Abstract
1. Impaired intracellular Ca2+ concentration ([Ca2+]i) regulation may underlie alterations in neuronal function during hypoxia or hypoglycaemia and may initiate cell damage. We have used the Ca2(+)-sensitive fluorophore, Fura-2, to study the regulation of [Ca2+]i in neurones isolated from mouse dorsal root ganglia. Mean resting [Ca2+]i was 163 +/- 11 nM (mean +/- S.E.M., n = 38). 2. Depolarization by exposure to 20 or 30 mM-K+ caused a rapid Co2(+)- and Cd2(+)-sensitive rise in [Ca2+]i, which subsequently declined with a time course usually fitted by the sum of two exponential functions. 3. Interference with mitochondrial function (by CN- or FCPP) or with glycolysis (by glucose removal) all raised [Ca2+]i by up to 220%. Addition of FCCP in the presence of CN- further increased [Ca2+]i. The response to CN- was still seen in the absence of extracellular Ca2+, although it attenuated rapidly, indicating release from an intracellular store. 4. Either CN- or glucose removal increased the rise in [Ca2+]i induced by K+ 2- to 3-fold and slowed recovery, suggesting interference with sequestration or extrusion of [Ca2+]i. 5. Resting [Ca2+]i rose when external Na+ was replaced by Li+ or N-methyl-D-glucamine, demonstrating the presence of a Na(+)-Ca2+ exchange process. However, Na+ replacement had only a slight effect on the handling of a Ca2+ load. 6. We conclude that (i) Ca2+ is released into the cytoplasm from intracellular organelles when energy supplies are reduced: (ii) that the extrusion or sequestration of Ca2+ entering the cell during electrical activity is rapidly impaired by interference with mitochondrial metabolism: and (iii) Na(+)-Ca2+ exchange makes only a small contribution to intracellular Ca2+ homeostasis. 7. [Ca2+]i would thus be expected to rise in vivo during hypoxia or hypoglycaemia and may initiate alterations in neuronal function. However, if a rise in Ca2+ is an important cause of cell damage in cerebral hypoxaemia, the combination of excitation and hypoxia will lead to the largest increases in [Ca2+]i, while hypoxia alone appears to cause only a small increase in [Ca2+]i in quiescent cells.
Full text
PDF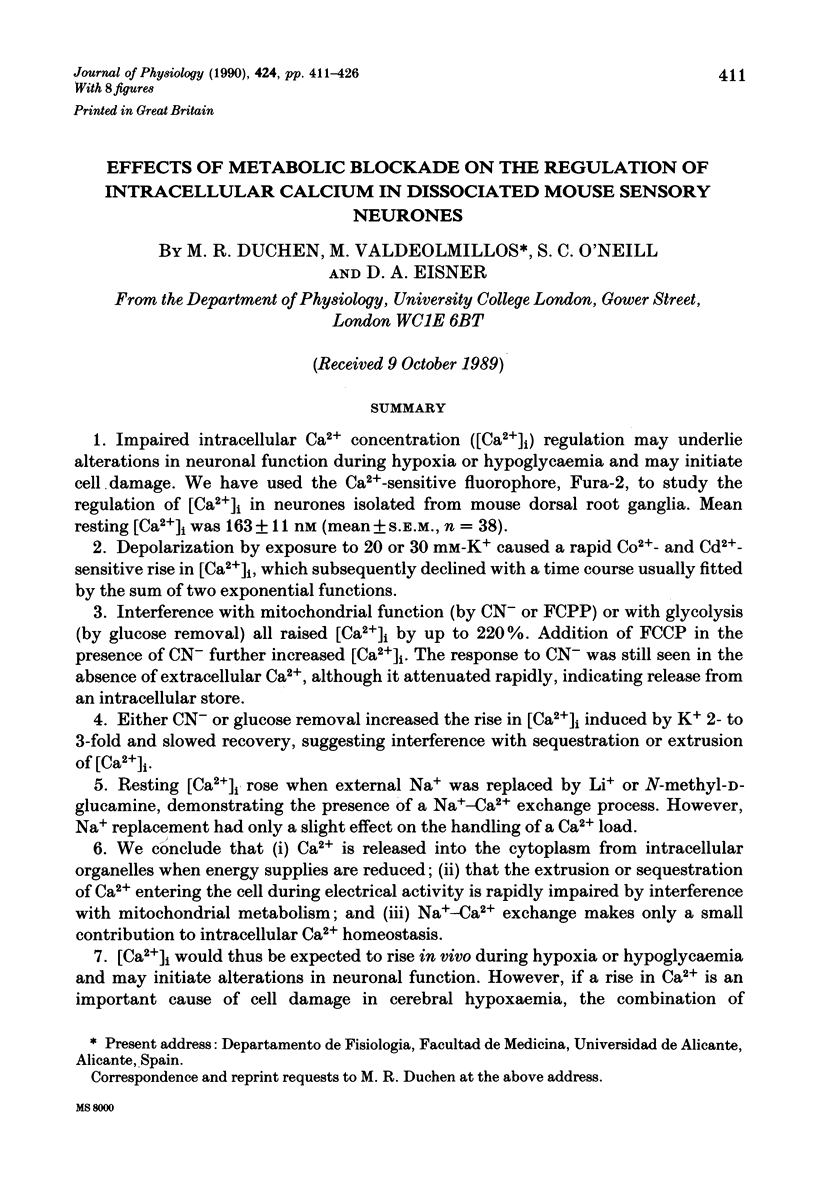
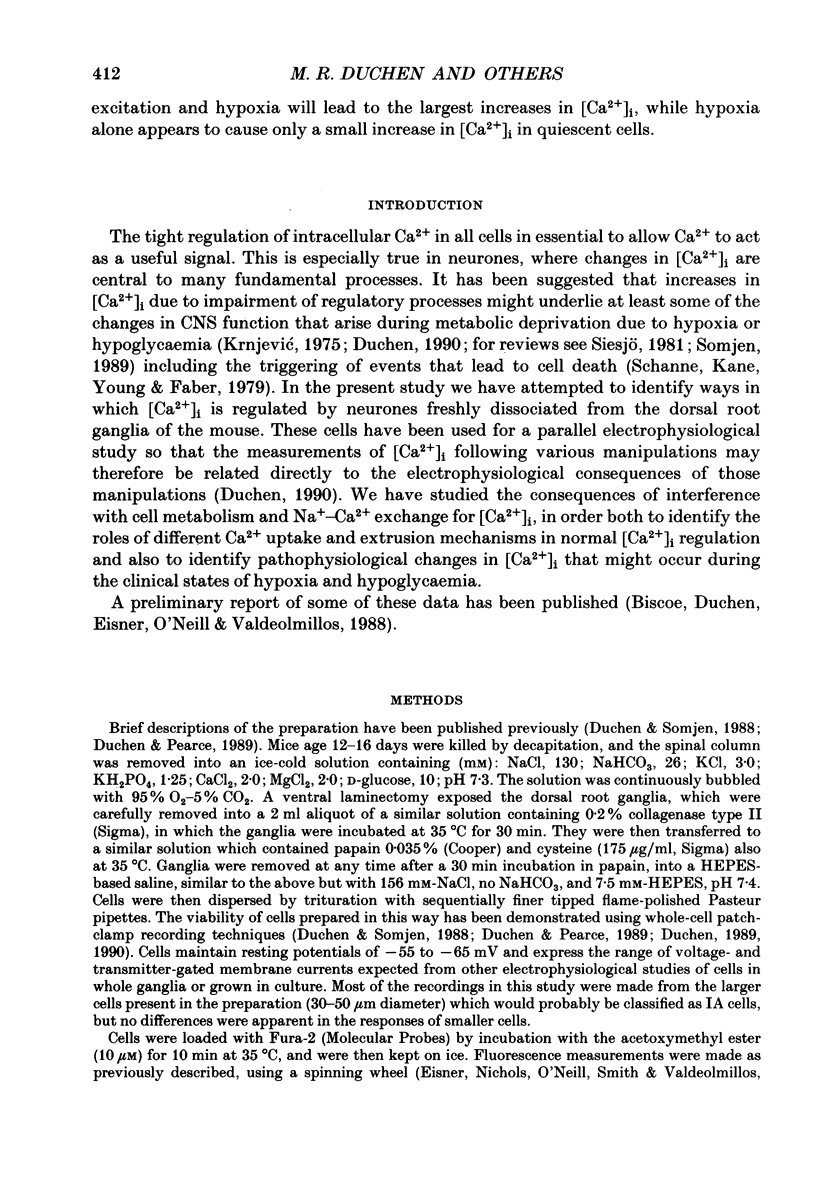
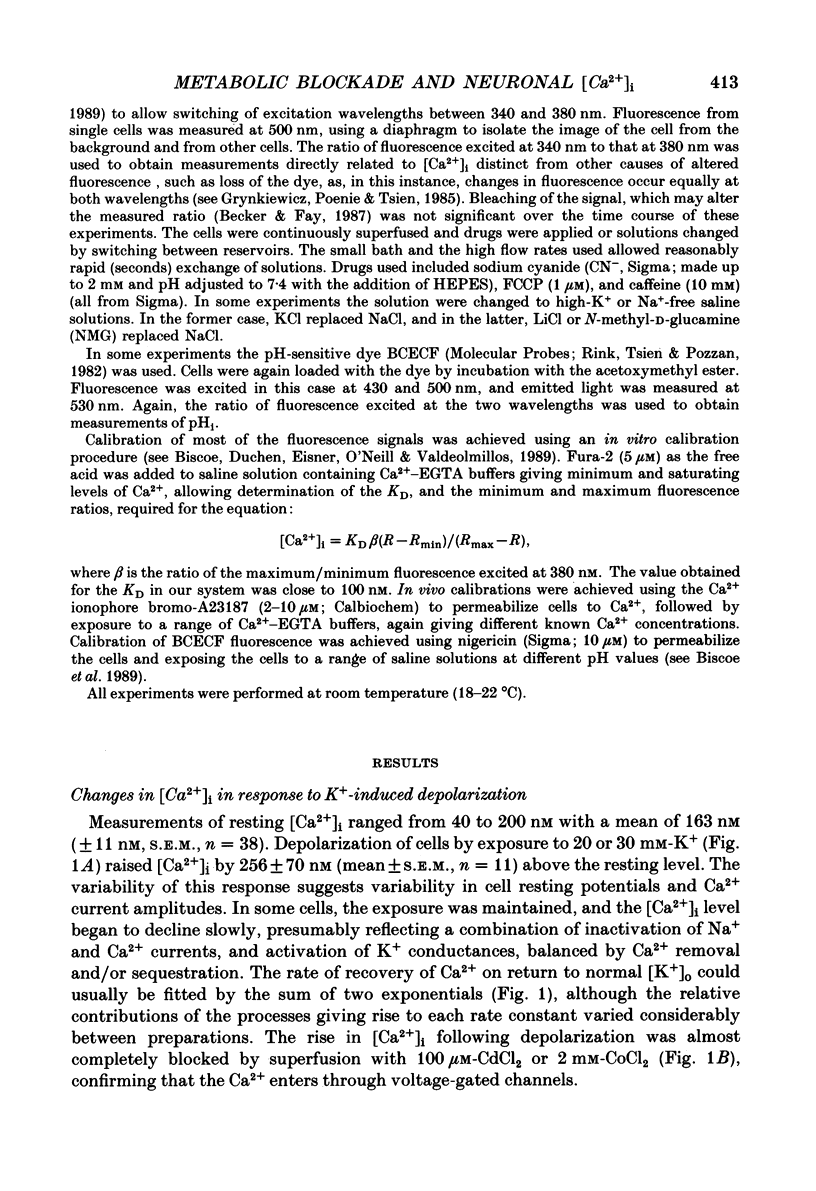
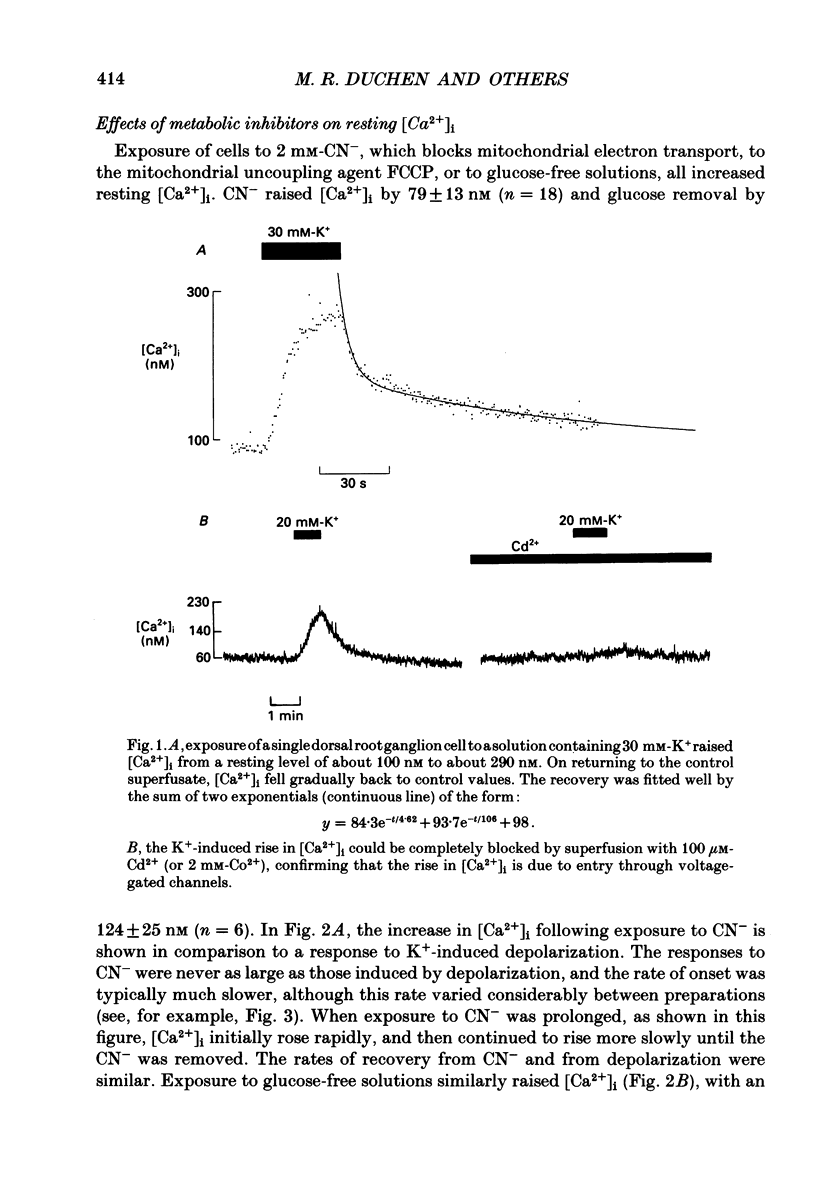
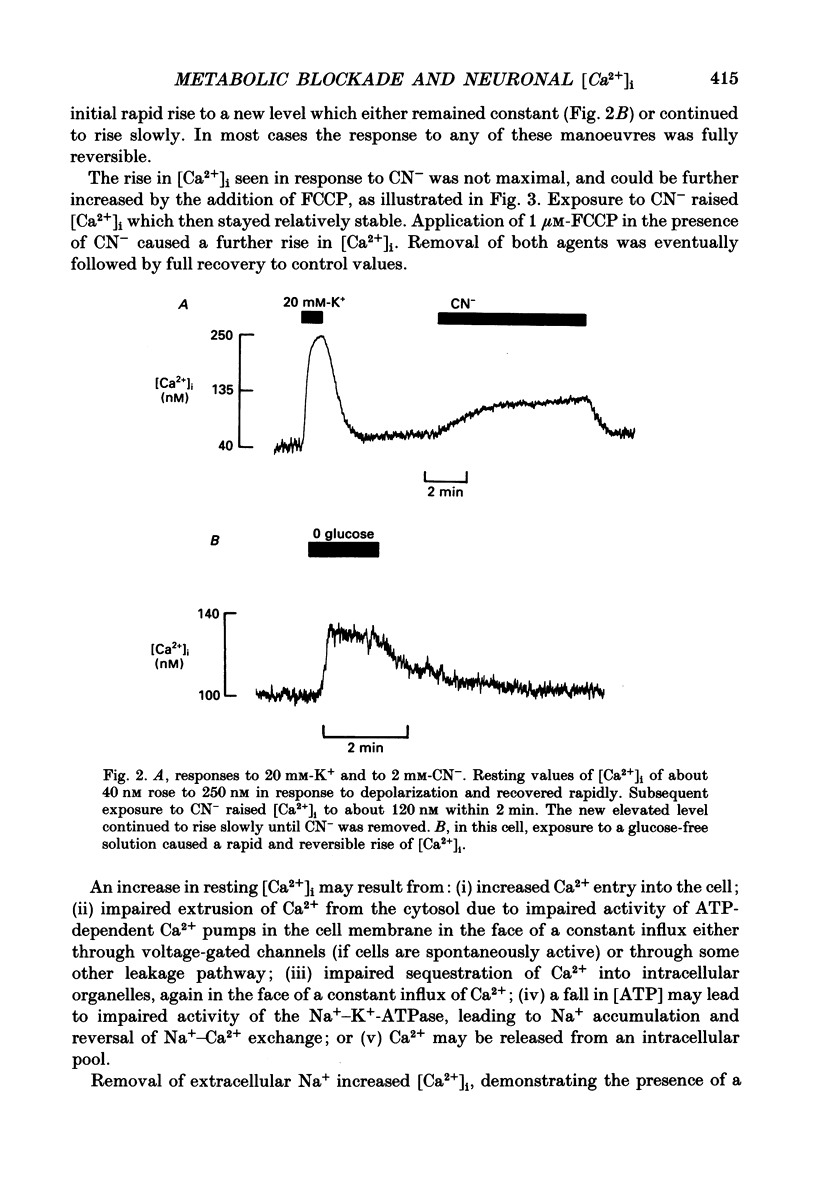
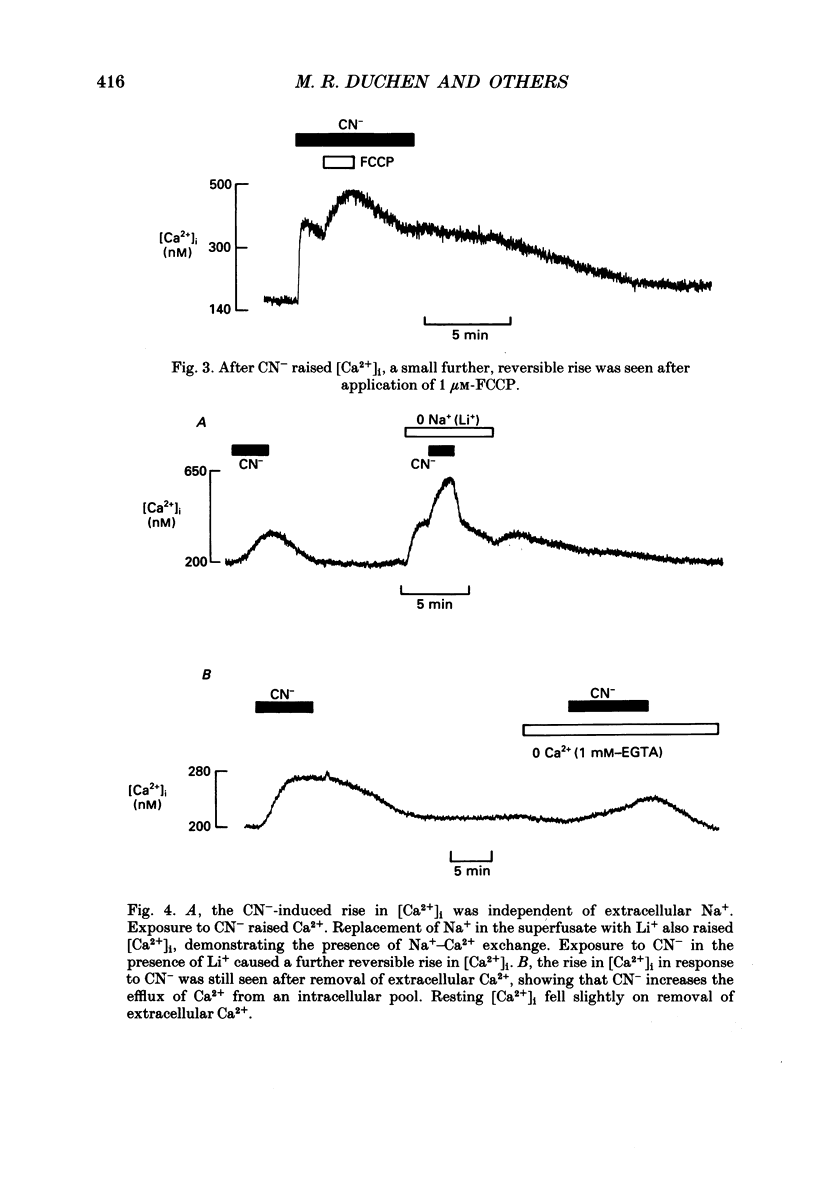
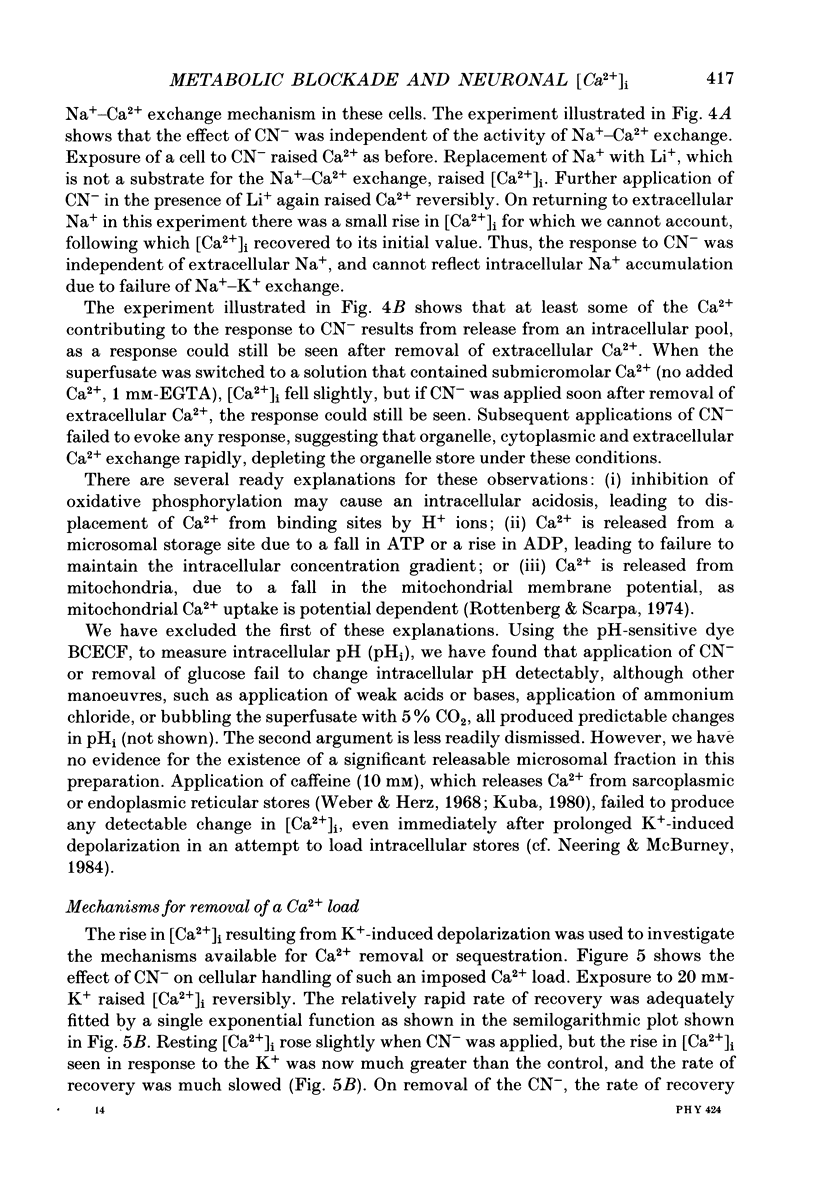
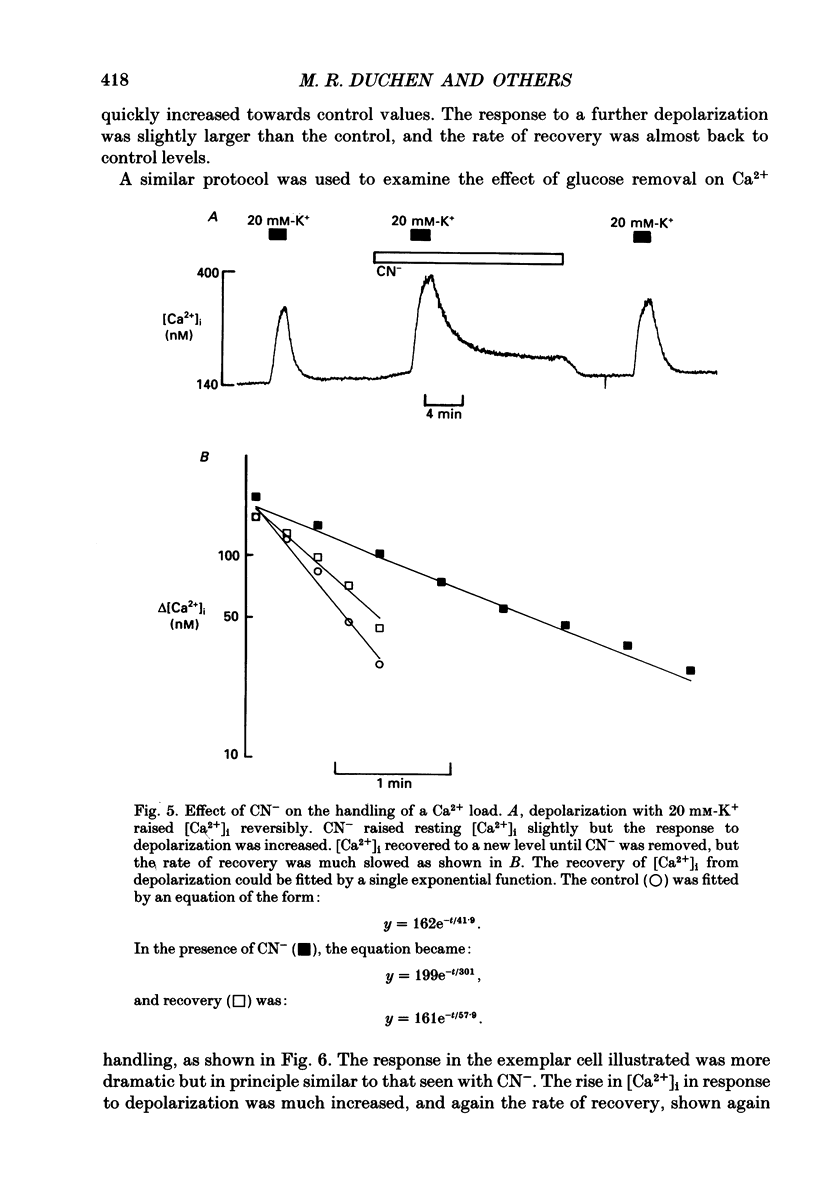
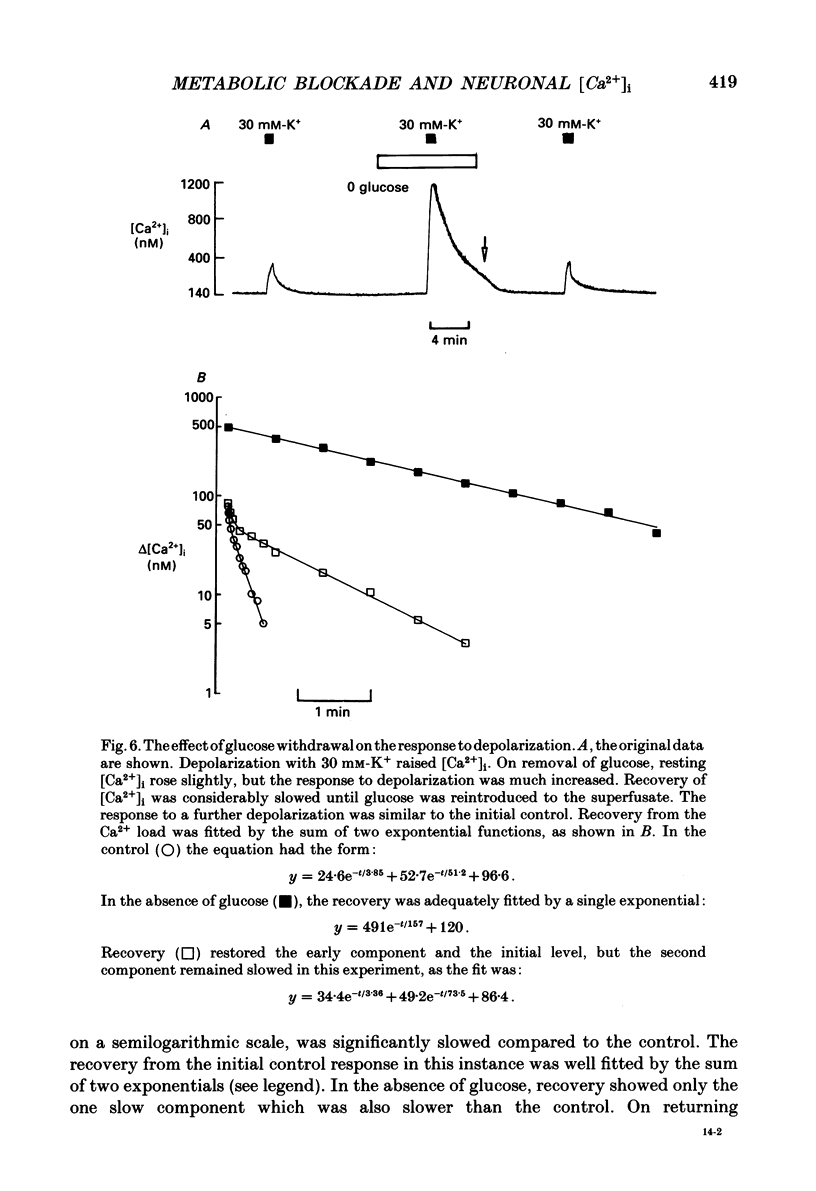
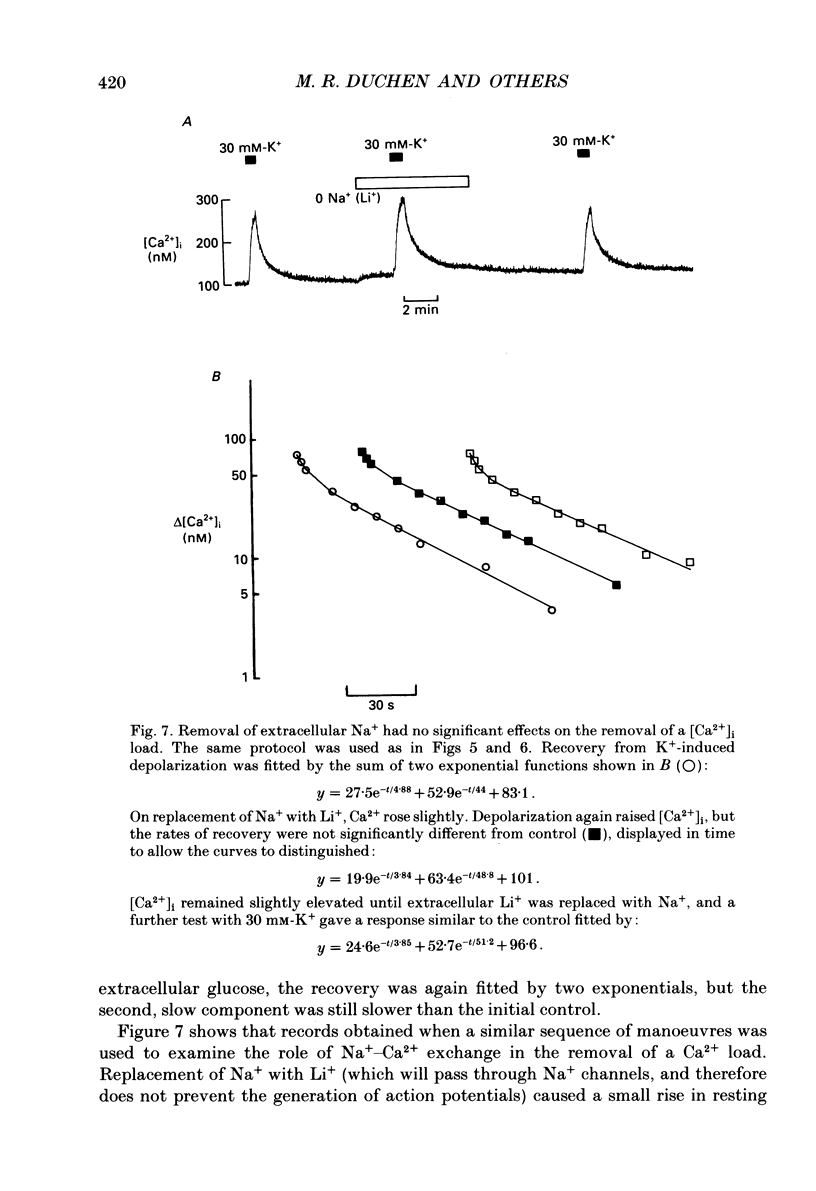
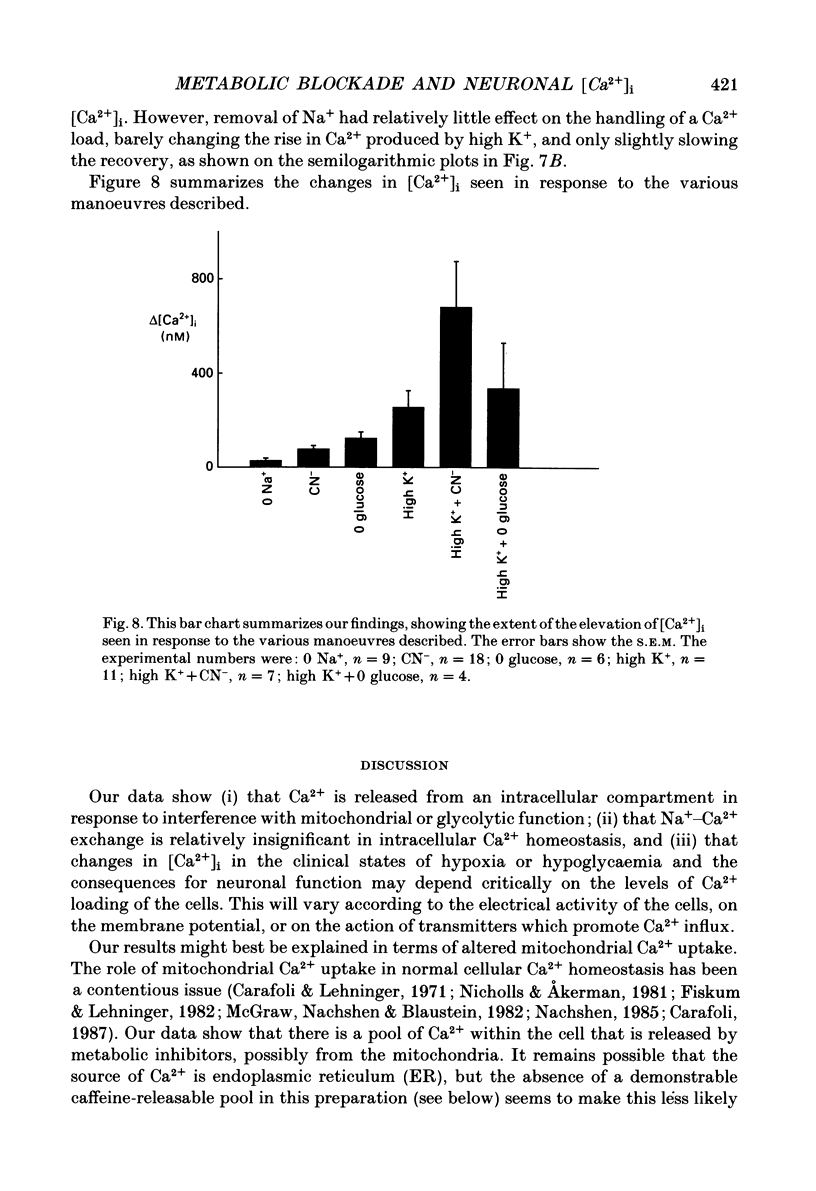
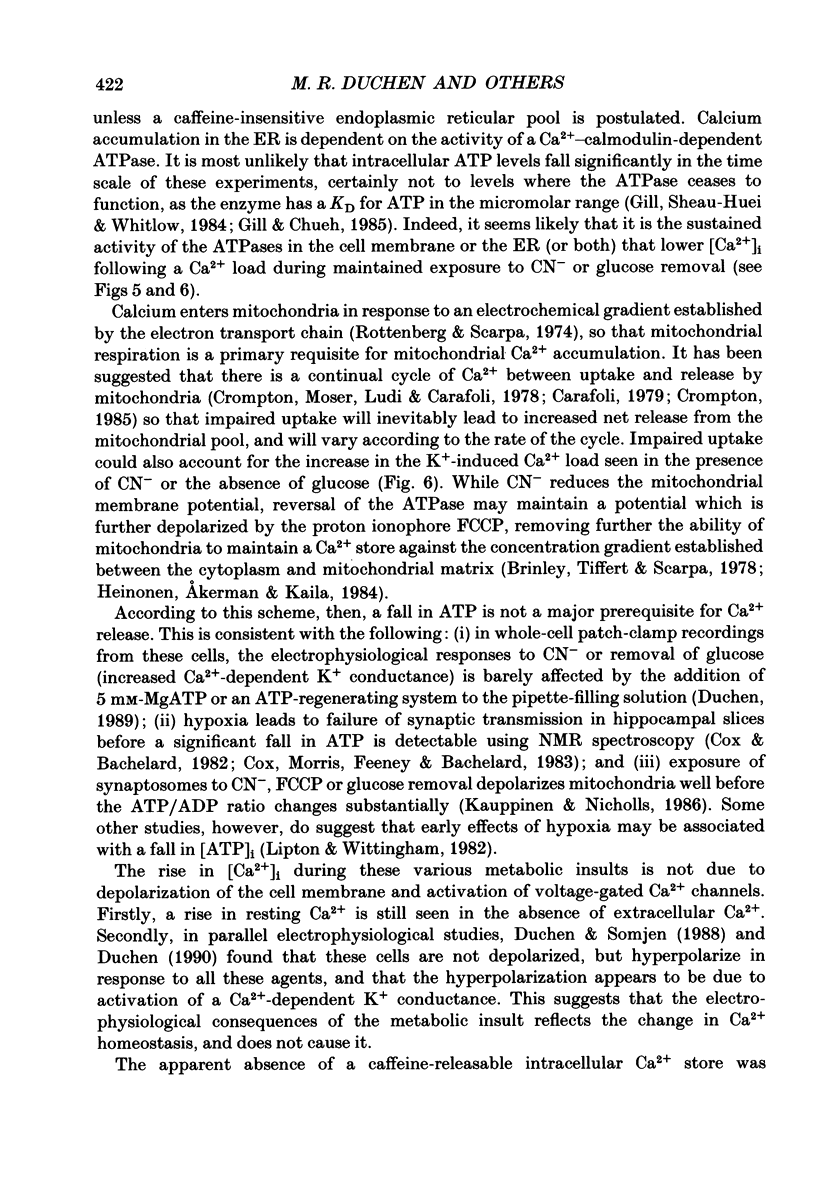
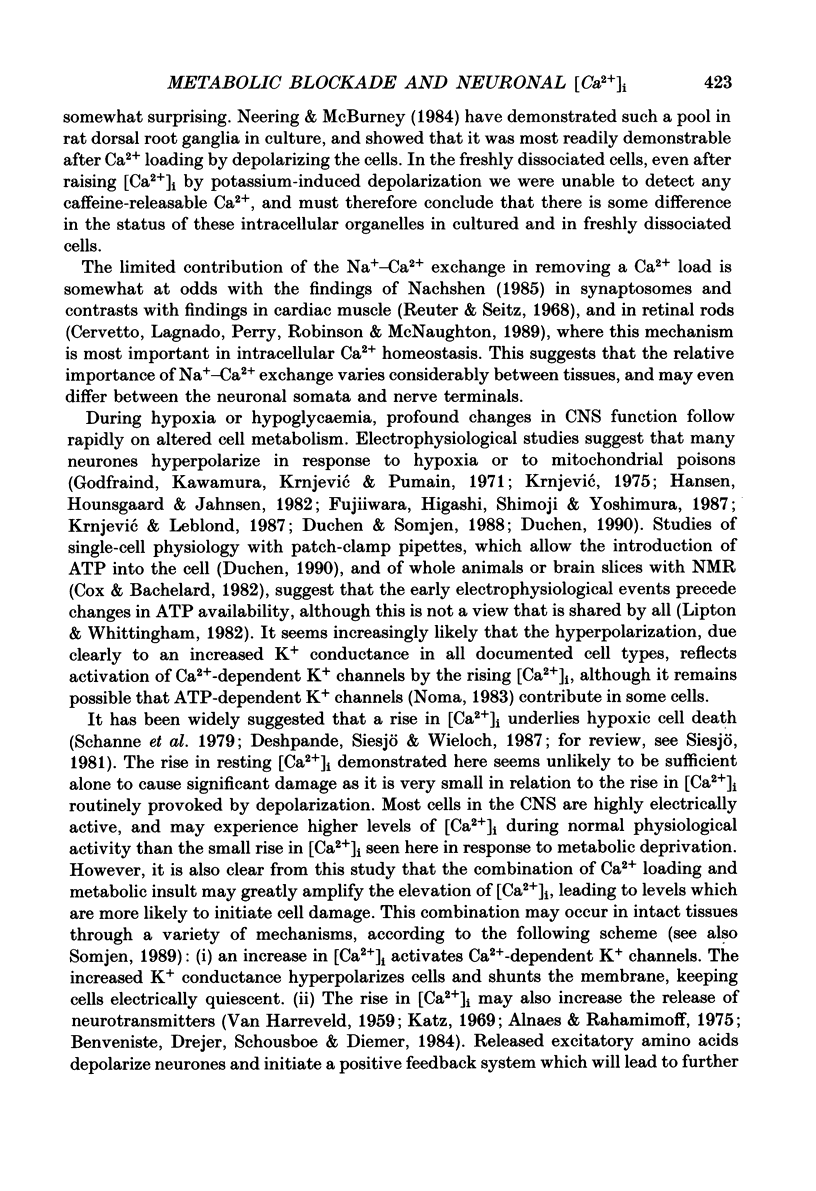
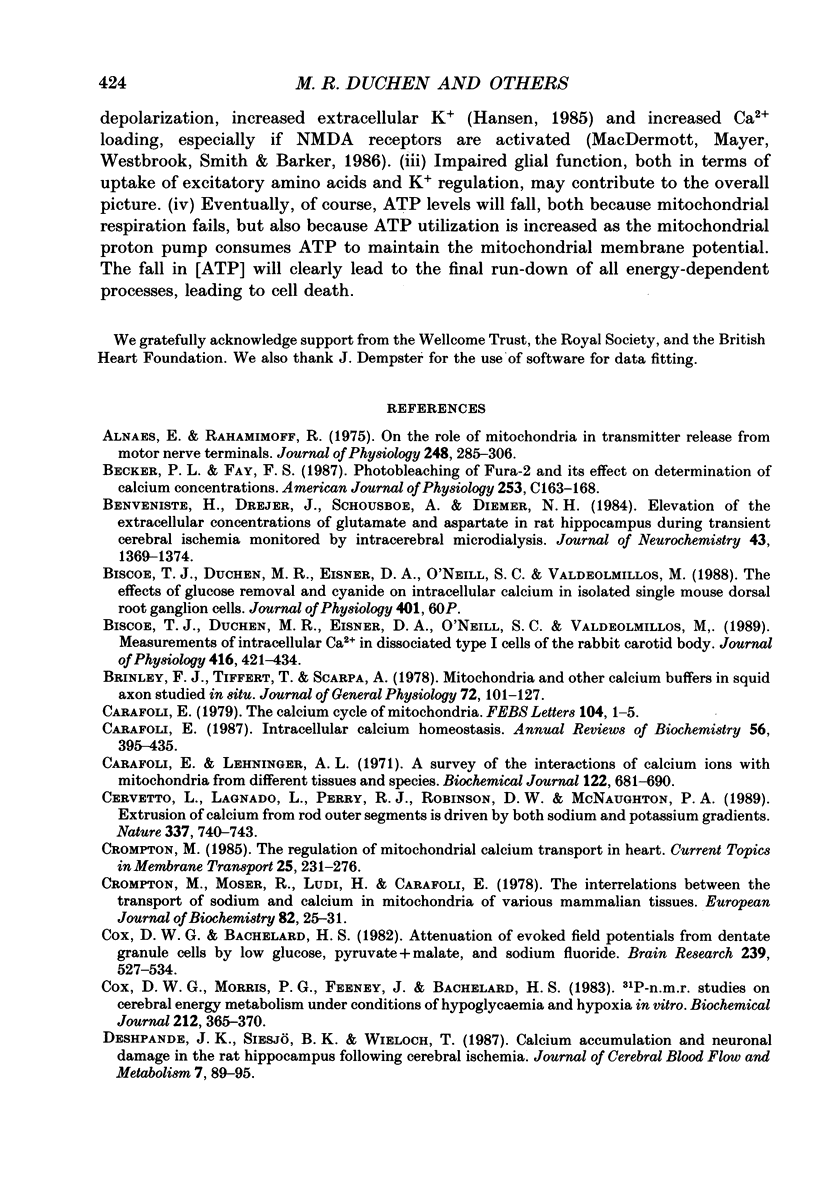
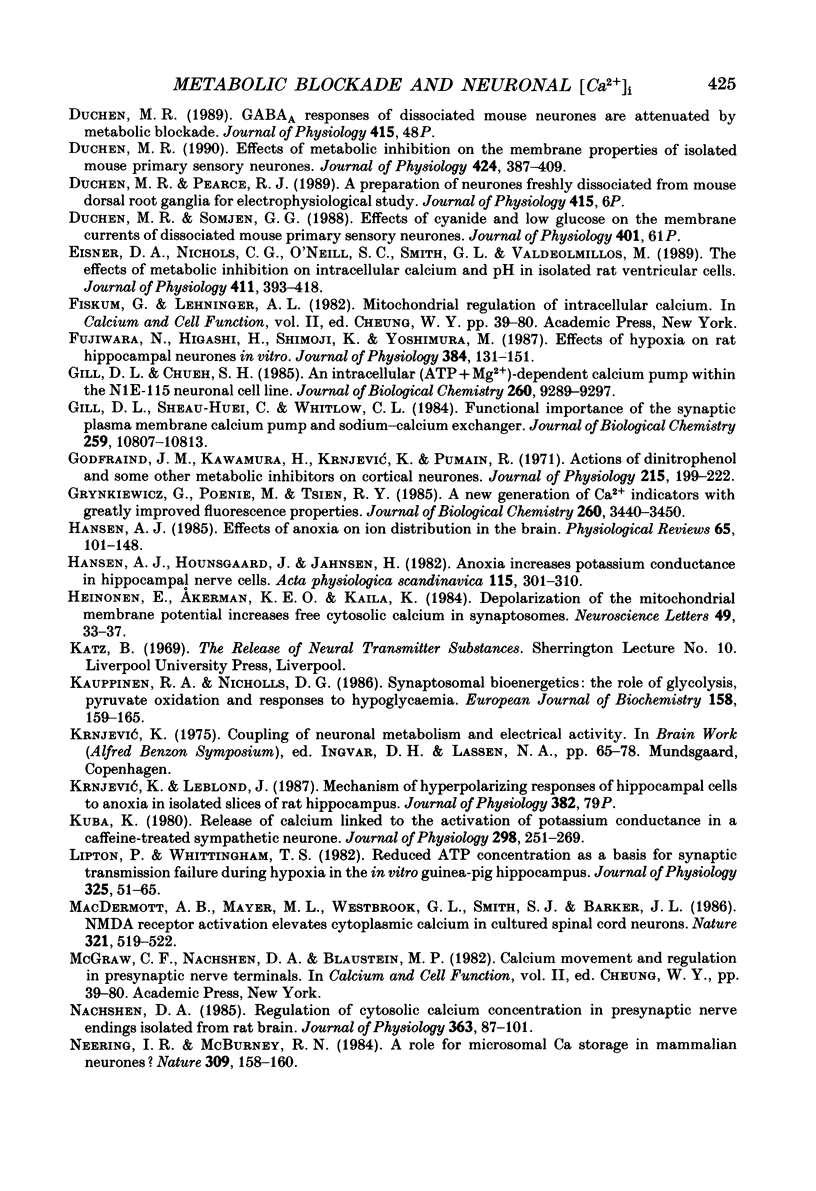
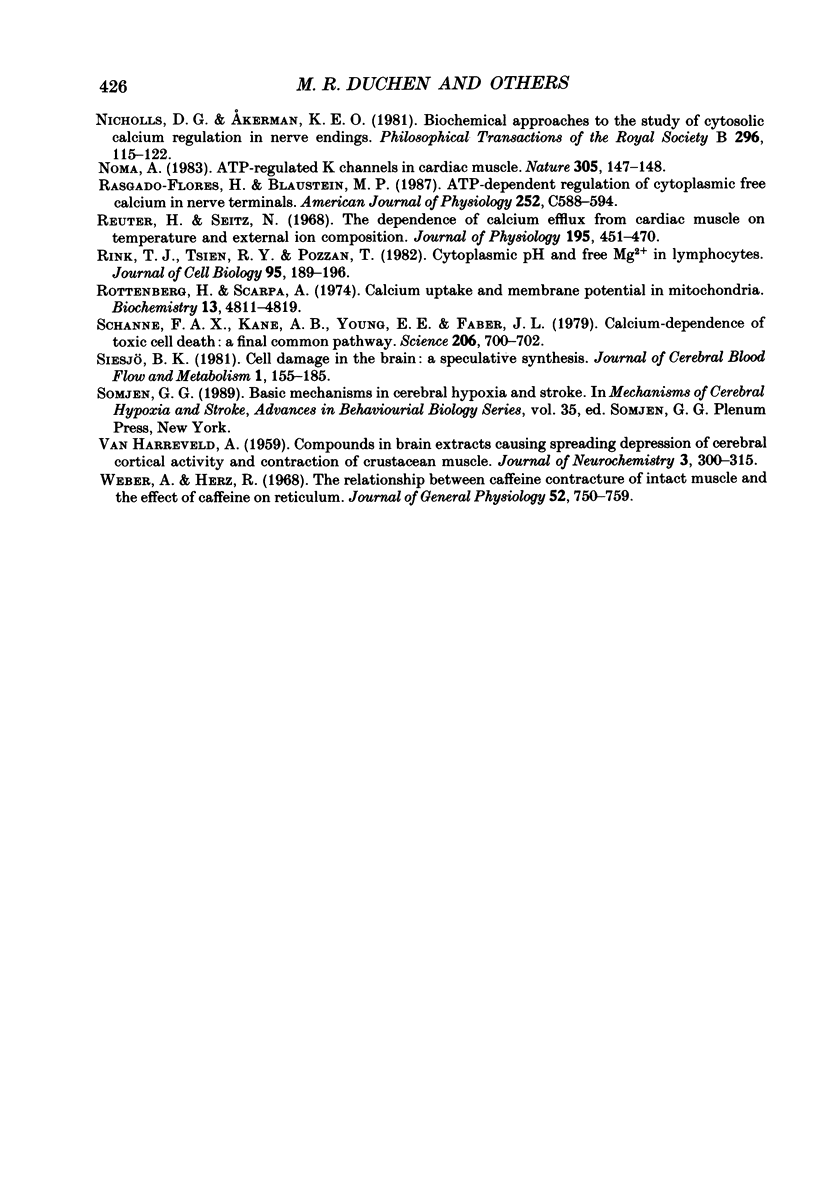
Selected References
These references are in PubMed. This may not be the complete list of references from this article.
- Alnaes E., Rahamimoff R. On the role of mitochondria in transmitter release from motor nerve terminals. J Physiol. 1975 Jun;248(2):285–306. doi: 10.1113/jphysiol.1975.sp010974. [DOI] [PMC free article] [PubMed] [Google Scholar]
- Benveniste H., Drejer J., Schousboe A., Diemer N. H. Elevation of the extracellular concentrations of glutamate and aspartate in rat hippocampus during transient cerebral ischemia monitored by intracerebral microdialysis. J Neurochem. 1984 Nov;43(5):1369–1374. doi: 10.1111/j.1471-4159.1984.tb05396.x. [DOI] [PubMed] [Google Scholar]
- Biscoe T. J., Duchen M. R., Eisner D. A., O'Neill S. C., Valdeolmillos M. Measurements of intracellular Ca2+ in dissociated type I cells of the rabbit carotid body. J Physiol. 1989 Sep;416:421–434. doi: 10.1113/jphysiol.1989.sp017769. [DOI] [PMC free article] [PubMed] [Google Scholar]
- Brinley F. J., Jr, Tiffert T., Scarpa A. Mitochondria and other calcium buffers of squid axon studied in situ. J Gen Physiol. 1978 Jul;72(1):101–127. doi: 10.1085/jgp.72.1.101. [DOI] [PMC free article] [PubMed] [Google Scholar]
- Carafoli E. Intracellular calcium homeostasis. Annu Rev Biochem. 1987;56:395–433. doi: 10.1146/annurev.bi.56.070187.002143. [DOI] [PubMed] [Google Scholar]
- Carafoli E., Lehninger A. L. A survey of the interaction of calcium ions with mitochondria from different tissues and species. Biochem J. 1971 May;122(5):681–690. doi: 10.1042/bj1220681. [DOI] [PMC free article] [PubMed] [Google Scholar]
- Carafoli E. The calcium cycle of mitochondria. FEBS Lett. 1979 Aug 1;104(1):1–5. doi: 10.1016/0014-5793(79)81073-x. [DOI] [PubMed] [Google Scholar]
- Cervetto L., Lagnado L., Perry R. J., Robinson D. W., McNaughton P. A. Extrusion of calcium from rod outer segments is driven by both sodium and potassium gradients. Nature. 1989 Feb 23;337(6209):740–743. doi: 10.1038/337740a0. [DOI] [PubMed] [Google Scholar]
- Cox D. W., Bachelard H. S. Attenuation of evoked field potentials from dentate granule cells by low glucose, pyruvate + malate, and sodium fluoride. Brain Res. 1982 May 13;239(2):527–534. doi: 10.1016/0006-8993(82)90527-3. [DOI] [PubMed] [Google Scholar]
- Cox D. W., Morris P. G., Feeney J., Bachelard H. S. 31P-n.m.r. studies on cerebral energy metabolism under conditions of hypoglycaemia and hypoxia in vitro. Biochem J. 1983 May 15;212(2):365–370. doi: 10.1042/bj2120365. [DOI] [PMC free article] [PubMed] [Google Scholar]
- Crompton M., Moser R., Lüdi H., Carafoli E. The interrelations between the transport of sodium and calcium in mitochondria of various mammalian tissues. Eur J Biochem. 1978 Jan 2;82(1):25–31. doi: 10.1111/j.1432-1033.1978.tb11993.x. [DOI] [PubMed] [Google Scholar]
- Deshpande J. K., Siesjö B. K., Wieloch T. Calcium accumulation and neuronal damage in the rat hippocampus following cerebral ischemia. J Cereb Blood Flow Metab. 1987 Feb;7(1):89–95. doi: 10.1038/jcbfm.1987.13. [DOI] [PubMed] [Google Scholar]
- Duchen M. R. Effects of metabolic inhibition on the membrane properties of isolated mouse primary sensory neurones. J Physiol. 1990 May;424:387–409. doi: 10.1113/jphysiol.1990.sp018073. [DOI] [PMC free article] [PubMed] [Google Scholar]
- Eisner D. A., Nichols C. G., O'Neill S. C., Smith G. L., Valdeolmillos M. The effects of metabolic inhibition on intracellular calcium and pH in isolated rat ventricular cells. J Physiol. 1989 Apr;411:393–418. doi: 10.1113/jphysiol.1989.sp017580. [DOI] [PMC free article] [PubMed] [Google Scholar]
- Fujiwara N., Higashi H., Shimoji K., Yoshimura M. Effects of hypoxia on rat hippocampal neurones in vitro. J Physiol. 1987 Mar;384:131–151. doi: 10.1113/jphysiol.1987.sp016447. [DOI] [PMC free article] [PubMed] [Google Scholar]
- Gill D. L., Chueh S. H. An intracellular (ATP + Mg2+)-dependent calcium pump within the N1E-115 neuronal cell line. J Biol Chem. 1985 Aug 5;260(16):9289–9297. [PubMed] [Google Scholar]
- Gill D. L., Chueh S. H., Whitlow C. L. Functional importance of the synaptic plasma membrane calcium pump and sodium-calcium exchanger. J Biol Chem. 1984 Sep 10;259(17):10807–10813. [PubMed] [Google Scholar]
- Godfraind J. M., Kawamura H., Krnjević K., Pumain R. Actions of dinitrophenol and some other metabolic inhibitors on cortical neurones. J Physiol. 1971 May;215(1):199–222. doi: 10.1113/jphysiol.1971.sp009465. [DOI] [PMC free article] [PubMed] [Google Scholar]
- Grynkiewicz G., Poenie M., Tsien R. Y. A new generation of Ca2+ indicators with greatly improved fluorescence properties. J Biol Chem. 1985 Mar 25;260(6):3440–3450. [PubMed] [Google Scholar]
- Hansen A. J. Effect of anoxia on ion distribution in the brain. Physiol Rev. 1985 Jan;65(1):101–148. doi: 10.1152/physrev.1985.65.1.101. [DOI] [PubMed] [Google Scholar]
- Hansen A. J., Hounsgaard J., Jahnsen H. Anoxia increases potassium conductance in hippocampal nerve cells. Acta Physiol Scand. 1982 Jul;115(3):301–310. doi: 10.1111/j.1748-1716.1982.tb07082.x. [DOI] [PubMed] [Google Scholar]
- Heinonen E., Akerman K. E., Kaila K. Depolarization of the mitochondrial membrane potential increases free cytosolic calcium in synaptosomes. Neurosci Lett. 1984 Aug 24;49(1-2):33–37. doi: 10.1016/0304-3940(84)90132-0. [DOI] [PubMed] [Google Scholar]
- Kauppinen R. A., Nicholls D. G. Synaptosomal bioenergetics. The role of glycolysis, pyruvate oxidation and responses to hypoglycaemia. Eur J Biochem. 1986 Jul 1;158(1):159–165. doi: 10.1111/j.1432-1033.1986.tb09733.x. [DOI] [PubMed] [Google Scholar]
- Kuba K. Release of calcium ions linked to the activation of potassium conductance in a caffeine-treated sympathetic neurone. J Physiol. 1980 Jan;298:251–269. doi: 10.1113/jphysiol.1980.sp013079. [DOI] [PMC free article] [PubMed] [Google Scholar]
- Lipton P., Whittingham T. S. Reduced ATP concentration as a basis for synaptic transmission failure during hypoxia in the in vitro guinea-pig hippocampus. J Physiol. 1982 Apr;325:51–65. doi: 10.1113/jphysiol.1982.sp014135. [DOI] [PMC free article] [PubMed] [Google Scholar]
- MacDermott A. B., Mayer M. L., Westbrook G. L., Smith S. J., Barker J. L. NMDA-receptor activation increases cytoplasmic calcium concentration in cultured spinal cord neurones. 1986 May 29-Jun 4Nature. 321(6069):519–522. doi: 10.1038/321519a0. [DOI] [PubMed] [Google Scholar]
- Nachshen D. A. Regulation of cytosolic calcium concentration in presynaptic nerve endings isolated from rat brain. J Physiol. 1985 Jun;363:87–101. doi: 10.1113/jphysiol.1985.sp015697. [DOI] [PMC free article] [PubMed] [Google Scholar]
- Neering I. R., McBurney R. N. Role for microsomal Ca storage in mammalian neurones? Nature. 1984 May 10;309(5964):158–160. doi: 10.1038/309158a0. [DOI] [PubMed] [Google Scholar]
- Nicholls D. G., Akerman K. E. Biochemical approaches to the study of cytosolic calcium regulation in nerve endings. Philos Trans R Soc Lond B Biol Sci. 1981 Dec 18;296(1080):115–122. doi: 10.1098/rstb.1981.0176. [DOI] [PubMed] [Google Scholar]
- Noma A. ATP-regulated K+ channels in cardiac muscle. Nature. 1983 Sep 8;305(5930):147–148. doi: 10.1038/305147a0. [DOI] [PubMed] [Google Scholar]
- Rasgado-Flores H., Blaustein M. P. ATP-dependent regulation of cytoplasmic free calcium in nerve terminals. Am J Physiol. 1987 Jun;252(6 Pt 1):C588–C594. doi: 10.1152/ajpcell.1987.252.6.C588. [DOI] [PubMed] [Google Scholar]
- Reuter H., Seitz N. The dependence of calcium efflux from cardiac muscle on temperature and external ion composition. J Physiol. 1968 Mar;195(2):451–470. doi: 10.1113/jphysiol.1968.sp008467. [DOI] [PMC free article] [PubMed] [Google Scholar]
- Rink T. J., Tsien R. Y., Pozzan T. Cytoplasmic pH and free Mg2+ in lymphocytes. J Cell Biol. 1982 Oct;95(1):189–196. doi: 10.1083/jcb.95.1.189. [DOI] [PMC free article] [PubMed] [Google Scholar]
- Rottenberg H., Scarpa A. Calcium uptake and membrane potential in mitochondria. Biochemistry. 1974 Nov 5;13(23):4811–4817. doi: 10.1021/bi00720a020. [DOI] [PubMed] [Google Scholar]
- Schanne F. A., Kane A. B., Young E. E., Farber J. L. Calcium dependence of toxic cell death: a final common pathway. Science. 1979 Nov 9;206(4419):700–702. doi: 10.1126/science.386513. [DOI] [PubMed] [Google Scholar]
- Siesjö B. K. Cell damage in the brain: a speculative synthesis. J Cereb Blood Flow Metab. 1981;1(2):155–185. doi: 10.1038/jcbfm.1981.18. [DOI] [PubMed] [Google Scholar]
- VAN HARREVELD A. Compounds in brain extracts causing spreading depression of cerebral cortical activity and contraction of crustacean muscle. J Neurochem. 1959 Feb;3(4):300–315. doi: 10.1111/j.1471-4159.1959.tb12636.x. [DOI] [PubMed] [Google Scholar]
- Weber A., Herz R. The relationship between caffeine contracture of intact muscle and the effect of caffeine on reticulum. J Gen Physiol. 1968 Nov;52(5):750–759. doi: 10.1085/jgp.52.5.750. [DOI] [PMC free article] [PubMed] [Google Scholar]