Abstract
1. Non-linear capacitative current (charge movement) was studied in isolated guinea-pig and rat ventricular myocytes. Linear capacitance was subtracted using standard procedures. Most of the experiments were done with guinea-pig myocytes, while rat myocytes were used for comparison. 2. When a myocyte was held at -100 mV, depolarizing clamp steps produced a rapid outward current transient, which was followed by an inward current transient upon repolarization. This current was identified as the movement of charged particles in the cell membrane, rather than ionic movement across the membrane, for the following reasons: (1) the current saturated at membrane potentials positive to +20 mV; (2) the current was capacitative in nature, having no reversal potential; (3) in general, the charge moved during depolarization (Qon) approximated the charge moved during repolarization (Qoff). 3. Qoff was significantly less than Qon for a depolarization from -100 mV to 0 mV. However, the Qoff/Qon ratio approached unity if the cell was instead repolarized to -140 mV. This was interpreted as being due to the immobilization of a fraction of the charge during the depolarization, which recovered rapidly enough to be measured at -140 mV, but recovered too slowly at -100 mV. 4. Charge movement in these cells had a sigmoidal dependence on the membrane potential, which could be empirically described by the two-state Boltzmann equation Q = Qmax/(1 + exp[-(V-V*)/kappa]), where Q is the charge movement at potential V, Qmax is the maximum charge, V* is the membrane potential at Q = Qmax/2, and kappa is a slope factor. Qmax was 11.7 nC/microF, V* was -18 mV and kappa was 16 mV in guinea-pig myocytes held at -100 mV, while the values in rat myocytes were 10.9 nC/microF, -32 mV and 13 mV. 5. The charge movement could be partially immobilized by a prior depolarization. This effect developed over a broad voltage range, from -120 to +20 mV. The fraction of charge that could be immobilized by a 10 s pre-pulse to +20 mV was 59%. 6. The time course of decay of both Qon and Qoff could basically be described as a single-exponential process. The time constant was largest at -40 mV and decreased at both more positive and negative test potentials. A second, slower Qoff time constant, possibly representing remobilization of immobilized charge, could be seen under some conditions. 7. The temperature dependence of charge movement was studied between 11 and 35 degrees C.(ABSTRACT TRUNCATED AT 400 WORDS)
Full text
PDF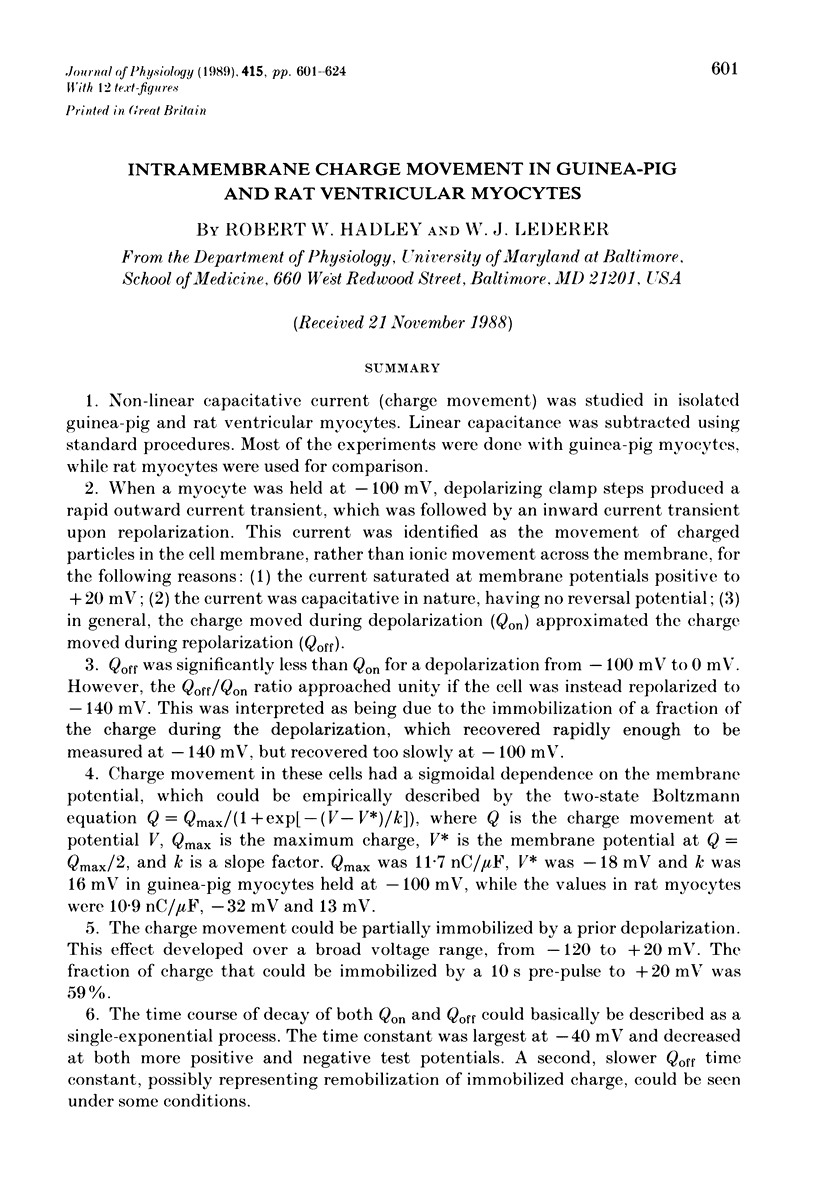
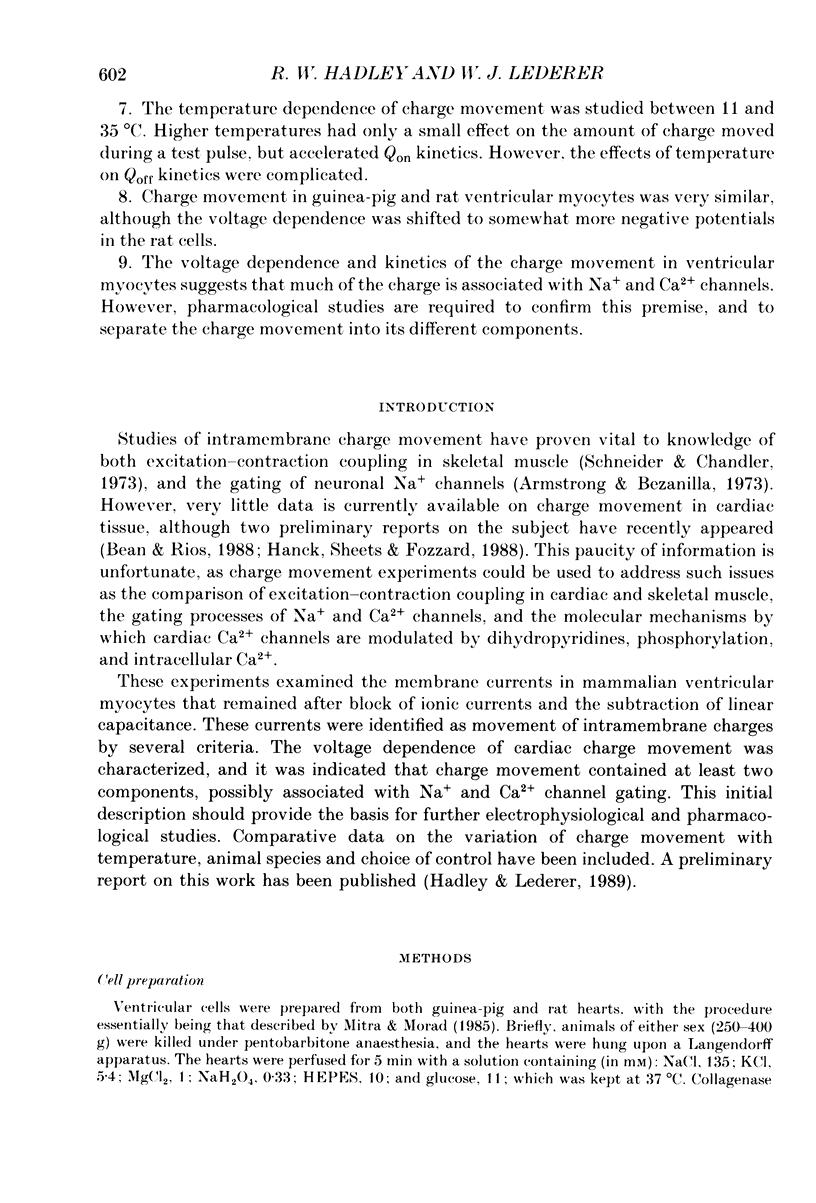
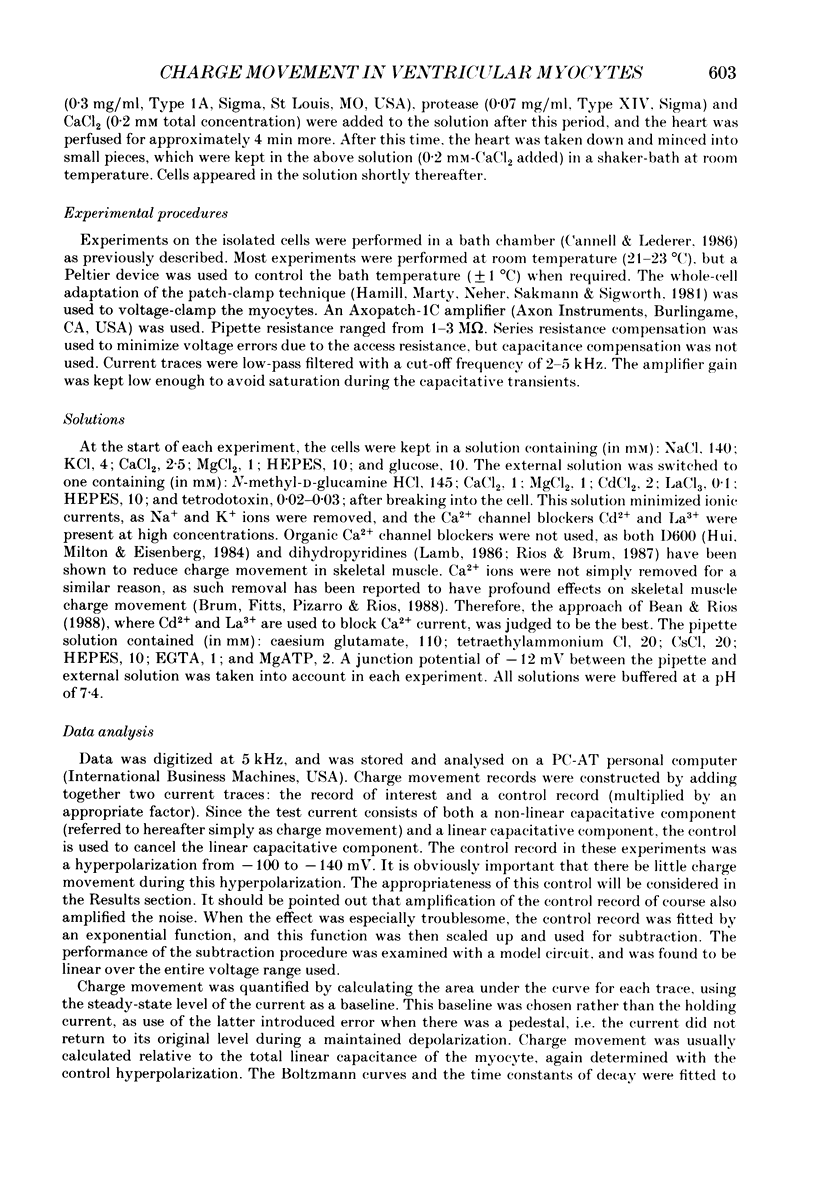
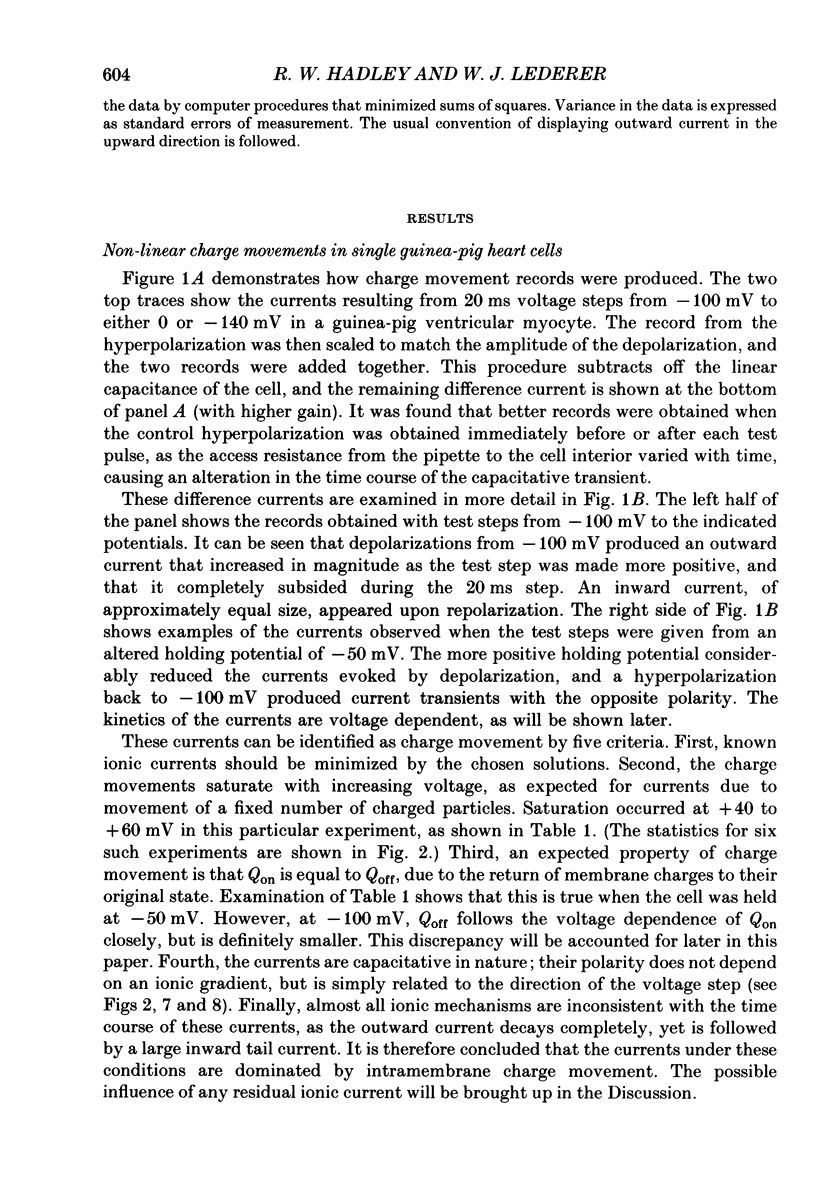
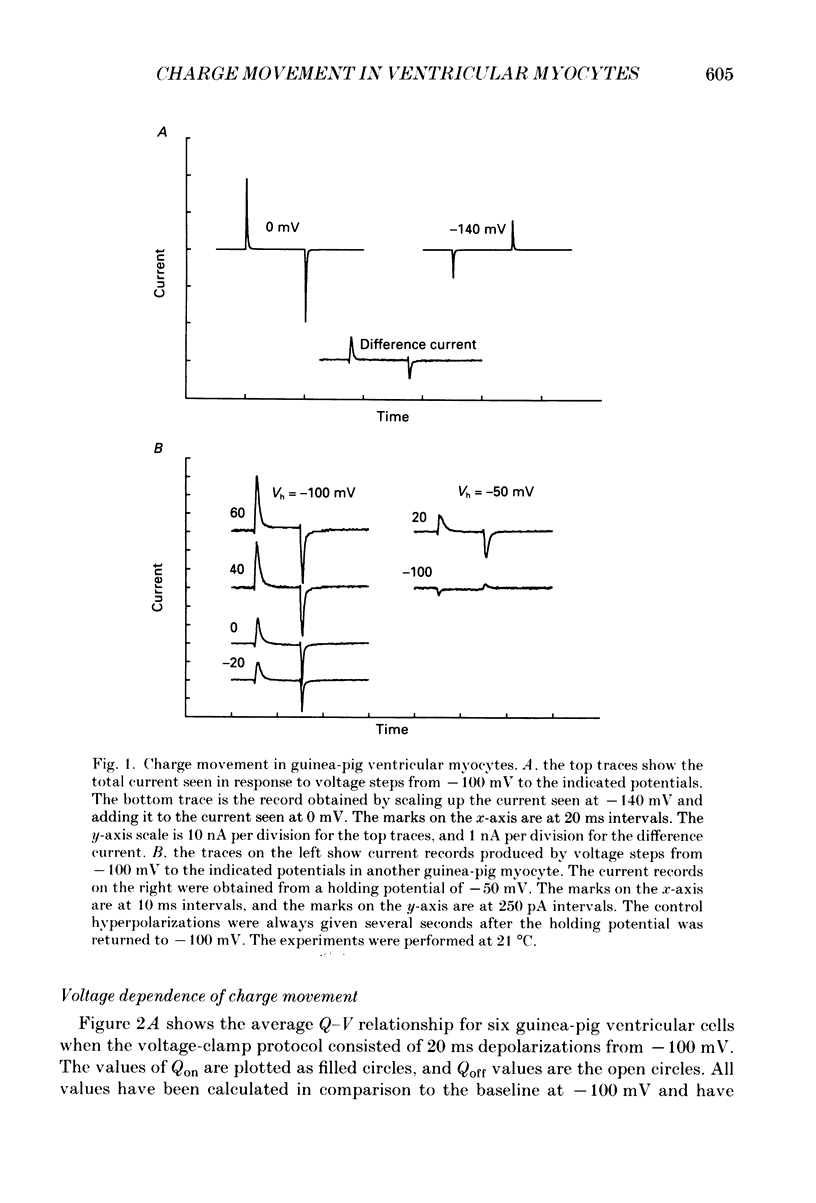
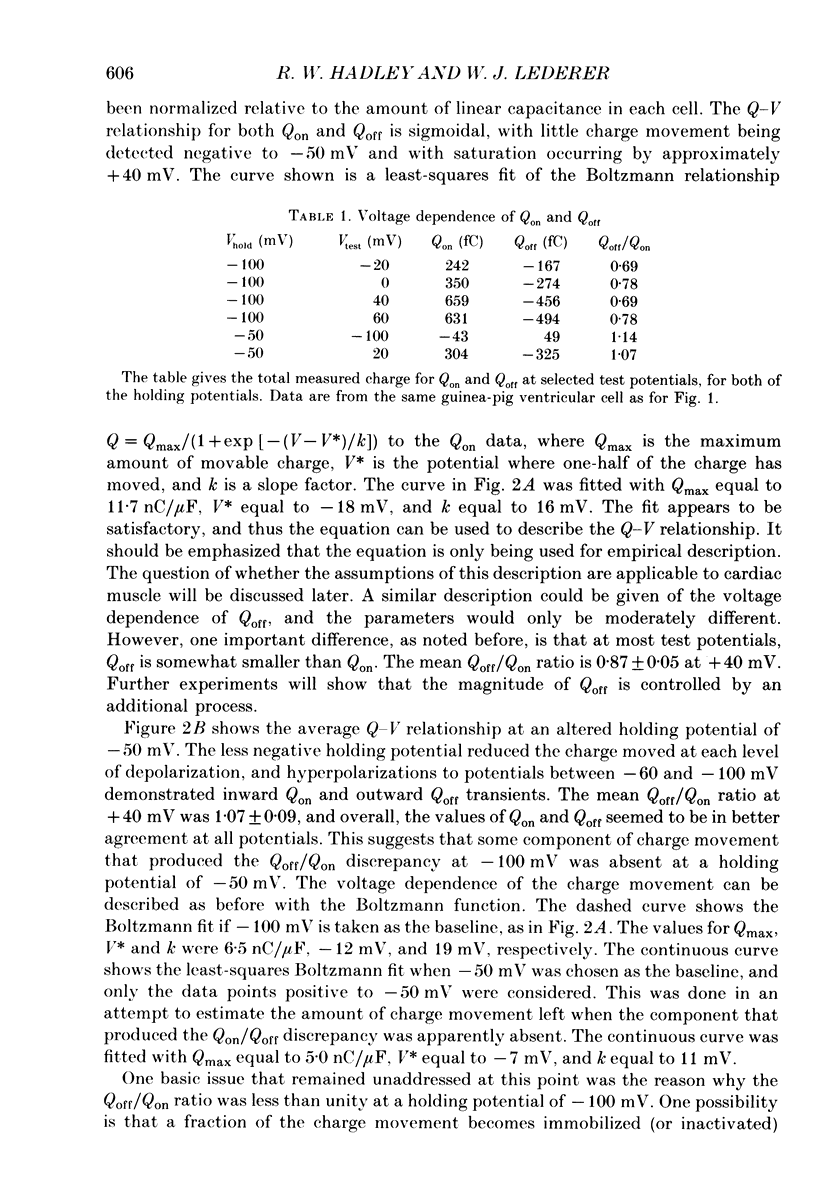
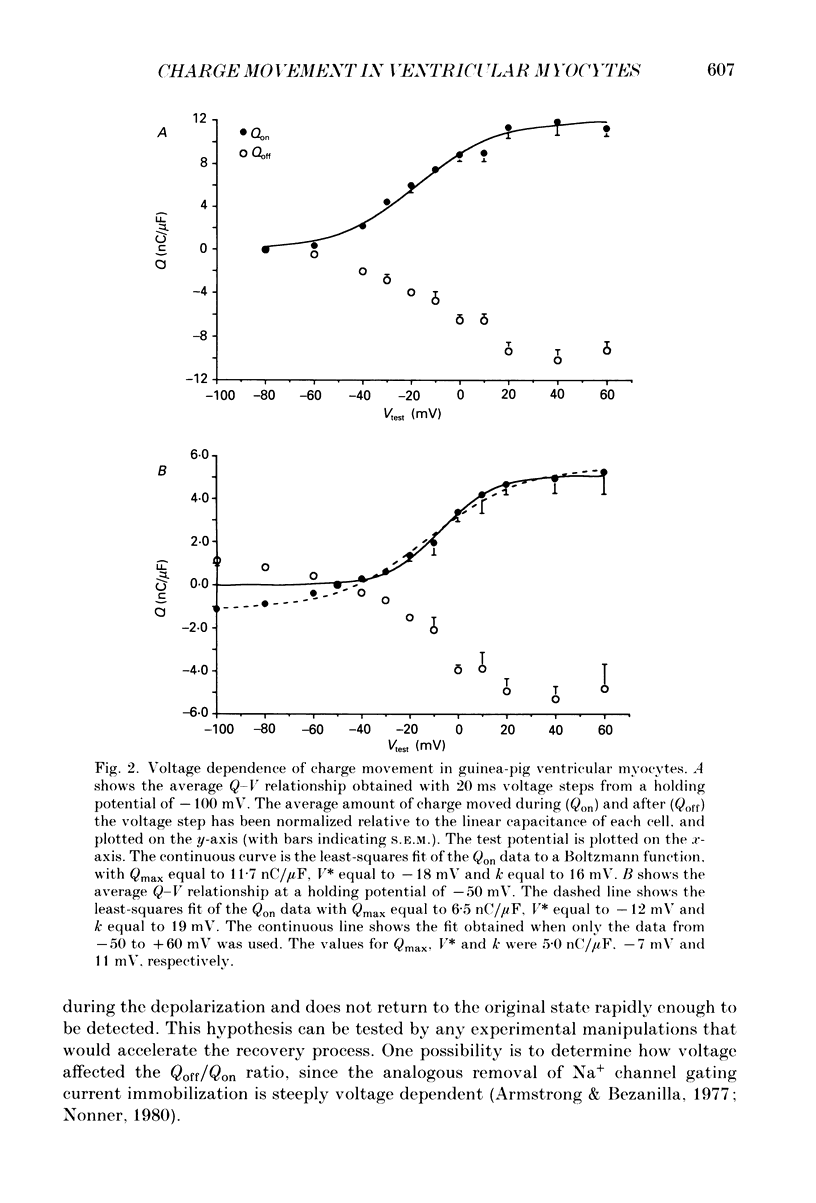
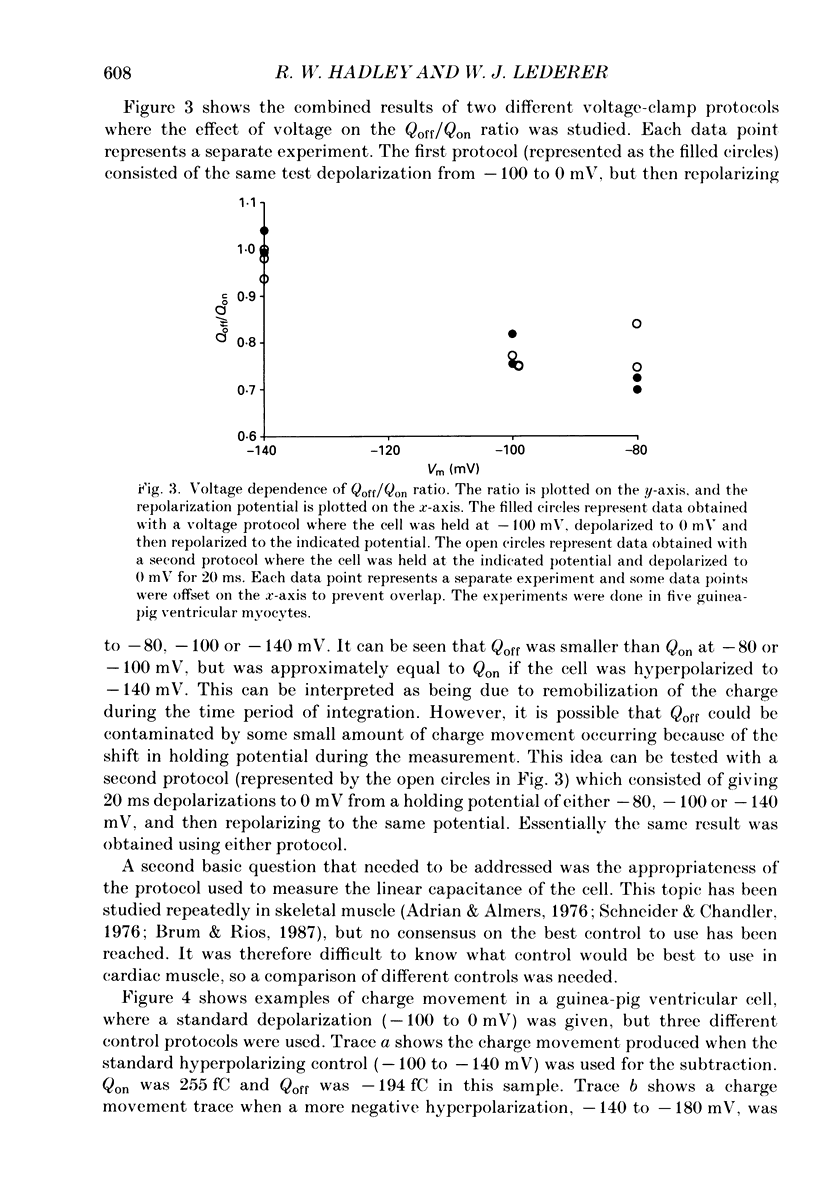
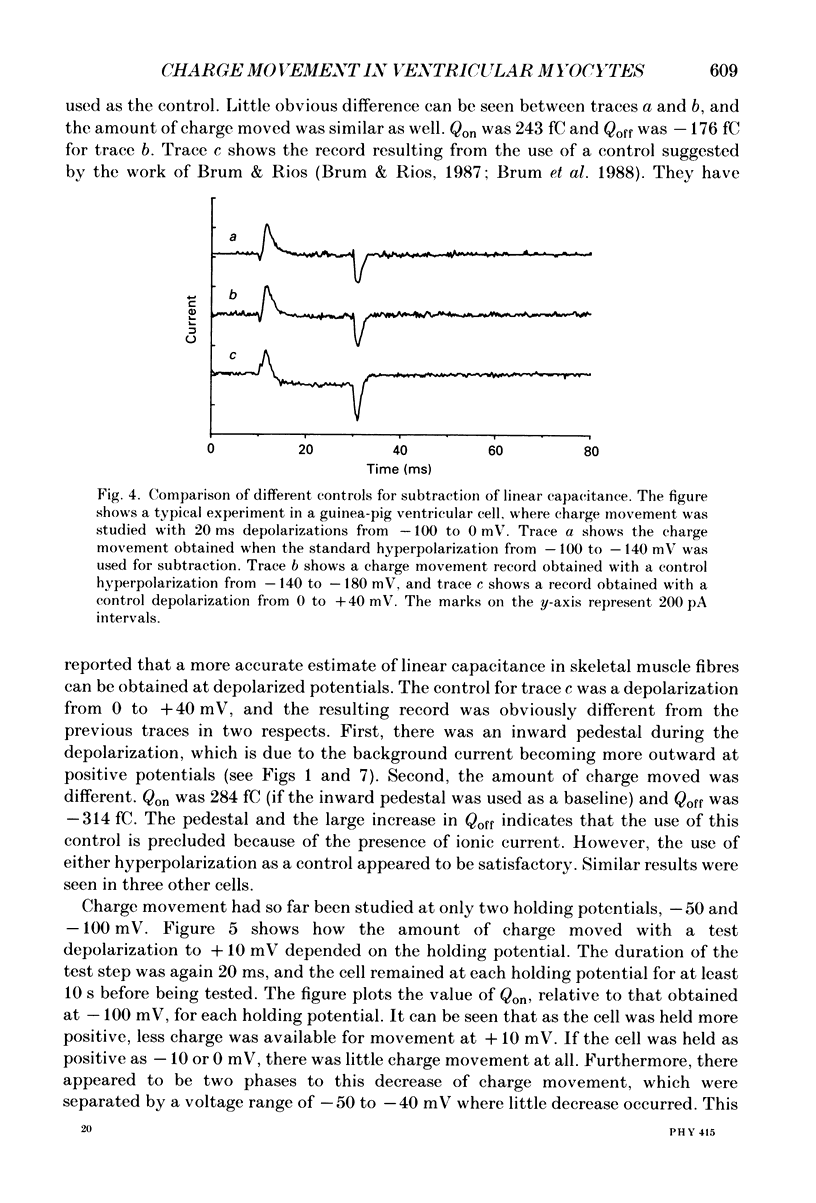
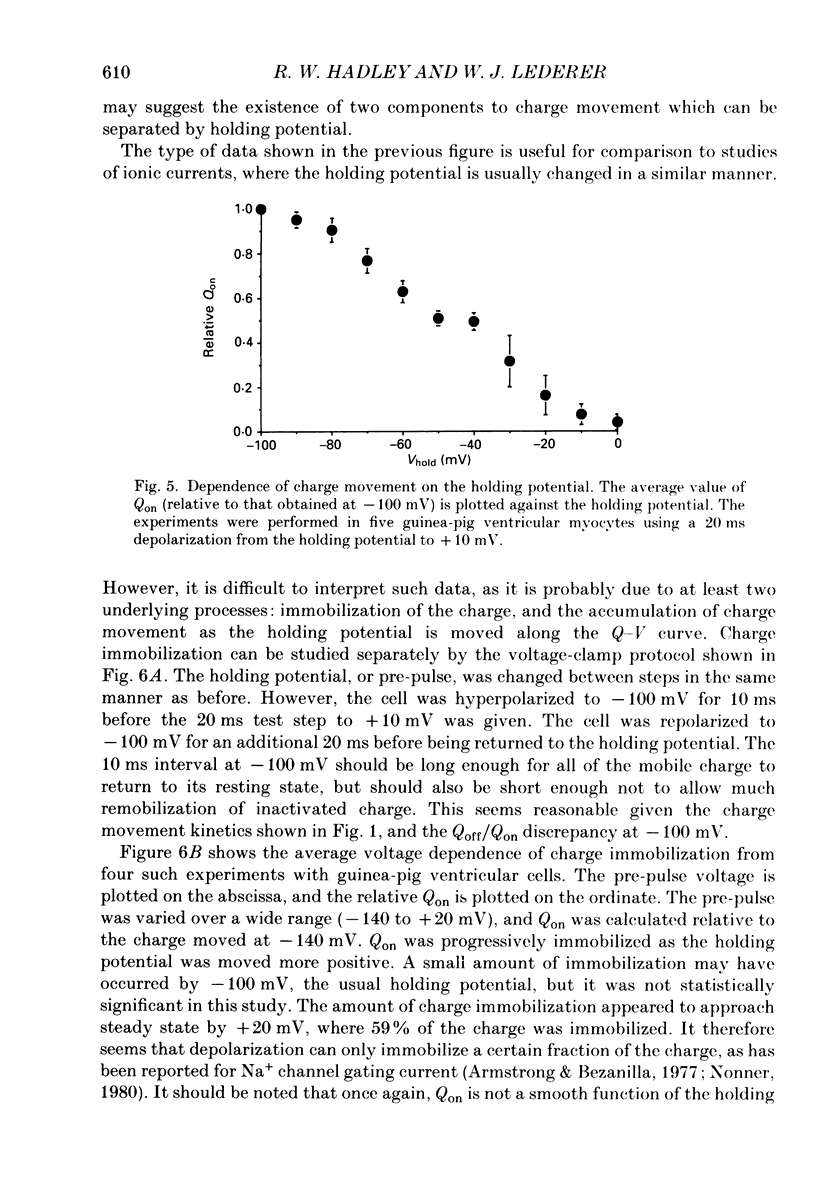
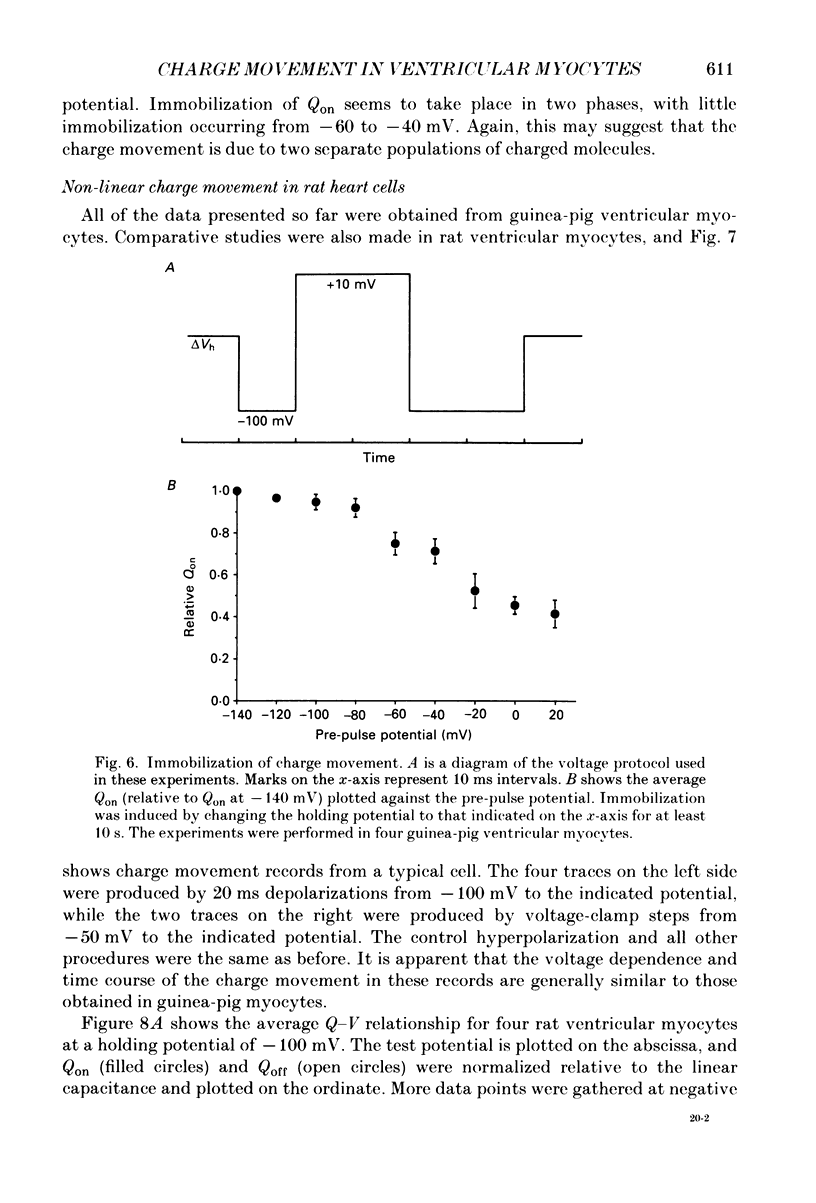
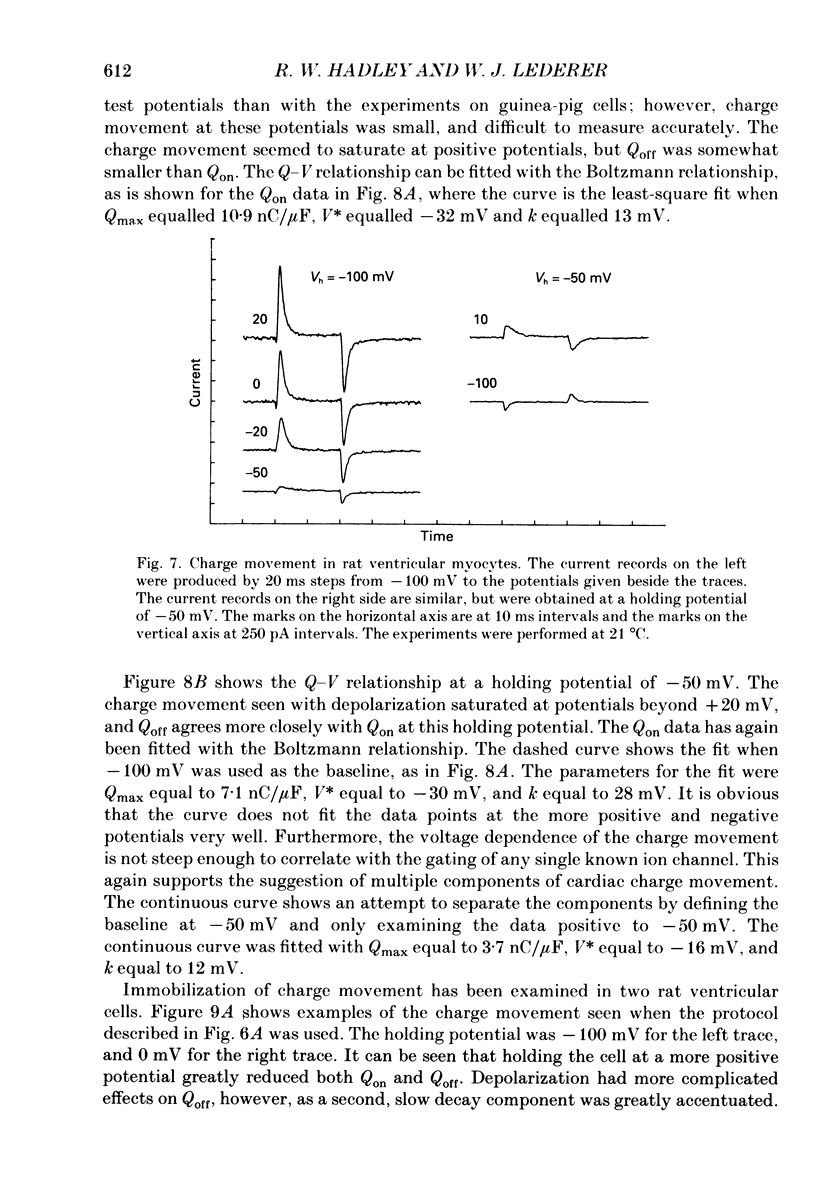
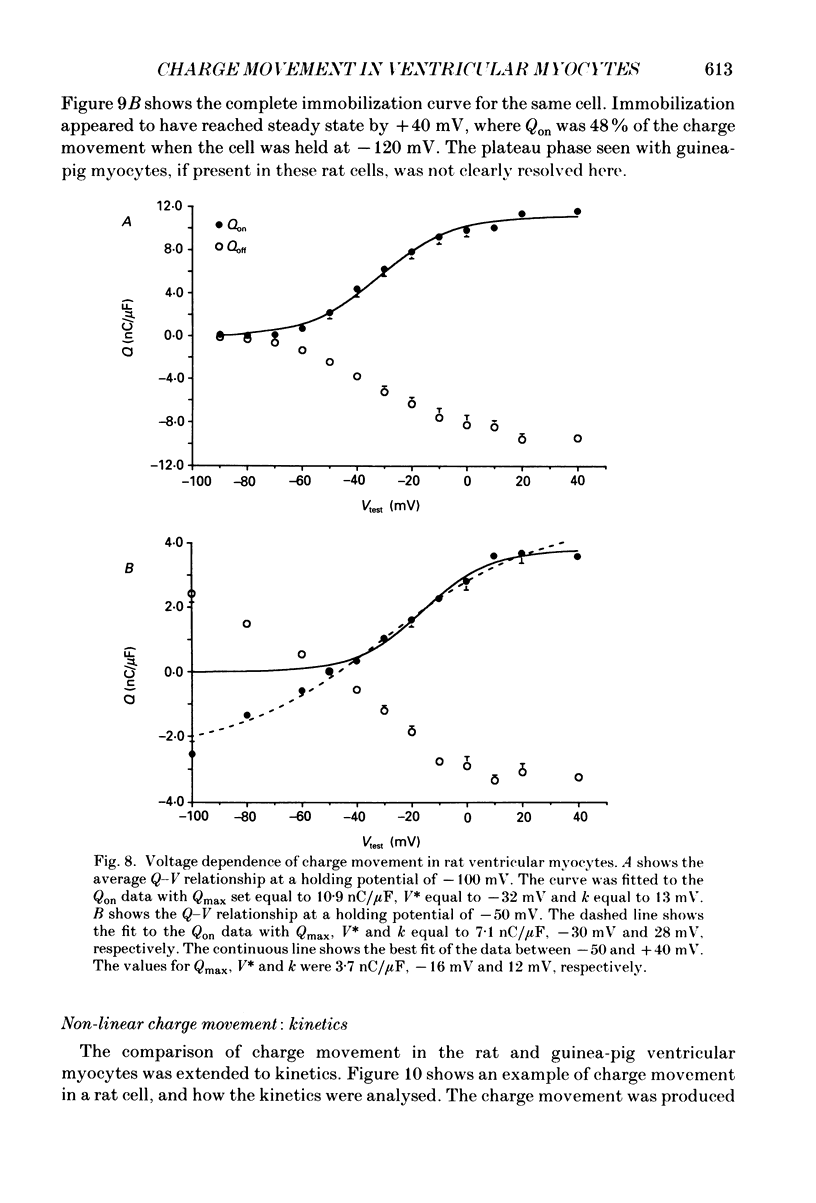
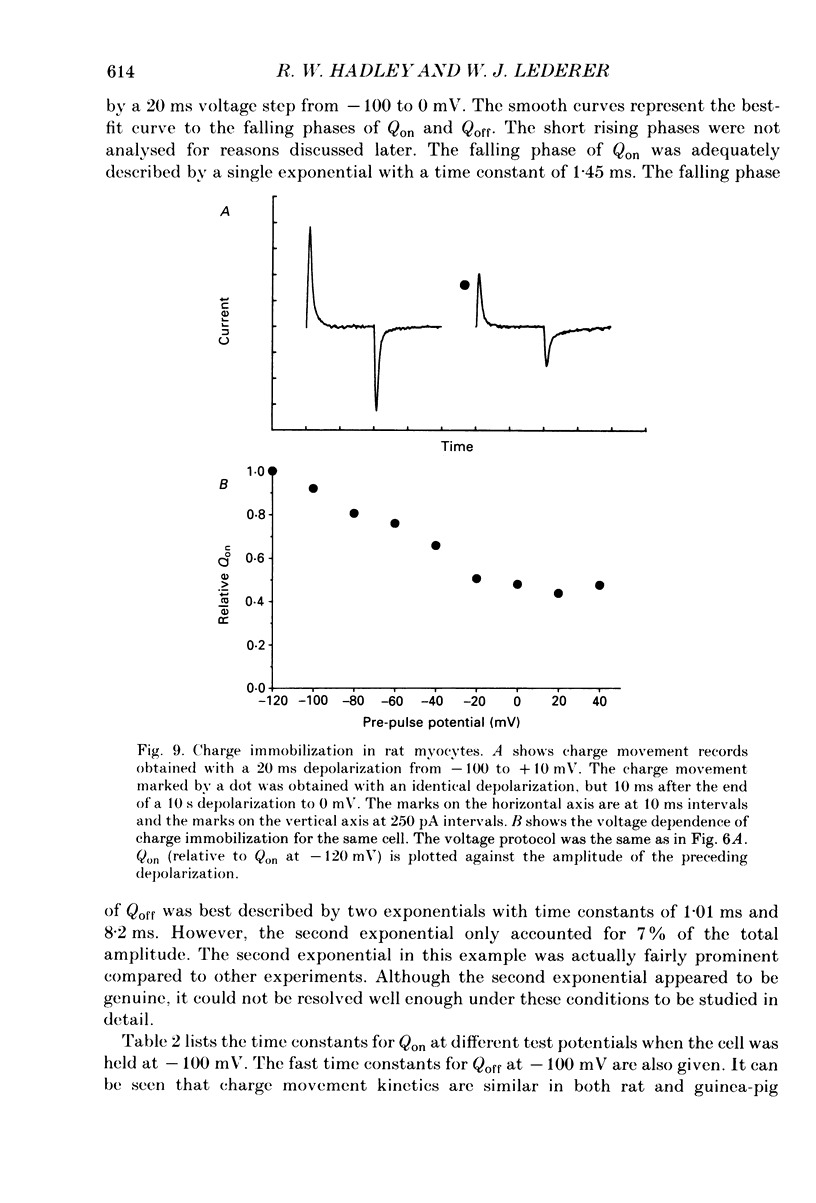
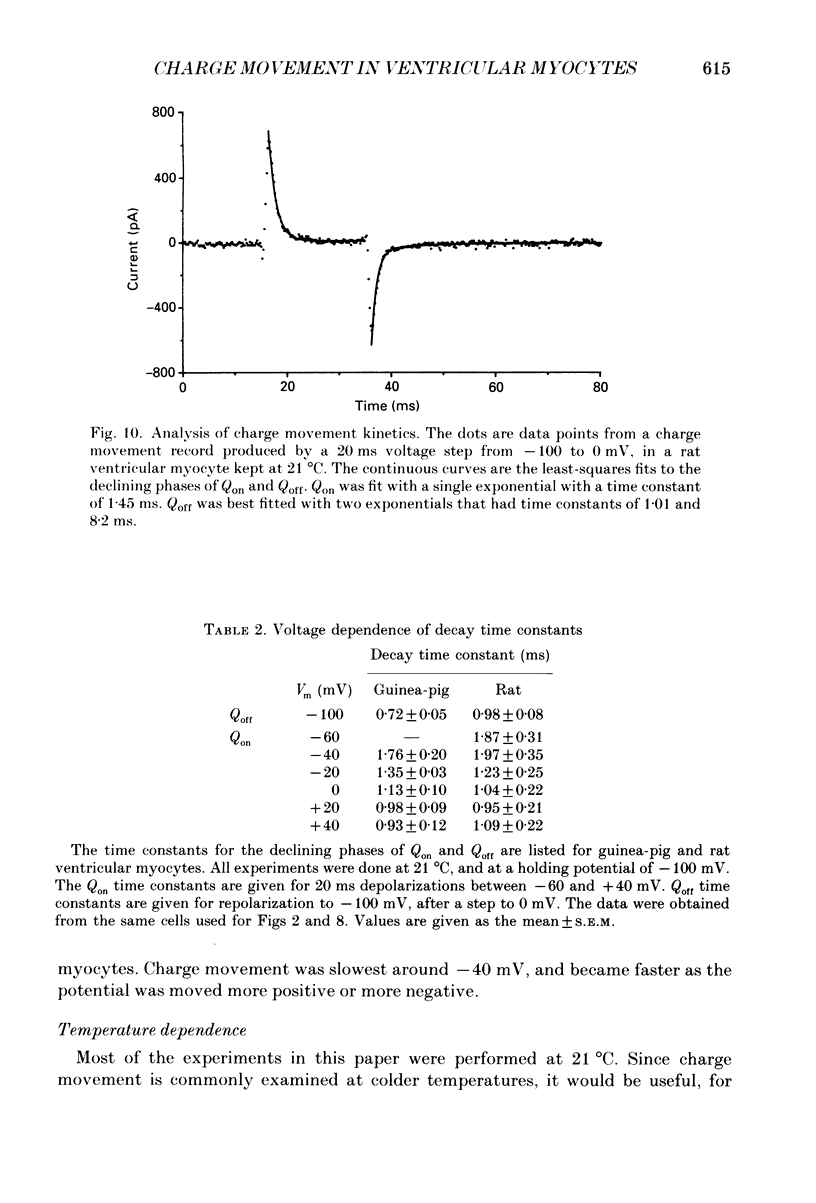
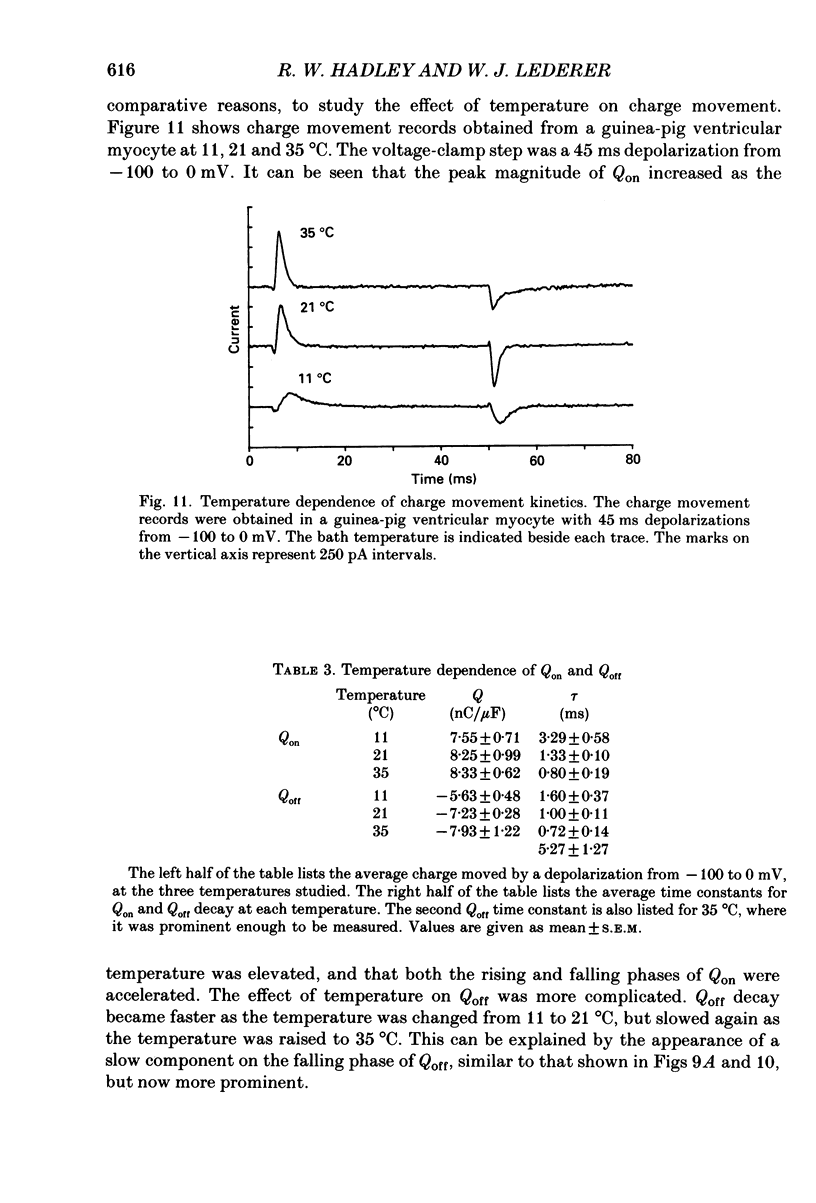
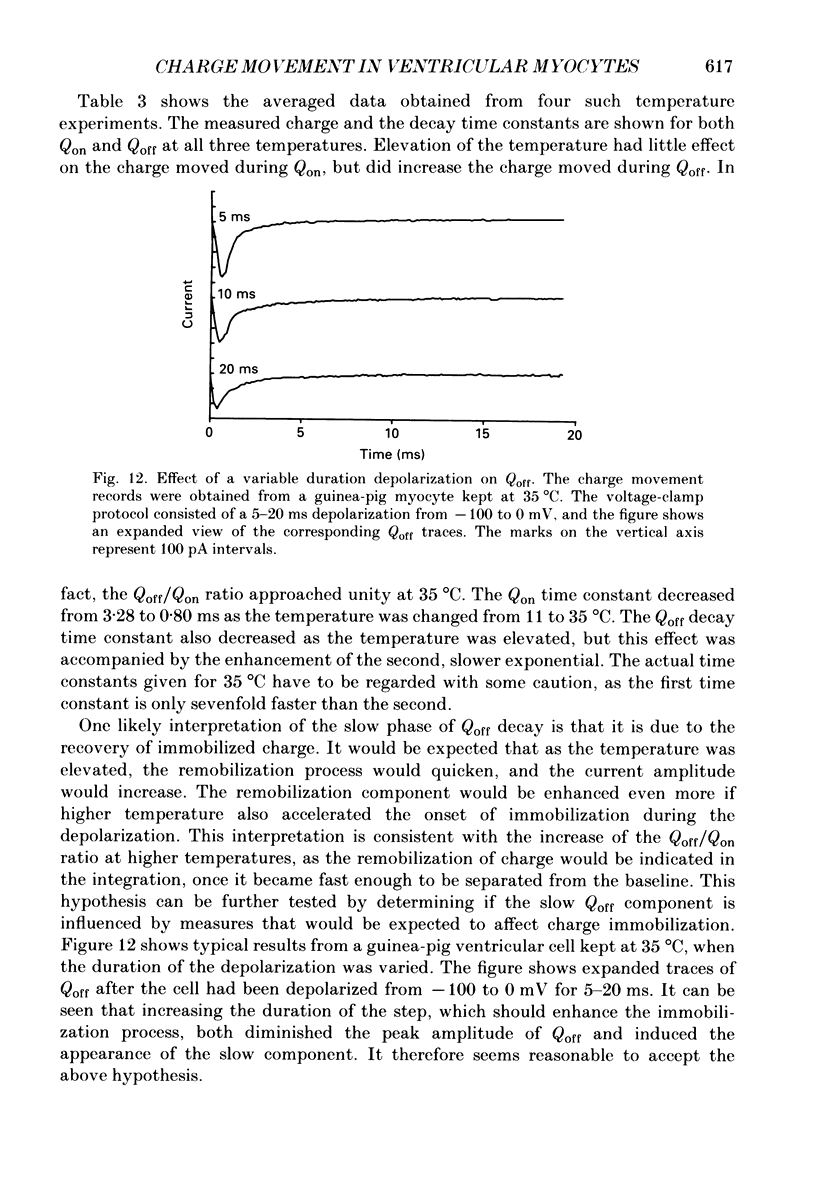
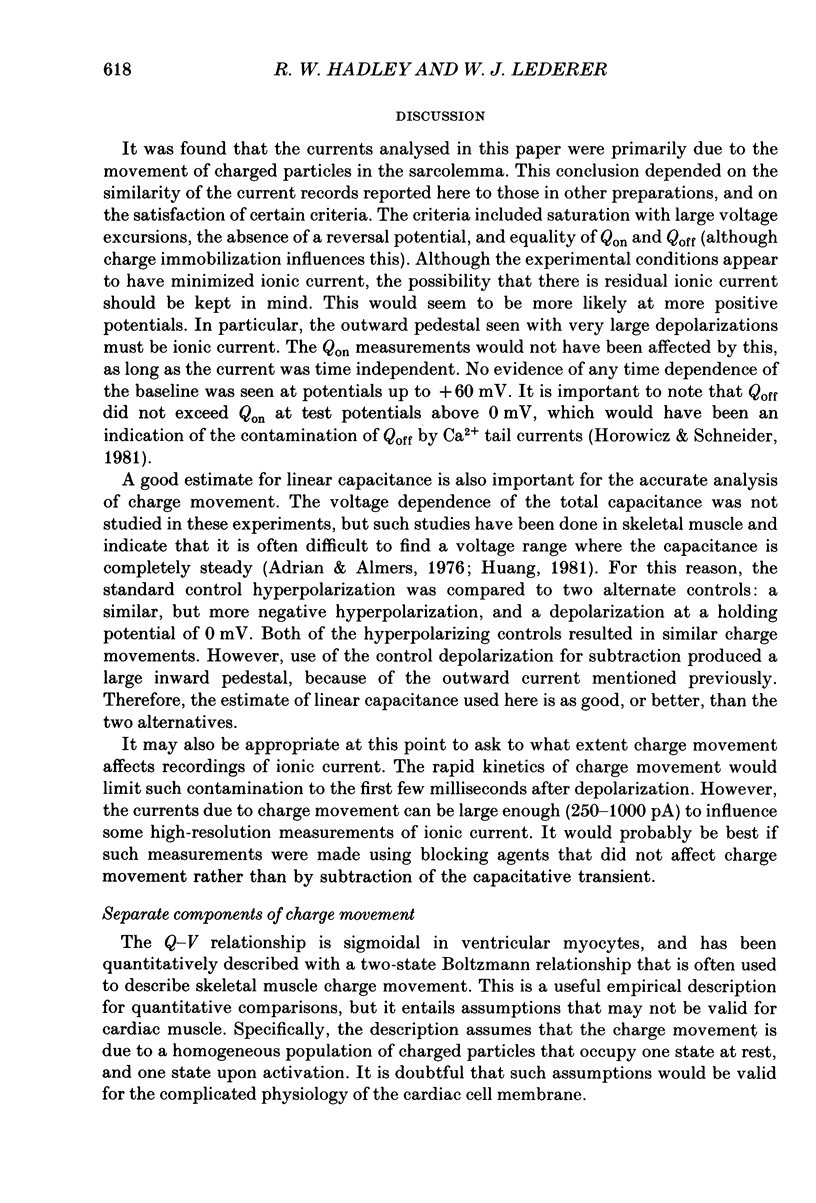
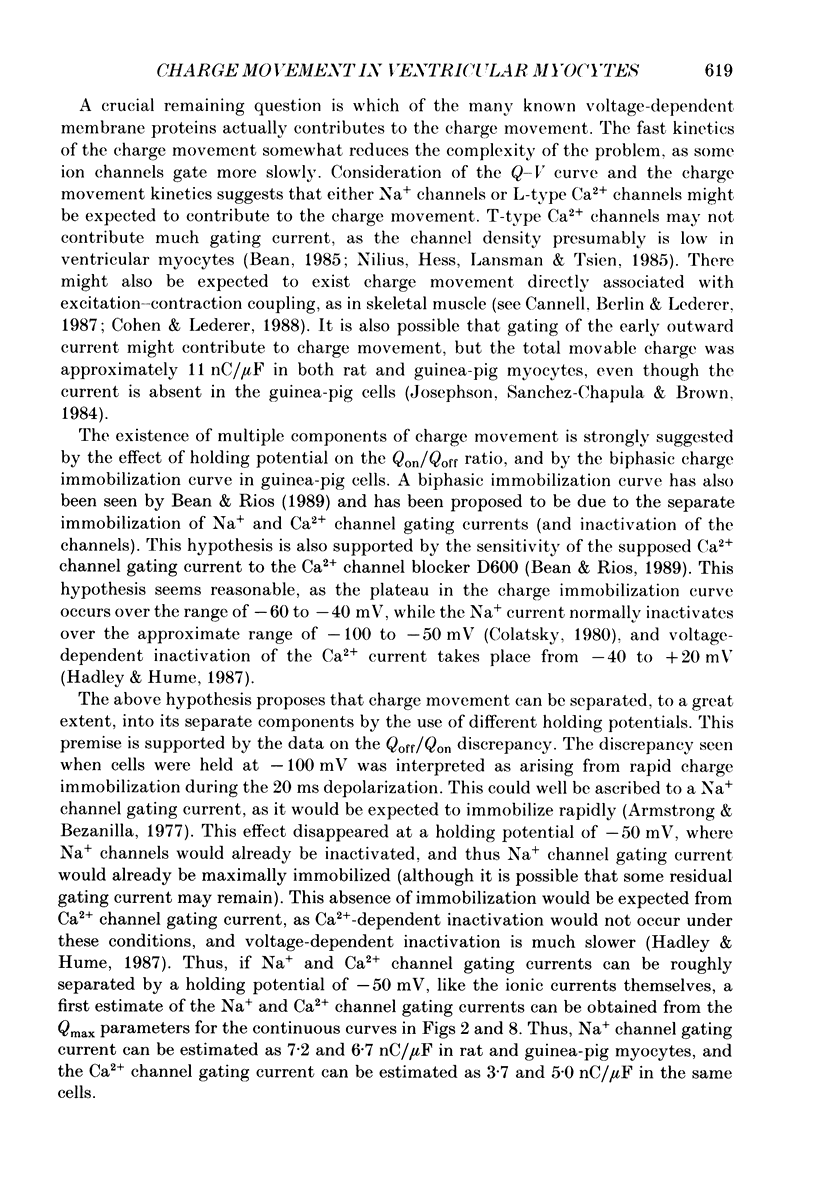
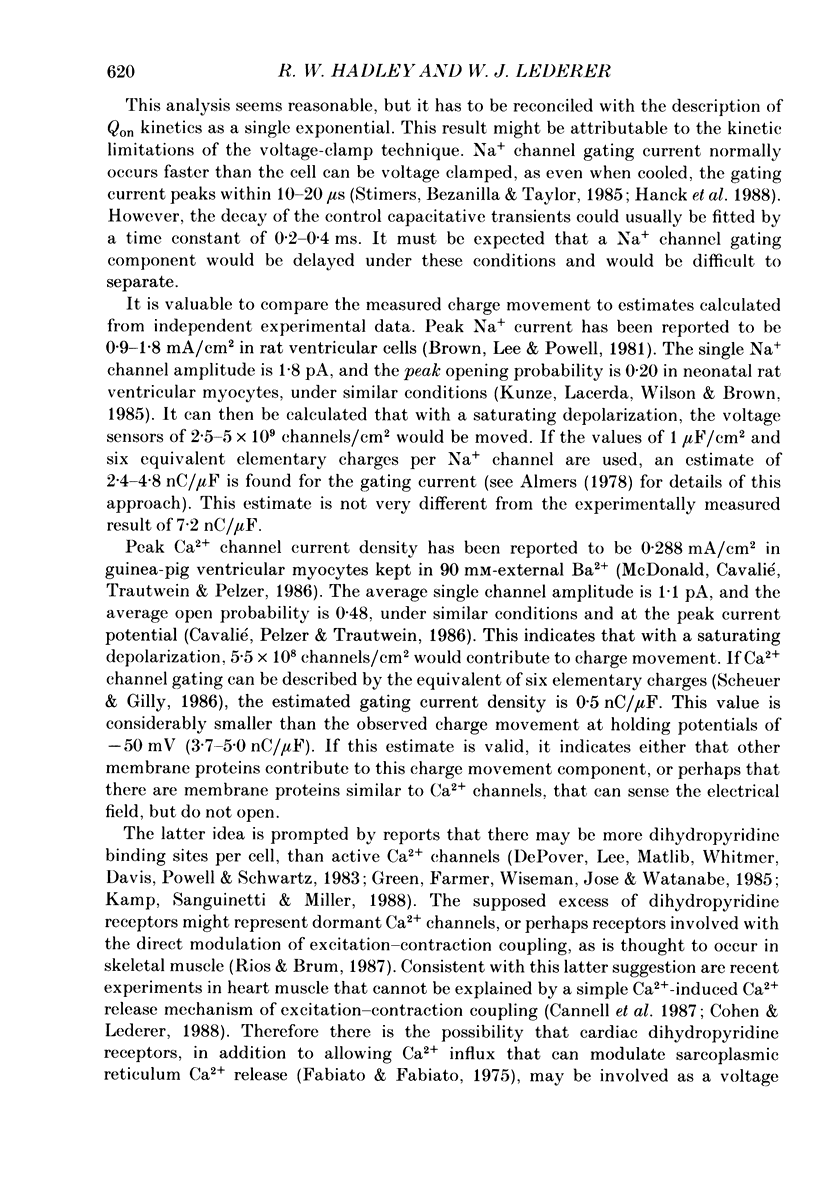
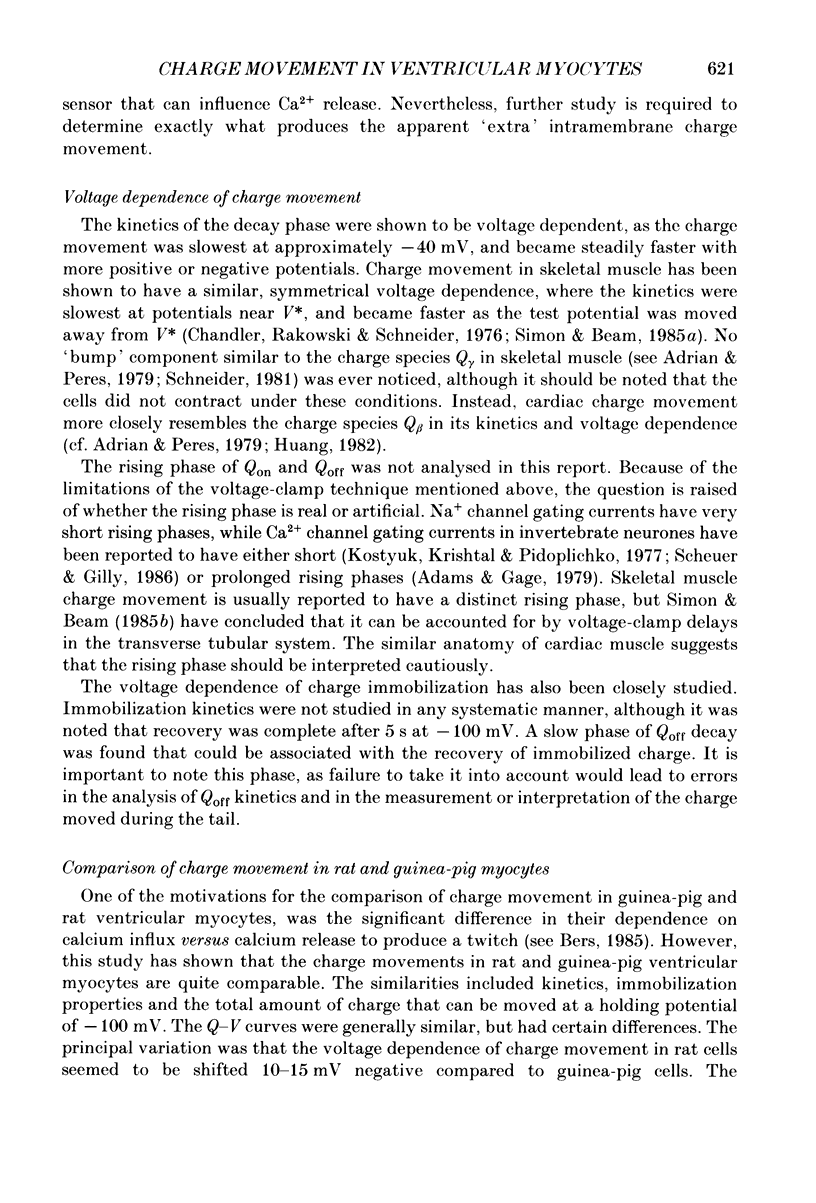
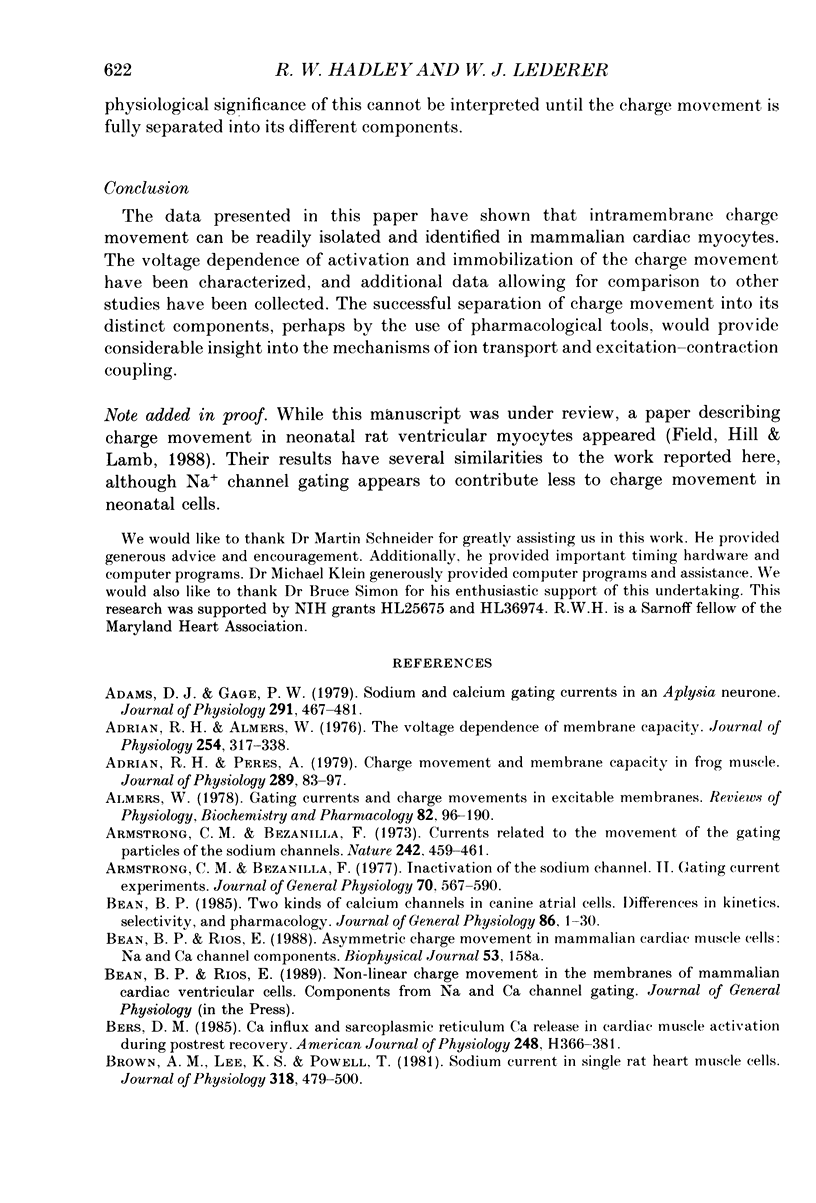
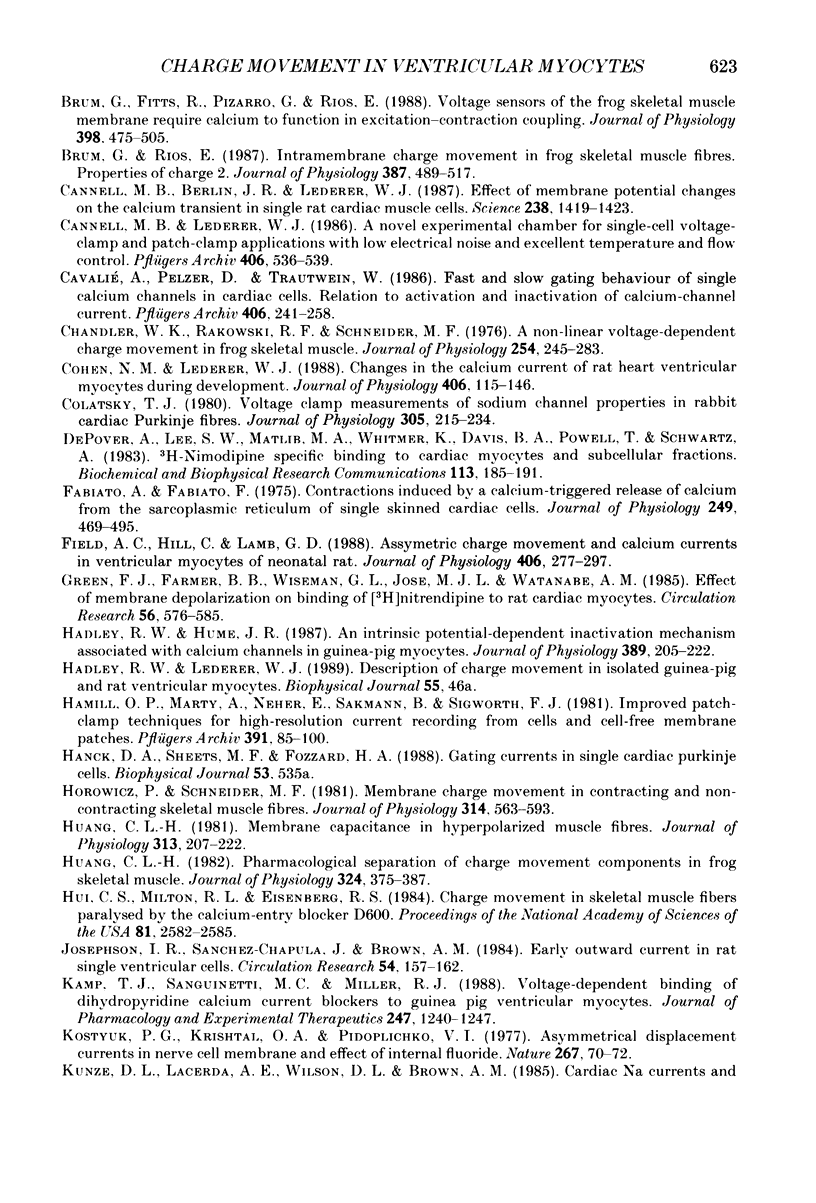
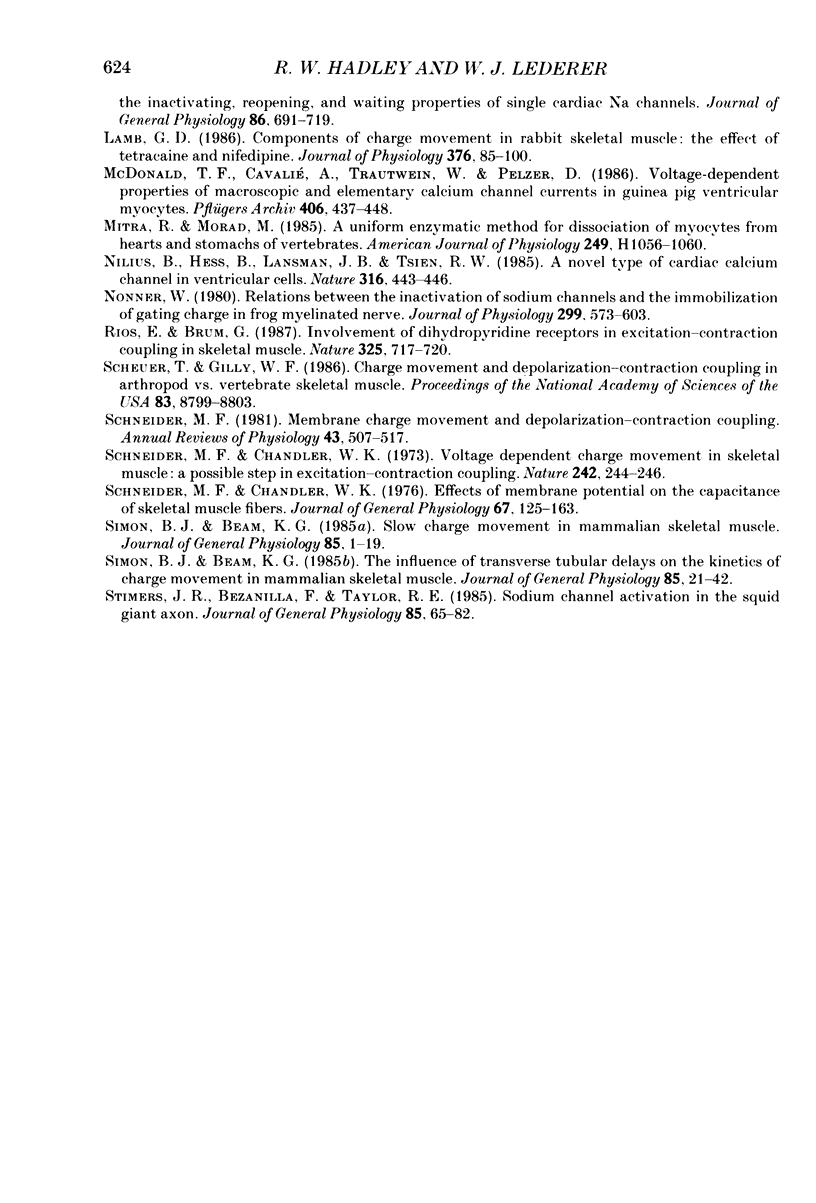
Selected References
These references are in PubMed. This may not be the complete list of references from this article.
- Adams D. J., Gage P. W. Sodium and calcium gating currents in an Aplysia neurone. J Physiol. 1979 Jun;291:467–481. doi: 10.1113/jphysiol.1979.sp012826. [DOI] [PMC free article] [PubMed] [Google Scholar]
- Adrian R. H., Almers W. The voltage dependence of membrane capacity. J Physiol. 1976 Jan;254(2):317–338. doi: 10.1113/jphysiol.1976.sp011234. [DOI] [PMC free article] [PubMed] [Google Scholar]
- Adrian R. H., Peres A. Charge movement and membrane capacity in frog muscle. J Physiol. 1979 Apr;289:83–97. doi: 10.1113/jphysiol.1979.sp012726. [DOI] [PMC free article] [PubMed] [Google Scholar]
- Almers W. Gating currents and charge movements in excitable membranes. Rev Physiol Biochem Pharmacol. 1978;82:96–190. doi: 10.1007/BFb0030498. [DOI] [PubMed] [Google Scholar]
- Armstrong C. M., Bezanilla F. Currents related to movement of the gating particles of the sodium channels. Nature. 1973 Apr 13;242(5398):459–461. doi: 10.1038/242459a0. [DOI] [PubMed] [Google Scholar]
- Armstrong C. M., Bezanilla F. Inactivation of the sodium channel. II. Gating current experiments. J Gen Physiol. 1977 Nov;70(5):567–590. doi: 10.1085/jgp.70.5.567. [DOI] [PMC free article] [PubMed] [Google Scholar]
- Bean B. P. Two kinds of calcium channels in canine atrial cells. Differences in kinetics, selectivity, and pharmacology. J Gen Physiol. 1985 Jul;86(1):1–30. doi: 10.1085/jgp.86.1.1. [DOI] [PMC free article] [PubMed] [Google Scholar]
- Bers D. M. Ca influx and sarcoplasmic reticulum Ca release in cardiac muscle activation during postrest recovery. Am J Physiol. 1985 Mar;248(3 Pt 2):H366–H381. doi: 10.1152/ajpheart.1985.248.3.H366. [DOI] [PubMed] [Google Scholar]
- Brown A. M., Lee K. S., Powell T. Sodium current in single rat heart muscle cells. J Physiol. 1981 Sep;318:479–500. doi: 10.1113/jphysiol.1981.sp013879. [DOI] [PMC free article] [PubMed] [Google Scholar]
- Brum G., Fitts R., Pizarro G., Ríos E. Voltage sensors of the frog skeletal muscle membrane require calcium to function in excitation-contraction coupling. J Physiol. 1988 Apr;398:475–505. doi: 10.1113/jphysiol.1988.sp017053. [DOI] [PMC free article] [PubMed] [Google Scholar]
- Brum G., Rios E. Intramembrane charge movement in frog skeletal muscle fibres. Properties of charge 2. J Physiol. 1987 Jun;387:489–517. doi: 10.1113/jphysiol.1987.sp016586. [DOI] [PMC free article] [PubMed] [Google Scholar]
- Cannell M. B., Berlin J. R., Lederer W. J. Effect of membrane potential changes on the calcium transient in single rat cardiac muscle cells. Science. 1987 Dec 4;238(4832):1419–1423. doi: 10.1126/science.2446391. [DOI] [PubMed] [Google Scholar]
- Cannell M. B., Lederer W. J. A novel experimental chamber for single-cell voltage-clamp and patch-clamp applications with low electrical noise and excellent temperature and flow control. Pflugers Arch. 1986 May;406(5):536–539. doi: 10.1007/BF00583378. [DOI] [PubMed] [Google Scholar]
- Cavalié A., Pelzer D., Trautwein W. Fast and slow gating behaviour of single calcium channels in cardiac cells. Relation to activation and inactivation of calcium-channel current. Pflugers Arch. 1986 Mar;406(3):241–258. doi: 10.1007/BF00640910. [DOI] [PubMed] [Google Scholar]
- Chandler W. K., Rakowski R. F., Schneider M. F. A non-linear voltage dependent charge movement in frog skeletal muscle. J Physiol. 1976 Jan;254(2):245–283. doi: 10.1113/jphysiol.1976.sp011232. [DOI] [PMC free article] [PubMed] [Google Scholar]
- Cohen N. M., Lederer W. J. Changes in the calcium current of rat heart ventricular myocytes during development. J Physiol. 1988 Dec;406:115–146. doi: 10.1113/jphysiol.1988.sp017372. [DOI] [PMC free article] [PubMed] [Google Scholar]
- Colatsky T. J. Voltage clamp measurements of sodium channel properties in rabbit cardiac Purkinje fibres. J Physiol. 1980 Aug;305:215–234. doi: 10.1113/jphysiol.1980.sp013359. [DOI] [PMC free article] [PubMed] [Google Scholar]
- DePover A., Lee S. W., Matlib M. A., Whitmer K., Davis B. A., Powell T., Schwartz A. [3H]nimodipine specific binding to cardiac myocytes and subcellular fractions. Biochem Biophys Res Commun. 1983 May 31;113(1):185–191. doi: 10.1016/0006-291x(83)90449-7. [DOI] [PubMed] [Google Scholar]
- Fabiato A., Fabiato F. Contractions induced by a calcium-triggered release of calcium from the sarcoplasmic reticulum of single skinned cardiac cells. J Physiol. 1975 Aug;249(3):469–495. doi: 10.1113/jphysiol.1975.sp011026. [DOI] [PMC free article] [PubMed] [Google Scholar]
- Field A. C., Hill C., Lamb G. D. Asymmetric charge movement and calcium currents in ventricular myocytes of neonatal rat. J Physiol. 1988 Dec;406:277–297. doi: 10.1113/jphysiol.1988.sp017380. [DOI] [PMC free article] [PubMed] [Google Scholar]
- Green F. J., Farmer B. B., Wiseman G. L., Jose M. J., Watanabe A. M. Effect of membrane depolarization on binding of [3H]nitrendipine to rat cardiac myocytes. Circ Res. 1985 Apr;56(4):576–585. doi: 10.1161/01.res.56.4.576. [DOI] [PubMed] [Google Scholar]
- Hadley R. W., Hume J. R. An intrinsic potential-dependent inactivation mechanism associated with calcium channels in guinea-pig myocytes. J Physiol. 1987 Aug;389:205–222. doi: 10.1113/jphysiol.1987.sp016654. [DOI] [PMC free article] [PubMed] [Google Scholar]
- Hamill O. P., Marty A., Neher E., Sakmann B., Sigworth F. J. Improved patch-clamp techniques for high-resolution current recording from cells and cell-free membrane patches. Pflugers Arch. 1981 Aug;391(2):85–100. doi: 10.1007/BF00656997. [DOI] [PubMed] [Google Scholar]
- Horowicz P., Schneider M. F. Membrane charge movement in contracting and non-contracting skeletal muscle fibres. J Physiol. 1981 May;314:565–593. doi: 10.1113/jphysiol.1981.sp013725. [DOI] [PMC free article] [PubMed] [Google Scholar]
- Huang C. L. Membrane capacitance in hyperpolarized muscle fibres. J Physiol. 1981;313:207–222. doi: 10.1113/jphysiol.1981.sp013659. [DOI] [PMC free article] [PubMed] [Google Scholar]
- Huang C. L. Pharmacological separation of charge movement components in frog skeletal muscle. J Physiol. 1982 Mar;324:375–387. doi: 10.1113/jphysiol.1982.sp014118. [DOI] [PMC free article] [PubMed] [Google Scholar]
- Hui C. S., Milton R. L., Eisenberg R. S. Charge movement in skeletal muscle fibers paralyzed by the calcium-entry blocker D600. Proc Natl Acad Sci U S A. 1984 Apr;81(8):2582–2585. doi: 10.1073/pnas.81.8.2582. [DOI] [PMC free article] [PubMed] [Google Scholar]
- Josephson I. R., Sanchez-Chapula J., Brown A. M. Early outward current in rat single ventricular cells. Circ Res. 1984 Feb;54(2):157–162. doi: 10.1161/01.res.54.2.157. [DOI] [PubMed] [Google Scholar]
- Kamp T. J., Sanguinetti M. C., Miller R. J. Voltage-dependent binding of dihydropyridine calcium channel blockers to guinea pig ventricular myocytes. J Pharmacol Exp Ther. 1988 Dec;247(3):1240–1247. [PubMed] [Google Scholar]
- Kostyuk P. G., Krishtal O. A., Pidoplichko V. I. Asymmetrical displacement currents in nerve cell membrane and effect of internal fluoride. Nature. 1977 May 5;267(5606):70–72. doi: 10.1038/267070a0. [DOI] [PubMed] [Google Scholar]
- Kunze D. L., Lacerda A. E., Wilson D. L., Brown A. M. Cardiac Na currents and the inactivating, reopening, and waiting properties of single cardiac Na channels. J Gen Physiol. 1985 Nov;86(5):691–719. doi: 10.1085/jgp.86.5.691. [DOI] [PMC free article] [PubMed] [Google Scholar]
- Lamb G. D. Components of charge movement in rabbit skeletal muscle: the effect of tetracaine and nifedipine. J Physiol. 1986 Jul;376:85–100. doi: 10.1113/jphysiol.1986.sp016143. [DOI] [PMC free article] [PubMed] [Google Scholar]
- McDonald T. F., Cavalié A., Trautwein W., Pelzer D. Voltage-dependent properties of macroscopic and elementary calcium channel currents in guinea pig ventricular myocytes. Pflugers Arch. 1986 May;406(5):437–448. doi: 10.1007/BF00583365. [DOI] [PubMed] [Google Scholar]
- Nilius B., Hess P., Lansman J. B., Tsien R. W. A novel type of cardiac calcium channel in ventricular cells. Nature. 1985 Aug 1;316(6027):443–446. doi: 10.1038/316443a0. [DOI] [PubMed] [Google Scholar]
- Nonner W. Relations between the inactivation of sodium channels and the immobilization of gating charge in frog myelinated nerve. J Physiol. 1980 Feb;299:573–603. doi: 10.1113/jphysiol.1980.sp013143. [DOI] [PMC free article] [PubMed] [Google Scholar]
- Rios E., Brum G. Involvement of dihydropyridine receptors in excitation-contraction coupling in skeletal muscle. Nature. 1987 Feb 19;325(6106):717–720. doi: 10.1038/325717a0. [DOI] [PubMed] [Google Scholar]
- Scheuer T., Gilly W. F. Charge movement and depolarization-contraction coupling in arthropod vs. vertebrate skeletal muscle. Proc Natl Acad Sci U S A. 1986 Nov;83(22):8799–8803. doi: 10.1073/pnas.83.22.8799. [DOI] [PMC free article] [PubMed] [Google Scholar]
- Schneider M. F., Chandler W. K. Effects of membrane potential on the capacitance of skeletal muscle fibers. J Gen Physiol. 1976 Feb;67(2):125–163. doi: 10.1085/jgp.67.2.125. [DOI] [PMC free article] [PubMed] [Google Scholar]
- Schneider M. F., Chandler W. K. Voltage dependent charge movement of skeletal muscle: a possible step in excitation-contraction coupling. Nature. 1973 Mar 23;242(5395):244–246. doi: 10.1038/242244a0. [DOI] [PubMed] [Google Scholar]
- Schneider M. F. Membrane charge movement and depolarization-contraction coupling. Annu Rev Physiol. 1981;43:507–517. doi: 10.1146/annurev.ph.43.030181.002451. [DOI] [PubMed] [Google Scholar]
- Simon B. J., Beam K. G. Slow charge movement in mammalian skeletal muscle. J Gen Physiol. 1985 Jan;85(1):1–19. doi: 10.1085/jgp.85.1.1. [DOI] [PMC free article] [PubMed] [Google Scholar]
- Simon B. J., Beam K. G. The influence of transverse tubular delays on the kinetics of charge movement in mammalian skeletal muscle. J Gen Physiol. 1985 Jan;85(1):21–42. doi: 10.1085/jgp.85.1.21. [DOI] [PMC free article] [PubMed] [Google Scholar]
- Stimers J. R., Bezanilla F., Taylor R. E. Sodium channel activation in the squid giant axon. Steady state properties. J Gen Physiol. 1985 Jan;85(1):65–82. doi: 10.1085/jgp.85.1.65. [DOI] [PMC free article] [PubMed] [Google Scholar]