Abstract
1. Transient and persistent tetrodotoxin-sensitive sodium currents were recorded in response to depolarizing voltage pulses in voltage clamped segments of rat extensor digitorum longus muscle fibres at 20-25 degrees C in a triple Vaseline gap. 2. Appreciable persistent sodium current but little or no transient current was seen in response to depolarizations of up to 15 mV from a holding potential of -100 mV. 3. The maximum amplitude of both transient and persistent sodium currents occurred with depolarizations to -40 mV: the average peak amplitude of the transient current in fibres with a holding potential of -90 mV was -0.22 +/- 0.03 mA/microF (mean +/- 1 S.E.M., seven fibres) and the average amplitude of the persistent current was -0.94 +/- 0.10 microA/microF (mean +/- 1 S.E.M., twelve fibres). With a holding potential of -100 mV, the average amplitudes of the transient and persistent currents were -0.46 +/- 0.10 mA/microF (four fibres) and -1.4 +/- 0.22 microA/microF (five fibres), respectively. 4. The average maximum persistent sodium conductance in seven fibres held at -90 mV was 0.13 +/- 0.0078 microS and the potential for half-maximum conductance was -53 +/- 0.74 mV (mean +/- 1 S.E.M.). 5. When the transient sodium current was completely inactivated with 100 ms conditioning depolarizations to potentials more positive than -50 to -60 mV, there was little inactivation of the persistent current. 6. In six denervated fibres, the average amplitudes of the transient and persistent sodium currents generated by pulses to -40 mV from a holding potential of -90 mV were -0.11 +/- 0.01 mA/microF and -0.88 +/- 0.12 microA/microF, respectively (mean +/- 1 S.E.M.). It was concluded that there was a decrease in transient current but not persistent current amplitude following denervation and that the persistent current in denervated fibres with an increased input resistance could give rise to the spontaneous action potentials responsible for fibrillation.
Full text
PDF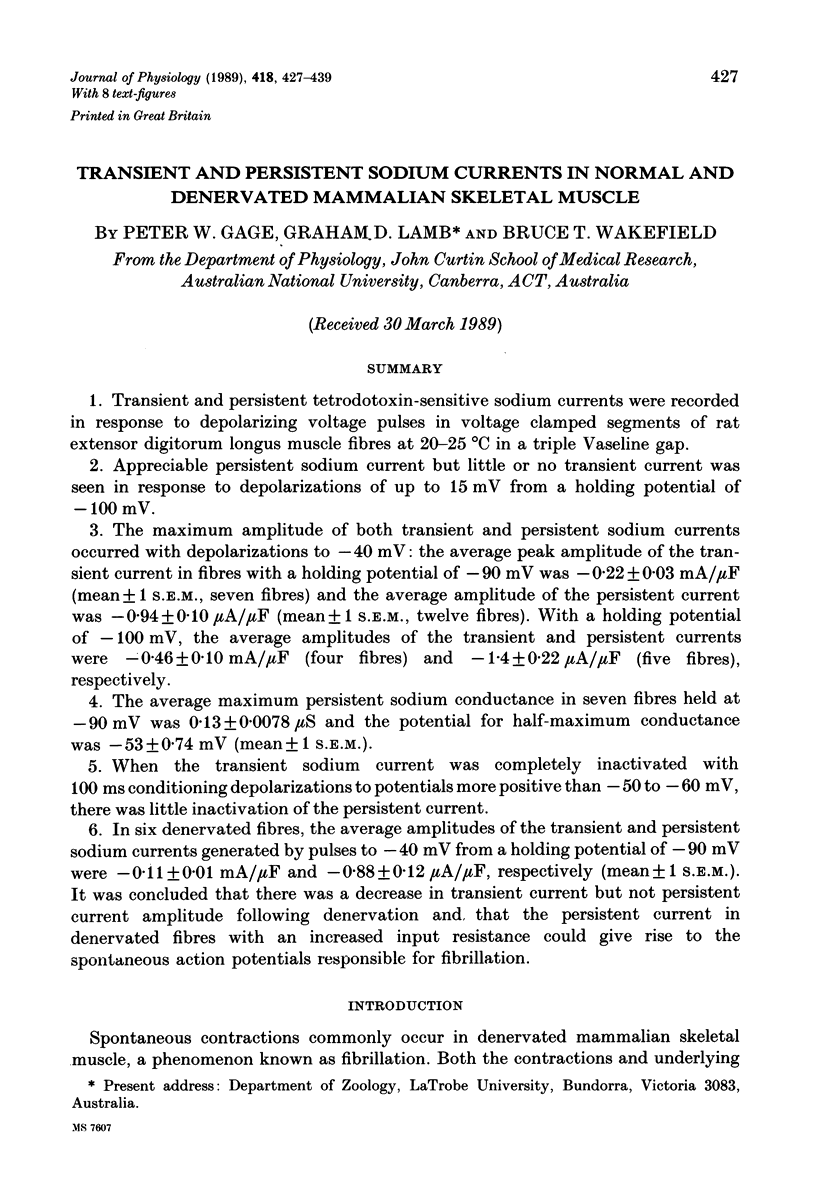
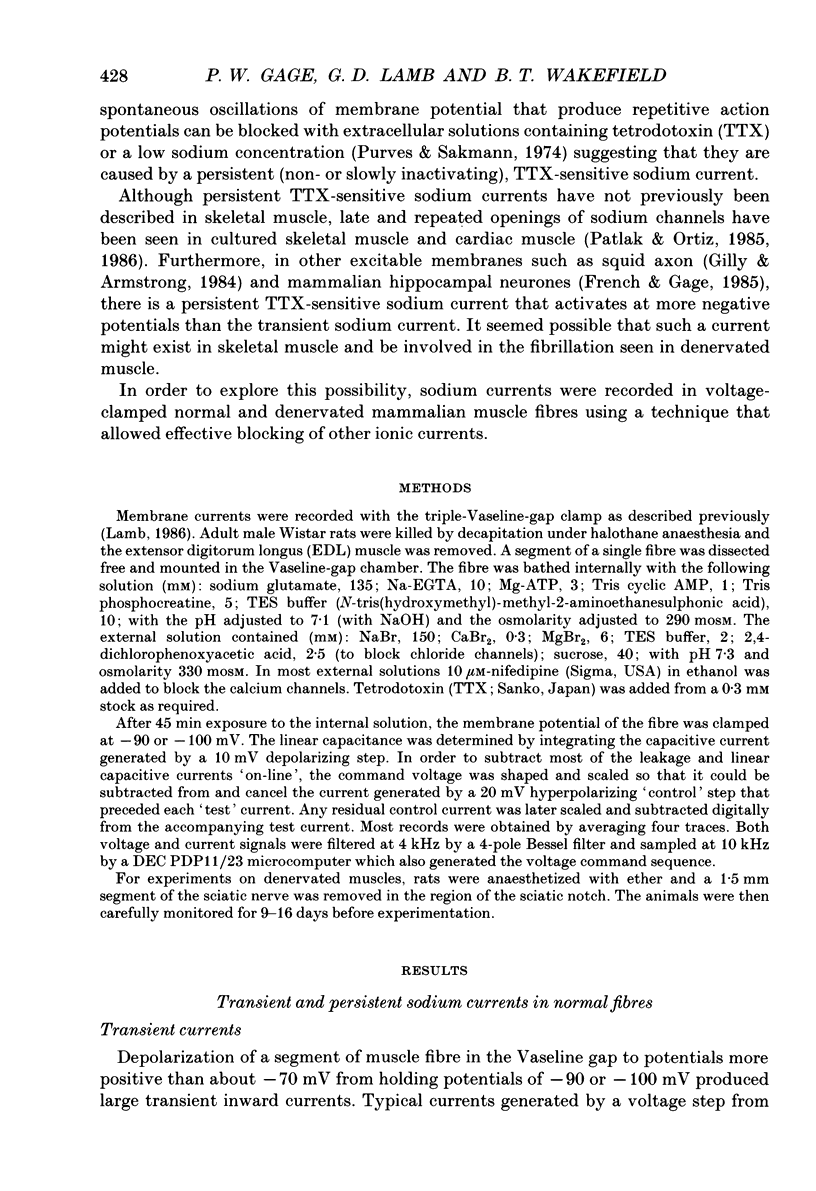
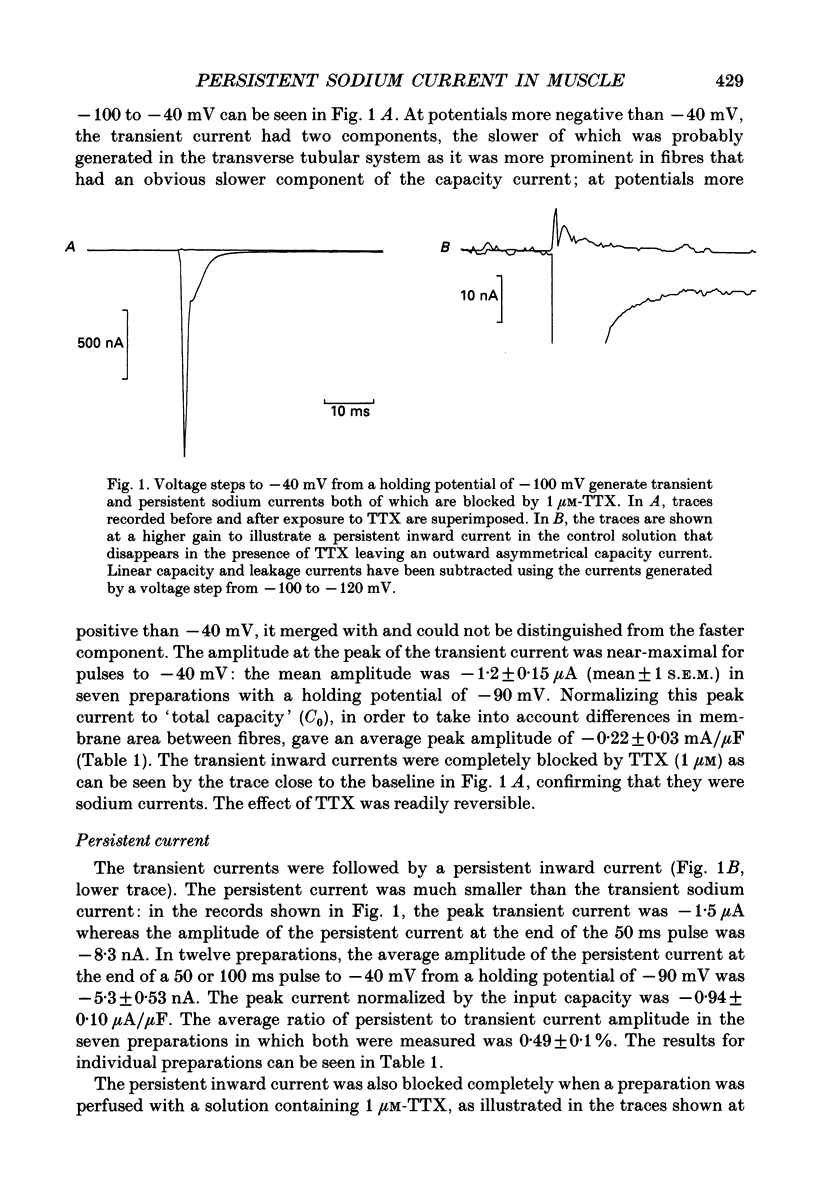
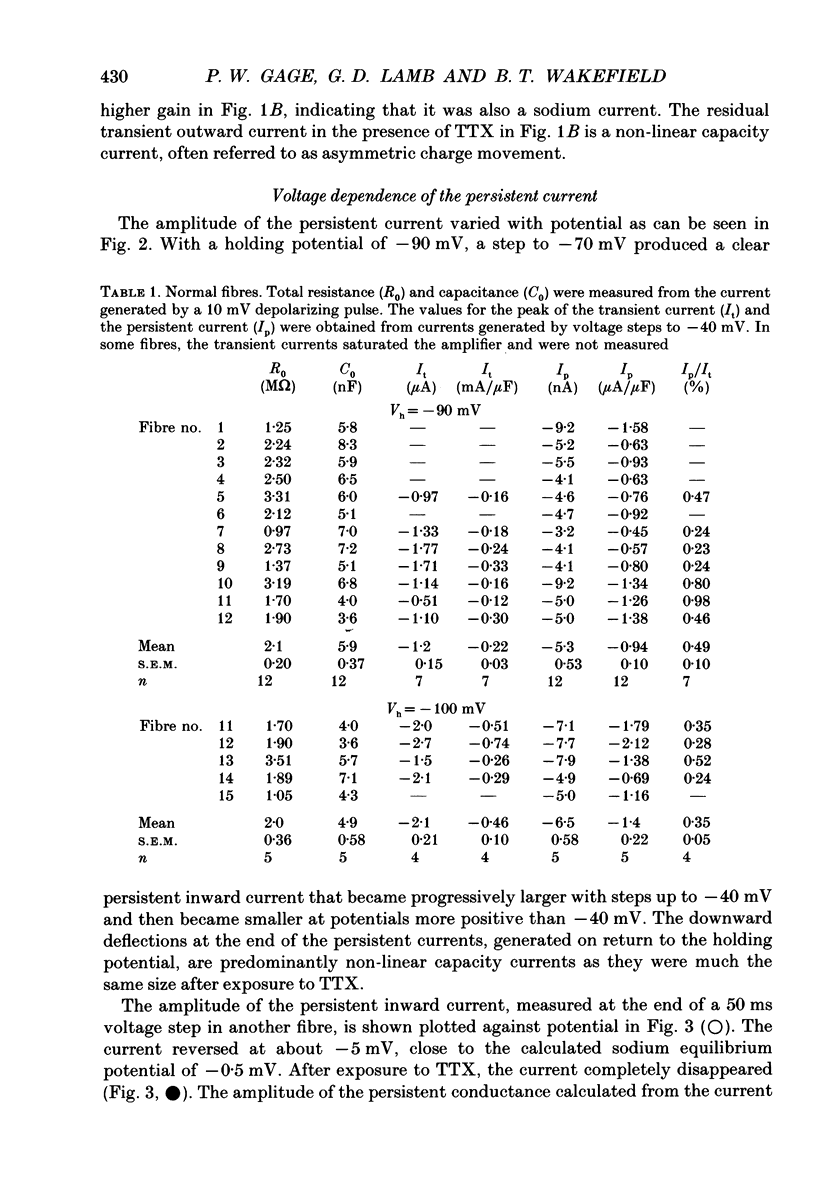
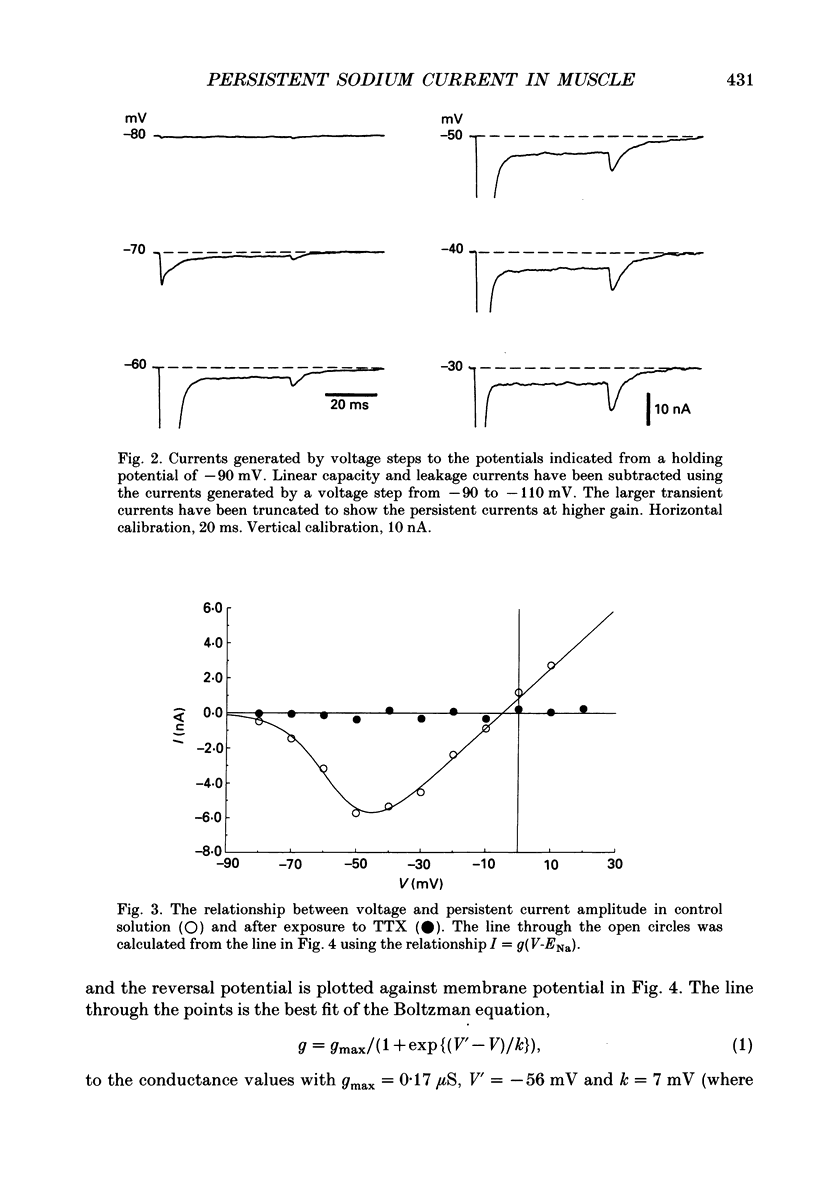
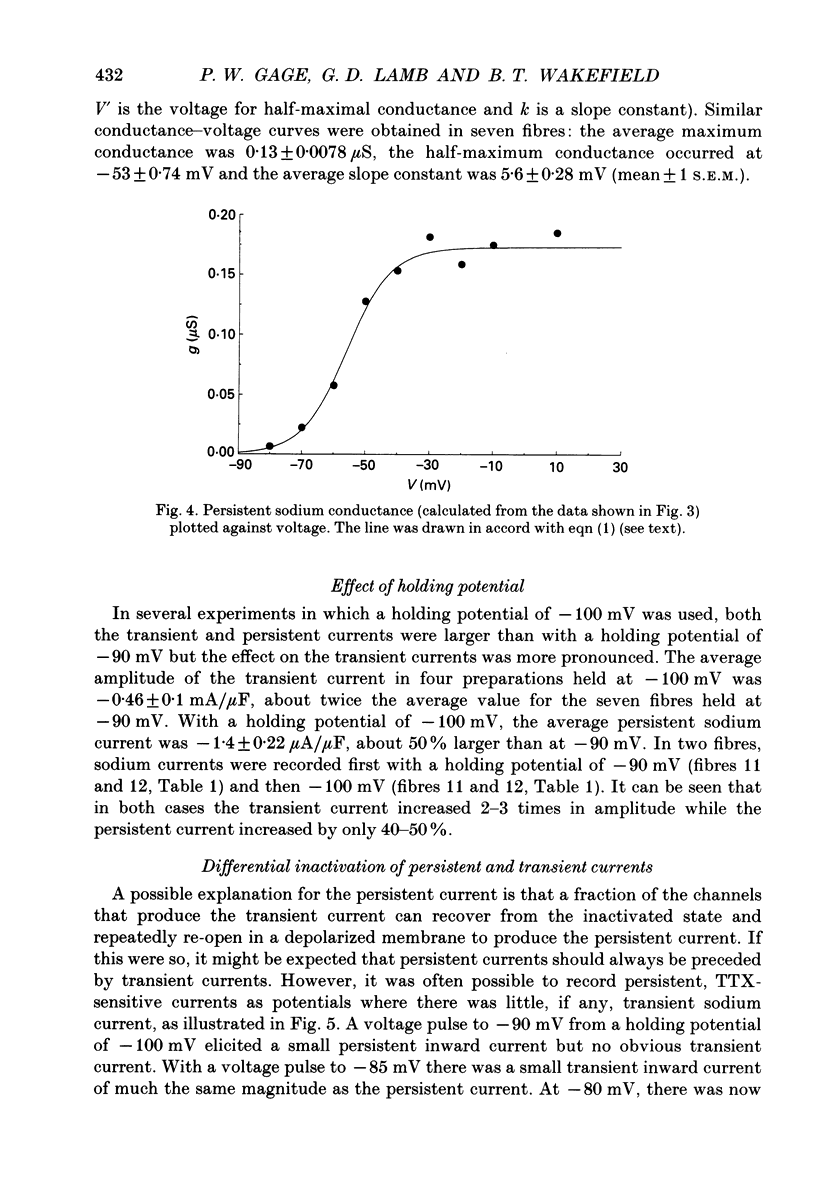
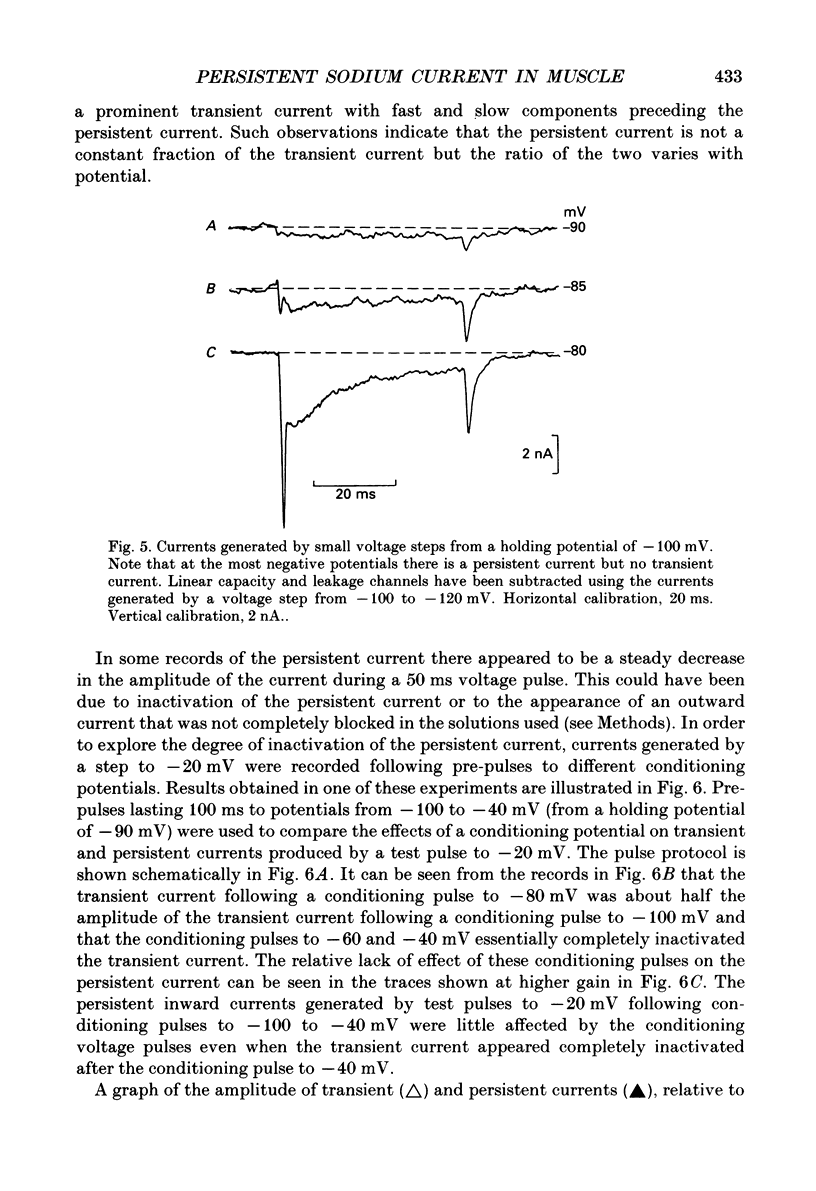
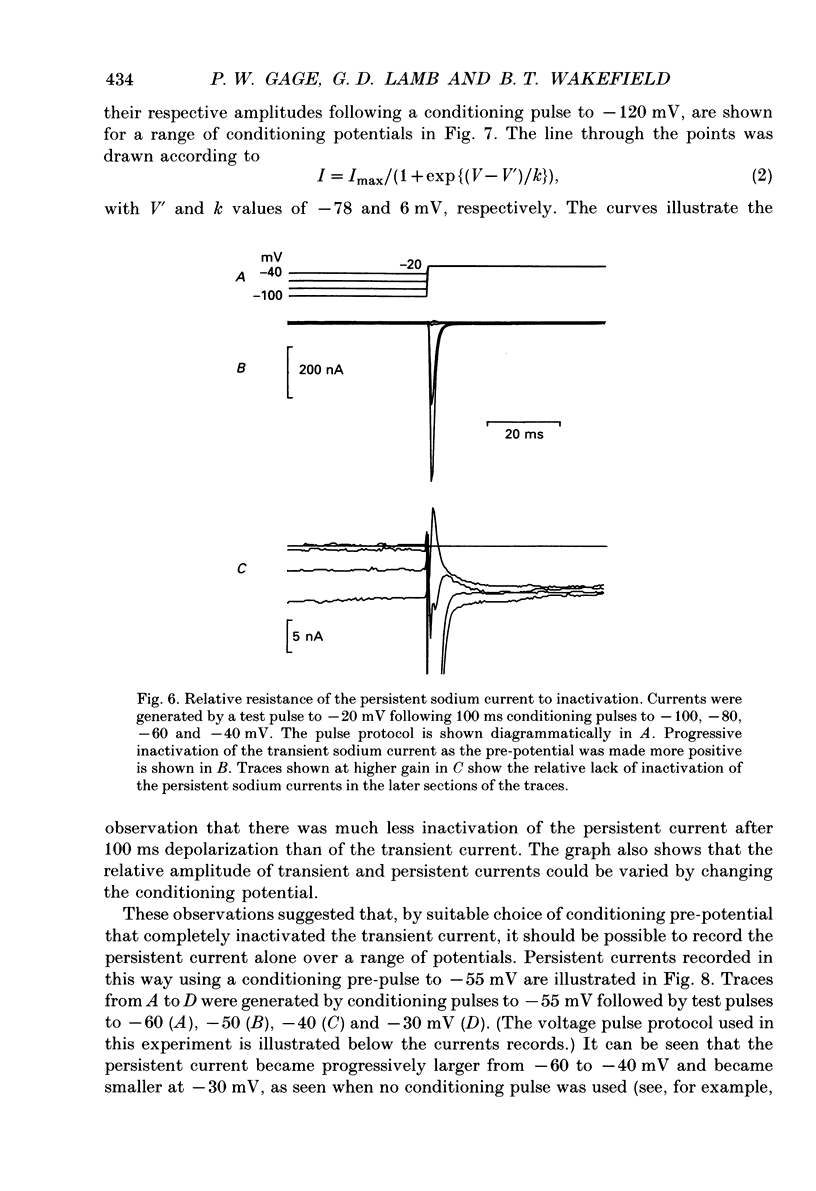
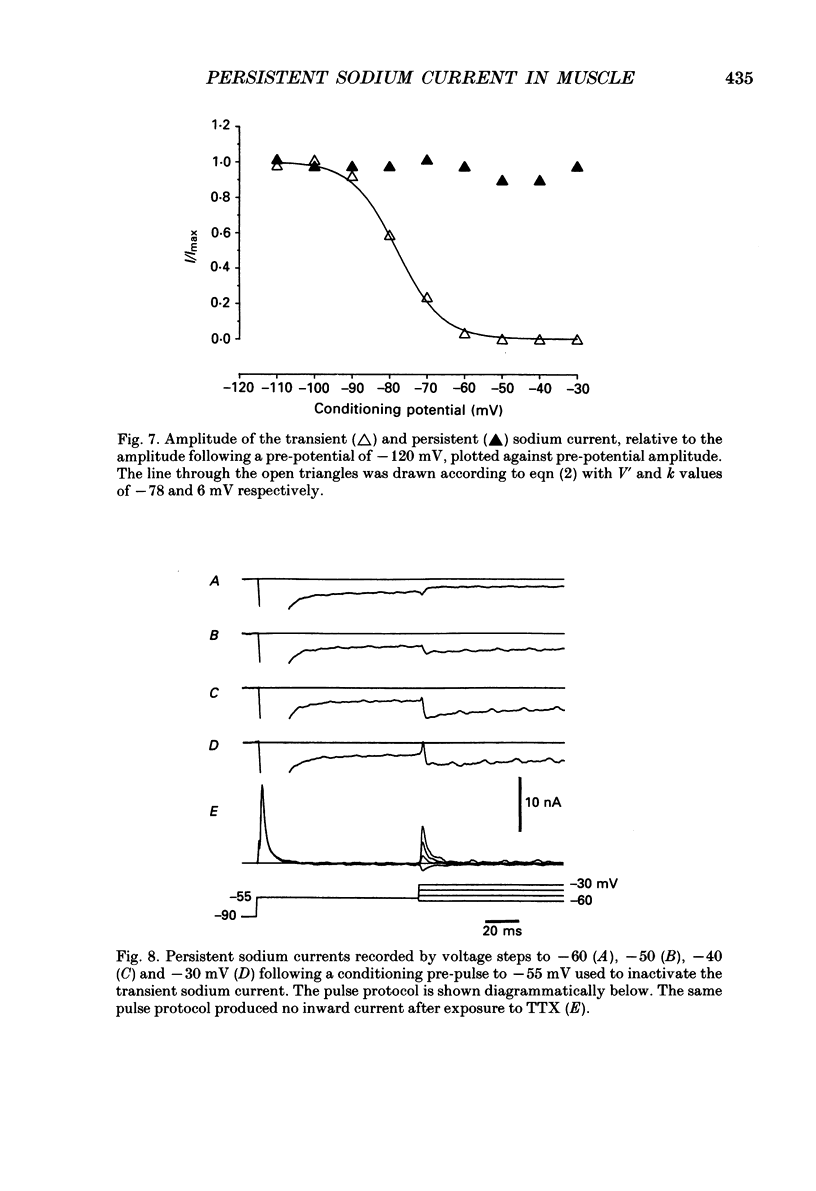
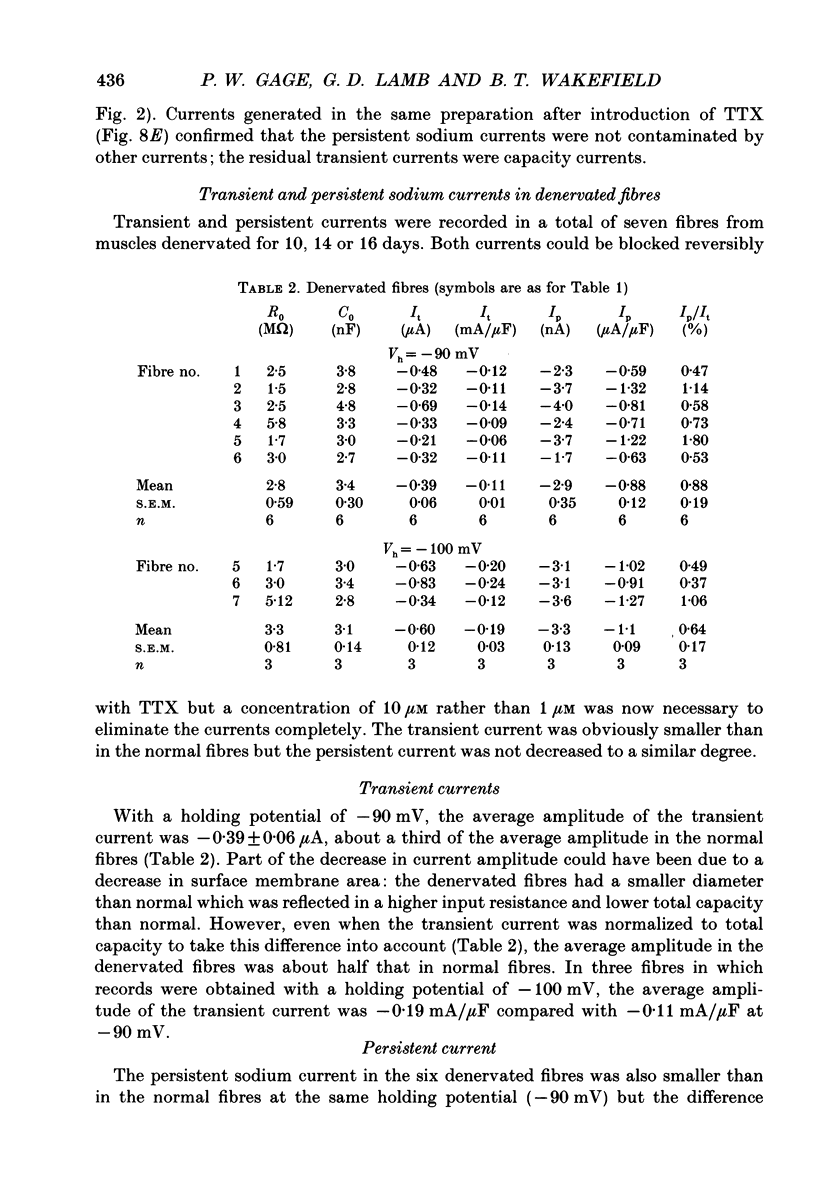
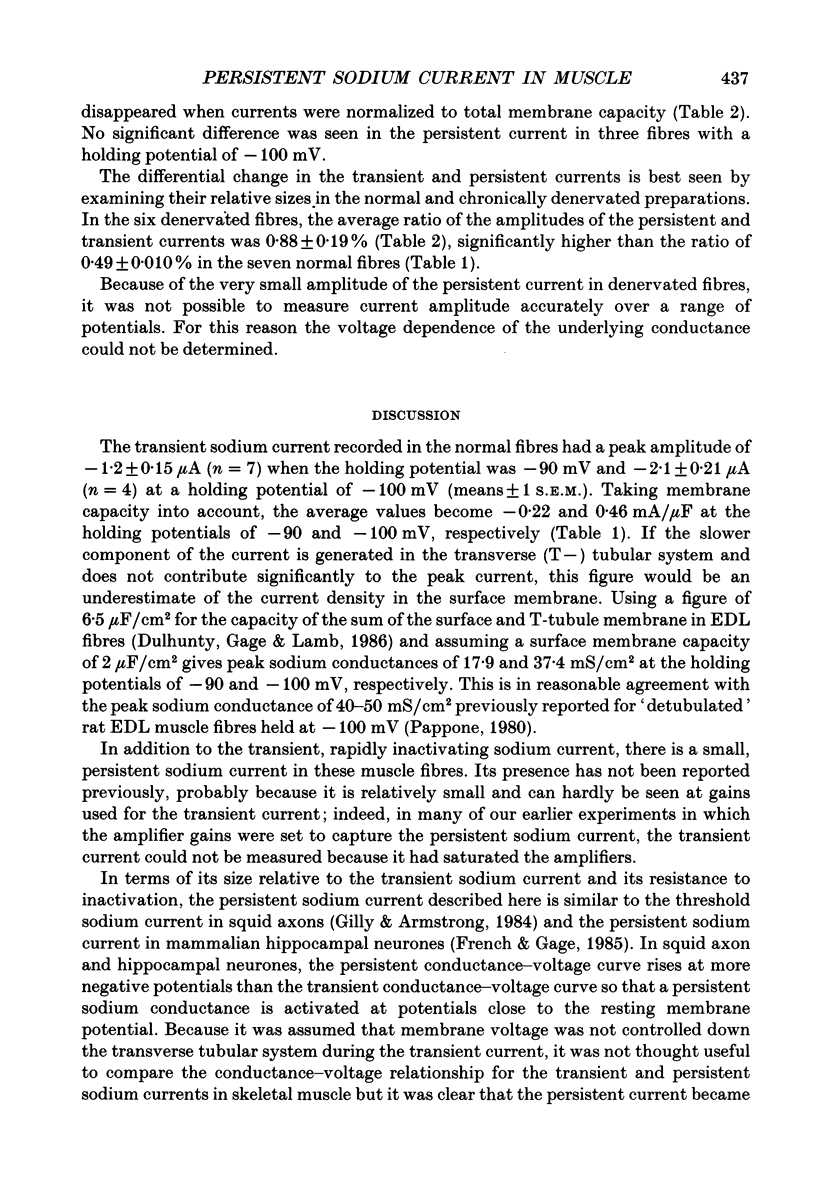
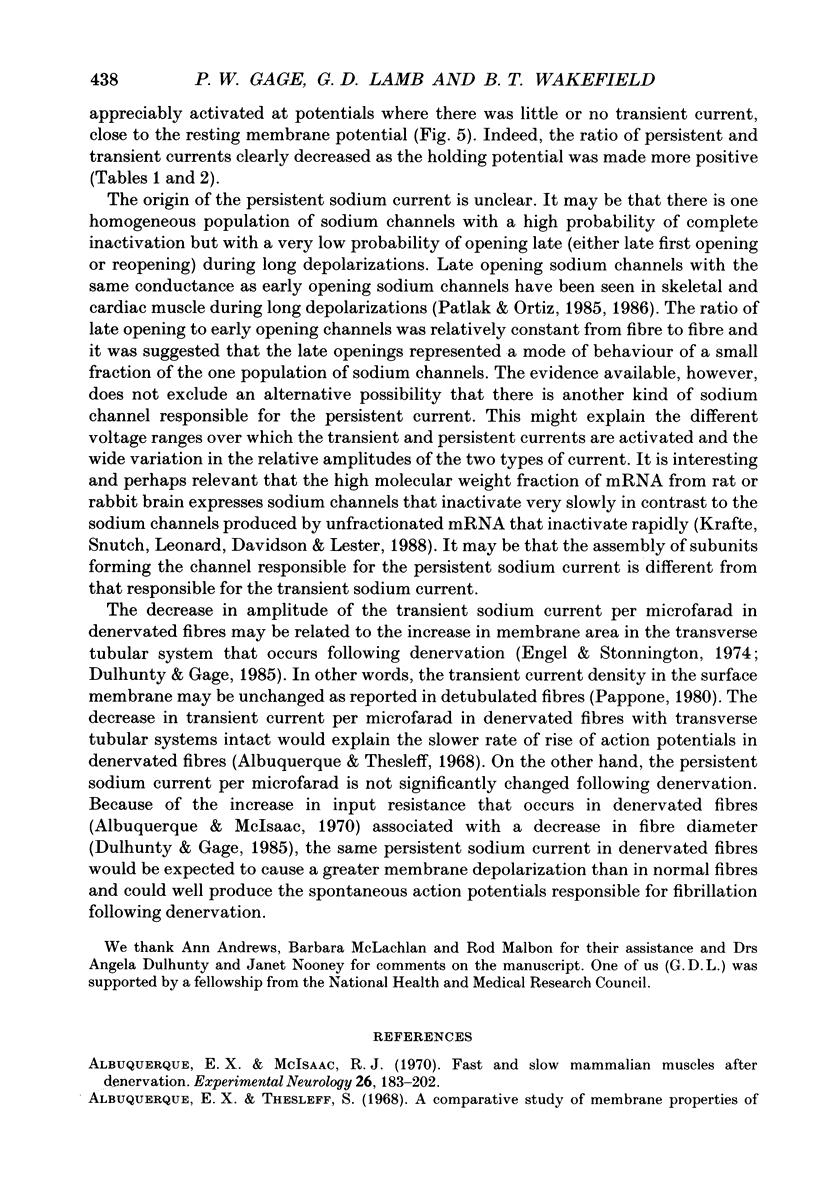
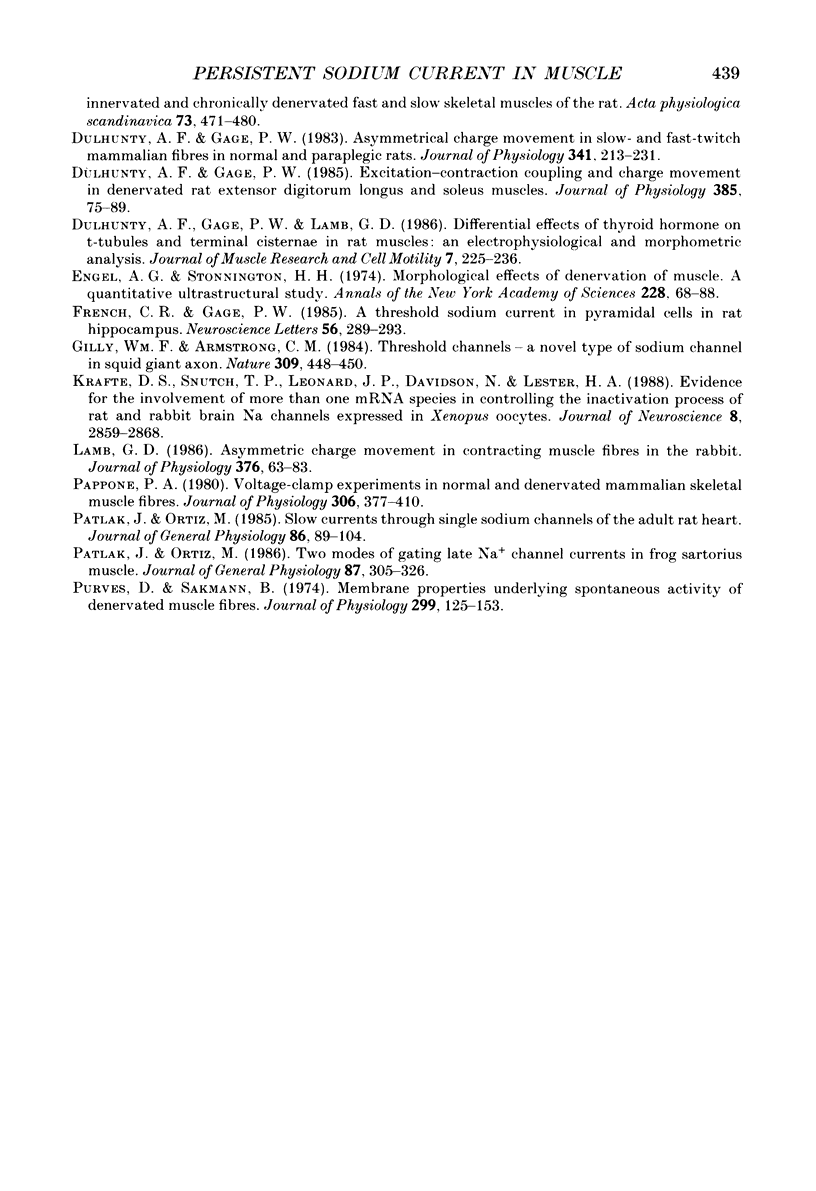
Selected References
These references are in PubMed. This may not be the complete list of references from this article.
- Albuquerque E. X., McIsaac R. J. Fast and slow mammalian muscles after denervation. Exp Neurol. 1970 Jan;26(1):183–202. doi: 10.1016/0014-4886(70)90099-3. [DOI] [PubMed] [Google Scholar]
- Dulhunty A. F., Gage P. W. Asymmetrical charge movement in slow- and fast-twitch mammalian muscle fibres in normal and paraplegic rats. J Physiol. 1983 Aug;341:213–231. doi: 10.1113/jphysiol.1983.sp014802. [DOI] [PMC free article] [PubMed] [Google Scholar]
- Dulhunty A. F., Gage P. W. Excitation-contraction coupling and charge movement in denervated rat extensor digitorum longus and soleus muscles. J Physiol. 1985 Jan;358:75–89. doi: 10.1113/jphysiol.1985.sp015541. [DOI] [PMC free article] [PubMed] [Google Scholar]
- Dulhunty A. F., Gage P. W., Lamb G. D. Differential effects of thyroid hormone on T-tubules and terminal cisternae in rat muscles: an electrophysiological and morphometric analysis. J Muscle Res Cell Motil. 1986 Jun;7(3):225–236. doi: 10.1007/BF01753555. [DOI] [PubMed] [Google Scholar]
- Engel A. G., Stonnington H. H. Trophic functions of the neuron. II. Denervation and regulation of muscle. Morphological effects of denervation of muscle. A quantitative ultrastructural study. Ann N Y Acad Sci. 1974 Mar 22;228(0):68–88. doi: 10.1111/j.1749-6632.1974.tb20503.x. [DOI] [PubMed] [Google Scholar]
- French C. R., Gage P. W. A threshold sodium current in pyramidal cells in rat hippocampus. Neurosci Lett. 1985 May 23;56(3):289–293. doi: 10.1016/0304-3940(85)90257-5. [DOI] [PubMed] [Google Scholar]
- Gilly W. F., Armstrong C. M. Threshold channels--a novel type of sodium channel in squid giant axon. 1984 May 31-Jun 6Nature. 309(5967):448–450. doi: 10.1038/309448a0. [DOI] [PubMed] [Google Scholar]
- Krafte D. S., Snutch T. P., Leonard J. P., Davidson N., Lester H. A. Evidence for the involvement of more than one mRNA species in controlling the inactivation process of rat and rabbit brain Na channels expressed in Xenopus oocytes. J Neurosci. 1988 Aug;8(8):2859–2868. doi: 10.1523/JNEUROSCI.08-08-02859.1988. [DOI] [PMC free article] [PubMed] [Google Scholar]
- Lamb G. D. Asymmetric charge movement in contracting muscle fibres in the rabbit. J Physiol. 1986 Jul;376:63–83. doi: 10.1113/jphysiol.1986.sp016142. [DOI] [PMC free article] [PubMed] [Google Scholar]
- Pappone P. A. Voltage-clamp experiments in normal and denervated mammalian skeletal muscle fibres. J Physiol. 1980 Sep;306:377–410. doi: 10.1113/jphysiol.1980.sp013403. [DOI] [PMC free article] [PubMed] [Google Scholar]
- Patlak J. B., Ortiz M. Slow currents through single sodium channels of the adult rat heart. J Gen Physiol. 1985 Jul;86(1):89–104. doi: 10.1085/jgp.86.1.89. [DOI] [PMC free article] [PubMed] [Google Scholar]
- Patlak J. B., Ortiz M. Two modes of gating during late Na+ channel currents in frog sartorius muscle. J Gen Physiol. 1986 Feb;87(2):305–326. doi: 10.1085/jgp.87.2.305. [DOI] [PMC free article] [PubMed] [Google Scholar]
- Purves D., Sakmann B. Membrane properties underlying spontaneous activity of denervated muscle fibres. J Physiol. 1974 May;239(1):125–153. doi: 10.1113/jphysiol.1974.sp010559. [DOI] [PMC free article] [PubMed] [Google Scholar]