Abstract
1. Depolarization of embryonic chick myotubes from negative potentials elicits a rapid spike followed by a long-duration after-potential. The ionic basis of the long-duration after-potential was examined by making intracellular recordings from cultured myotubes, and by making whole-cell patch-clamp recordings from myoblasts and myoballs. 2. The peak potential of the long-duration after-potential varied with the chloride gradient, suggesting that a conductance increase to chloride is involved in generating the after-potential. However, a calcium current was also implicated, since lowering the extracellular calcium or replacing extracellular calcium with cobalt abolished the after-potential. 3. When extracellular calcium was replaced with strontium or barium, short-duration spikes similar to calcium spikes were observed, but only strontium was able to support activation of long-duration after-potentials. Intracellular injection of calcium or strontium into myotubes bathed in calcium-free extracellular solutions restored the ability of depolarization to evoke an after-potential. Intracellular injection of magnesium, barium, nickel or cobalt did not restore this ability. These experiments strongly suggested that the long-duration after-potential was due to a calcium- and voltage-activated chloride current. 4. Whole-cell voltage-clamp recordings from myoballs and myoblasts showed that a large chloride conductance could be activated by depolarization when the internal free calcium concentration was buffered at levels greater than 10(-7) M. At 2.5 x 10(-7) M-calcium, the voltage dependence of activation was steepest in the range of -30 to -20 mV and the activation kinetics varied with the membrane potential. The time to half-maximal activation ranged from 0.1 s at positive potentials to greater than 1 s at more negative potentials. The time constant for deactivation was approximately 1 s at -50 mV. No inactivation was observed. 5. The selectivity of the chloride current was measured by substituting other anions for chloride. The following permeability series was found: I- greater than NO3- greater than Br- greater than Cl- greater than acetate greater than F- greater than SO4- = glucuronate. Thus anion permeability decreased as the hydration radius increased. 6. Measurements of the resting potential of developing myoblasts and myotubes under 'physiological' conditions (37 degrees C, bicarbonate buffer) suggest that the after-potential acts to depolarize these cells 10-20 mV above their resting potential (approximately -60 mV) for several seconds. 7. We discuss the possibility that the long-duration after-potential may be involved in triggering myoblast fusion and in the generation of bursts of spontaneous contractions in developing myotubes.
Full text
PDF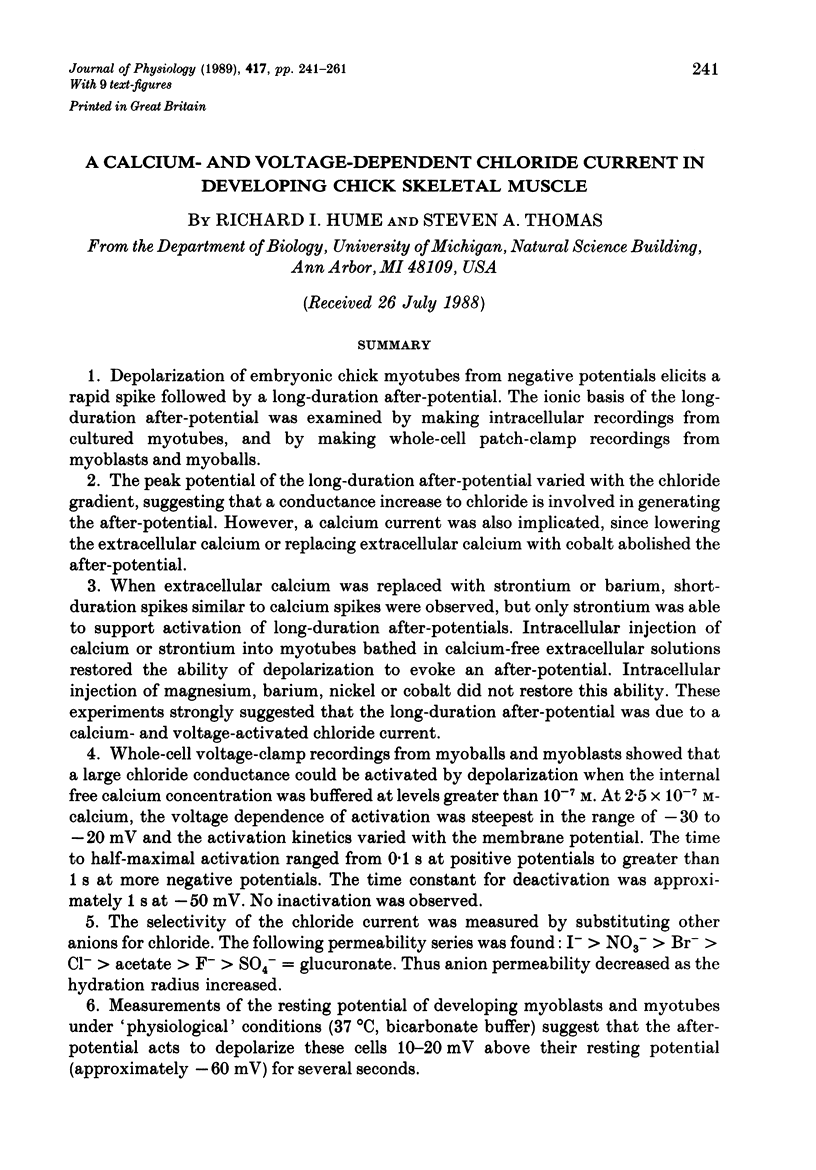
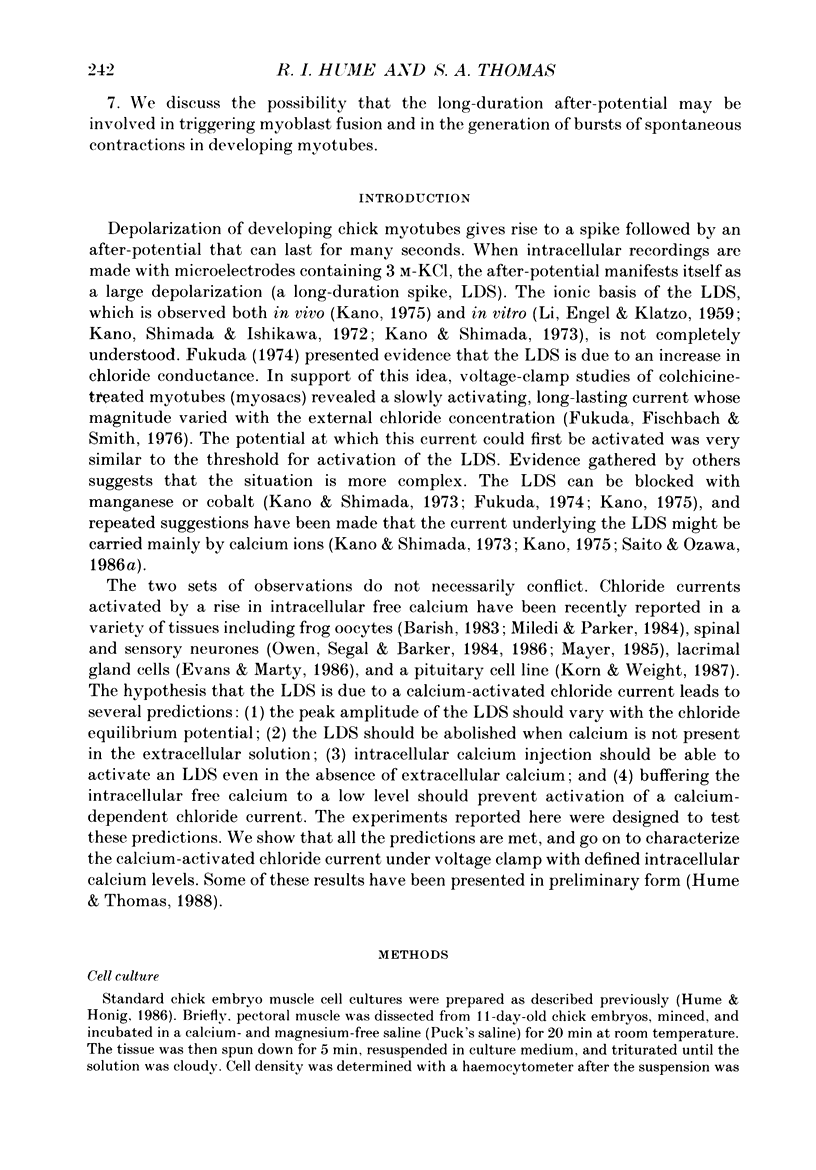
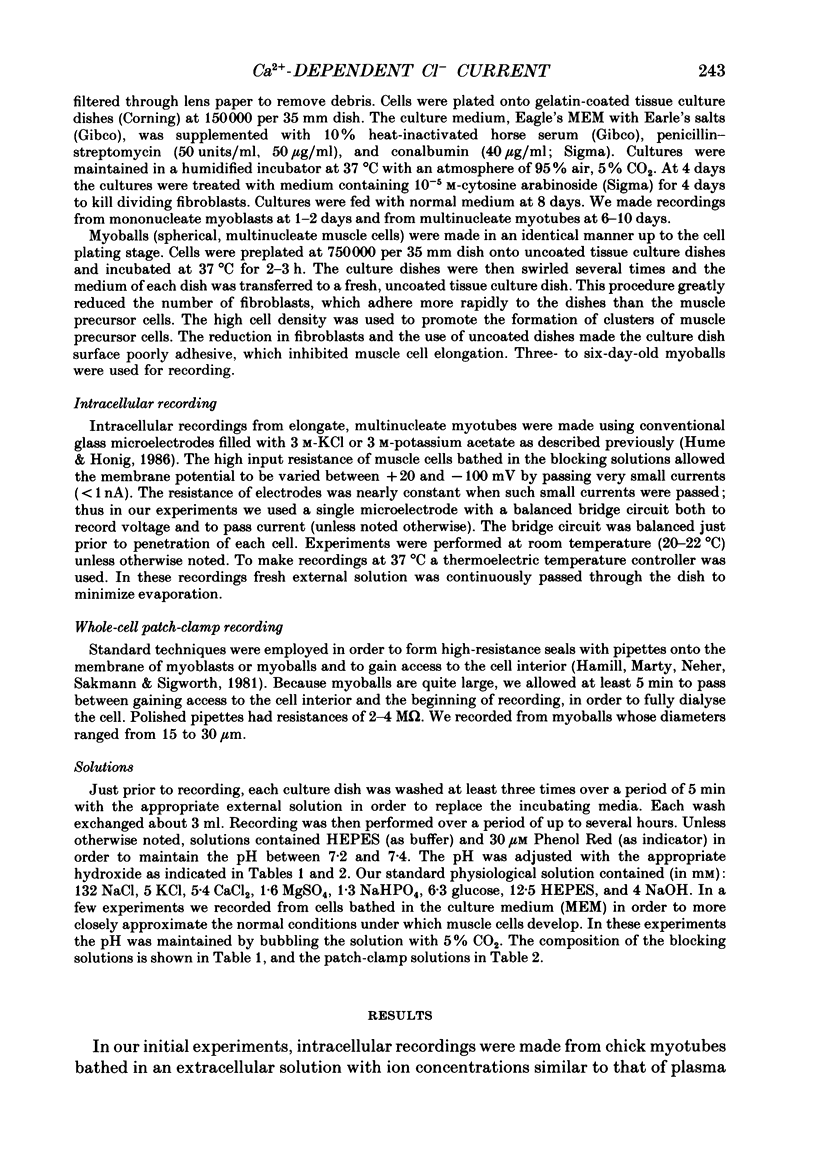
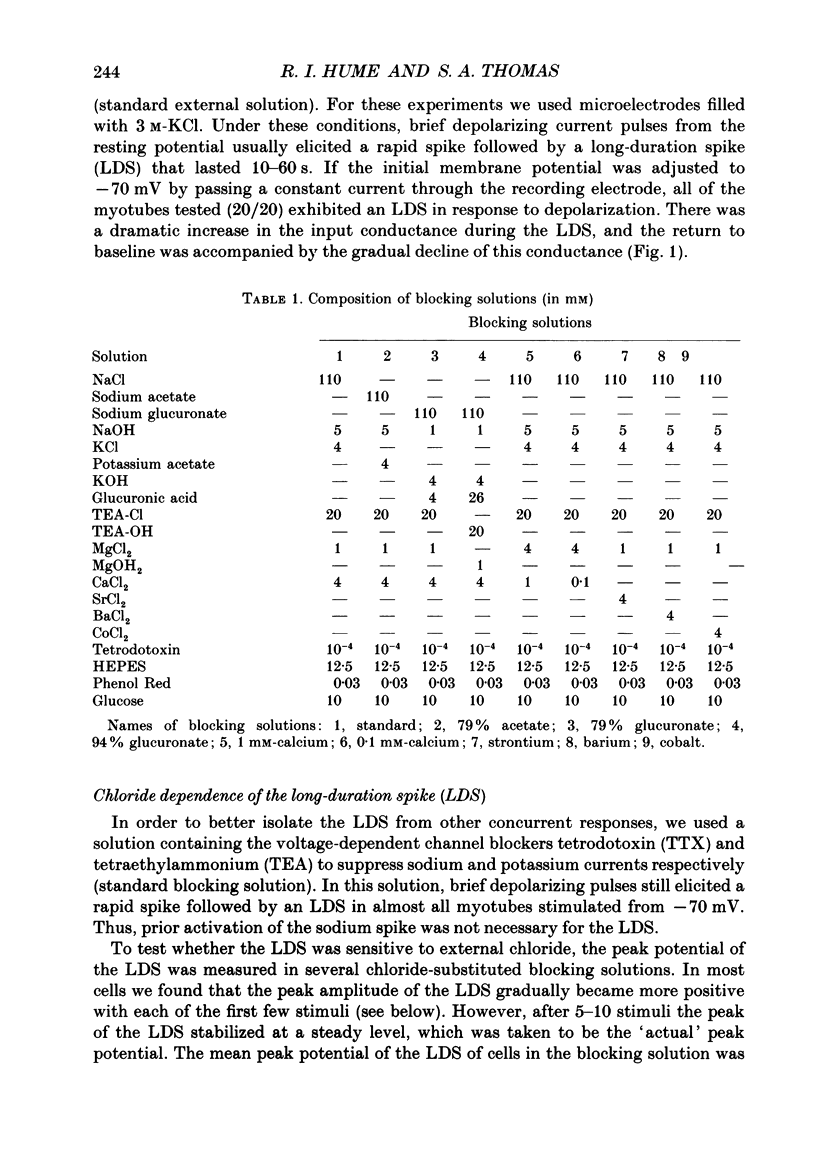
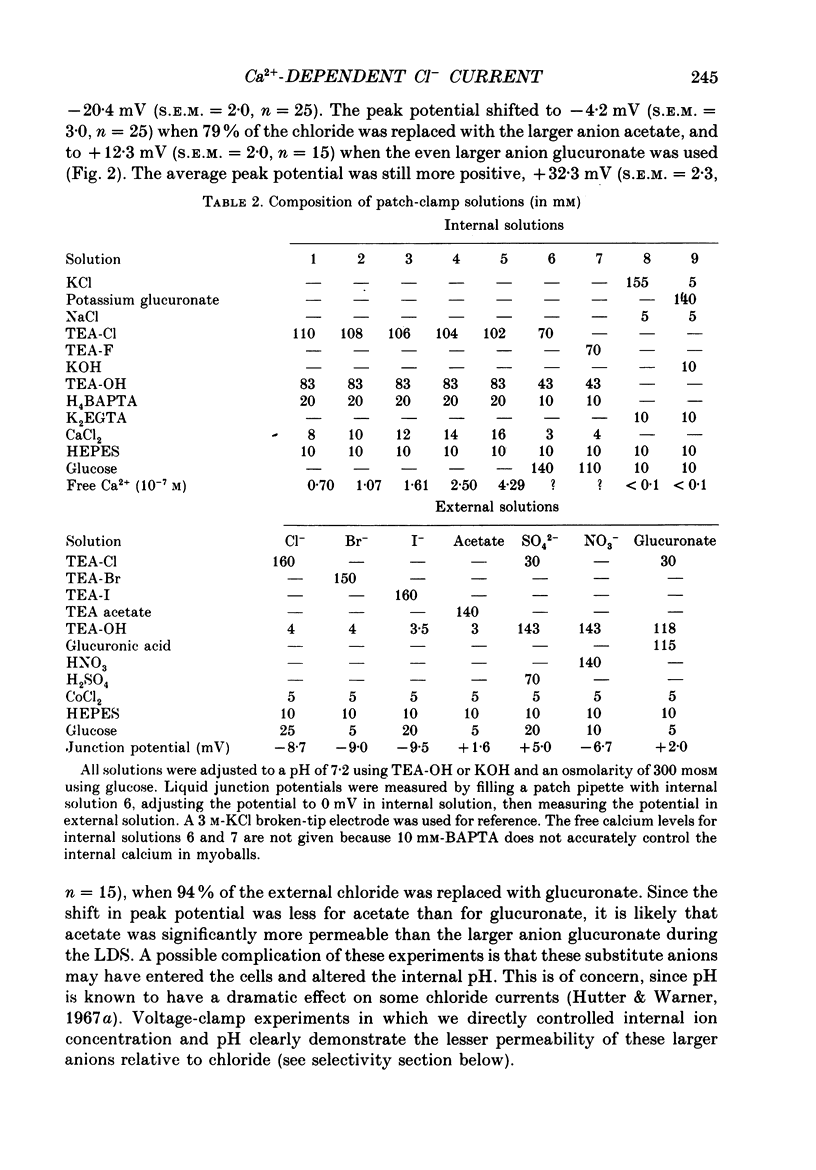
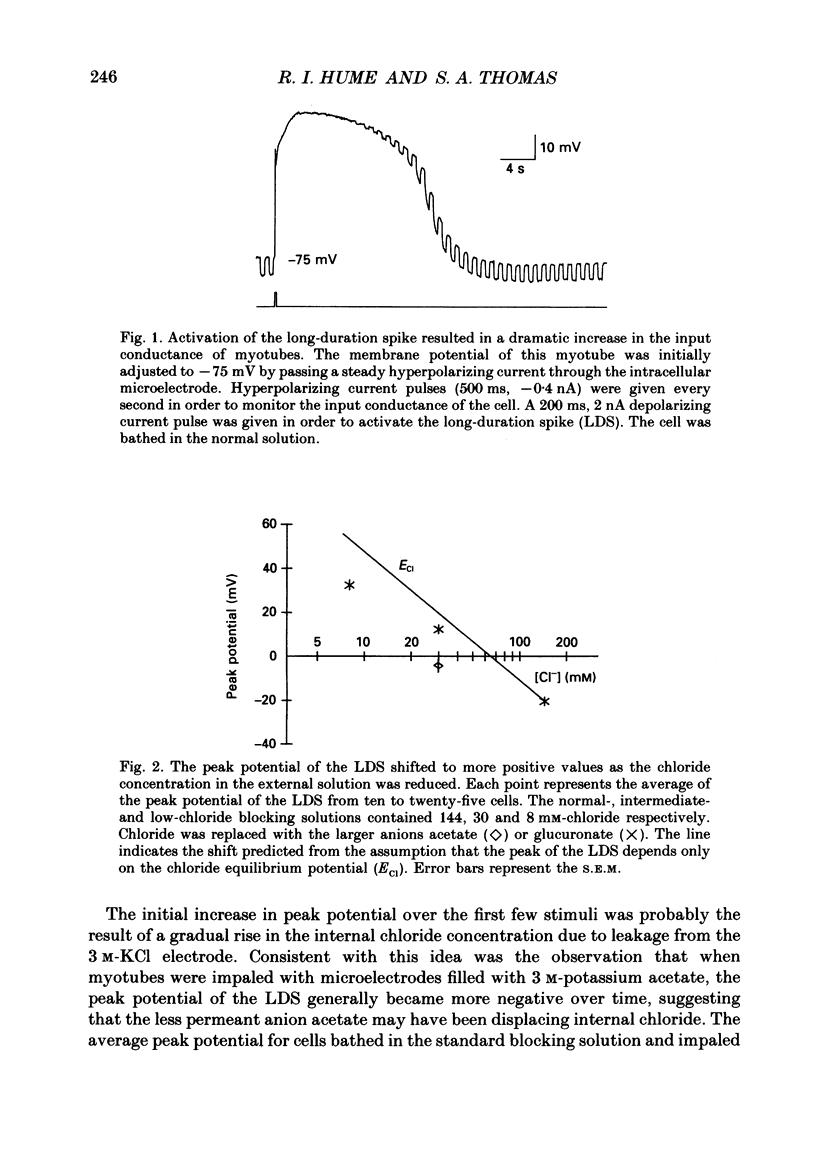
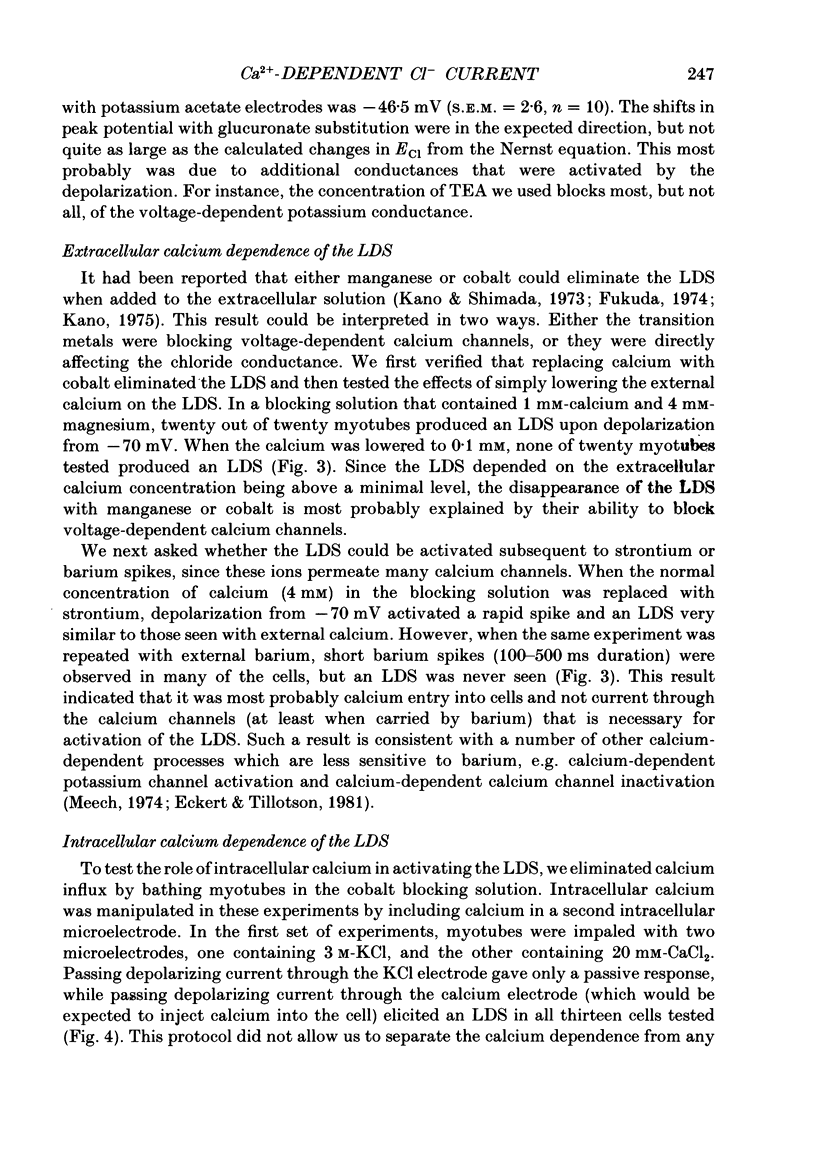
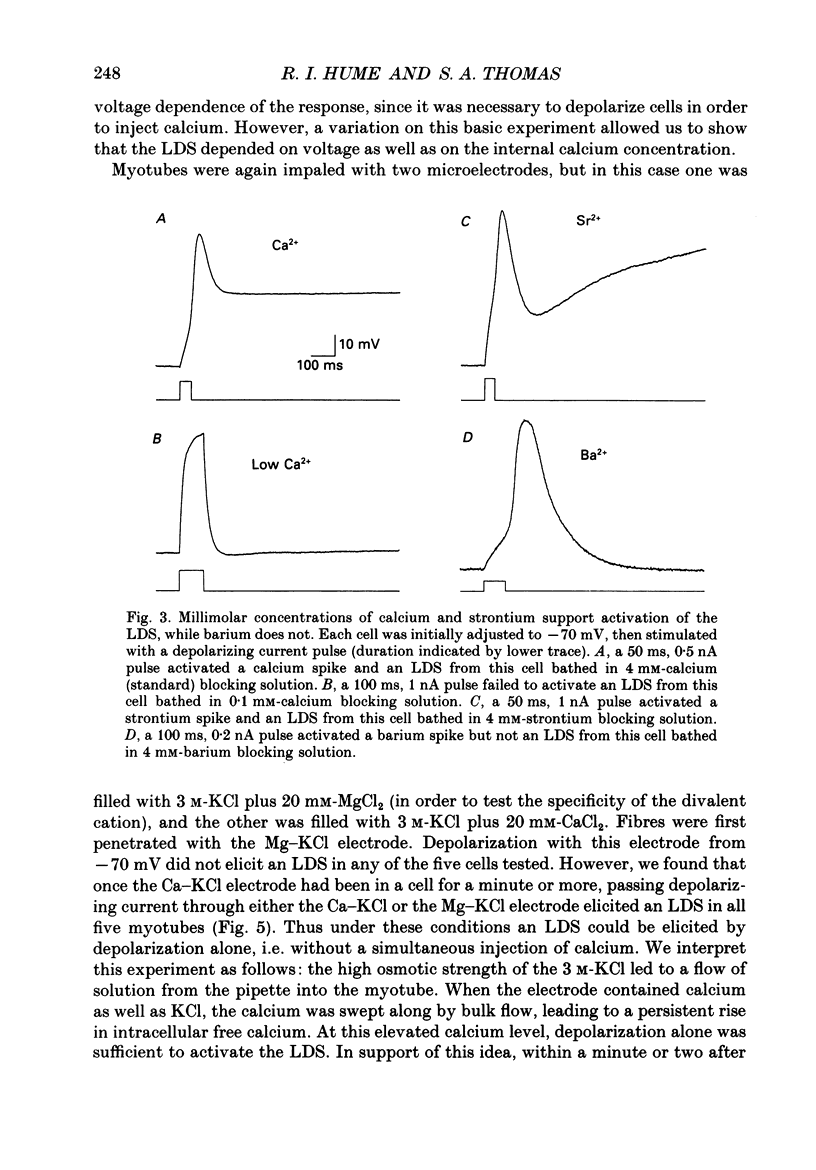
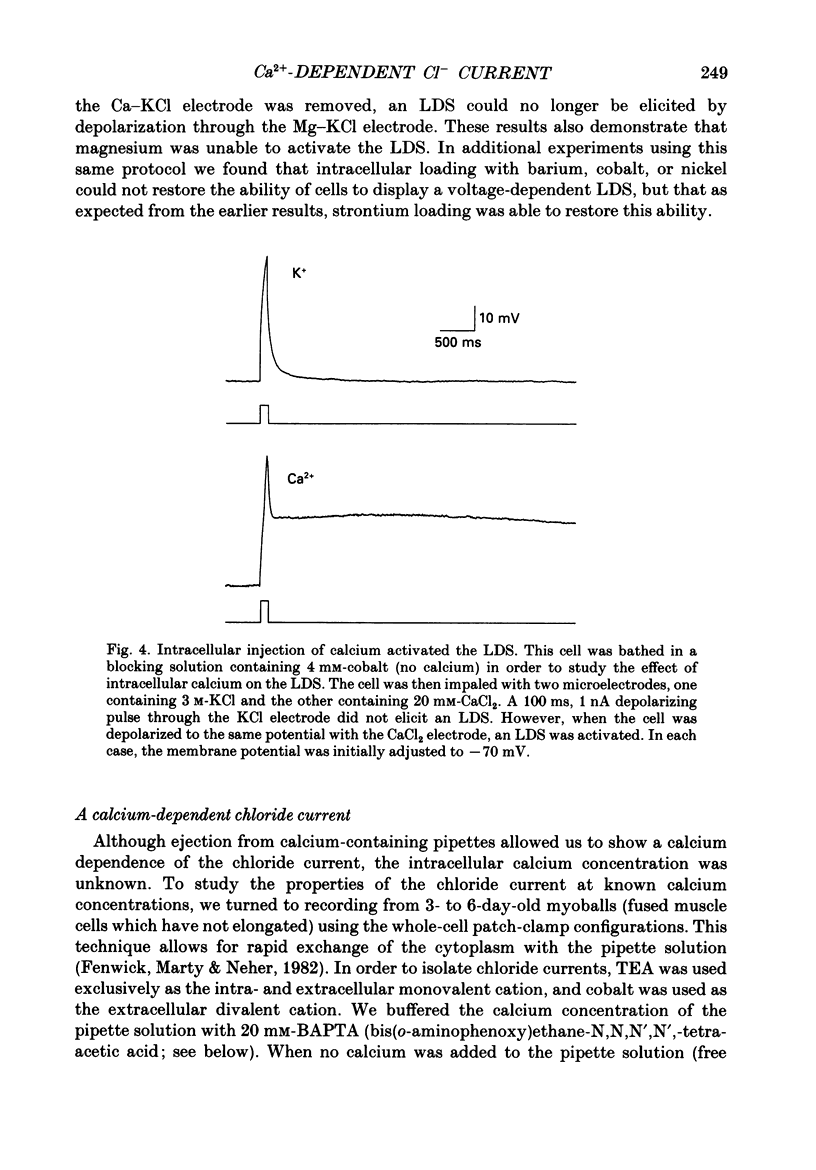
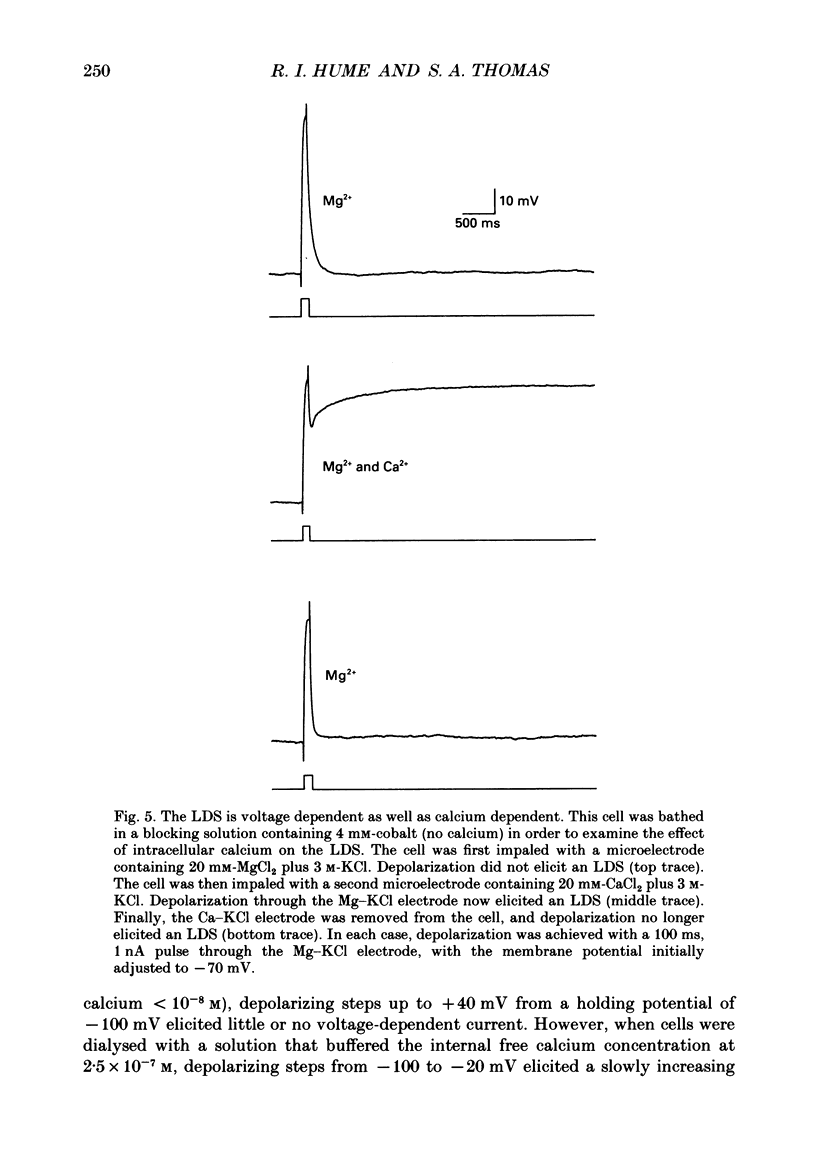
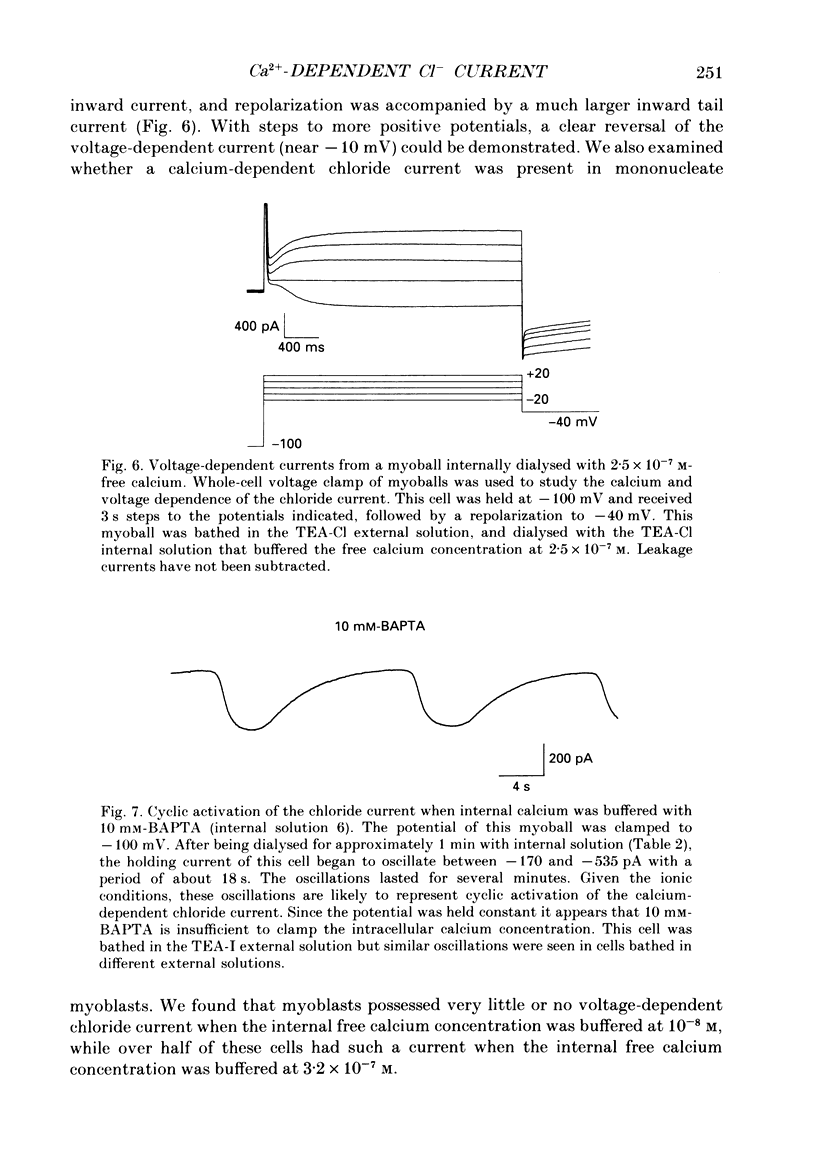
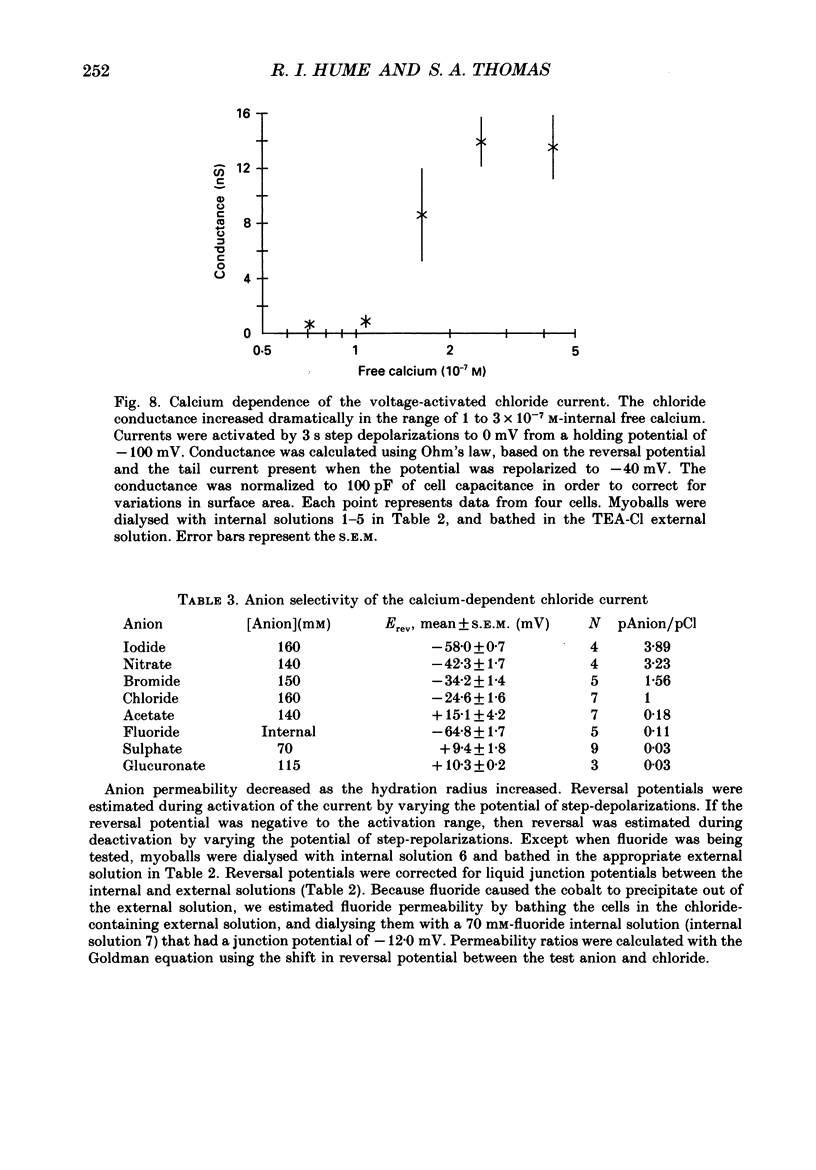
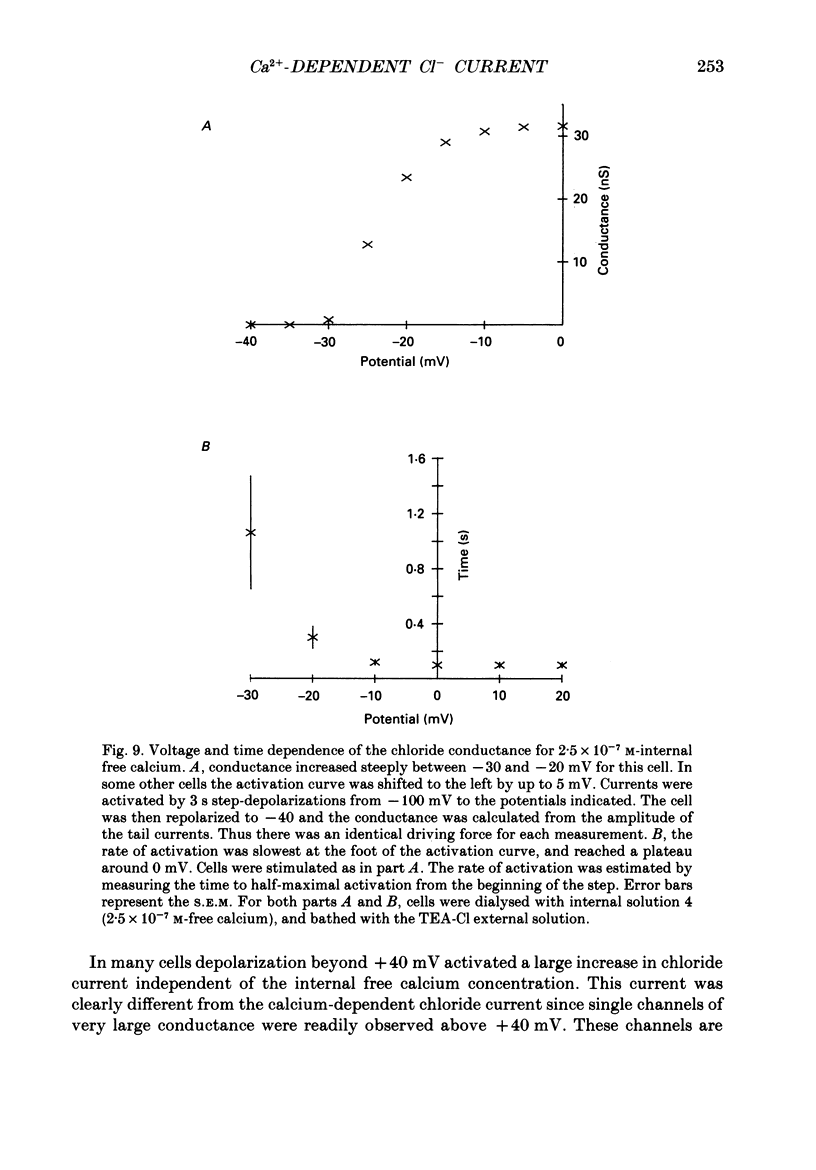
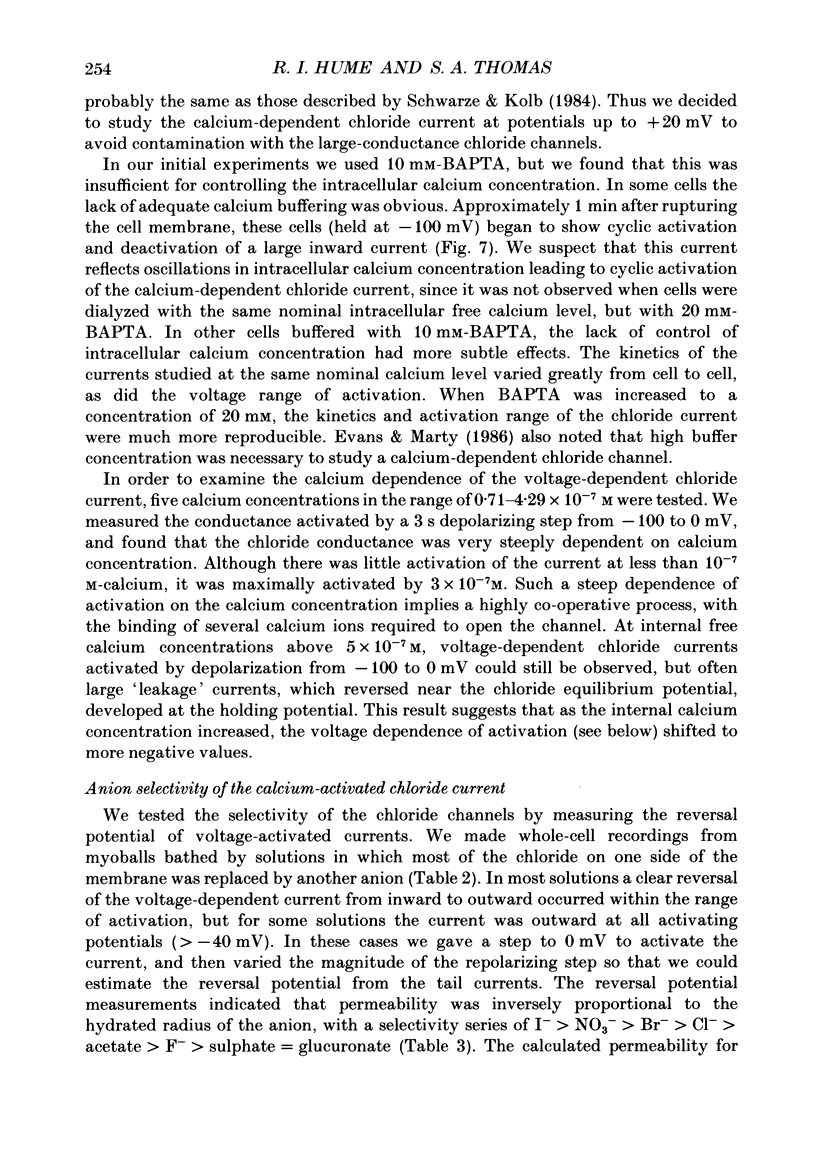
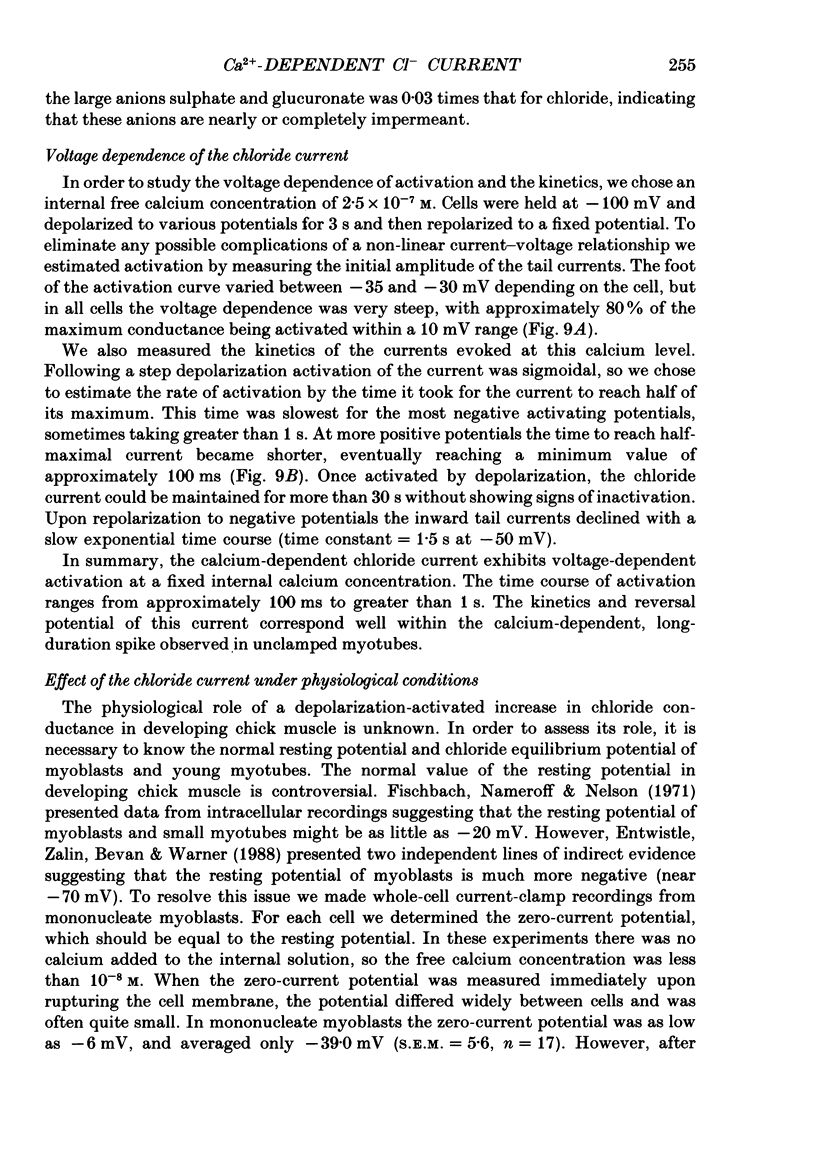
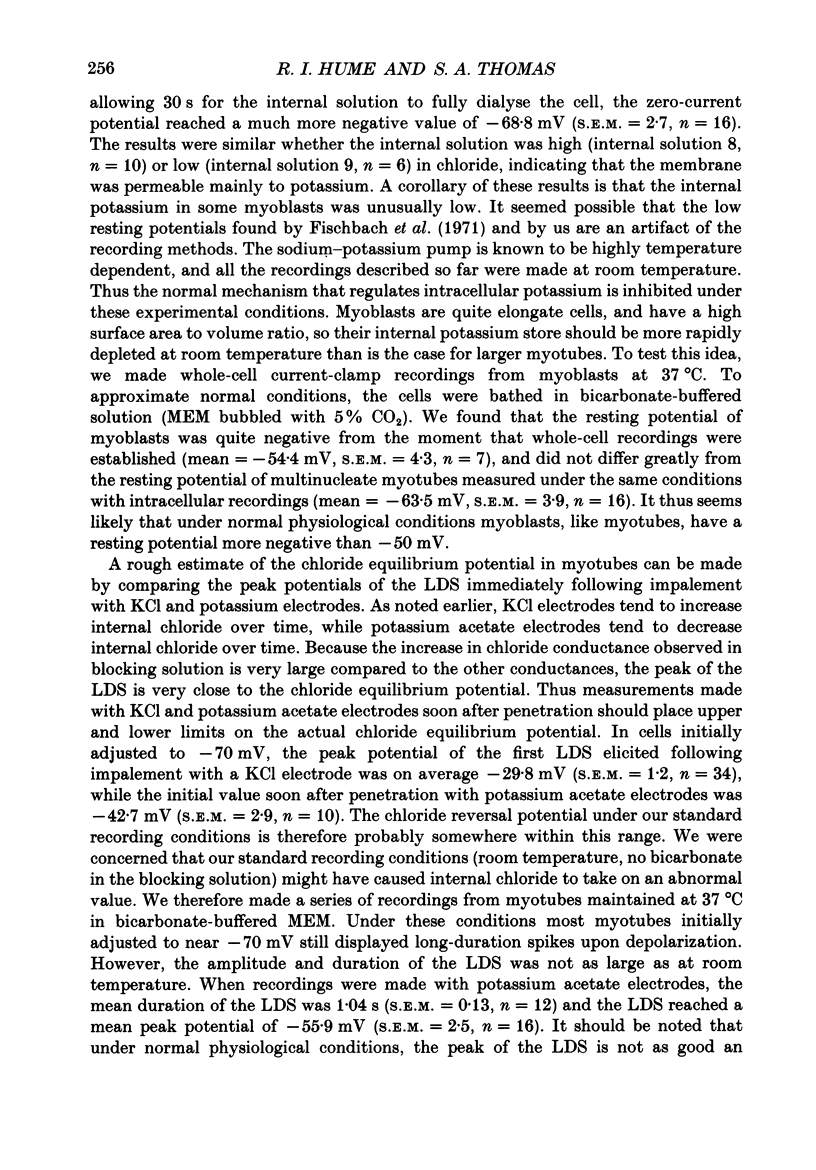
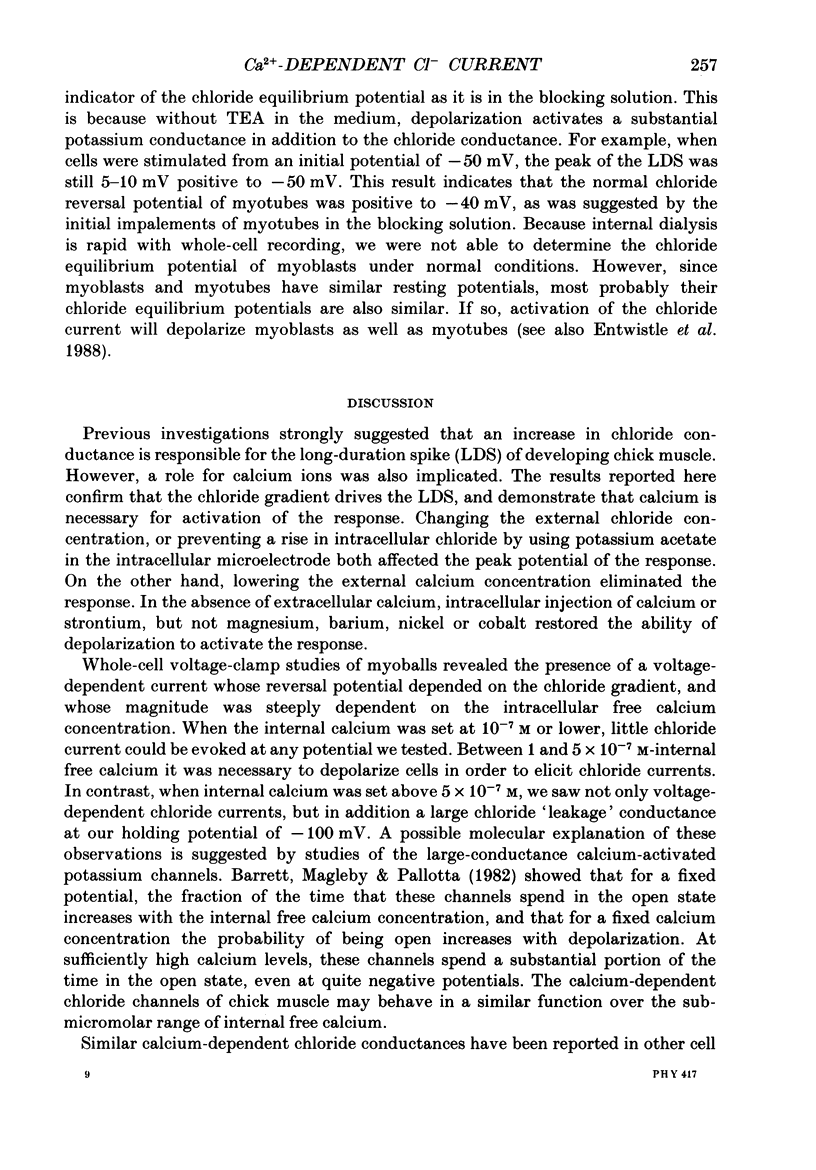
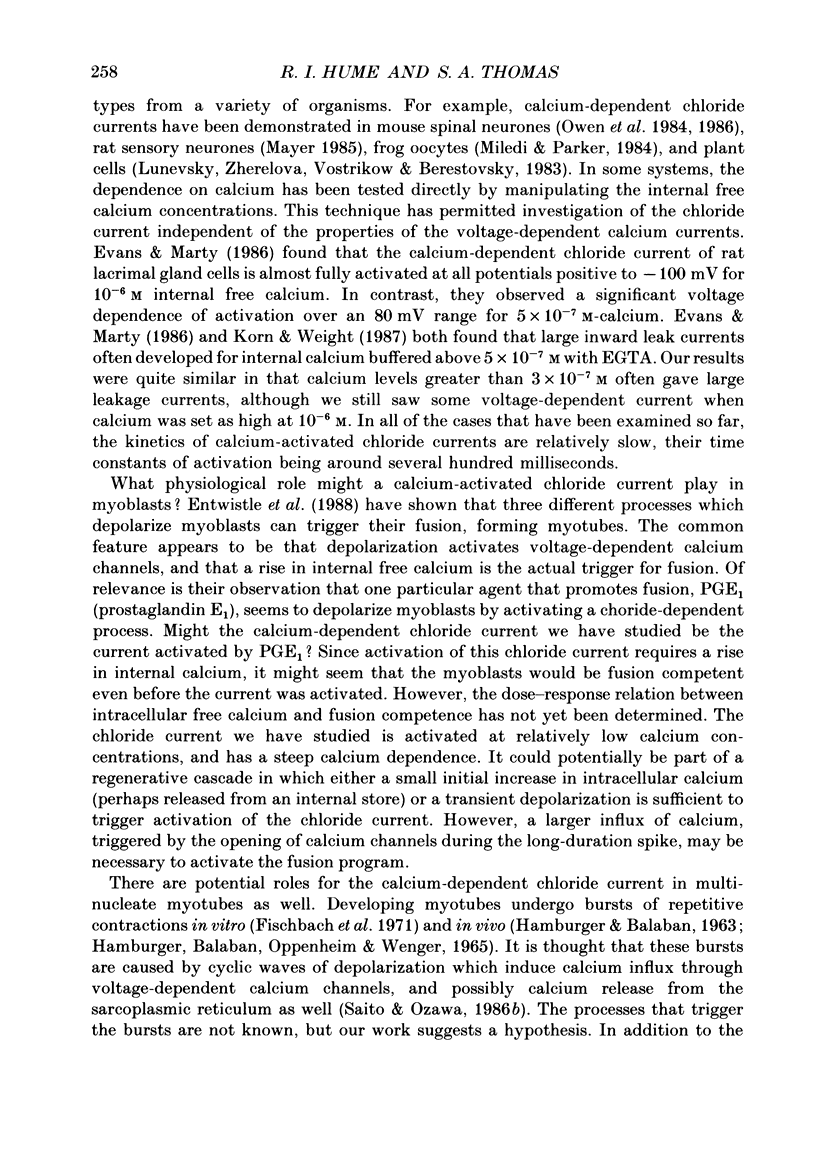
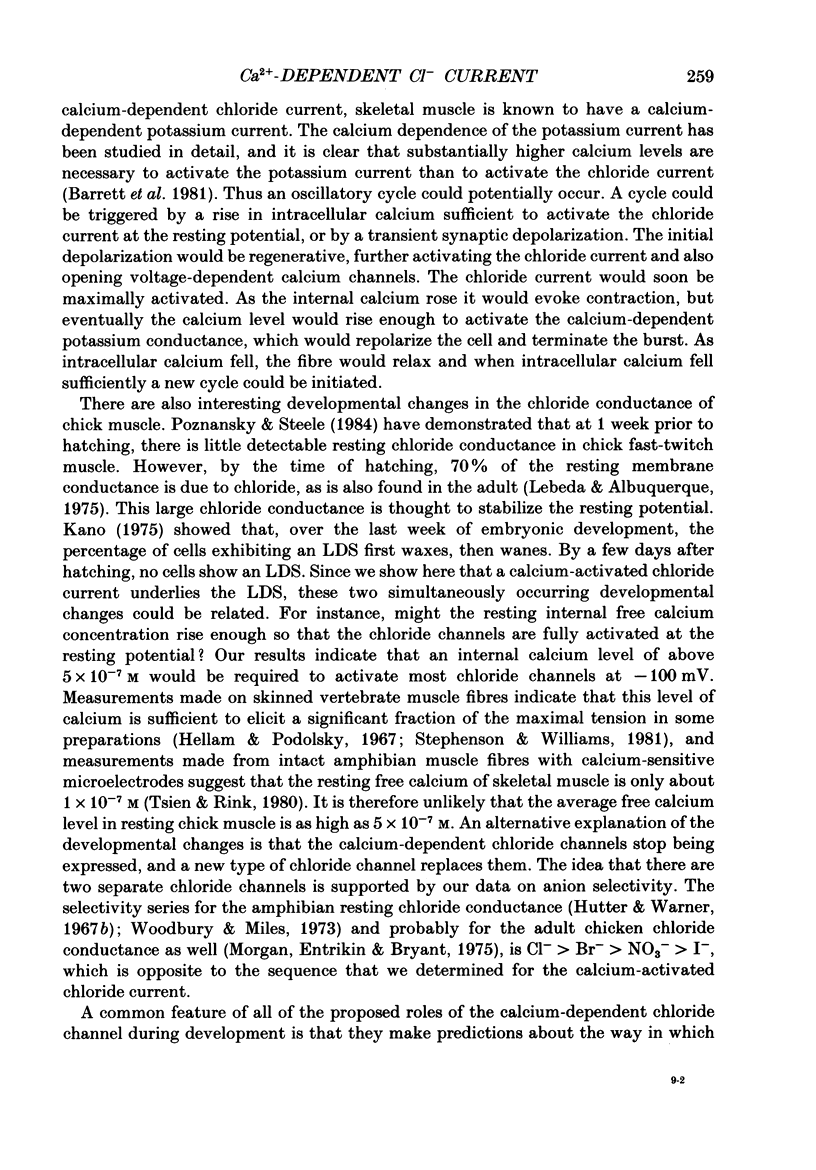
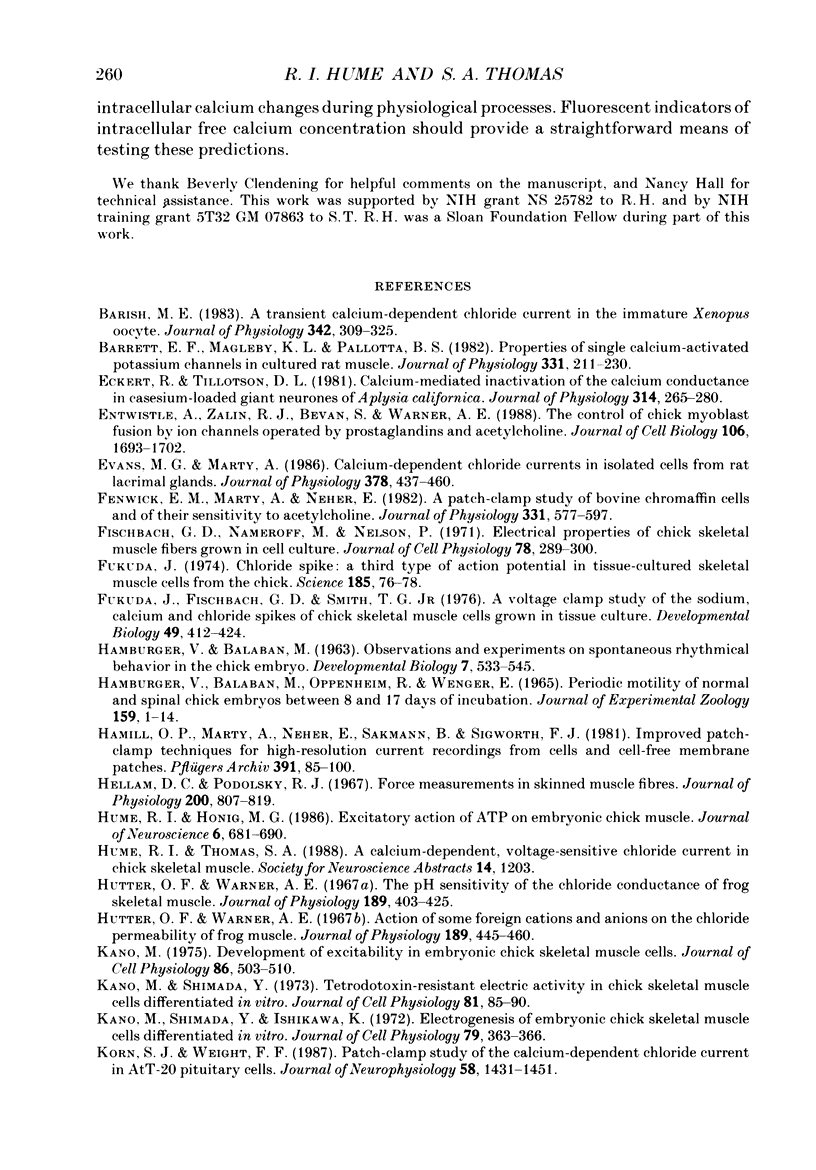
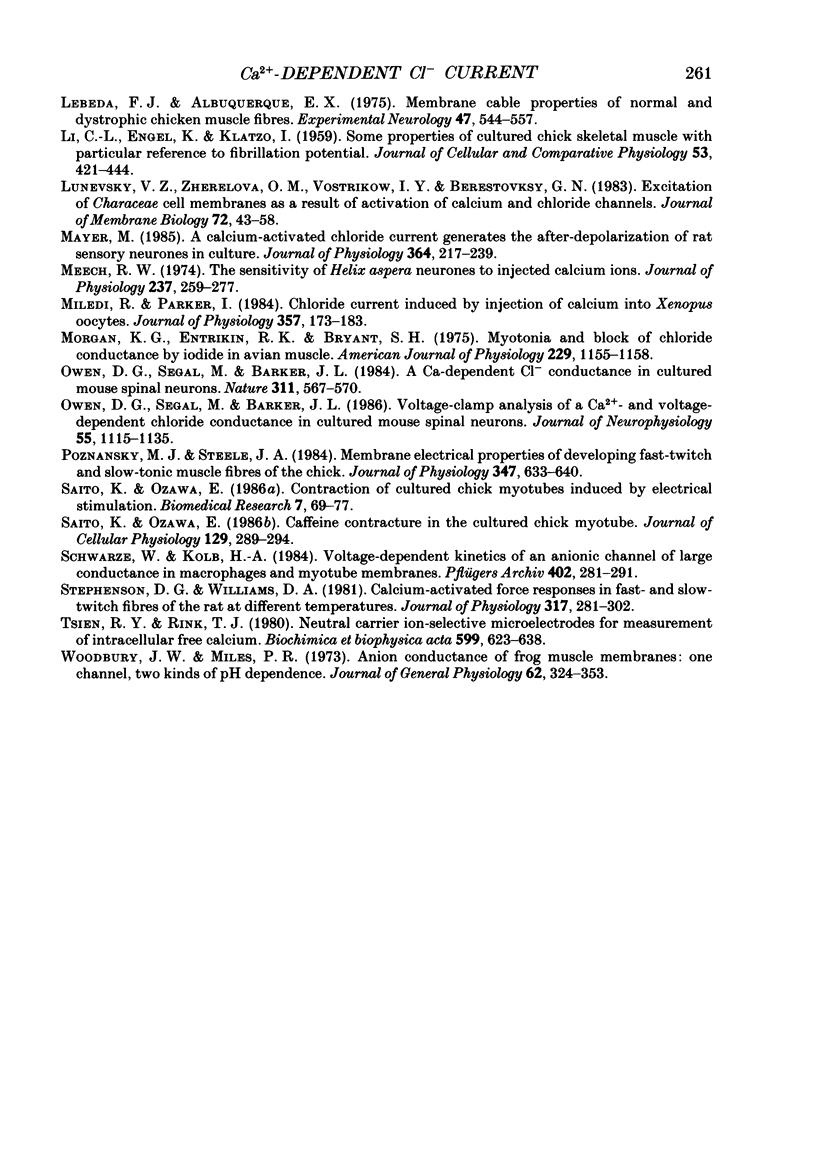
Selected References
These references are in PubMed. This may not be the complete list of references from this article.
- Barish M. E. A transient calcium-dependent chloride current in the immature Xenopus oocyte. J Physiol. 1983 Sep;342:309–325. doi: 10.1113/jphysiol.1983.sp014852. [DOI] [PMC free article] [PubMed] [Google Scholar]
- Barrett J. N., Magleby K. L., Pallotta B. S. Properties of single calcium-activated potassium channels in cultured rat muscle. J Physiol. 1982 Oct;331:211–230. doi: 10.1113/jphysiol.1982.sp014370. [DOI] [PMC free article] [PubMed] [Google Scholar]
- Eckert R., Tillotson D. L. Calcium-mediated inactivation of the calcium conductance in caesium-loaded giant neurones of Aplysia californica. J Physiol. 1981 May;314:265–280. doi: 10.1113/jphysiol.1981.sp013706. [DOI] [PMC free article] [PubMed] [Google Scholar]
- Evans M. G., Marty A. Calcium-dependent chloride currents in isolated cells from rat lacrimal glands. J Physiol. 1986 Sep;378:437–460. doi: 10.1113/jphysiol.1986.sp016229. [DOI] [PMC free article] [PubMed] [Google Scholar]
- Fenwick E. M., Marty A., Neher E. A patch-clamp study of bovine chromaffin cells and of their sensitivity to acetylcholine. J Physiol. 1982 Oct;331:577–597. doi: 10.1113/jphysiol.1982.sp014393. [DOI] [PMC free article] [PubMed] [Google Scholar]
- Fischbach G. D., Nameroff M., Nelson P. G. Electrical properties of chick skeletal muscle fibers developing in cell culture. J Cell Physiol. 1971 Oct;78(2):289–299. doi: 10.1002/jcp.1040780218. [DOI] [PubMed] [Google Scholar]
- Fukuda J. Chloride spike: a third type of action potential in tissue-cultured skeletal muscle cells from the chick. Science. 1974 Jul 5;185(4145):76–78. doi: 10.1126/science.185.4145.76. [DOI] [PubMed] [Google Scholar]
- Fukuda J., Fischbach G. D., Smith T. G., Jr A voltage clamp study of the sodium, calcium and chloride spikes of chick skeletal muscle cells grown in tissue culture. Dev Biol. 1976 Apr;49(2):412–424. doi: 10.1016/0012-1606(76)90184-6. [DOI] [PubMed] [Google Scholar]
- HAMBURGER V., BALABAN M. Observations and experiments on spontaneous rhythmical behavior in the chick embryo. Dev Biol. 1963 Mar;6:533–545. doi: 10.1016/0012-1606(63)90140-4. [DOI] [PubMed] [Google Scholar]
- Hamill O. P., Marty A., Neher E., Sakmann B., Sigworth F. J. Improved patch-clamp techniques for high-resolution current recording from cells and cell-free membrane patches. Pflugers Arch. 1981 Aug;391(2):85–100. doi: 10.1007/BF00656997. [DOI] [PubMed] [Google Scholar]
- Hellam D. C., Podolsky R. J. Force measurements in skinned muscle fibres. J Physiol. 1969 Feb;200(3):807–819. doi: 10.1113/jphysiol.1969.sp008723. [DOI] [PMC free article] [PubMed] [Google Scholar]
- Hume R. I., Honig M. G. Excitatory action of ATP on embryonic chick muscle. J Neurosci. 1986 Mar;6(3):681–690. doi: 10.1523/JNEUROSCI.06-03-00681.1986. [DOI] [PMC free article] [PubMed] [Google Scholar]
- Hutter O. F., Warner A. E. Action of some foreign cations and anions on the chloride permeability of frog muscle. J Physiol. 1967 Apr;189(3):445–460. doi: 10.1113/jphysiol.1967.sp008178. [DOI] [PMC free article] [PubMed] [Google Scholar]
- Hutter O. F., Warner A. E. The pH sensitivity of the chloride conductance of frog skeletal muscle. J Physiol. 1967 Apr;189(3):403–425. doi: 10.1113/jphysiol.1967.sp008176. [DOI] [PMC free article] [PubMed] [Google Scholar]
- Kano M. Development of excitability in embryonic chick skeletal muscle cells. J Cell Physiol. 1975 Dec;86(3 Pt 1):503–510. doi: 10.1002/jcp.1040860307. [DOI] [PubMed] [Google Scholar]
- Kano M., Shimada Y., Ishikawa K. Electrogenesis of embryonic chick skeletal muscle cells differentiated in vitro. J Cell Physiol. 1972 Jun;79(3):363–366. doi: 10.1002/jcp.1040790306. [DOI] [PubMed] [Google Scholar]
- Kano M., Shimada Y. Tetrodotoxin-resistant electric activity in chick skeletal muscle cells differentiated in vitro. J Cell Physiol. 1973 Feb;81(1):85–89. doi: 10.1002/jcp.1040810110. [DOI] [PubMed] [Google Scholar]
- Korn S. J., Weight F. F. Patch-clamp study of the calcium-dependent chloride current in AtT-20 pituitary cells. J Neurophysiol. 1987 Dec;58(6):1431–1451. doi: 10.1152/jn.1987.58.6.1431. [DOI] [PubMed] [Google Scholar]
- LI C. L., ENGEL K., KLATZO I. Some properties of cultured chick skeletal muscle with particular reference to fibrillation potential. J Cell Comp Physiol. 1959 Jun;53:421–444. doi: 10.1002/jcp.1030530307. [DOI] [PubMed] [Google Scholar]
- Lebeda F. J., Albuquerque E. X. Membrane cable properties of normal and dystrophic chicken muscle fibers. Exp Neurol. 1975 Jun;47(3):544–557. doi: 10.1016/0014-4886(75)90087-4. [DOI] [PubMed] [Google Scholar]
- Mayer M. L. A calcium-activated chloride current generates the after-depolarization of rat sensory neurones in culture. J Physiol. 1985 Jul;364:217–239. doi: 10.1113/jphysiol.1985.sp015740. [DOI] [PMC free article] [PubMed] [Google Scholar]
- Meech R. W. The sensitivity of Helix aspersa neurones to injected calcium ions. J Physiol. 1974 Mar;237(2):259–277. doi: 10.1113/jphysiol.1974.sp010481. [DOI] [PMC free article] [PubMed] [Google Scholar]
- Miledi R., Parker I. Chloride current induced by injection of calcium into Xenopus oocytes. J Physiol. 1984 Dec;357:173–183. doi: 10.1113/jphysiol.1984.sp015495. [DOI] [PMC free article] [PubMed] [Google Scholar]
- Morgan K. G., Entrikin R. K., Bryant S. H. Myotonia and block of chloride conductance by iodide in avian muscle. Am J Physiol. 1975 Nov;229(5):1155–1158. doi: 10.1152/ajplegacy.1975.229.5.1155. [DOI] [PubMed] [Google Scholar]
- Owen D. G., Segal M., Barker J. L. A Ca-dependent Cl- conductance in cultured mouse spinal neurones. Nature. 1984 Oct 11;311(5986):567–570. doi: 10.1038/311567a0. [DOI] [PubMed] [Google Scholar]
- Owen D. G., Segal M., Barker J. L. Voltage-clamp analysis of a Ca2+- and voltage-dependent chloride conductance in cultured mouse spinal neurons. J Neurophysiol. 1986 Jun;55(6):1115–1135. doi: 10.1152/jn.1986.55.6.1115. [DOI] [PubMed] [Google Scholar]
- Poznansky M. J., Steele J. A. Membrane electrical properties of developing fast-twitch and slow-tonic muscle fibres of the chick. J Physiol. 1984 Feb;347:633–640. doi: 10.1113/jphysiol.1984.sp015087. [DOI] [PMC free article] [PubMed] [Google Scholar]
- Saito K., Ozawa E. Caffeine contracture in the cultured chick myotube. J Cell Physiol. 1986 Dec;129(3):289–294. doi: 10.1002/jcp.1041290304. [DOI] [PubMed] [Google Scholar]
- Schwarze W., Kolb H. A. Voltage-dependent kinetics of an anionic channel of large unit conductance in macrophages and myotube membranes. Pflugers Arch. 1984 Nov;402(3):281–291. doi: 10.1007/BF00585511. [DOI] [PubMed] [Google Scholar]
- Stephenson D. G., Williams D. A. Calcium-activated force responses in fast- and slow-twitch skinned muscle fibres of the rat at different temperatures. J Physiol. 1981 Aug;317:281–302. doi: 10.1113/jphysiol.1981.sp013825. [DOI] [PMC free article] [PubMed] [Google Scholar]
- Tsien R. Y., Rink T. J. Neutral carrier ion-selective microelectrodes for measurement of intracellular free calcium. Biochim Biophys Acta. 1980 Jul;599(2):623–638. doi: 10.1016/0005-2736(80)90205-9. [DOI] [PubMed] [Google Scholar]
- Woodbury J. W., Miles P. R. Anion conductance of frog muscle membranes: one channel, two kinds of pH dependence. J Gen Physiol. 1973 Sep;62(3):324–353. doi: 10.1085/jgp.62.3.324. [DOI] [PMC free article] [PubMed] [Google Scholar]