Abstract
1. We measured intracellular Ca2+ transients during rapid cooling contractures (RCCs) in guinea-pig ventricular myocytes using the fluorescent Ca2+ indicator, Indo-1. 2. Rapid cooling of myocytes from 22 to 0-1 degrees C induced a rapid increase in [Ca2+]i which preceded the peak of the contraction and was sometimes large enough to saturate Indo-1. This indicates that [Ca2+]i may reach greater than 10 microM during an RCC. 3. The [Ca2+]i during the RCC slowly declined from its peak value and most of this decline in [Ca2+]i can be attributed to slow reaccumulation of Ca2+ by the sarcoplasmic reticulum (SR) in the cold. RCCs induced in the absence of Cao2+, were not different from control, supporting previous conclusions that RCCs depend exclusively on intracellular Ca2+ stores. 4. RCCs are depressed by long rest periods (rest decay) or by exposure to ryanodine or caffeine, which supports conclusions that RCCs are due to Ca2+ release from the SR. The rest decay of RCCs can be almost completely prevented by applying Nao(+)-free solution during the rest period. This implies that the loss of SR Ca2+ during rest depends on the sarcolemmal Na(+)-Ca2+ exchange (and not the sarcolemmal Ca2(+)-ATPase pump). 5. Rapid rewarming during an RCC normally leads to an additional transient contraction (or rewarming spike), without any increase in [Ca2+]i. Thus, the rewarming spike might be attributable to an increase in myofilament Ca2+ sensitivity induced by rewarming. 6. A second RCC is used to assess the fraction of Ca2+ which is re-sequestered by the SR during relaxation from the first RCC. In control solution progressive RCCs decline in amplitude, but in Na(+)-free, Ca2(+)-free solution they are of constant amplitude. We conclude that the SR Ca2+ pump and Na(+)-Ca2+ exchange are responsible for relaxation and that the latter may account for 20-50% of relaxation. 7. These results support the use of RCCs as a useful means of assessing SR Ca2+ content in intact cardiac muscle cells.
Full text
PDF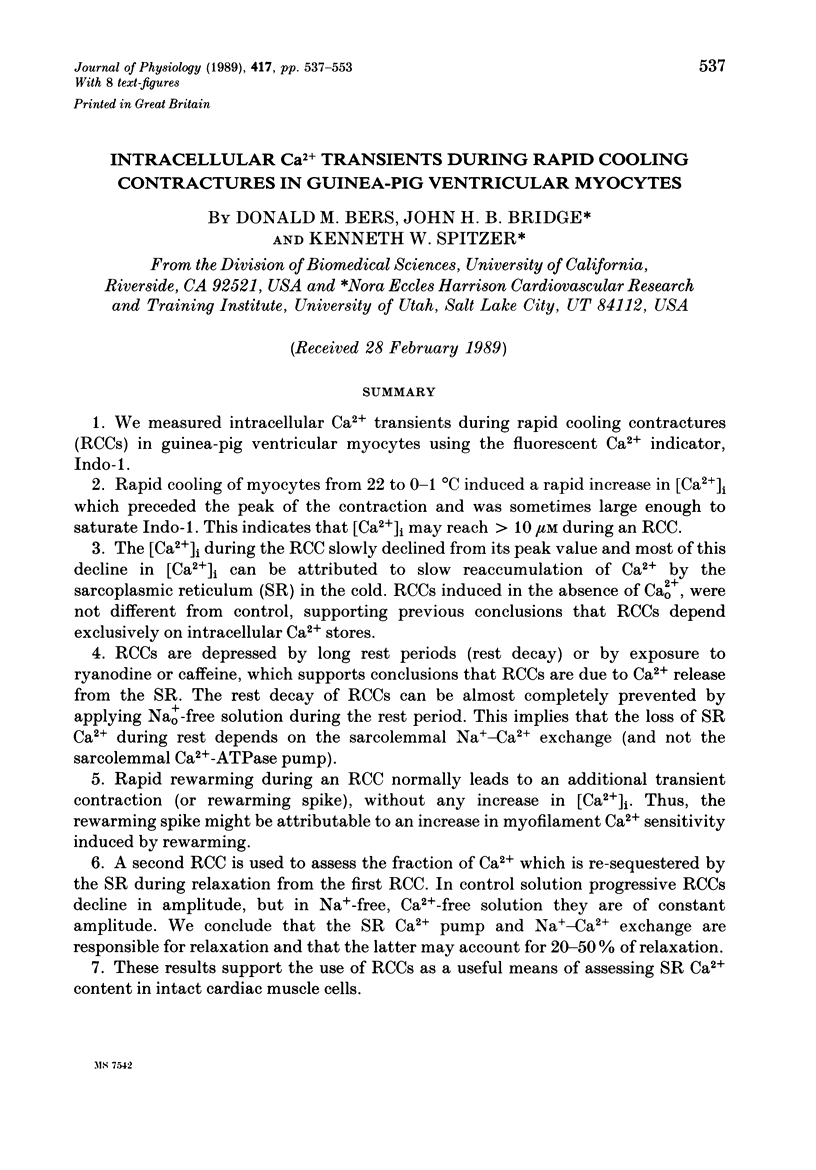
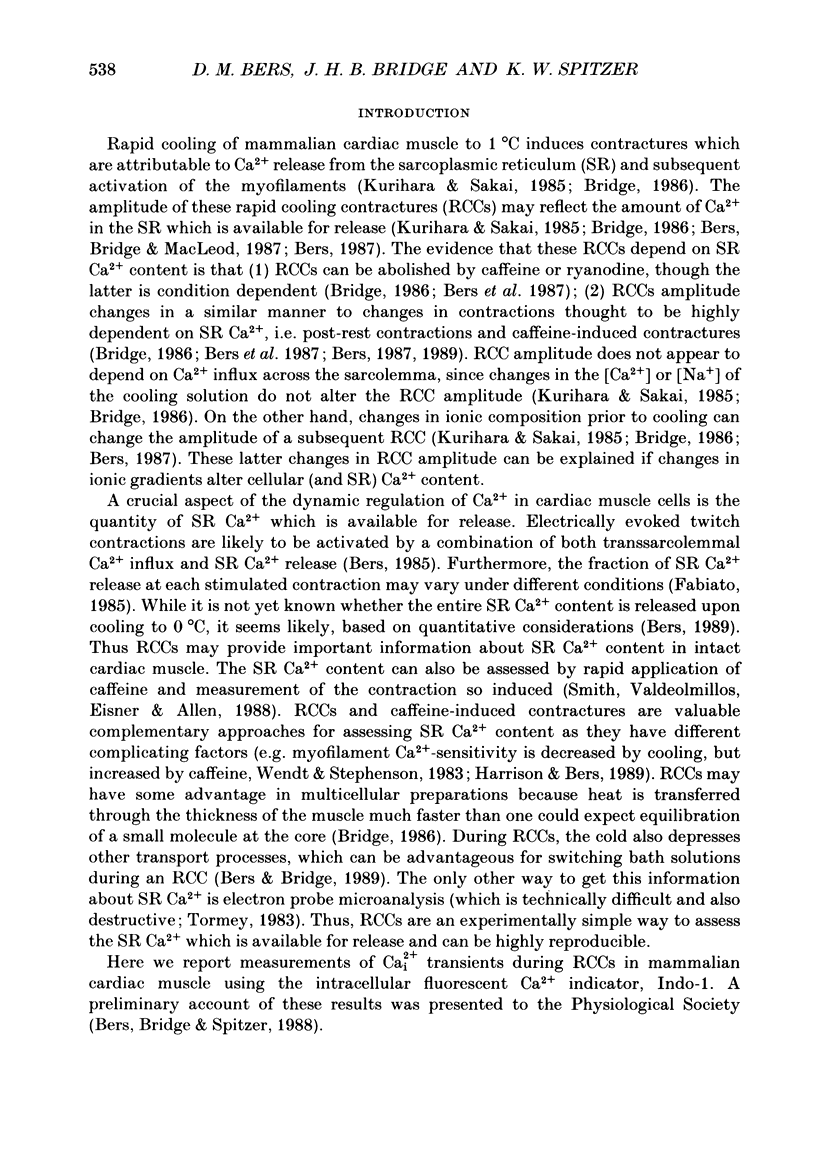
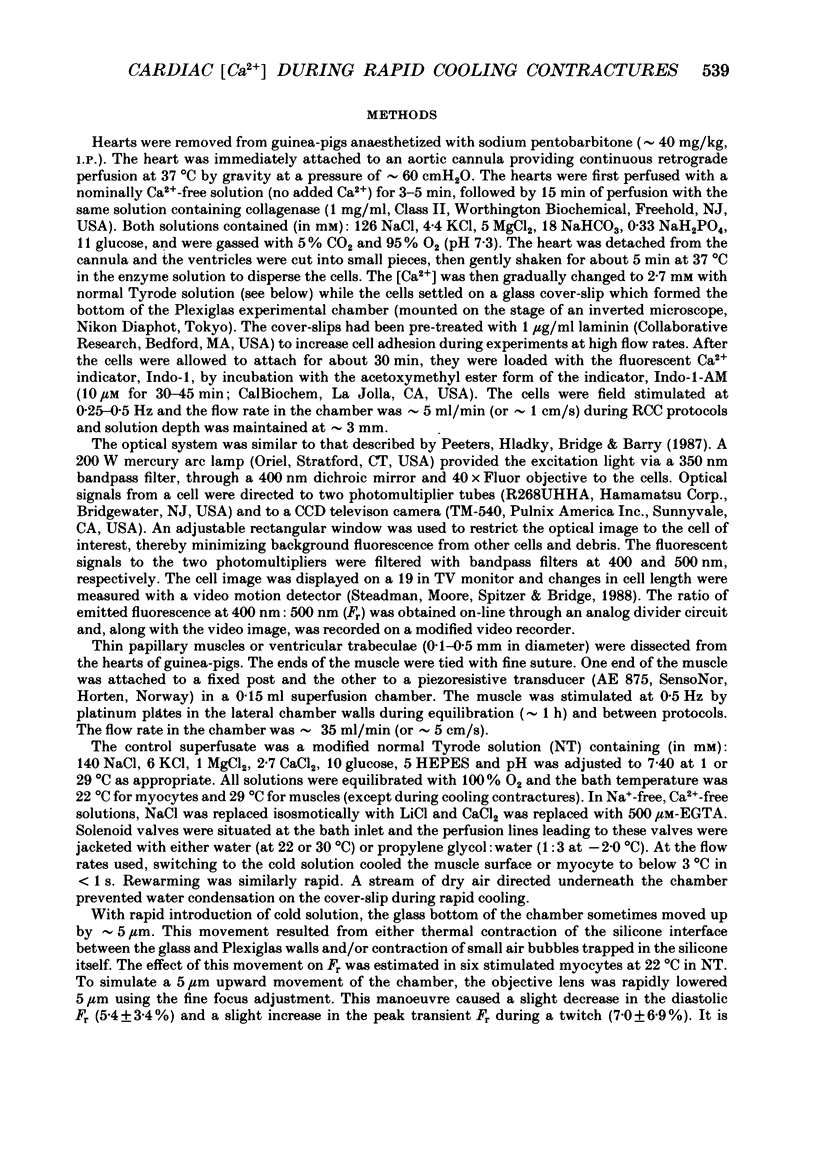
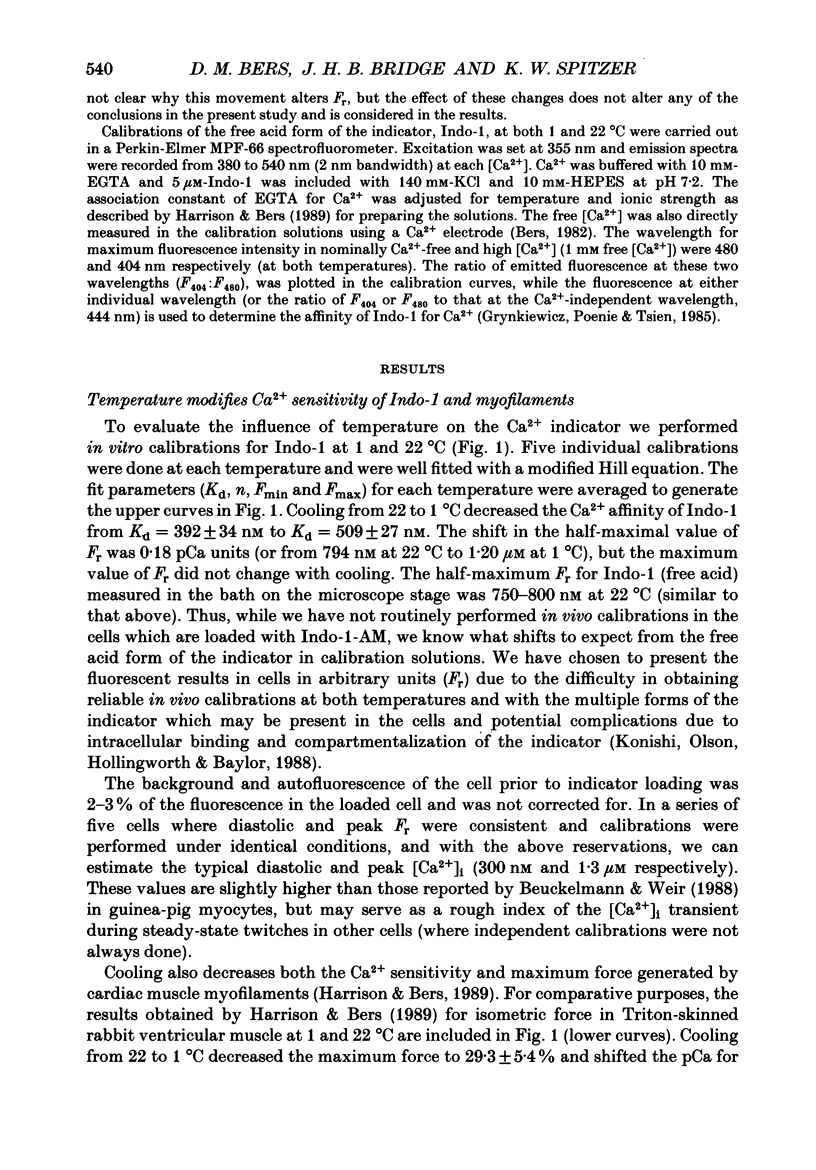
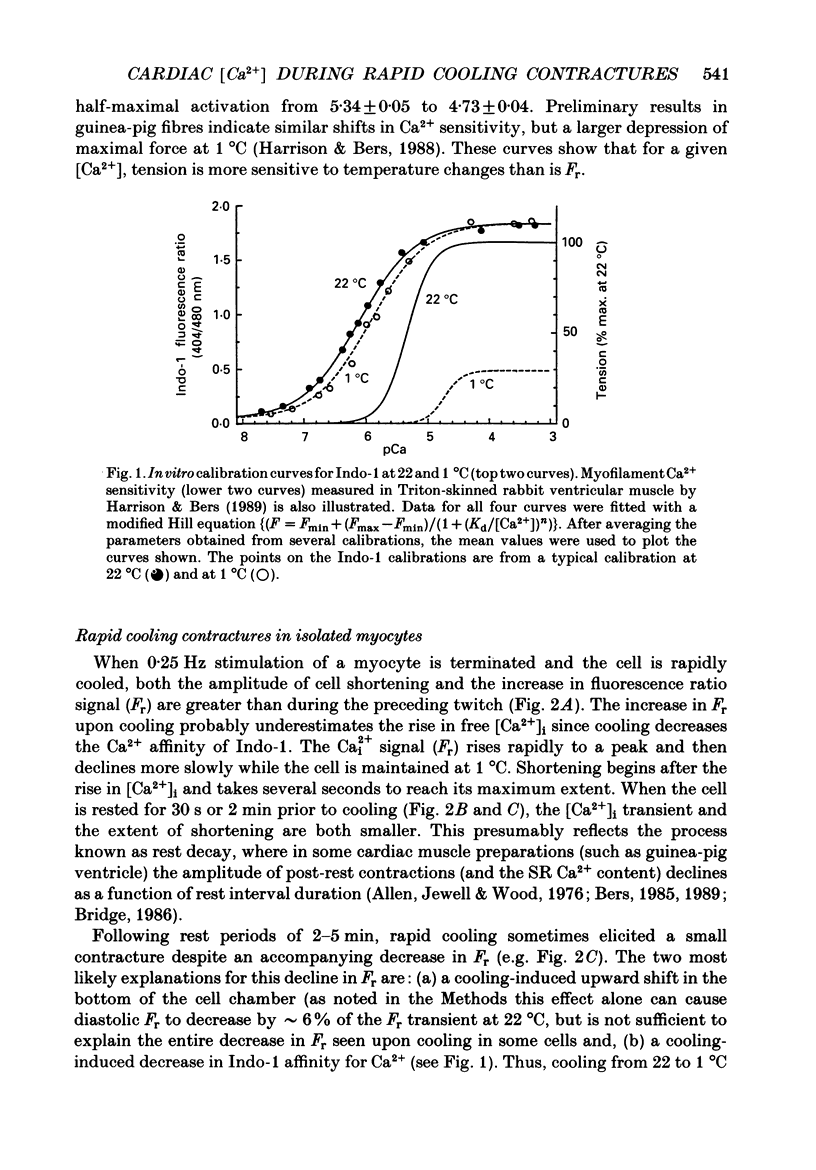
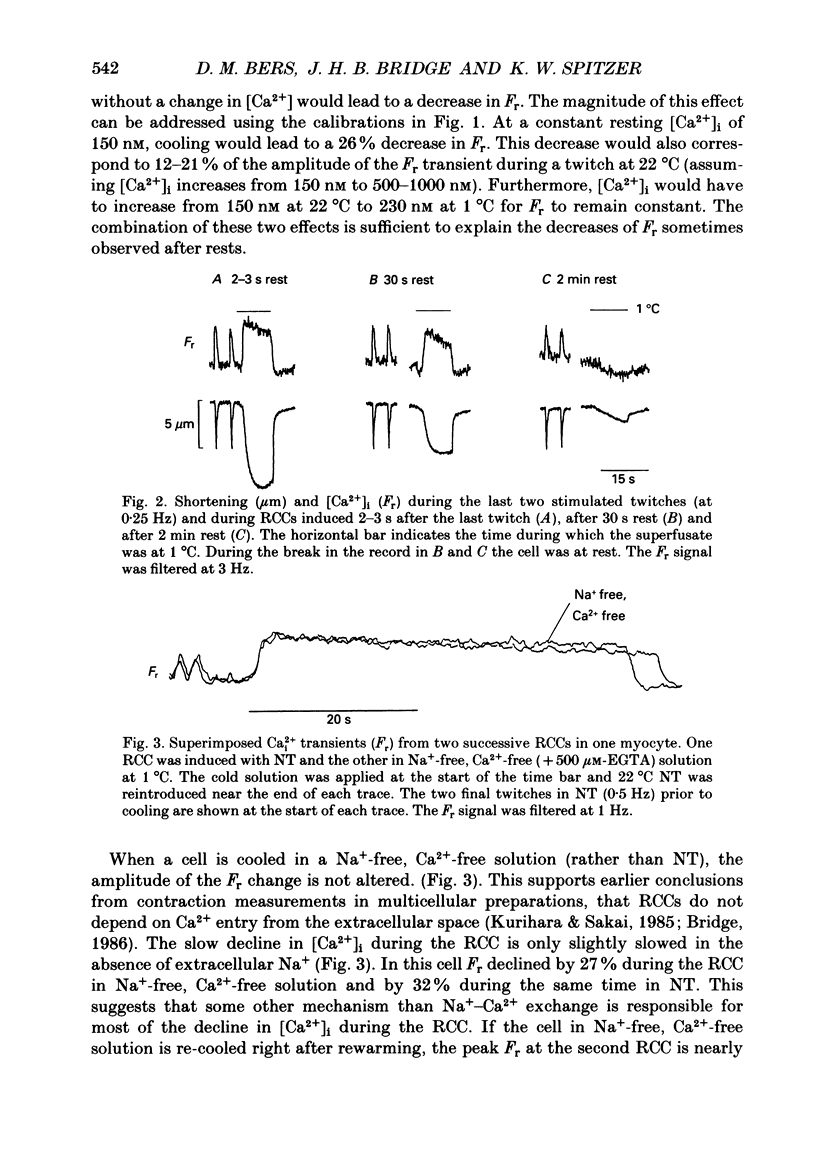
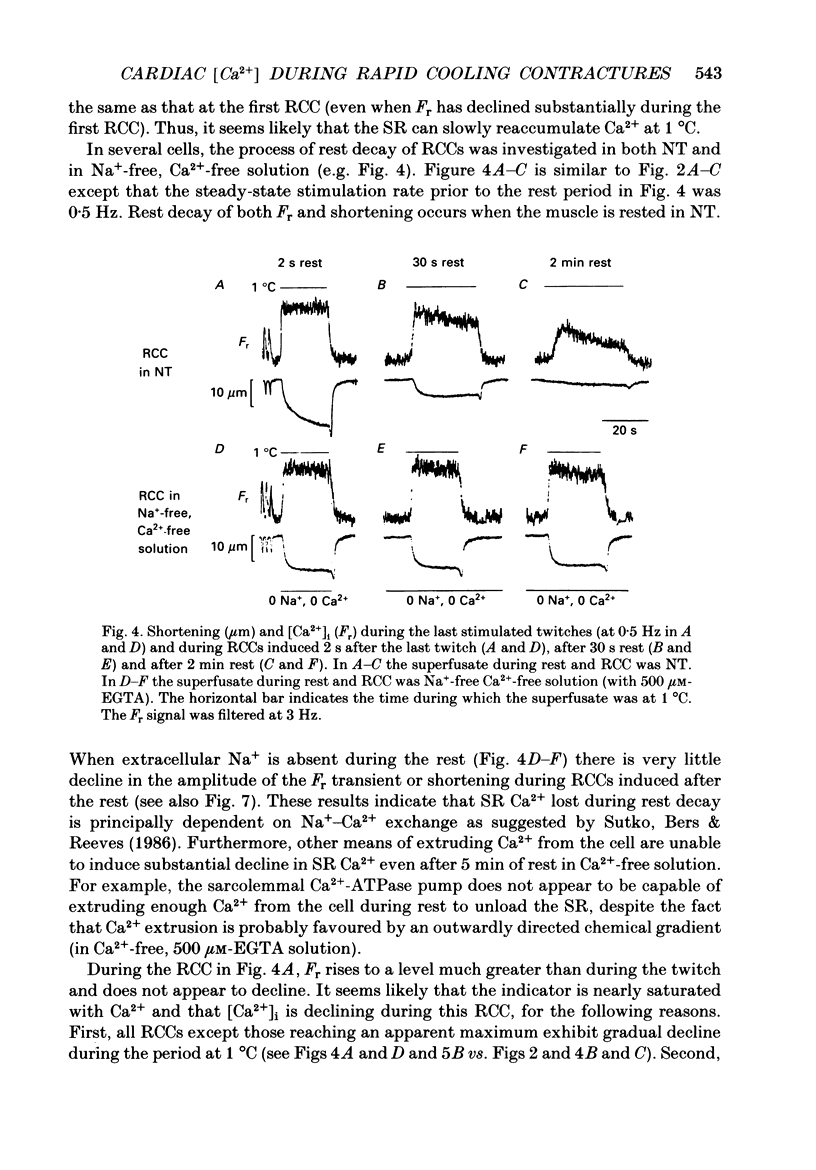
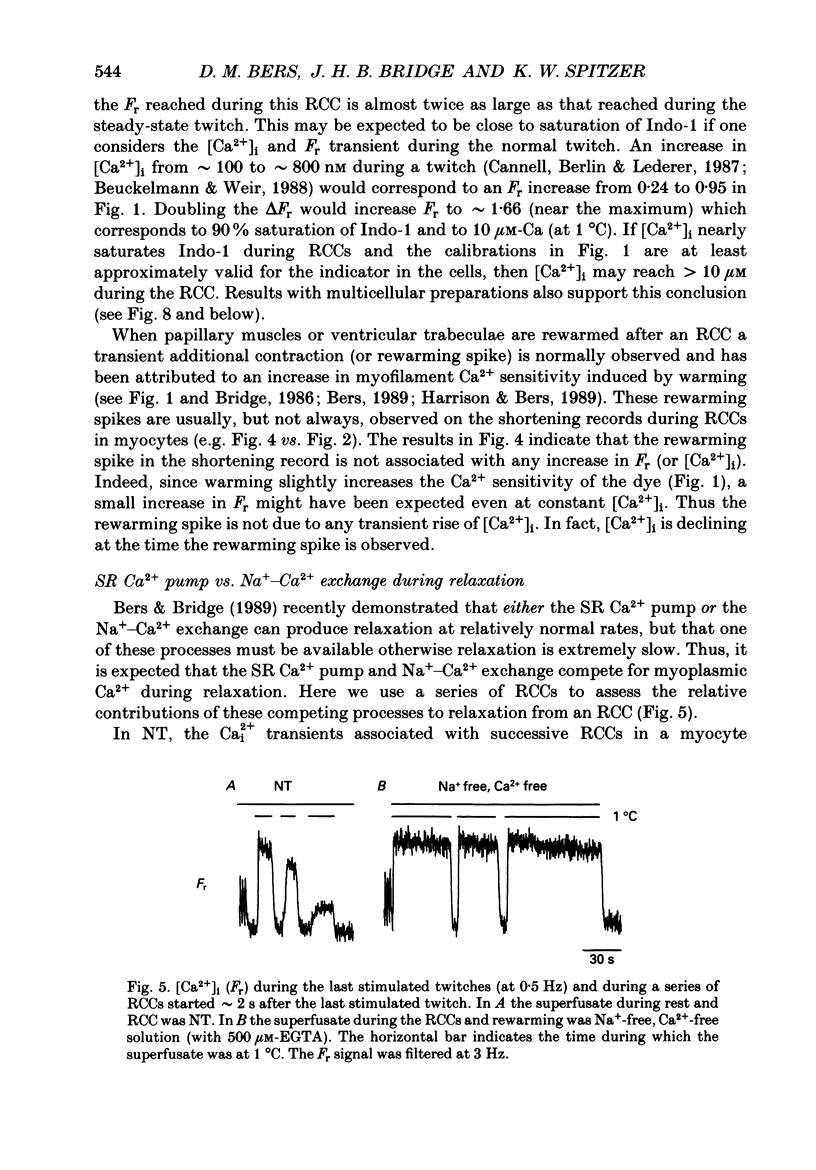
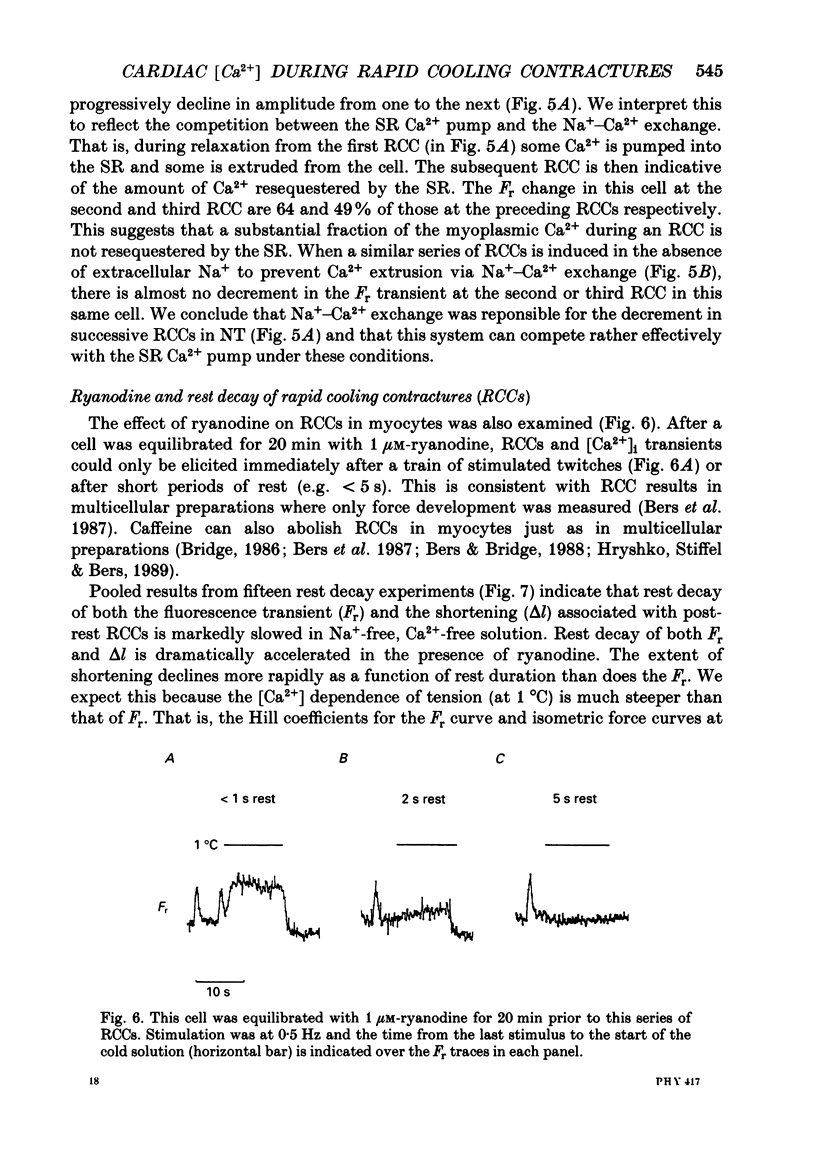
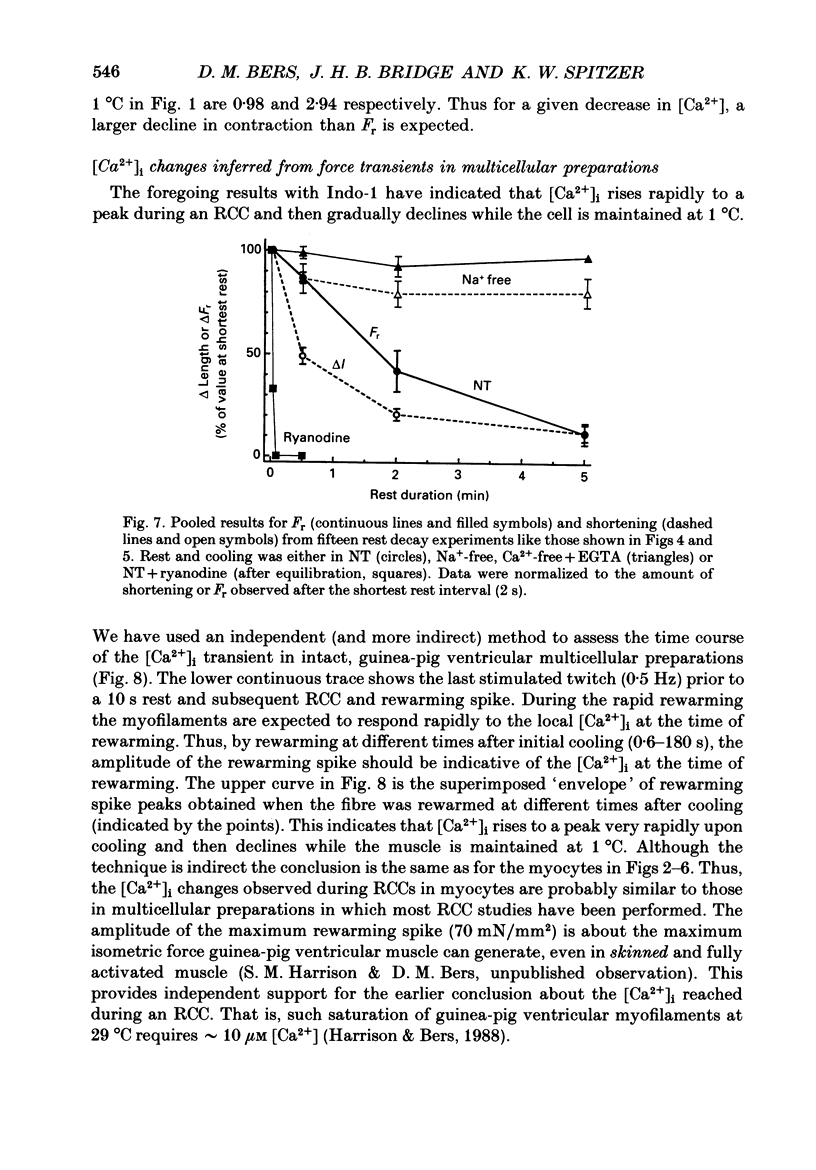
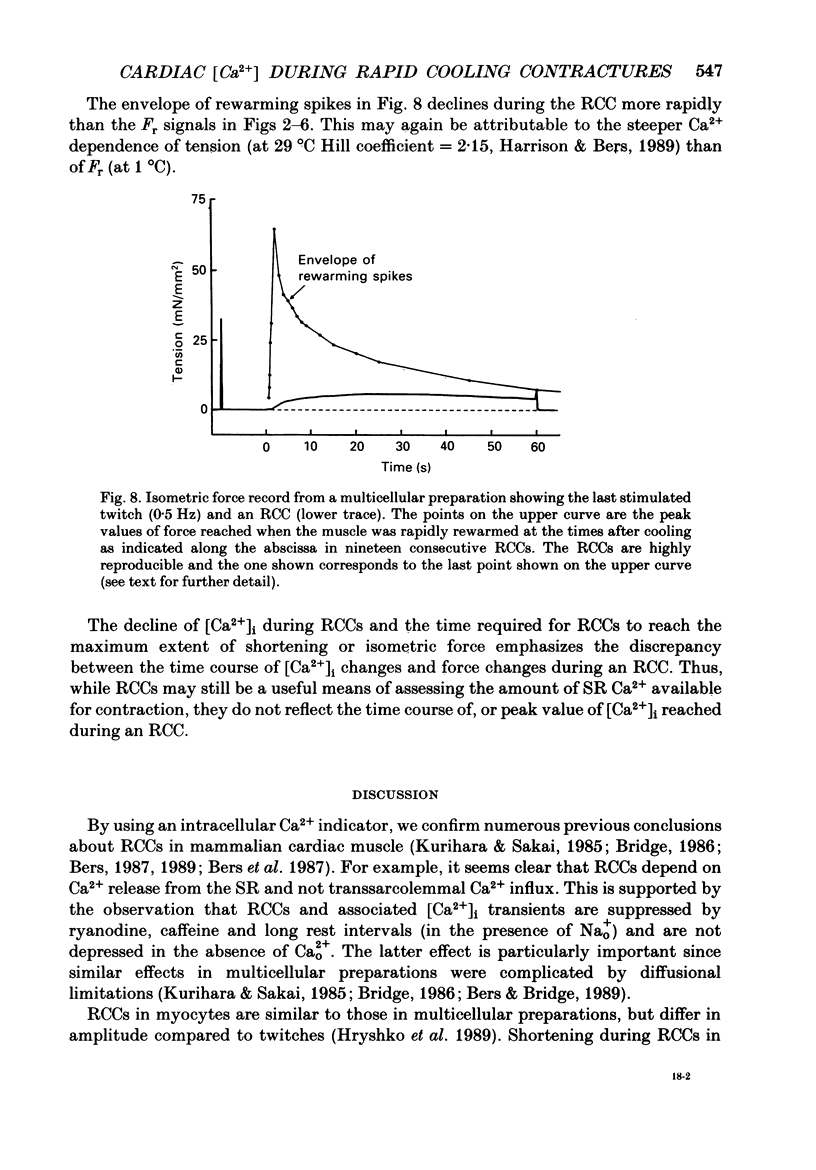
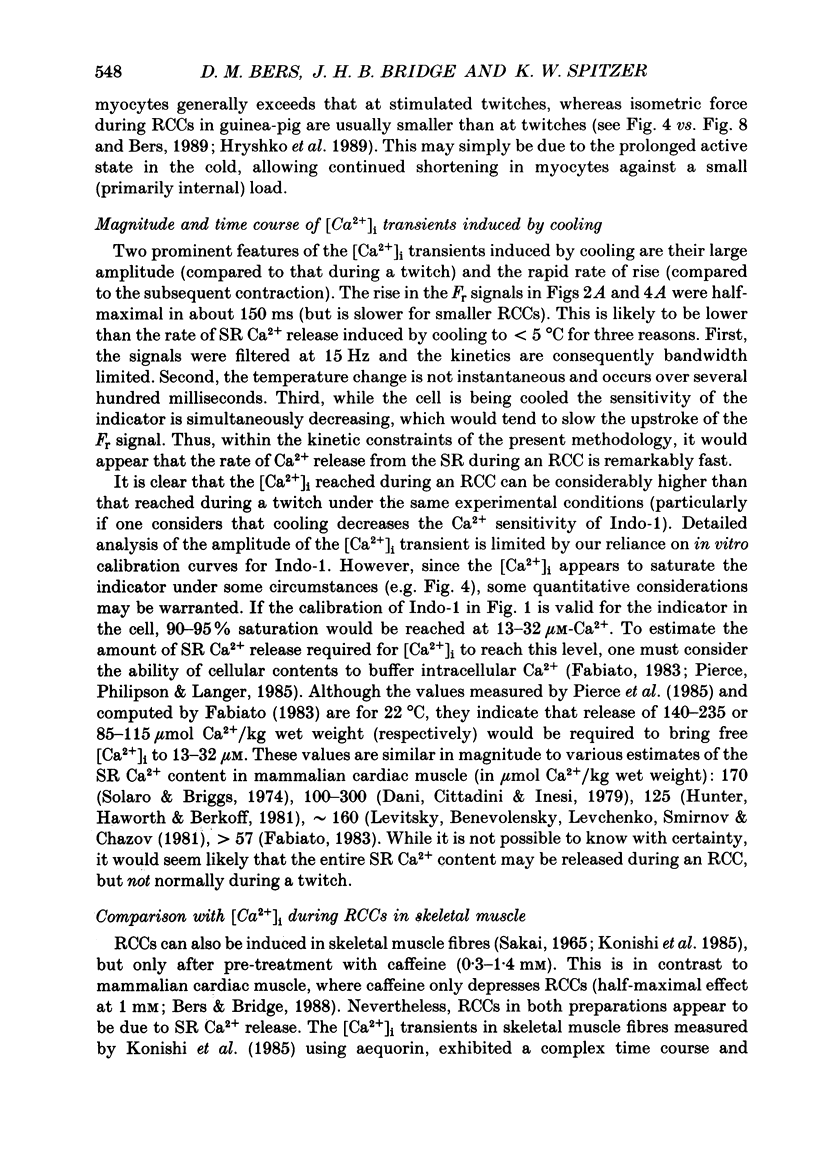
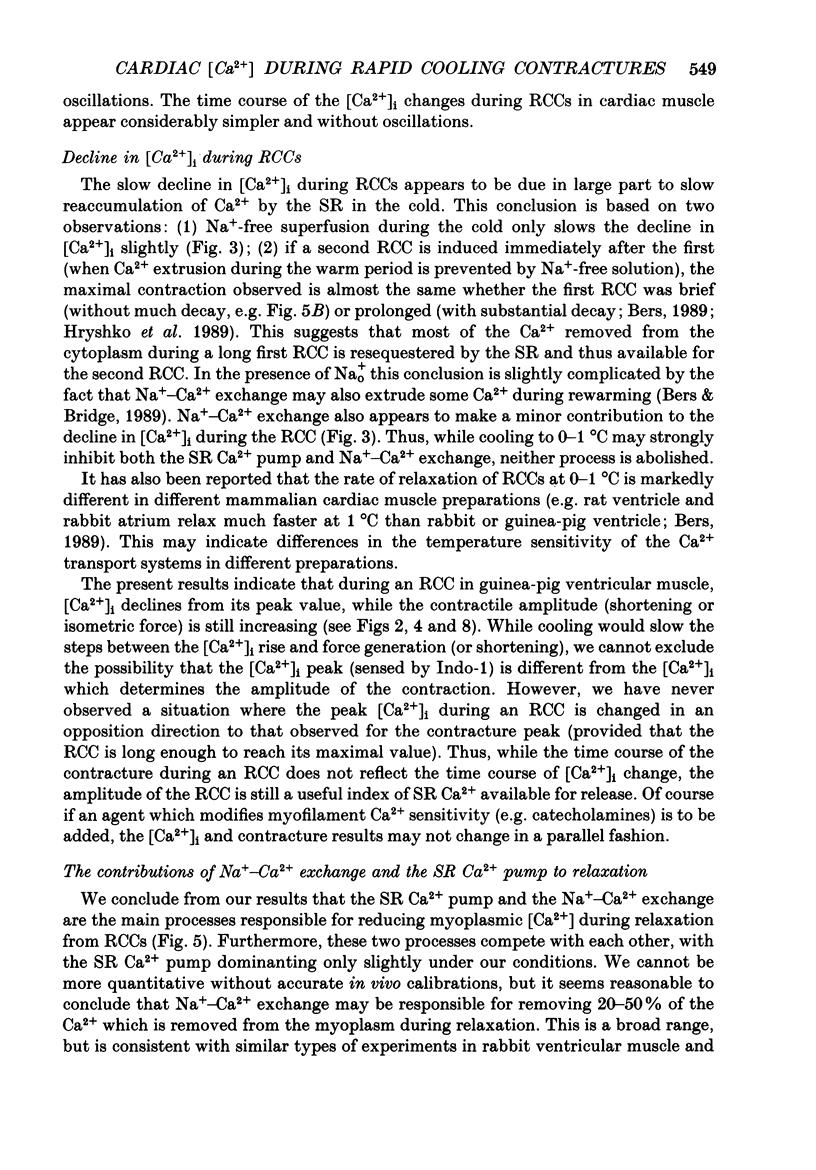
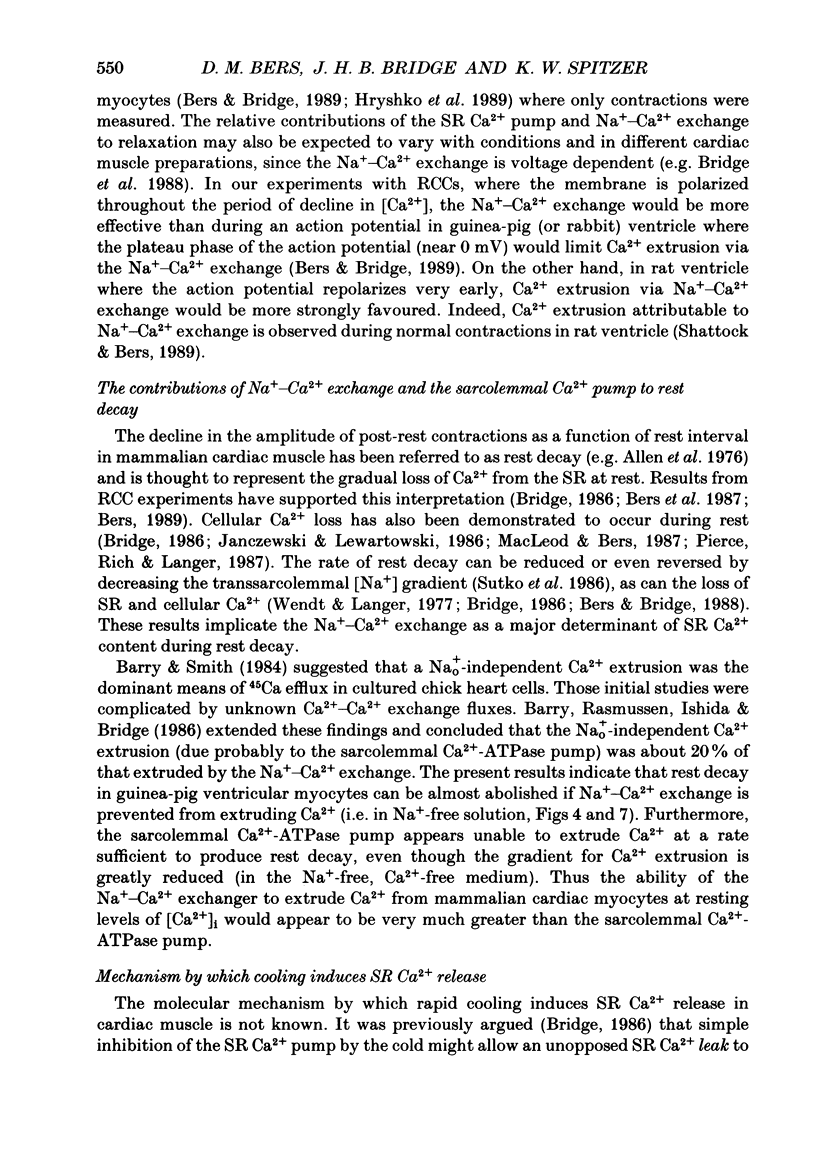
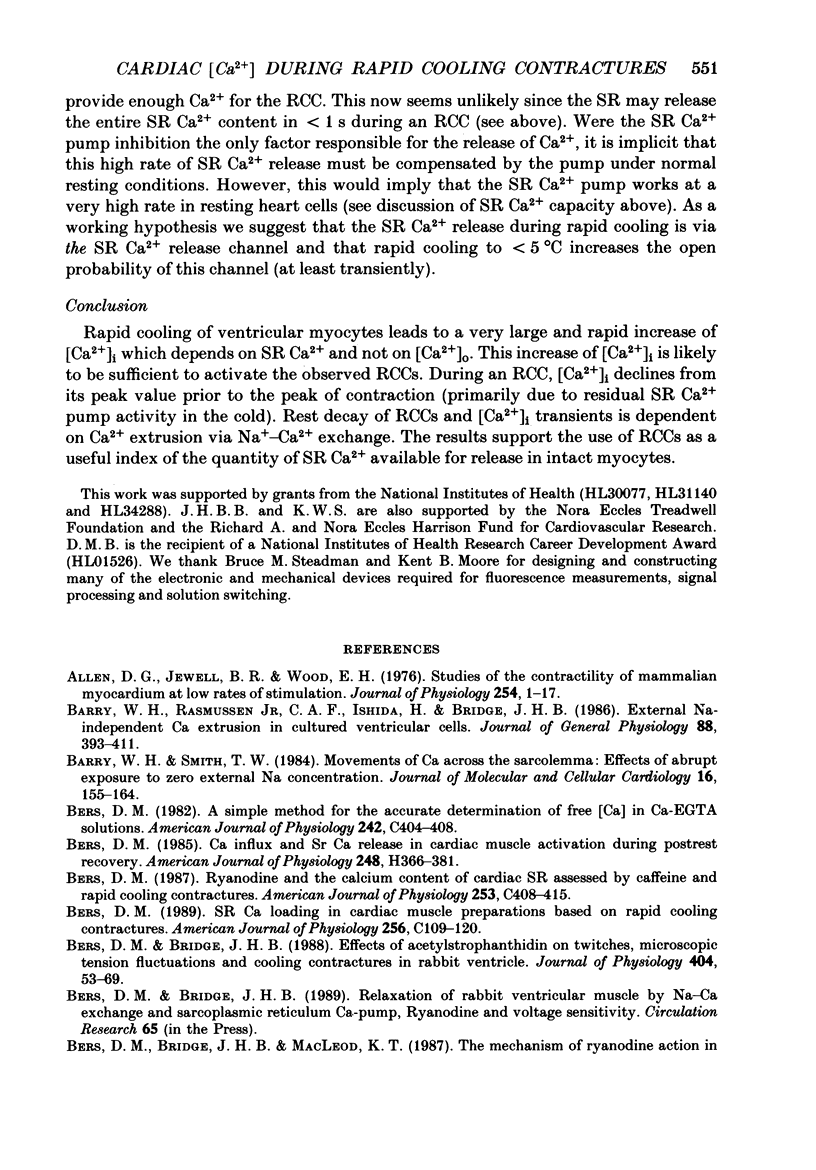
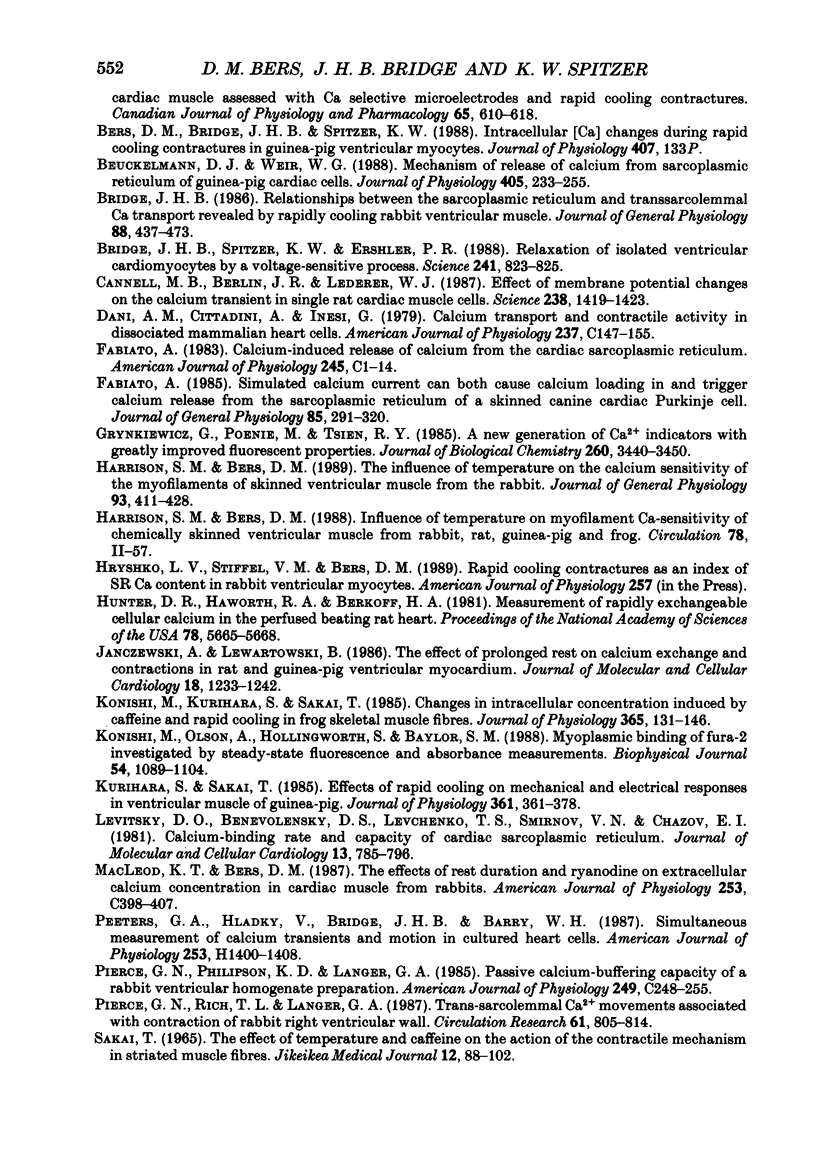
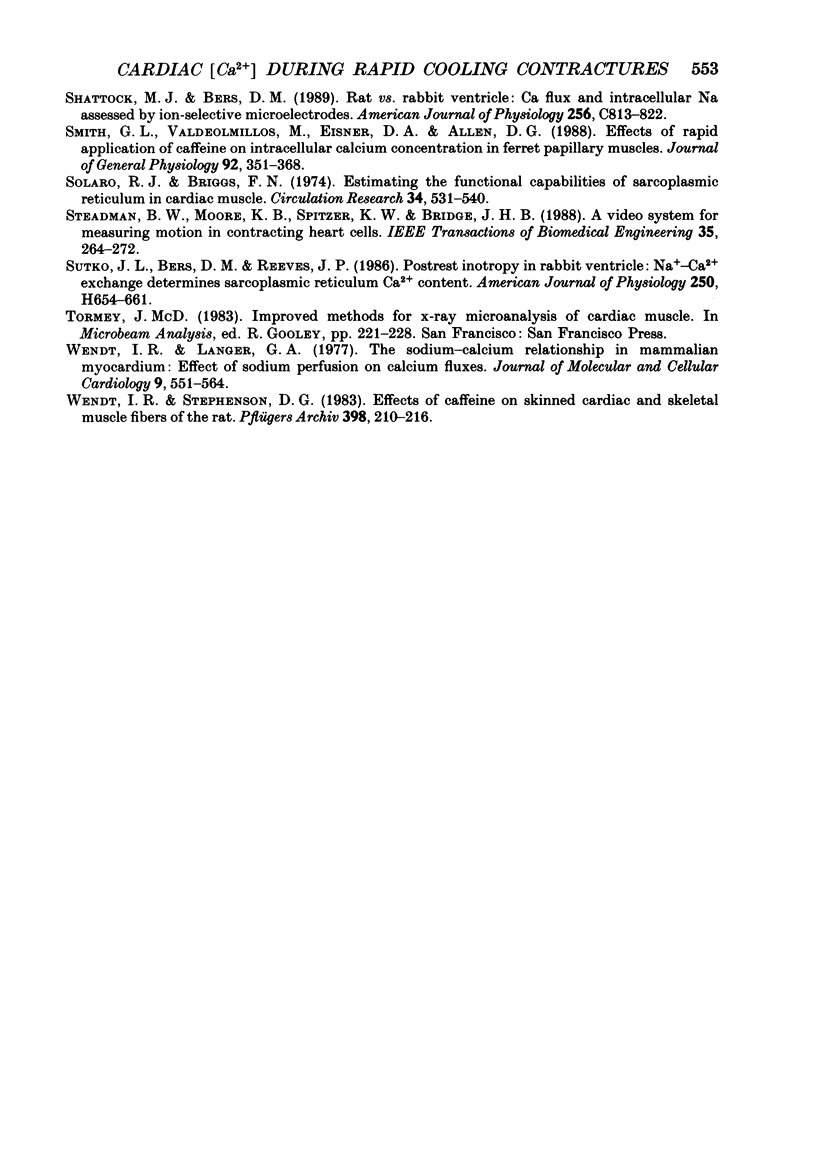
Selected References
These references are in PubMed. This may not be the complete list of references from this article.
- Allen D. G., Jewell B. R., Wood E. H. Studies of the contractility of mammalian myocardium at low rates of stimulation. J Physiol. 1976 Jan;254(1):1–17. doi: 10.1113/jphysiol.1976.sp011217. [DOI] [PMC free article] [PubMed] [Google Scholar]
- Barry W. H., Rasmussen C. A., Jr, Ishida H., Bridge J. H. External Na-independent Ca extrusion in cultured ventricular cells. Magnitude and functional significance. J Gen Physiol. 1986 Sep;88(3):393–411. doi: 10.1085/jgp.88.3.393. [DOI] [PMC free article] [PubMed] [Google Scholar]
- Barry W. H., Smith T. W. Movement of Ca2+ across the sarcolemma: effects of abrupt exposure to zero external Na concentration. J Mol Cell Cardiol. 1984 Feb;16(2):155–164. doi: 10.1016/s0022-2828(84)80704-x. [DOI] [PubMed] [Google Scholar]
- Bers D. M. A simple method for the accurate determination of free [Ca] in Ca-EGTA solutions. Am J Physiol. 1982 May;242(5):C404–C408. doi: 10.1152/ajpcell.1982.242.5.C404. [DOI] [PubMed] [Google Scholar]
- Bers D. M., Bridge J. H. Effect of acetylstrophanthidin on twitches, microscopic tension fluctuations and cooling contractures in rabbit ventricle. J Physiol. 1988 Oct;404:53–69. doi: 10.1113/jphysiol.1988.sp017278. [DOI] [PMC free article] [PubMed] [Google Scholar]
- Bers D. M., Bridge J. H., MacLeod K. T. The mechanism of ryanodine action in rabbit ventricular muscle evaluated with Ca-selective microelectrodes and rapid cooling contractures. Can J Physiol Pharmacol. 1987 Apr;65(4):610–618. doi: 10.1139/y87-103. [DOI] [PubMed] [Google Scholar]
- Bers D. M. Ca influx and sarcoplasmic reticulum Ca release in cardiac muscle activation during postrest recovery. Am J Physiol. 1985 Mar;248(3 Pt 2):H366–H381. doi: 10.1152/ajpheart.1985.248.3.H366. [DOI] [PubMed] [Google Scholar]
- Bers D. M. Ryanodine and the calcium content of cardiac SR assessed by caffeine and rapid cooling contractures. Am J Physiol. 1987 Sep;253(3 Pt 1):C408–C415. doi: 10.1152/ajpcell.1987.253.3.C408. [DOI] [PubMed] [Google Scholar]
- Bers D. M. SR Ca loading in cardiac muscle preparations based on rapid-cooling contractures. Am J Physiol. 1989 Jan;256(1 Pt 1):C109–C120. doi: 10.1152/ajpcell.1989.256.1.C109. [DOI] [PubMed] [Google Scholar]
- Beuckelmann D. J., Wier W. G. Mechanism of release of calcium from sarcoplasmic reticulum of guinea-pig cardiac cells. J Physiol. 1988 Nov;405:233–255. doi: 10.1113/jphysiol.1988.sp017331. [DOI] [PMC free article] [PubMed] [Google Scholar]
- Bridge J. H. Relationships between the sarcoplasmic reticulum and sarcolemmal calcium transport revealed by rapidly cooling rabbit ventricular muscle. J Gen Physiol. 1986 Oct;88(4):437–473. doi: 10.1085/jgp.88.4.437. [DOI] [PMC free article] [PubMed] [Google Scholar]
- Bridge J. H., Spitzer K. W., Ershler P. R. Relaxation of isolated ventricular cardiomyocytes by a voltage-dependent process. Science. 1988 Aug 12;241(4867):823–825. doi: 10.1126/science.3406740. [DOI] [PubMed] [Google Scholar]
- Cannell M. B., Berlin J. R., Lederer W. J. Effect of membrane potential changes on the calcium transient in single rat cardiac muscle cells. Science. 1987 Dec 4;238(4832):1419–1423. doi: 10.1126/science.2446391. [DOI] [PubMed] [Google Scholar]
- Dani A. M., Cittadini A., Inesi G. Calcium transport and contractile activity in dissociated mammalian heart cells. Am J Physiol. 1979 Sep;237(3):C147–C155. doi: 10.1152/ajpcell.1979.237.3.C147. [DOI] [PubMed] [Google Scholar]
- Fabiato A. Calcium-induced release of calcium from the cardiac sarcoplasmic reticulum. Am J Physiol. 1983 Jul;245(1):C1–14. doi: 10.1152/ajpcell.1983.245.1.C1. [DOI] [PubMed] [Google Scholar]
- Fabiato A. Simulated calcium current can both cause calcium loading in and trigger calcium release from the sarcoplasmic reticulum of a skinned canine cardiac Purkinje cell. J Gen Physiol. 1985 Feb;85(2):291–320. doi: 10.1085/jgp.85.2.291. [DOI] [PMC free article] [PubMed] [Google Scholar]
- Grynkiewicz G., Poenie M., Tsien R. Y. A new generation of Ca2+ indicators with greatly improved fluorescence properties. J Biol Chem. 1985 Mar 25;260(6):3440–3450. [PubMed] [Google Scholar]
- Harrison S. M., Bers D. M. Influence of temperature on the calcium sensitivity of the myofilaments of skinned ventricular muscle from the rabbit. J Gen Physiol. 1989 Mar;93(3):411–428. doi: 10.1085/jgp.93.3.411. [DOI] [PMC free article] [PubMed] [Google Scholar]
- Hunter D. R., Haworth R. A., Berkoff H. A. Measurement of rapidly exchangeable cellular calcium in the perfused beating rat heart. Proc Natl Acad Sci U S A. 1981 Sep;78(9):5665–5668. doi: 10.1073/pnas.78.9.5665. [DOI] [PMC free article] [PubMed] [Google Scholar]
- Janczewski A. M., Lewartowski B. The effect of prolonged rest on calcium exchange and contractions in rat and guinea-pig ventricular myocardium. J Mol Cell Cardiol. 1986 Dec;18(12):1233–1242. doi: 10.1016/s0022-2828(86)80427-8. [DOI] [PubMed] [Google Scholar]
- Konishi M., Kurihara S., Sakai T. Change in intracellular calcium ion concentration induced by caffeine and rapid cooling in frog skeletal muscle fibres. J Physiol. 1985 Aug;365:131–146. doi: 10.1113/jphysiol.1985.sp015763. [DOI] [PMC free article] [PubMed] [Google Scholar]
- Konishi M., Olson A., Hollingworth S., Baylor S. M. Myoplasmic binding of fura-2 investigated by steady-state fluorescence and absorbance measurements. Biophys J. 1988 Dec;54(6):1089–1104. doi: 10.1016/S0006-3495(88)83045-5. [DOI] [PMC free article] [PubMed] [Google Scholar]
- Kurihara S., Sakai T. Effects of rapid cooling on mechanical and electrical responses in ventricular muscle of guinea-pig. J Physiol. 1985 Apr;361:361–378. doi: 10.1113/jphysiol.1985.sp015650. [DOI] [PMC free article] [PubMed] [Google Scholar]
- Levitsky D. O., Benevolensky D. S., Levchenko T. S., Smirnov V. N., Chazov E. I. Calcium-binding rate and capacity of cardiac sarcoplasmic reticulum. J Mol Cell Cardiol. 1981 Sep;13(9):785–796. doi: 10.1016/0022-2828(81)90236-4. [DOI] [PubMed] [Google Scholar]
- MacLeod K. T., Bers D. M. Effects of rest duration and ryanodine on changes of extracellular [Ca] in cardiac muscle from rabbits. Am J Physiol. 1987 Sep;253(3 Pt 1):C398–C407. doi: 10.1152/ajpcell.1987.253.3.C398. [DOI] [PubMed] [Google Scholar]
- Peeters G. A., Hlady V., Bridge J. H., Barry W. H. Simultaneous measurement of calcium transients and motion in cultured heart cells. Am J Physiol. 1987 Dec;253(6 Pt 2):H1400–H1408. doi: 10.1152/ajpheart.1987.253.6.H1400. [DOI] [PubMed] [Google Scholar]
- Pierce G. N., Philipson K. D., Langer G. A. Passive calcium-buffering capacity of a rabbit ventricular homogenate preparation. Am J Physiol. 1985 Sep;249(3 Pt 1):C248–C255. doi: 10.1152/ajpcell.1985.249.3.C248. [DOI] [PubMed] [Google Scholar]
- Pierce G. N., Rich T. L., Langer G. A. Trans-sarcolemmal Ca2+ movements associated with contraction of the rabbit right ventricular wall. Circ Res. 1987 Dec;61(6):805–814. doi: 10.1161/01.res.61.6.805. [DOI] [PubMed] [Google Scholar]
- Shattock M. J., Bers D. M. Rat vs. rabbit ventricle: Ca flux and intracellular Na assessed by ion-selective microelectrodes. Am J Physiol. 1989 Apr;256(4 Pt 1):C813–C822. doi: 10.1152/ajpcell.1989.256.4.C813. [DOI] [PubMed] [Google Scholar]
- Smith G. L., Valdeolmillos M., Eisner D. A., Allen D. G. Effects of rapid application of caffeine on intracellular calcium concentration in ferret papillary muscles. J Gen Physiol. 1988 Sep;92(3):351–368. doi: 10.1085/jgp.92.3.351. [DOI] [PMC free article] [PubMed] [Google Scholar]
- Solaro R. J., Briggs F. N. Estimating the functional capabilities of sarcoplasmic reticulum in cardiac muscle. Calcium binding. Circ Res. 1974 Apr;34(4):531–540. doi: 10.1161/01.res.34.4.531. [DOI] [PubMed] [Google Scholar]
- Steadman B. W., Moore K. B., Spitzer K. W., Bridge J. H. A video system for measuring motion in contracting heart cells. IEEE Trans Biomed Eng. 1988 Apr;35(4):264–272. doi: 10.1109/10.1375. [DOI] [PubMed] [Google Scholar]
- Sutko J. L., Bers D. M., Reeves J. P. Postrest inotropy in rabbit ventricle: Na+-Ca2+ exchange determines sarcoplasmic reticulum Ca2+ content. Am J Physiol. 1986 Apr;250(4 Pt 2):H654–H661. doi: 10.1152/ajpheart.1986.250.4.H654. [DOI] [PubMed] [Google Scholar]
- Wendt I. R., Langer G. A. The sodium-calcium relationship in mammalian myocardium: effect of sodium deficient perfusion on calcium fluxes. J Mol Cell Cardiol. 1977 Jul;9(7):551–564. doi: 10.1016/s0022-2828(77)80370-2. [DOI] [PubMed] [Google Scholar]
- Wendt I. R., Stephenson D. G. Effects of caffeine on Ca-activated force production in skinned cardiac and skeletal muscle fibres of the rat. Pflugers Arch. 1983 Aug;398(3):210–216. doi: 10.1007/BF00657153. [DOI] [PubMed] [Google Scholar]