Abstract
1. A method of estimating absolute ionic permeability coefficients which does not depend on the use of impermeant substitutes is reported. 2. The method is based on a pump leak model of the Xenopus laevis oocyte membrane. The procedure consists of measuring, in the same experiment, the pump current and the currents generated under voltage clamp by the partial substitution of one or two ions at a time. For each experimental condition, the measured currents are substituted in a Goldman-Hodgkin-Katz type equation with two unknowns (the permeability coefficients). The set of equations thus generated enables the computation of all the ionic permeability coefficients. 3. The Xenopus oocyte membrane (stages IV and V, Dumont, 1972) has been found to be permeable to conventional ion substitutes such as N-methyl-D-glucamine (NMG), sulphate, isethionate and gluconate. 4. The values for sodium, potassium and chloride permeability coefficients obtained from sixty-eight pooled experiments were, respectively, 5.44, 17.41 and 1.49 x 10(-8) cm s-1. 5. The diffusional currents for sodium, potassium and chloride computed from the experiments referred to above were, respectively, -1.16, 0.69 and -0.038 microA cm-2. 6. A stoichiometry of the Na+-K+ pump exchange of 3/1.8 was computed. 7. The intracellular concentrations of sodium, potassium and chloride ions, as determined by ion-selective microelectrodes, were, respectively, 10.1 +/- 0.66 mM (n = 12), 109.5 +/- 3.3 mM (n = 13) and 37.7 +/- 1.18 mM (n = 19), corresponding to equilibrium potentials of 61, -95 and -28 mV. 8. Since chloride is not at equilibrium across the membrane, we propose that there is an inward uphill Cl- transport.
Full text
PDF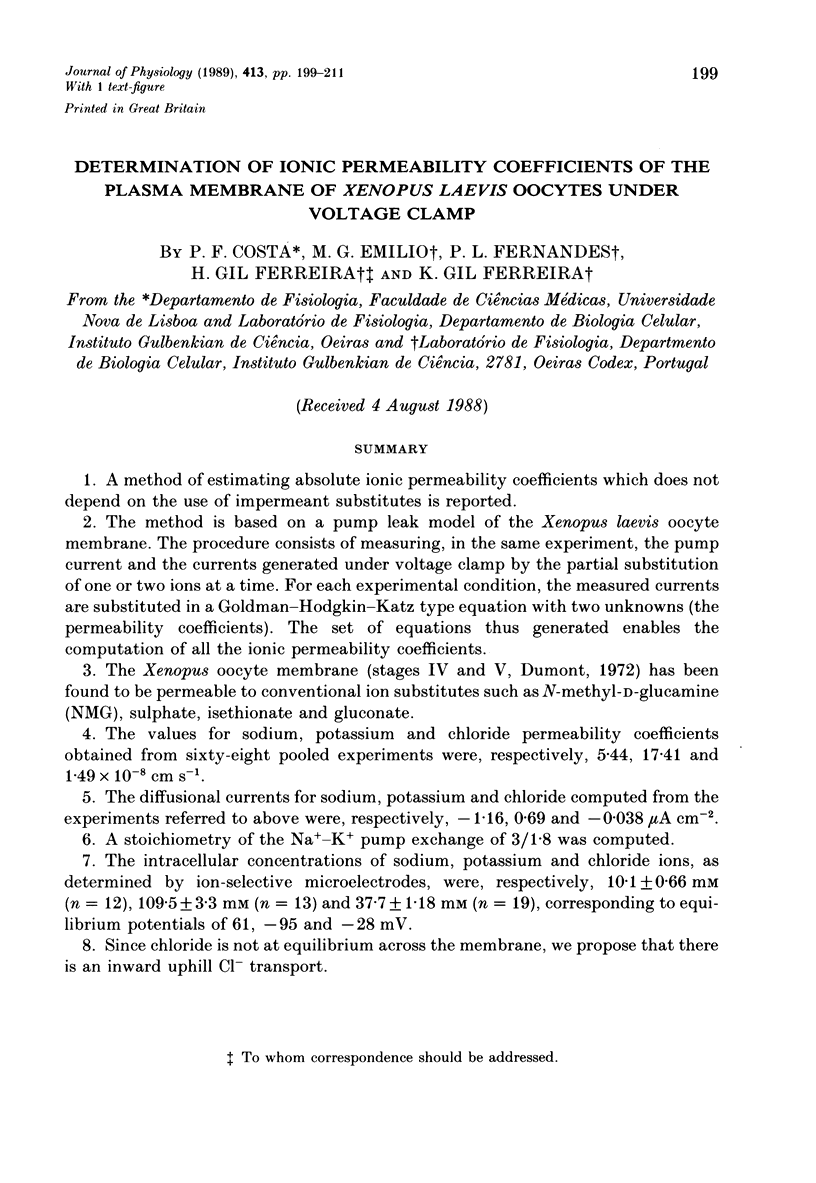
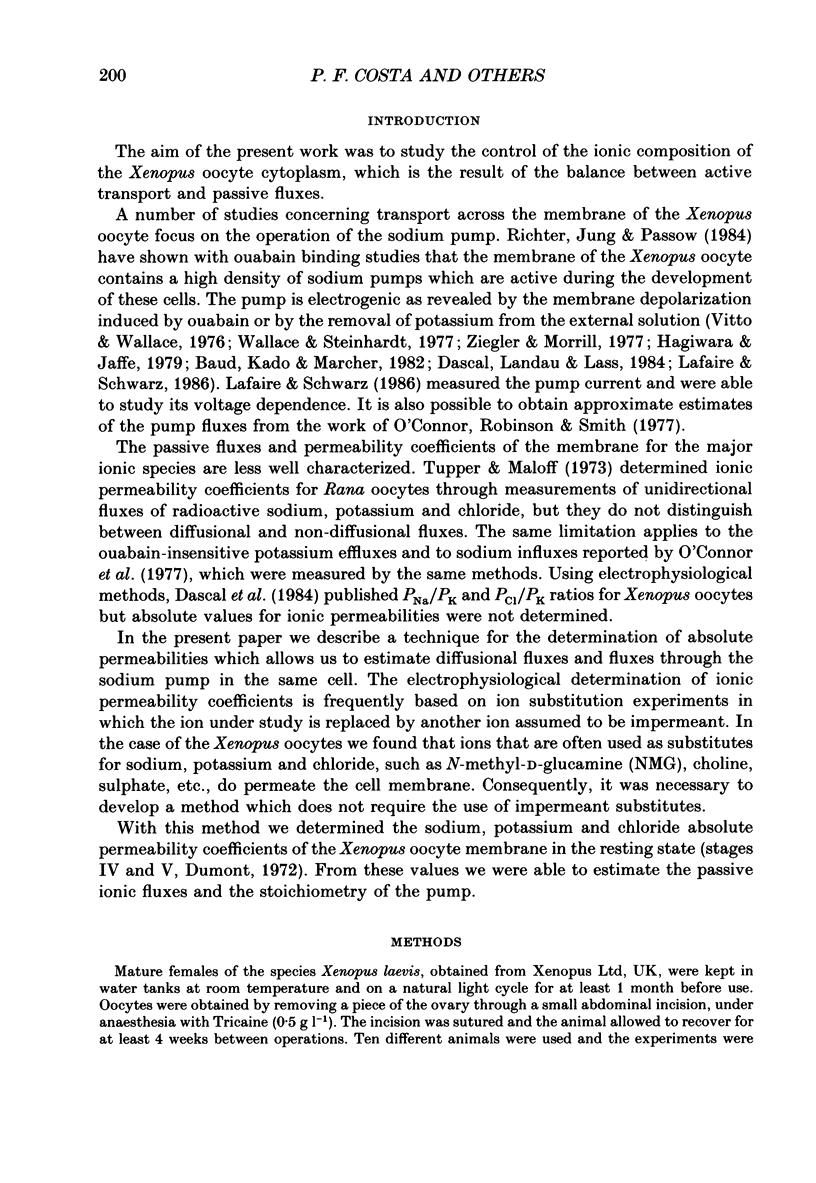
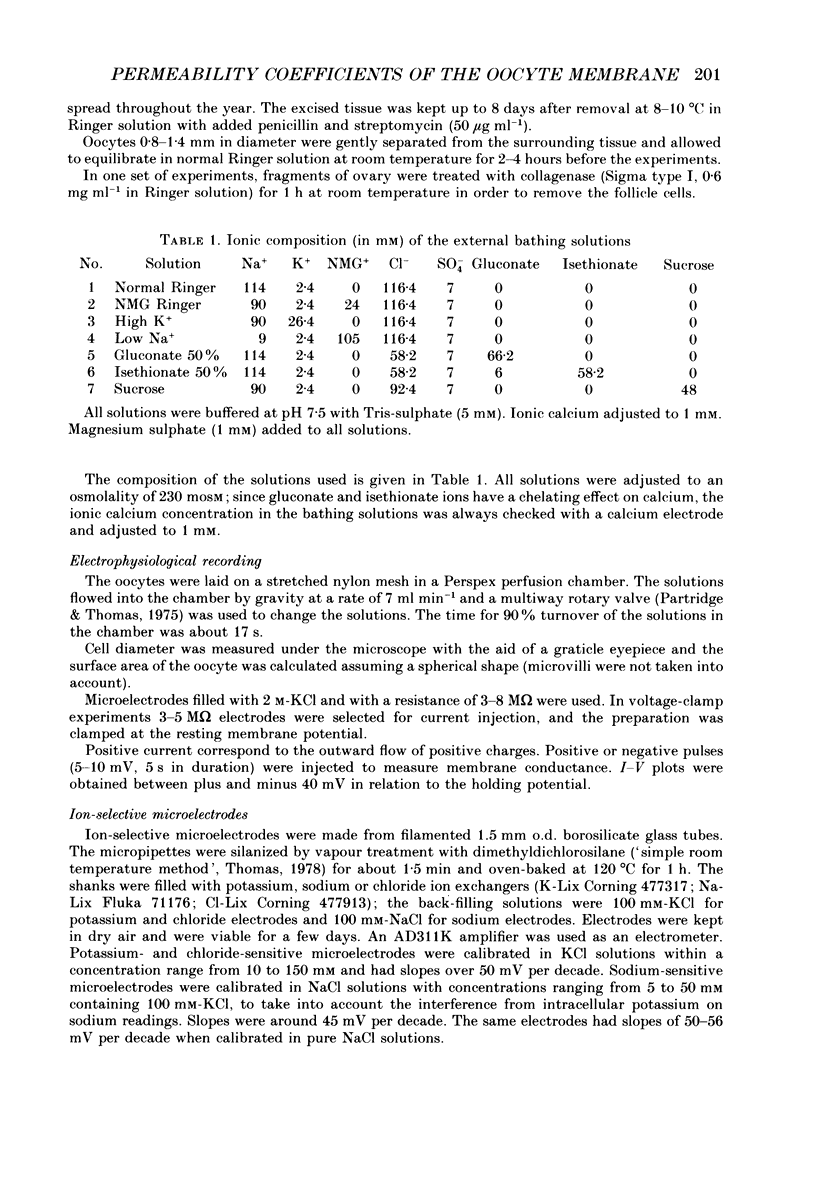
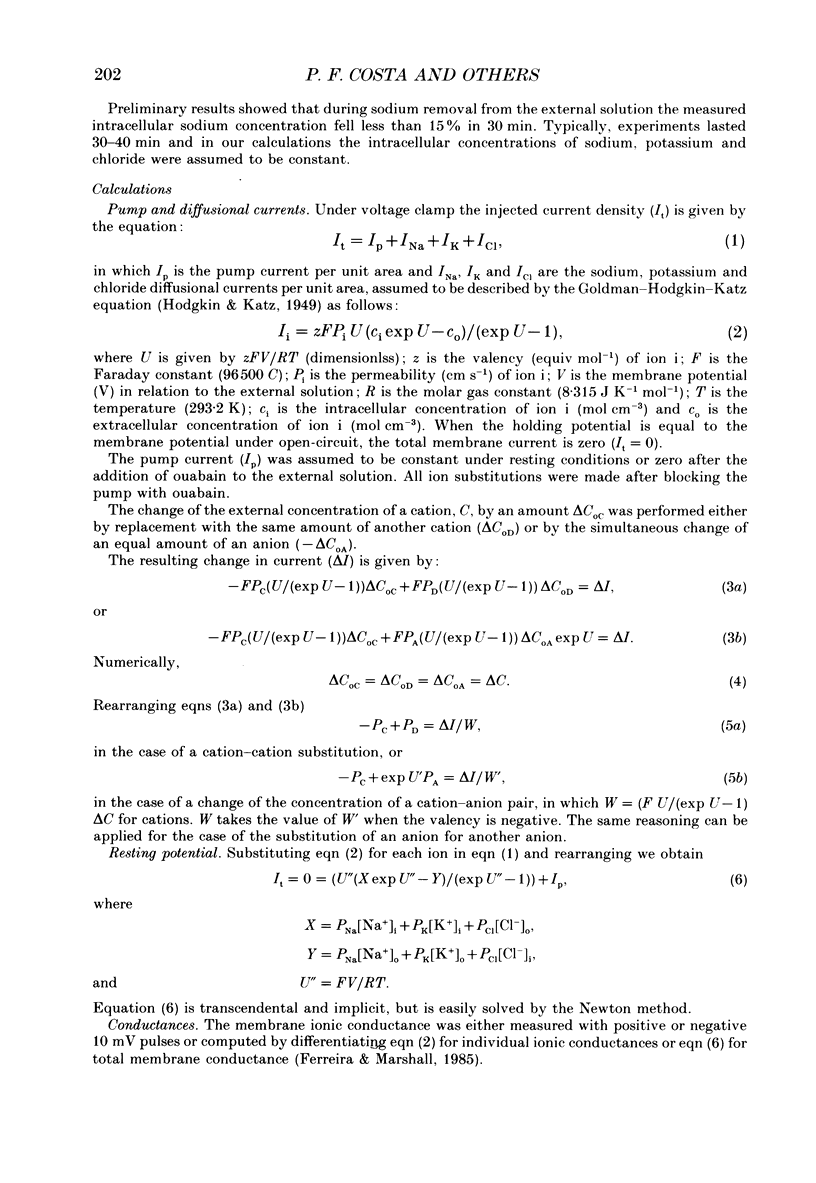
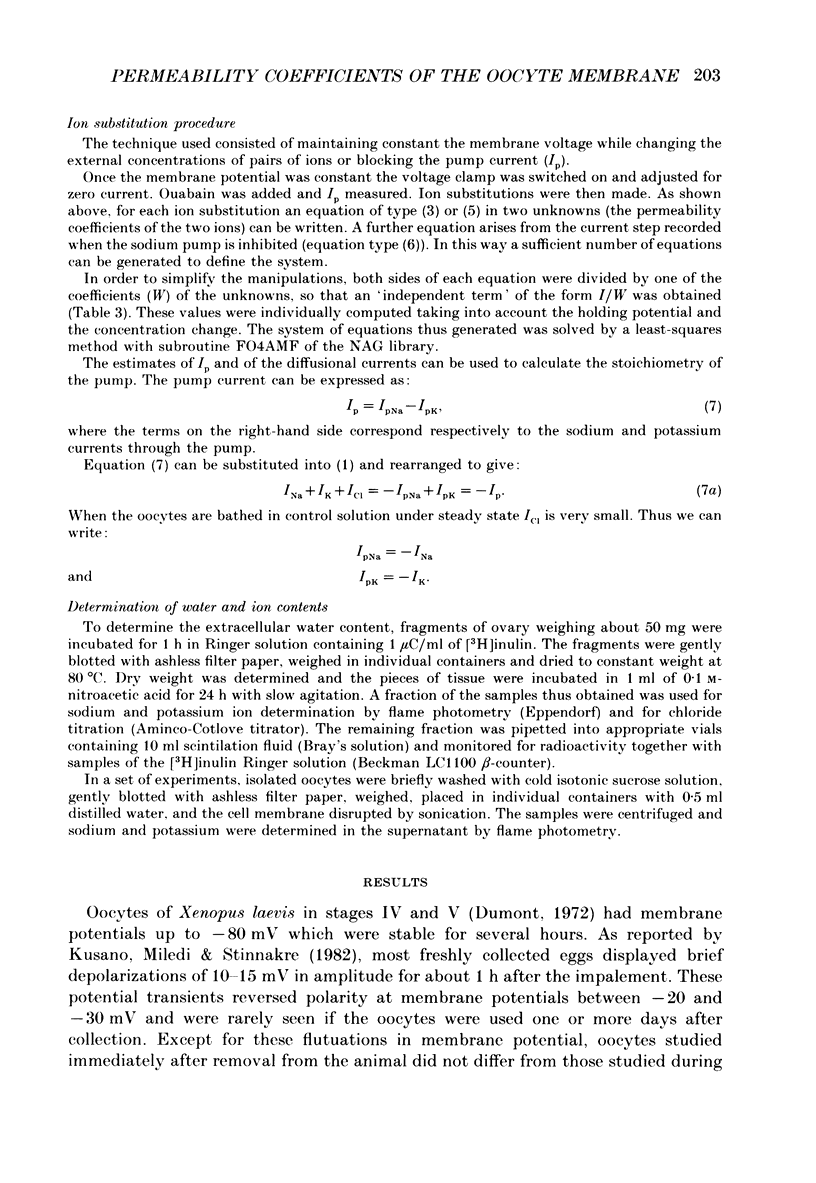
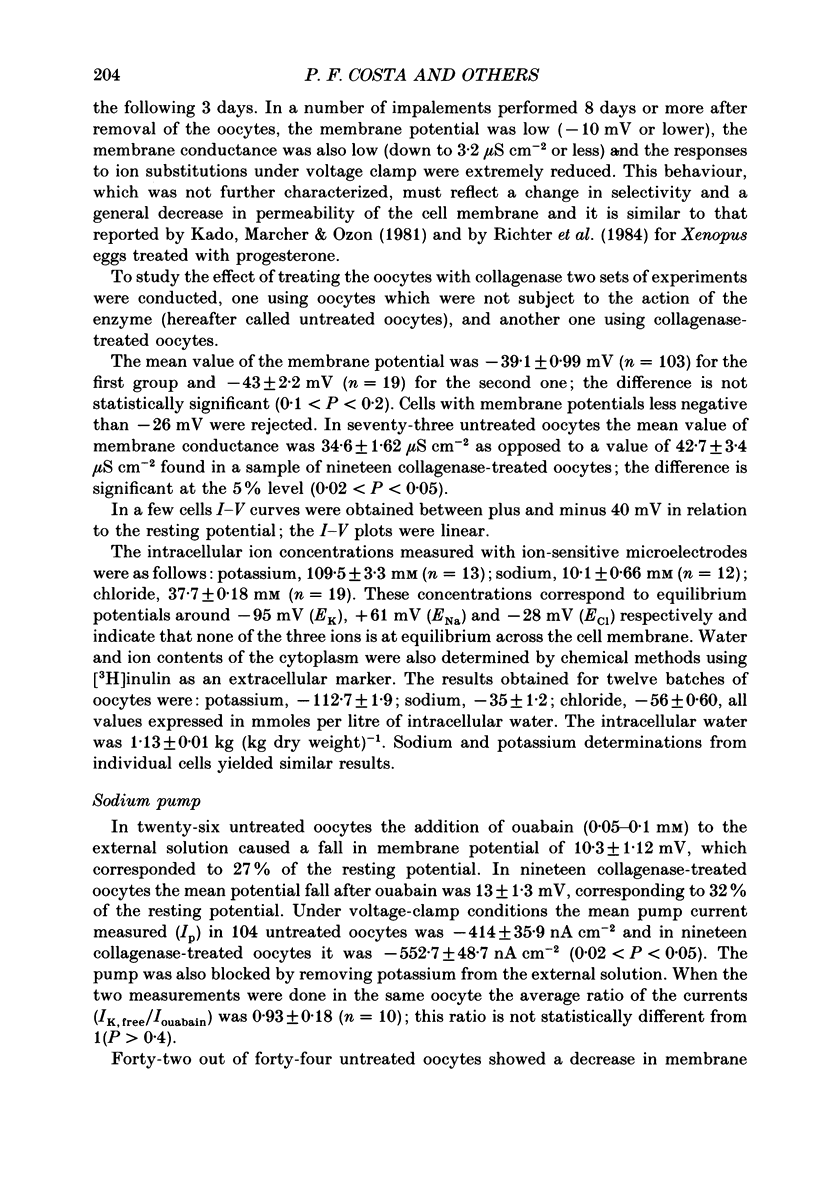
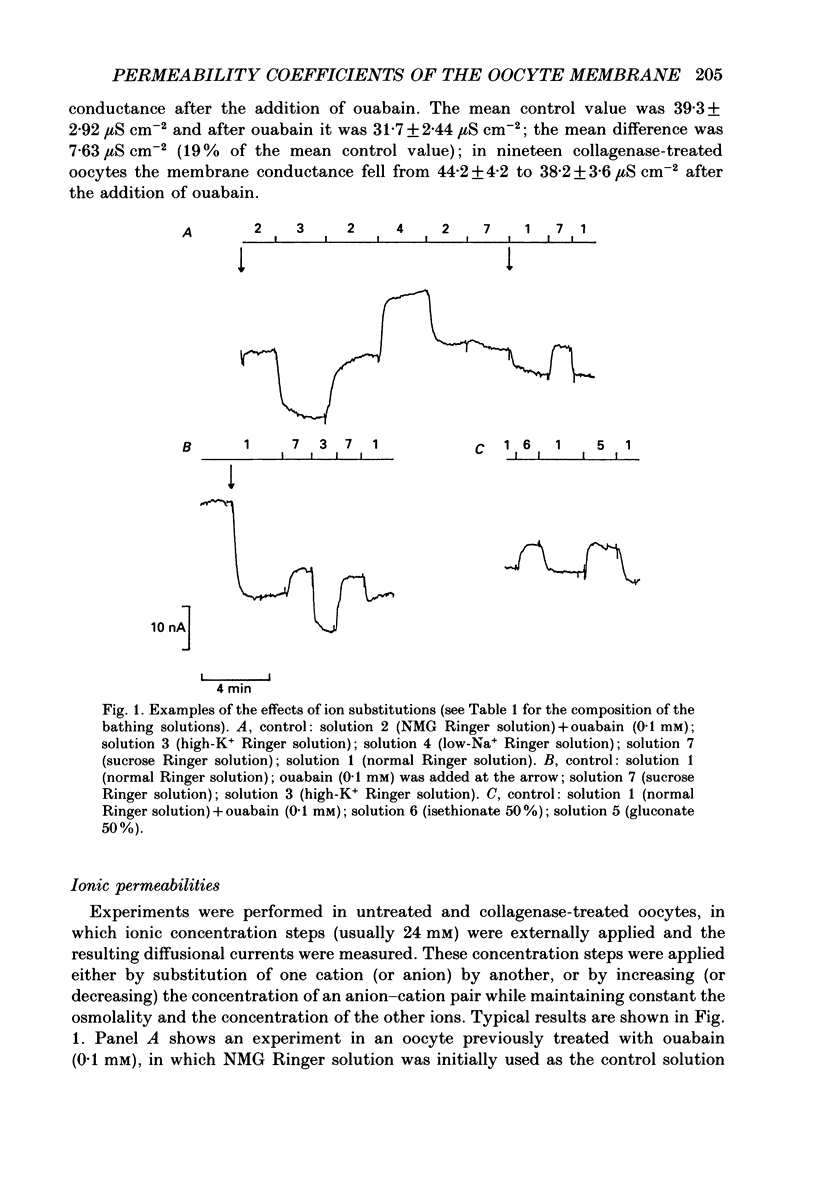
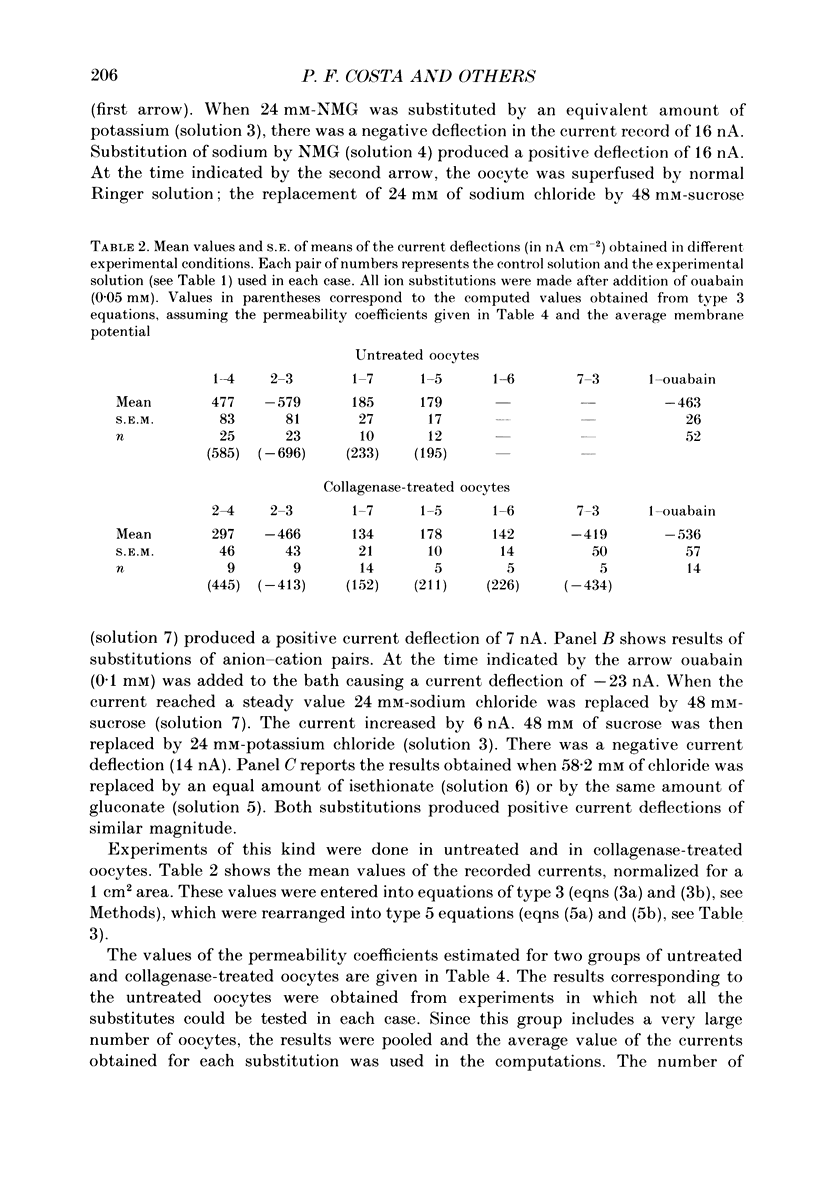
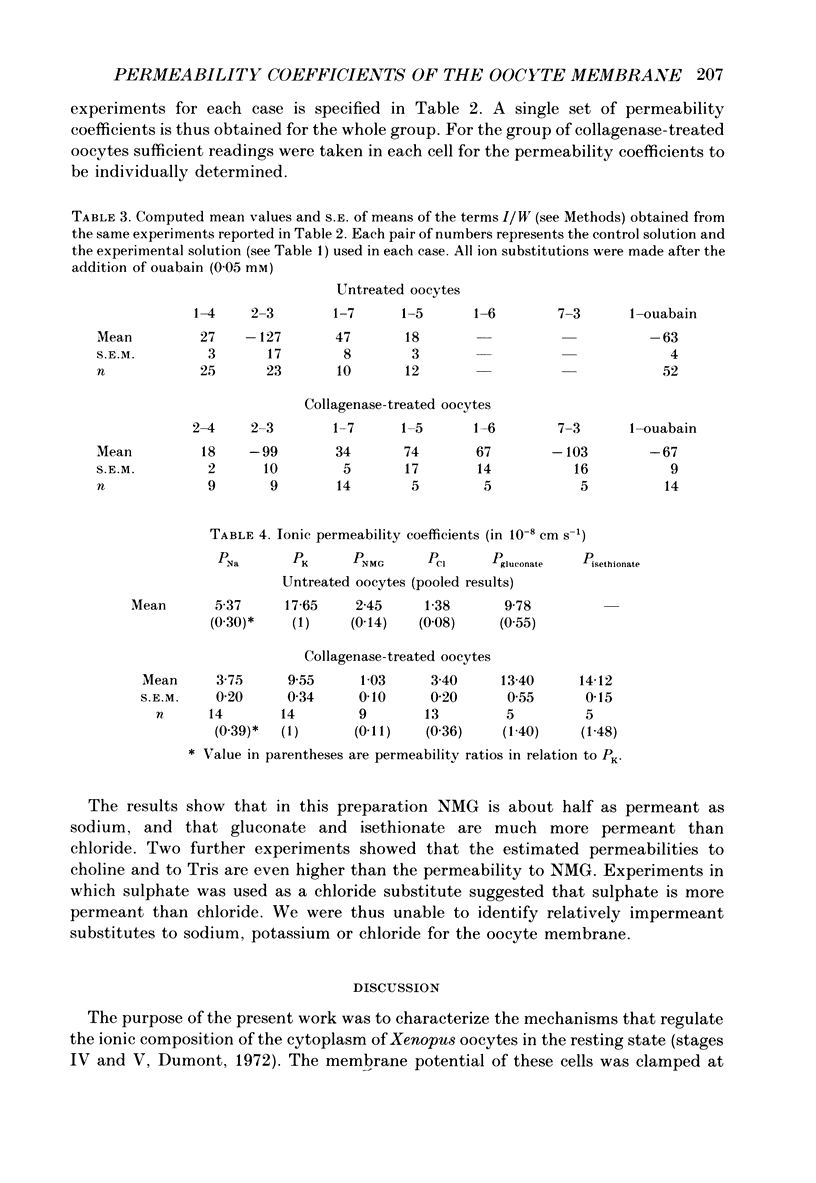
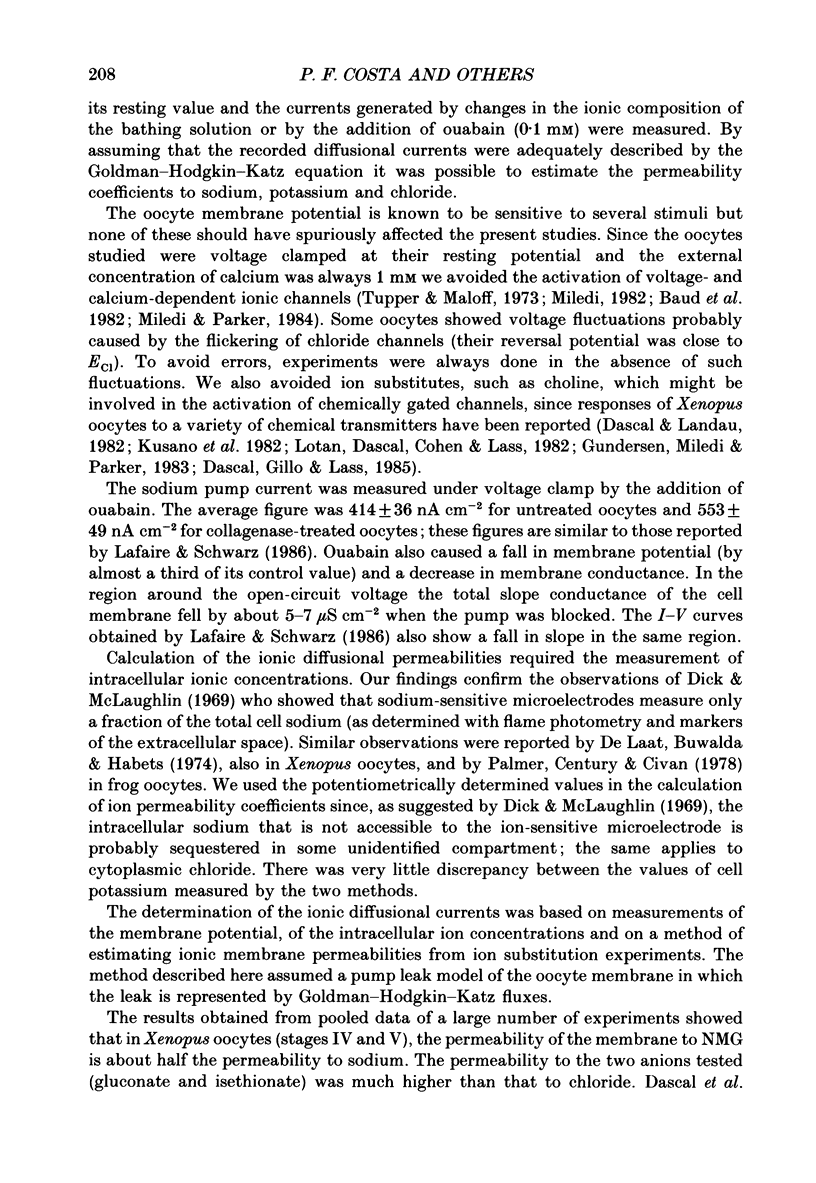
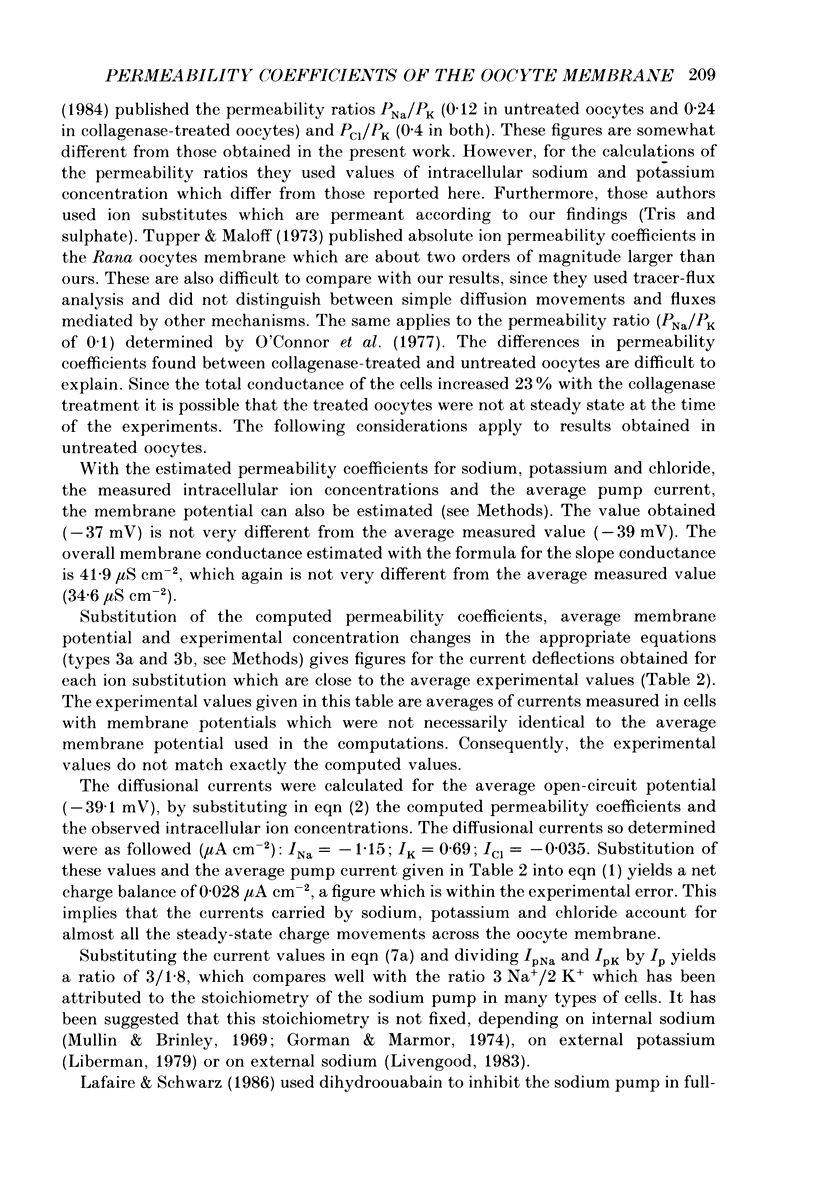
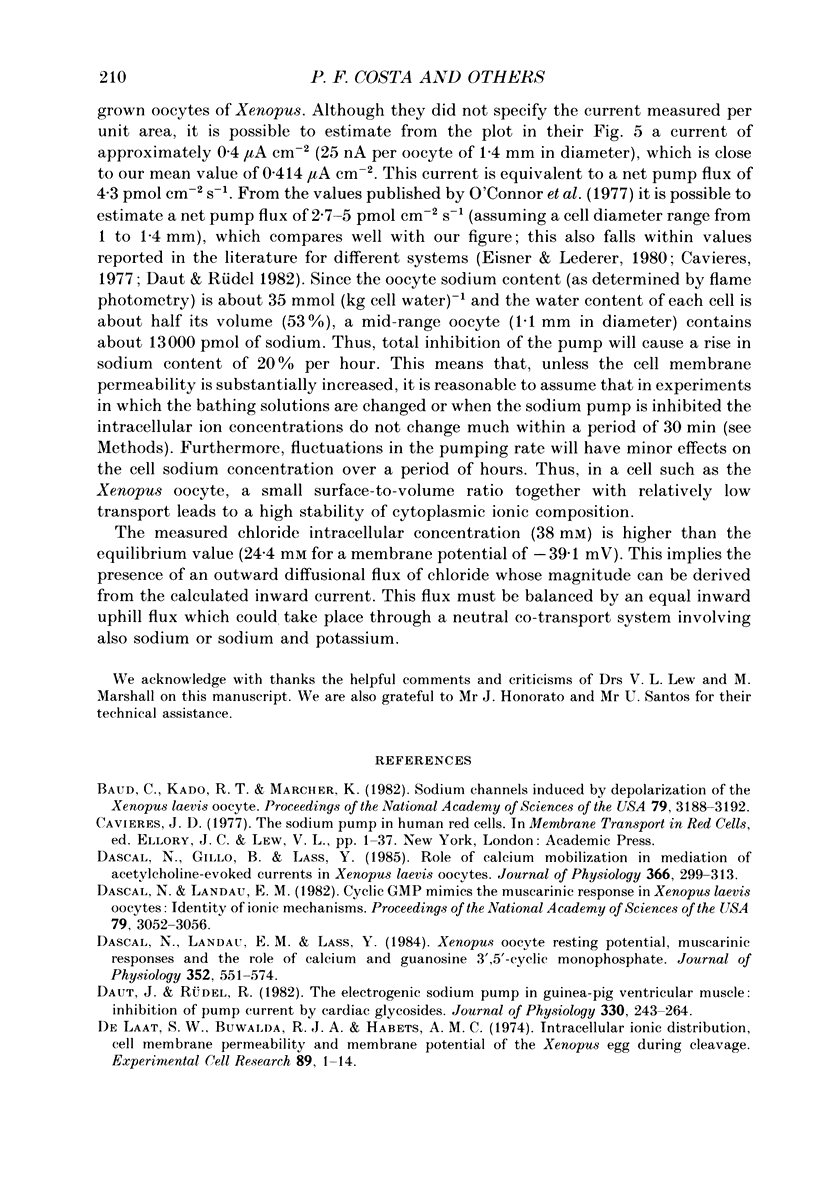
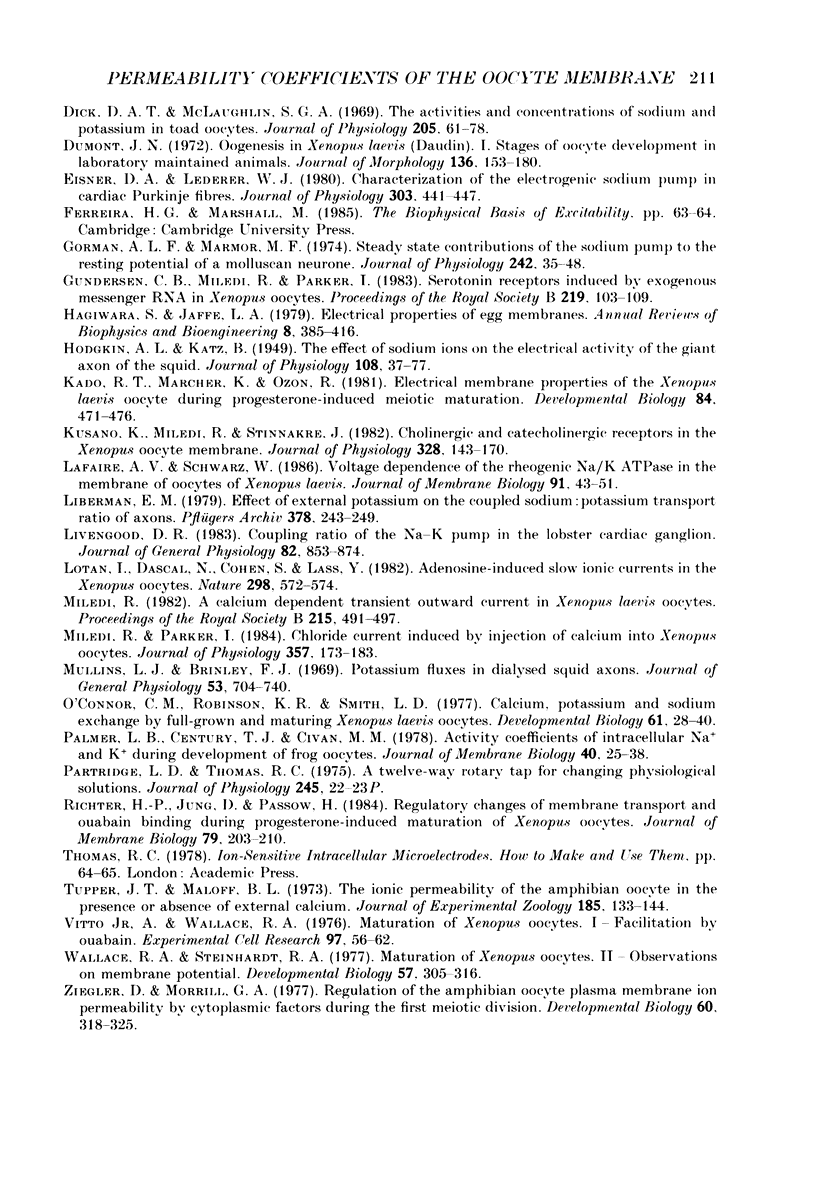
Selected References
These references are in PubMed. This may not be the complete list of references from this article.
- Baud C., Kado R. T., Marcher K. Sodium channels induced by depolarization of the Xenopus laevis oocyte. Proc Natl Acad Sci U S A. 1982 May;79(10):3188–3192. doi: 10.1073/pnas.79.10.3188. [DOI] [PMC free article] [PubMed] [Google Scholar]
- Dascal N., Gillo B., Lass Y. Role of calcium mobilization in mediation of acetylcholine-evoked chloride currents in Xenopus laevis oocytes. J Physiol. 1985 Sep;366:299–313. doi: 10.1113/jphysiol.1985.sp015799. [DOI] [PMC free article] [PubMed] [Google Scholar]
- Dascal N., Landau E. M. Cyclic GMP mimics the muscarinic response in Xenopus oocytes: identity of ionic mechanisms. Proc Natl Acad Sci U S A. 1982 May;79(9):3052–3056. doi: 10.1073/pnas.79.9.3052. [DOI] [PMC free article] [PubMed] [Google Scholar]
- Dascal N., Landau E. M., Lass Y. Xenopus oocyte resting potential, muscarinic responses and the role of calcium and guanosine 3',5'-cyclic monophosphate. J Physiol. 1984 Jul;352:551–574. doi: 10.1113/jphysiol.1984.sp015310. [DOI] [PMC free article] [PubMed] [Google Scholar]
- Daut J., Rüdel R. The electrogenic sodium pump in guinea-pig ventricular muscle: inhibition of pump current by cardiac glycosides. J Physiol. 1982 Sep;330:243–264. doi: 10.1113/jphysiol.1982.sp014339. [DOI] [PMC free article] [PubMed] [Google Scholar]
- Dick D. A., McLaughlin S. G. The activities and concentrations of sodium and potassium in toad oocytes. J Physiol. 1969 Nov;205(1):61–78. doi: 10.1113/jphysiol.1969.sp008951. [DOI] [PMC free article] [PubMed] [Google Scholar]
- Dumont J. N. Oogenesis in Xenopus laevis (Daudin). I. Stages of oocyte development in laboratory maintained animals. J Morphol. 1972 Feb;136(2):153–179. doi: 10.1002/jmor.1051360203. [DOI] [PubMed] [Google Scholar]
- Eisner D. A., Lederer W. J. Characterization of the electrogenic sodium pump in cardiac Purkinje fibres. J Physiol. 1980 Jun;303:441–474. doi: 10.1113/jphysiol.1980.sp013298. [DOI] [PMC free article] [PubMed] [Google Scholar]
- Gorman A. L., Marmor M. F. Steady-state contribution of the sodium pump to the resting potential of a molluscan neurone. J Physiol. 1974 Oct;242(1):35–48. doi: 10.1113/jphysiol.1974.sp010692. [DOI] [PMC free article] [PubMed] [Google Scholar]
- Gundersen C. B., Miledi R., Parker I. Serotonin receptors induced by exogenous messenger RNA in Xenopus oocytes. Proc R Soc Lond B Biol Sci. 1983 Aug 22;219(1214):103–109. doi: 10.1098/rspb.1983.0062. [DOI] [PubMed] [Google Scholar]
- HODGKIN A. L., KATZ B. The effect of sodium ions on the electrical activity of giant axon of the squid. J Physiol. 1949 Mar 1;108(1):37–77. doi: 10.1113/jphysiol.1949.sp004310. [DOI] [PMC free article] [PubMed] [Google Scholar]
- Hagiwara S., Jaffe L. A. Electrical properties of egg cell membranes. Annu Rev Biophys Bioeng. 1979;8:385–416. doi: 10.1146/annurev.bb.08.060179.002125. [DOI] [PubMed] [Google Scholar]
- Kusano K., Miledi R., Stinnakre J. Cholinergic and catecholaminergic receptors in the Xenopus oocyte membrane. J Physiol. 1982 Jul;328:143–170. doi: 10.1113/jphysiol.1982.sp014257. [DOI] [PMC free article] [PubMed] [Google Scholar]
- Lafaire A. V., Schwarz W. Voltage dependence of the rheogenic Na+/K+ ATPase in the membrane of oocytes of Xenopus laevis. J Membr Biol. 1986;91(1):43–51. doi: 10.1007/BF01870213. [DOI] [PubMed] [Google Scholar]
- Livengood D. R. Coupling ratio of the Na-K pump in the lobster cardiac ganglion. J Gen Physiol. 1983 Dec;82(6):853–874. doi: 10.1085/jgp.82.6.853. [DOI] [PMC free article] [PubMed] [Google Scholar]
- Lotan I., Dascal N., Cohen S., Lass Y. Adenosine-induced slow ionic currents in the Xenopus oocyte. Nature. 1982 Aug 5;298(5874):572–574. doi: 10.1038/298572a0. [DOI] [PubMed] [Google Scholar]
- Miledi R. A calcium-dependent transient outward current in Xenopus laevis oocytes. Proc R Soc Lond B Biol Sci. 1982 Jul 22;215(1201):491–497. doi: 10.1098/rspb.1982.0056. [DOI] [PubMed] [Google Scholar]
- Miledi R., Parker I. Chloride current induced by injection of calcium into Xenopus oocytes. J Physiol. 1984 Dec;357:173–183. doi: 10.1113/jphysiol.1984.sp015495. [DOI] [PMC free article] [PubMed] [Google Scholar]
- Mullins L. J., Brinley F. J., Jr Potassium fluxes in dialyzed squid axons. J Gen Physiol. 1969 Jun;53(6):704–740. doi: 10.1085/jgp.53.6.704. [DOI] [PMC free article] [PubMed] [Google Scholar]
- O'Connor C. M., Robinson K. R., Smith L. D. Calcium, potassium, and sodium exchange by full-grown and maturing Xenopus laevis oocytes. Dev Biol. 1977 Nov;61(1):28–40. doi: 10.1016/0012-1606(77)90339-6. [DOI] [PubMed] [Google Scholar]
- Palmer L. G., Century T. J., Civan M. M. Activity coefficients of intracellular Na+ and K+ during development of frog oocytes. J Membr Biol. 1978 Apr 20;40(1):25–38. doi: 10.1007/BF01909737. [DOI] [PubMed] [Google Scholar]
- Richter H. P., Jung D., Passow H. Regulatory changes of membrane transport and ouabain binding during progesterone-induced maturation of Xenopus oocytes. J Membr Biol. 1984;79(3):203–210. doi: 10.1007/BF01871059. [DOI] [PubMed] [Google Scholar]
- Tupper J. T., Maloff B. L. The ionic permeability of the amphibian oocyte in the presence or absence of external calcium. J Exp Zool. 1973 Jul;185(1):133–144. doi: 10.1002/jez.1401850113. [DOI] [PubMed] [Google Scholar]
- Vitto A., Jr, wallace R. A. Maturation of Xenopus oocytes. I. Facilitation by ouabain. Exp Cell Res. 1976 Jan;97:56–62. doi: 10.1016/0014-4827(76)90654-6. [DOI] [PubMed] [Google Scholar]
- Wallace R. A., Steinhardt R. A. Maturation of Xenopus oocytes. II. Observations on membrane potential. Dev Biol. 1977 Jun;57(2):305–316. doi: 10.1016/0012-1606(77)90217-2. [DOI] [PubMed] [Google Scholar]
- Ziegler D., Morrill G. A. Regulation of the amphibian oocyte plasma membrane ion permeability by cytoplasmic factors during the first meiotic division. Dev Biol. 1977 Oct 1;60(1):318–325. doi: 10.1016/0012-1606(77)90129-4. [DOI] [PubMed] [Google Scholar]
- de Laat S. W., Buwalda R. J., Habets A. M. Intracellular ionic distribution, cell membrane permeability and membrane potential of the Xenopus egg during first cleavage. Exp Cell Res. 1974 Nov;89(1):1–14. doi: 10.1016/0014-4827(74)90180-3. [DOI] [PubMed] [Google Scholar]