Abstract
1. The blocking actions of Zn2+ on currents carried by Ba2+ through single dihydropyridine-sensitive Ca2+ channels were recorded from cell-attached patches on myotubes from the mouse C2 cell line. 2. Adding 100 microM-Zn2+ to the patch electrode containing 110 mM-BaCl2 produced an increase in the open channel noise, presumably arising from unresolved blocking and unblocking of the open channel by Zn2+. Adding between 200 and 1000 microM-Zn2+ to the electrode reduced the amplitude of the unitary current in a concentration-dependent manner. 3. The single-channel current-voltage (i-V) relations showed that Zn2+ reduced the amplitude of the unitary Ba2+ currents at all potentials more negative than 0 mV. A plot of the amplitude of the unitary current in the presence of Zn2+, normalized to the amplitude in its absence, showed that block of the current depended on voltage, decreasing as the patch potential was made more negative. 4. The normalized amplitudes of the unitary currents were plotted as a function of the logarithm of [Zn2+] in the electrode. The relation for currents recorded at different potentials were fitted to an expression for binding to a single site with a KD at 0 mV of approximately 500 microM. The KD changed approximately e-fold per 83 mV with hyperpolarization. The results suggest Zn2+ binds to a site located at approximately 15% of the potential drop from the surface membrane. 5. Reducing the concentration of Ba2+ in the patch electrode enhanced the steady-state block of unitary currents by Zn2+. The inverse of the unitary current was plotted as a function of [Ba2+]o in the presence and absence of Zn2+; both were linear and intersected at the ordinate, indicating Ba2+ and Zn2+ compete for a channel site. 6. The kinetics of Zn2+ block of unitary Ba2+ currents were studied by amplitude distribution analysis. As expected for a simple reaction between blocking ion and open channel, the blocking rate depended linearly on the concentration of Zn2+, while the exit rate was independent of concentration. The second-order rate coefficient for Zn2+ entry in the presence of 110 mM-BaCl2 at 0 mV was approximately 2.0 X 10(7) M-1S-1, while the exit rate was approximately 16000 s-1. 7. Both entry and exit rates increased as the membrane potential was made more negative. The entry rate increased approximately e-fold per 66 mV, while the exit rate increased approximately e-fold per 41 mV.(ABSTRACT TRUNCATED AT 400 WORDS)
Full text
PDF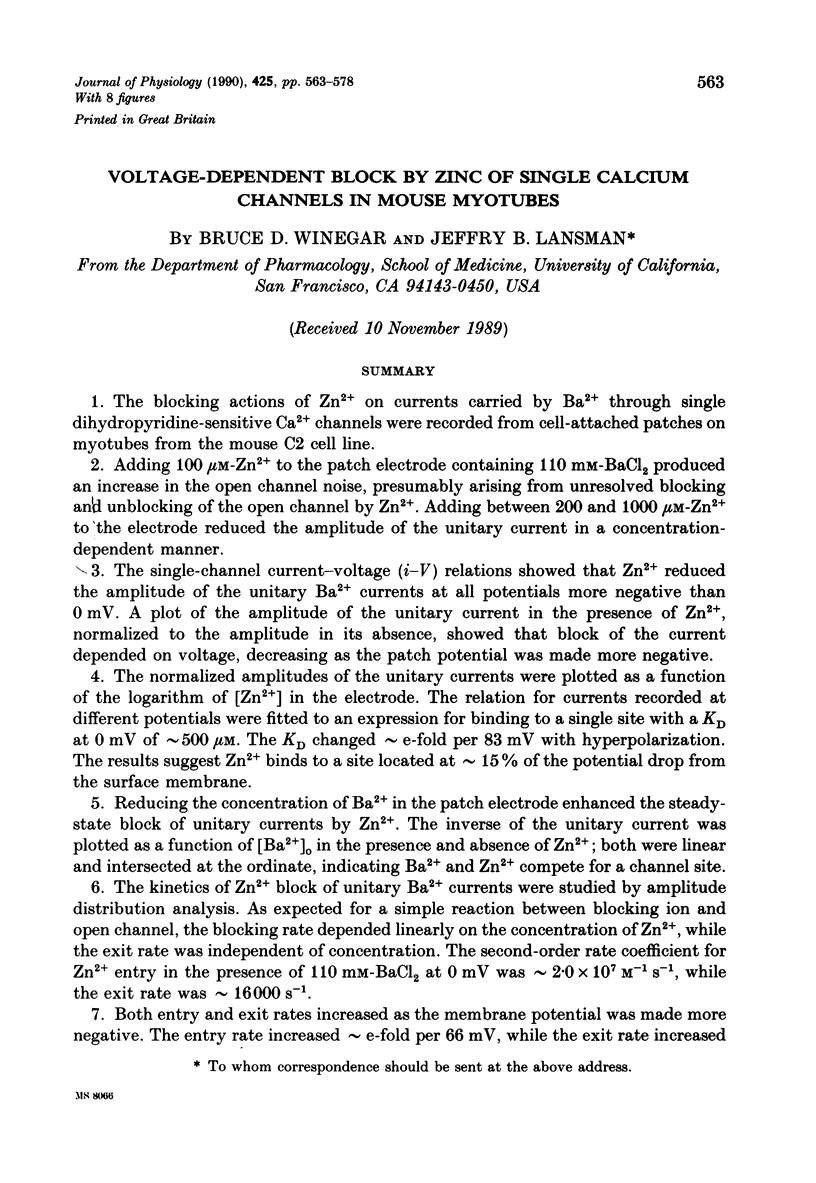
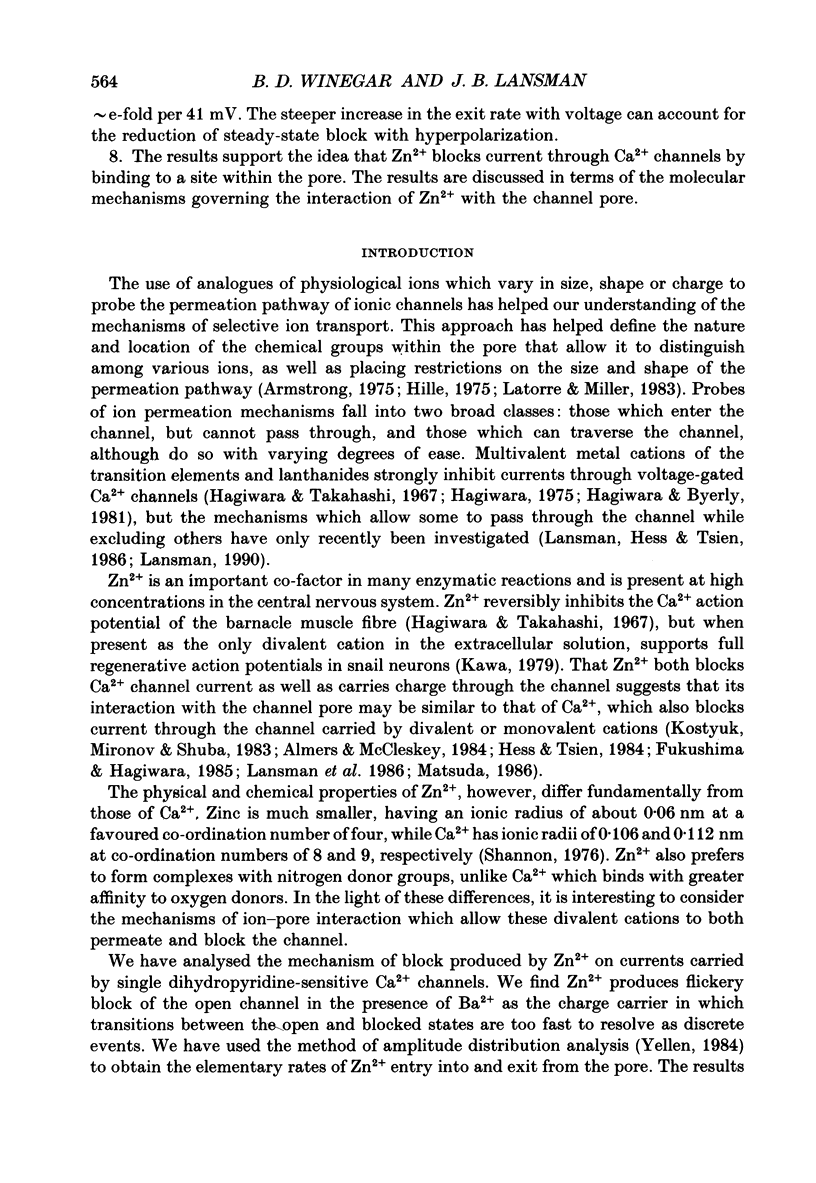
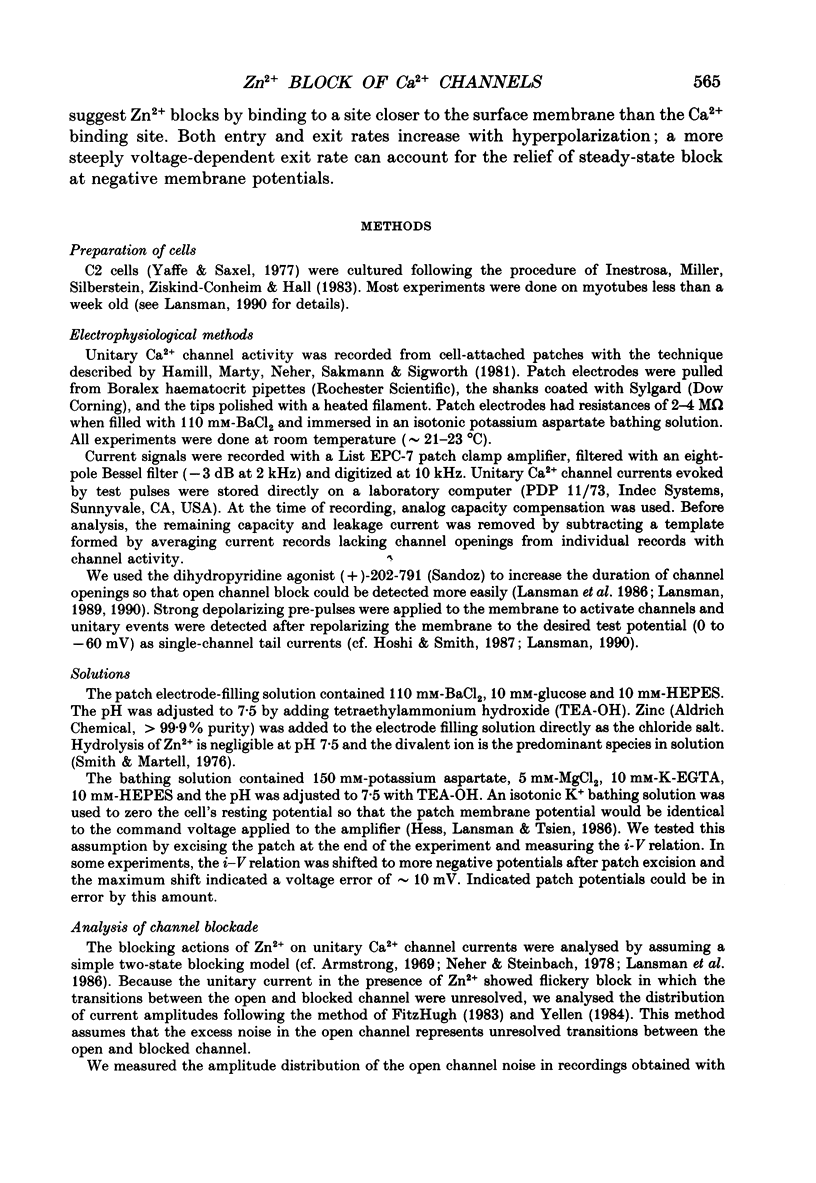
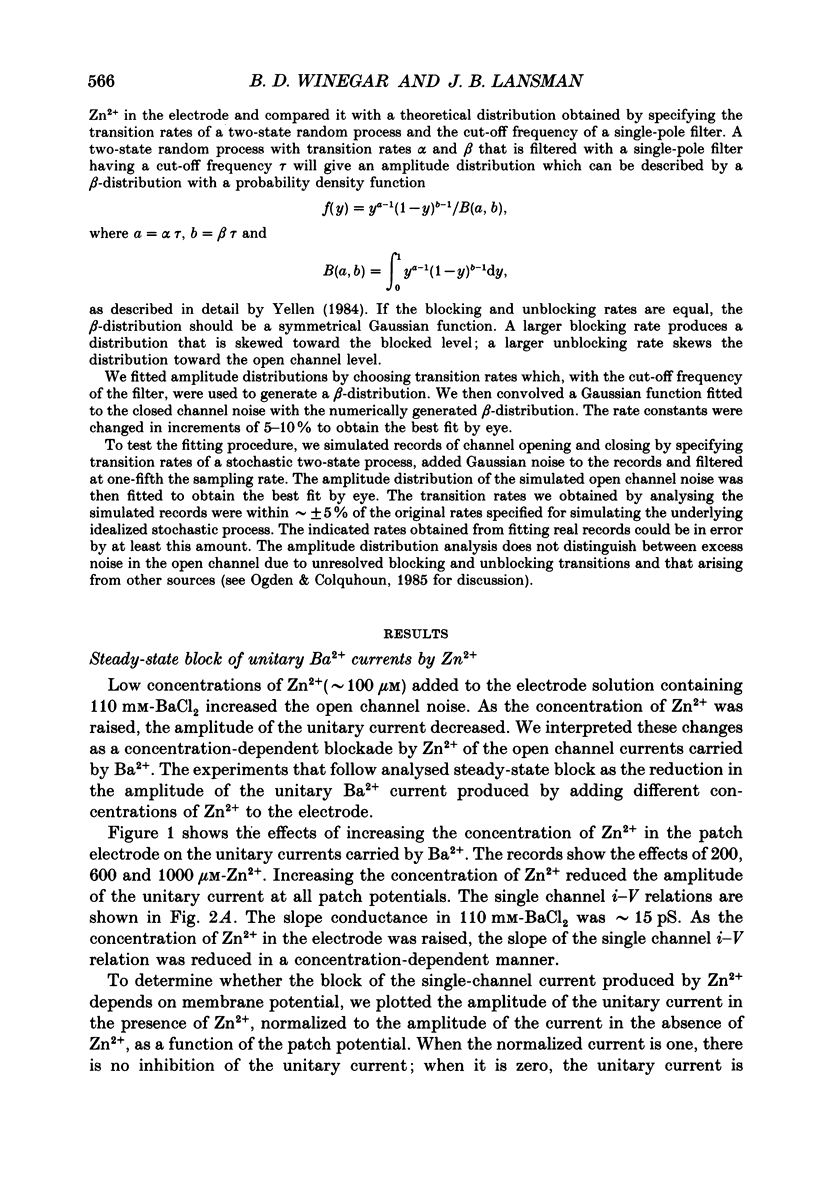
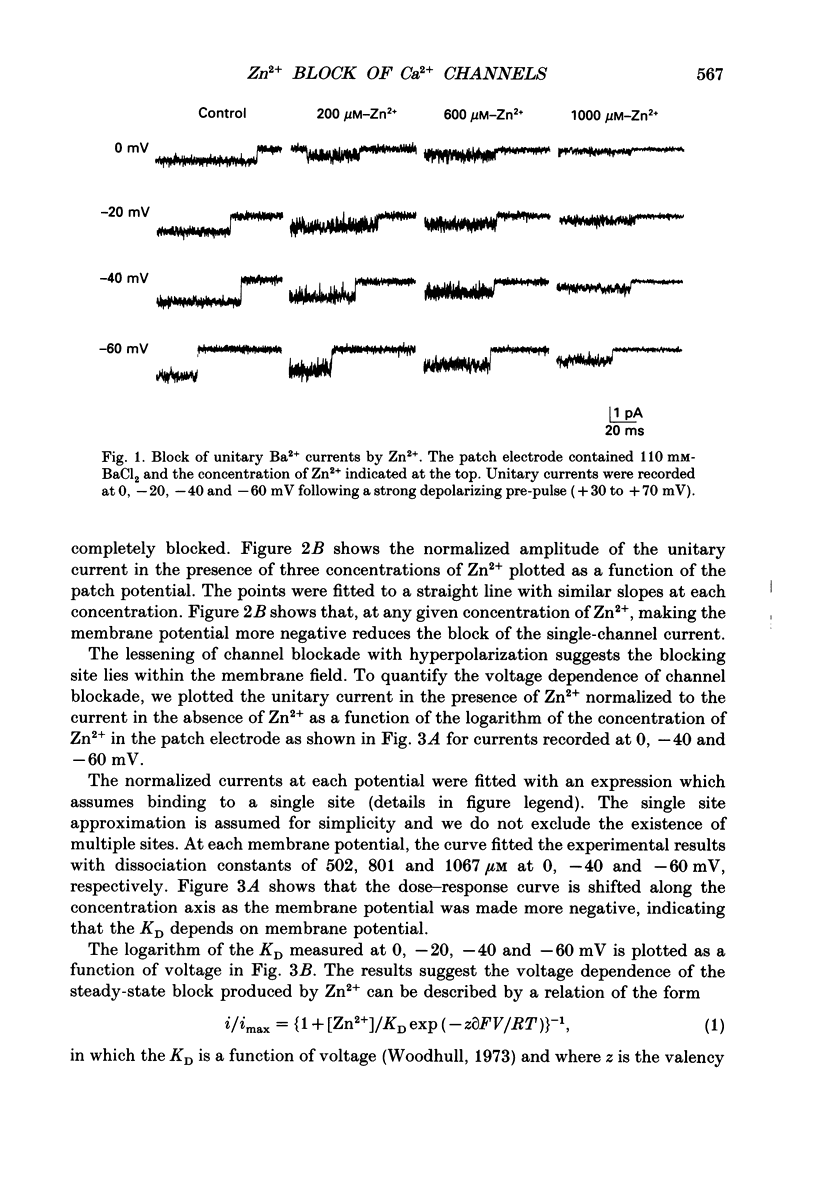
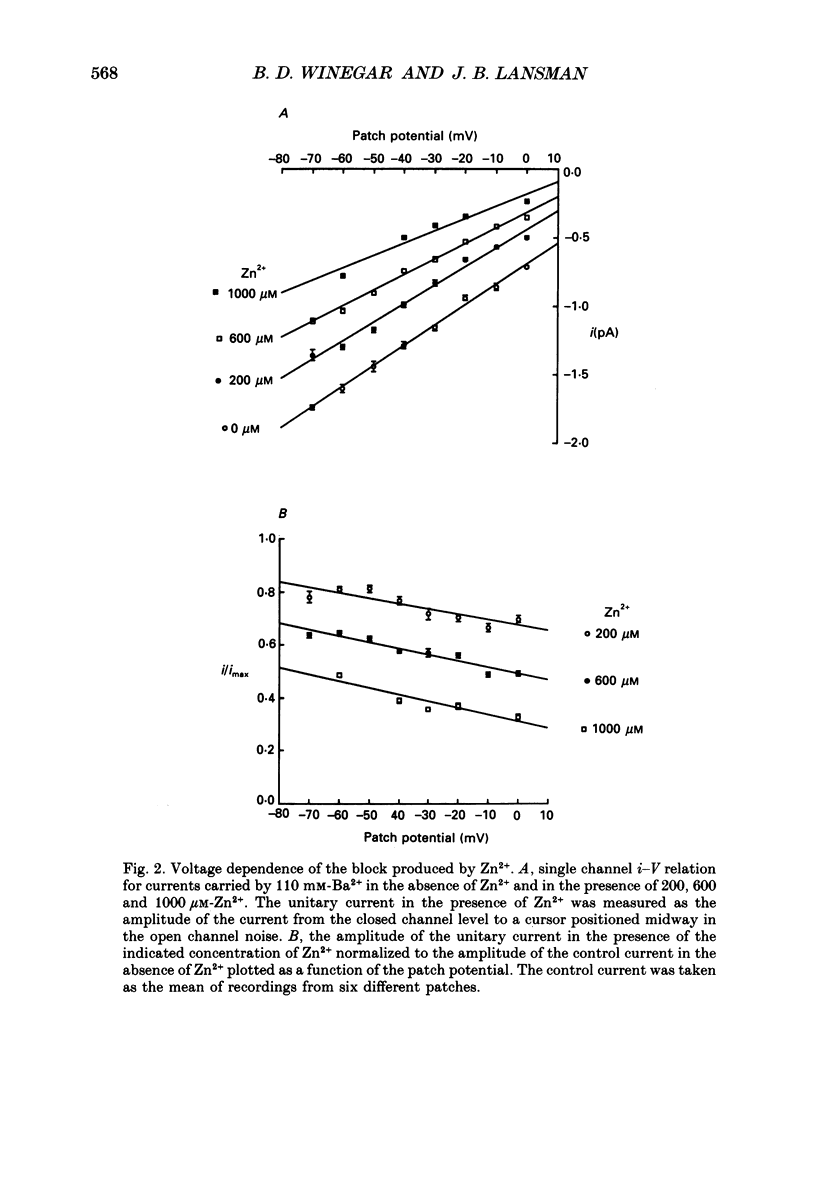
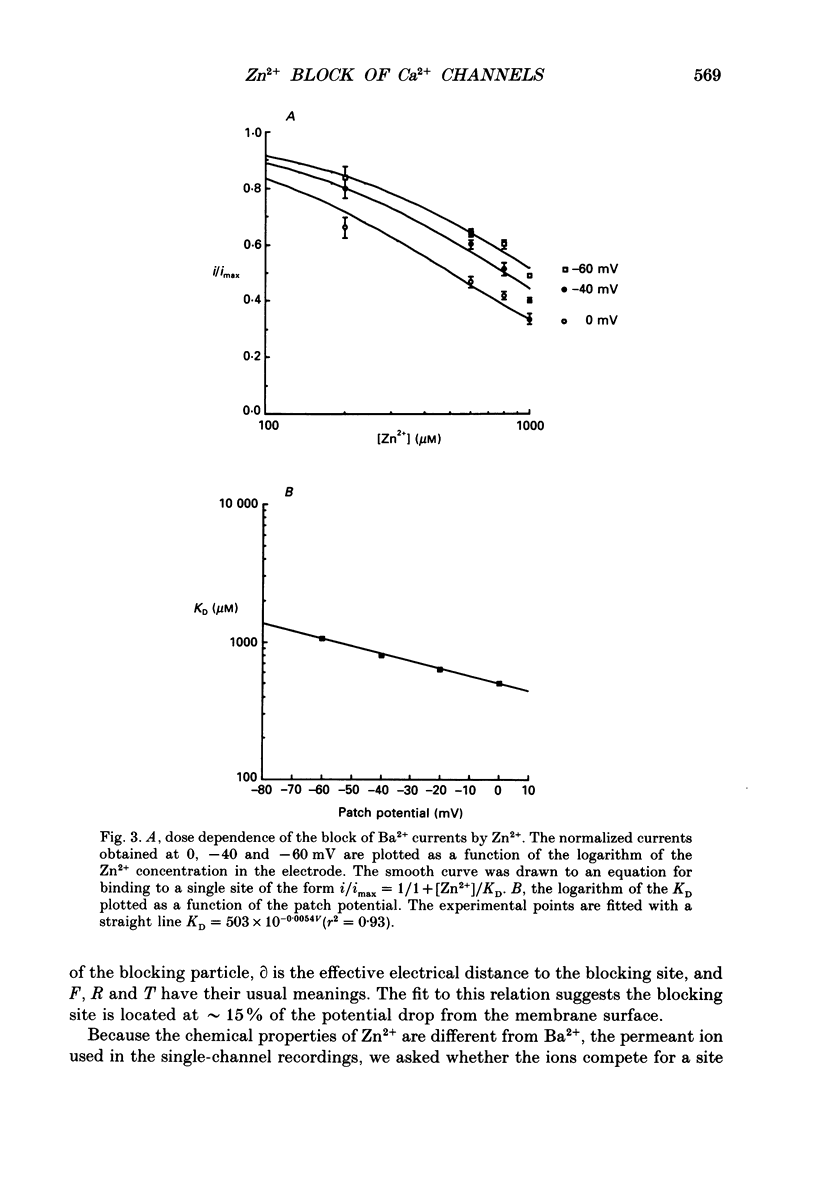
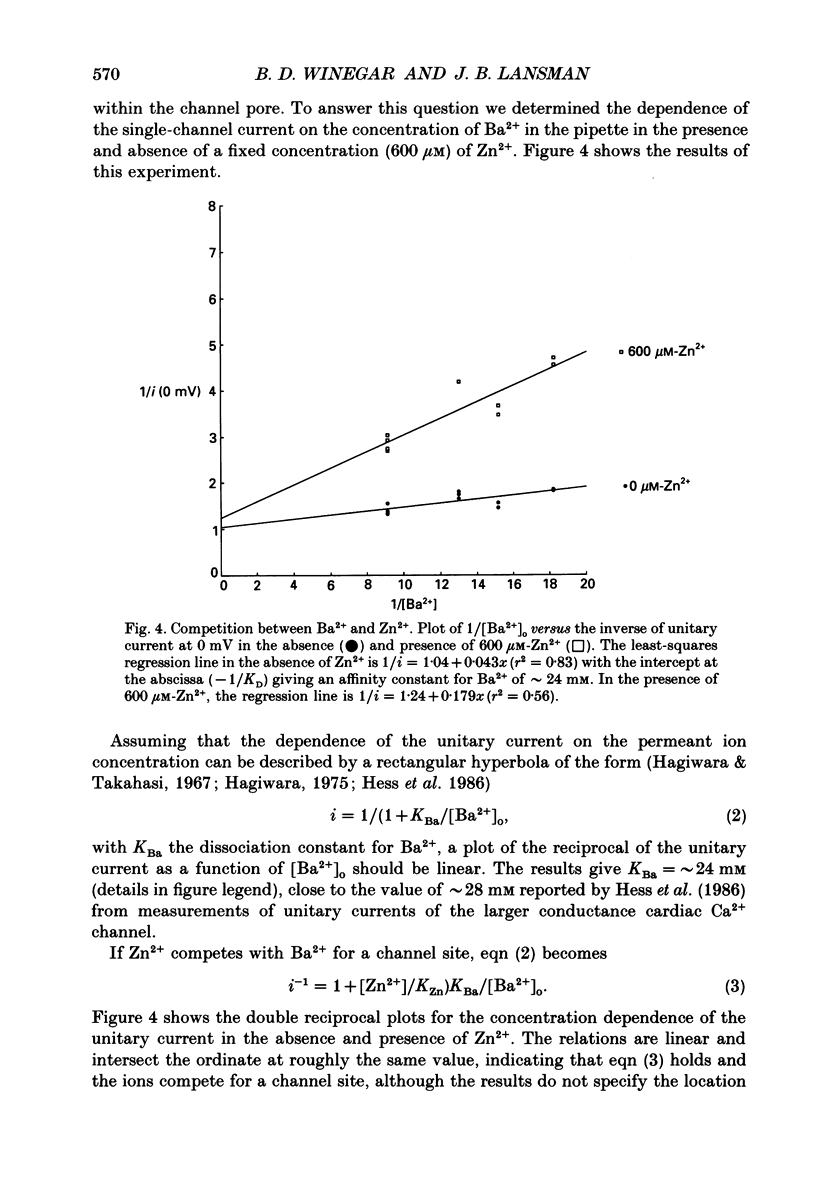
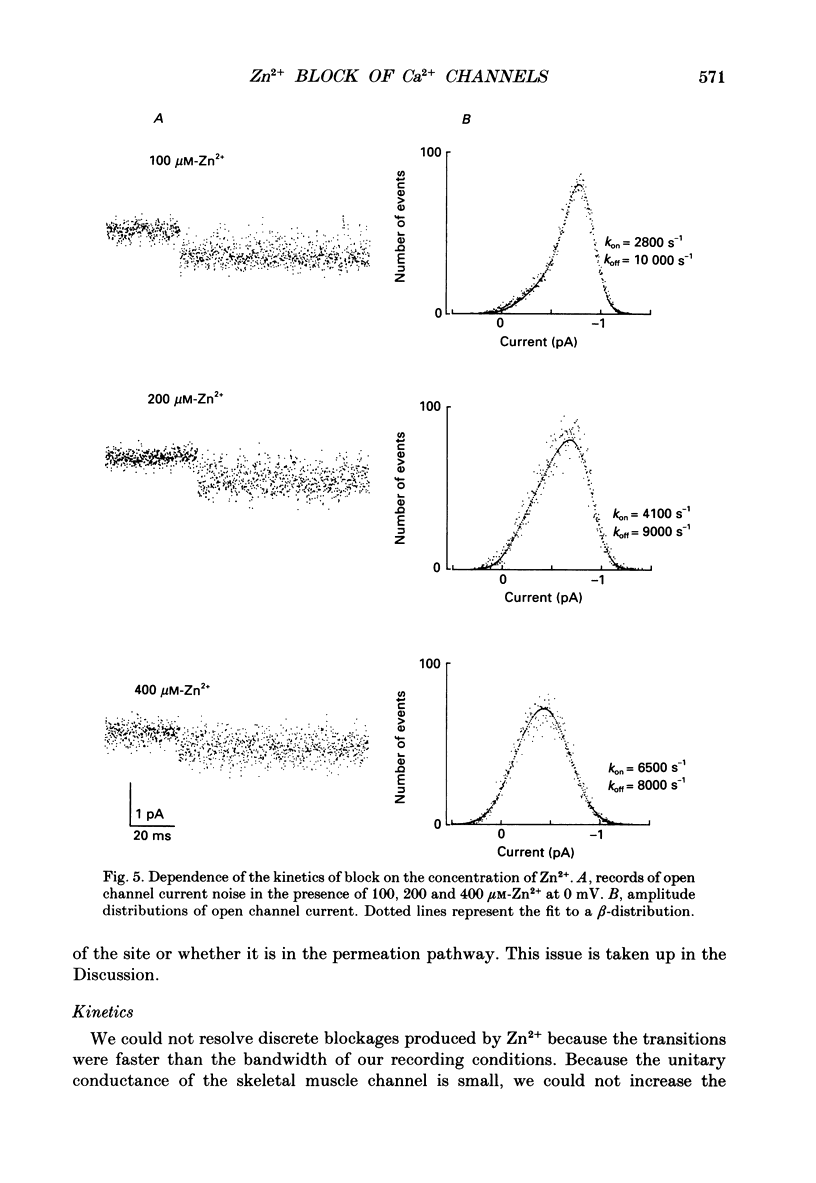
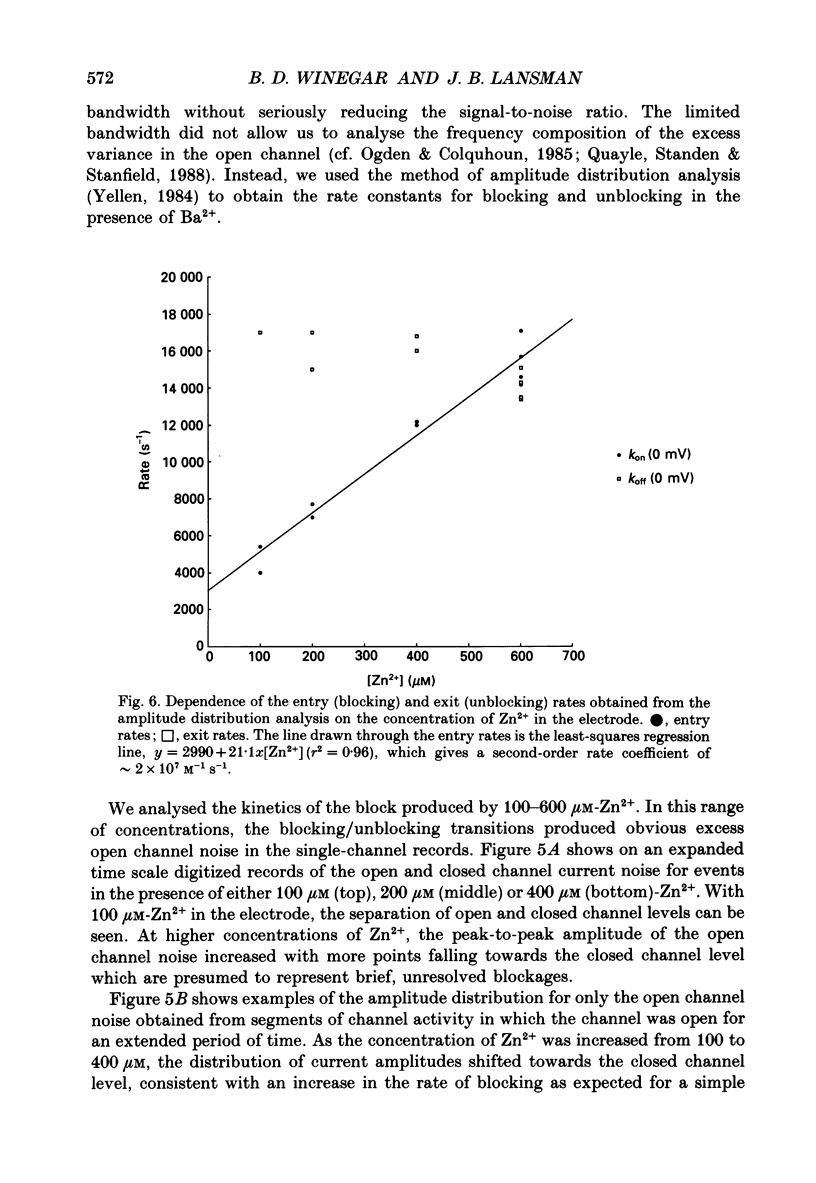
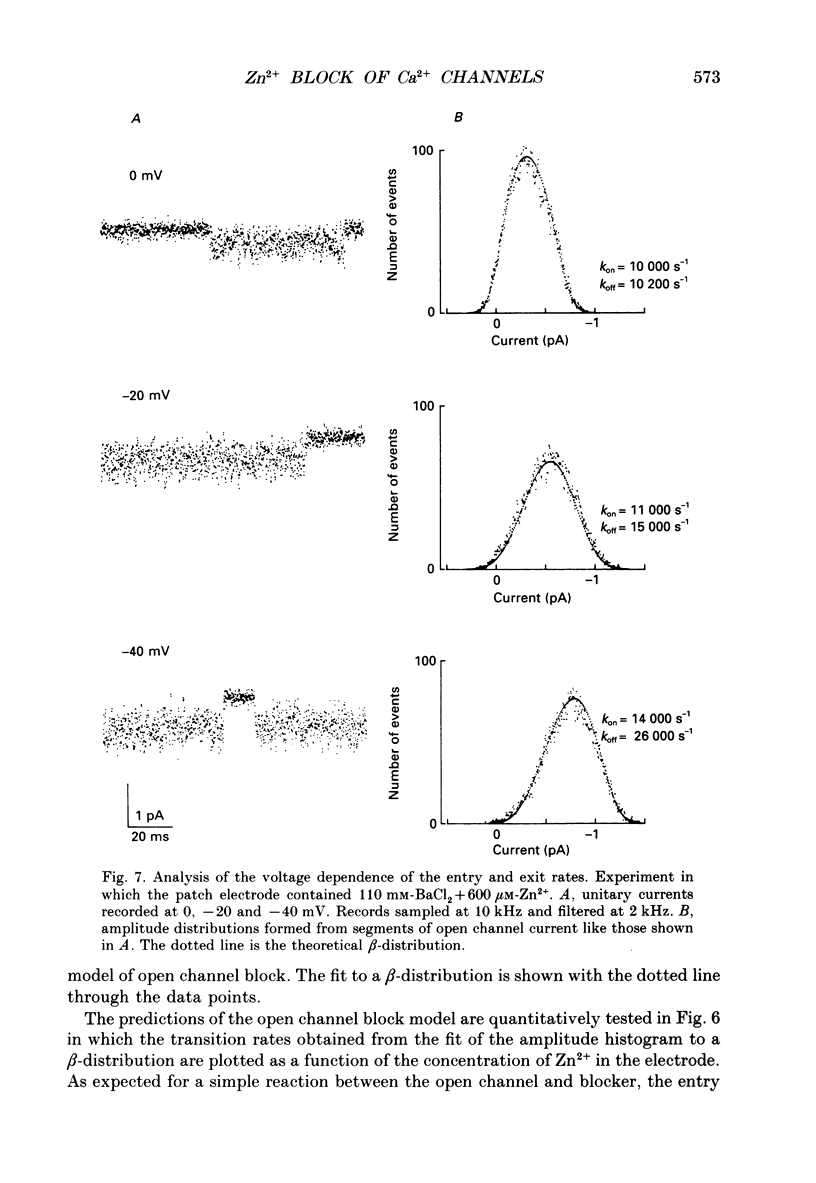
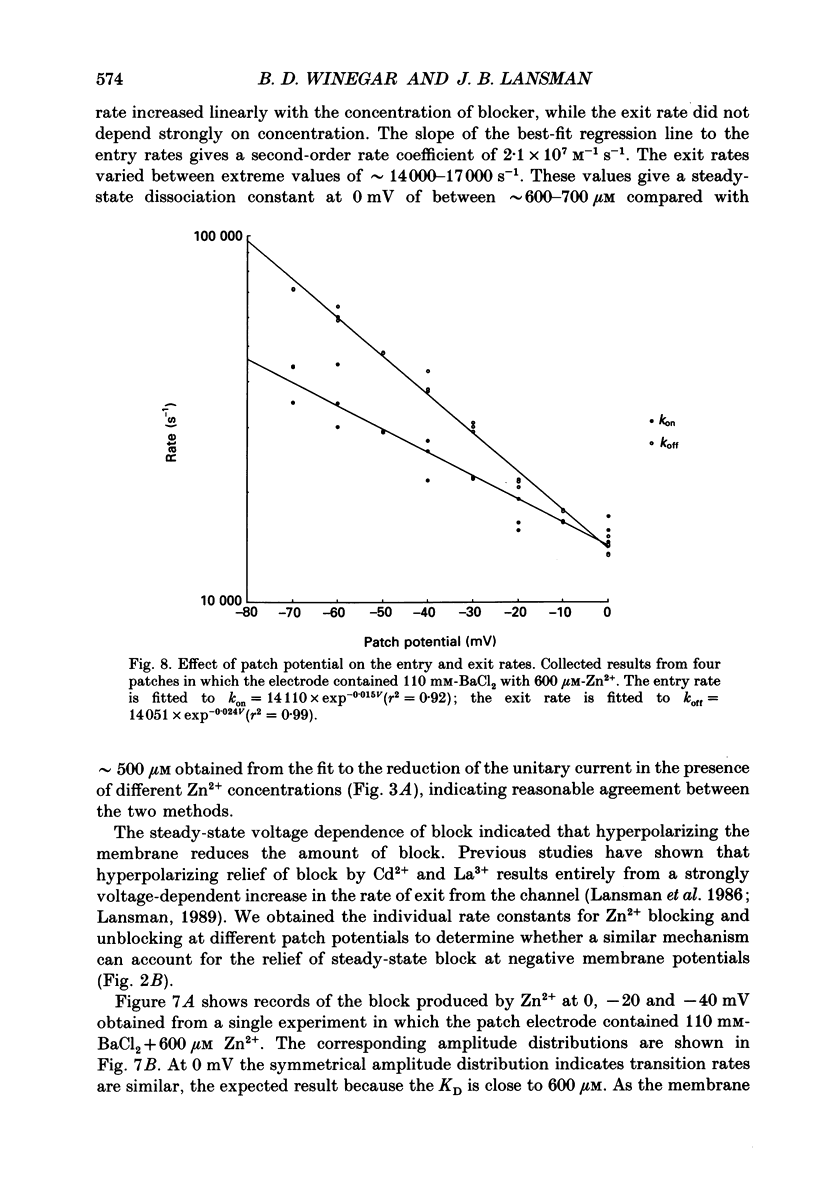
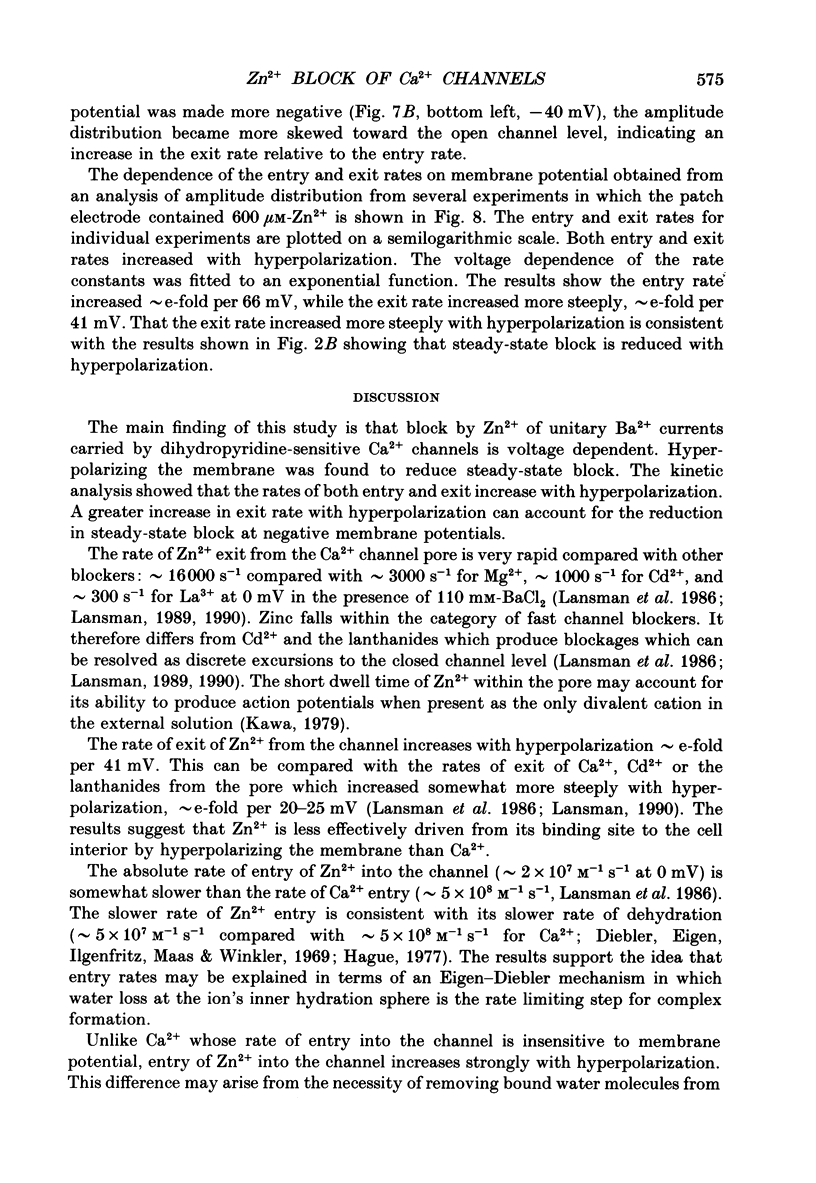
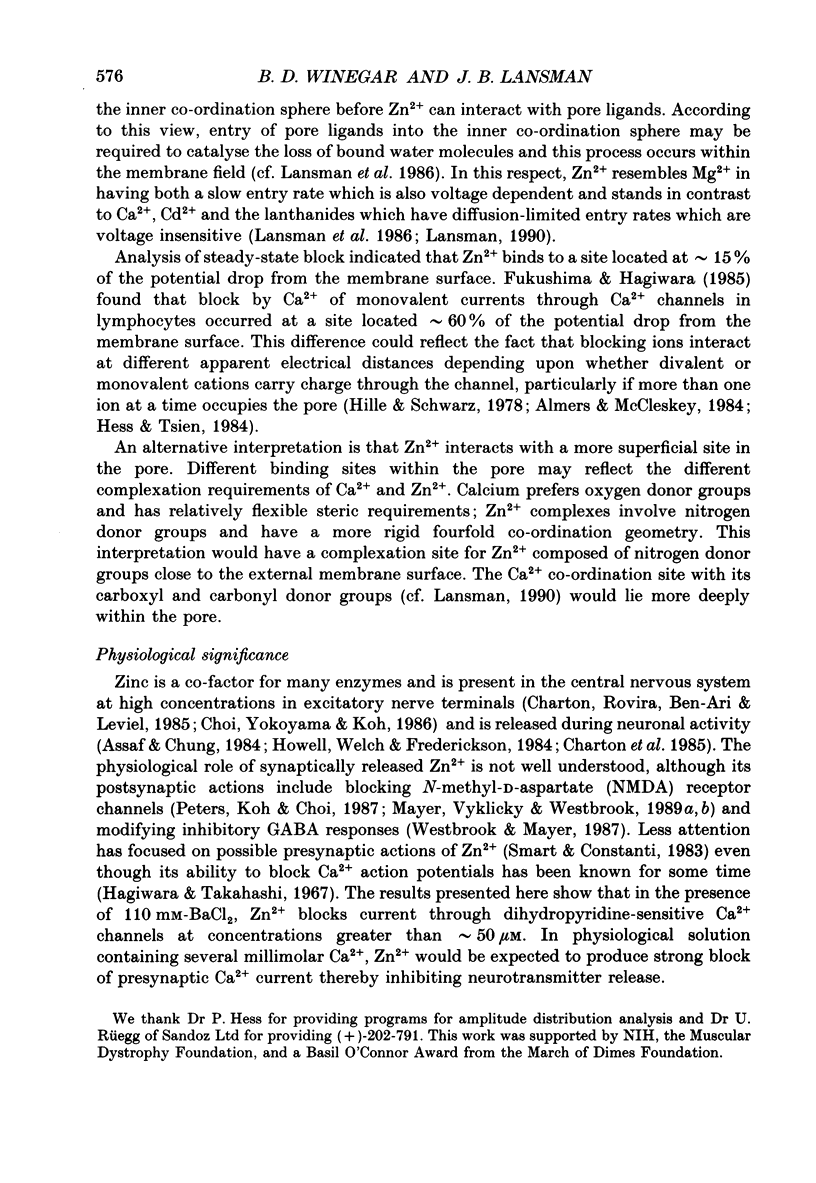
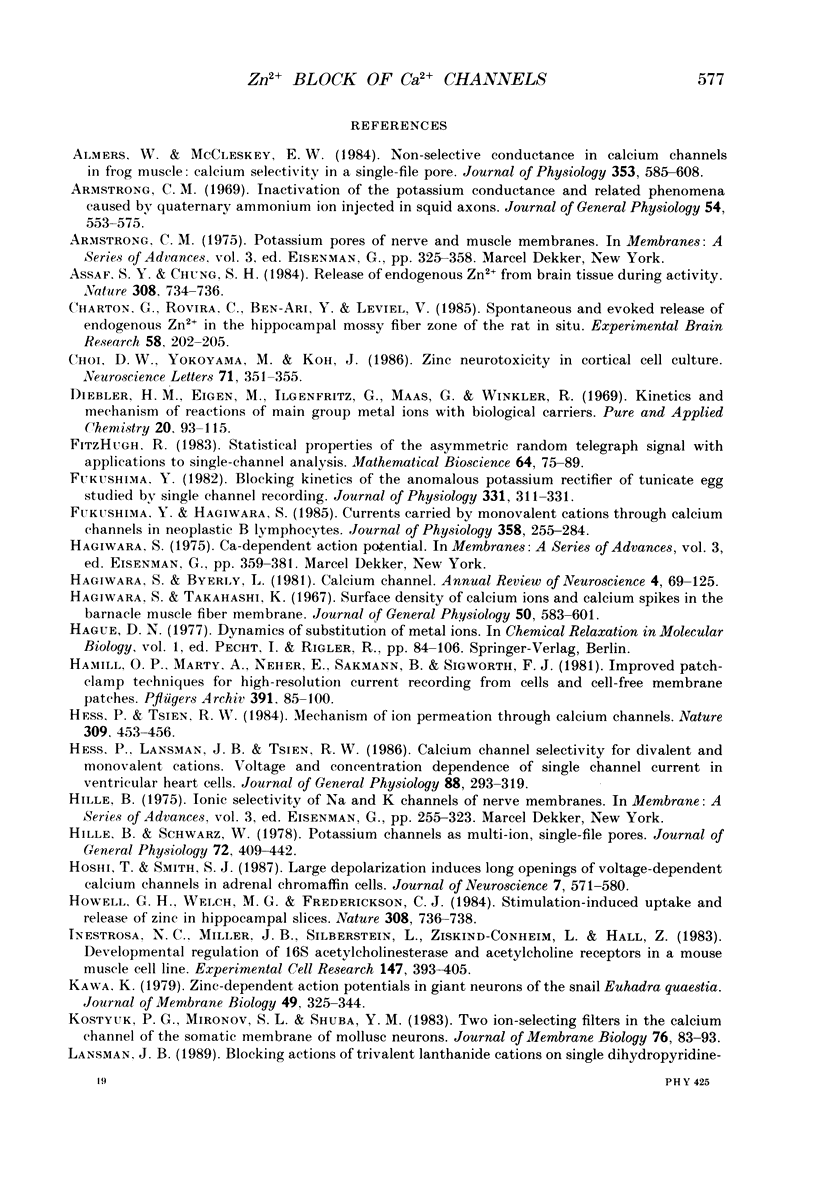
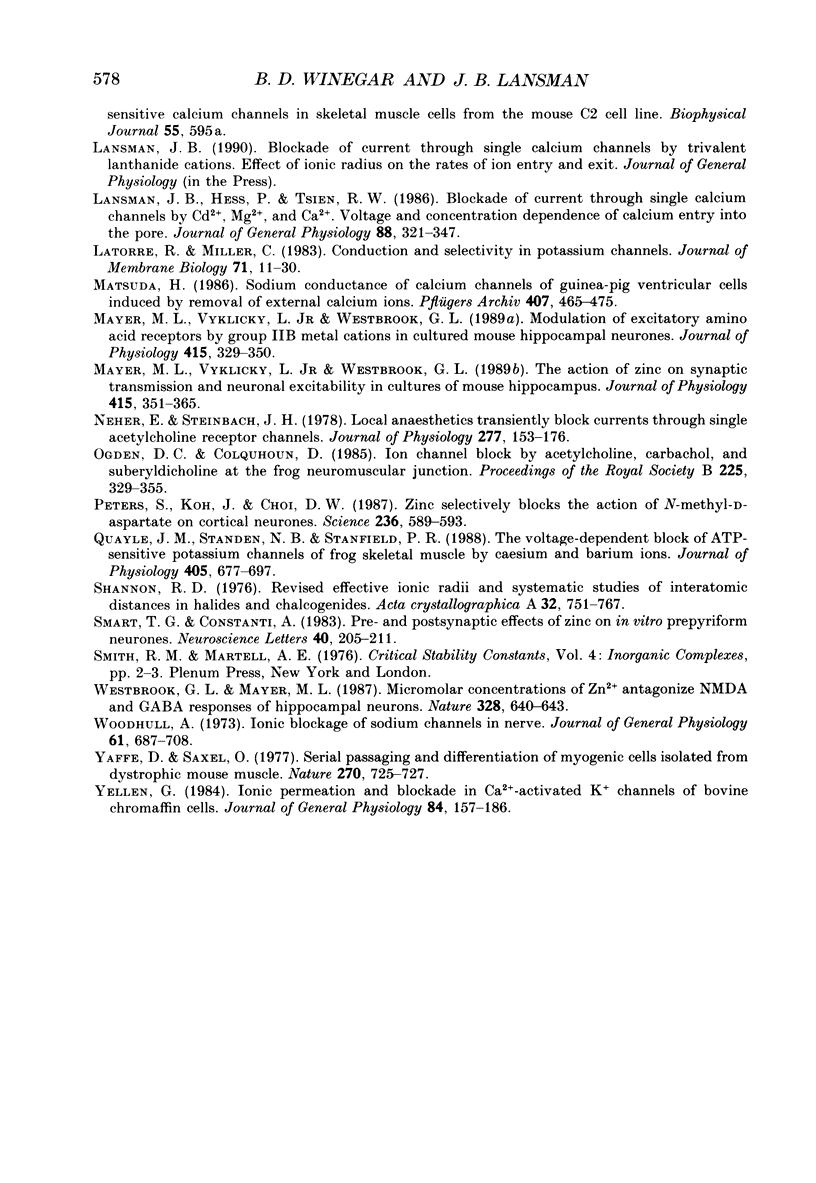
Selected References
These references are in PubMed. This may not be the complete list of references from this article.
- Almers W., McCleskey E. W. Non-selective conductance in calcium channels of frog muscle: calcium selectivity in a single-file pore. J Physiol. 1984 Aug;353:585–608. doi: 10.1113/jphysiol.1984.sp015352. [DOI] [PMC free article] [PubMed] [Google Scholar]
- Armstrong C. M. Inactivation of the potassium conductance and related phenomena caused by quaternary ammonium ion injection in squid axons. J Gen Physiol. 1969 Nov;54(5):553–575. doi: 10.1085/jgp.54.5.553. [DOI] [PMC free article] [PubMed] [Google Scholar]
- Armstrong C. M. Potassium pores of nerve and muscle membranes. Membranes. 1975;3:325–358. [PubMed] [Google Scholar]
- Assaf S. Y., Chung S. H. Release of endogenous Zn2+ from brain tissue during activity. Nature. 1984 Apr 19;308(5961):734–736. doi: 10.1038/308734a0. [DOI] [PubMed] [Google Scholar]
- Charton G., Rovira C., Ben-Ari Y., Leviel V. Spontaneous and evoked release of endogenous Zn2+ in the hippocampal mossy fiber zone of the rat in situ. Exp Brain Res. 1985;58(1):202–205. doi: 10.1007/BF00238969. [DOI] [PubMed] [Google Scholar]
- Fukushima Y. Blocking kinetics of the anomalous potassium rectifier of tunicate egg studied by single channel recording. J Physiol. 1982 Oct;331:311–331. doi: 10.1113/jphysiol.1982.sp014374. [DOI] [PMC free article] [PubMed] [Google Scholar]
- Fukushima Y., Hagiwara S. Currents carried by monovalent cations through calcium channels in mouse neoplastic B lymphocytes. J Physiol. 1985 Jan;358:255–284. doi: 10.1113/jphysiol.1985.sp015550. [DOI] [PMC free article] [PubMed] [Google Scholar]
- Hagiwara S., Byerly L. Calcium channel. Annu Rev Neurosci. 1981;4:69–125. doi: 10.1146/annurev.ne.04.030181.000441. [DOI] [PubMed] [Google Scholar]
- Hagiwara S. Ca-dependent action potential. Membranes. 1975;3:359–381. [PubMed] [Google Scholar]
- Hagiwara S., Takahashi K. Surface density of calcium ions and calcium spikes in the barnacle muscle fiber membrane. J Gen Physiol. 1967 Jan;50(3):583–601. doi: 10.1085/jgp.50.3.583. [DOI] [PMC free article] [PubMed] [Google Scholar]
- Hague D. N. Dynamics of substitution at metal ions. Mol Biol Biochem Biophys. 1977;24:84–106. doi: 10.1007/978-3-642-81117-3_3. [DOI] [PubMed] [Google Scholar]
- Hamill O. P., Marty A., Neher E., Sakmann B., Sigworth F. J. Improved patch-clamp techniques for high-resolution current recording from cells and cell-free membrane patches. Pflugers Arch. 1981 Aug;391(2):85–100. doi: 10.1007/BF00656997. [DOI] [PubMed] [Google Scholar]
- Hess P., Lansman J. B., Tsien R. W. Calcium channel selectivity for divalent and monovalent cations. Voltage and concentration dependence of single channel current in ventricular heart cells. J Gen Physiol. 1986 Sep;88(3):293–319. doi: 10.1085/jgp.88.3.293. [DOI] [PMC free article] [PubMed] [Google Scholar]
- Hess P., Tsien R. W. Mechanism of ion permeation through calcium channels. 1984 May 31-Jun 6Nature. 309(5967):453–456. doi: 10.1038/309453a0. [DOI] [PubMed] [Google Scholar]
- Hille B. Ionic selectivity of Na and K channels of nerve membranes. Membranes. 1975;3:255–323. [PubMed] [Google Scholar]
- Hille B., Schwarz W. Potassium channels as multi-ion single-file pores. J Gen Physiol. 1978 Oct;72(4):409–442. doi: 10.1085/jgp.72.4.409. [DOI] [PMC free article] [PubMed] [Google Scholar]
- Hoshi T., Smith S. J. Large depolarization induces long openings of voltage-dependent calcium channels in adrenal chromaffin cells. J Neurosci. 1987 Feb;7(2):571–580. doi: 10.1523/JNEUROSCI.07-02-00571.1987. [DOI] [PMC free article] [PubMed] [Google Scholar]
- Howell G. A., Welch M. G., Frederickson C. J. Stimulation-induced uptake and release of zinc in hippocampal slices. Nature. 1984 Apr 19;308(5961):736–738. doi: 10.1038/308736a0. [DOI] [PubMed] [Google Scholar]
- Inestrosa N. C., Miller J. B., Silberstein L., Ziskind-Conhaim L., Hall Z. W. Developmental regulation of 16S acetylcholinesterase and acetylcholine receptors in a mouse muscle cell line. Exp Cell Res. 1983 Sep;147(2):393–405. doi: 10.1016/0014-4827(83)90221-5. [DOI] [PubMed] [Google Scholar]
- Kawa K. Zinc-dependent action potentials in giant neurons of the snail, Euhadra quaestia. J Membr Biol. 1979 Sep 14;49(4):325–344. doi: 10.1007/BF01868990. [DOI] [PubMed] [Google Scholar]
- Lansman J. B., Hess P., Tsien R. W. Blockade of current through single calcium channels by Cd2+, Mg2+, and Ca2+. Voltage and concentration dependence of calcium entry into the pore. J Gen Physiol. 1986 Sep;88(3):321–347. doi: 10.1085/jgp.88.3.321. [DOI] [PMC free article] [PubMed] [Google Scholar]
- Latorre R., Miller C. Conduction and selectivity in potassium channels. J Membr Biol. 1983;71(1-2):11–30. doi: 10.1007/BF01870671. [DOI] [PubMed] [Google Scholar]
- Matsuda H. Sodium conductance in calcium channels of guinea-pig ventricular cells induced by removal of external calcium ions. Pflugers Arch. 1986 Nov;407(5):465–475. doi: 10.1007/BF00657502. [DOI] [PubMed] [Google Scholar]
- Mayer M. L., Vyklicky L., Jr The action of zinc on synaptic transmission and neuronal excitability in cultures of mouse hippocampus. J Physiol. 1989 Aug;415:351–365. doi: 10.1113/jphysiol.1989.sp017725. [DOI] [PMC free article] [PubMed] [Google Scholar]
- Mayer M. L., Vyklicky L., Jr, Westbrook G. L. Modulation of excitatory amino acid receptors by group IIB metal cations in cultured mouse hippocampal neurones. J Physiol. 1989 Aug;415:329–350. doi: 10.1113/jphysiol.1989.sp017724. [DOI] [PMC free article] [PubMed] [Google Scholar]
- Neher E., Steinbach J. H. Local anaesthetics transiently block currents through single acetylcholine-receptor channels. J Physiol. 1978 Apr;277:153–176. doi: 10.1113/jphysiol.1978.sp012267. [DOI] [PMC free article] [PubMed] [Google Scholar]
- Ogden D. C., Colquhoun D. Ion channel block by acetylcholine, carbachol and suberyldicholine at the frog neuromuscular junction. Proc R Soc Lond B Biol Sci. 1985 Sep 23;225(1240):329–355. doi: 10.1098/rspb.1985.0065. [DOI] [PubMed] [Google Scholar]
- Peters S., Koh J., Choi D. W. Zinc selectively blocks the action of N-methyl-D-aspartate on cortical neurons. Science. 1987 May 1;236(4801):589–593. doi: 10.1126/science.2883728. [DOI] [PubMed] [Google Scholar]
- Quayle J. M., Standen N. B., Stanfield P. R. The voltage-dependent block of ATP-sensitive potassium channels of frog skeletal muscle by caesium and barium ions. J Physiol. 1988 Nov;405:677–697. doi: 10.1113/jphysiol.1988.sp017355. [DOI] [PMC free article] [PubMed] [Google Scholar]
- Smart T. G., Constanti A. Pre- and postsynaptic effects of zinc on in vitro prepyriform neurones. Neurosci Lett. 1983 Sep 30;40(2):205–211. doi: 10.1016/0304-3940(83)90303-8. [DOI] [PubMed] [Google Scholar]
- Westbrook G. L., Mayer M. L. Micromolar concentrations of Zn2+ antagonize NMDA and GABA responses of hippocampal neurons. Nature. 1987 Aug 13;328(6131):640–643. doi: 10.1038/328640a0. [DOI] [PubMed] [Google Scholar]
- Woodhull A. M. Ionic blockage of sodium channels in nerve. J Gen Physiol. 1973 Jun;61(6):687–708. doi: 10.1085/jgp.61.6.687. [DOI] [PMC free article] [PubMed] [Google Scholar]
- Yaffe D., Saxel O. Serial passaging and differentiation of myogenic cells isolated from dystrophic mouse muscle. Nature. 1977 Dec 22;270(5639):725–727. doi: 10.1038/270725a0. [DOI] [PubMed] [Google Scholar]
- Yellen G. Ionic permeation and blockade in Ca2+-activated K+ channels of bovine chromaffin cells. J Gen Physiol. 1984 Aug;84(2):157–186. doi: 10.1085/jgp.84.2.157. [DOI] [PMC free article] [PubMed] [Google Scholar]
- Yokoyama M., Koh J., Choi D. W. Brief exposure to zinc is toxic to cortical neurons. Neurosci Lett. 1986 Nov 21;71(3):351–355. doi: 10.1016/0304-3940(86)90646-4. [DOI] [PubMed] [Google Scholar]