Abstract
1. Intracellular microelectrodes have been used to record the electrical activity of smooth muscle cells of the circular layer from full length strips of mouse colon in vitro. The membrane potential was unstable and showed slow depolarizations (mean amplitude, 10.9 mV; mean frequency, 0.008 Hz; mean duration, 56.4 s). 2. A variable number (mean fifty-six) of rapid oscillations in membrane potential (mean amplitude, 10.2 mV) with a frequency of approximately 2 Hz and a duration of approximately 400 ms were superimposed on each slow depolarization. Occasionally, action potentials arose from the rapid oscillations. The action potentials, but neither the slow depolarizations nor the rapid oscillations, were abolished by 1.0 microM-nifedipine. 3. The majority of the slow depolarizations and the associated rapid oscillations migrated aborally along the colon at a velocity of between 0.5 and 1.5 mm s-1; in the distal colon the slow depolarization was often preceded by a small hyperpolarization. 4. During the rising and plateau phase of the slow depolarization the amplitude of electronic potentials was decreased. Hyperpolarization induced by passing current during the slow depolarization increased the amplitude of the rapid oscillations. 5. Transmural electrical stimulation (single pulses) in the presence of nifedipine evoked (1 mm anal to the stimulating electrodes) an inhibitory junction potential which was sometimes preceded by an excitatory junction potential. The amplitude, of the evoked inhibitory junction potential was decreased during the rising and plateau phase of the slow depolarization. 6. The slow depolarization and the rapid oscillations were abolished by hexamethonium (500 microM), morphine (1-10 microM) and tetrodotoxin (3.1 microM). Atropine (3.5 microM) abolished the rapid oscillations and reduced the amplitude of the slow depolarization. 7. Atropine (3.5 microM) and morphine (10 microM) abolished the evoked excitatory junction potential whilst tetrodotoxin (3.1 microM) abolished both the excitatory and the inhibitory junction potential. 8. It is suggested that the migrating depolarization and accompanying oscillations, which are neurogenic in origin, represent the electrical correlate in the circular muscle layer of the migrating colonic motor complex which has been associated with the propulsion of faecal pellets along the colon.
Full text
PDF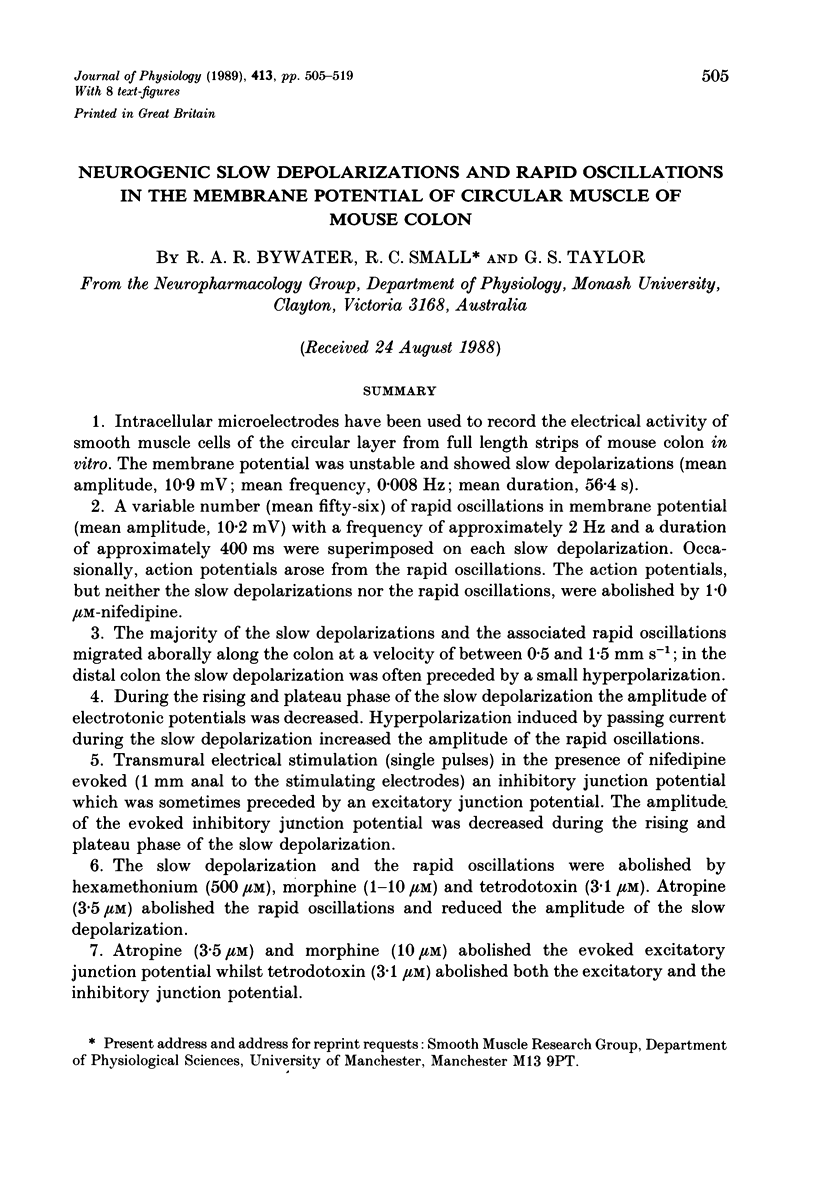
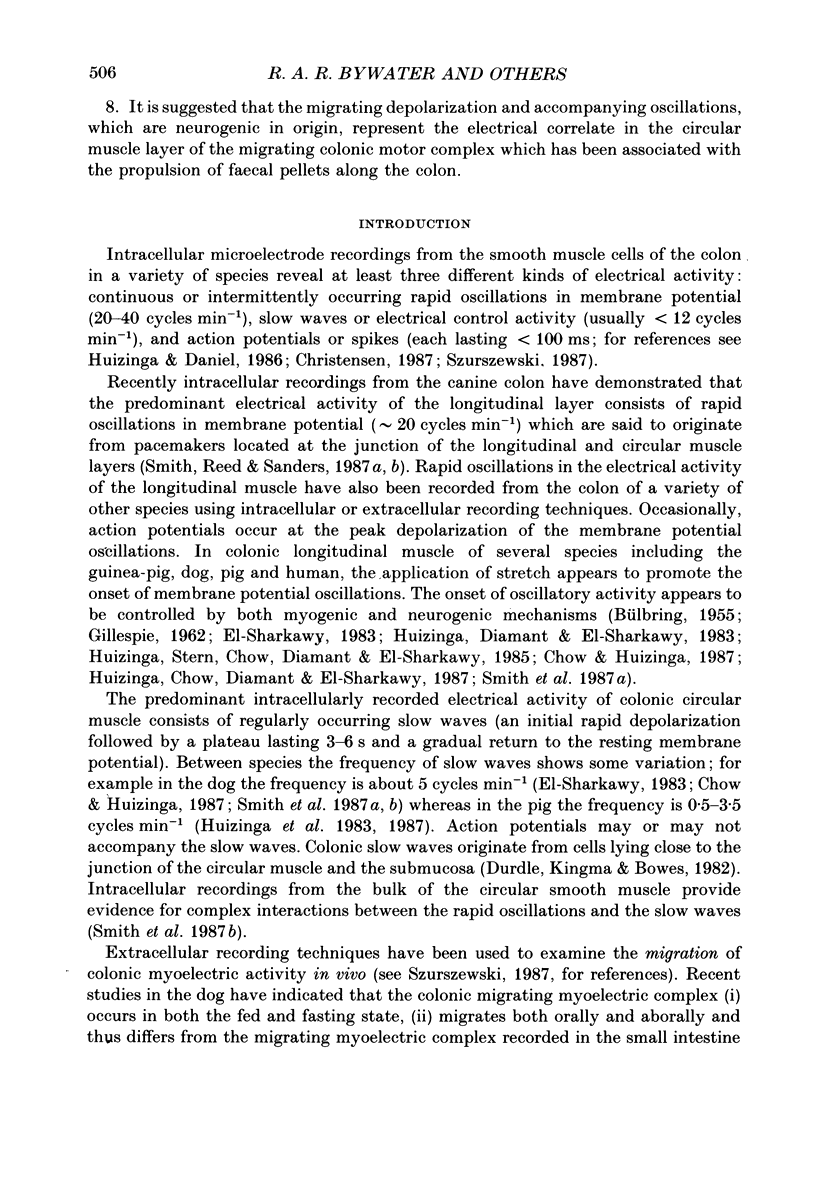
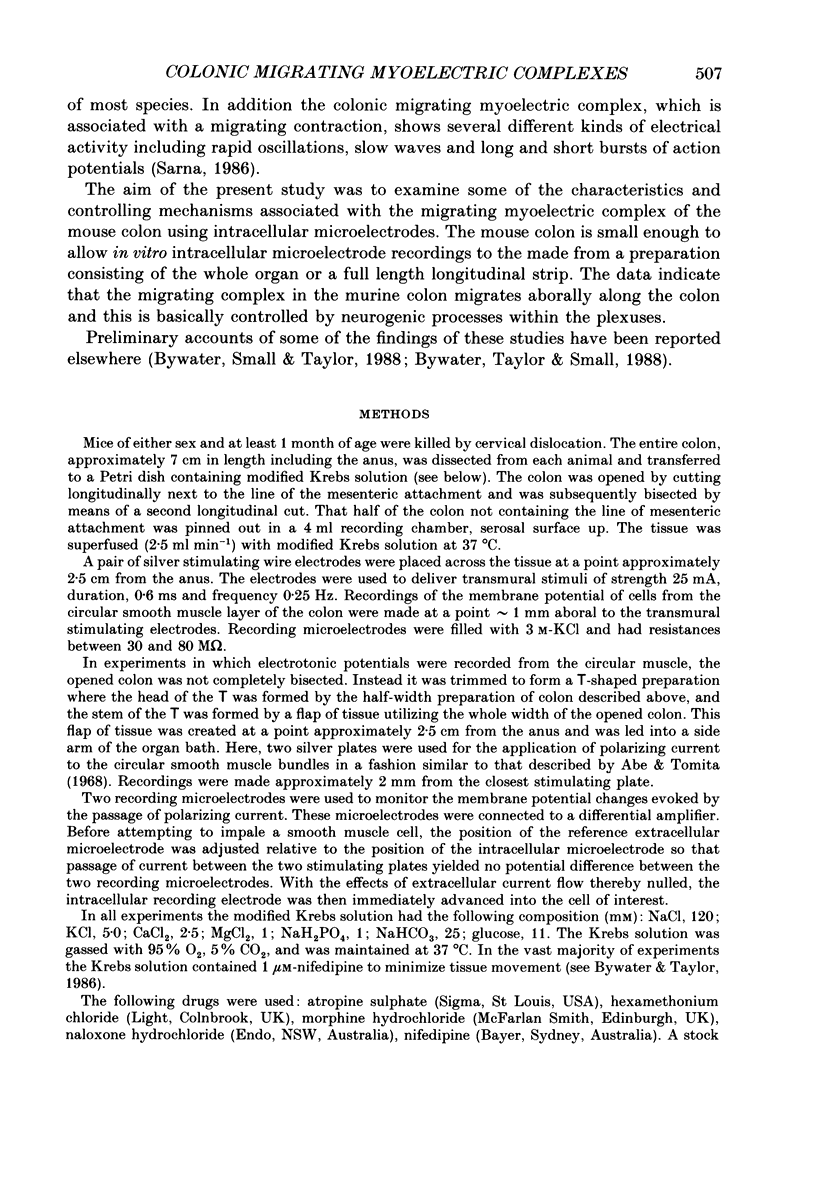
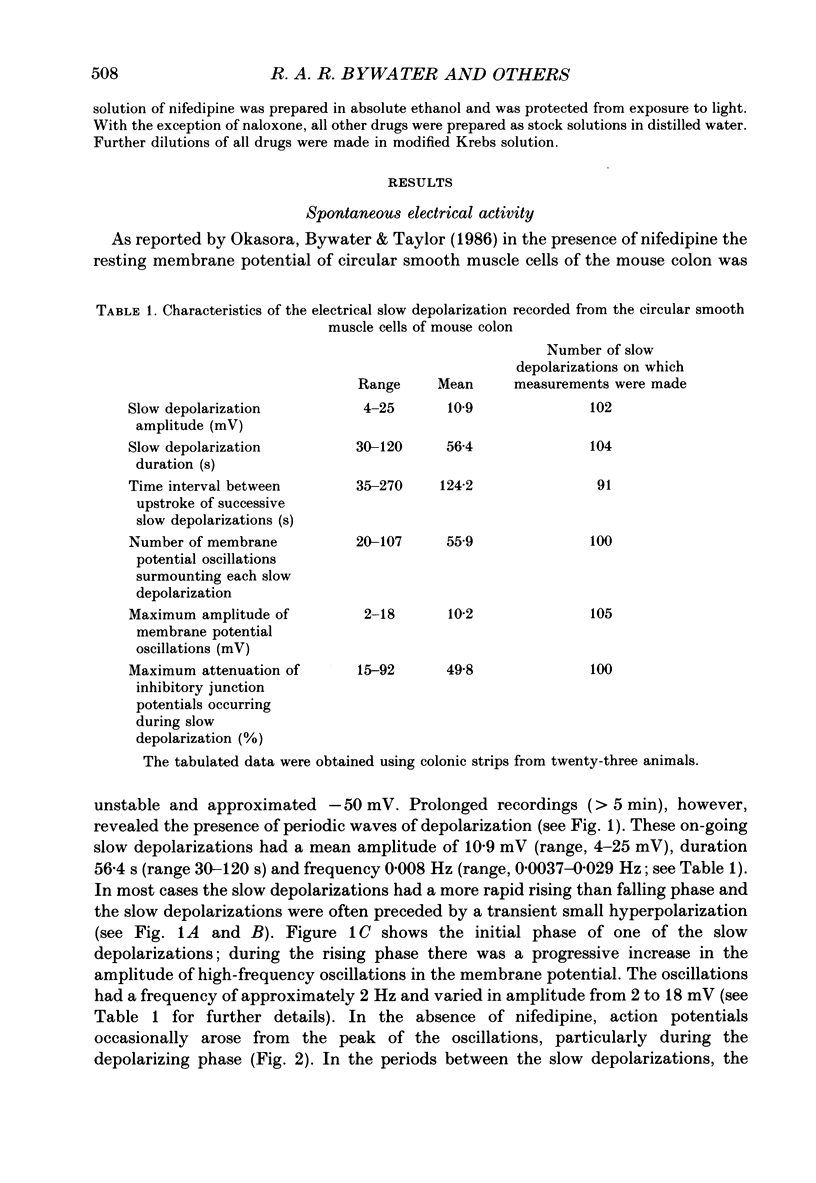
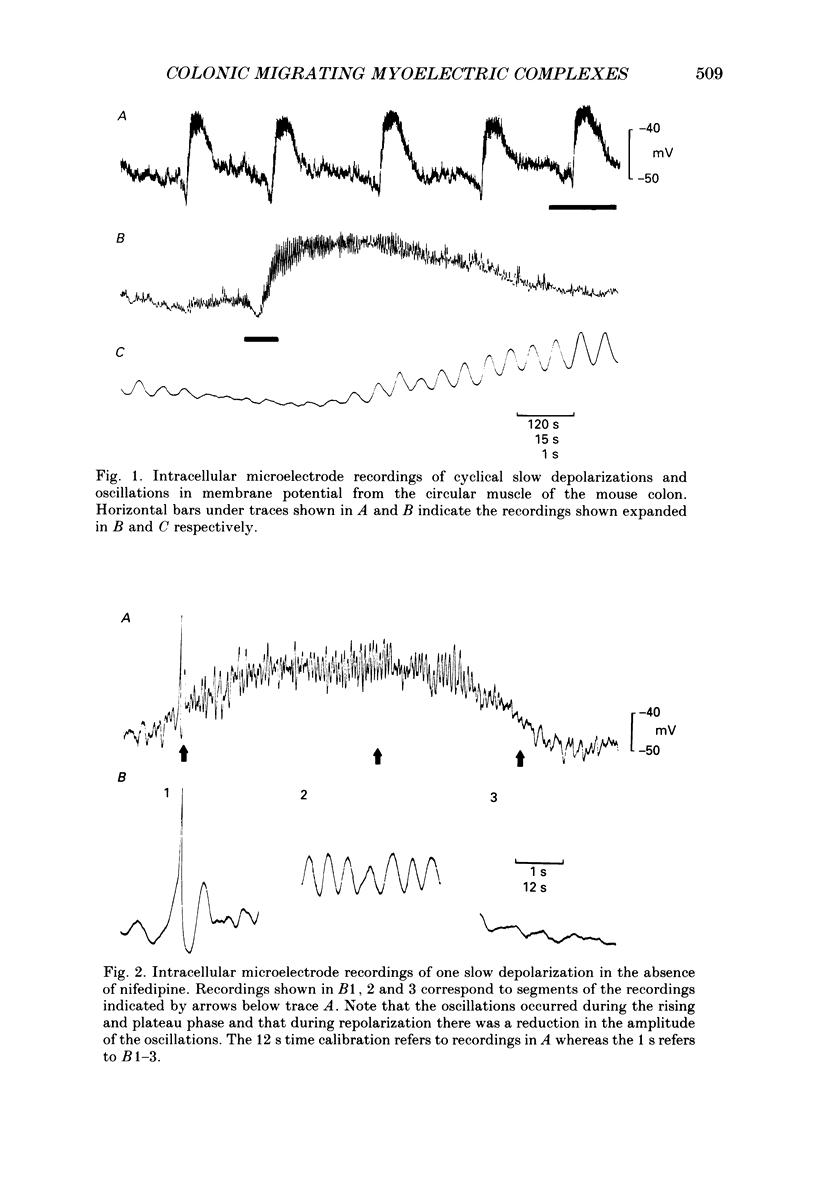
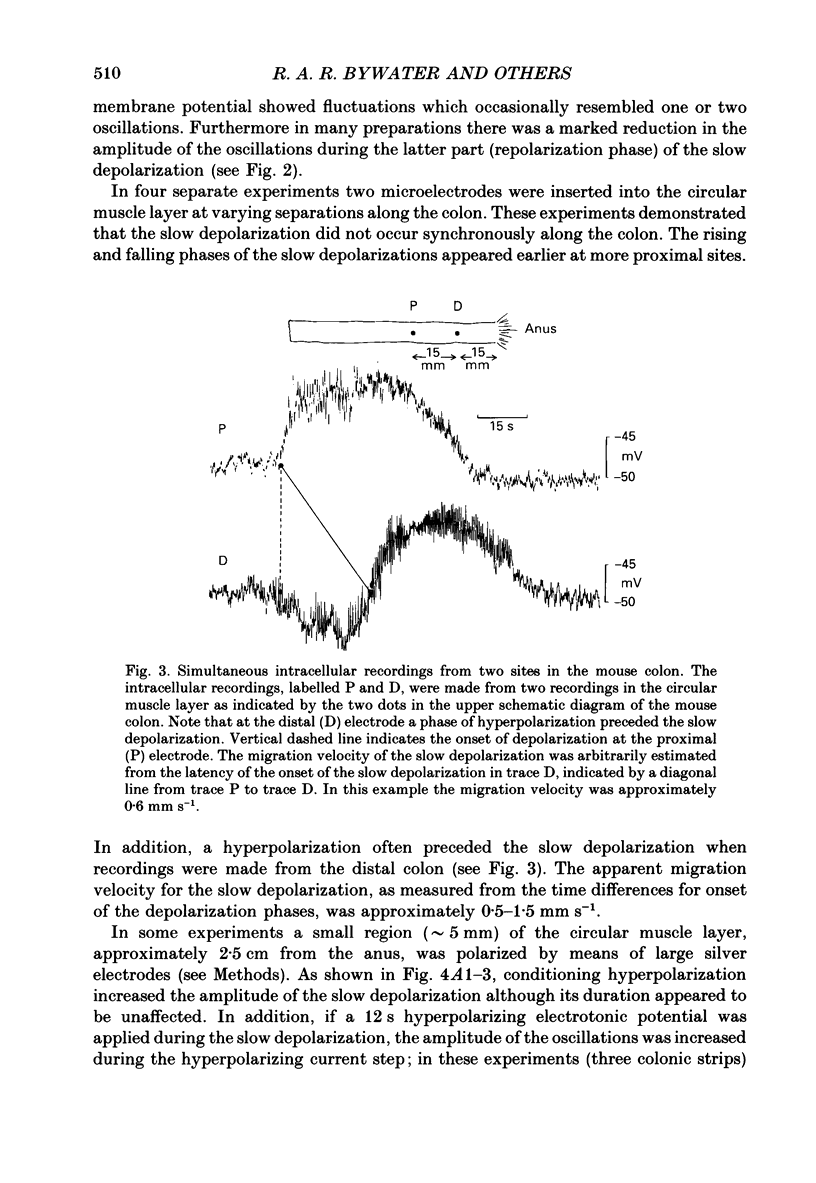
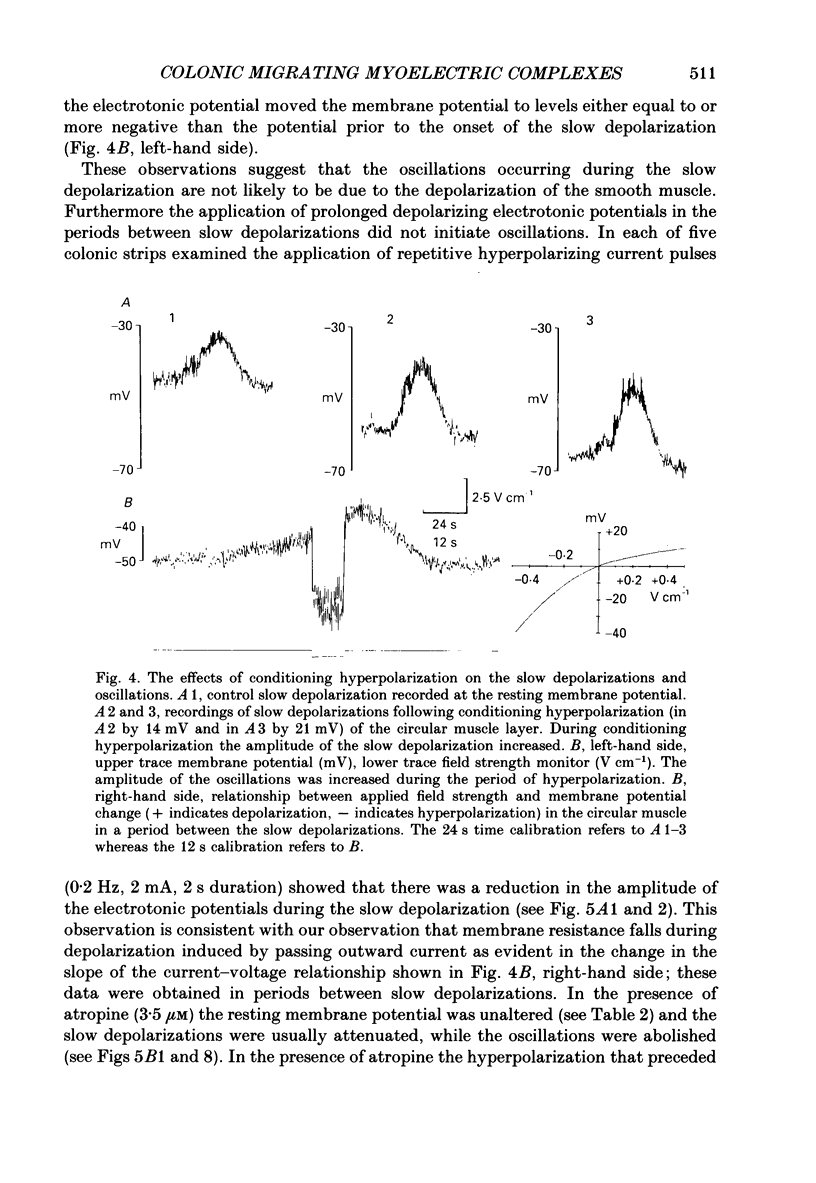
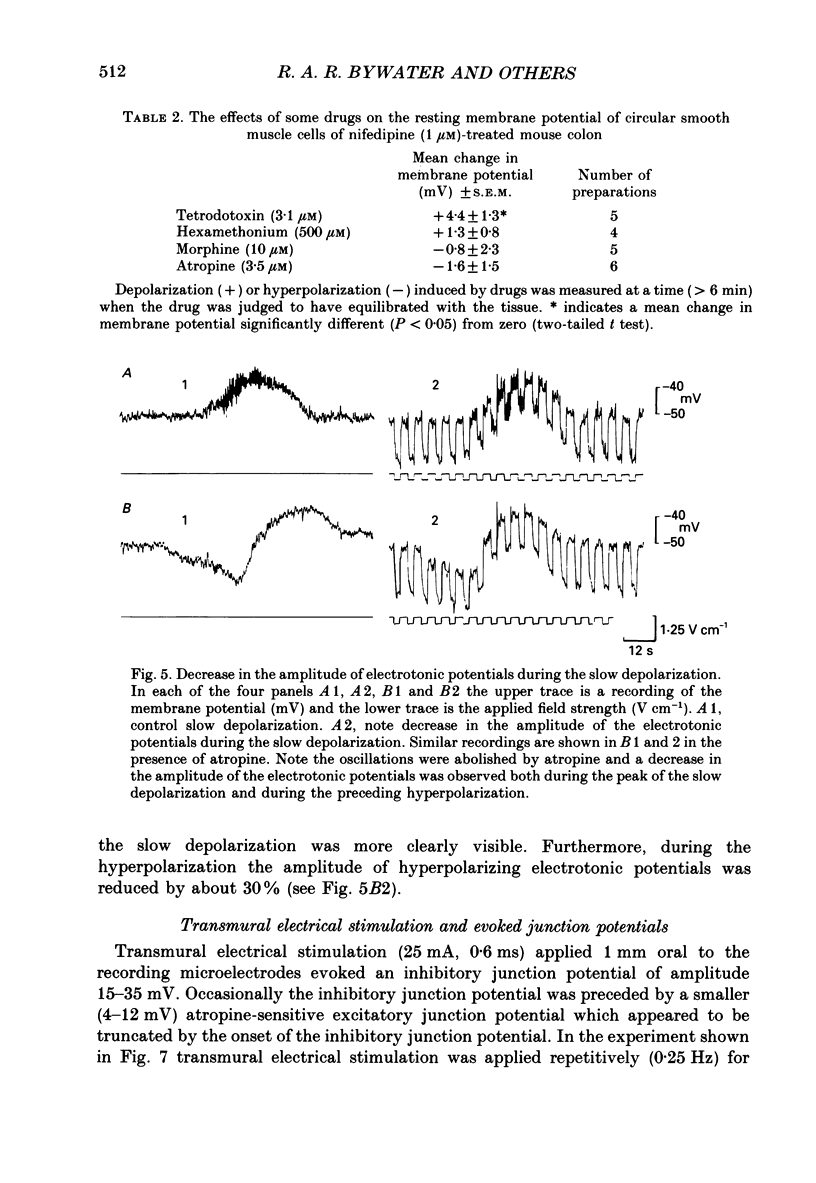
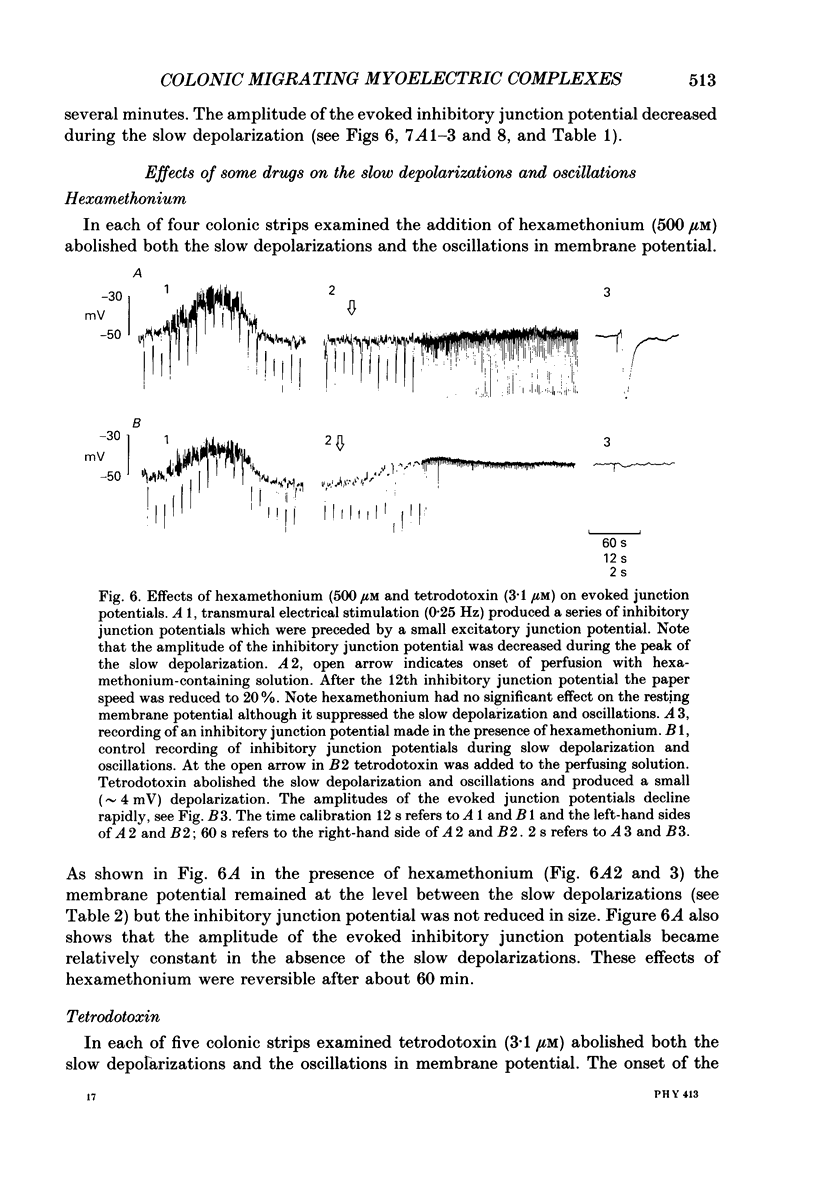
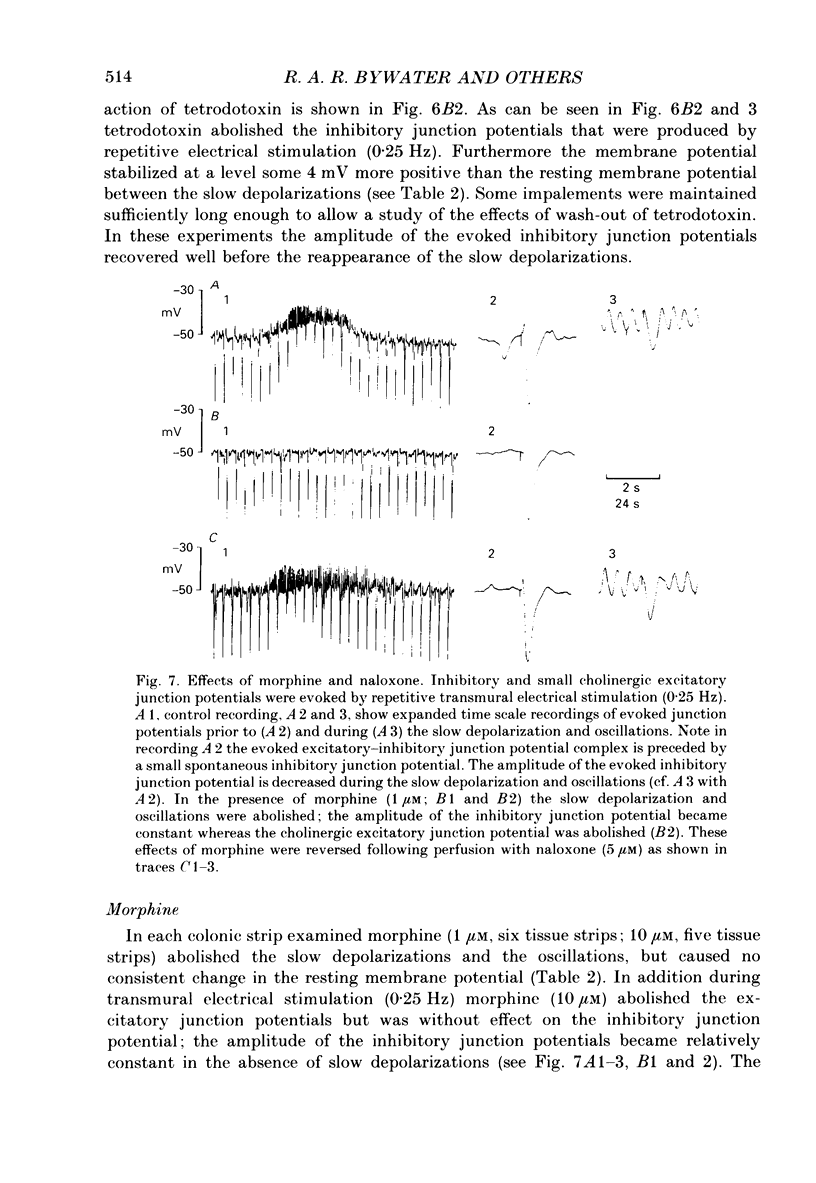
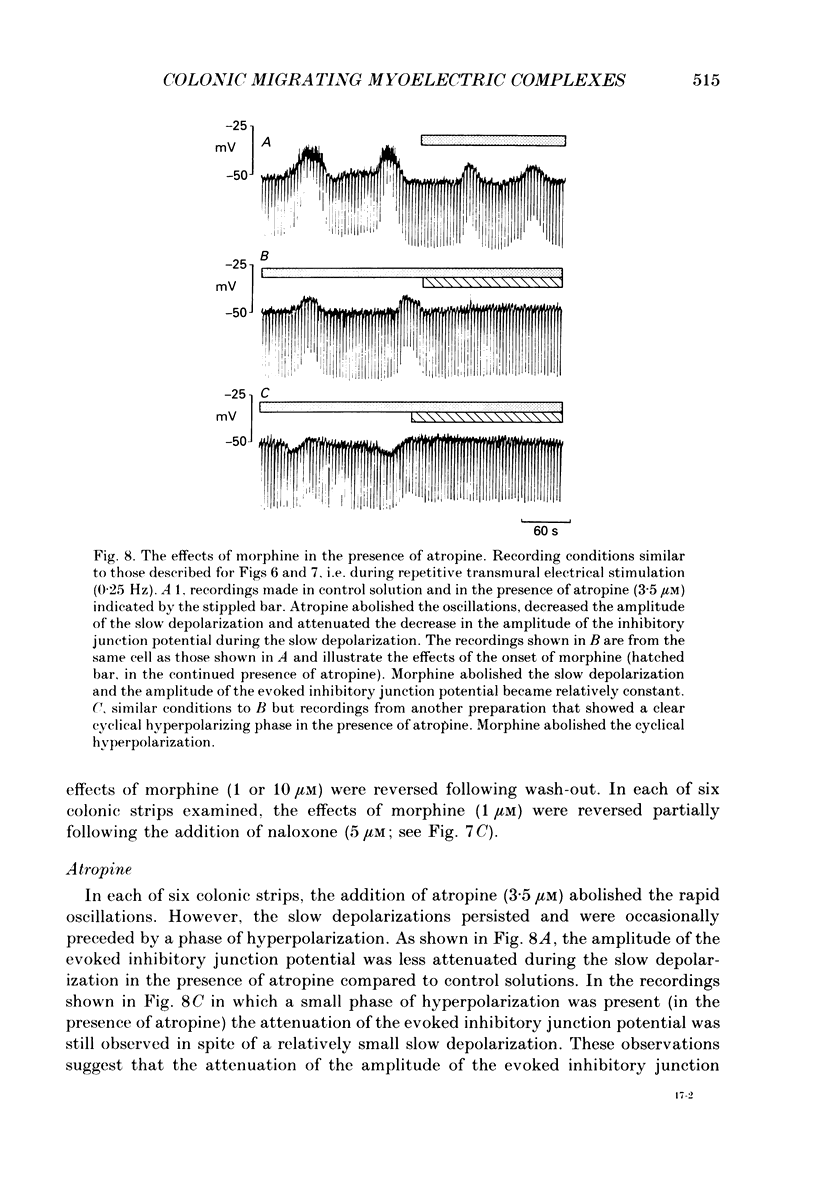
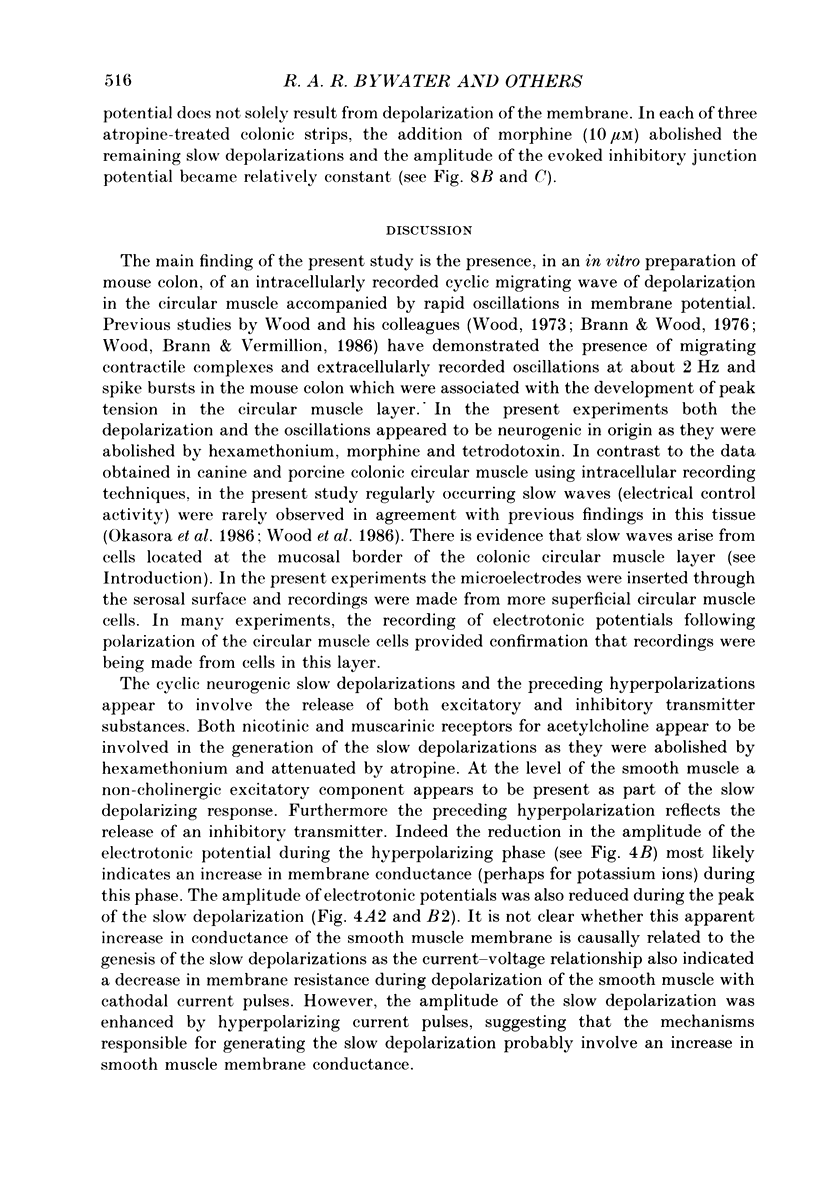
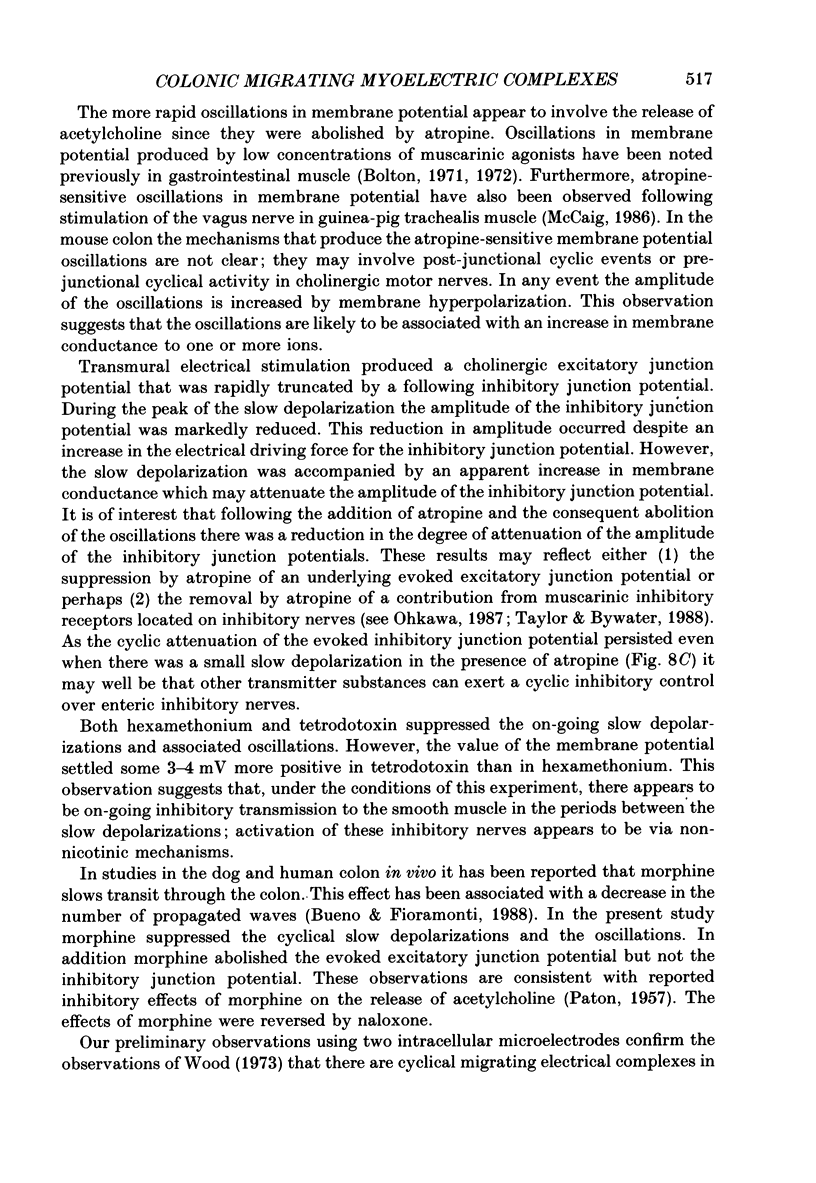
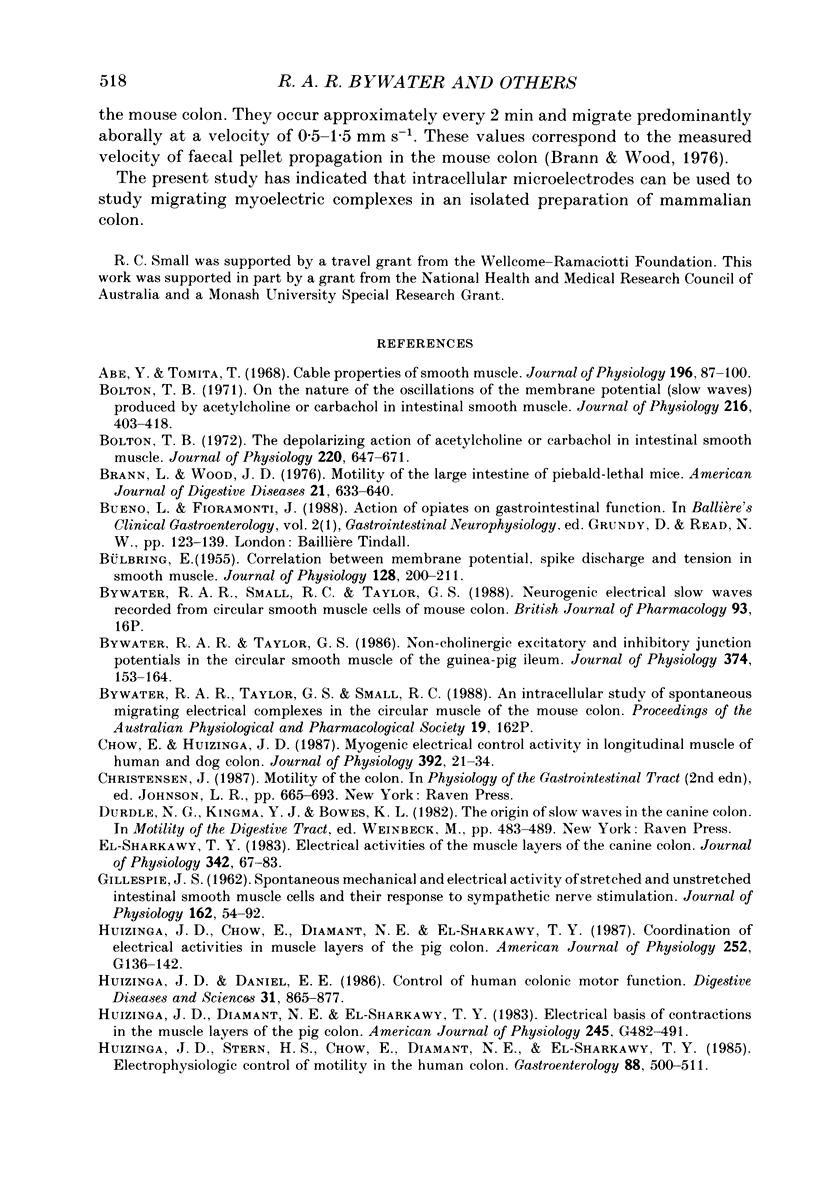
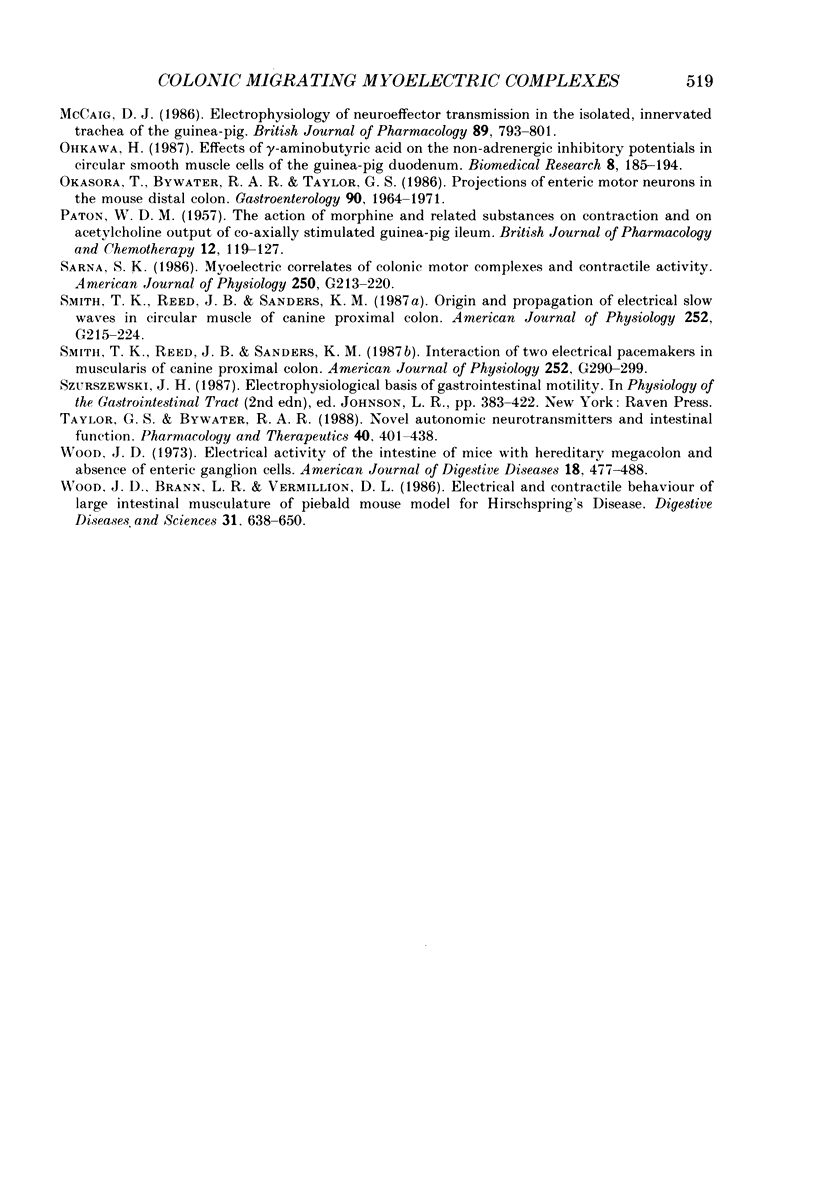
Selected References
These references are in PubMed. This may not be the complete list of references from this article.
- Abe Y., Tomita T. Cable properties of smooth muscle. J Physiol. 1968 May;196(1):87–100. doi: 10.1113/jphysiol.1968.sp008496. [DOI] [PMC free article] [PubMed] [Google Scholar]
- BULBRING E. Correlation between membrane potential, spike discharge and tension in smooth muscle. J Physiol. 1955 Apr 28;128(1):200–221. doi: 10.1113/jphysiol.1955.sp005299. [DOI] [PMC free article] [PubMed] [Google Scholar]
- Bolton T. B. On the nature of the oscillations of the membrane potential (slow waves) produced by acetylcholine or carbachol in intestinal smooth muscle. J Physiol. 1971 Jul;216(2):403–418. doi: 10.1113/jphysiol.1971.sp009532. [DOI] [PMC free article] [PubMed] [Google Scholar]
- Bolton T. B. The depolarizing action of acetylcholine or carbachol in intestinal smooth muscle. J Physiol. 1972 Feb;220(3):647–671. doi: 10.1113/jphysiol.1972.sp009728. [DOI] [PMC free article] [PubMed] [Google Scholar]
- Brann L., Wood J. D. Motility of the large intestine of piebald-lethal mice. Am J Dig Dis. 1976 Aug;21(8):633–640. doi: 10.1007/BF01071956. [DOI] [PubMed] [Google Scholar]
- Bywater R. A., Taylor G. S. Non-cholinergic excitatory and inhibitory junction potentials in the circular smooth muscle of the guinea-pig ileum. J Physiol. 1986 May;374:153–164. doi: 10.1113/jphysiol.1986.sp016072. [DOI] [PMC free article] [PubMed] [Google Scholar]
- Chow E., Huizinga J. D. Myogenic electrical control activity in longitudinal muscle of human and dog colon. J Physiol. 1987 Nov;392:21–34. doi: 10.1113/jphysiol.1987.sp016767. [DOI] [PMC free article] [PubMed] [Google Scholar]
- El-Sharkawy T. Y. Electrical activities of the muscle layers of the canine colon. J Physiol. 1983 Sep;342:67–83. doi: 10.1113/jphysiol.1983.sp014840. [DOI] [PMC free article] [PubMed] [Google Scholar]
- GILLESPIE J. S. Spontaneous mechanical and electrical activity of stretched and unstretched intestinal smooth muscle cells and their response to sympathetic-nerve stimulation. J Physiol. 1962 Jun;162:54–75. doi: 10.1113/jphysiol.1962.sp006914. [DOI] [PMC free article] [PubMed] [Google Scholar]
- Huizinga J. D., Chow E., Diamant N. E., el-Sharkaway T. Y. Coordination of electrical activities in muscle layers of the pig colon. Am J Physiol. 1987 Jan;252(1 Pt 1):G136–G142. doi: 10.1152/ajpgi.1987.252.1.G136. [DOI] [PubMed] [Google Scholar]
- Huizinga J. D., Daniel E. E. Control of human colonic motor function. Dig Dis Sci. 1986 Aug;31(8):865–877. doi: 10.1007/BF01296057. [DOI] [PubMed] [Google Scholar]
- Huizinga J. D., Diamant N. E., El-Sharkawy T. Y. Electrical basis of contractions in the muscle layers of the pig colon. Am J Physiol. 1983 Oct;245(4):G482–G491. doi: 10.1152/ajpgi.1983.245.4.G482. [DOI] [PubMed] [Google Scholar]
- Huizinga J. D., Stern H. S., Chow E., Diamant N. E., El-Sharkawy T. Y. Electrophysiologic control of motility in the human colon. Gastroenterology. 1985 Feb;88(2):500–511. doi: 10.1016/0016-5085(85)90513-x. [DOI] [PubMed] [Google Scholar]
- McCaig D. J. Electrophysiology of neuroeffector transmission in the isolated, innervated trachea of the guinea-pig. Br J Pharmacol. 1986 Dec;89(4):793–801. doi: 10.1111/j.1476-5381.1986.tb11184.x. [DOI] [PMC free article] [PubMed] [Google Scholar]
- Okasora T., Bywater R. A., Taylor G. S. Projections of enteric motor neurons in the mouse distal colon. Gastroenterology. 1986 Jun;90(6):1964–1971. doi: 10.1016/0016-5085(86)90268-4. [DOI] [PubMed] [Google Scholar]
- PATON W. D. The action of morphine and related substances on contraction and on acetylcholine output of coaxially stimulated guinea-pig ileum. Br J Pharmacol Chemother. 1957 Mar;12(1):119–127. doi: 10.1111/j.1476-5381.1957.tb01373.x. [DOI] [PMC free article] [PubMed] [Google Scholar]
- Sarna S. K. Myoelectric correlates of colonic motor complexes and contractile activity. Am J Physiol. 1986 Feb;250(2 Pt 1):G213–G220. doi: 10.1152/ajpgi.1986.250.2.G213. [DOI] [PubMed] [Google Scholar]
- Taylor G. S., Bywater R. A. Novel autonomic neurotransmitters and intestinal function. Pharmacol Ther. 1989;40(3):401–438. doi: 10.1016/0163-7258(89)90087-9. [DOI] [PubMed] [Google Scholar]
- Wood J. D., Brann L. R., Vermillion D. L. Electrical and contractile behavior of large intestinal musculature of piebald mouse model for Hirschsprung's disease. Dig Dis Sci. 1986 Jun;31(6):638–650. doi: 10.1007/BF01318696. [DOI] [PubMed] [Google Scholar]