Abstract
1. Intracellular Na+ activity (aiNa) has been measured in Purkinje fibres from sheep heart and in ventricular muscle from rabbit heart during hypoxia and metabolic inhibition. The aiNa was measured using liquid sensor ion-sensitive microelectrodes. 2. Hypoxia, produced by replacement of O2 with N2 in the superfusate, produced an increase in aiNa. This increase was larger if sucrose replaced glucose in the superfusing Tyrode solution. The increase in aiNa was accompanied by a small depolarization. Upon reoxygenation aiNa decreased and cells rapidly repolarized. 3. When oxidative phosphorylation was inhibited by application of 2 mM-cyanide, aiNa increased. This increase was also accompanied by a small depolarization. Upon removal of cyanide, aiNa and membrane potential recovered to control levels. 4. After inhibiting glycolysis, by replacing glucose with 2-deoxy-D-glucose, inhibition of oxidative phosphorylation (by addition of cyanide or exposure to hypoxia) produced a much more rapid increase in aiNa and a large contracture. The rise in aiNa and the occurrence of a contracture could not be inhibited by application of amiloride (1 mM) or tetrodotoxin (1 microgram ml-1). Removal of cyanide or reoxygenation and replacement of glucose resulted in a rapid relaxation of the contracture and a slower decrease in aiNa. 5. The relative rates of increase in aiNa during metabolic inhibition were compared with the rate observed when Na+-K+-ATPase was inhibited by application of 10 mumols l-1 of the cardio-active steroid strophanthidin. The rate of increase of aiNa when both oxidative phosphorylation and glycolysis were inhibited was approximately twice that observed with only oxidative phosphorylation inhibited and approximately half that observed in the presence of 10 microM-strophanthidin. 6. Cyanide, applied when aiNa had been elevated (i.e. during exposure to 10 microM-strophanthidin to inhibit Na+-K+-ATPase), did not produce a contracture. The contracture observed in the presence of cyanide and 2-deoxy-D-glucose still occurred when Ca2+ was removed from the superfusate.
Full text
PDF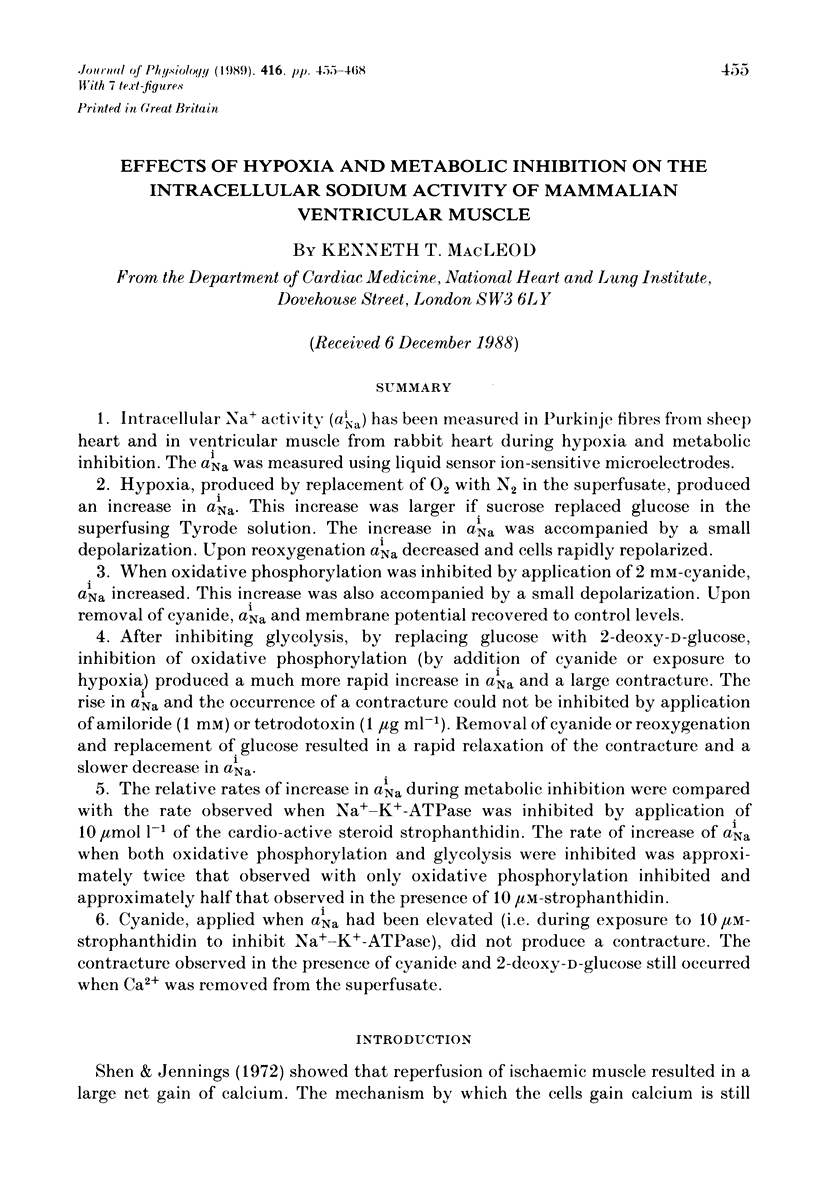
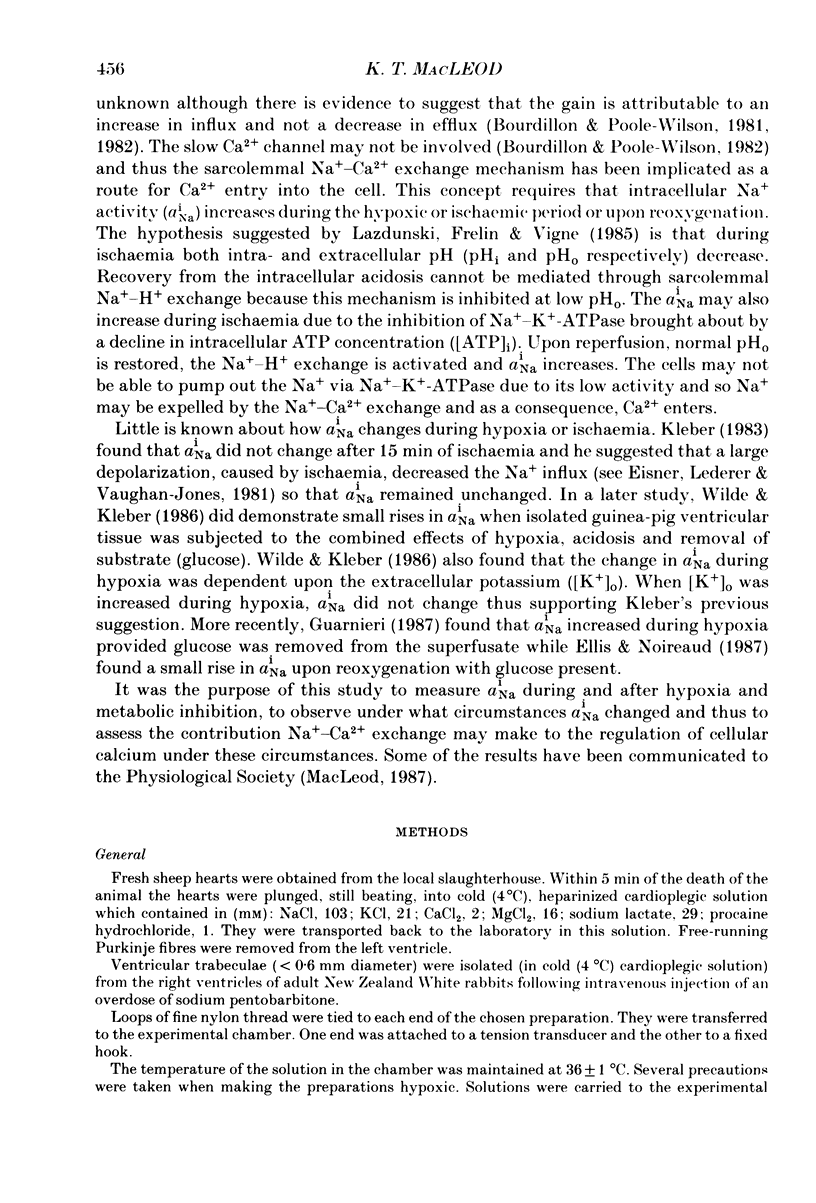
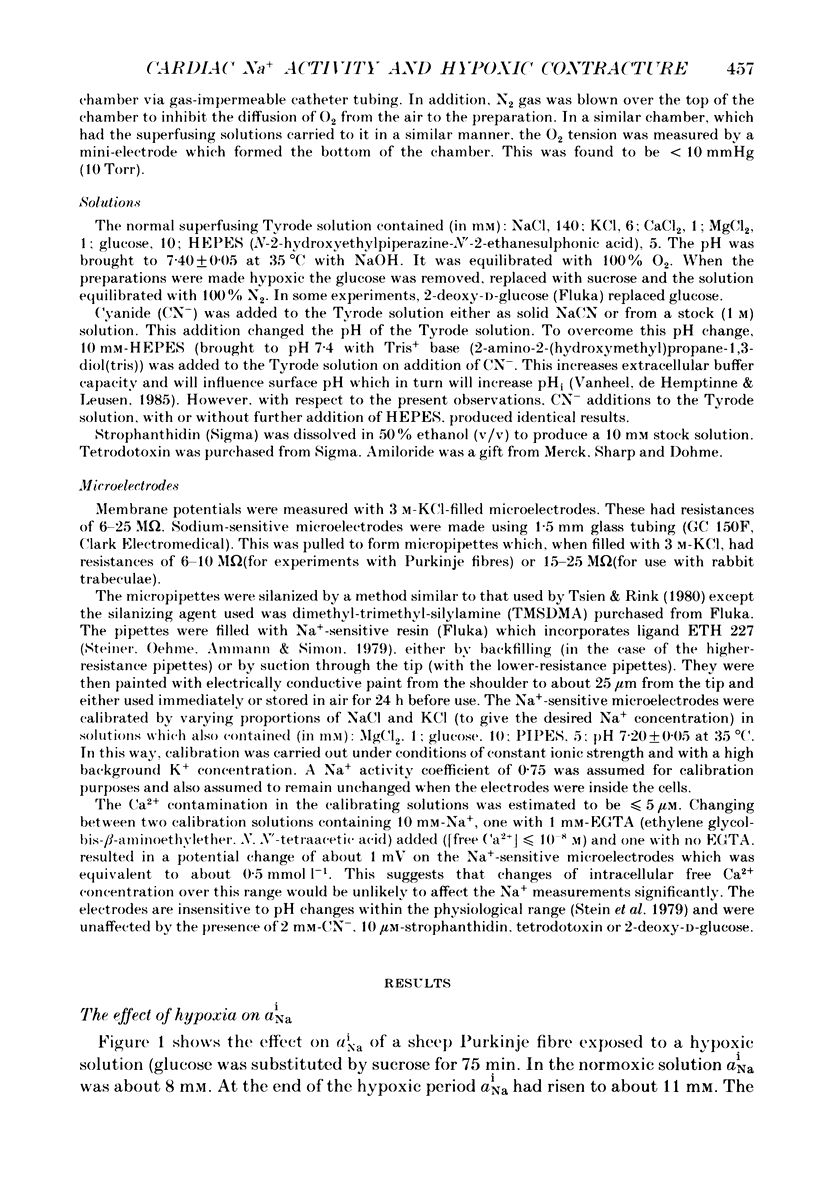
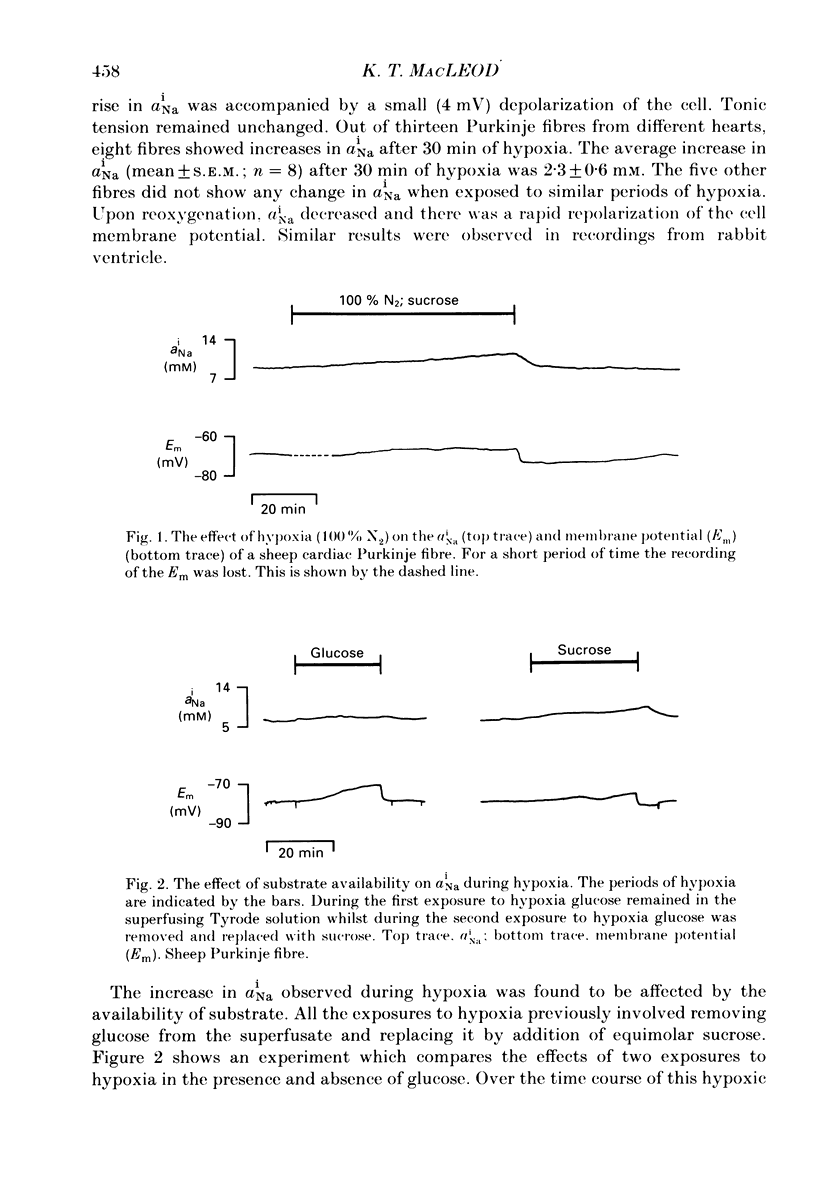
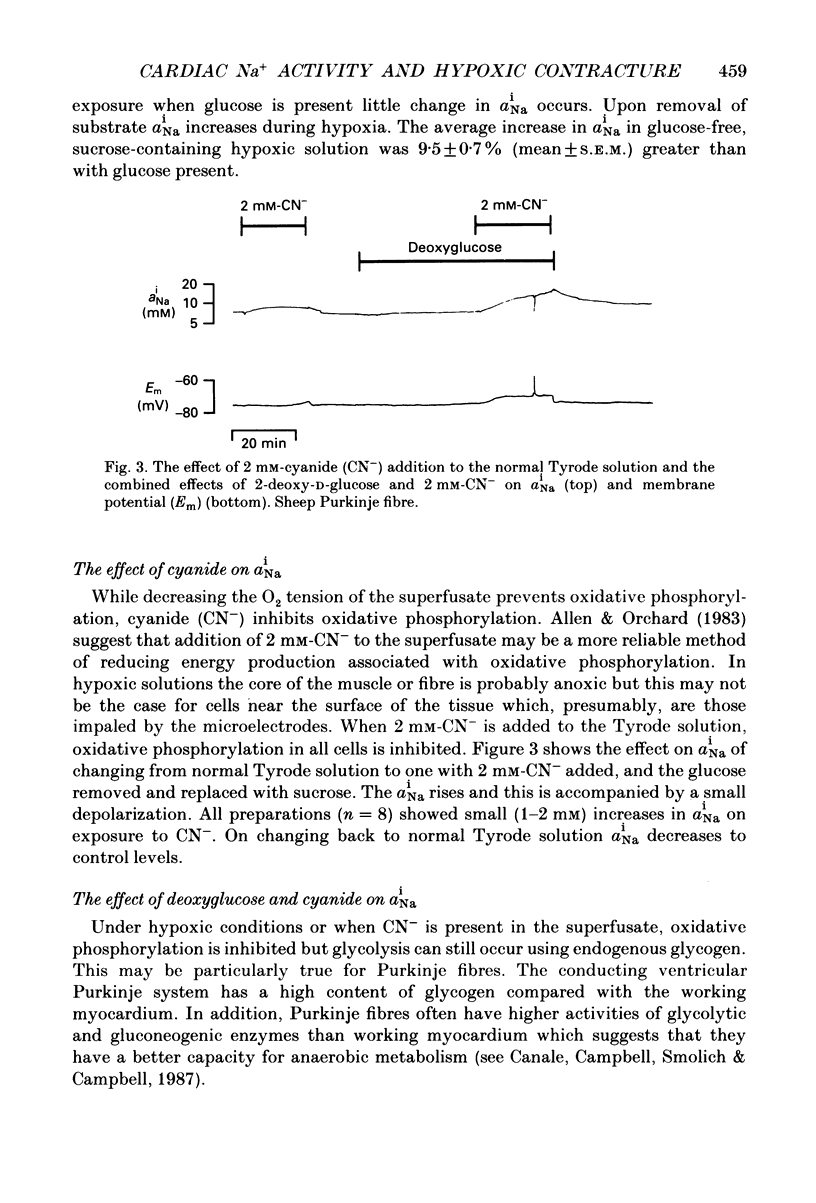
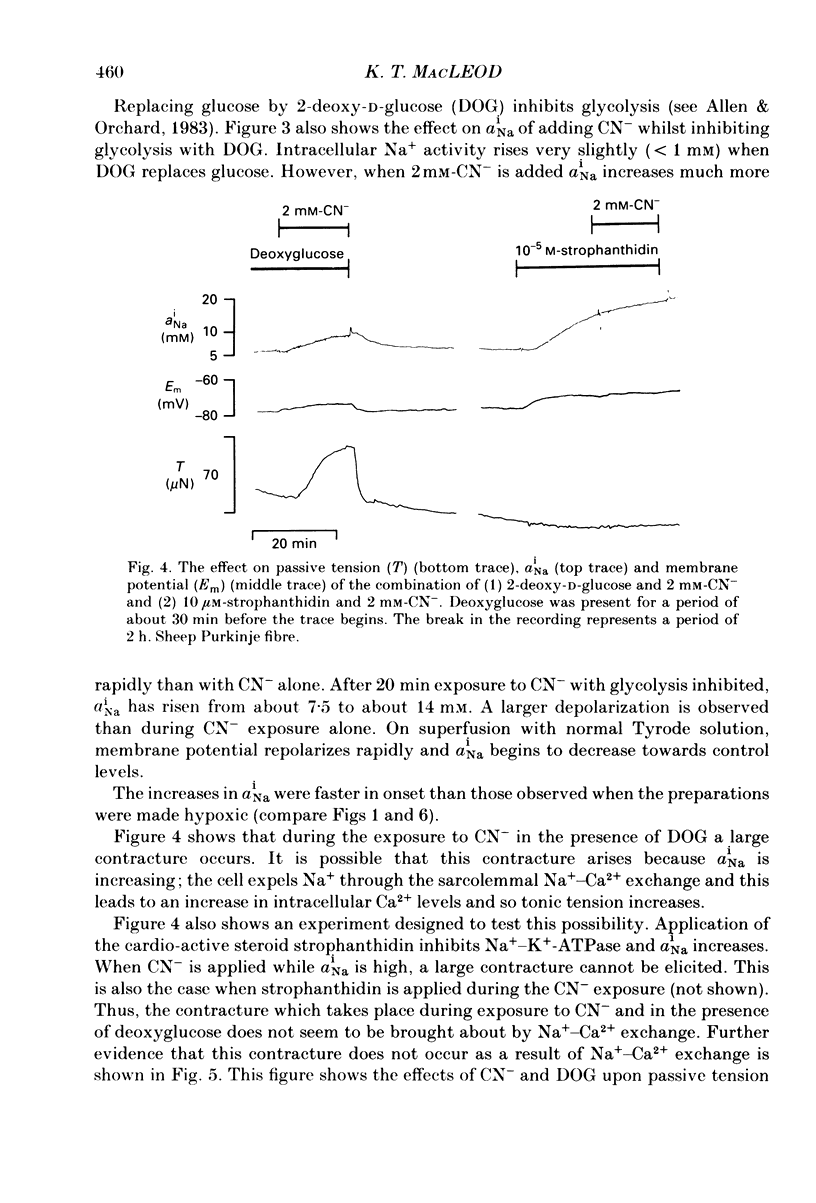
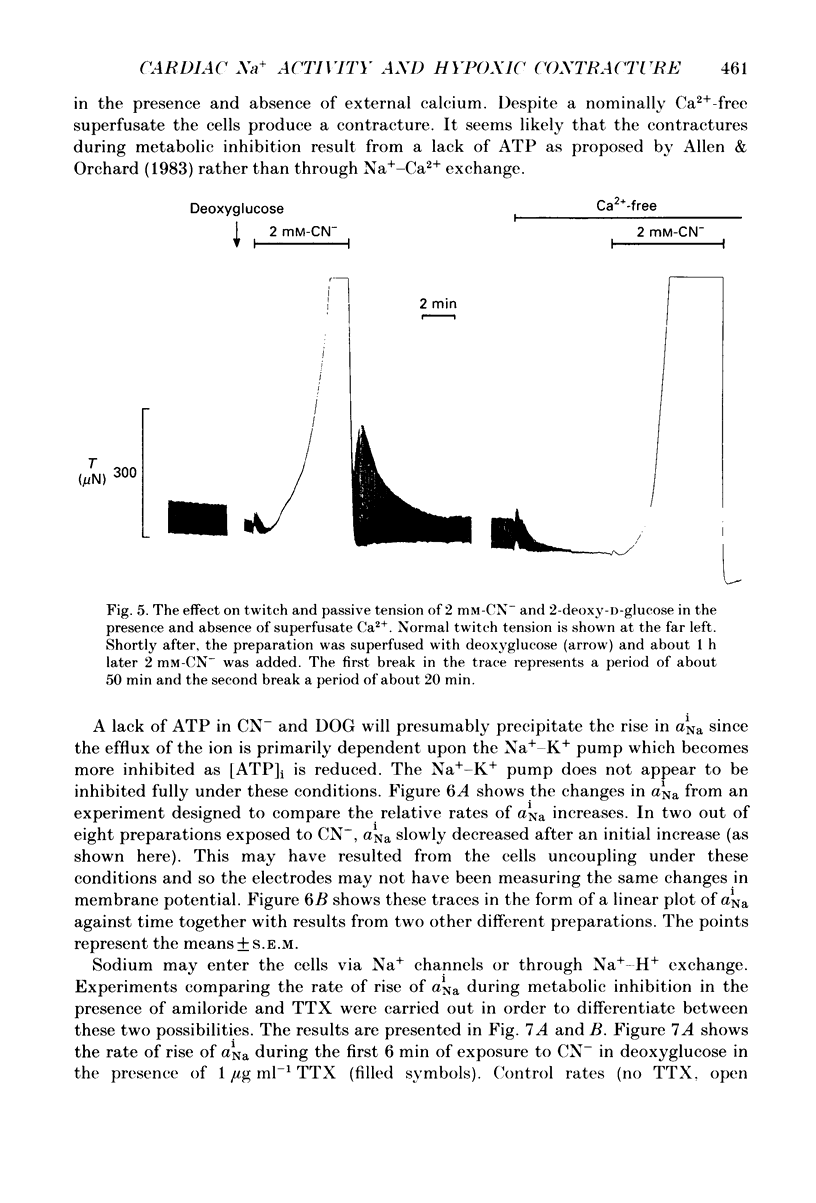
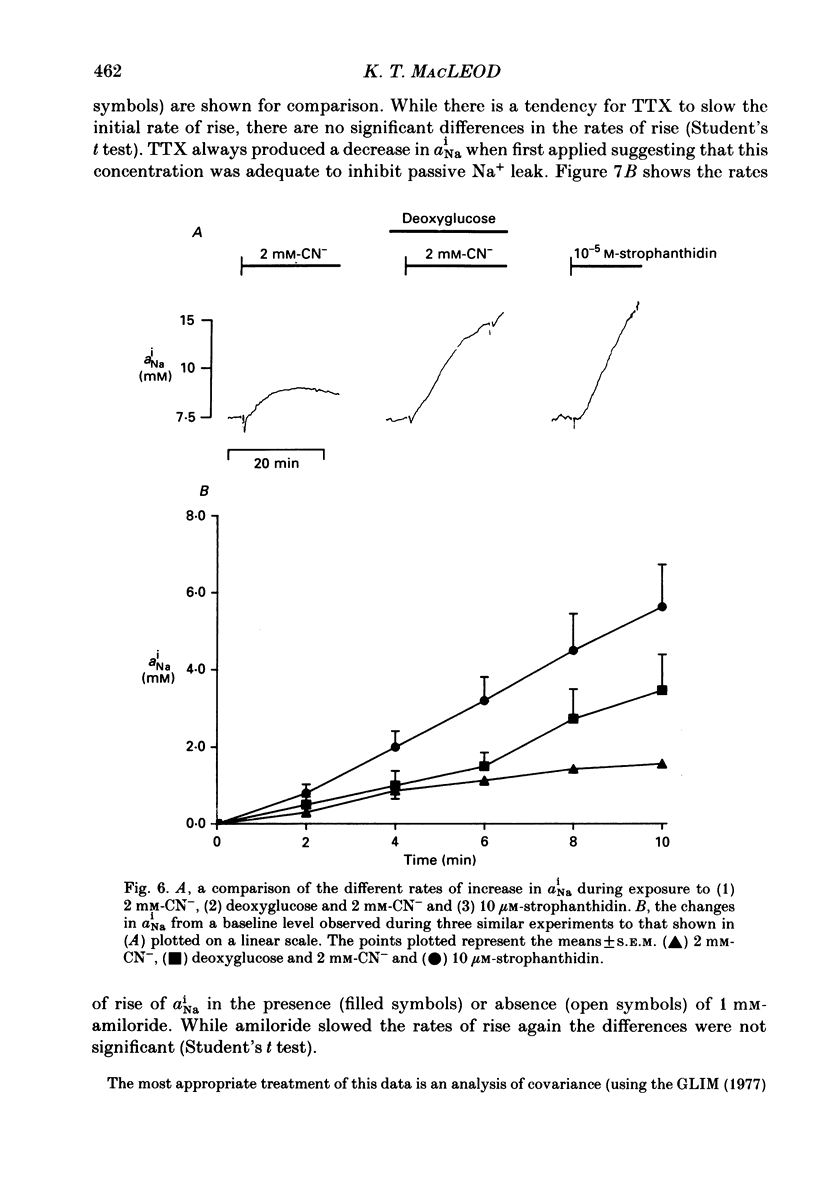
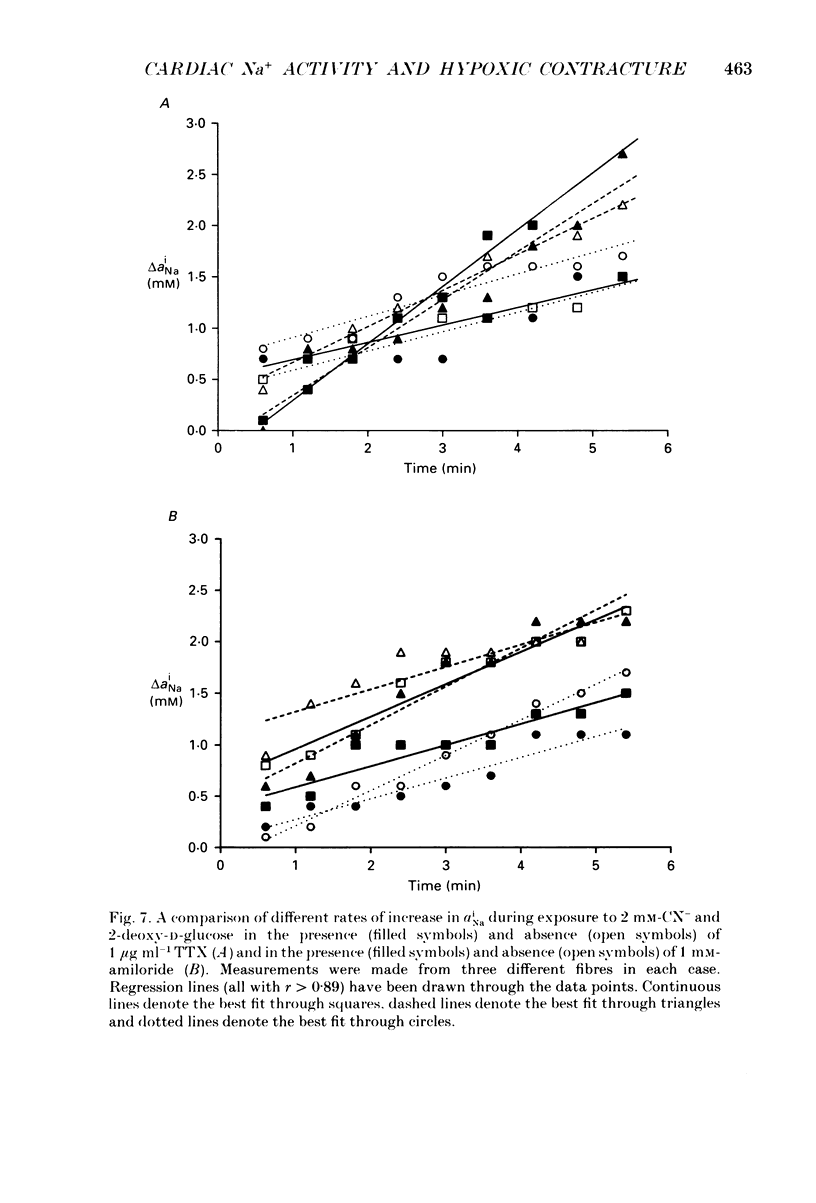
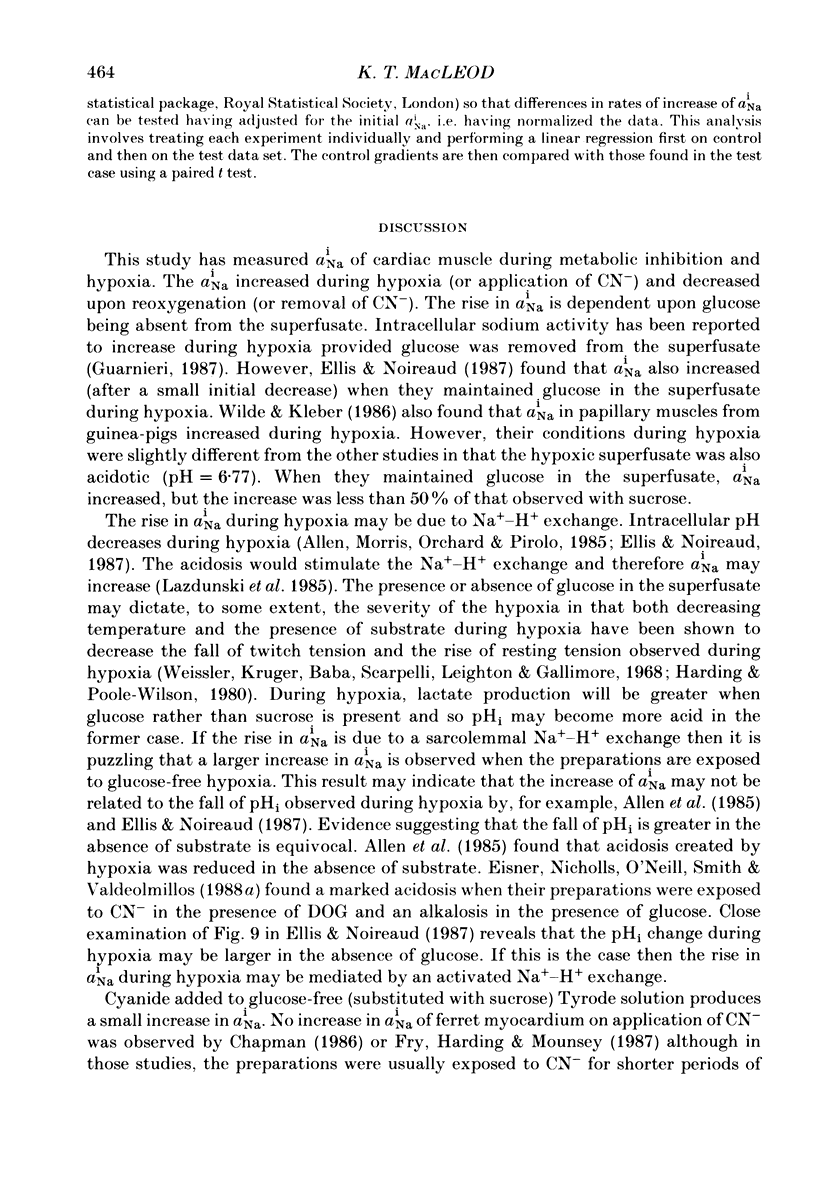
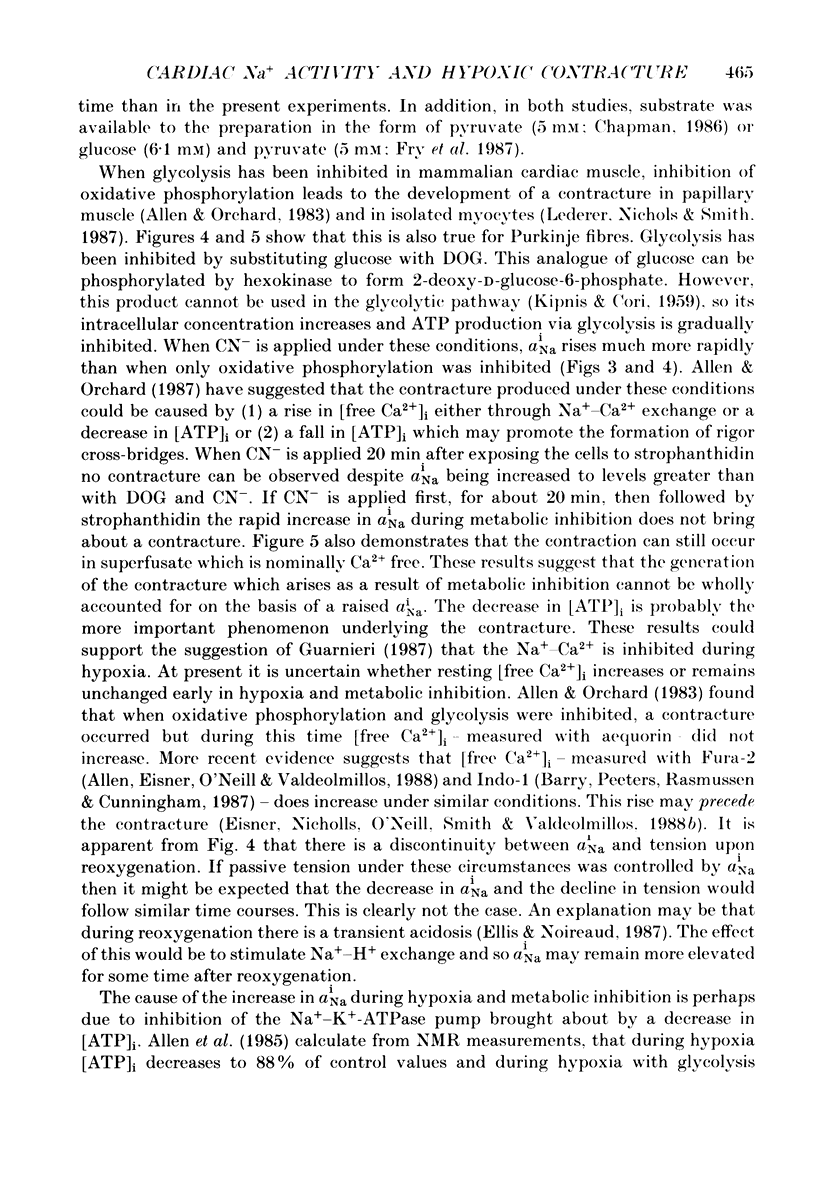
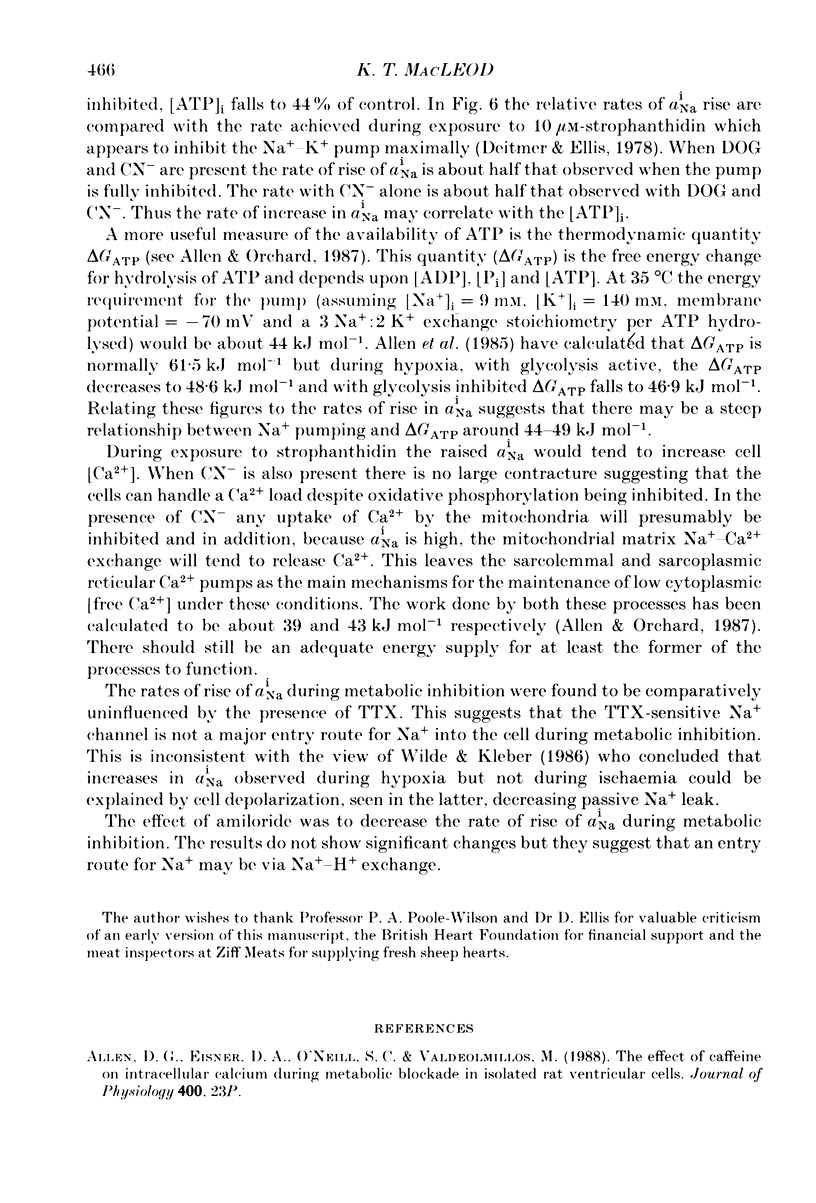
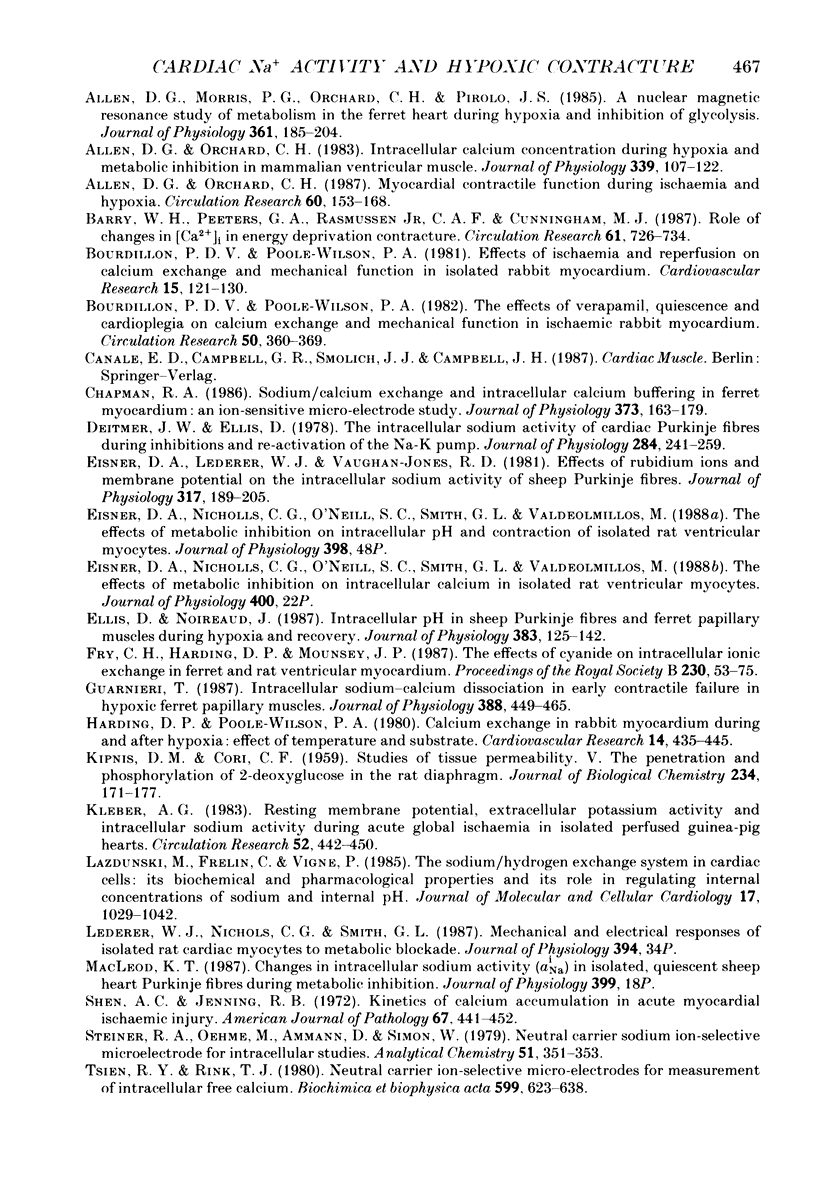
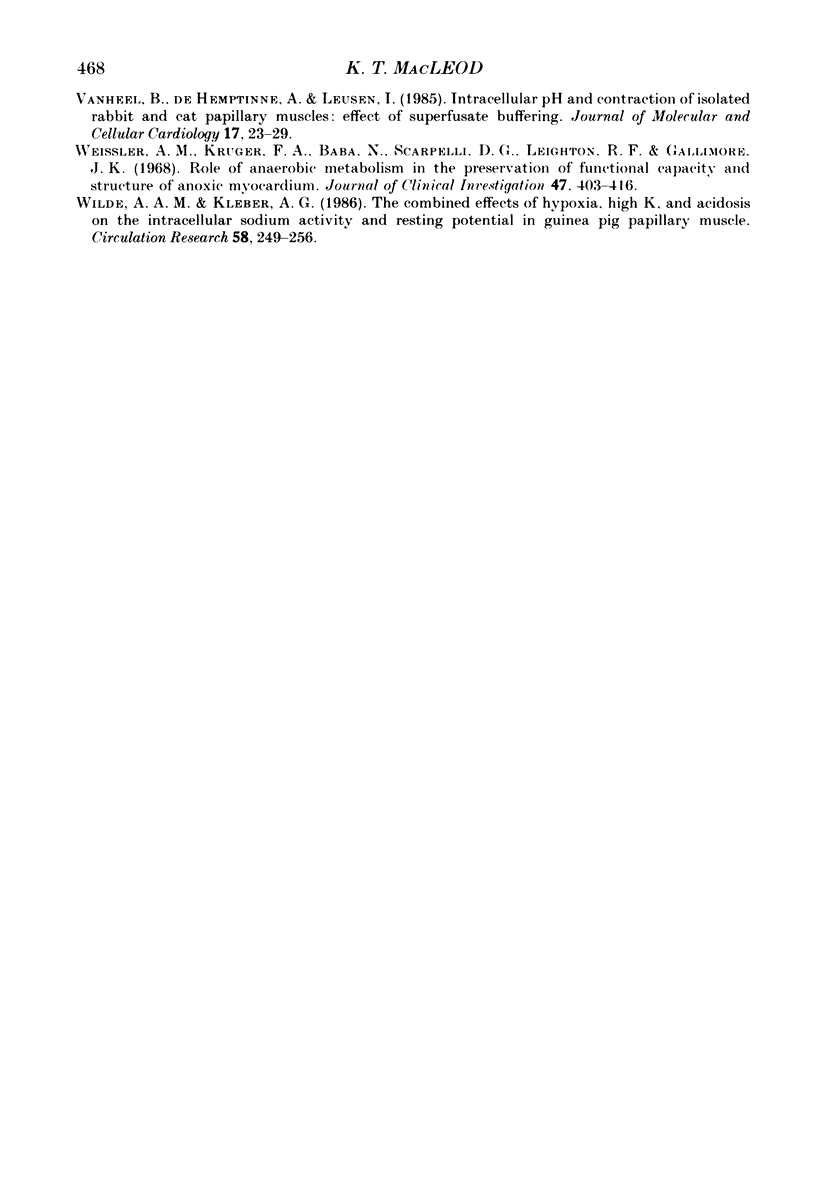
Selected References
These references are in PubMed. This may not be the complete list of references from this article.
- Allen D. G., Morris P. G., Orchard C. H., Pirolo J. S. A nuclear magnetic resonance study of metabolism in the ferret heart during hypoxia and inhibition of glycolysis. J Physiol. 1985 Apr;361:185–204. doi: 10.1113/jphysiol.1985.sp015640. [DOI] [PMC free article] [PubMed] [Google Scholar]
- Allen D. G., Orchard C. H. Intracellular calcium concentration during hypoxia and metabolic inhibition in mammalian ventricular muscle. J Physiol. 1983 Jun;339:107–122. doi: 10.1113/jphysiol.1983.sp014706. [DOI] [PMC free article] [PubMed] [Google Scholar]
- Allen D. G., Orchard C. H. Myocardial contractile function during ischemia and hypoxia. Circ Res. 1987 Feb;60(2):153–168. doi: 10.1161/01.res.60.2.153. [DOI] [PubMed] [Google Scholar]
- Barry W. H., Peeters G. A., Rasmussen C. A., Jr, Cunningham M. J. Role of changes in [Ca2+]i in energy deprivation contracture. Circ Res. 1987 Nov;61(5):726–734. doi: 10.1161/01.res.61.5.726. [DOI] [PubMed] [Google Scholar]
- Bourdillon P. D., Poole-Wilson P. A. Effects of ischaemia and reperfusion on calcium exchange and mechanical function in isolated rabbit myocardium. Cardiovasc Res. 1981 Mar;15(3):121–130. doi: 10.1093/cvr/15.3.121. [DOI] [PubMed] [Google Scholar]
- Bourdillon P. D., Poole-Wilson P. A. The effects of verapamil, quiescence, and cardioplegia on calcium exchange and mechanical function in ischemic rabbit myocardium. Circ Res. 1982 Mar;50(3):360–368. doi: 10.1161/01.res.50.3.360. [DOI] [PubMed] [Google Scholar]
- Chapman R. A. Sodium/calcium exchange and intracellular calcium buffering in ferret myocardium: an ion-sensitive micro-electrode study. J Physiol. 1986 Apr;373:163–179. doi: 10.1113/jphysiol.1986.sp016040. [DOI] [PMC free article] [PubMed] [Google Scholar]
- Deitmer J. W., Ellis D. The intracellular sodium activity of cardiac Purkinje fibres during inhibition and re-activation of the Na-K pump. J Physiol. 1978 Nov;284:241–259. doi: 10.1113/jphysiol.1978.sp012539. [DOI] [PMC free article] [PubMed] [Google Scholar]
- Eisner D. A., Lederer W. J., Vaughan-Jones R. D. The effects of rubidium ions and membrane potentials on the intracellular sodium activity of sheep Purkinje fibres. J Physiol. 1981 Aug;317:189–205. doi: 10.1113/jphysiol.1981.sp013820. [DOI] [PMC free article] [PubMed] [Google Scholar]
- Ellis D., Noireaud J. Intracellular pH in sheep Purkinje fibres and ferret papillary muscles during hypoxia and recovery. J Physiol. 1987 Feb;383:125–141. doi: 10.1113/jphysiol.1987.sp016400. [DOI] [PMC free article] [PubMed] [Google Scholar]
- Fry C. H., Harding D. P., Mounsey J. P. The effects of cyanide on intracellular ionic exchange in ferret and rat ventricular myocardium. Proc R Soc Lond B Biol Sci. 1987 Feb 23;230(1258):53–75. doi: 10.1098/rspb.1987.0009. [DOI] [PubMed] [Google Scholar]
- Guarnieri T. Intracellular sodium-calcium dissociation in early contractile failure in hypoxic ferret papillary muscles. J Physiol. 1987 Jul;388:449–465. doi: 10.1113/jphysiol.1987.sp016624. [DOI] [PMC free article] [PubMed] [Google Scholar]
- Harding D. P., Poole-Wilson P. A. Calcium exchange in rabbit myocardium during and after hypoxia: effect of temperature and substrate. Cardiovasc Res. 1980 Aug;14(8):435–445. doi: 10.1093/cvr/14.8.435. [DOI] [PubMed] [Google Scholar]
- KIPNIS D. M., CORI C. F. Studies of tissue permeability. V. The penetration and phosphorylation of 2-deoxyglucose in the rat diaphragm. J Biol Chem. 1959 Jan;234(1):171–177. [PubMed] [Google Scholar]
- Kléber A. G. Resting membrane potential, extracellular potassium activity, and intracellular sodium activity during acute global ischemia in isolated perfused guinea pig hearts. Circ Res. 1983 Apr;52(4):442–450. doi: 10.1161/01.res.52.4.442. [DOI] [PubMed] [Google Scholar]
- Lazdunski M., Frelin C., Vigne P. The sodium/hydrogen exchange system in cardiac cells: its biochemical and pharmacological properties and its role in regulating internal concentrations of sodium and internal pH. J Mol Cell Cardiol. 1985 Nov;17(11):1029–1042. doi: 10.1016/s0022-2828(85)80119-x. [DOI] [PubMed] [Google Scholar]
- Shen A. C., Jennings R. B. Kinetics of calcium accumulation in acute myocardial ischemic injury. Am J Pathol. 1972 Jun;67(3):441–452. [PMC free article] [PubMed] [Google Scholar]
- Tsien R. Y., Rink T. J. Neutral carrier ion-selective microelectrodes for measurement of intracellular free calcium. Biochim Biophys Acta. 1980 Jul;599(2):623–638. doi: 10.1016/0005-2736(80)90205-9. [DOI] [PubMed] [Google Scholar]
- Vanheel B., de Hemptinne A., Leusen I. Intracellular pH and contraction of isolated rabbit and cat papillary muscle: effect of superfusate buffering. J Mol Cell Cardiol. 1985 Jan;17(1):23–29. doi: 10.1016/s0022-2828(85)80089-4. [DOI] [PubMed] [Google Scholar]
- Weissler A. M., Kruger F. A., Baba N., Scarpelli D. G., Leighton R. F., Gallimore J. K. Role of anaerobic metabolism in the preservation of functional capacity and structure of anoxic myocardium. J Clin Invest. 1968 Feb;47(2):403–416. doi: 10.1172/JCI105737. [DOI] [PMC free article] [PubMed] [Google Scholar]
- Wilde A. A., Kléber A. G. The combined effects of hypoxia, high K+, and acidosis on the intracellular sodium activity and resting potential in guinea pig papillary muscle. Circ Res. 1986 Feb;58(2):249–256. doi: 10.1161/01.res.58.2.249. [DOI] [PubMed] [Google Scholar]