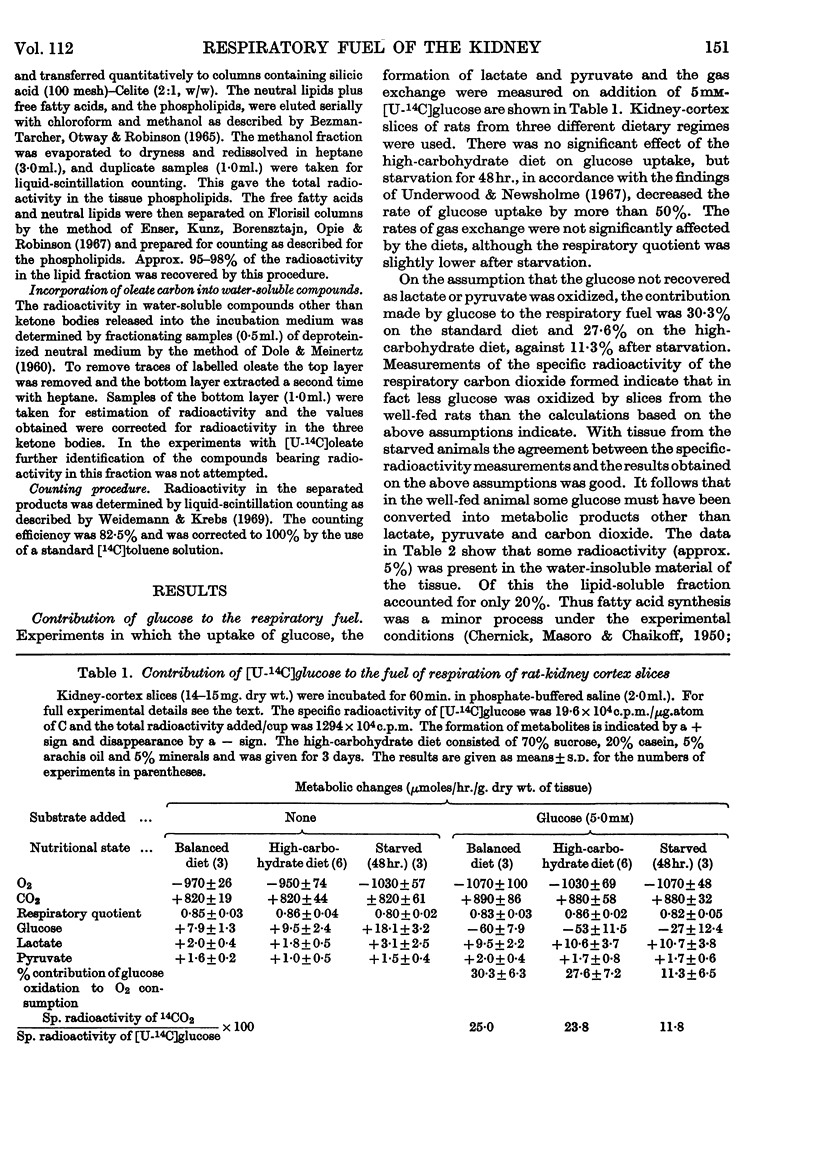
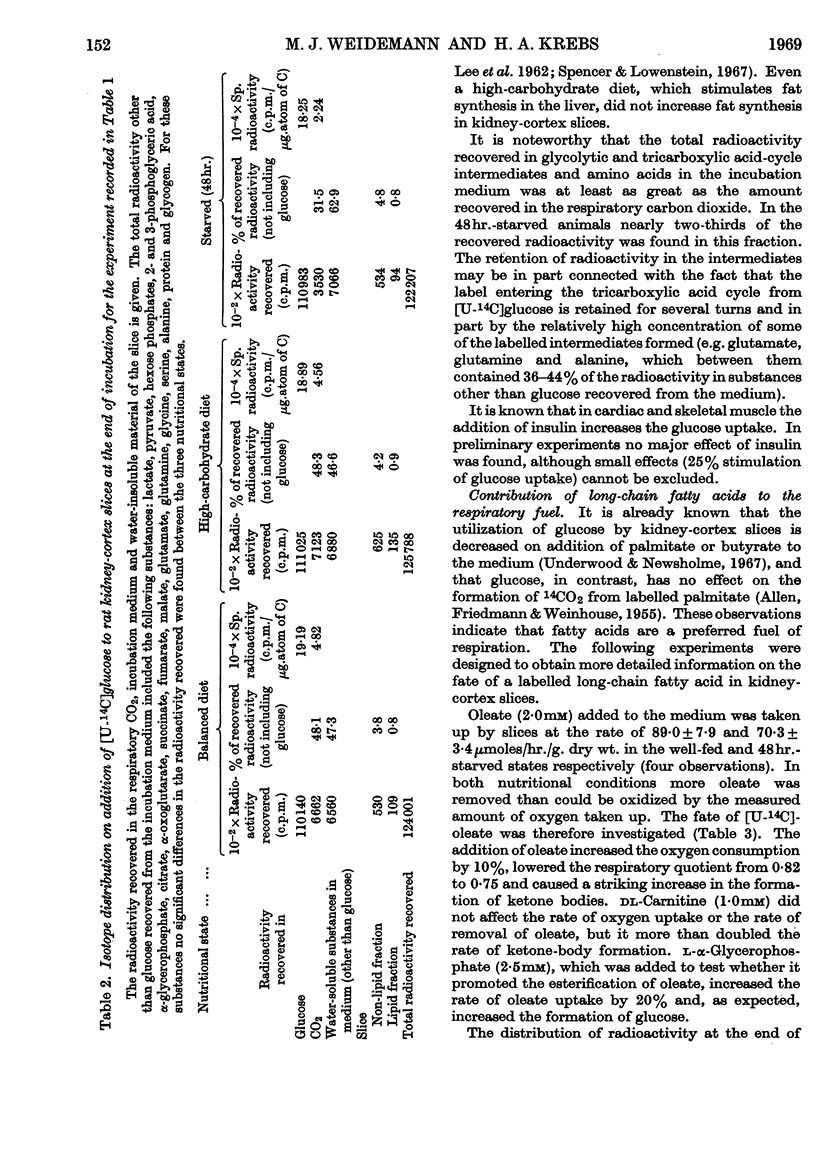
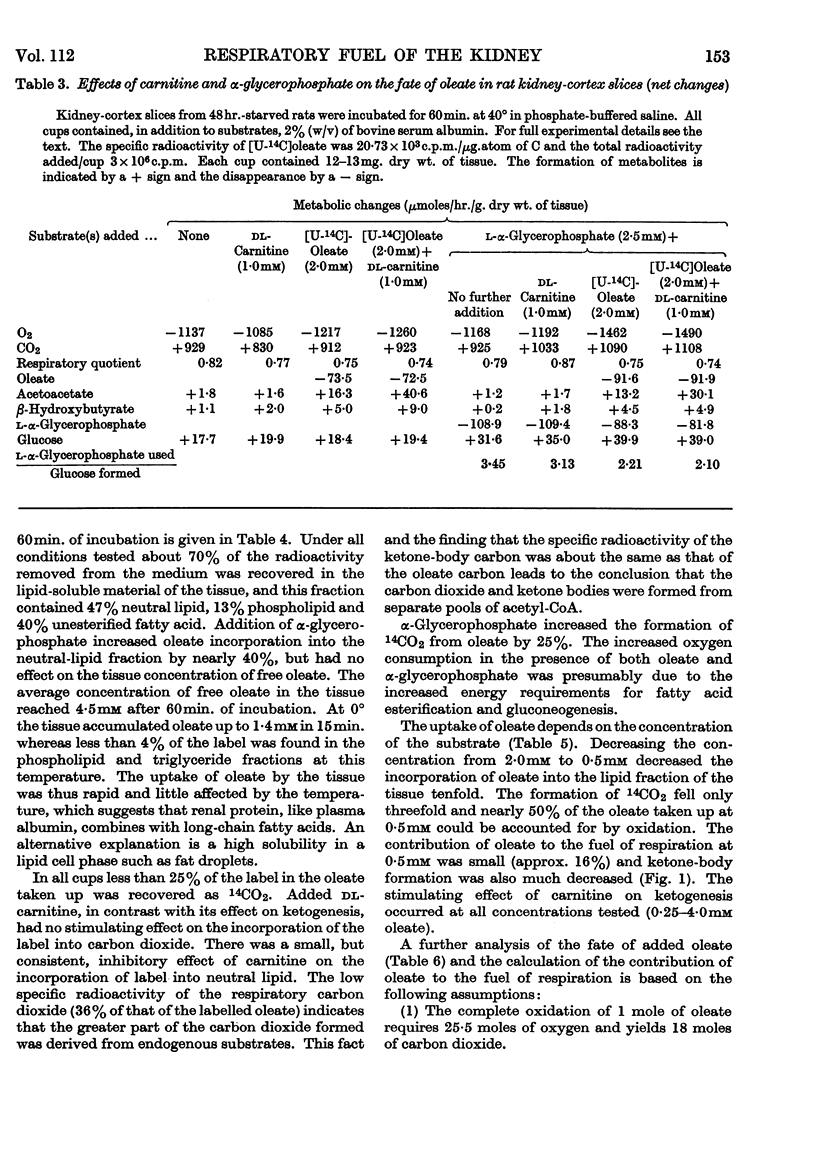
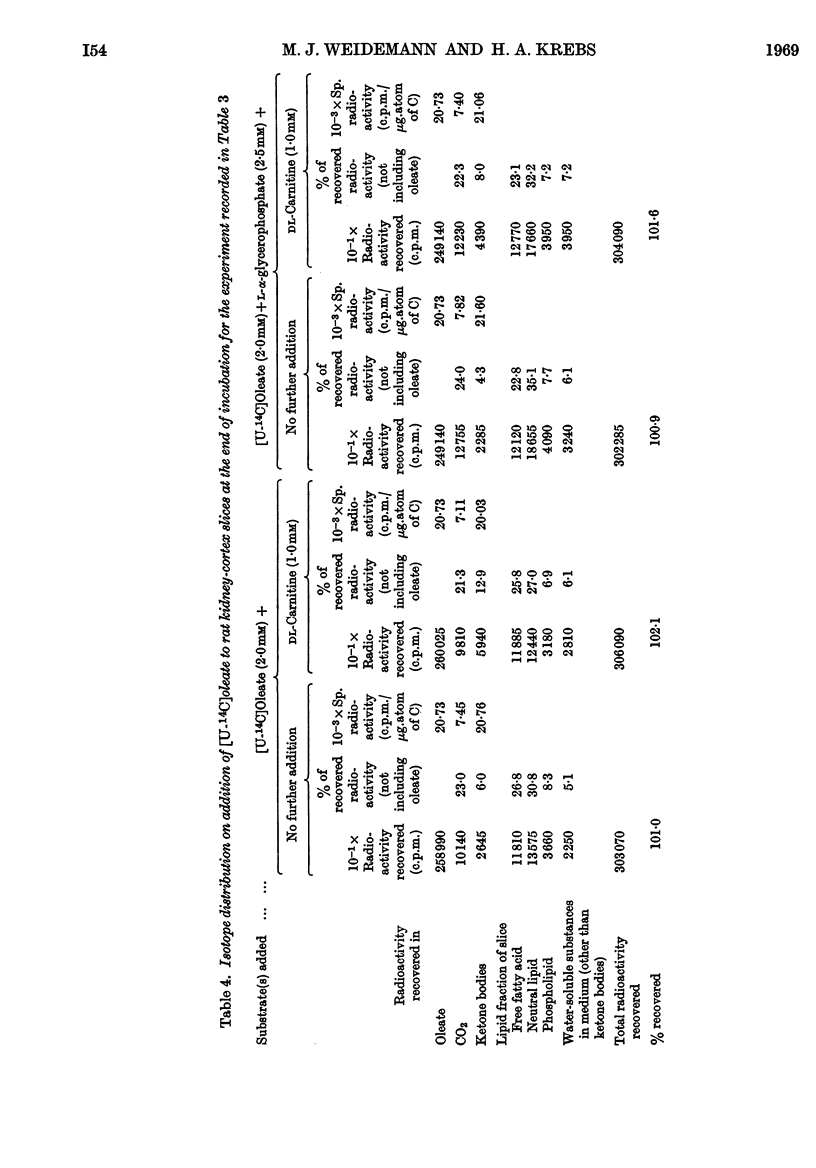
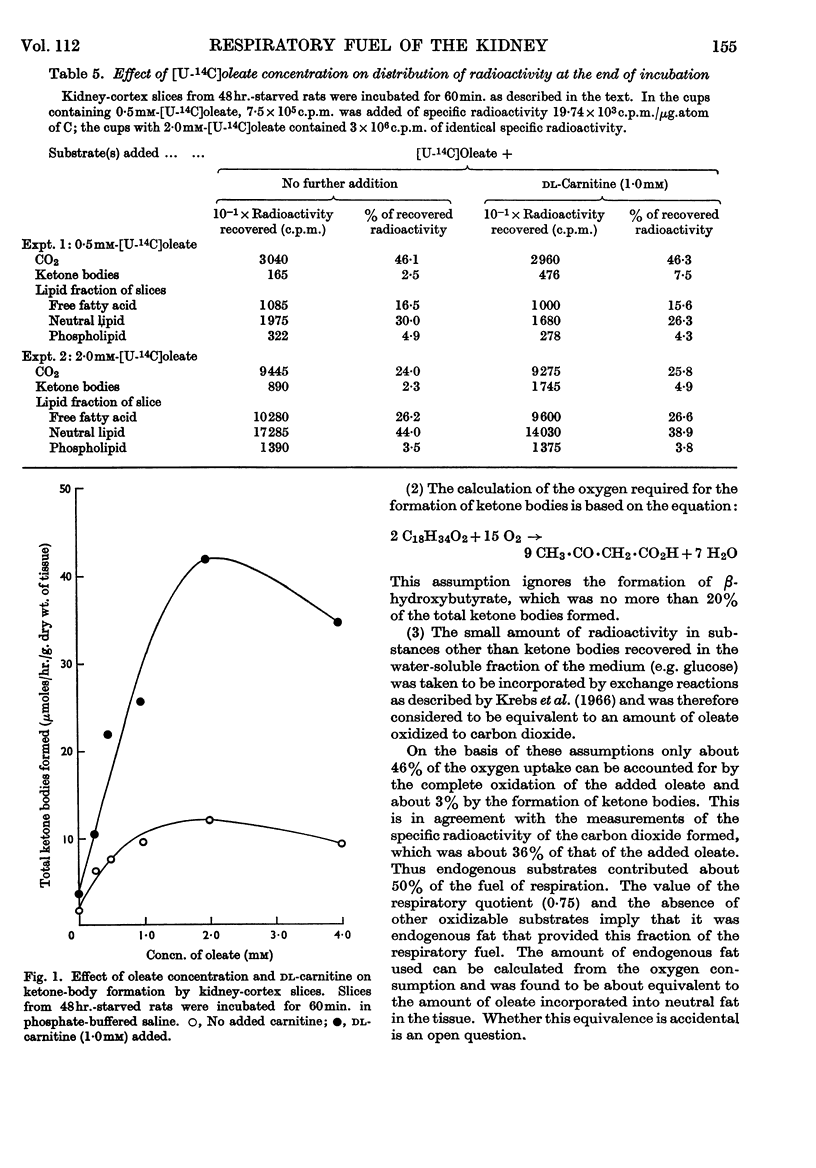
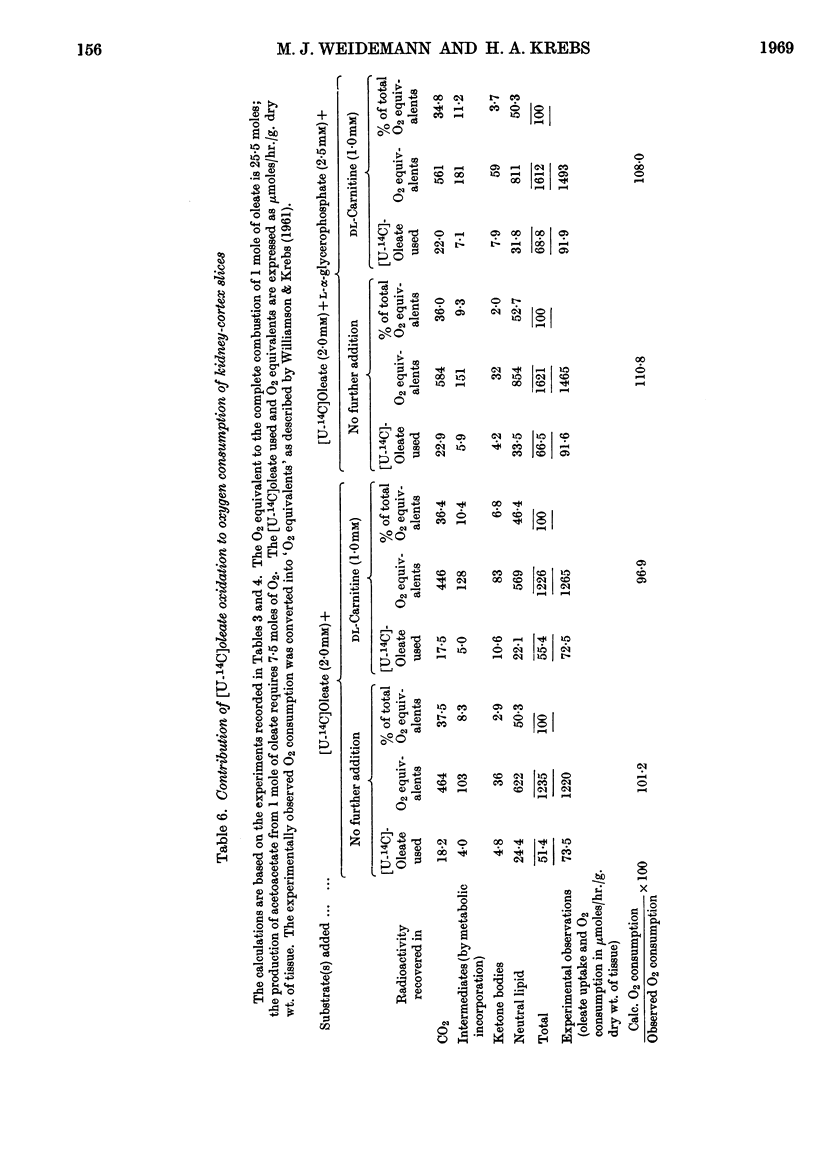
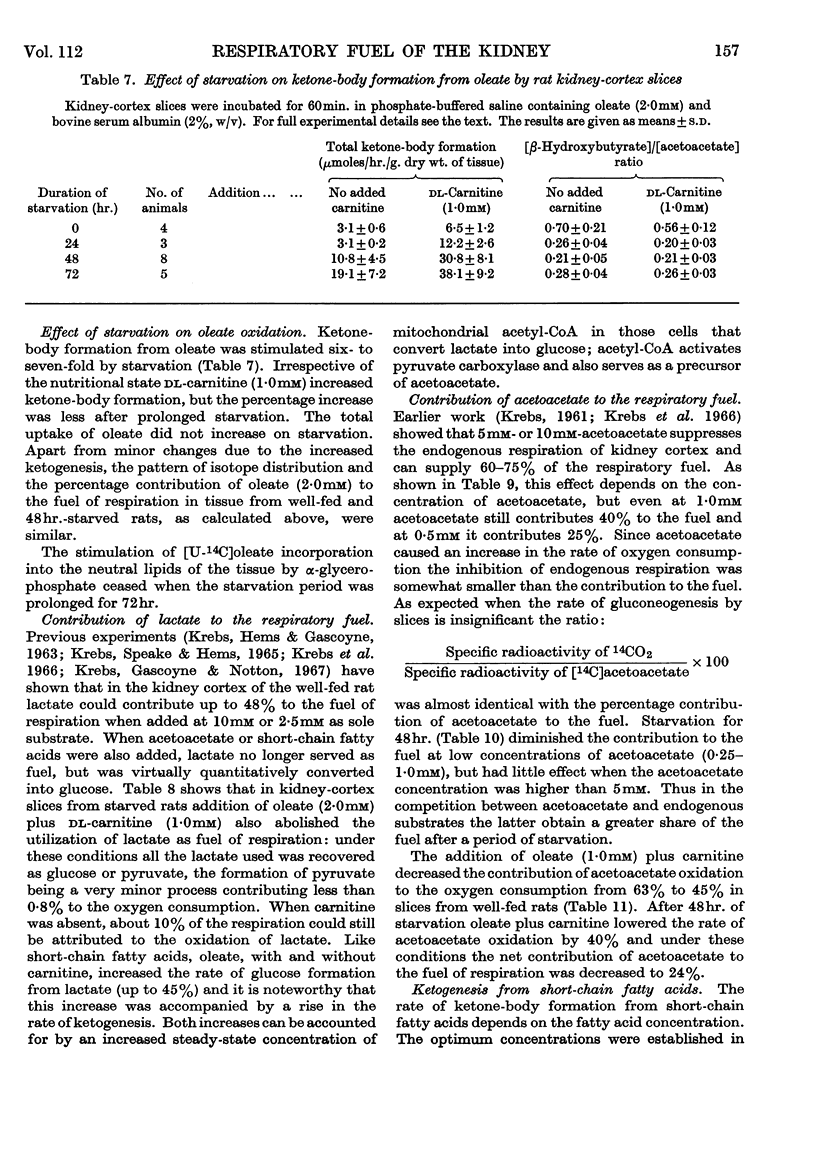
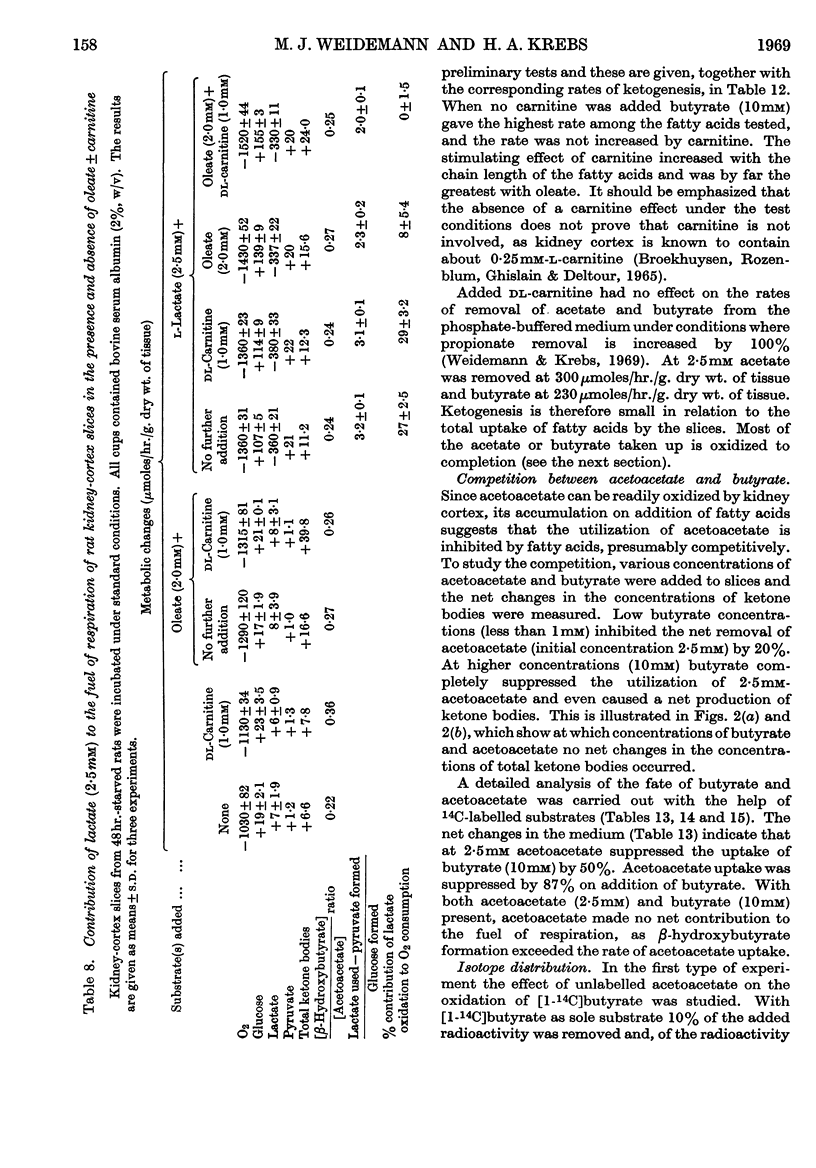
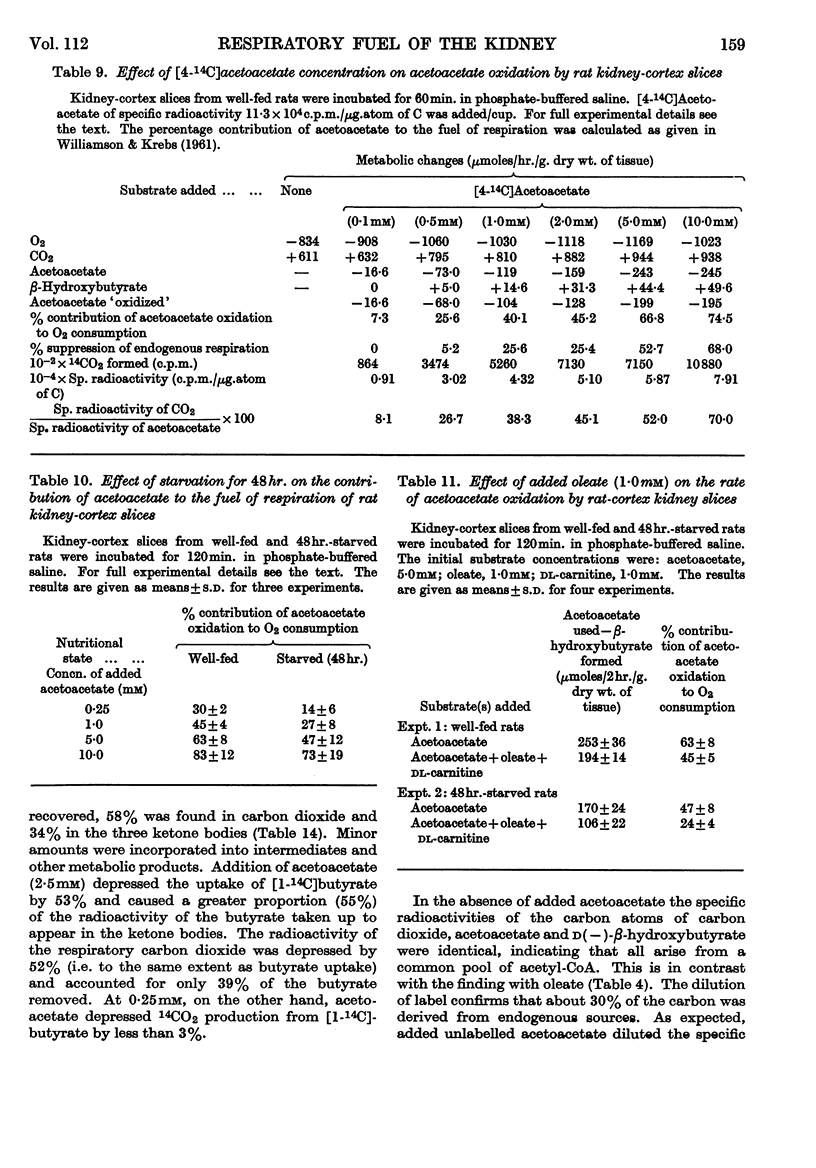
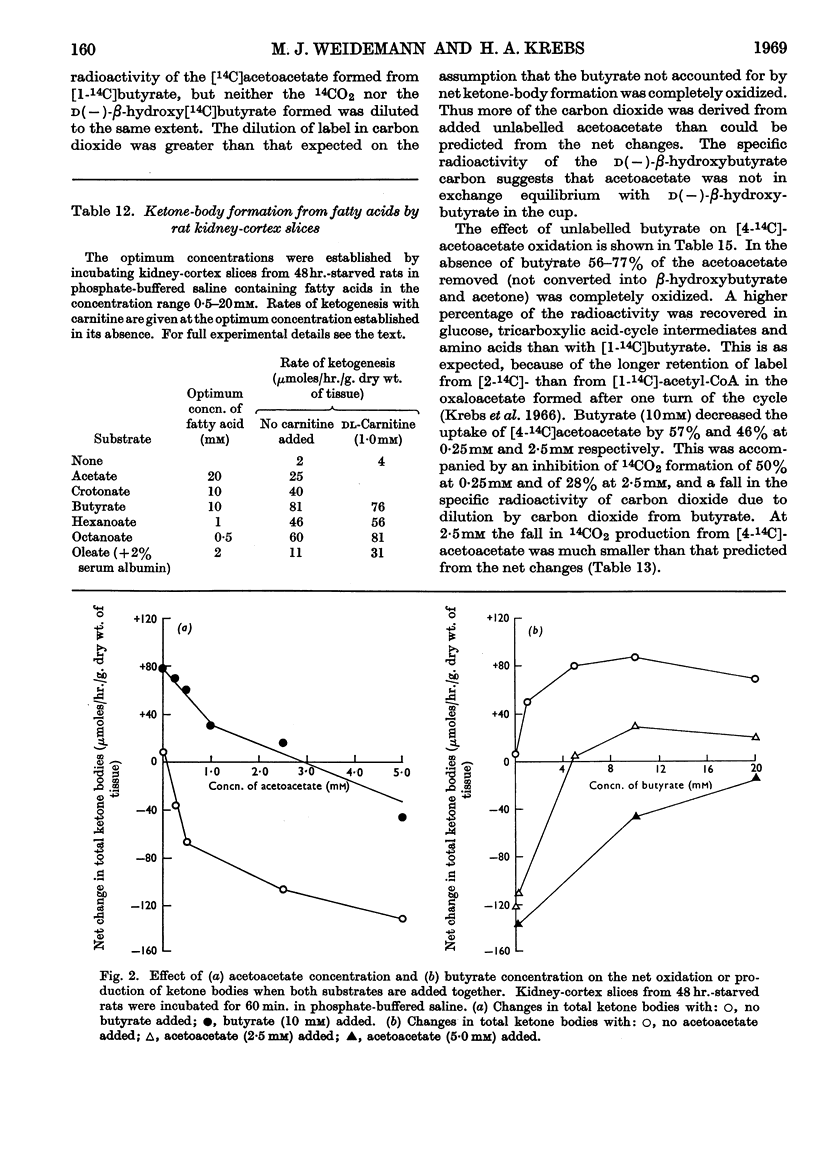
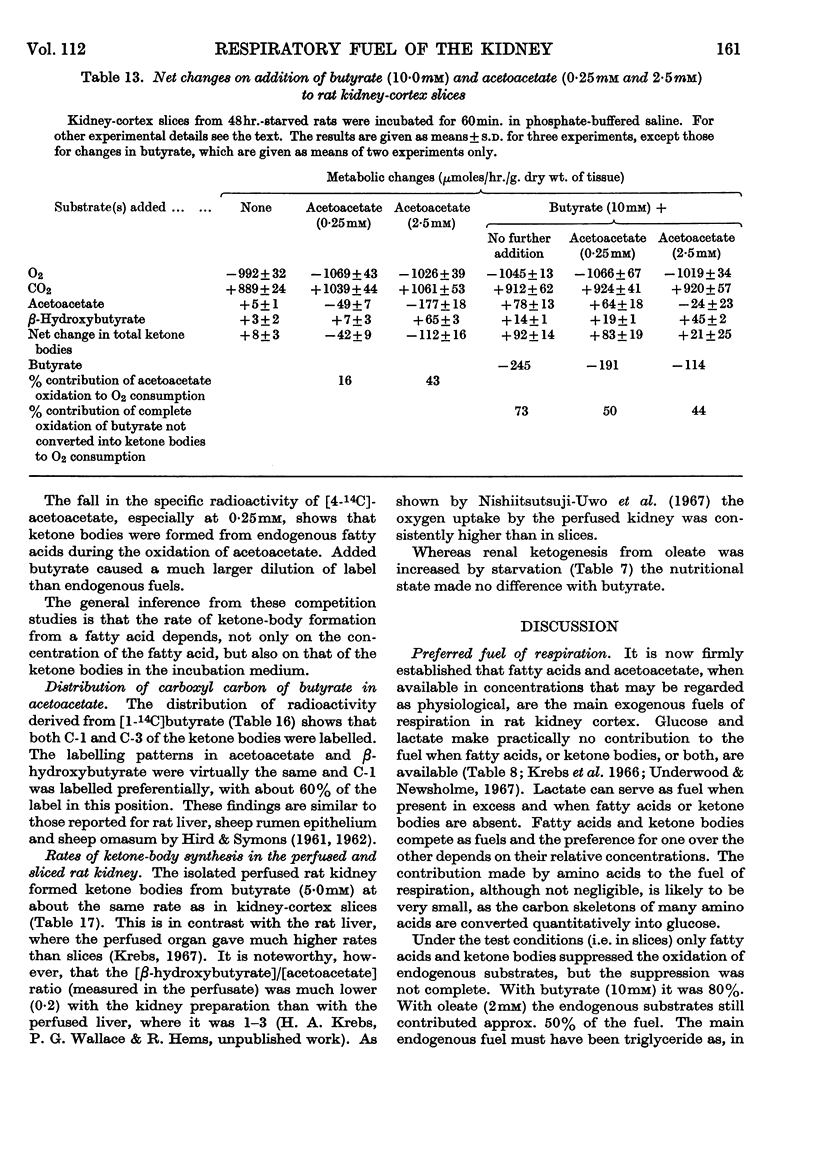
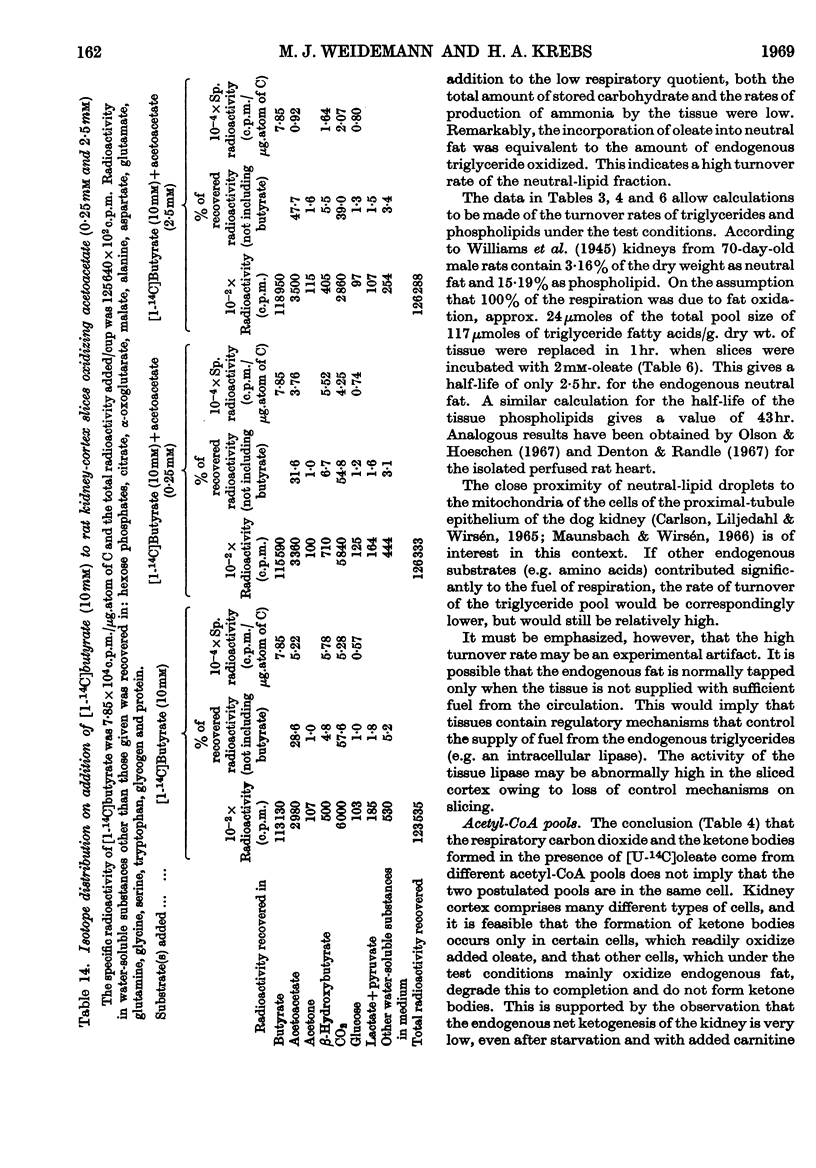
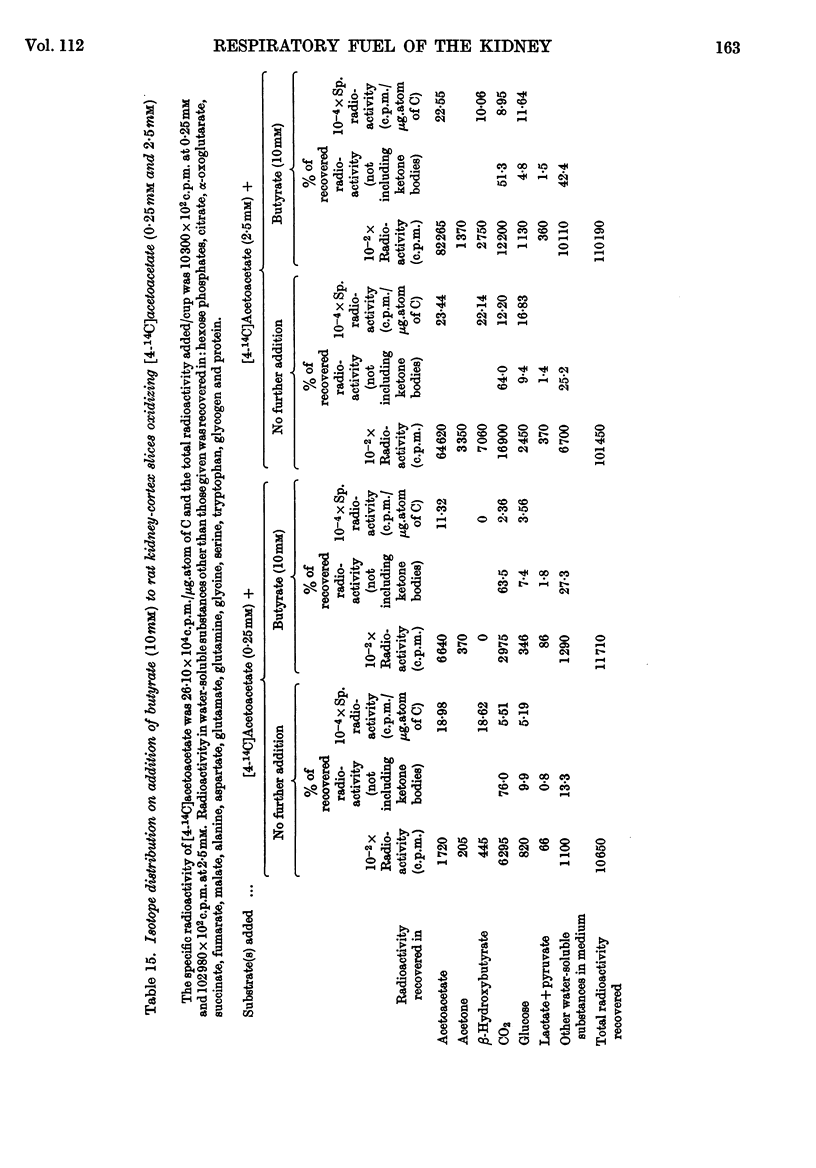
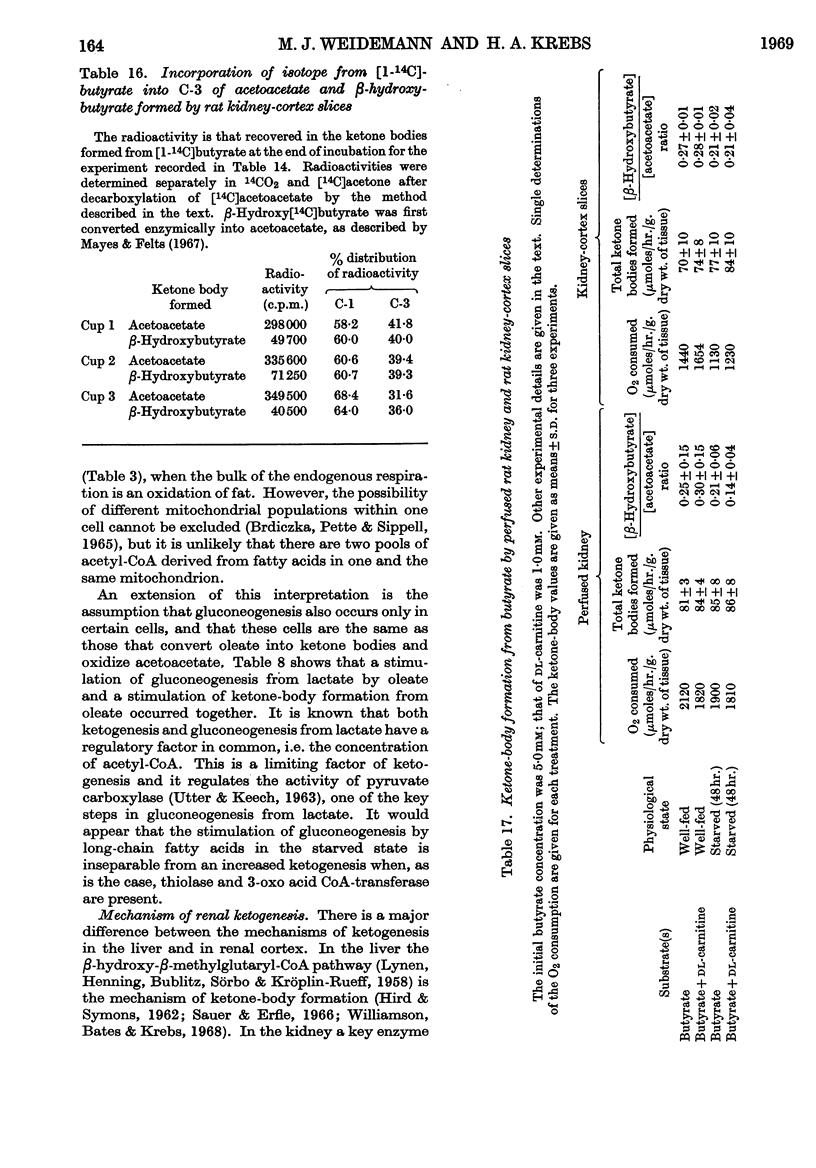
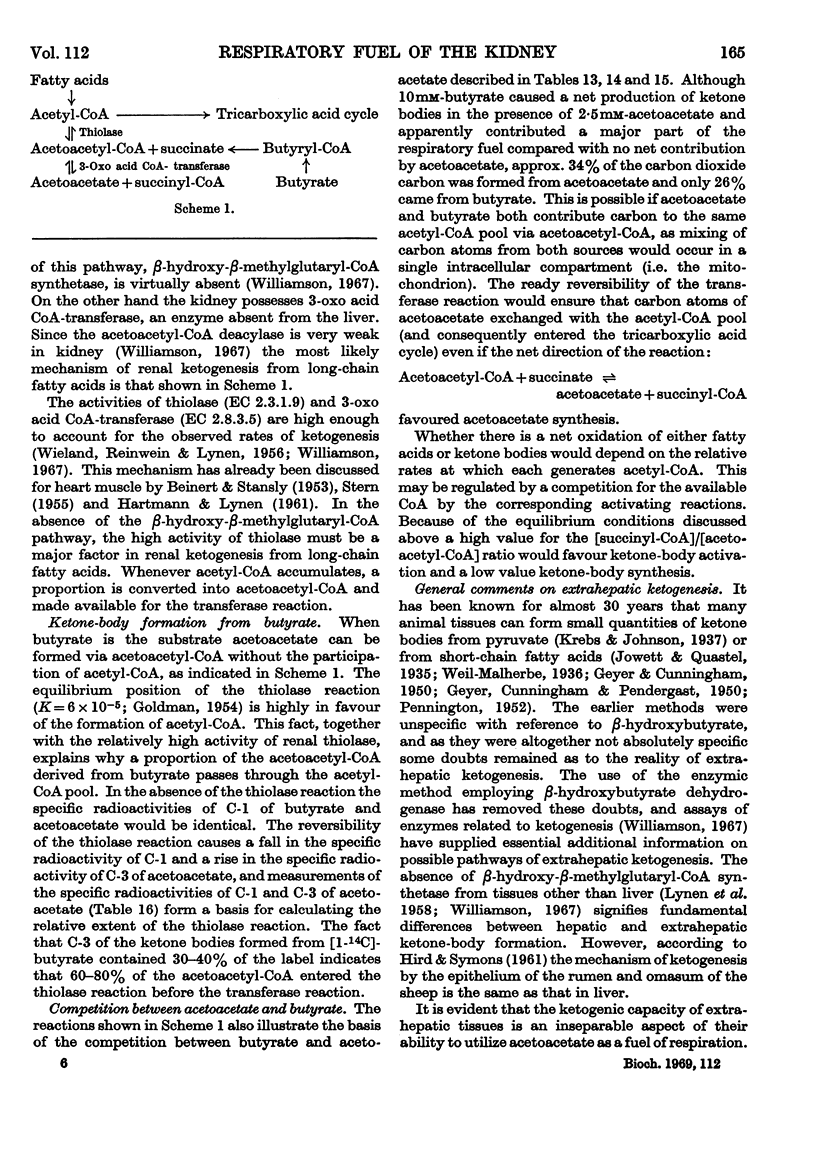
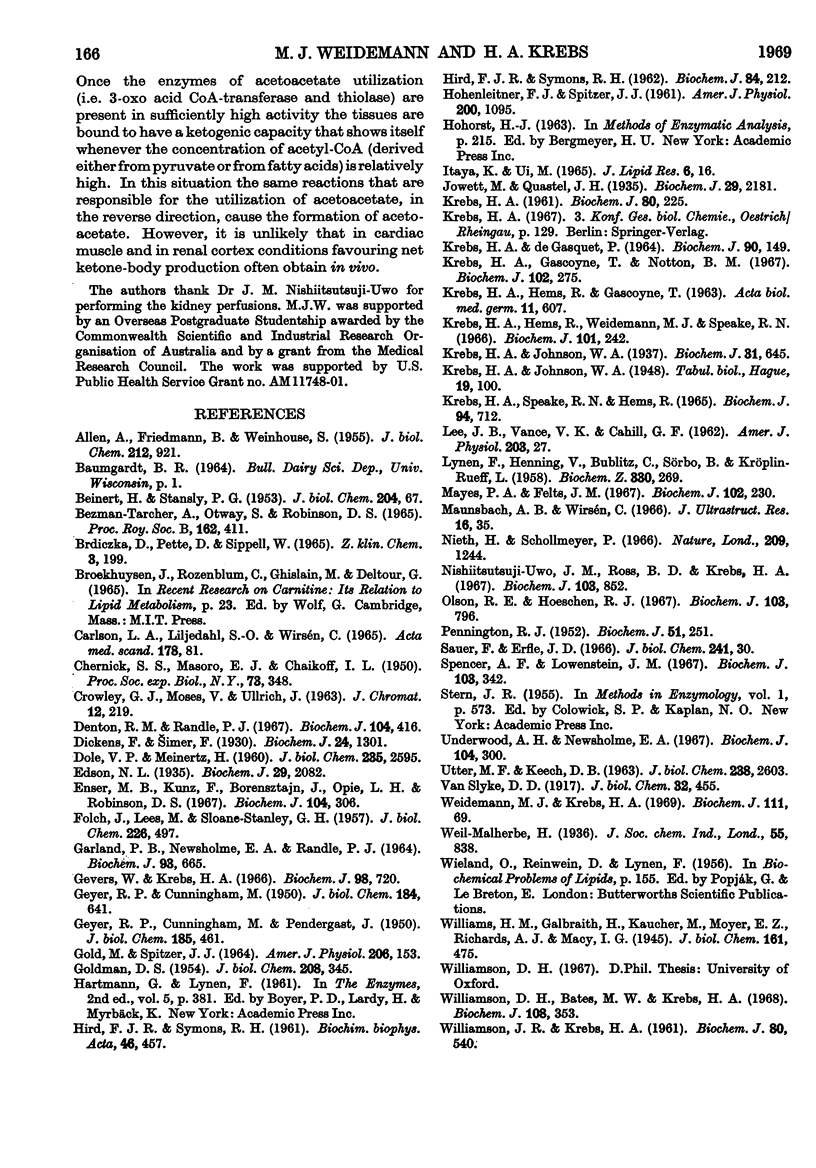
Selected References
These references are in PubMed. This may not be the complete list of references from this article.
- ALLEN A., FRIEDMANN B., WEINHOUSE S. Tissue preferences for fatty acid and glucose oxidation. J Biol Chem. 1955 Feb;212(2):921–933. [PubMed] [Google Scholar]
- BEINERT H., STANSLY P. G. Asymmetric labeling of acetoacetate by enzymatic acetyl exchange with acetyl coenzyme A. J Biol Chem. 1953 Sep;204(1):67–76. [PubMed] [Google Scholar]
- CROWLEY G. J., MOSES V., ULLRICH J. A VERSATILE SOLVENT TO REPLACE PHENOL FOR THE PAPER CHROMATOGRAPHY OF RADIOACTIVE INTERMEDIARY METABOLITES. J Chromatogr. 1963 Oct;12:219–228. doi: 10.1016/s0021-9673(01)83673-6. [DOI] [PubMed] [Google Scholar]
- DOLE V. P., MEINERTZ H. Microdetermination of long-chain fatty acids in plasma and tissues. J Biol Chem. 1960 Sep;235:2595–2599. [PubMed] [Google Scholar]
- Denton R. M., Randle P. J. Concentrations of glycerides and phospholipids in rat heart and gastrocnemius muscles. Effects of alloxan-diabetes and perfusion. Biochem J. 1967 Aug;104(2):416–422. doi: 10.1042/bj1040416. [DOI] [PMC free article] [PubMed] [Google Scholar]
- Dickens F., Simer F. The metabolism of normal and tumour tissue: The respiratory quotient, and the relationship of respiration to glycolysis. Biochem J. 1930;24(5):1301–1326. doi: 10.1042/bj0241301. [DOI] [PMC free article] [PubMed] [Google Scholar]
- Edson N. L. Ketogenesis-antiketogenesis: The influence of ammonium chloride on ketone-body formation in liver. Biochem J. 1935 Sep;29(9):2082–2094. doi: 10.1042/bj0292082. [DOI] [PMC free article] [PubMed] [Google Scholar]
- Enser M. B., Kunz F., Borensztajn J., Opie L. H., Robinson D. S. Metabolism of triglyceride fatty acid by the perfused rat heart. Biochem J. 1967 Jul;104(1):306–317. doi: 10.1042/bj1040306. [DOI] [PMC free article] [PubMed] [Google Scholar]
- FOLCH J., LEES M., SLOANE STANLEY G. H. A simple method for the isolation and purification of total lipides from animal tissues. J Biol Chem. 1957 May;226(1):497–509. [PubMed] [Google Scholar]
- GEYER R. P., CUNNINGHAM M. Metabolism of fatty acids in vitro, studied with odd and even members of the RC14OOH series. J Biol Chem. 1950 Jun;184(2):641–646. [PubMed] [Google Scholar]
- GEYER R. P., CUNNINGHAM M., PENDERGAST J. Acetoacetic acid formation in vitro from odd and even numbered radioactive fatty acids. J Biol Chem. 1950 Aug;185(2):461–468. [PubMed] [Google Scholar]
- GOLD M., SPITZER J. J. METABOLISM OF FREE FATTY ACIDS BY MYOCARDIUM AND KIDNEY. Am J Physiol. 1964 Jan;206:153–158. doi: 10.1152/ajplegacy.1964.206.1.153. [DOI] [PubMed] [Google Scholar]
- GOLDMAN D. S. Studies on the fatty acid oxidizing system of animal tissues. VII. The beta-ketoacyl coenzyme A cleavage enzyme. J Biol Chem. 1954 May;208(1):345–357. [PubMed] [Google Scholar]
- Gevers W., Krebs H. A. The effects of adenine nucleotides on carbohydrate metabolism in pigeon-liver homogenates. Biochem J. 1966 Mar;98(3):720–735. doi: 10.1042/bj0980720. [DOI] [PMC free article] [PubMed] [Google Scholar]
- HIRD F. J., SYMONS R. H. The mechanism of ketone-body formation from butyrate in rat liver. Biochem J. 1962 Jul;84:212–216. doi: 10.1042/bj0840212. [DOI] [PMC free article] [PubMed] [Google Scholar]
- HIRD F. J., SYMONS R. H. The mode of formation of ketone bodies from butyrate by tissue from the rumen and omasum of the sheep. Biochim Biophys Acta. 1961 Jan 29;46:457–467. doi: 10.1016/0006-3002(61)90577-7. [DOI] [PubMed] [Google Scholar]
- HOHENLEITNER F. J., SPITZER J. J. Changes in plasma free fatty acid concentrations on passage through the dog kidney. Am J Physiol. 1961 May;200:1095–1098. doi: 10.1152/ajplegacy.1961.200.5.1095. [DOI] [PubMed] [Google Scholar]
- ITAYA K., UI M. COLORIMETRIC DETERMINATION OF FREE FATTY ACIDS IN BIOLOGICAL FLUIDS. J Lipid Res. 1965 Jan;6:16–20. [PubMed] [Google Scholar]
- Jowett M., Quastel J. H. Studies in fat metabolism: The formation and breakdown of acetoacetic acid in animal tissues. Biochem J. 1935 Sep;29(9):2181–2191. doi: 10.1042/bj0292181. [DOI] [PMC free article] [PubMed] [Google Scholar]
- KREBS H. A., HEMS R., GASCOYNE T. RENAL GLUCONEOGENESIS. IV. GLUCONEOGENESIS FROM SUBSTRATE COMBINATIONS. Acta Biol Med Ger. 1963;11:607–615. [PubMed] [Google Scholar]
- KREBS H. A., SPEAKE R. N., HEMS R. ACCELERATION OF RENAL GLUCONEOGENESIS BY KETONE BODIES AND FATTY ACIDS. Biochem J. 1965 Mar;94:712–720. doi: 10.1042/bj0940712. [DOI] [PMC free article] [PubMed] [Google Scholar]
- KREBS H. A. The physiological role of the ketone bodies. Biochem J. 1961 Aug;80:225–233. doi: 10.1042/bj0800225. [DOI] [PMC free article] [PubMed] [Google Scholar]
- Krebs H. A., De Gasquet P. Inhibition of gluconeogenesis by alpha-oxo acids. Biochem J. 1964 Jan;90(1):149–154. doi: 10.1042/bj0900149. [DOI] [PMC free article] [PubMed] [Google Scholar]
- Krebs H. A., Gascoyne T., Notton B. M. Generation of extramitochondrial reducing power in gluconeogenesis. Biochem J. 1967 Jan;102(1):275–282. doi: 10.1042/bj1020275. [DOI] [PMC free article] [PubMed] [Google Scholar]
- Krebs H. A., Hems R., Weidemann M. J., Speake R. N. The fate of isotopic carbon in kidney cortex synthesizing glucose from lactate. Biochem J. 1966 Oct;101(1):242–249. doi: 10.1042/bj1010242. [DOI] [PMC free article] [PubMed] [Google Scholar]
- Krebs H. A., Johnson W. A. Metabolism of ketonic acids in animal tissues. Biochem J. 1937 Apr;31(4):645–660. doi: 10.1042/bj0310645. [DOI] [PMC free article] [PubMed] [Google Scholar]
- LEE J. B., VANCE V. K., CAHILL G. F., Jr Metabolism of C14-labeled substrates by rabbit kidney cortex and medulla. Am J Physiol. 1962 Jul;203:27–36. doi: 10.1152/ajplegacy.1962.203.1.27. [DOI] [PubMed] [Google Scholar]
- LYNEN F., HENNING U., BUBLITZ C., SORBO B., KROPLIN-RUEFF L. Der chemische Mechanismus der Acetessigsäurebildung in der Leber. Biochem Z. 1958;330(4):269–295. [PubMed] [Google Scholar]
- Mayes P. A., Felts J. M. Determination of 14C-labelled ketone bodies by liquid-scintillation counting. Biochem J. 1967 Jan;102(1):230–235. doi: 10.1042/bj1020230. [DOI] [PMC free article] [PubMed] [Google Scholar]
- Nieth H., Schollmeyer P. Substrate-utilization of the human kidney. Nature. 1966 Mar 19;209(5029):1244–1245. doi: 10.1038/2091244a0. [DOI] [PubMed] [Google Scholar]
- Nishiitsutsuji-Uwo J. M., Ross B. D., Krebs H. A. Metabolic activities of the isolated perfused rat kidney. Biochem J. 1967 Jun;103(3):852–862. doi: 10.1042/bj1030852. [DOI] [PMC free article] [PubMed] [Google Scholar]
- Olson R. E., Hoeschen R. J. Utilization of endogenous lipid by the isolated perfused rat heart. Biochem J. 1967 Jun;103(3):796–801. doi: 10.1042/bj1030796. [DOI] [PMC free article] [PubMed] [Google Scholar]
- PENNINGTON R. J. The metabolism of short-chain fatty acids in the sheep. I. Fatty acid utilization and ketone body production by rumen epithelium and other tissues. Biochem J. 1952 May;51(2):251–258. doi: 10.1042/bj0510251. [DOI] [PMC free article] [PubMed] [Google Scholar]
- Randle P. J., Newsholme E. A., Garland P. B. Regulation of glucose uptake by muscle. 8. Effects of fatty acids, ketone bodies and pyruvate, and of alloxan-diabetes and starvation, on the uptake and metabolic fate of glucose in rat heart and diaphragm muscles. Biochem J. 1964 Dec;93(3):652–665. doi: 10.1042/bj0930652. [DOI] [PMC free article] [PubMed] [Google Scholar]
- Sauer F., Erfle J. D. On the mechanism of acetoacetate synthesis by guinea pig liver fractions. J Biol Chem. 1966 Jan 10;241(1):30–37. [PubMed] [Google Scholar]
- Spencer A. F., Lowenstein J. M. Citrate content of liver and kidney of rat in various metabolic states and in fluoroacetate poisoning. Biochem J. 1967 May;103(2):342–348. doi: 10.1042/bj1030342. [DOI] [PMC free article] [PubMed] [Google Scholar]
- UTTER M. F., KEECH D. B. PYRUVATE CARBOXYLASE. I. NATURE OF THE REACTION. J Biol Chem. 1963 Aug;238:2603–2608. [PubMed] [Google Scholar]
- Underwood A. H., Newsholme E. A. Control of glycolysis and gluconeogenesis in rat kidney cortex slices. Biochem J. 1967 Jul;104(1):300–305. doi: 10.1042/bj1040300. [DOI] [PMC free article] [PubMed] [Google Scholar]
- WILLIAMSON J. R., KREBS H. A. Acetoacetate as fuel of respiration in the perfused rat heart. Biochem J. 1961 Sep;80:540–547. doi: 10.1042/bj0800540. [DOI] [PMC free article] [PubMed] [Google Scholar]
- Weidemann M. J., Krebs H. A. Acceleration of gluconeogenesis from propionate by Dl-carnitine in the rat kidney cortex. Biochem J. 1969 Jan;111(1):69–81. doi: 10.1042/bj1110069. [DOI] [PMC free article] [PubMed] [Google Scholar]
- Williamson D. H., Bates M. W., Krebs H. A. Activity and intracellular distribution of enzymes of ketone-body metabolism in rat liver. Biochem J. 1968 Jul;108(3):353–361. doi: 10.1042/bj1080353. [DOI] [PMC free article] [PubMed] [Google Scholar]