Abstract
1. Electrical and pharmacological properties of the low-voltage-activated Ca2+ current (ICa, LVA) in rat aorta smooth muscle cells (SMC) in primary culture were examined, particularly in comparison with the high-voltage-activated Ca2+ current (ICa, HVA). Both types of Ca2+ currents were recorded in external solution containing 20 mM-Ca2+, using the whole-cell voltage-clamp technique. 2. ICa, LVA was evoked by step depolarizations to potentials more positive than -60 mV from a holding potential of -100 mV, and reached a peak in the current-voltage (I-V) relationship around -30 mV. ICa, HVA was activated at -20 mV, and reached a peak at +20 mV. 3. The intracellular dialysis of 5 mM-F- irreversibly suppressed ICa, HVA, with time, while it has little effect on the ICa, LVA. The ICa, LVA could be separated from the ICa, HVA by either selecting the holding and test potential levels or by perfusing intracellularly with F-. 4. The ratio of peak amplitude of Ba2+, Sr2+ and Ca2+ currents in the respective I-V relationship was 1.6:1.2:1.0 for high-voltage-activated Ca2+ channels and was 1.0:1.4:1.0 for low-voltage-activated ones. 5. The inactivation phase of ICa, HVA was fitted by a sum of double-exponential functions, the time constants of which were larger when the current was carried by Ba2+ than by Ca2+. The inactivation time course of ICa, LVA was fitted by a single-exponential function, and the time constant was practically the same when the current was carried by Ba2+ or by Ca2+. Activation and inactivation processes of ICa, LVA were potential-dependent. 6. The steady-state inactivation curve of ICa, LVA was fitted by the Boltzmann equation, having a mid-potential of -80 mV and a slope factor of 5.0. The recovery time course from steady-state inactivation was fitted by a sum of two exponential functions. The time constants of the faster phase were 230 and 380 ms, and those of slower phase were 2.8 and 1.8 s at the repolarization potentials of -120 and -100 mV, respectively. 7. The amplitude of ICa, LVA depended on the external Ca2+ concentration ([Ca2+]o), approaching saturation at 95 mM [Ca2+]o. 8. Various polyvalent cations blocked both types of Ca2+ current reversibly in the order (IC50 in M): La3+ (8 x 10(-8)) greater than Cd2+ (6 x 10(-6)) greater than Ni2+ (1 x 10(-5)) greater than Zn2+ (2 x 10(-5)) for ICa, HVA, and La3+ (6 x 10(-7)) greater than Zn2+ (3 x 10(-5)) greater than Cd2+ (4 x 10(-4)) greater than Ni2+ (6 x 10(-4)) for ICa, LVA.(ABSTRACT TRUNCATED AT 400 WORDS)
Full text
PDF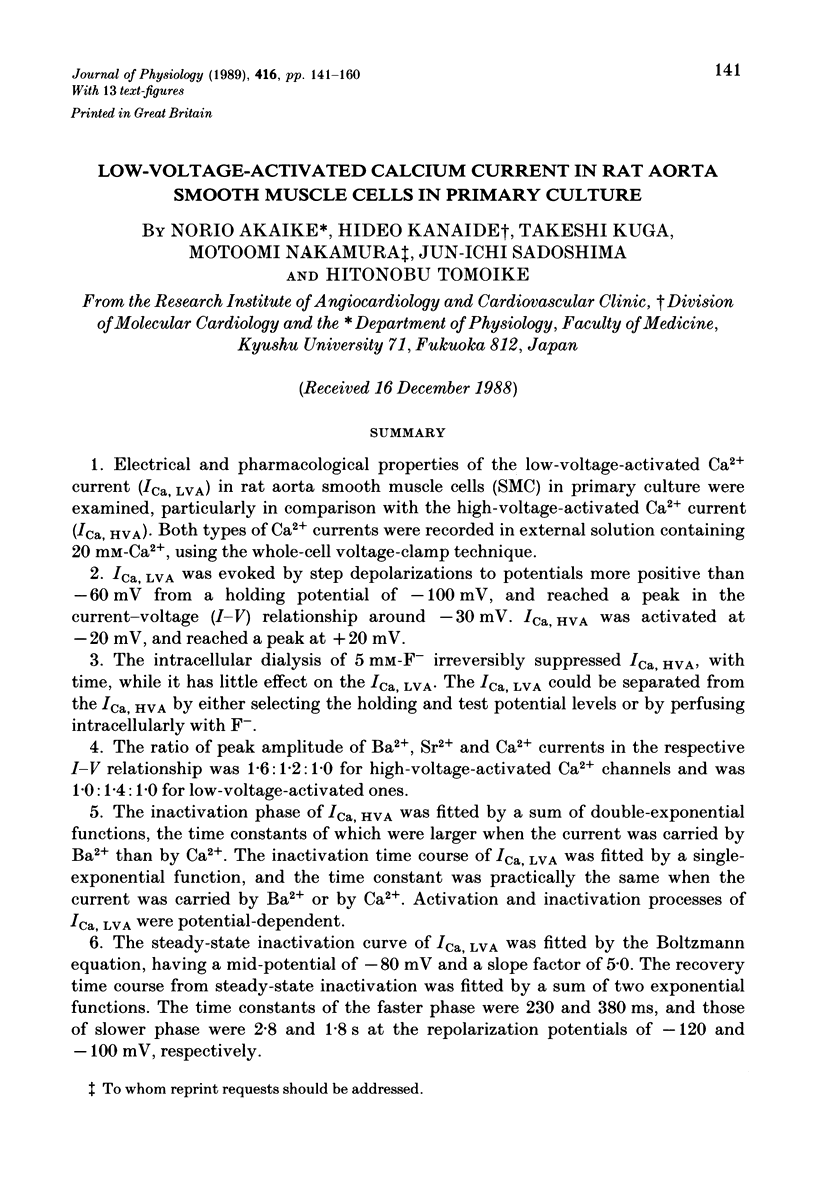
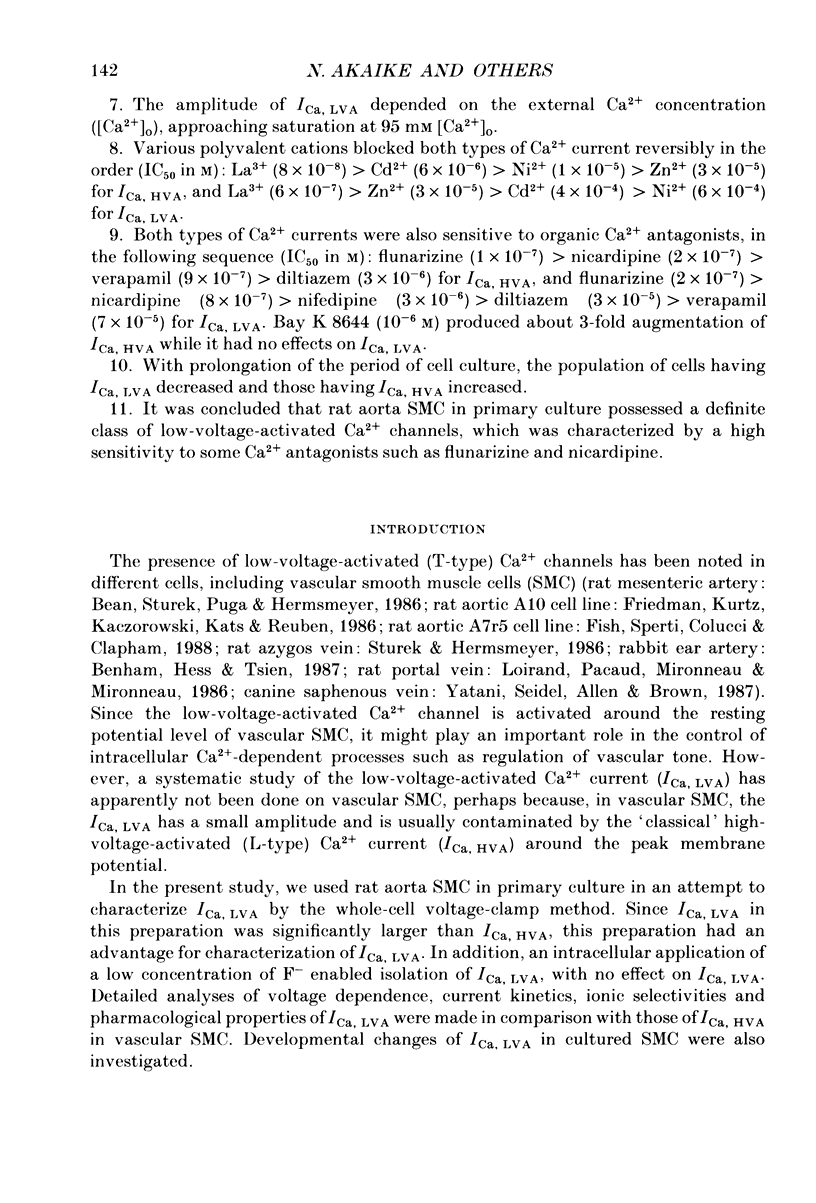
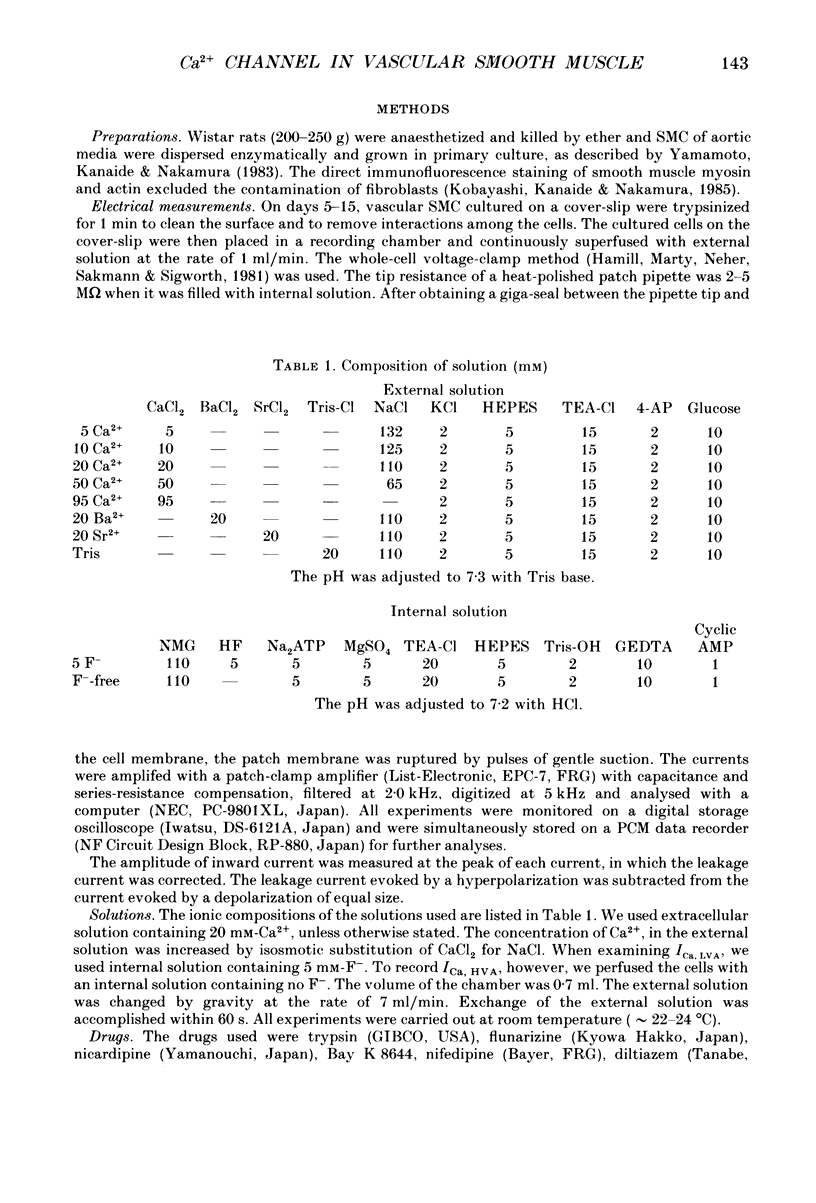
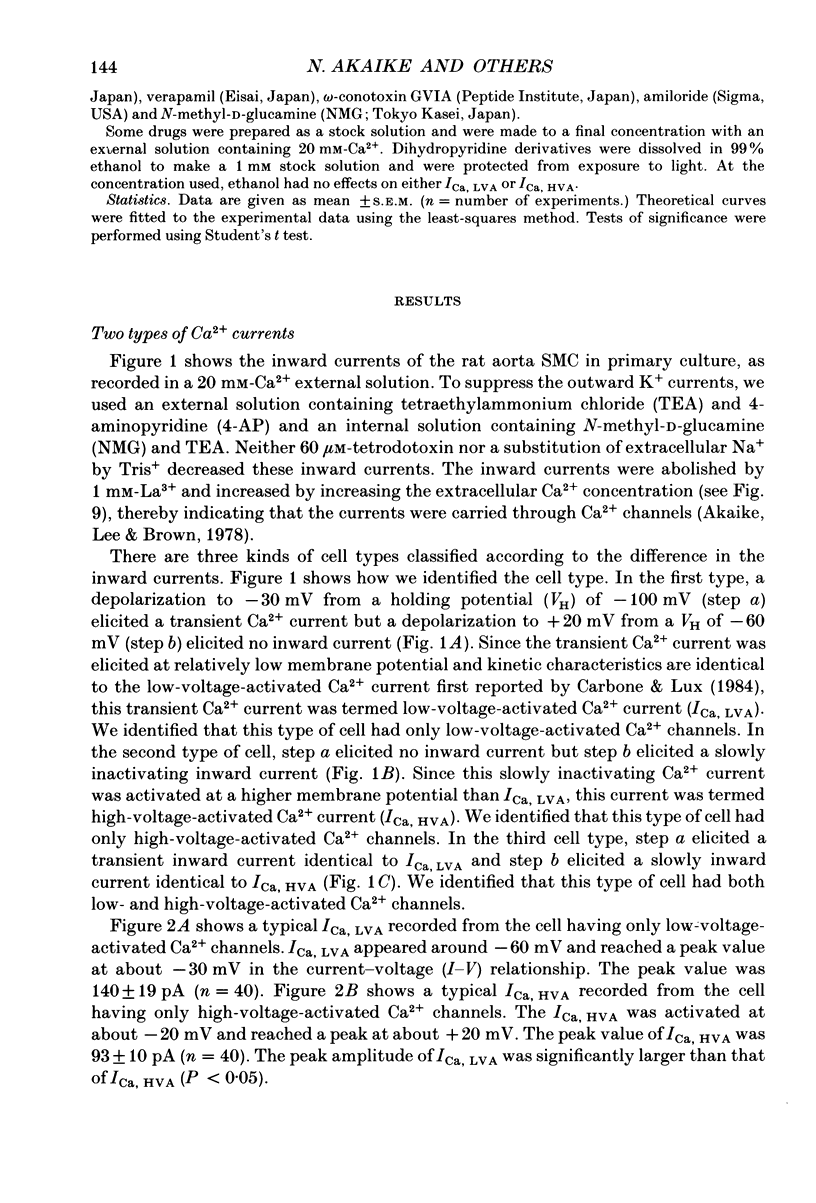
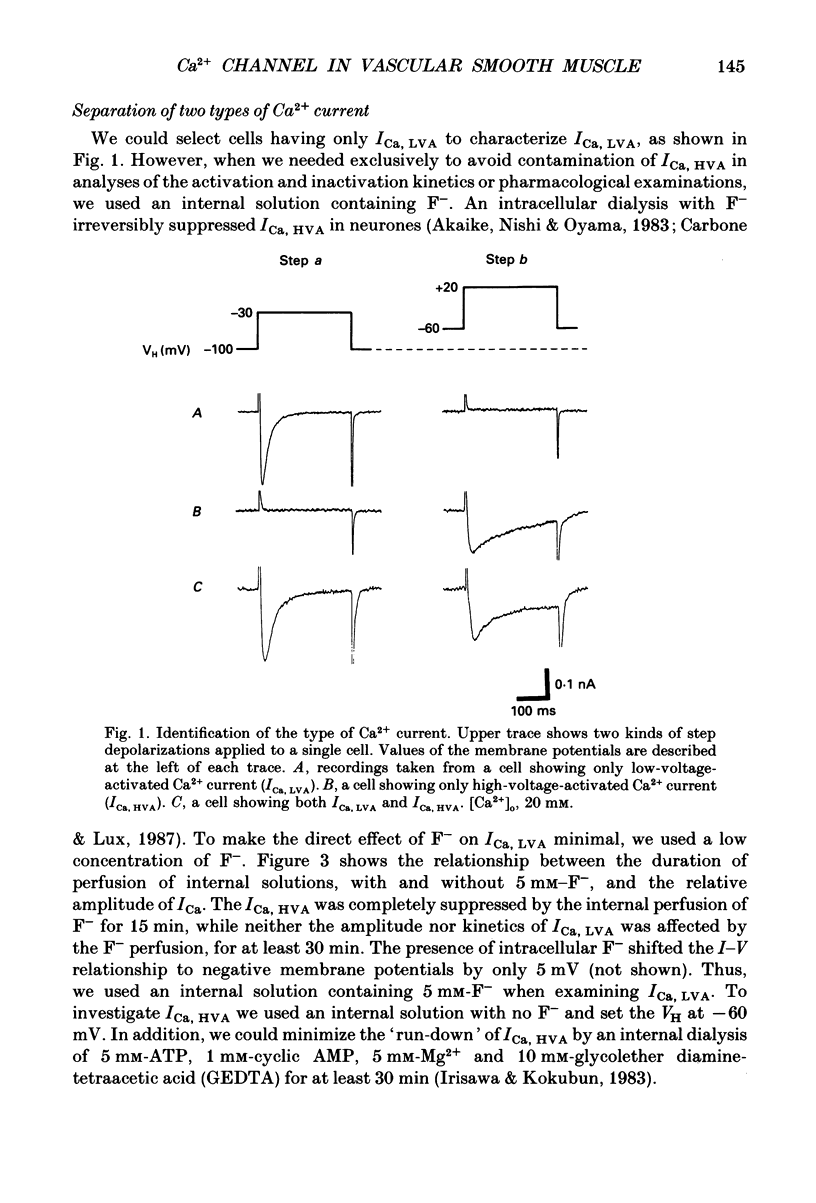
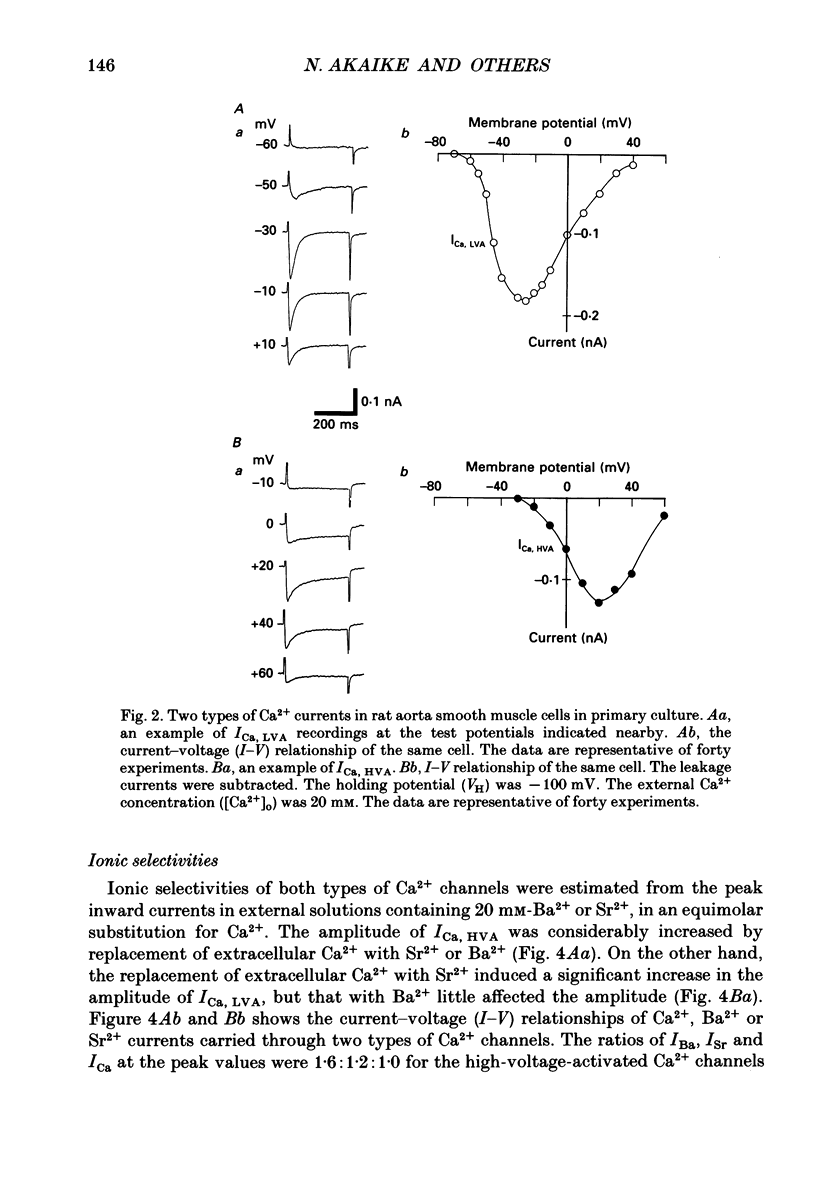
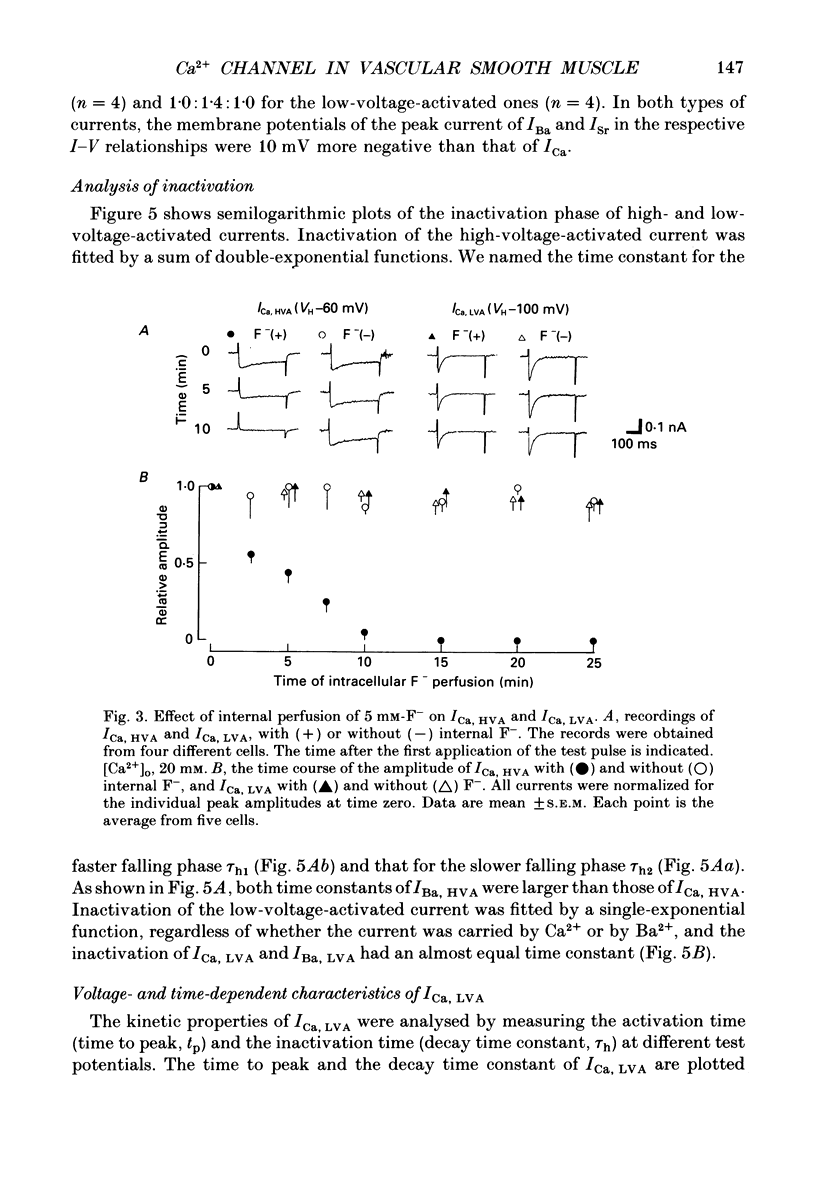
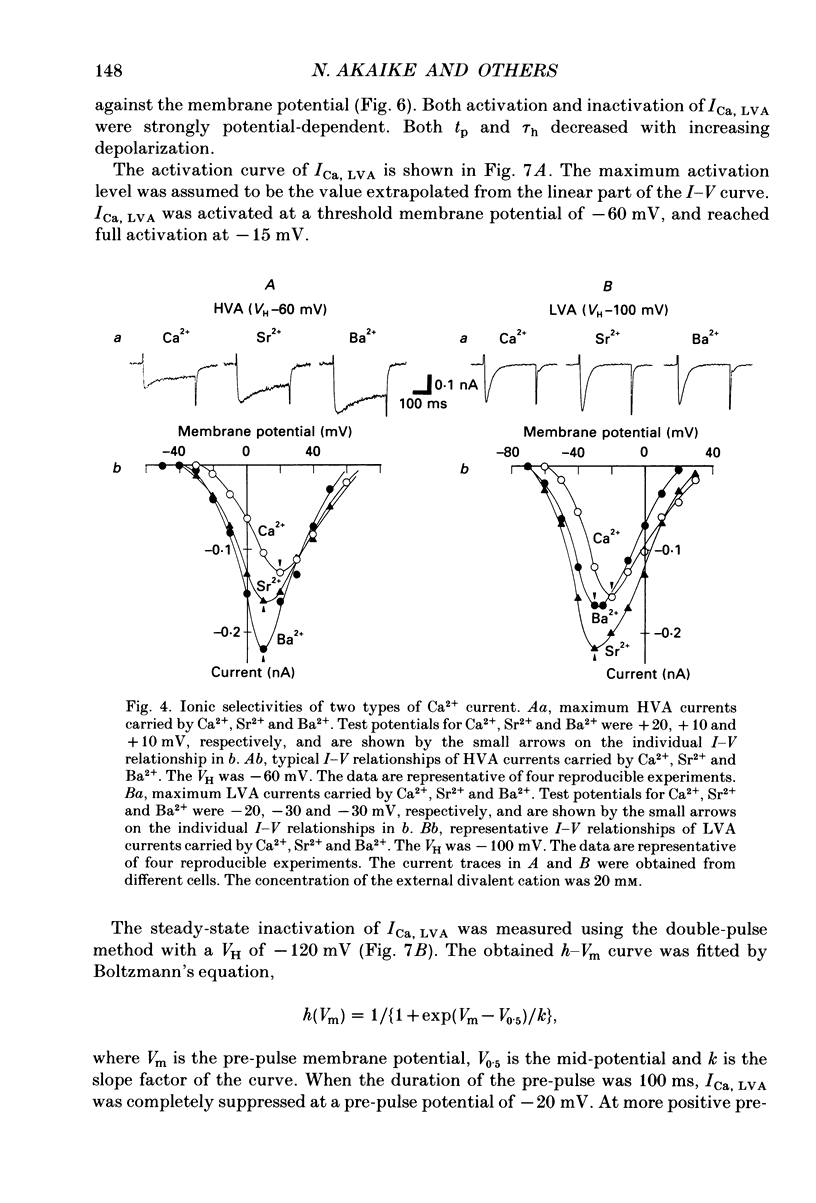
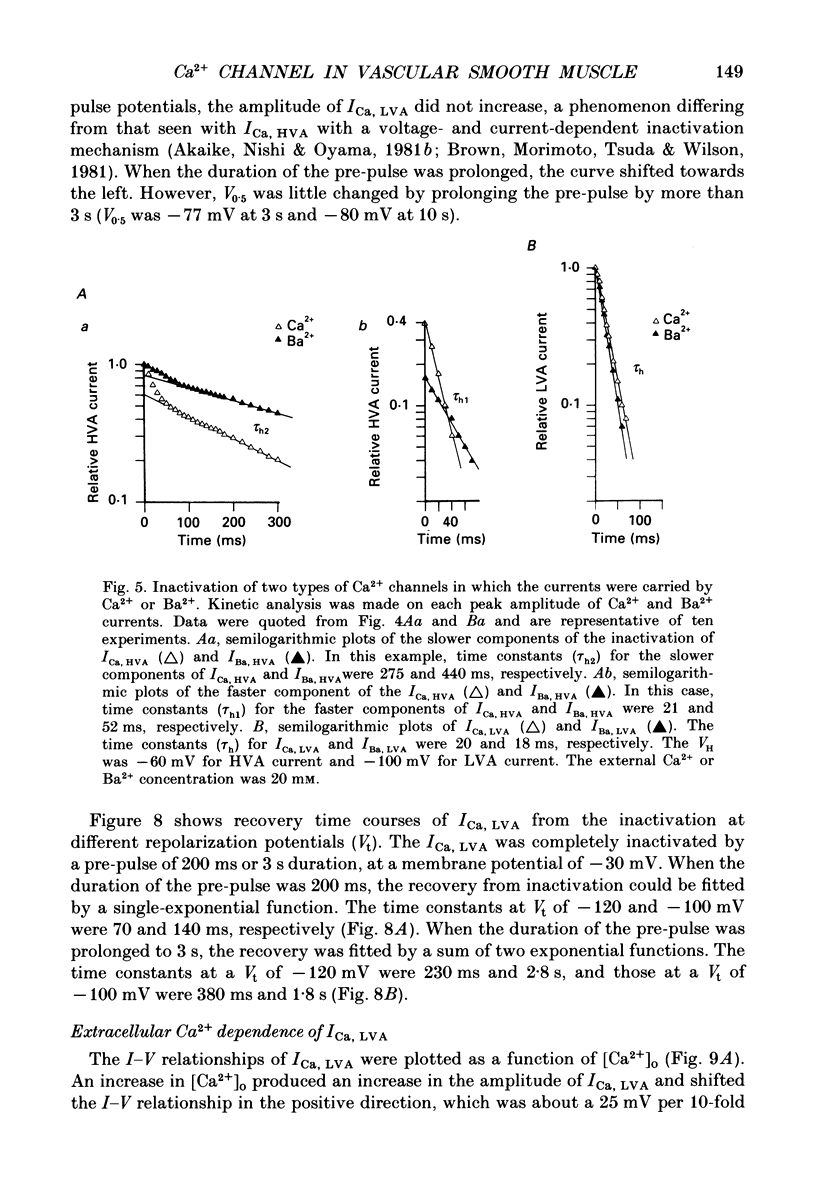
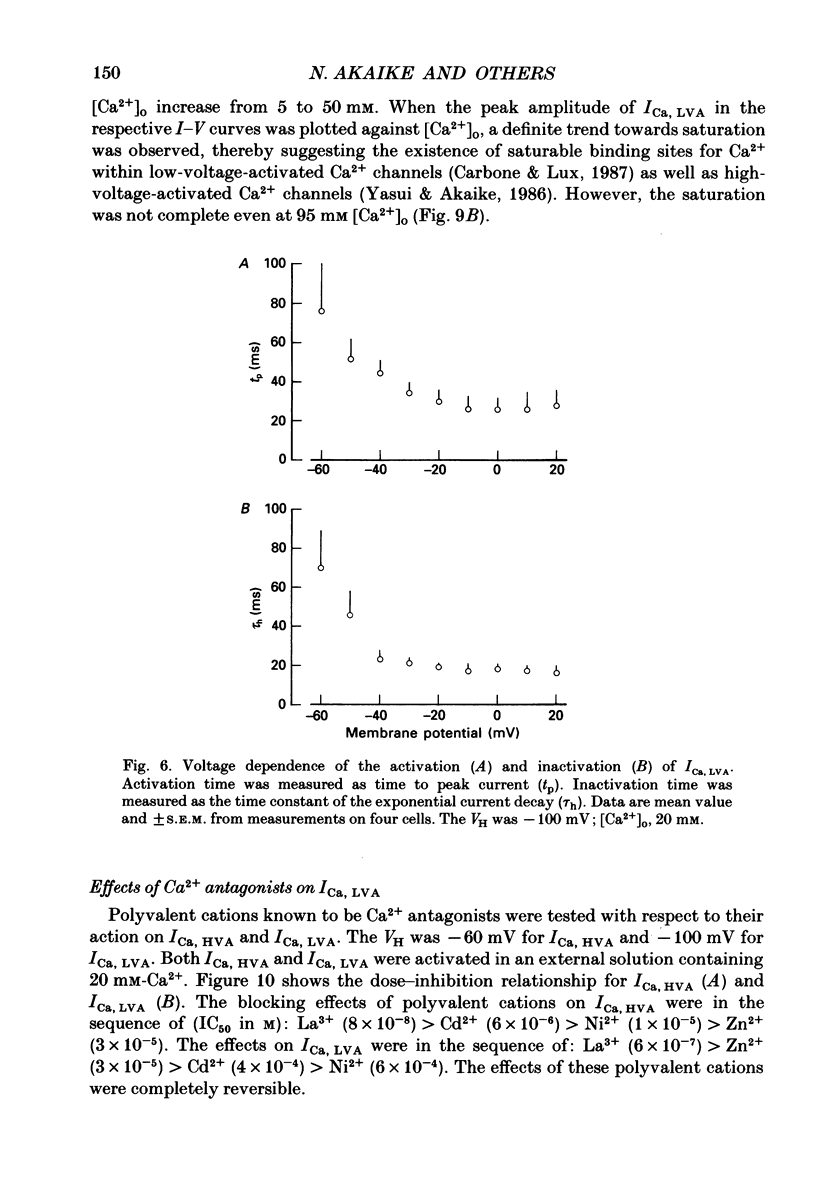
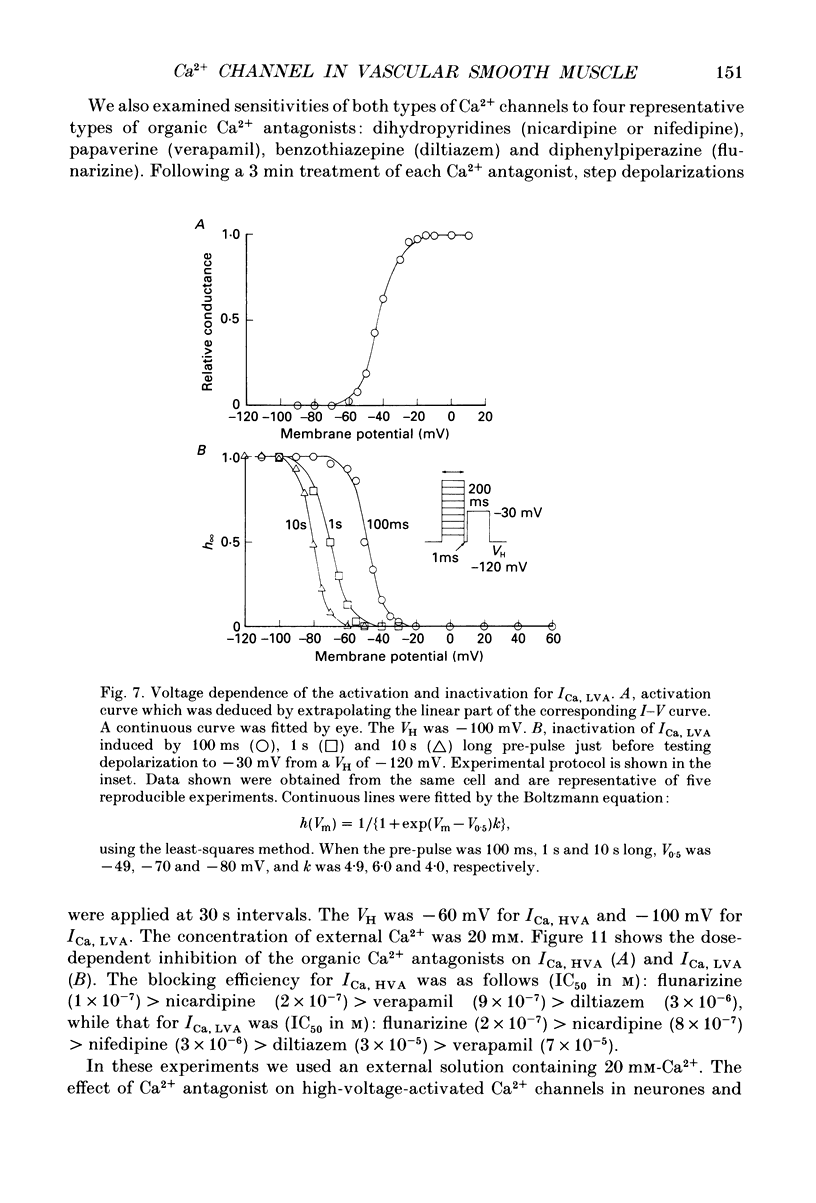
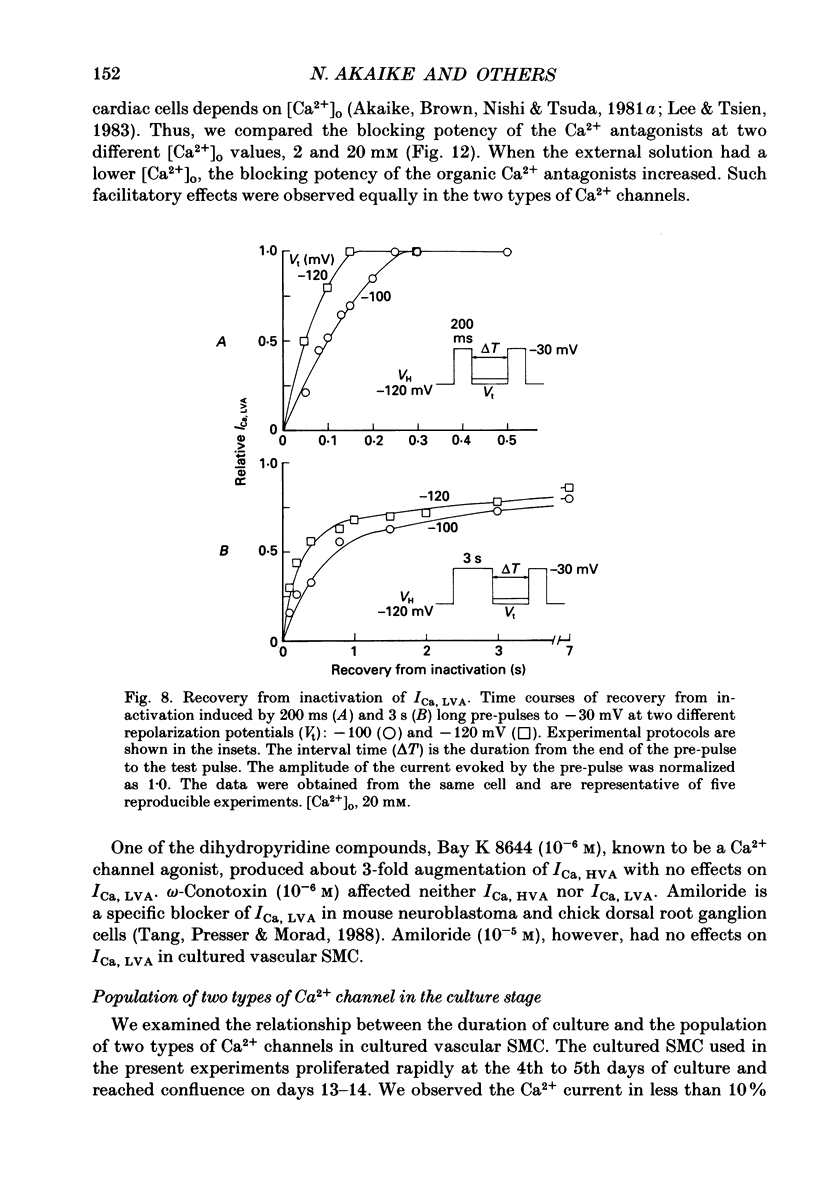
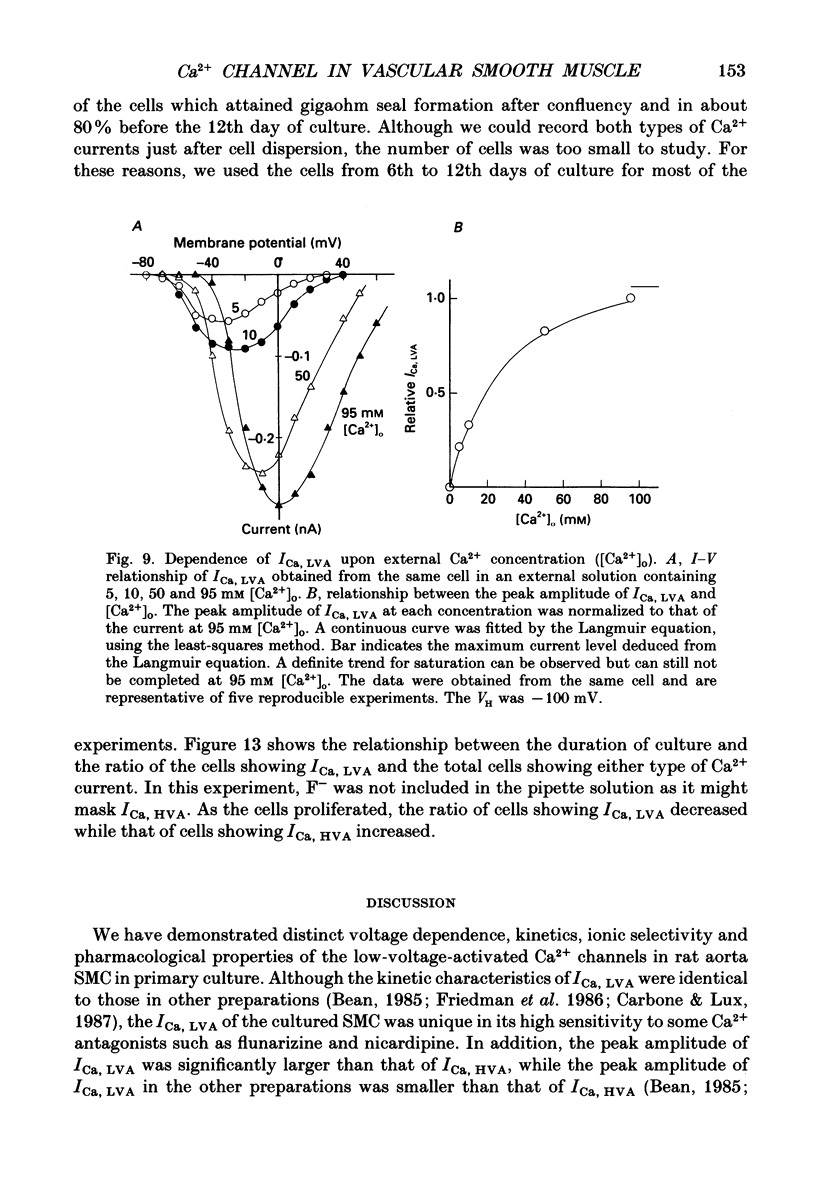
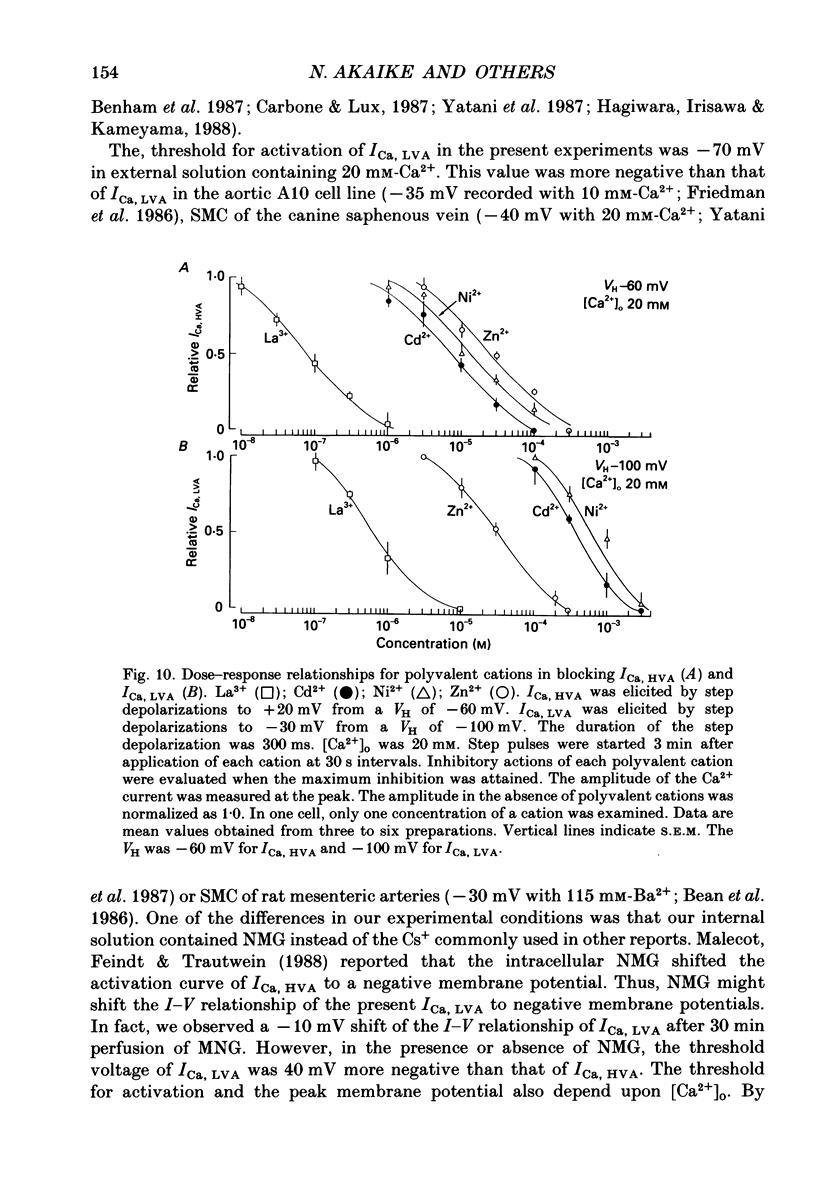
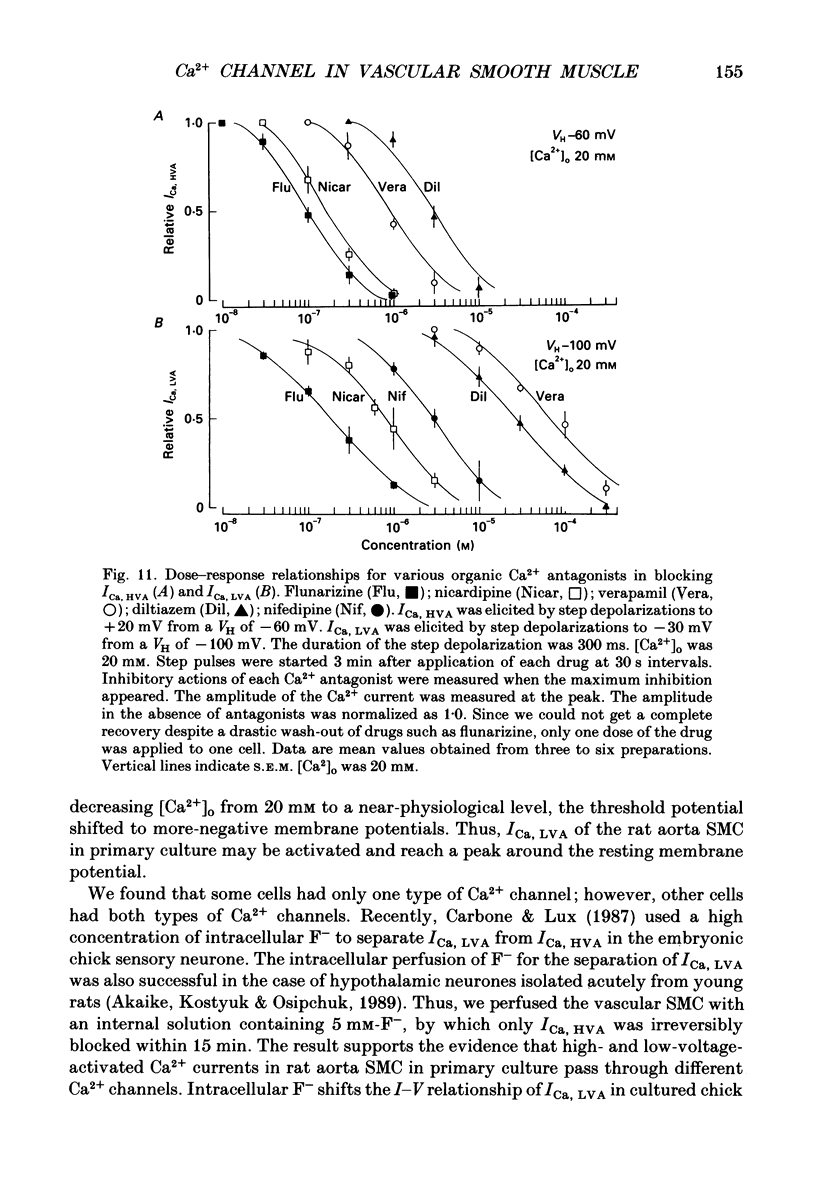
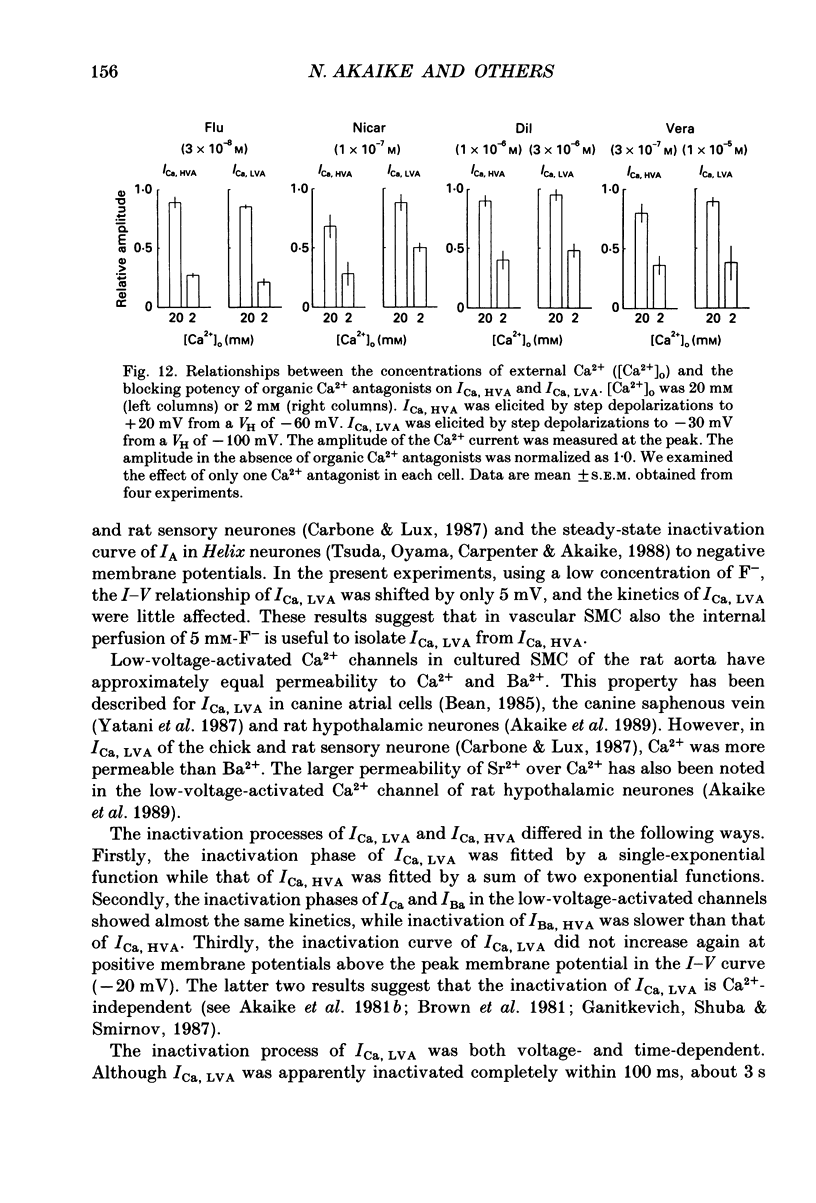
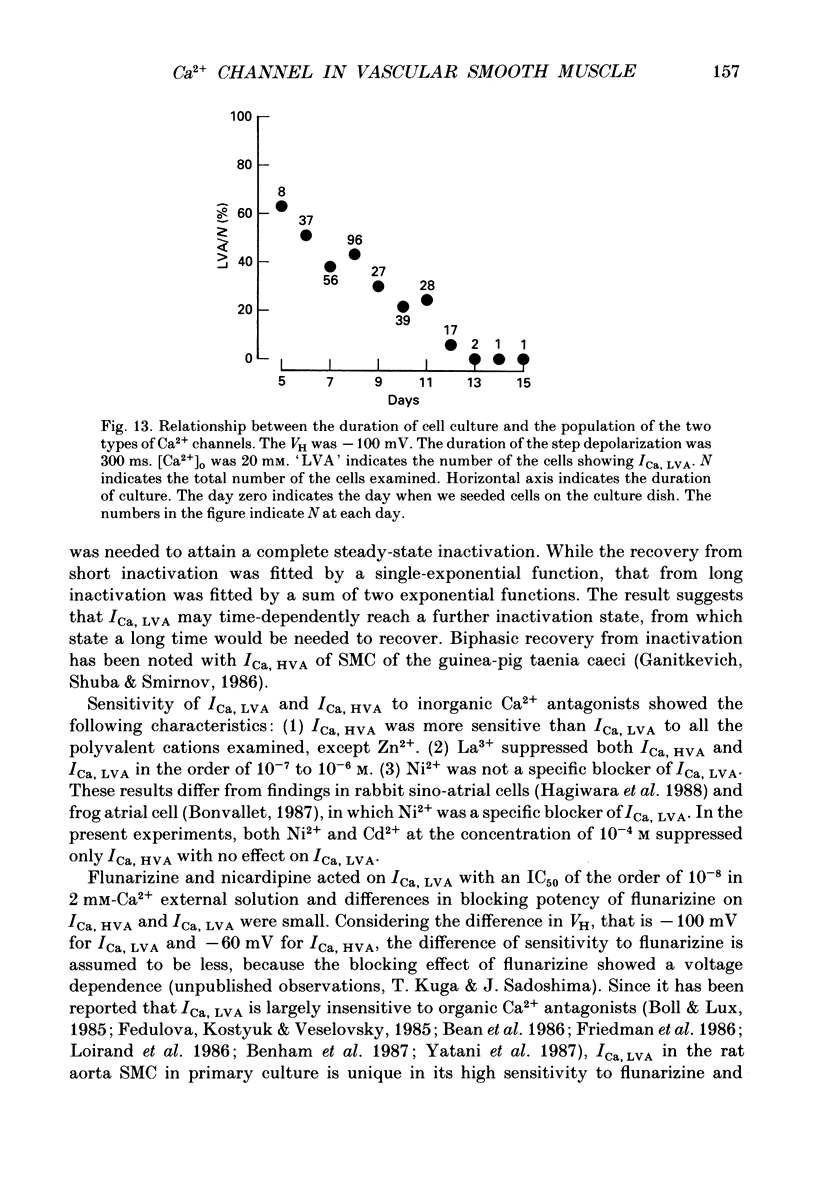
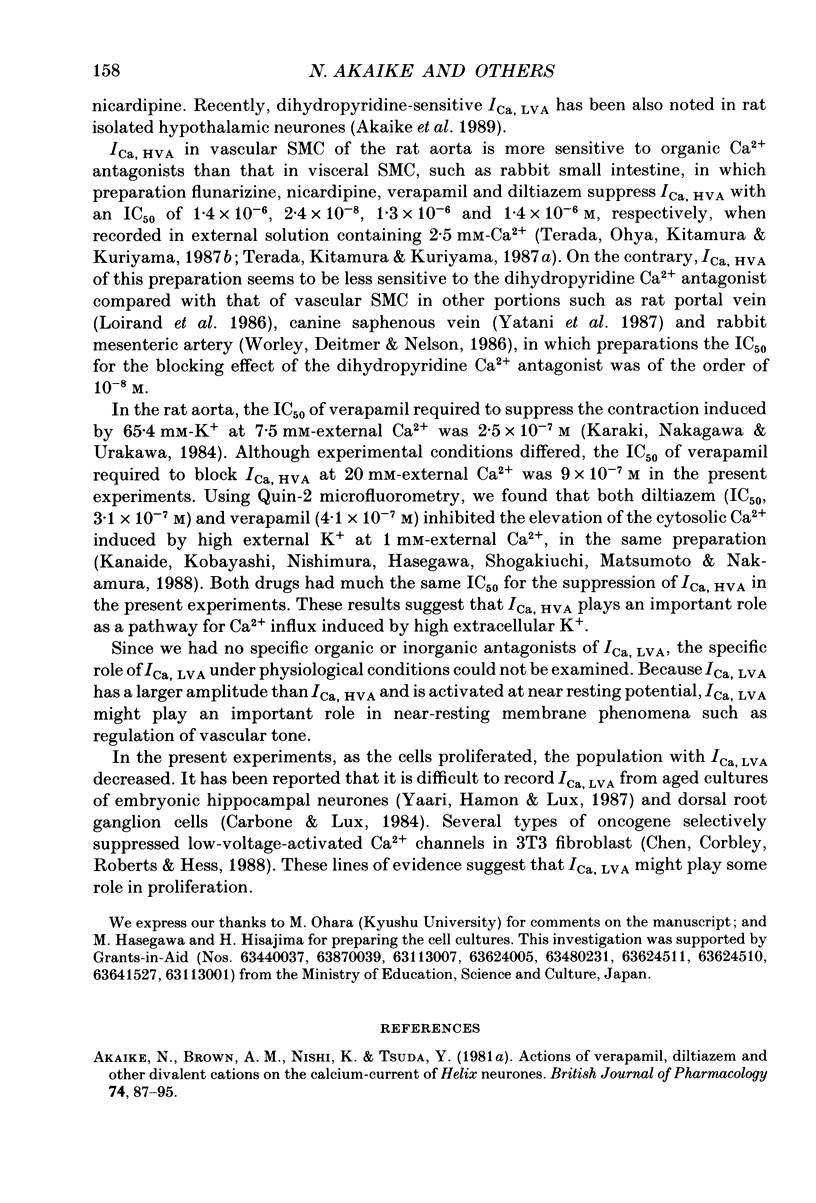
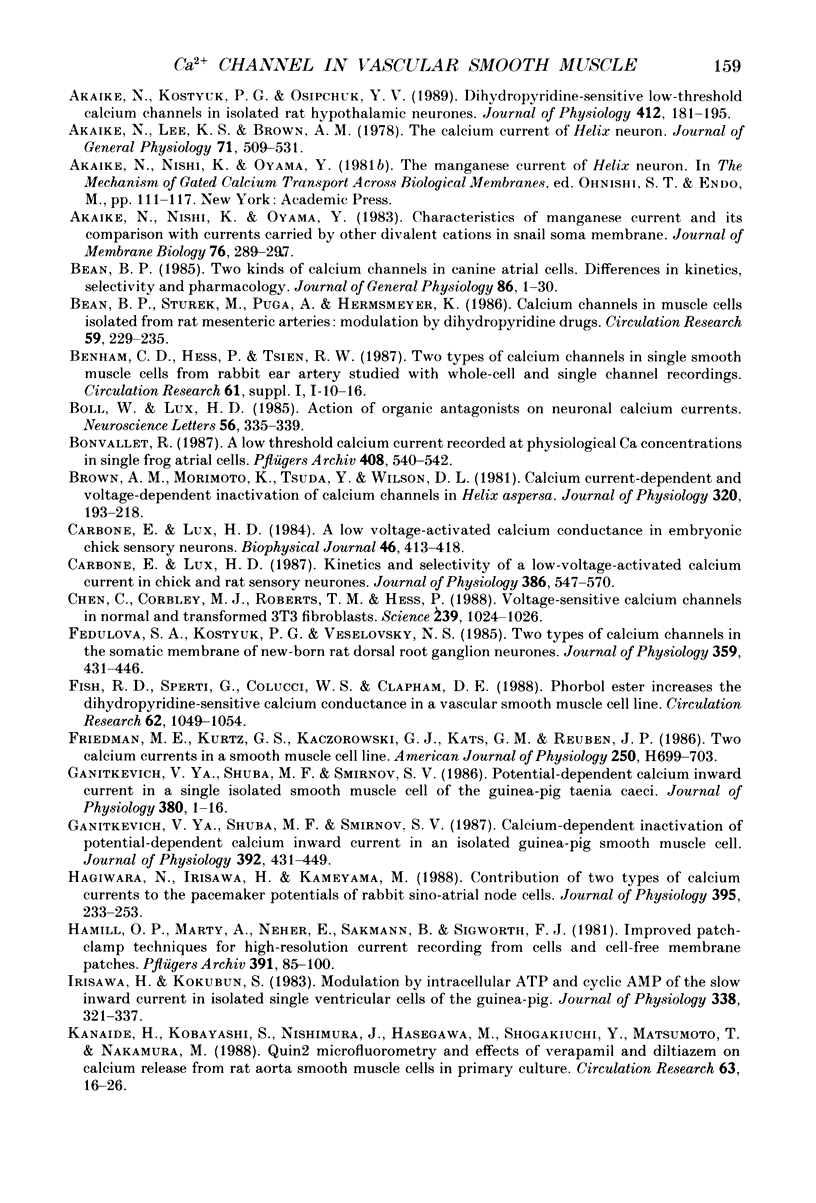
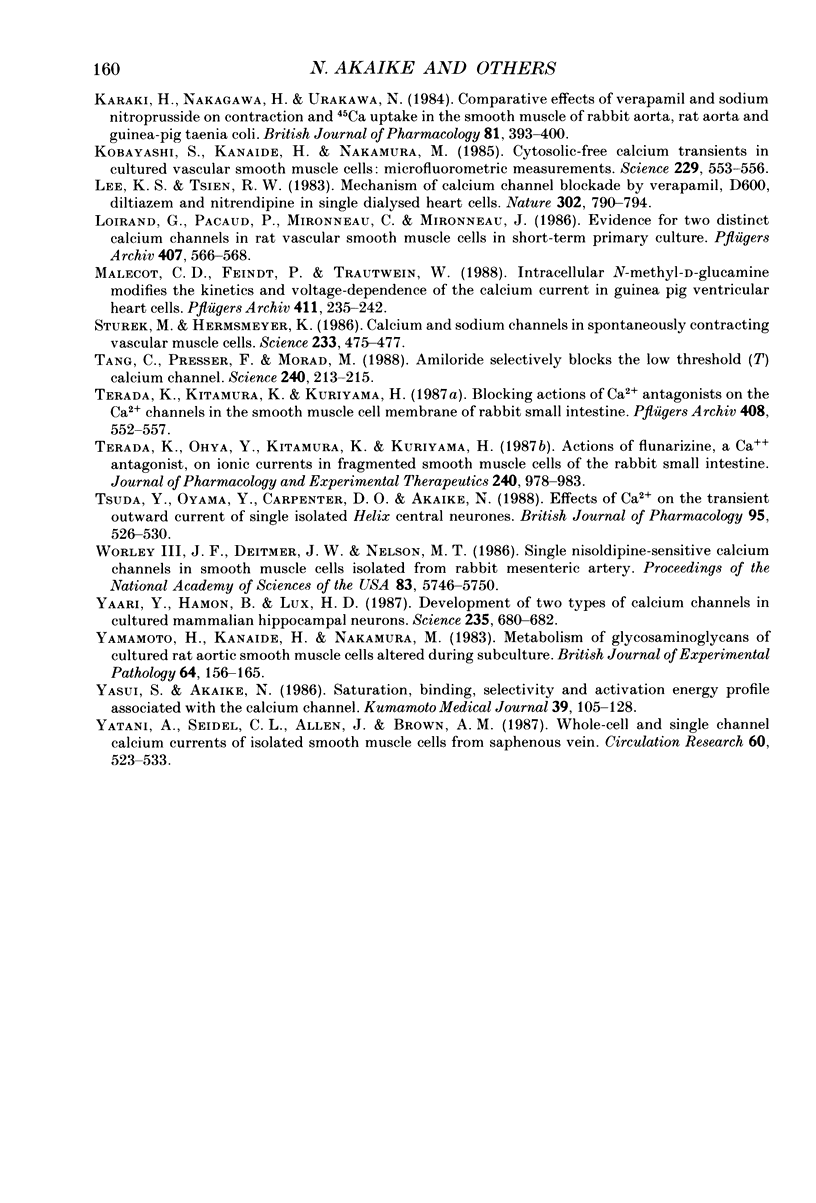
Selected References
These references are in PubMed. This may not be the complete list of references from this article.
- Akaike N., Brown A. M., Nishi K., Tsuda Y. Actions of verapamil, diltiazem and other divalent cations on the calcium-current of Helix neurones. Br J Pharmacol. 1981 Sep;74(1):87–95. doi: 10.1111/j.1476-5381.1981.tb09958.x. [DOI] [PMC free article] [PubMed] [Google Scholar]
- Akaike N., Kostyuk P. G., Osipchuk Y. V. Dihydropyridine-sensitive low-threshold calcium channels in isolated rat hypothalamic neurones. J Physiol. 1989 May;412:181–195. doi: 10.1113/jphysiol.1989.sp017610. [DOI] [PMC free article] [PubMed] [Google Scholar]
- Akaike N., Lee K. S., Brown A. M. The calcium current of Helix neuron. J Gen Physiol. 1978 May;71(5):509–531. doi: 10.1085/jgp.71.5.509. [DOI] [PMC free article] [PubMed] [Google Scholar]
- Bean B. P., Sturek M., Puga A., Hermsmeyer K. Calcium channels in muscle cells isolated from rat mesenteric arteries: modulation by dihydropyridine drugs. Circ Res. 1986 Aug;59(2):229–235. doi: 10.1161/01.res.59.2.229. [DOI] [PubMed] [Google Scholar]
- Bean B. P. Two kinds of calcium channels in canine atrial cells. Differences in kinetics, selectivity, and pharmacology. J Gen Physiol. 1985 Jul;86(1):1–30. doi: 10.1085/jgp.86.1.1. [DOI] [PMC free article] [PubMed] [Google Scholar]
- Boll W., Lux H. D. Action of organic antagonists on neuronal calcium currents. Neurosci Lett. 1985 May 23;56(3):335–339. doi: 10.1016/0304-3940(85)90265-4. [DOI] [PubMed] [Google Scholar]
- Bonvallet R. A low threshold calcium current recorded at physiological Ca concentrations in single frog atrial cells. Pflugers Arch. 1987 May;408(5):540–542. doi: 10.1007/BF00585084. [DOI] [PubMed] [Google Scholar]
- Brown A. M., Morimoto K., Tsuda Y., wilson D. L. Calcium current-dependent and voltage-dependent inactivation of calcium channels in Helix aspersa. J Physiol. 1981 Nov;320:193–218. doi: 10.1113/jphysiol.1981.sp013944. [DOI] [PMC free article] [PubMed] [Google Scholar]
- Carbone E., Lux H. D. A low voltage-activated calcium conductance in embryonic chick sensory neurons. Biophys J. 1984 Sep;46(3):413–418. doi: 10.1016/S0006-3495(84)84037-0. [DOI] [PMC free article] [PubMed] [Google Scholar]
- Carbone E., Lux H. D. Kinetics and selectivity of a low-voltage-activated calcium current in chick and rat sensory neurones. J Physiol. 1987 May;386:547–570. doi: 10.1113/jphysiol.1987.sp016551. [DOI] [PMC free article] [PubMed] [Google Scholar]
- Chen C. F., Corbley M. J., Roberts T. M., Hess P. Voltage-sensitive calcium channels in normal and transformed 3T3 fibroblasts. Science. 1988 Feb 26;239(4843):1024–1026. doi: 10.1126/science.2449730. [DOI] [PubMed] [Google Scholar]
- Fedulova S. A., Kostyuk P. G., Veselovsky N. S. Two types of calcium channels in the somatic membrane of new-born rat dorsal root ganglion neurones. J Physiol. 1985 Feb;359:431–446. doi: 10.1113/jphysiol.1985.sp015594. [DOI] [PMC free article] [PubMed] [Google Scholar]
- Fish R. D., Sperti G., Colucci W. S., Clapham D. E. Phorbol ester increases the dihydropyridine-sensitive calcium conductance in a vascular smooth muscle cell line. Circ Res. 1988 May;62(5):1049–1054. doi: 10.1161/01.res.62.5.1049. [DOI] [PubMed] [Google Scholar]
- Friedman M. E., Suarez-Kurtz G., Kaczorowski G. J., Katz G. M., Reuben J. P. Two calcium currents in a smooth muscle cell line. Am J Physiol. 1986 Apr;250(4 Pt 2):H699–H703. doi: 10.1152/ajpheart.1986.250.4.H699. [DOI] [PubMed] [Google Scholar]
- Ganitkevich VYa, Shuba M. F., Smirnov S. V. Calcium-dependent inactivation of potential-dependent calcium inward current in an isolated guinea-pig smooth muscle cell. J Physiol. 1987 Nov;392:431–449. doi: 10.1113/jphysiol.1987.sp016789. [DOI] [PMC free article] [PubMed] [Google Scholar]
- Ganitkevich VYa, Shuba M. F., Smirnov S. V. Potential-dependent calcium inward current in a single isolated smooth muscle cell of the guinea-pig taenia caeci. J Physiol. 1986 Nov;380:1–16. doi: 10.1113/jphysiol.1986.sp016268. [DOI] [PMC free article] [PubMed] [Google Scholar]
- Hagiwara N., Irisawa H., Kameyama M. Contribution of two types of calcium currents to the pacemaker potentials of rabbit sino-atrial node cells. J Physiol. 1988 Jan;395:233–253. doi: 10.1113/jphysiol.1988.sp016916. [DOI] [PMC free article] [PubMed] [Google Scholar]
- Hamill O. P., Marty A., Neher E., Sakmann B., Sigworth F. J. Improved patch-clamp techniques for high-resolution current recording from cells and cell-free membrane patches. Pflugers Arch. 1981 Aug;391(2):85–100. doi: 10.1007/BF00656997. [DOI] [PubMed] [Google Scholar]
- Irisawa H., Kokubun S. Modulation by intracellular ATP and cyclic AMP of the slow inward current in isolated single ventricular cells of the guinea-pig. J Physiol. 1983 May;338:321–337. doi: 10.1113/jphysiol.1983.sp014675. [DOI] [PMC free article] [PubMed] [Google Scholar]
- Kanaide H., Kobayashi S., Nishimura J., Hasegawa M., Shogakiuchi Y., Matsumoto T., Nakamura M. Quin2 microfluorometry and effects of verapamil and diltiazem on calcium release from rat aorta smooth muscle cells in primary culture. Circ Res. 1988 Jul;63(1):16–26. doi: 10.1161/01.res.63.1.16. [DOI] [PubMed] [Google Scholar]
- Karaki H., Nakagawa H., Urakawa N. Comparative effects of verapamil and sodium nitroprusside on contraction and 45Ca uptake in the smooth muscle of rabbit aorta, rat aorta and guinea-pig taenia coli. Br J Pharmacol. 1984 Feb;81(2):393–400. doi: 10.1111/j.1476-5381.1984.tb10091.x. [DOI] [PMC free article] [PubMed] [Google Scholar]
- Kobayashi S., Kanaide H., Nakamura M. Cytosolic-free calcium transients in cultured vascular smooth muscle cells: microfluorometric measurements. Science. 1985 Aug 9;229(4713):553–556. doi: 10.1126/science.3927484. [DOI] [PubMed] [Google Scholar]
- Lee K. S., Tsien R. W. Mechanism of calcium channel blockade by verapamil, D600, diltiazem and nitrendipine in single dialysed heart cells. Nature. 1983 Apr 28;302(5911):790–794. doi: 10.1038/302790a0. [DOI] [PubMed] [Google Scholar]
- Loirand G., Pacaud P., Mironneau C., Mironneau J. Evidence for two distinct calcium channels in rat vascular smooth muscle cells in short-term primary culture. Pflugers Arch. 1986 Nov;407(5):566–568. doi: 10.1007/BF00657519. [DOI] [PubMed] [Google Scholar]
- Malécot C. O., Feindt P., Trautwein W. Intracellular N-methyl-D-glucamine modifies the kinetics and voltage-dependence of the calcium current in guinea pig ventricular heart cells. Pflugers Arch. 1988 Mar;411(3):235–242. doi: 10.1007/BF00585109. [DOI] [PubMed] [Google Scholar]
- Sturek M., Hermsmeyer K. Calcium and sodium channels in spontaneously contracting vascular muscle cells. Science. 1986 Jul 25;233(4762):475–478. doi: 10.1126/science.2425434. [DOI] [PubMed] [Google Scholar]
- Tang C. M., Presser F., Morad M. Amiloride selectively blocks the low threshold (T) calcium channel. Science. 1988 Apr 8;240(4849):213–215. doi: 10.1126/science.2451291. [DOI] [PubMed] [Google Scholar]
- Terada K., Kitamura K., Kuriyama H. Blocking actions of Ca2+ antagonists on the Ca2+ channels in the smooth muscle cell membrane of rabbit small intestine. Pflugers Arch. 1987 May;408(6):552–557. doi: 10.1007/BF00581155. [DOI] [PubMed] [Google Scholar]
- Terada K., Ohya Y., Kitamura K., Kuriyama H. Actions of flunarizine, a Ca++ antagonist, on ionic currents in fragmented smooth muscle cells of the rabbit small intestine. J Pharmacol Exp Ther. 1987 Mar;240(3):978–983. [PubMed] [Google Scholar]
- Tsuda Y., Oyama Y., Carpenter D. O., Akaike N. Effects of Ca2+ on the transient outward current of single isolated Helix central neurones. Br J Pharmacol. 1988 Oct;95(2):526–530. doi: 10.1111/j.1476-5381.1988.tb11673.x. [DOI] [PMC free article] [PubMed] [Google Scholar]
- Worley J. F., 3rd, Deitmer J. W., Nelson M. T. Single nisoldipine-sensitive calcium channels in smooth muscle cells isolated from rabbit mesenteric artery. Proc Natl Acad Sci U S A. 1986 Aug;83(15):5746–5750. doi: 10.1073/pnas.83.15.5746. [DOI] [PMC free article] [PubMed] [Google Scholar]
- Yaari Y., Hamon B., Lux H. D. Development of two types of calcium channels in cultured mammalian hippocampal neurons. Science. 1987 Feb 6;235(4789):680–682. doi: 10.1126/science.2433765. [DOI] [PubMed] [Google Scholar]
- Yamamoto H., Kanaide H., Nakamura M. Metabolism of glycosaminoglycans of cultured rat aortic smooth muscle cells altered during subculture. Br J Exp Pathol. 1983 Apr;64(2):156–165. [PMC free article] [PubMed] [Google Scholar]
- Yatani A., Seidel C. L., Allen J., Brown A. M. Whole-cell and single-channel calcium currents of isolated smooth muscle cells from saphenous vein. Circ Res. 1987 Apr;60(4):523–533. doi: 10.1161/01.res.60.4.523. [DOI] [PubMed] [Google Scholar]