Abstract
1. Calcium currents were recorded with whole-cell voltage-clamp procedures in relay neurones of the rat thalamus which had been acutely isolated by an enzymatic dissociation procedure. 2. Low-threshold and high-threshold Ca2+ currents were elicited by depolarizing voltage steps from holding potentials more negative than -60 mV. A transient current, analogous to the T-current in sensory neurones, was activated at low threshold near -65 mV and was completely inactivating at command steps up to -35 mV. Voltage steps to more depolarized levels activated a high-threshold current that inactivated slowly and incompletely during a 200 ms step depolarization. 3. The high-threshold current contained both non-inactivating and slowly inactivating components which were insensitive and sensitive to holding potential, respectively. 4. A 'T-type' current was prominent in relay neurones, in both absolute terms (350 pA peak current average) and in relation to high-threshold currents. The average ratio of maximum transient to maximum sustained current was greater than 2. 5. T-current could be modelled in a manner analogous to that employed for the fast Na+ current underlying action potential generation, using the m3h format. The rate of activation of T-current was voltage dependent, with a time constant (tau m) varying between 8 and 2 ms at command potentials of -60 to -10 mV at 23 degrees C. The rate of inactivation was also voltage dependent, and the time constant tau h varied between 50 and 20 ms over the same voltage range. With command potentials more positive than -35 mV, the inactivation of Ca2+ current could no longer be fitted by a single exponential. 6. Steady-state inactivation of T-current could be well fitted by a Boltzman equation with slope factor of 6.3 and half-inactivated voltage of -83.5 mV. 7. Recovery from inactivation of T-current was not exponential. The major component of recovery (70-80% of total) was not very voltage sensitive at potentials more negative than -90 mV, with tau r of 251 ms at -92 mV and 23 degrees C, compared to 225 ms at -112 mV. A smaller, voltage-sensitive component accounted for the remainder of recovery. 8. All kinetic properties, including rates of activation, inactivation, and recovery from inactivation, as well as the amplitude of T-current, were temperature sensitive with Q10 (temperature coefficient) values of greater than 2.5.(ABSTRACT TRUNCATED AT 400 WORDS)
Full text
PDF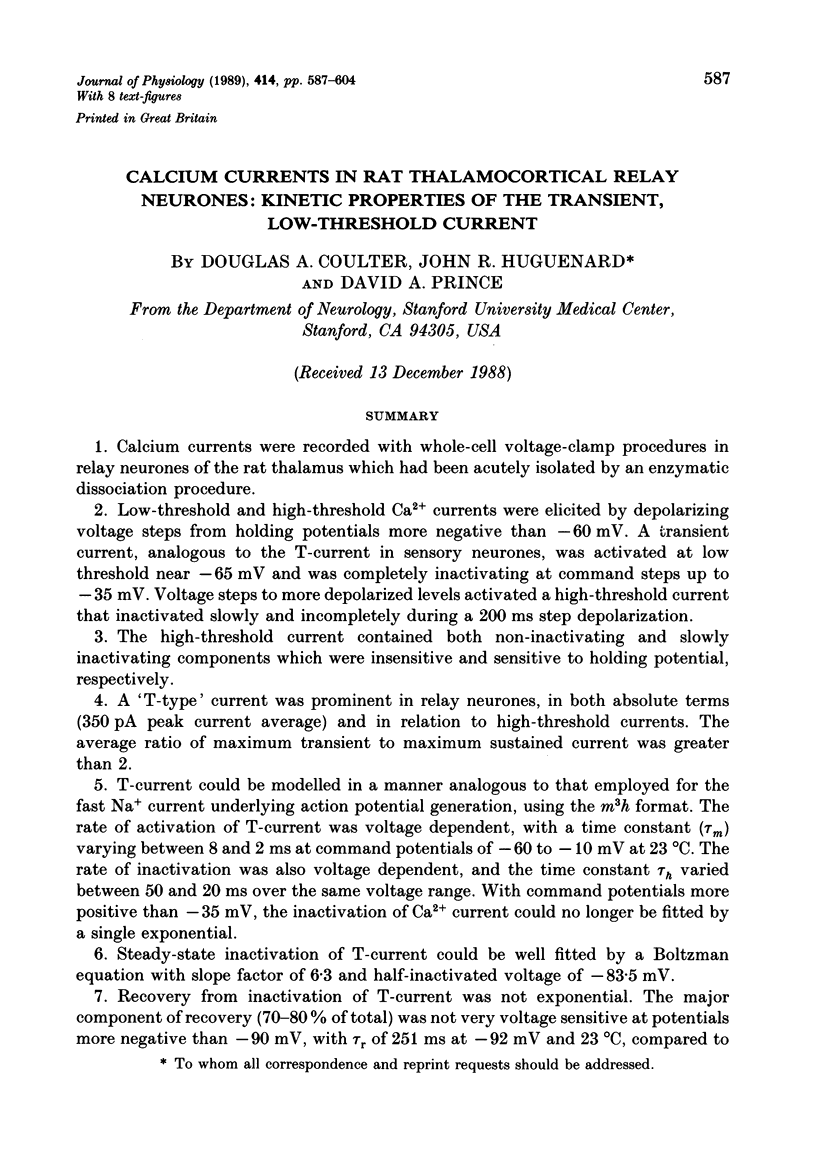
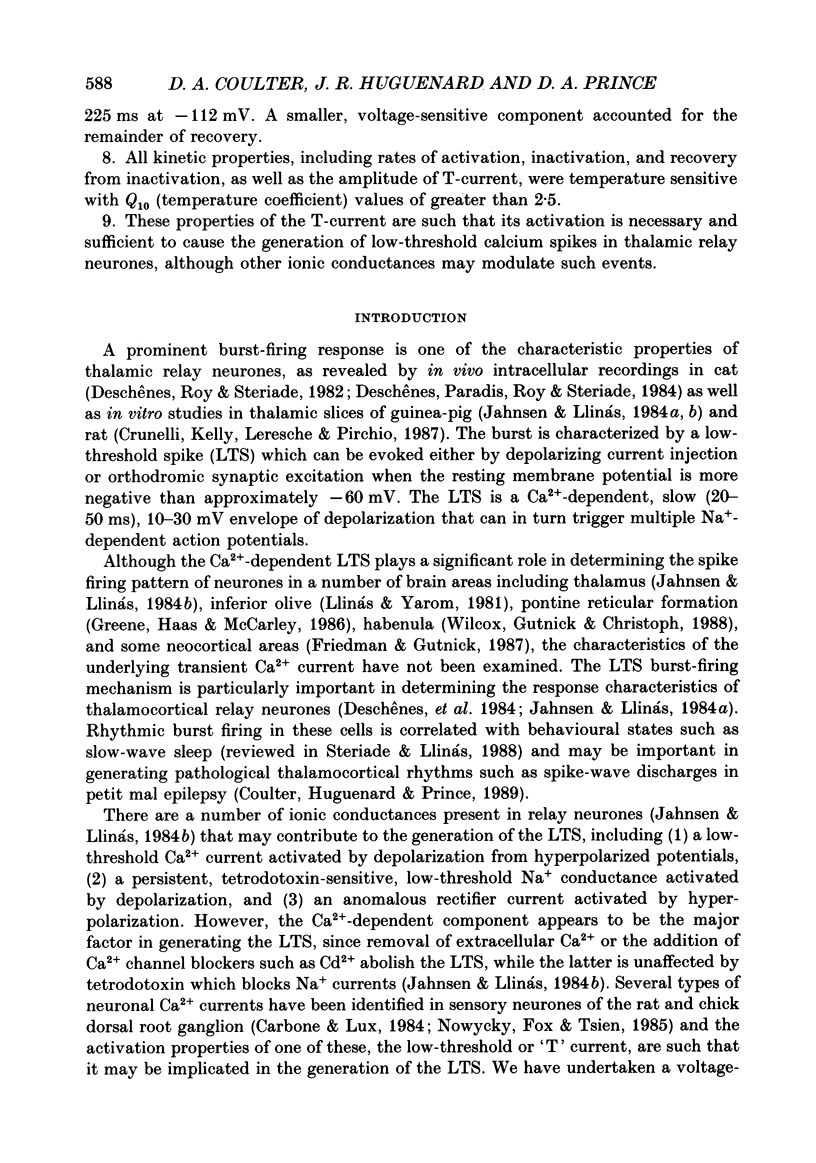
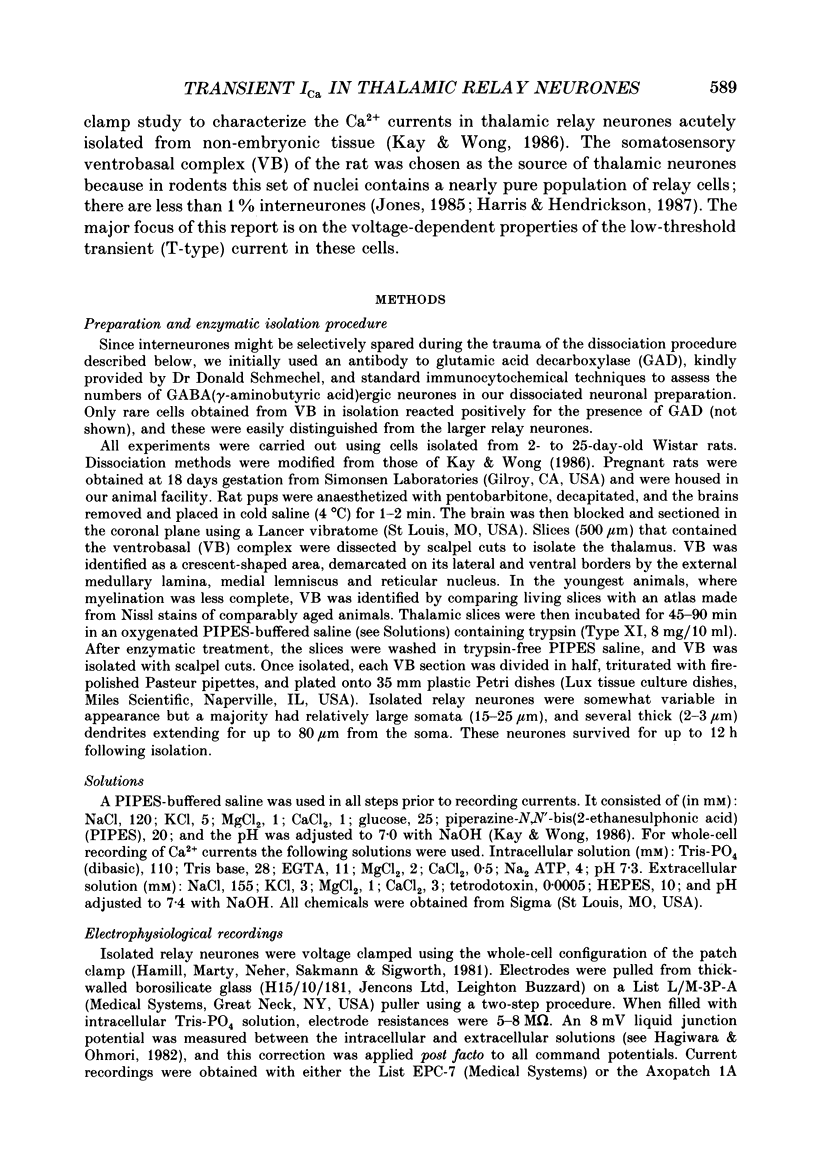
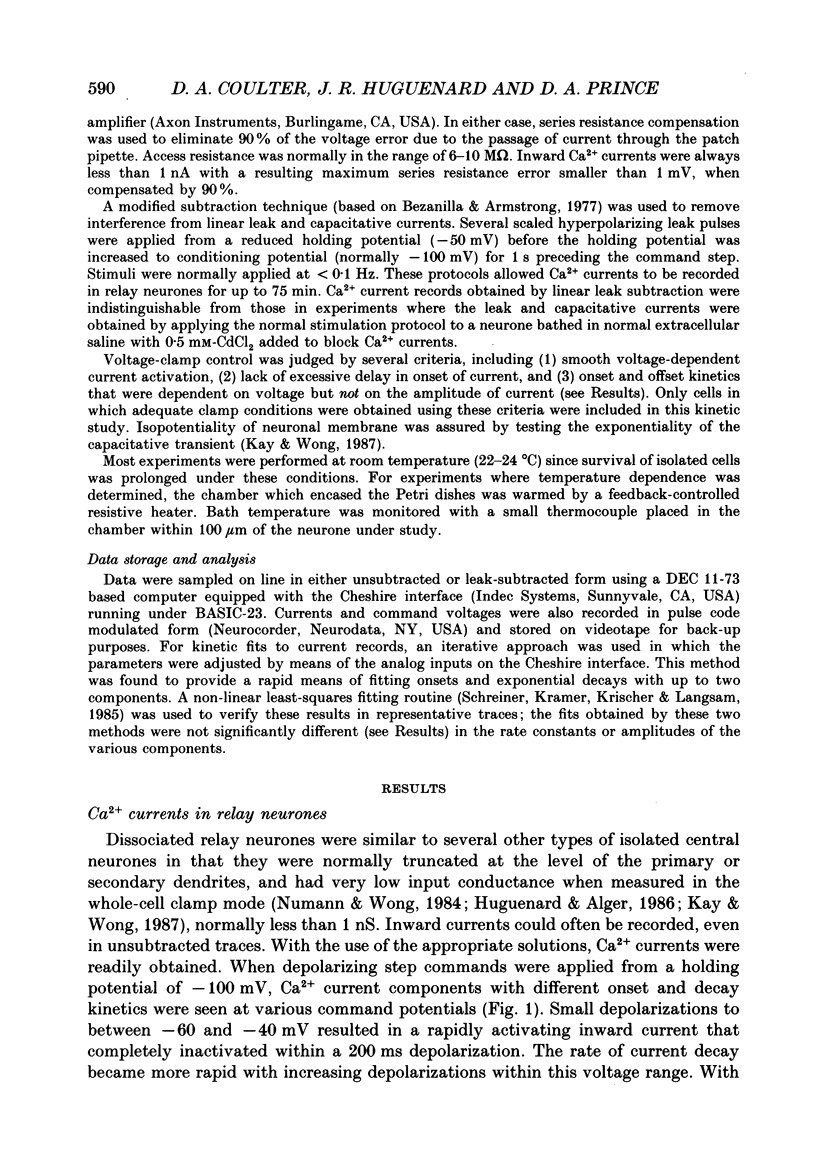
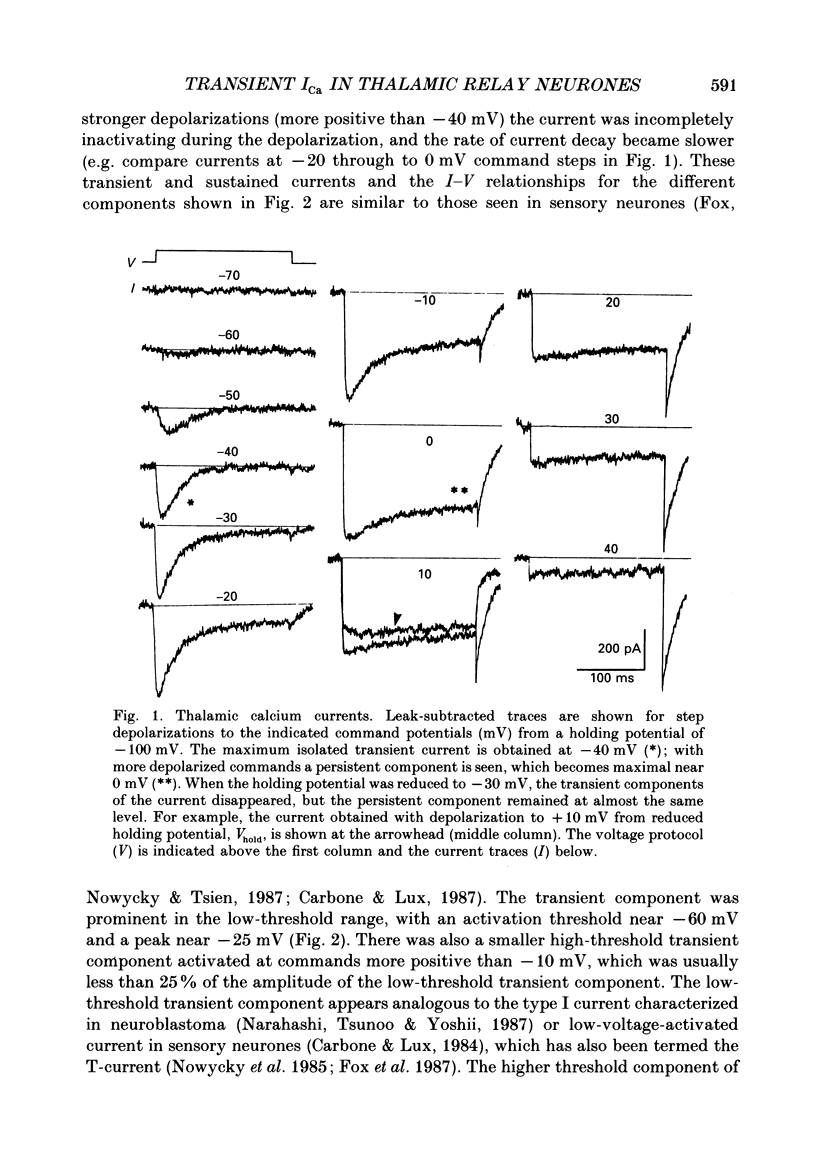
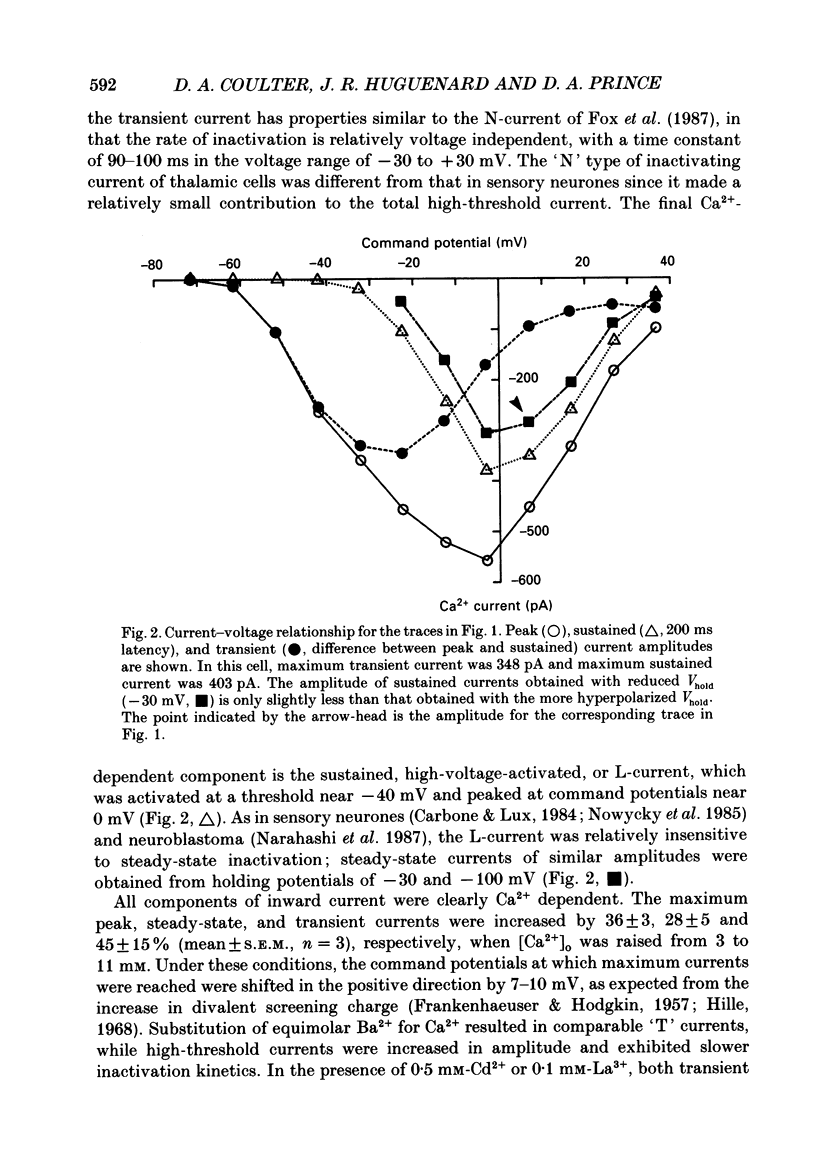
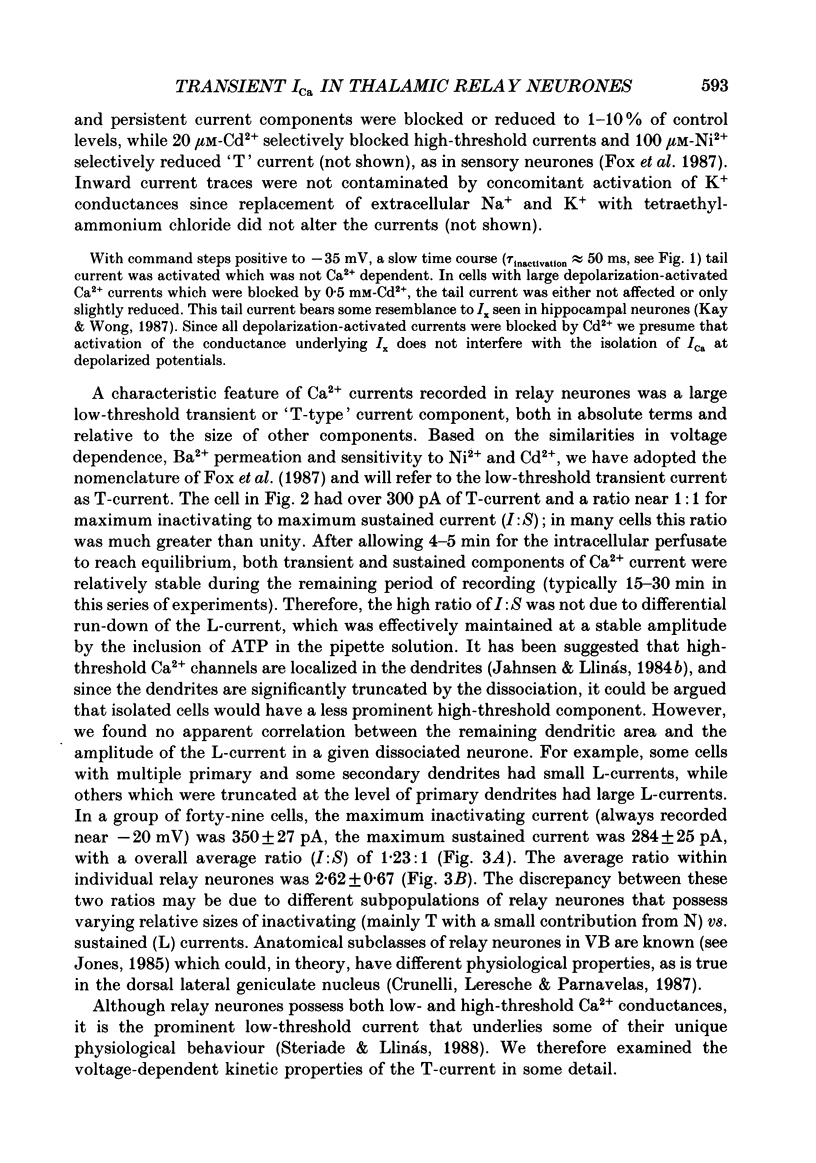
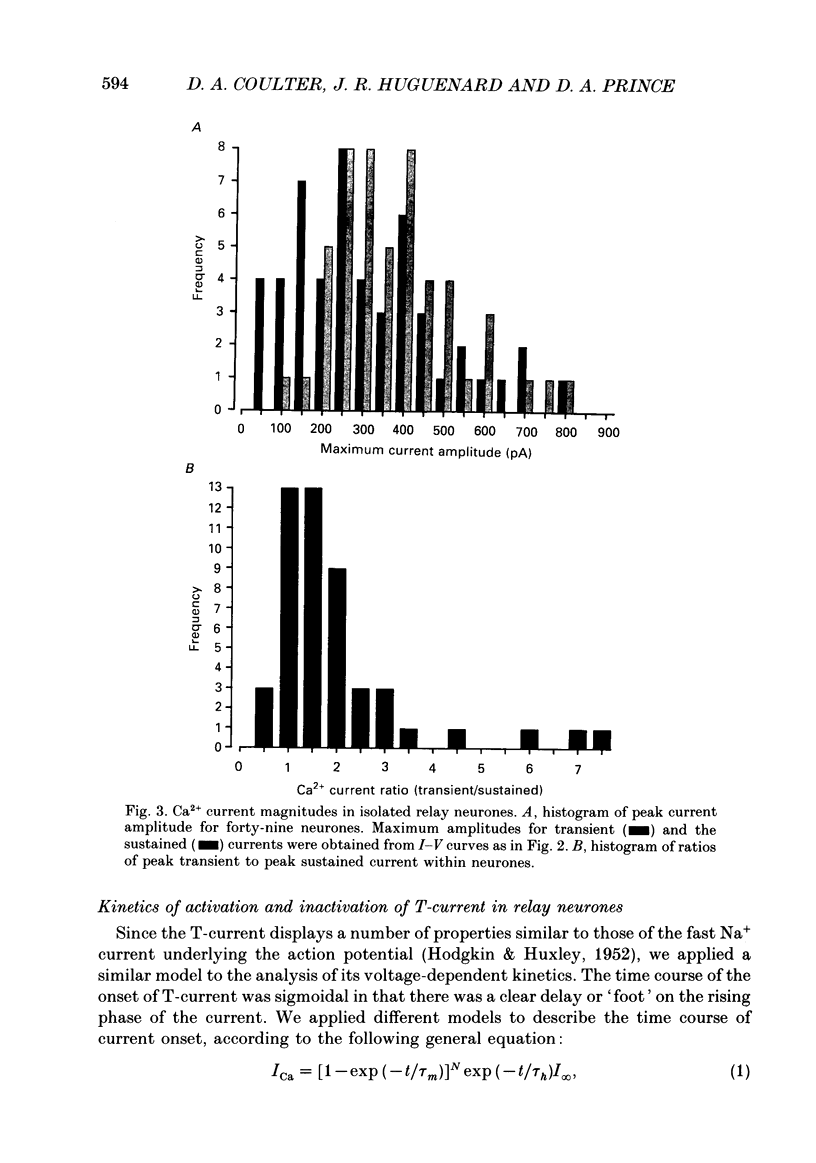
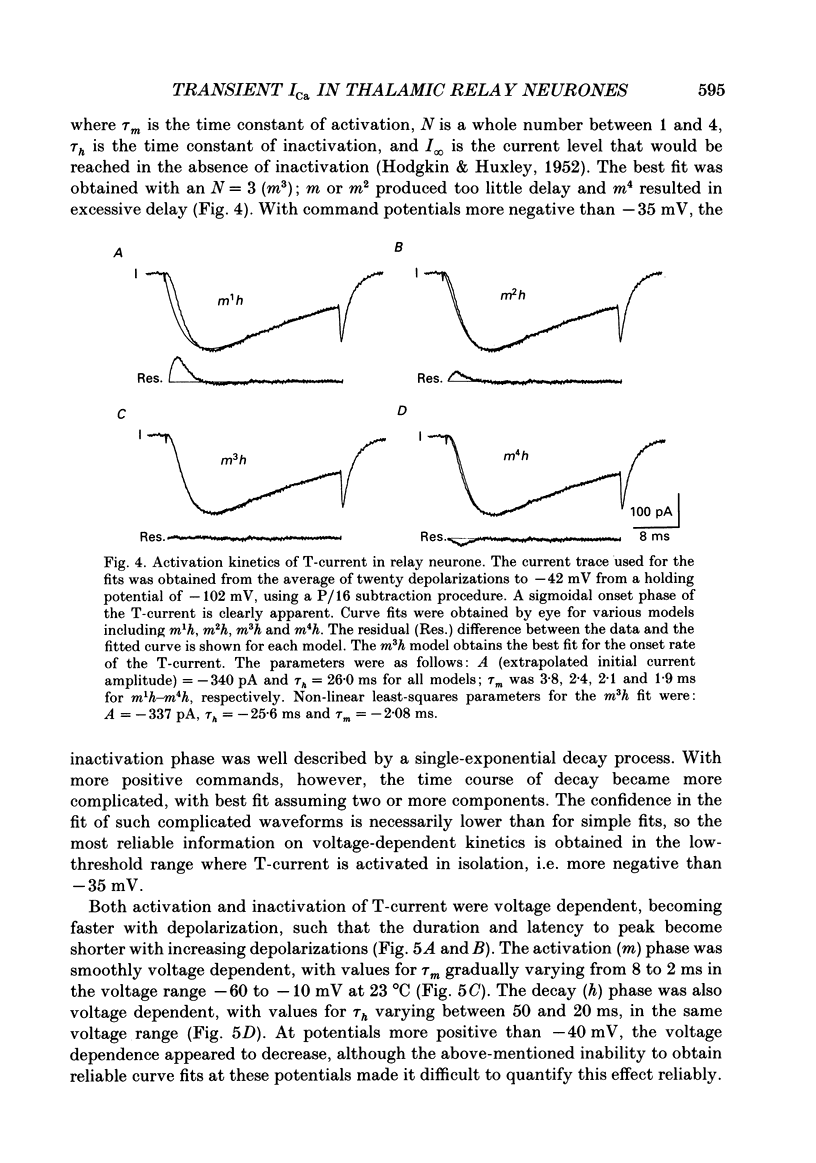
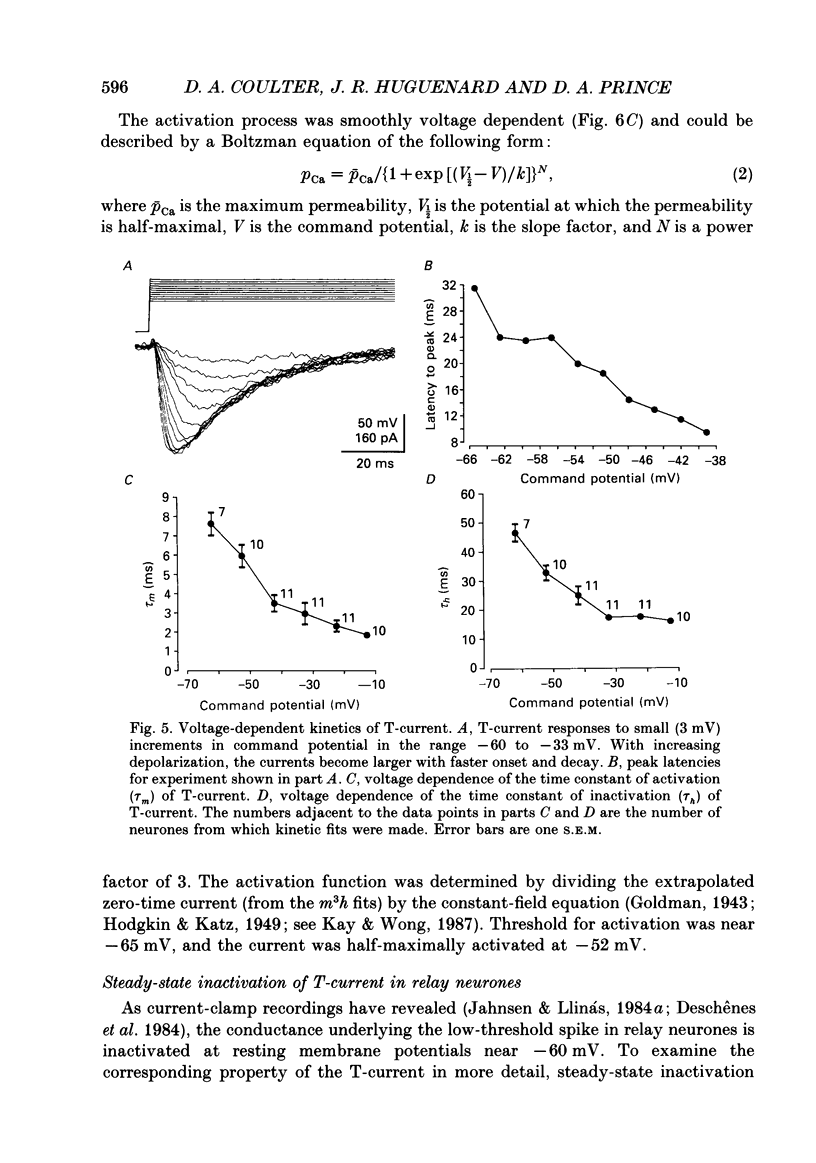
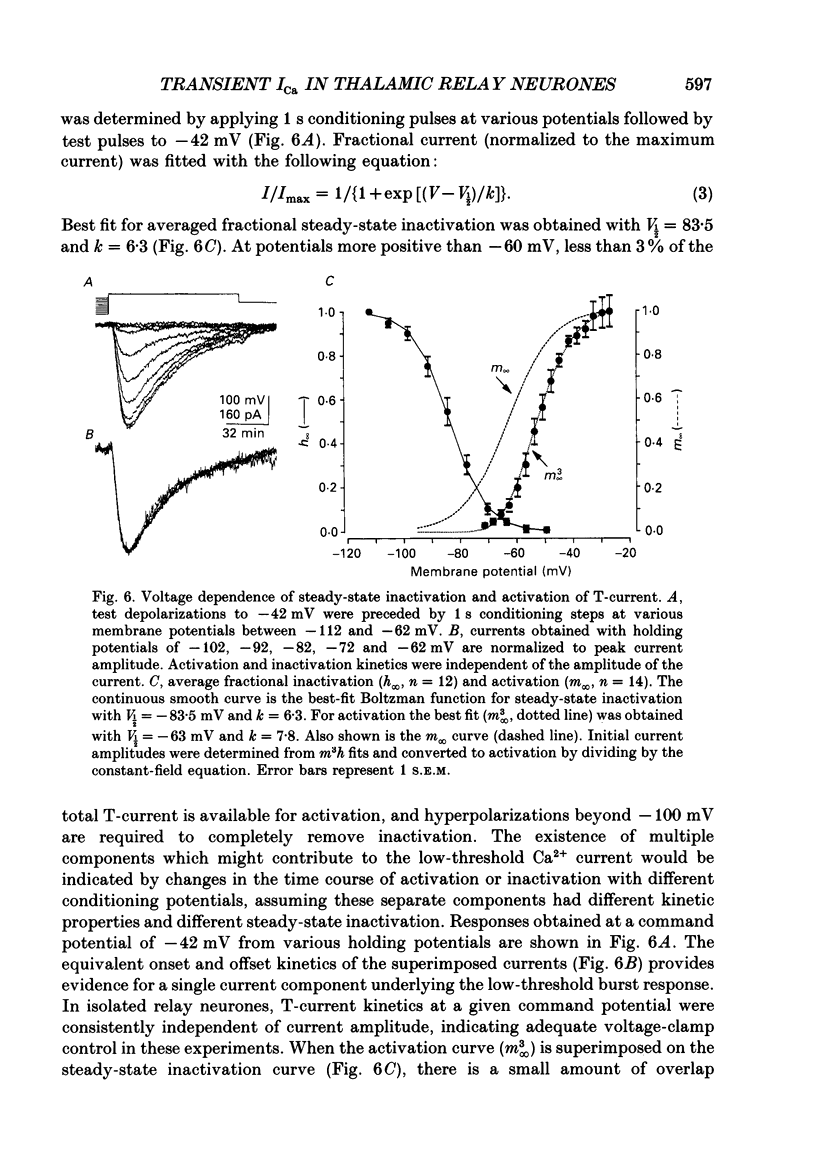
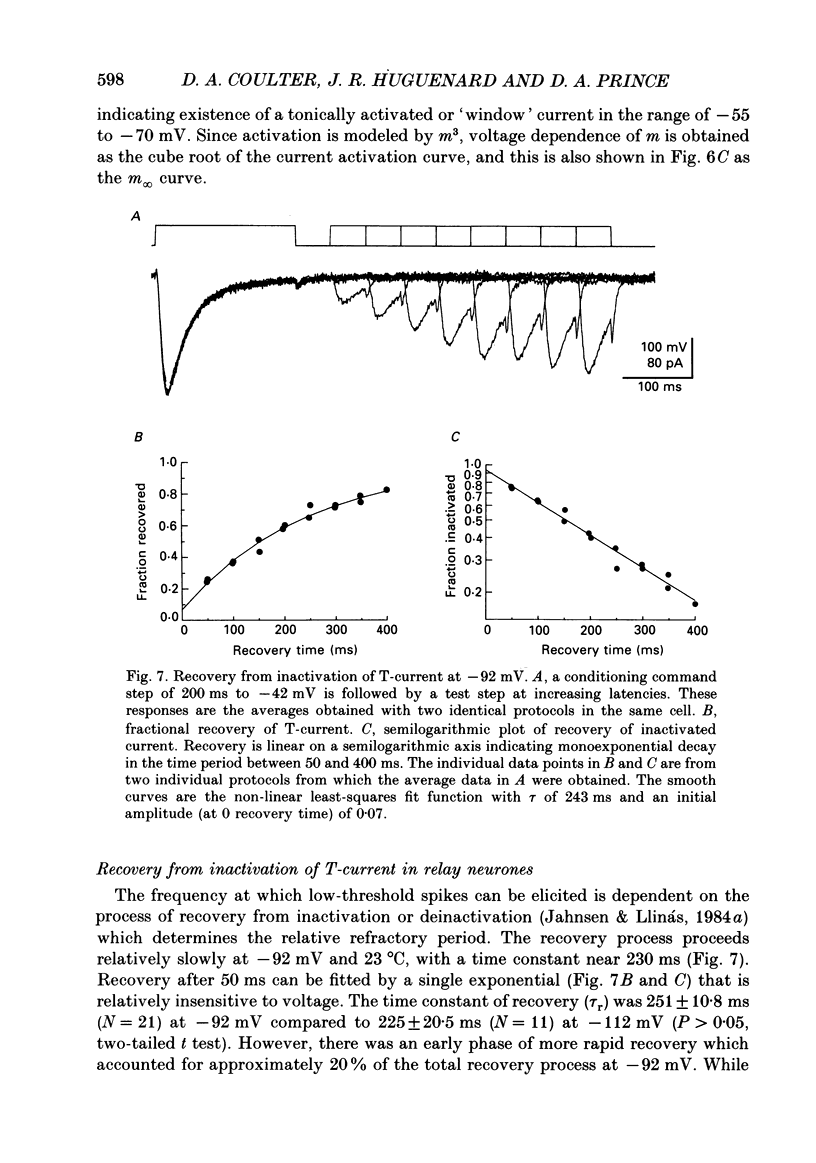
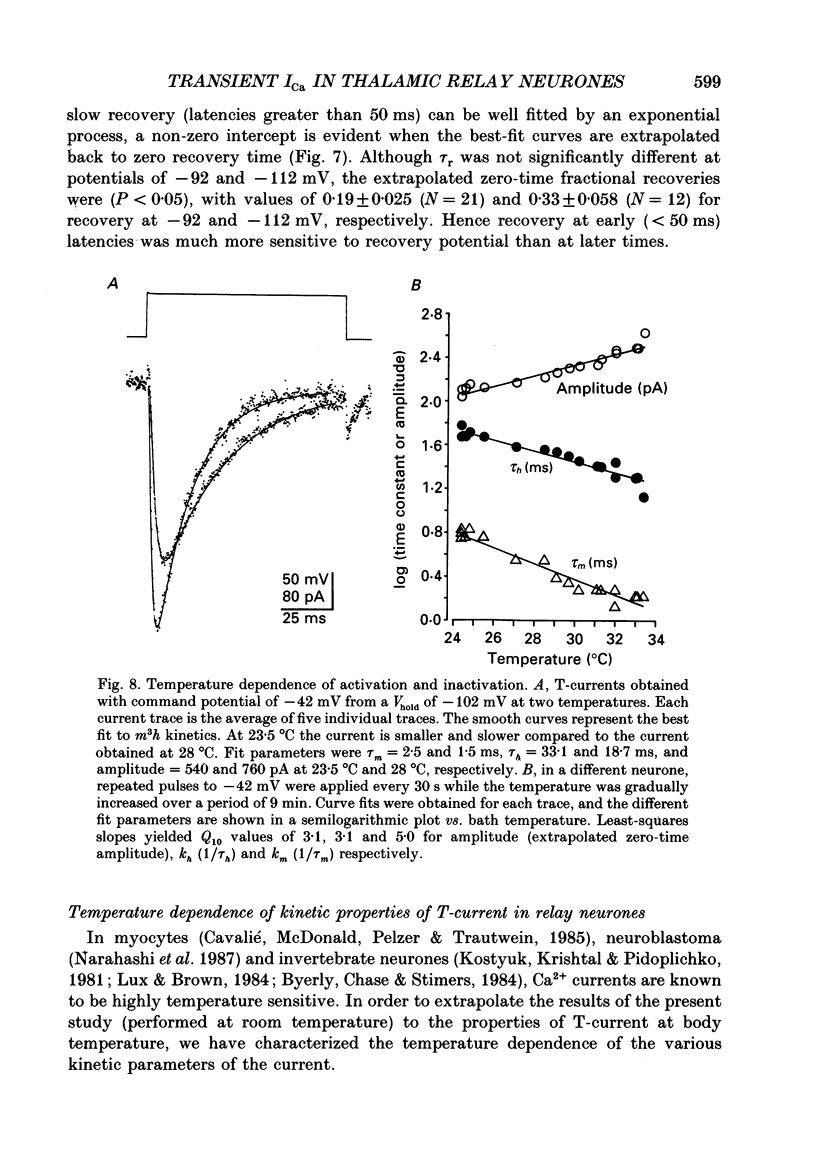
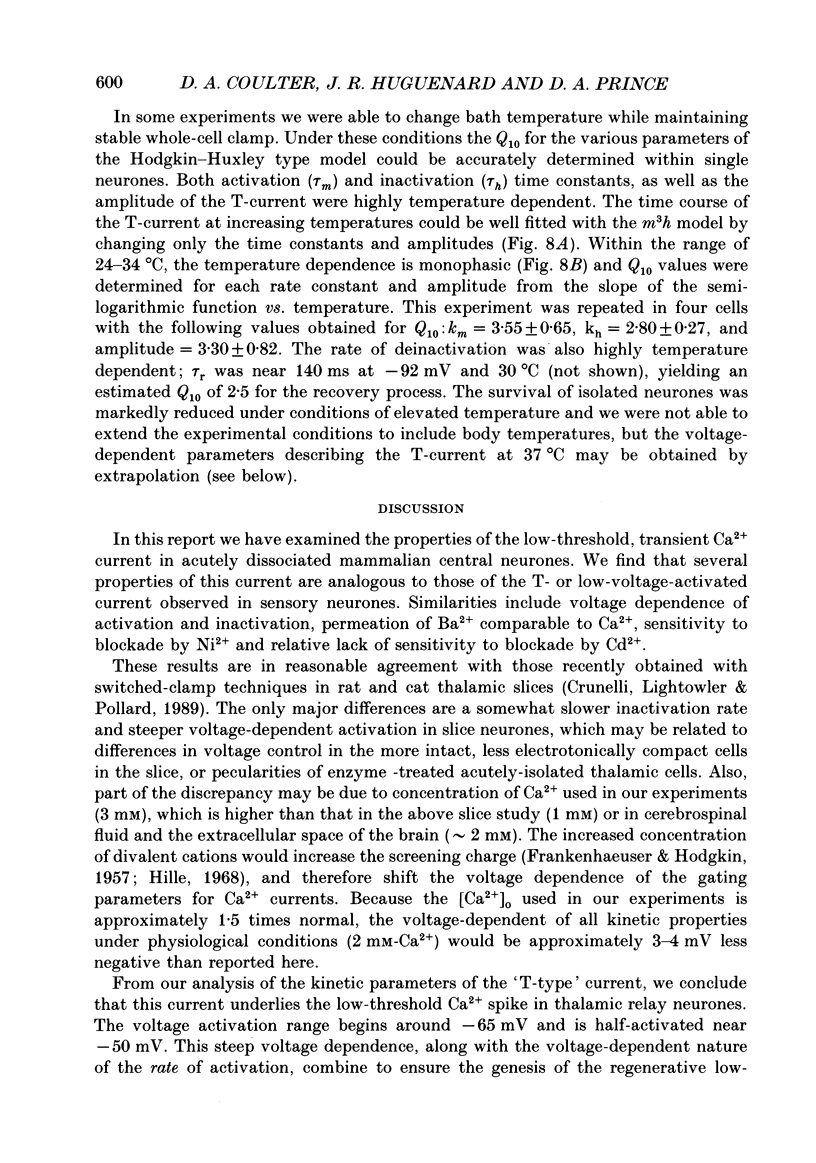
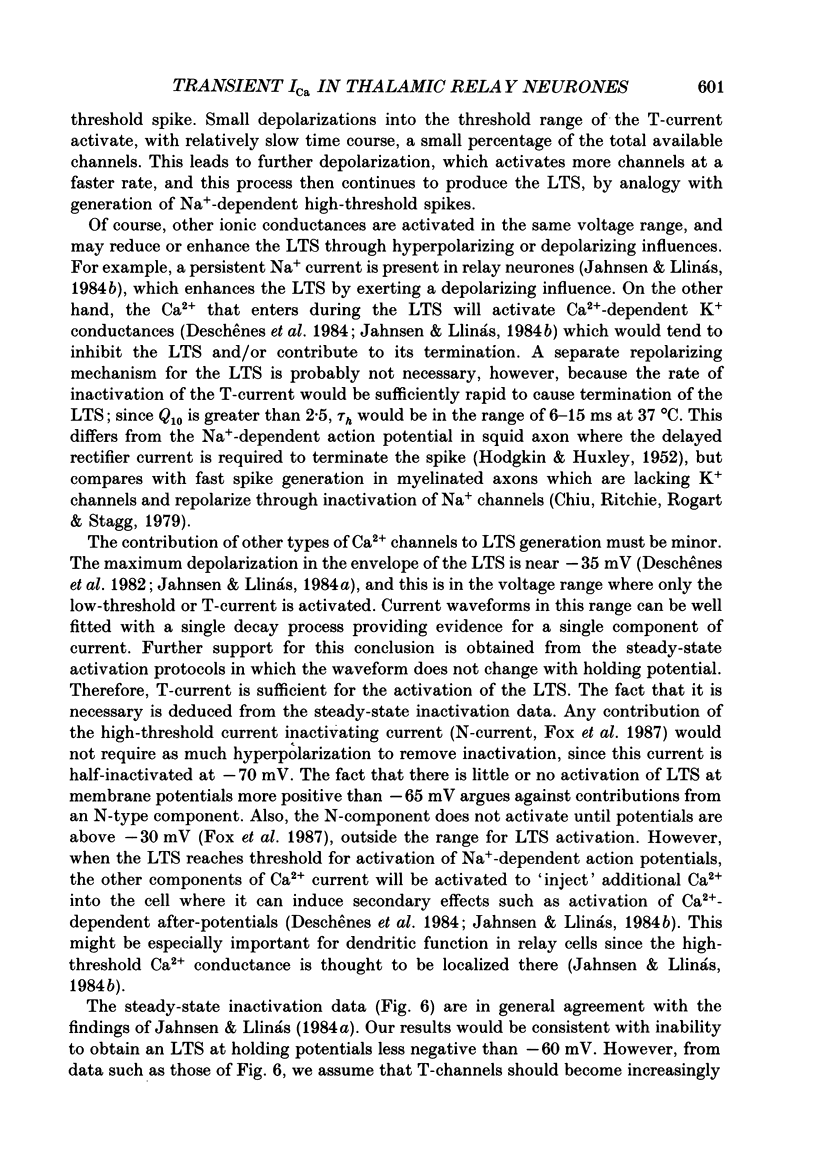
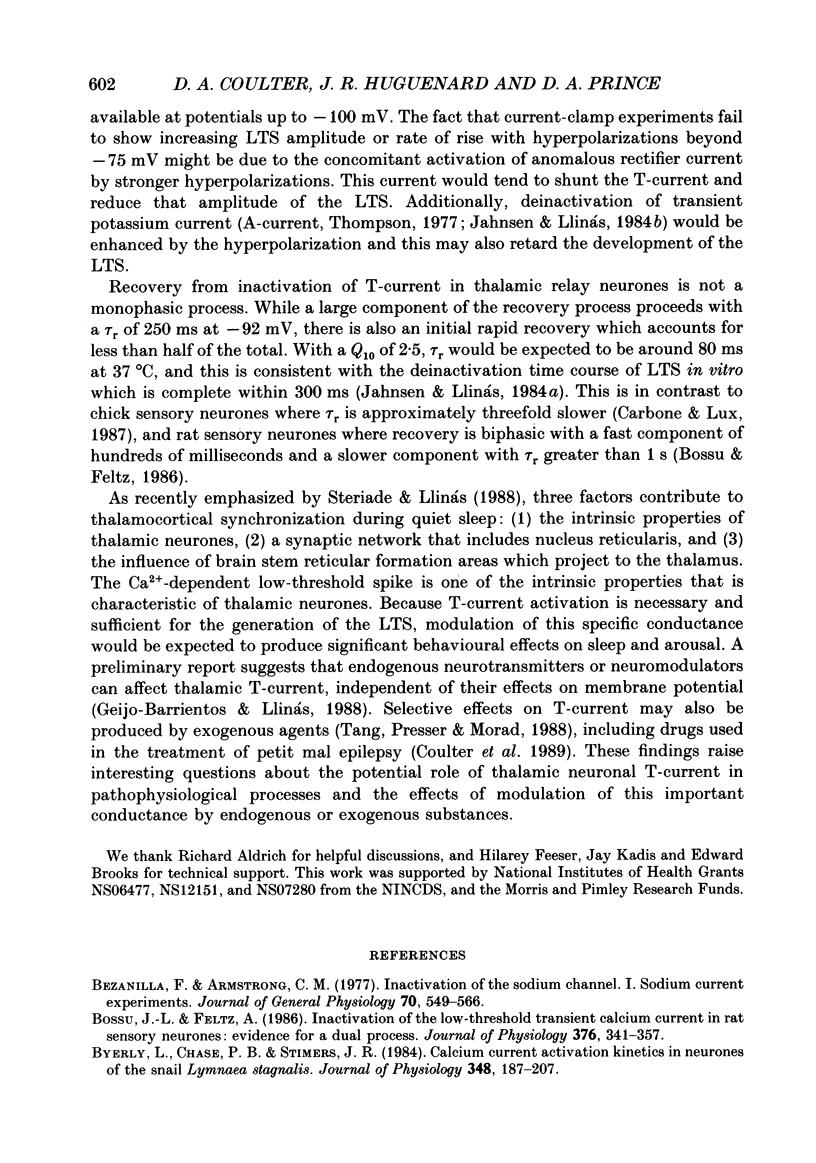
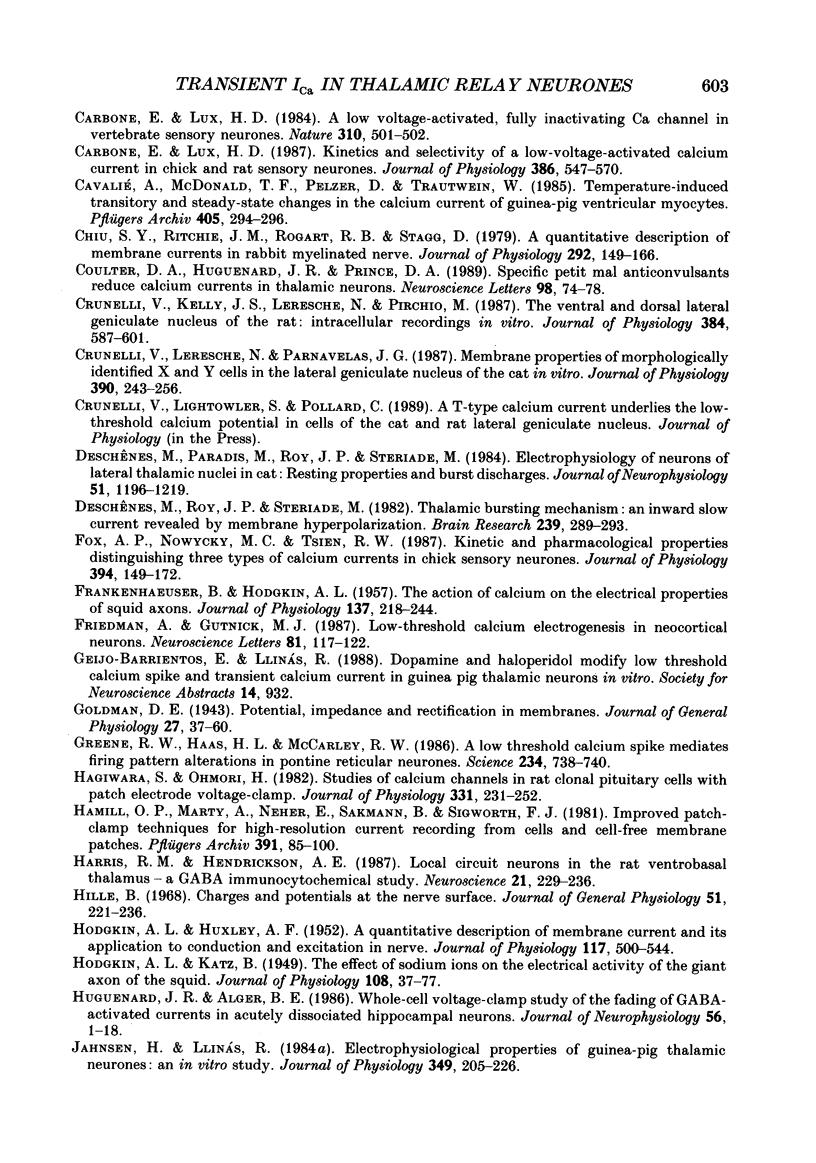
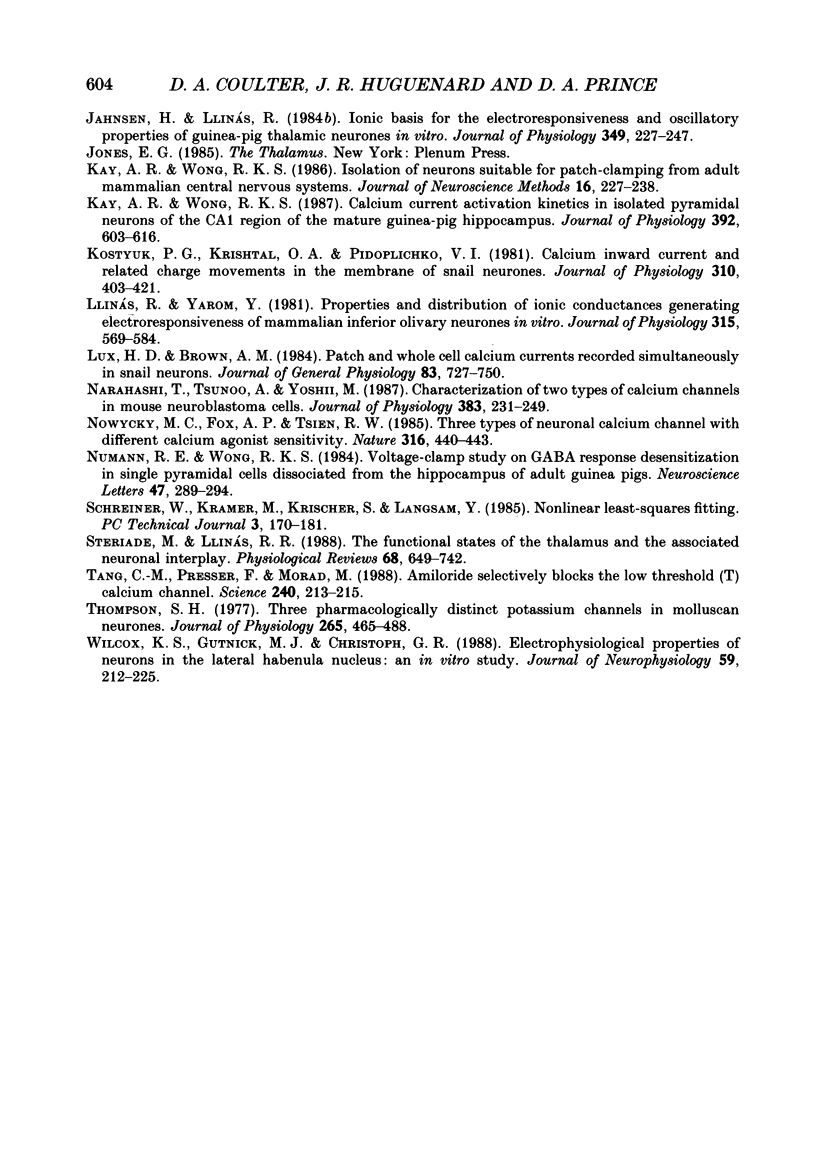
Images in this article
Selected References
These references are in PubMed. This may not be the complete list of references from this article.
- Bezanilla F., Armstrong C. M. Inactivation of the sodium channel. I. Sodium current experiments. J Gen Physiol. 1977 Nov;70(5):549–566. doi: 10.1085/jgp.70.5.549. [DOI] [PMC free article] [PubMed] [Google Scholar]
- Bossu J. L., Feltz A. Inactivation of the low-threshold transient calcium current in rat sensory neurones: evidence for a dual process. J Physiol. 1986 Jul;376:341–357. doi: 10.1113/jphysiol.1986.sp016157. [DOI] [PMC free article] [PubMed] [Google Scholar]
- Byerly L., Chase P. B., Stimers J. R. Calcium current activation kinetics in neurones of the snail Lymnaea stagnalis. J Physiol. 1984 Mar;348:187–207. doi: 10.1113/jphysiol.1984.sp015105. [DOI] [PMC free article] [PubMed] [Google Scholar]
- Carbone E., Lux H. D. A low voltage-activated, fully inactivating Ca channel in vertebrate sensory neurones. Nature. 1984 Aug 9;310(5977):501–502. doi: 10.1038/310501a0. [DOI] [PubMed] [Google Scholar]
- Carbone E., Lux H. D. Kinetics and selectivity of a low-voltage-activated calcium current in chick and rat sensory neurones. J Physiol. 1987 May;386:547–570. doi: 10.1113/jphysiol.1987.sp016551. [DOI] [PMC free article] [PubMed] [Google Scholar]
- Cavalié A., McDonald T. F., Pelzer D., Trautwein W. Temperature-induced transitory and steady-state changes in the calcium current of guinea pig ventricular myocytes. Pflugers Arch. 1985 Oct;405(3):294–296. doi: 10.1007/BF00582574. [DOI] [PubMed] [Google Scholar]
- Chiu S. Y., Ritchie J. M., Rogart R. B., Stagg D. A quantitative description of membrane currents in rabbit myelinated nerve. J Physiol. 1979 Jul;292:149–166. doi: 10.1113/jphysiol.1979.sp012843. [DOI] [PMC free article] [PubMed] [Google Scholar]
- Coulter D. A., Huguenard J. R., Prince D. A. Specific petit mal anticonvulsants reduce calcium currents in thalamic neurons. Neurosci Lett. 1989 Mar 13;98(1):74–78. doi: 10.1016/0304-3940(89)90376-5. [DOI] [PubMed] [Google Scholar]
- Crunelli V., Kelly J. S., Leresche N., Pirchio M. The ventral and dorsal lateral geniculate nucleus of the rat: intracellular recordings in vitro. J Physiol. 1987 Mar;384:587–601. doi: 10.1113/jphysiol.1987.sp016471. [DOI] [PMC free article] [PubMed] [Google Scholar]
- Crunelli V., Leresche N., Parnavelas J. G. Membrane properties of morphologically identified X and Y cells in the lateral geniculate nucleus of the cat in vitro. J Physiol. 1987 Sep;390:243–256. doi: 10.1113/jphysiol.1987.sp016697. [DOI] [PMC free article] [PubMed] [Google Scholar]
- Deschênes M., Paradis M., Roy J. P., Steriade M. Electrophysiology of neurons of lateral thalamic nuclei in cat: resting properties and burst discharges. J Neurophysiol. 1984 Jun;51(6):1196–1219. doi: 10.1152/jn.1984.51.6.1196. [DOI] [PubMed] [Google Scholar]
- Deschênes M., Roy J. P., Steriade M. Thalamic bursting mechanism: an inward slow current revealed by membrane hyperpolarization. Brain Res. 1982 May 6;239(1):289–293. doi: 10.1016/0006-8993(82)90854-x. [DOI] [PubMed] [Google Scholar]
- FRANKENHAEUSER B., HODGKIN A. L. The action of calcium on the electrical properties of squid axons. J Physiol. 1957 Jul 11;137(2):218–244. doi: 10.1113/jphysiol.1957.sp005808. [DOI] [PMC free article] [PubMed] [Google Scholar]
- Fox A. P., Nowycky M. C., Tsien R. W. Kinetic and pharmacological properties distinguishing three types of calcium currents in chick sensory neurones. J Physiol. 1987 Dec;394:149–172. doi: 10.1113/jphysiol.1987.sp016864. [DOI] [PMC free article] [PubMed] [Google Scholar]
- Friedman A., Gutnick M. J. Low-threshold calcium electrogenesis in neocortical neurons. Neurosci Lett. 1987 Oct 16;81(1-2):117–122. doi: 10.1016/0304-3940(87)90350-8. [DOI] [PubMed] [Google Scholar]
- Goldman D. E. POTENTIAL, IMPEDANCE, AND RECTIFICATION IN MEMBRANES. J Gen Physiol. 1943 Sep 20;27(1):37–60. doi: 10.1085/jgp.27.1.37. [DOI] [PMC free article] [PubMed] [Google Scholar]
- Greene R. W., Haas H. L., McCarley R. W. A low threshold calcium spike mediates firing pattern alterations in pontine reticular neurons. Science. 1986 Nov 7;234(4777):738–740. doi: 10.1126/science.3775364. [DOI] [PubMed] [Google Scholar]
- HODGKIN A. L., HUXLEY A. F. A quantitative description of membrane current and its application to conduction and excitation in nerve. J Physiol. 1952 Aug;117(4):500–544. doi: 10.1113/jphysiol.1952.sp004764. [DOI] [PMC free article] [PubMed] [Google Scholar]
- HODGKIN A. L., KATZ B. The effect of sodium ions on the electrical activity of giant axon of the squid. J Physiol. 1949 Mar 1;108(1):37–77. doi: 10.1113/jphysiol.1949.sp004310. [DOI] [PMC free article] [PubMed] [Google Scholar]
- Hagiwara S., Ohmori H. Studies of calcium channels in rat clonal pituitary cells with patch electrode voltage clamp. J Physiol. 1982 Oct;331:231–252. doi: 10.1113/jphysiol.1982.sp014371. [DOI] [PMC free article] [PubMed] [Google Scholar]
- Hamill O. P., Marty A., Neher E., Sakmann B., Sigworth F. J. Improved patch-clamp techniques for high-resolution current recording from cells and cell-free membrane patches. Pflugers Arch. 1981 Aug;391(2):85–100. doi: 10.1007/BF00656997. [DOI] [PubMed] [Google Scholar]
- Harris R. M., Hendrickson A. E. Local circuit neurons in the rat ventrobasal thalamus--a GABA immunocytochemical study. Neuroscience. 1987 Apr;21(1):229–236. doi: 10.1016/0306-4522(87)90335-6. [DOI] [PubMed] [Google Scholar]
- Hille B. Charges and potentials at the nerve surface. Divalent ions and pH. J Gen Physiol. 1968 Feb;51(2):221–236. doi: 10.1085/jgp.51.2.221. [DOI] [PMC free article] [PubMed] [Google Scholar]
- Huguenard J. R., Alger B. E. Whole-cell voltage-clamp study of the fading of GABA-activated currents in acutely dissociated hippocampal neurons. J Neurophysiol. 1986 Jul;56(1):1–18. doi: 10.1152/jn.1986.56.1.1. [DOI] [PubMed] [Google Scholar]
- Jahnsen H., Llinás R. Electrophysiological properties of guinea-pig thalamic neurones: an in vitro study. J Physiol. 1984 Apr;349:205–226. doi: 10.1113/jphysiol.1984.sp015153. [DOI] [PMC free article] [PubMed] [Google Scholar]
- Jahnsen H., Llinás R. Ionic basis for the electro-responsiveness and oscillatory properties of guinea-pig thalamic neurones in vitro. J Physiol. 1984 Apr;349:227–247. doi: 10.1113/jphysiol.1984.sp015154. [DOI] [PMC free article] [PubMed] [Google Scholar]
- Kay A. R., Wong R. K. Calcium current activation kinetics in isolated pyramidal neurones of the Ca1 region of the mature guinea-pig hippocampus. J Physiol. 1987 Nov;392:603–616. doi: 10.1113/jphysiol.1987.sp016799. [DOI] [PMC free article] [PubMed] [Google Scholar]
- Kay A. R., Wong R. K. Isolation of neurons suitable for patch-clamping from adult mammalian central nervous systems. J Neurosci Methods. 1986 May;16(3):227–238. doi: 10.1016/0165-0270(86)90040-3. [DOI] [PubMed] [Google Scholar]
- Kostyuk P. G., Krishtal O. A., Pidoplichko V. I. Calcium inward current and related charge movements in the membrane of snail neurones. J Physiol. 1981 Jan;310:403–421. doi: 10.1113/jphysiol.1981.sp013557. [DOI] [PMC free article] [PubMed] [Google Scholar]
- Llinás R., Yarom Y. Properties and distribution of ionic conductances generating electroresponsiveness of mammalian inferior olivary neurones in vitro. J Physiol. 1981 Jun;315:569–584. doi: 10.1113/jphysiol.1981.sp013764. [DOI] [PMC free article] [PubMed] [Google Scholar]
- Lux H. D., Brown A. M. Patch and whole cell calcium currents recorded simultaneously in snail neurons. J Gen Physiol. 1984 May;83(5):727–750. doi: 10.1085/jgp.83.5.727. [DOI] [PMC free article] [PubMed] [Google Scholar]
- Narahashi T., Tsunoo A., Yoshii M. Characterization of two types of calcium channels in mouse neuroblastoma cells. J Physiol. 1987 Feb;383:231–249. doi: 10.1113/jphysiol.1987.sp016406. [DOI] [PMC free article] [PubMed] [Google Scholar]
- Nowycky M. C., Fox A. P., Tsien R. W. Three types of neuronal calcium channel with different calcium agonist sensitivity. Nature. 1985 Aug 1;316(6027):440–443. doi: 10.1038/316440a0. [DOI] [PubMed] [Google Scholar]
- Numann R. E., Wong R. K. Voltage-clamp study on GABA response desensitization in single pyramidal cells dissociated from the hippocampus of adult guinea pigs. Neurosci Lett. 1984 Jun 29;47(3):289–294. doi: 10.1016/0304-3940(84)90528-7. [DOI] [PubMed] [Google Scholar]
- Steriade M., Llinás R. R. The functional states of the thalamus and the associated neuronal interplay. Physiol Rev. 1988 Jul;68(3):649–742. doi: 10.1152/physrev.1988.68.3.649. [DOI] [PubMed] [Google Scholar]
- Tang C. M., Presser F., Morad M. Amiloride selectively blocks the low threshold (T) calcium channel. Science. 1988 Apr 8;240(4849):213–215. doi: 10.1126/science.2451291. [DOI] [PubMed] [Google Scholar]
- Thompson S. H. Three pharmacologically distinct potassium channels in molluscan neurones. J Physiol. 1977 Feb;265(2):465–488. doi: 10.1113/jphysiol.1977.sp011725. [DOI] [PMC free article] [PubMed] [Google Scholar]
- Wilcox K. S., Gutnick M. J., Christoph G. R. Electrophysiological properties of neurons in the lateral habenula nucleus: an in vitro study. J Neurophysiol. 1988 Jan;59(1):212–225. doi: 10.1152/jn.1988.59.1.212. [DOI] [PubMed] [Google Scholar]