Abstract
1. The effects of chlorpromazine on the voltage-activated sodium and type I (transient) calcium channels were studied in cultured mouse neuroblastoma cells (N1E-115) using the whole-cell patch-clamp technique. 2. Chlorpromazine (2-10 x 10(-6) M) blocked both the sodium channel current and the calcium channel current as carried by Ba2+ in a reversible and dose-dependent manner. 3. The block was not associated with any change in the time course of the activation and inactivation of the sodium and calcium channel currents. 4. The dose-response relationships for the block of these channels measured with a holding potential of -120 mV indicated a 1:1 binding stoichiometry with apparent dissociation constants of 2.5 +/- 10(-6) M and 1.5 +/- 10(-5) M for the sodium and calcium channels, respectively. 5. The block was dependent on the holding potential for both channel currents. The apparent dissociation constant for the sodium channel was decreased to 0.65 +/- 10(-6) M when the membrane was held at -80 mV. The apparent dissociation constant for the calcium channel was decreased to 3.2 +/- 10(-6) M when the membrane was held at -60 mV. 6. The steady-state inactivation curve for the sodium channel was shifted by 12.4 +/- 1.8 mV to more negative potentials by exposure to 1 x 10(-6) M-chlorpromazine. The inactivation curve for the calcium channel was also shifted by 15.4 +/- 3.2 mV to more negative potentials by exposure to 1 x 10(-5) M-chlorpromazine. These results indicate a greater affinity of chlorpromazine for the inactivated state of the channels than for the resting state. 7. Chlorpromazine caused a marked use-dependent block of the sodium channel current, as demonstrated by a cumulative increase of the block during a train of depolarizing pulses. The use-dependent block was observed even with an interpulse interval as long as 2 s. 8. On the other hand, the block of the calcium channel current did not notably accumulate during a train of depolarizing pulses even when extremely prolonged (1 s) pulses were applied at a very short interpulse interval (200 ms). 9. The marked use dependence of the sodium channel block was due to a very slow repriming of the drug-bound sodium channels from inactivation, whereas the lack of use dependence of the calcium channel block was due to a rapid repriming of the drug-bound calcium channels.(ABSTRACT TRUNCATED AT 400 WORDS)
Full text
PDF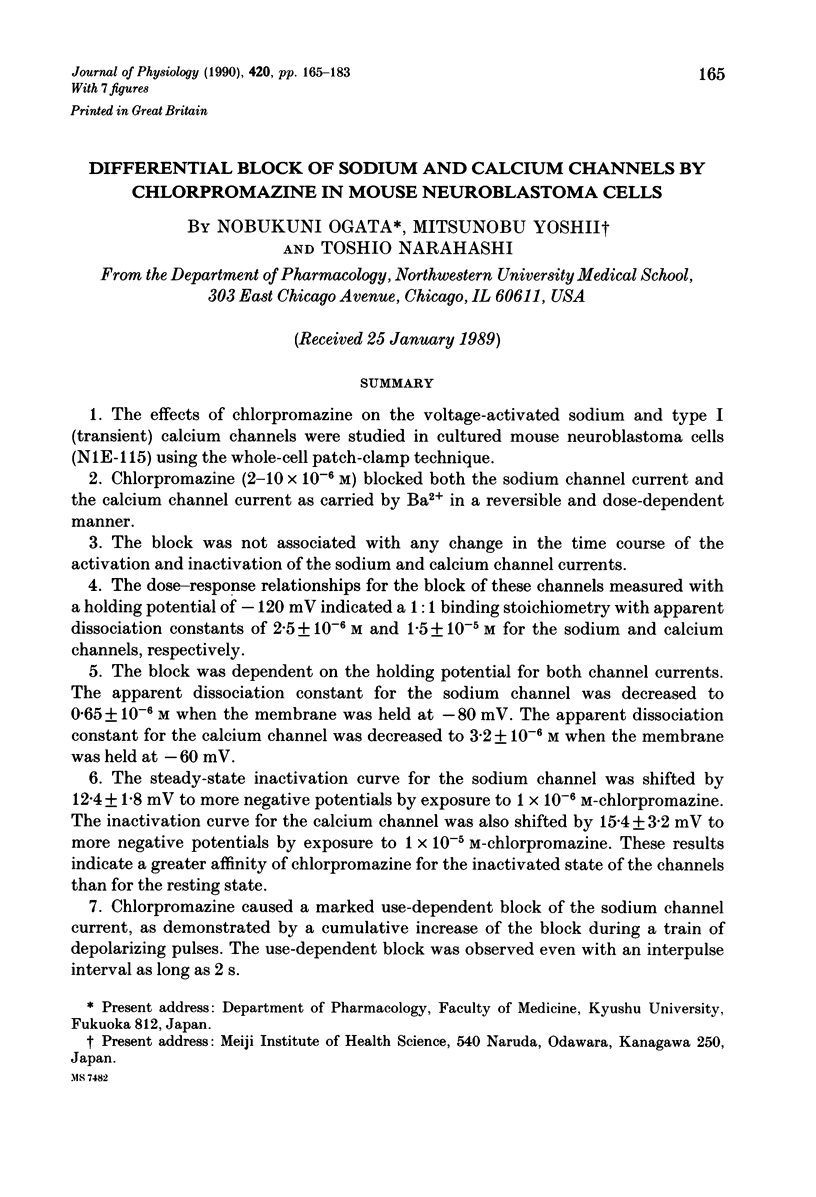
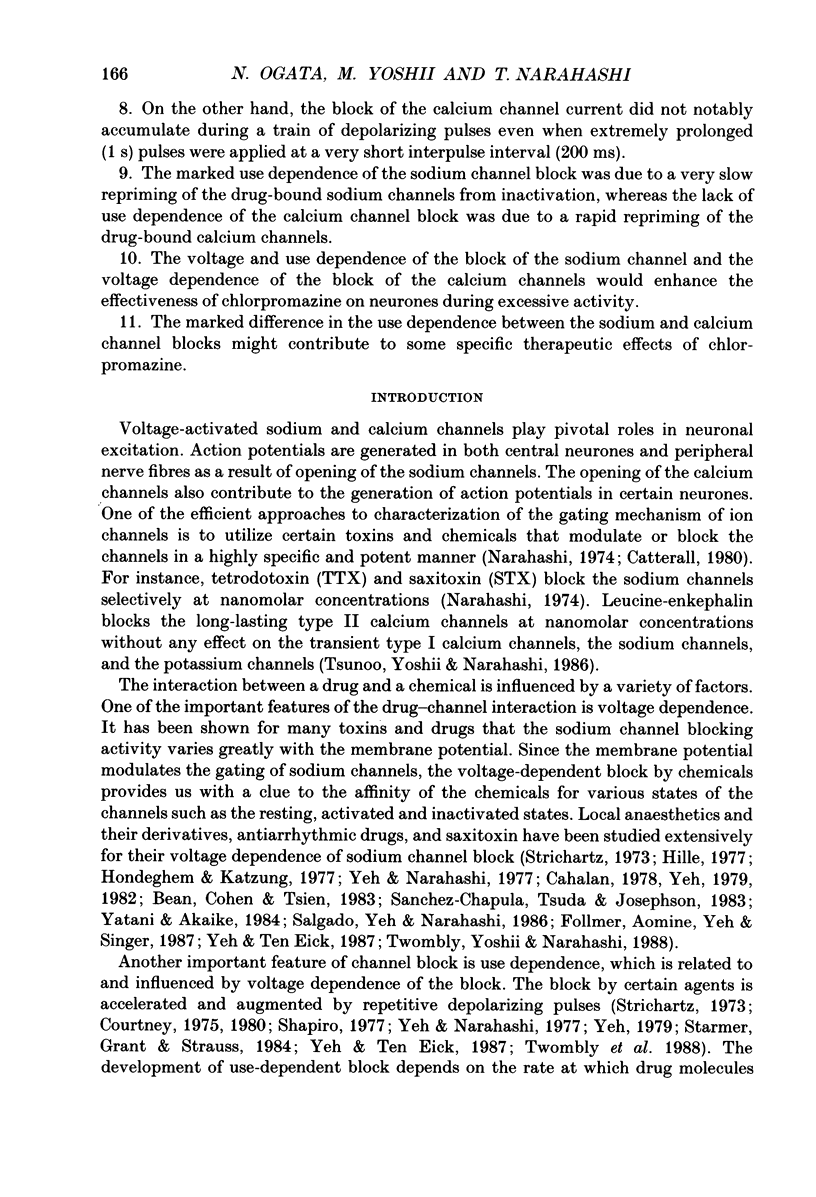
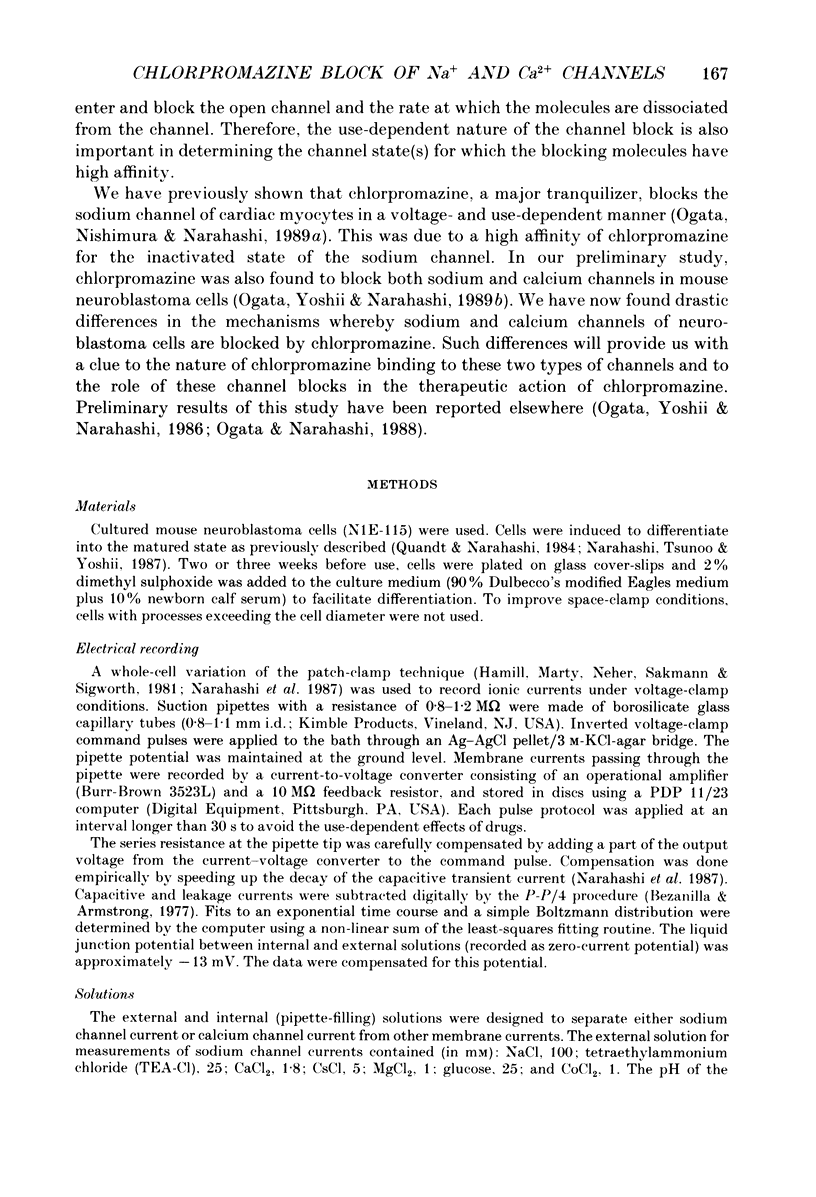
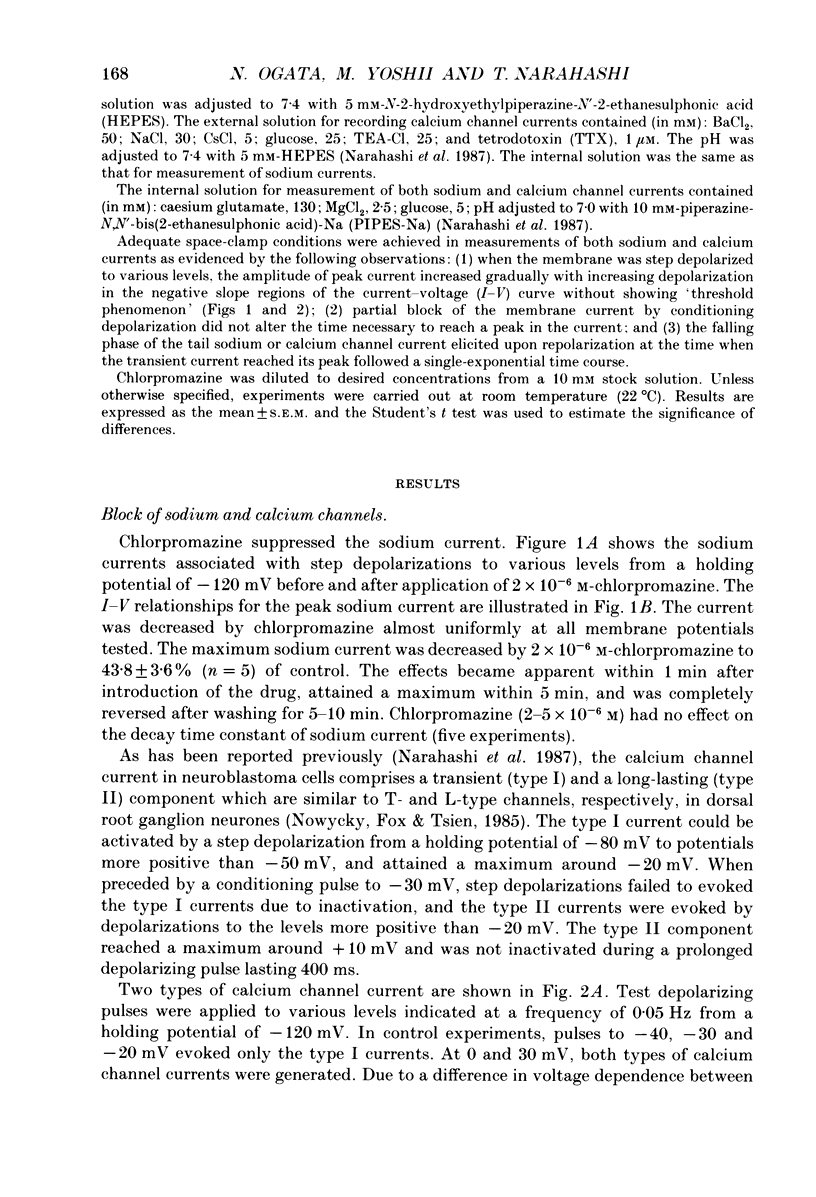
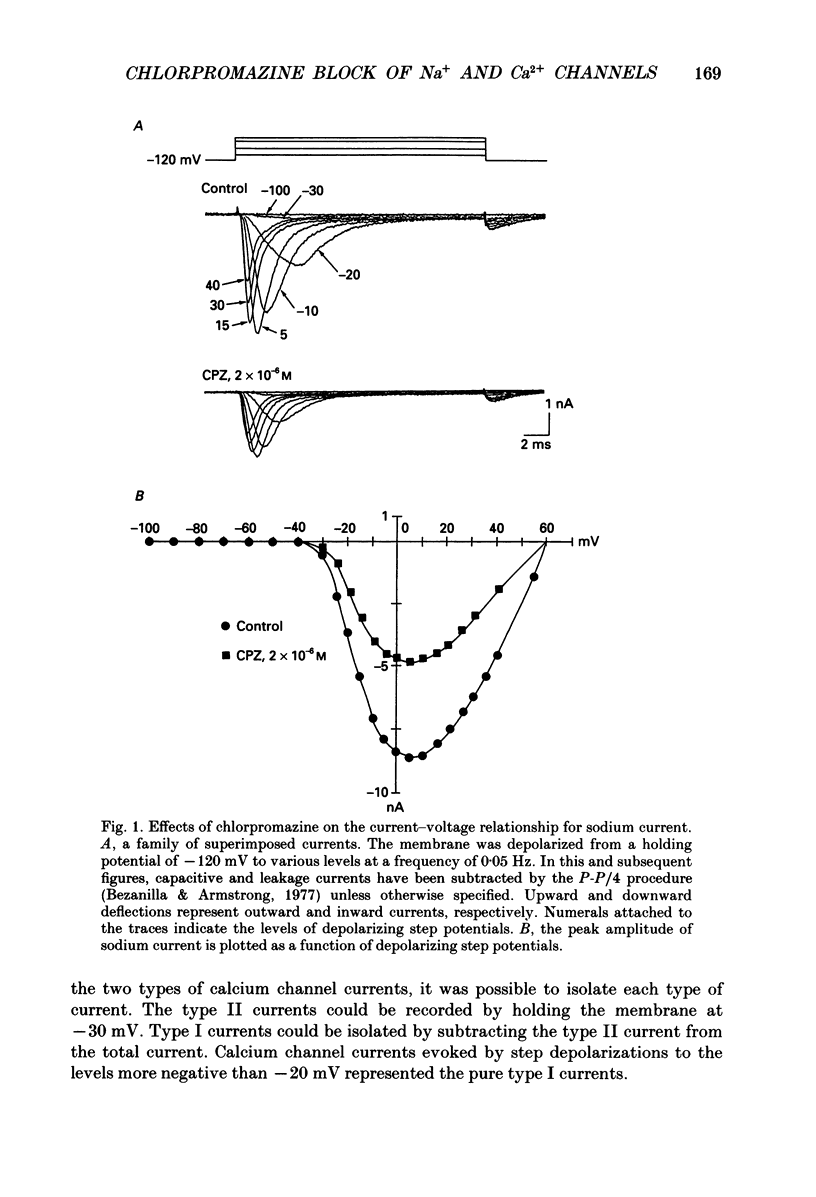
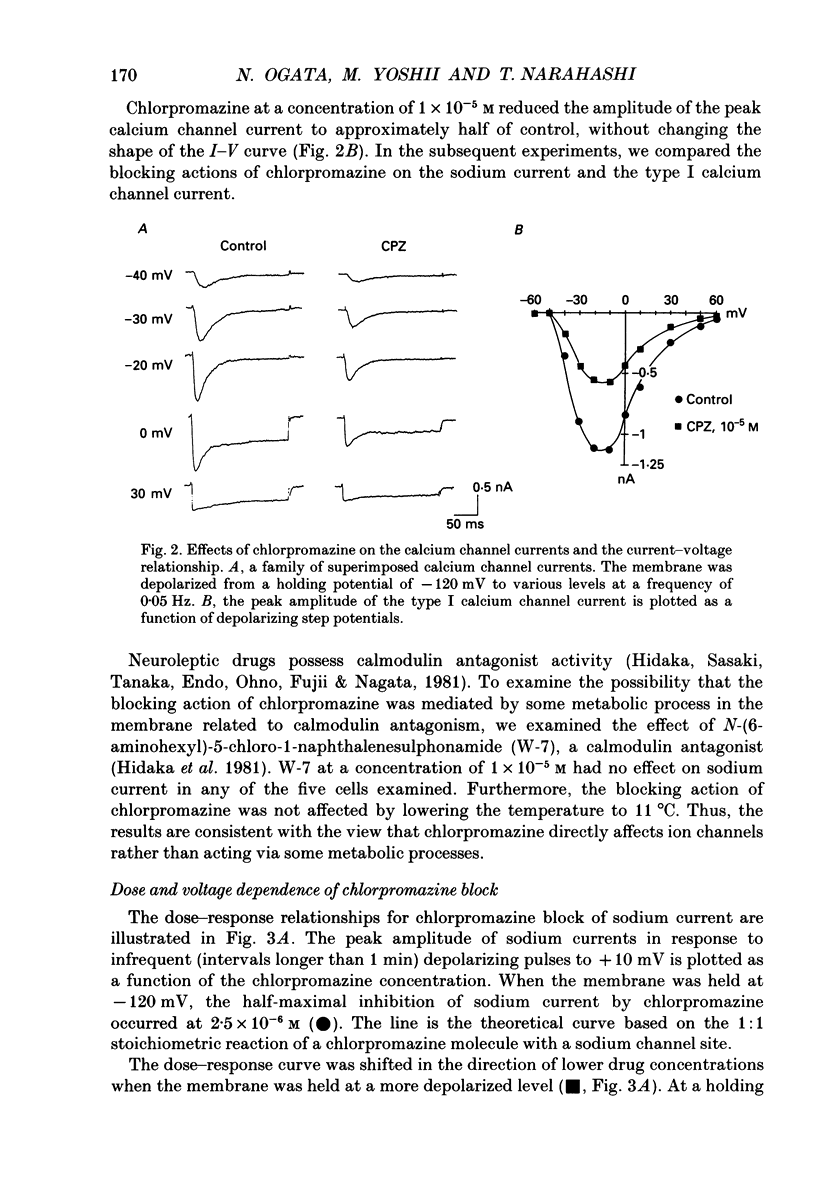
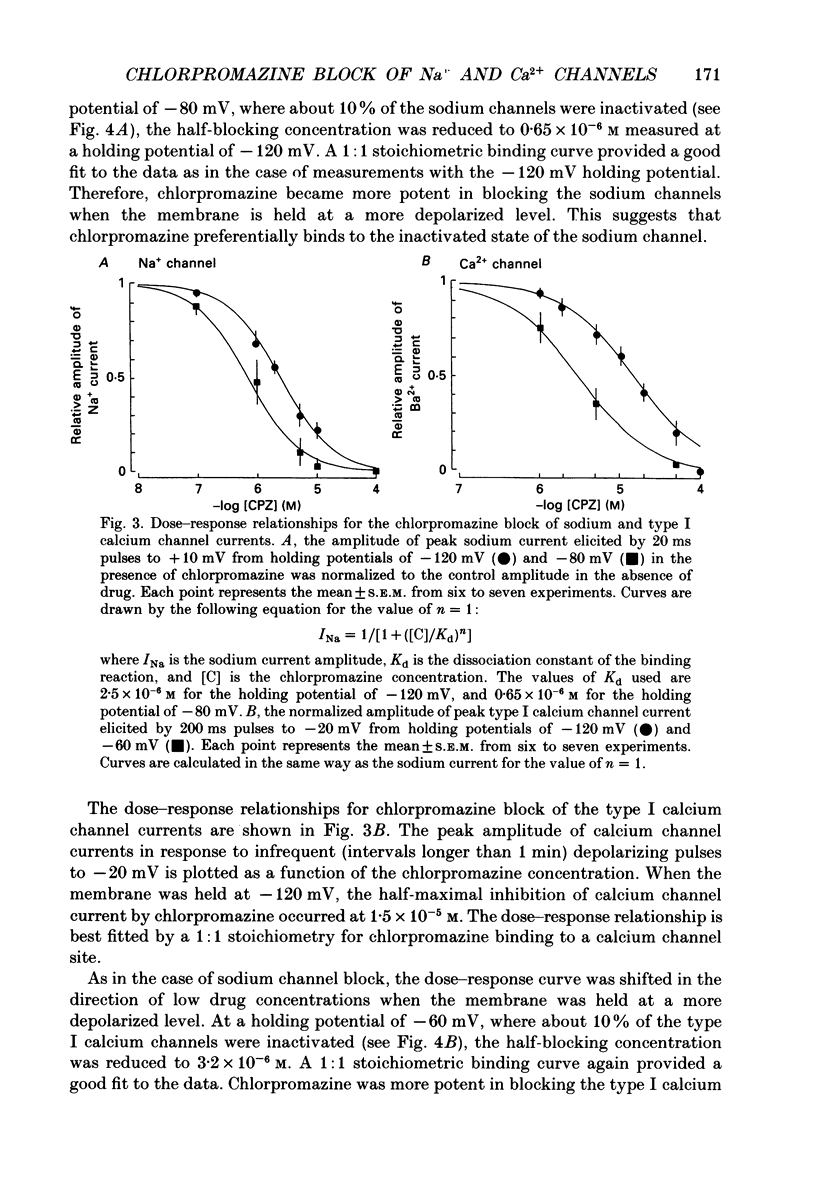
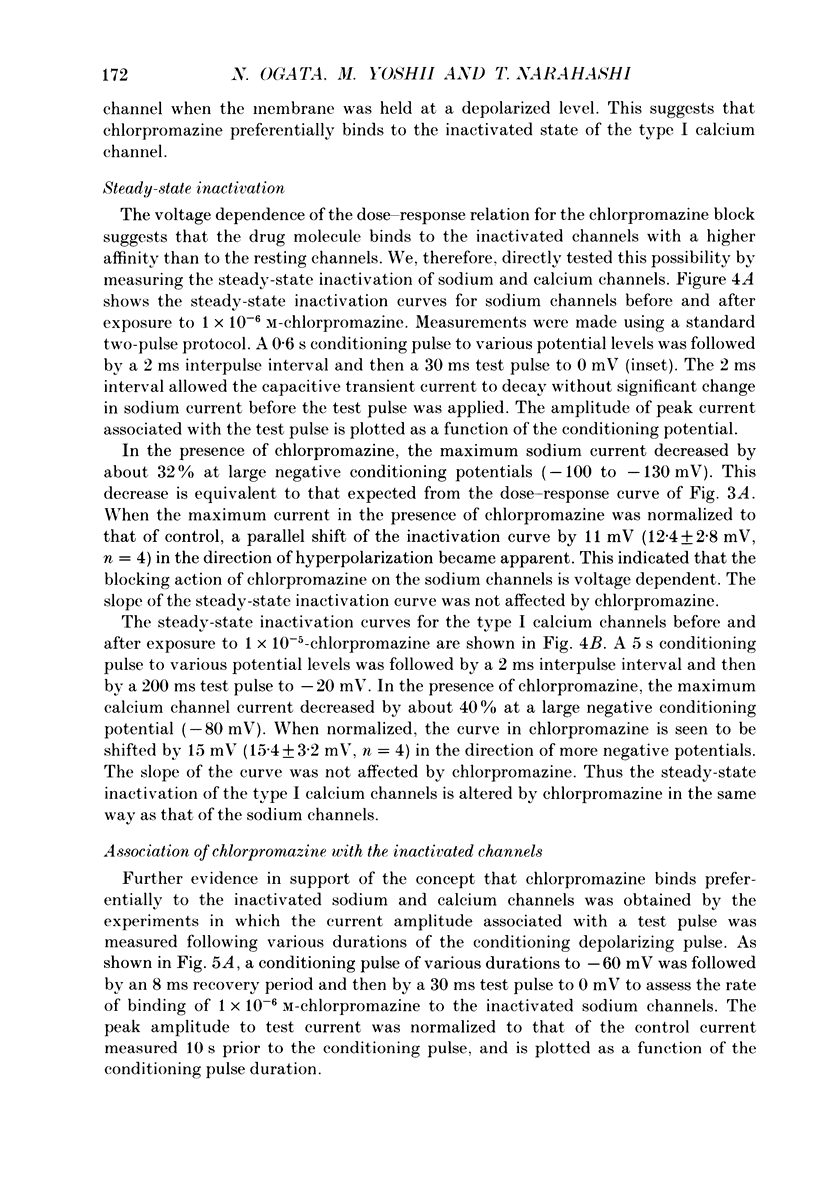
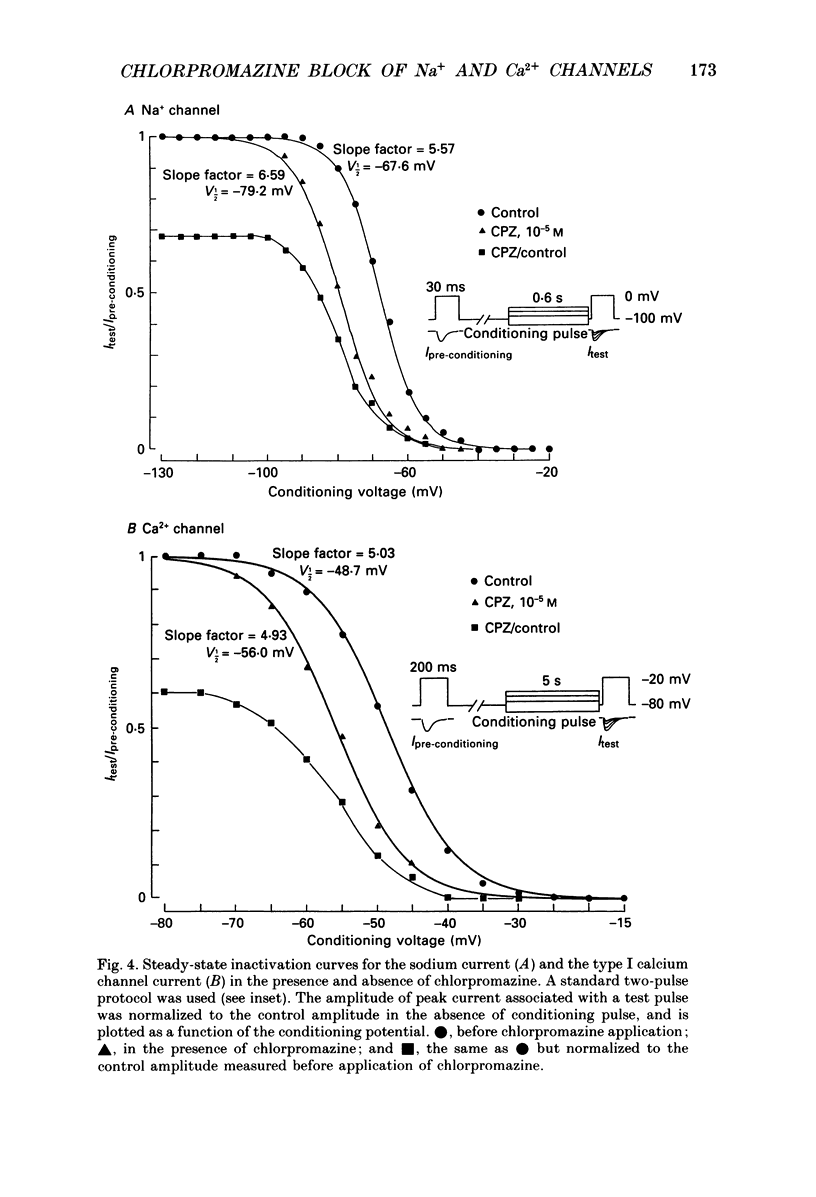
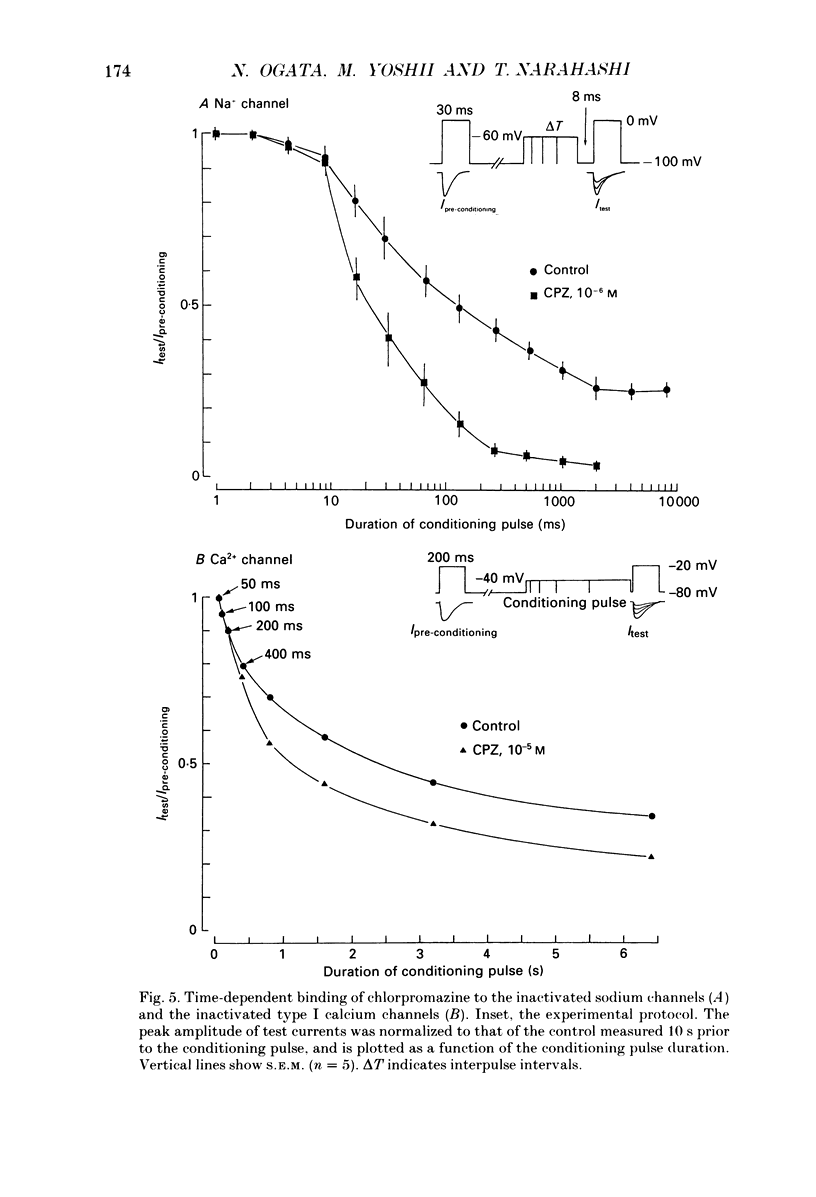
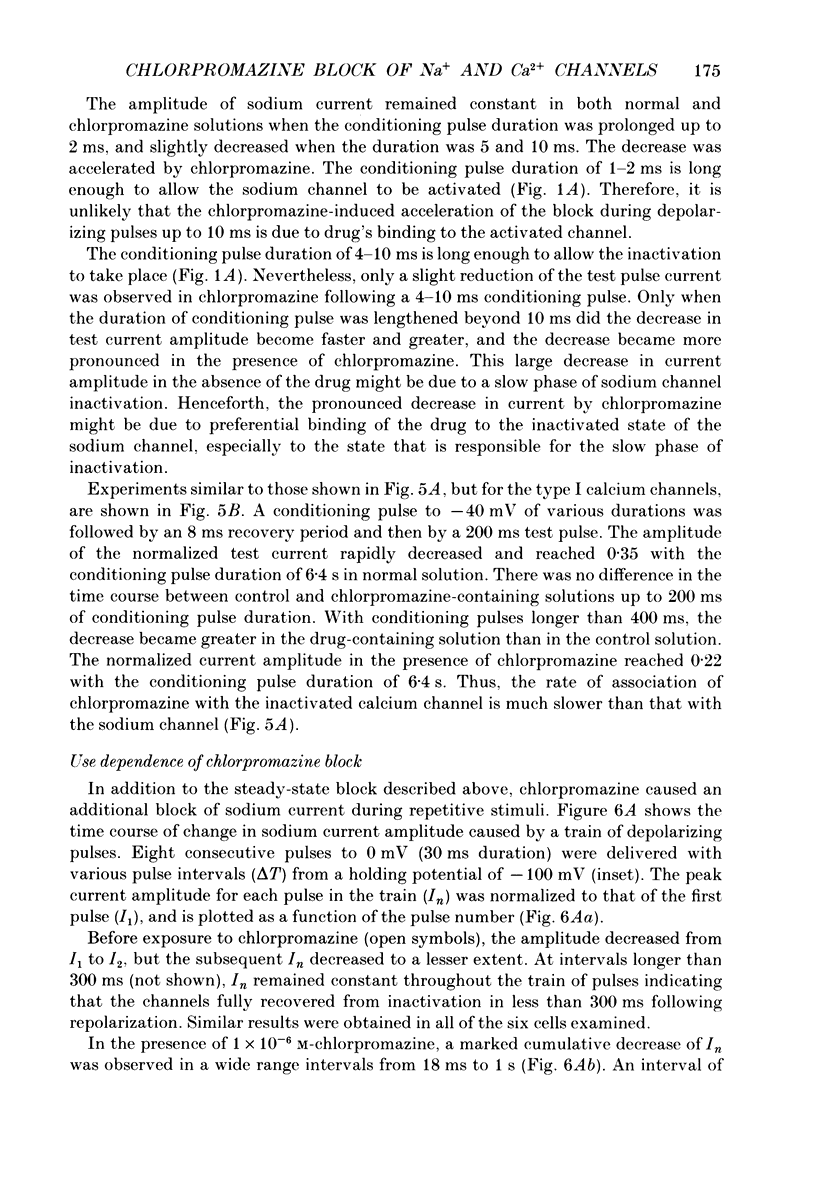
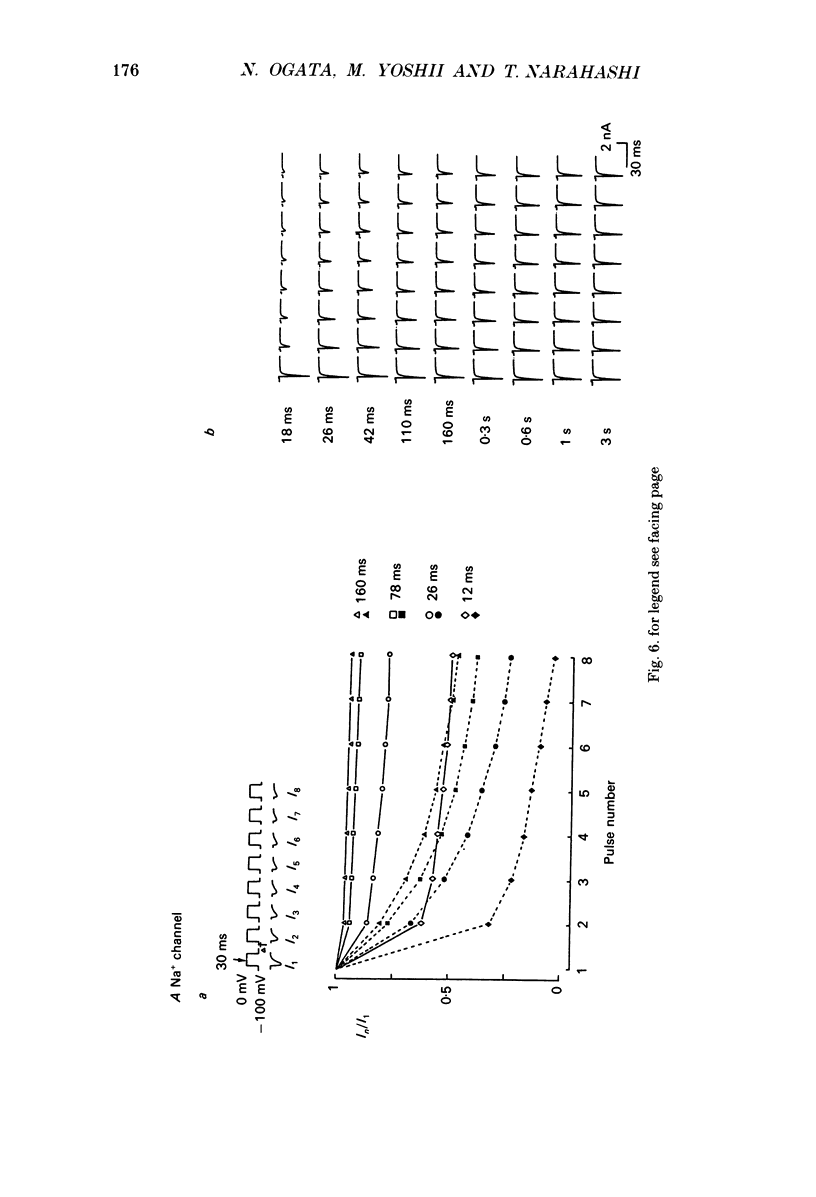
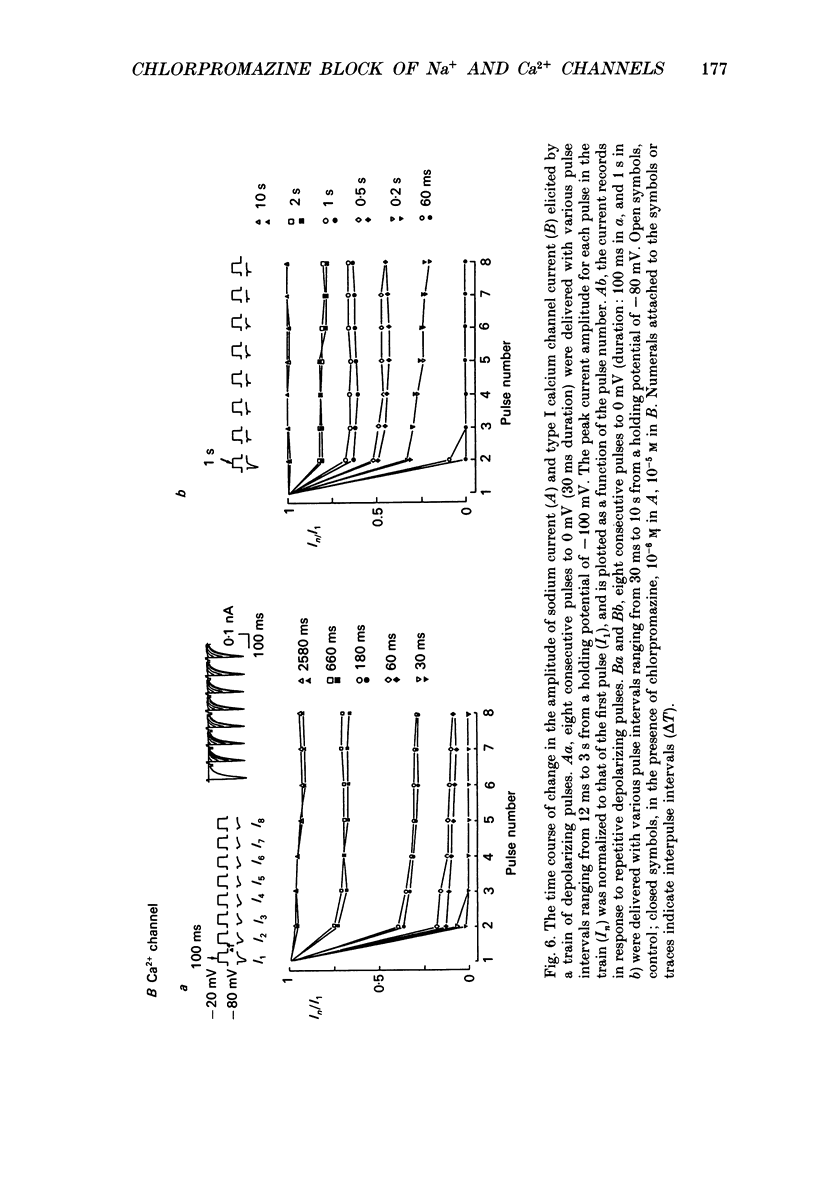
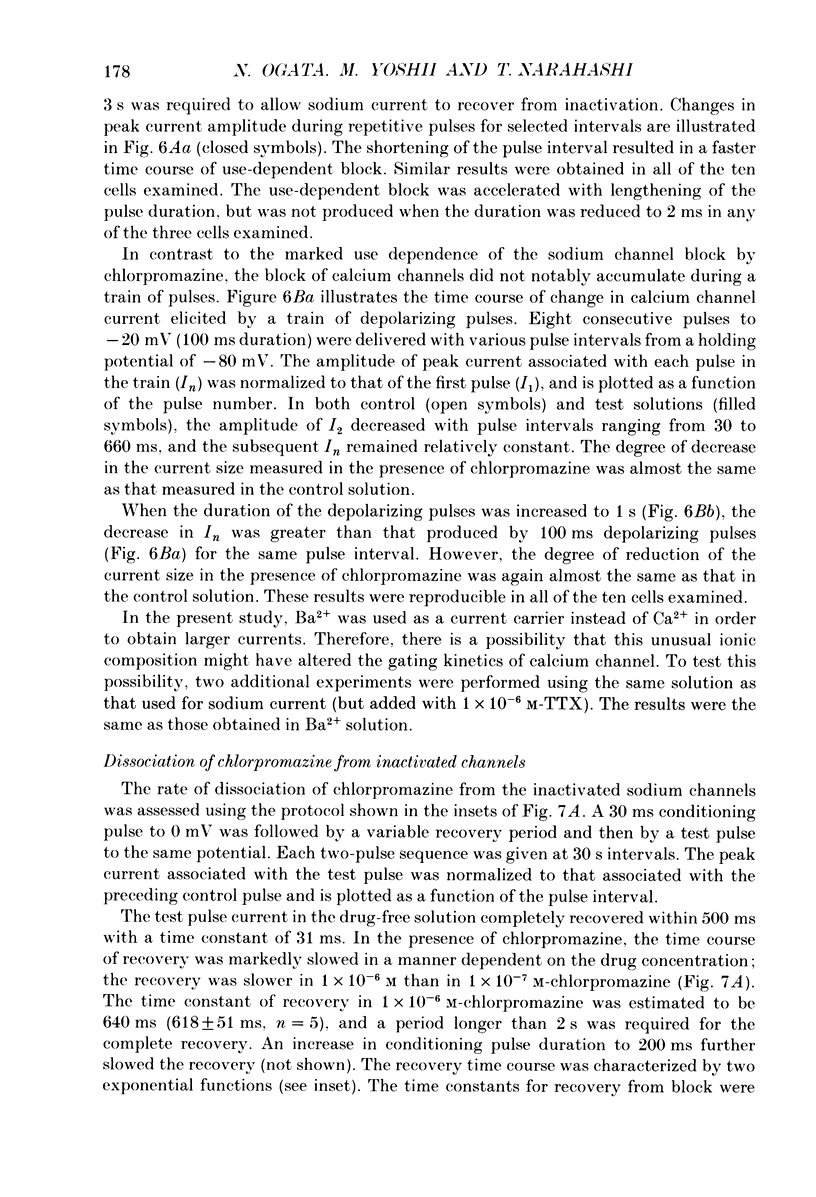
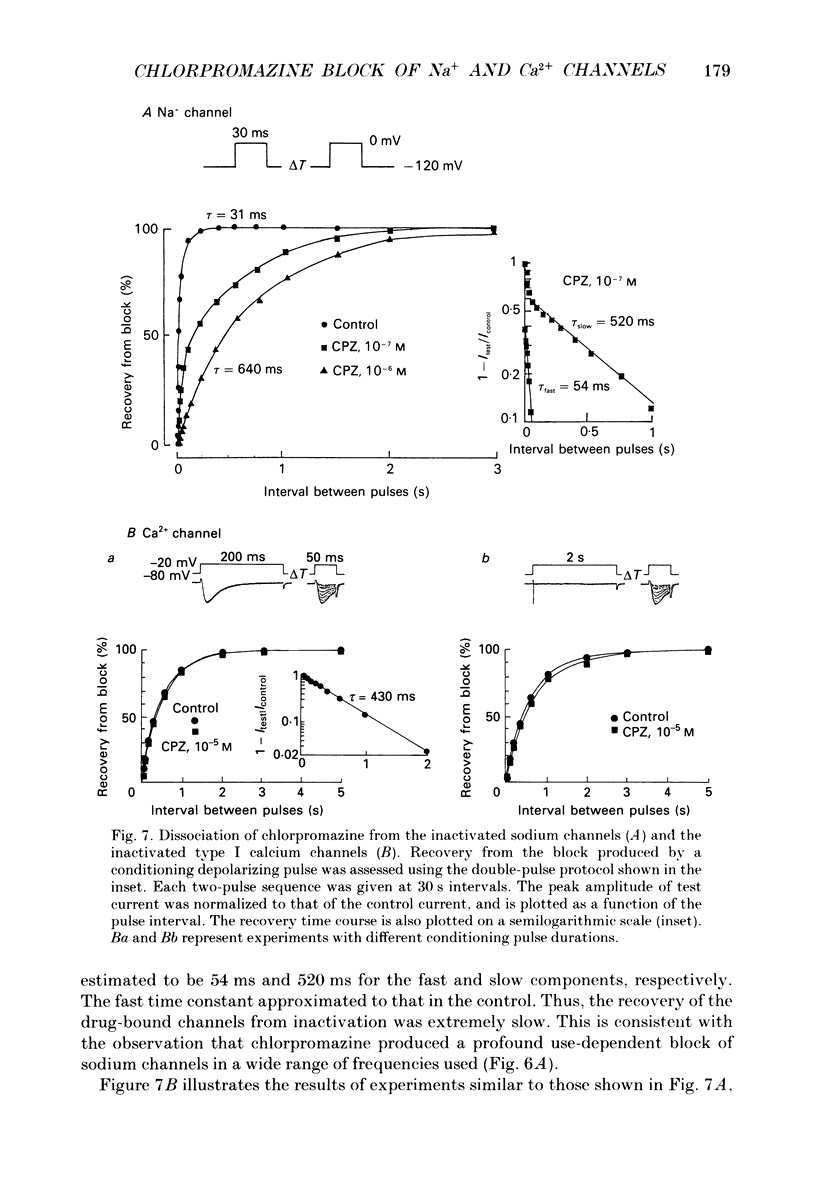
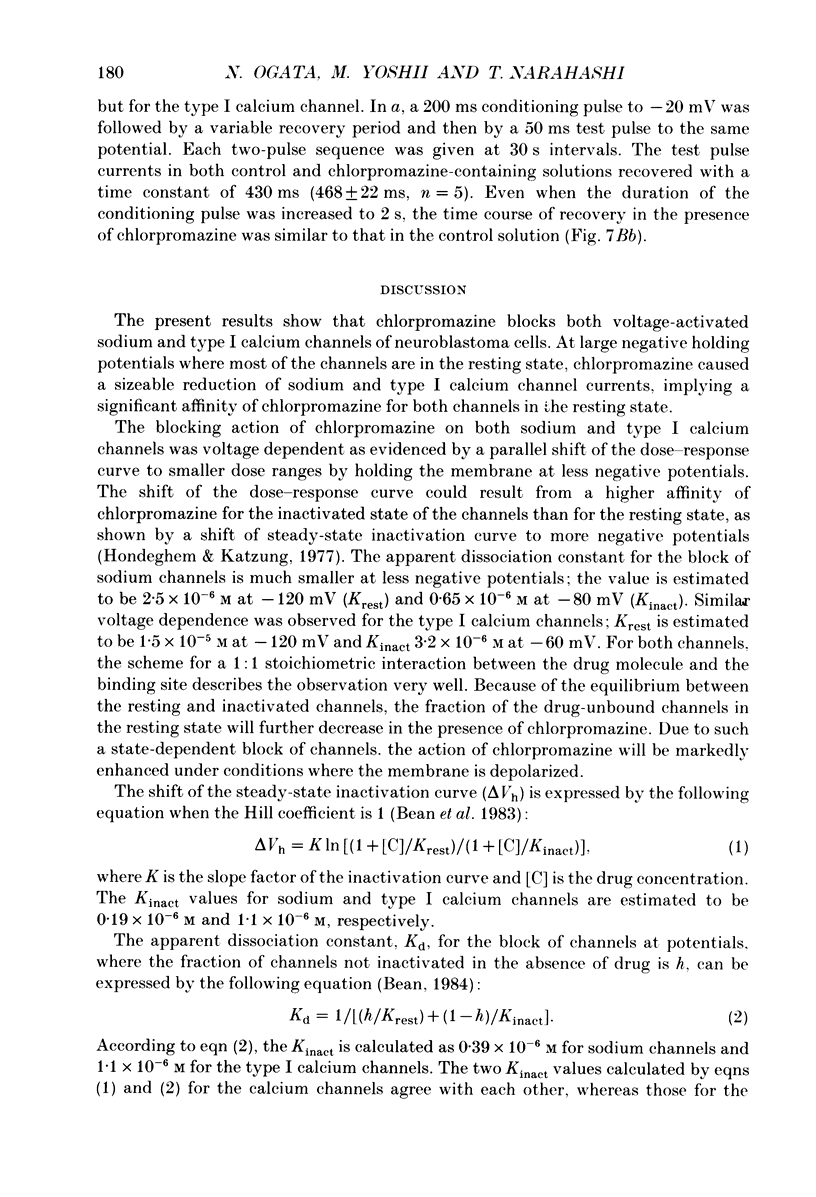
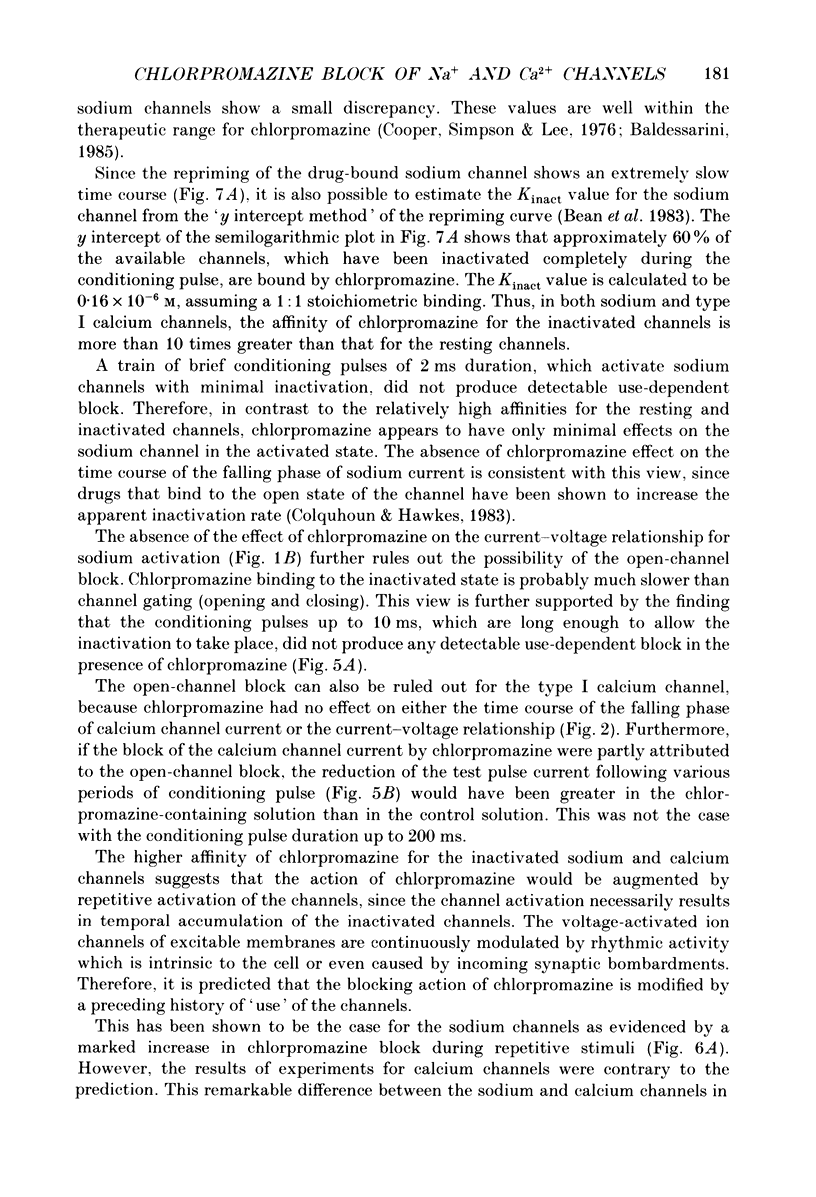
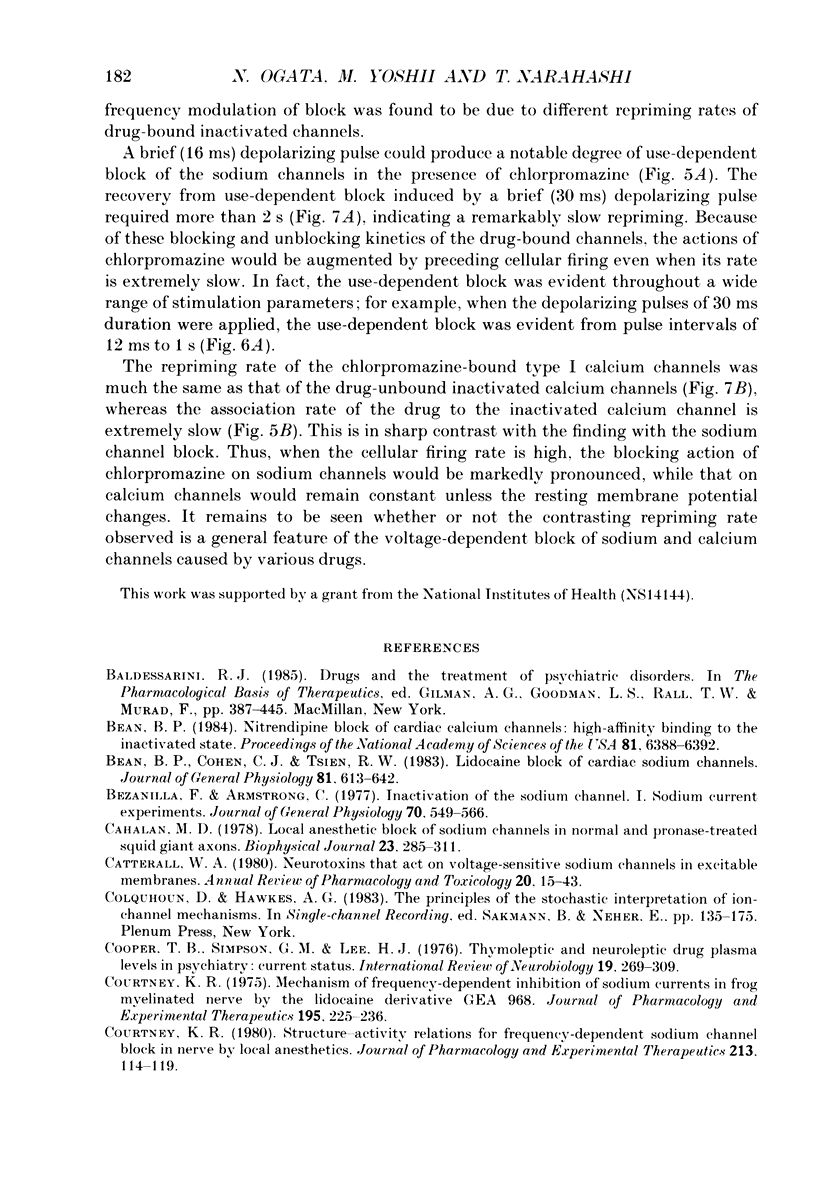
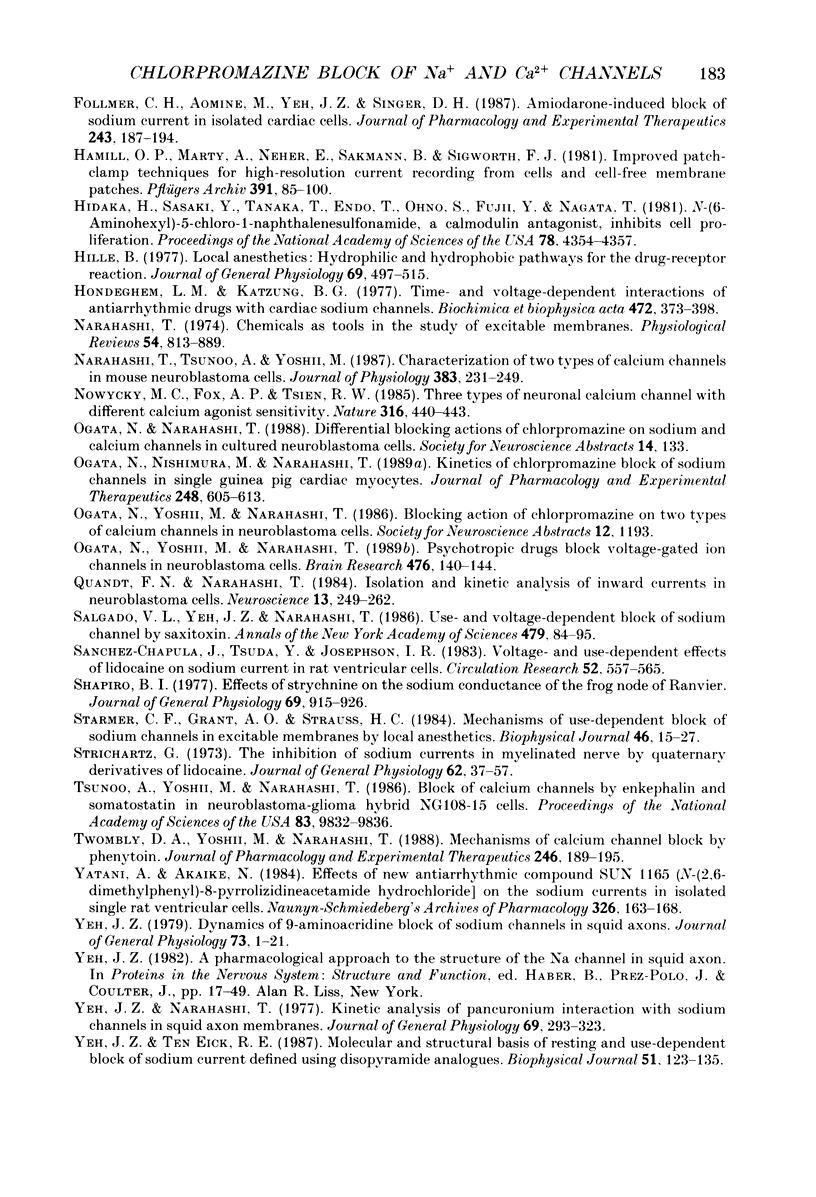
Selected References
These references are in PubMed. This may not be the complete list of references from this article.
- Bean B. P., Cohen C. J., Tsien R. W. Lidocaine block of cardiac sodium channels. J Gen Physiol. 1983 May;81(5):613–642. doi: 10.1085/jgp.81.5.613. [DOI] [PMC free article] [PubMed] [Google Scholar]
- Bean B. P. Nitrendipine block of cardiac calcium channels: high-affinity binding to the inactivated state. Proc Natl Acad Sci U S A. 1984 Oct;81(20):6388–6392. doi: 10.1073/pnas.81.20.6388. [DOI] [PMC free article] [PubMed] [Google Scholar]
- Bezanilla F., Armstrong C. M. Inactivation of the sodium channel. I. Sodium current experiments. J Gen Physiol. 1977 Nov;70(5):549–566. doi: 10.1085/jgp.70.5.549. [DOI] [PMC free article] [PubMed] [Google Scholar]
- Cahalan M. D. Local anesthetic block of sodium channels in normal and pronase-treated squid giant axons. Biophys J. 1978 Aug;23(2):285–311. doi: 10.1016/S0006-3495(78)85449-6. [DOI] [PMC free article] [PubMed] [Google Scholar]
- Catterall W. A. Neurotoxins that act on voltage-sensitive sodium channels in excitable membranes. Annu Rev Pharmacol Toxicol. 1980;20:15–43. doi: 10.1146/annurev.pa.20.040180.000311. [DOI] [PubMed] [Google Scholar]
- Cooper T. B., Simpson G. M., Lee J. H. Thymoleptic and neuroleptic drug plasma levels in psychiatry: current status. Int Rev Neurobiol. 1976;19:269–309. doi: 10.1016/s0074-7742(08)60706-0. [DOI] [PubMed] [Google Scholar]
- Courtney K. R. Structure-activity relations for frequency-dependent sodium channel block in nerve by local anesthetics. J Pharmacol Exp Ther. 1980 Apr;213(1):114–119. [PubMed] [Google Scholar]
- Follmer C. H., Aomine M., Yeh J. Z., Singer D. H. Amiodarone-induced block of sodium current in isolated cardiac cells. J Pharmacol Exp Ther. 1987 Oct;243(1):187–194. [PubMed] [Google Scholar]
- Hamill O. P., Marty A., Neher E., Sakmann B., Sigworth F. J. Improved patch-clamp techniques for high-resolution current recording from cells and cell-free membrane patches. Pflugers Arch. 1981 Aug;391(2):85–100. doi: 10.1007/BF00656997. [DOI] [PubMed] [Google Scholar]
- Hidaka H., Sasaki Y., Tanaka T., Endo T., Ohno S., Fujii Y., Nagata T. N-(6-aminohexyl)-5-chloro-1-naphthalenesulfonamide, a calmodulin antagonist, inhibits cell proliferation. Proc Natl Acad Sci U S A. 1981 Jul;78(7):4354–4357. doi: 10.1073/pnas.78.7.4354. [DOI] [PMC free article] [PubMed] [Google Scholar]
- Hille B. Local anesthetics: hydrophilic and hydrophobic pathways for the drug-receptor reaction. J Gen Physiol. 1977 Apr;69(4):497–515. doi: 10.1085/jgp.69.4.497. [DOI] [PMC free article] [PubMed] [Google Scholar]
- Hondeghem L. M., Katzung B. G. Time- and voltage-dependent interactions of antiarrhythmic drugs with cardiac sodium channels. Biochim Biophys Acta. 1977 Nov 14;472(3-4):373–398. doi: 10.1016/0304-4157(77)90003-x. [DOI] [PubMed] [Google Scholar]
- Narahashi T. Chemicals as tools in the study of excitable membranes. Physiol Rev. 1974 Oct;54(4):813–889. doi: 10.1152/physrev.1974.54.4.813. [DOI] [PubMed] [Google Scholar]
- Narahashi T., Tsunoo A., Yoshii M. Characterization of two types of calcium channels in mouse neuroblastoma cells. J Physiol. 1987 Feb;383:231–249. doi: 10.1113/jphysiol.1987.sp016406. [DOI] [PMC free article] [PubMed] [Google Scholar]
- Nowycky M. C., Fox A. P., Tsien R. W. Three types of neuronal calcium channel with different calcium agonist sensitivity. Nature. 1985 Aug 1;316(6027):440–443. doi: 10.1038/316440a0. [DOI] [PubMed] [Google Scholar]
- Ogata N., Nishimura M., Narahashi T. Kinetics of chlorpromazine block of sodium channels in single guinea pig cardiac myocytes. J Pharmacol Exp Ther. 1989 Feb;248(2):605–613. [PubMed] [Google Scholar]
- Ogata N., Yoshii M., Narahashi T. Psychotropic drugs block voltage-gated ion channels in neuroblastoma cells. Brain Res. 1989 Jan 2;476(1):140–144. doi: 10.1016/0006-8993(89)91546-1. [DOI] [PubMed] [Google Scholar]
- Quandt F. N., Narahashi T. Isolation and kinetic analysis of inward currents in neuroblastoma cells. Neuroscience. 1984 Sep;13(1):249–262. doi: 10.1016/0306-4522(84)90275-6. [DOI] [PubMed] [Google Scholar]
- Salgado V. L., Yeh J. Z., Narahashi T. Use- and voltage-dependent block of the sodium channel by saxitoxin. Ann N Y Acad Sci. 1986;479:84–95. doi: 10.1111/j.1749-6632.1986.tb15563.x. [DOI] [PubMed] [Google Scholar]
- Sanchez-Chapula J., Tsuda Y., Josephson I. R. Voltage- and use-dependent effects of lidocaine on sodium current in rat single ventricular cells. Circ Res. 1983 May;52(5):557–565. doi: 10.1161/01.res.52.5.557. [DOI] [PubMed] [Google Scholar]
- Shapiro B. I. Effects of strychnine on the sodium conductance of the frog node of Ranvier. J Gen Physiol. 1977 Jun;69(6):915–926. doi: 10.1085/jgp.69.6.915. [DOI] [PMC free article] [PubMed] [Google Scholar]
- Starmer C. F., Grant A. O., Strauss H. C. Mechanisms of use-dependent block of sodium channels in excitable membranes by local anesthetics. Biophys J. 1984 Jul;46(1):15–27. doi: 10.1016/S0006-3495(84)83994-6. [DOI] [PMC free article] [PubMed] [Google Scholar]
- Strichartz G. R. The inhibition of sodium currents in myelinated nerve by quaternary derivatives of lidocaine. J Gen Physiol. 1973 Jul;62(1):37–57. doi: 10.1085/jgp.62.1.37. [DOI] [PMC free article] [PubMed] [Google Scholar]
- Tsunoo A., Yoshii M., Narahashi T. Block of calcium channels by enkephalin and somatostatin in neuroblastoma-glioma hybrid NG108-15 cells. Proc Natl Acad Sci U S A. 1986 Dec;83(24):9832–9836. doi: 10.1073/pnas.83.24.9832. [DOI] [PMC free article] [PubMed] [Google Scholar]
- Twombly D. A., Yoshii M., Narahashi T. Mechanisms of calcium channel block by phenytoin. J Pharmacol Exp Ther. 1988 Jul;246(1):189–195. [PubMed] [Google Scholar]
- Yatani A., Akaike N. Effects of a new antiarrhythmic compound SUN 1165 [N-(2,6-dimethylphenyl)-8-pyrrolizidineacetamide hydrochloride] on the sodium currents in isolated single rat ventricular cells. Naunyn Schmiedebergs Arch Pharmacol. 1984 Jun;326(2):163–168. doi: 10.1007/BF00517314. [DOI] [PubMed] [Google Scholar]
- Yeh J. Z. Dynamics of 9-aminoacridine block of sodium channels in squid axons. J Gen Physiol. 1979 Jan;73(1):1–21. doi: 10.1085/jgp.73.1.1. [DOI] [PMC free article] [PubMed] [Google Scholar]
- Yeh J. Z., Narahashi T. Kinetic analysis of pancuronium interaction with sodium channels in squid axon membranes. J Gen Physiol. 1977 Mar;69(3):293–323. doi: 10.1085/jgp.69.3.293. [DOI] [PMC free article] [PubMed] [Google Scholar]
- Yeh J. Z., TenEick R. E. Molecular and structural basis of resting and use-dependent block of sodium current defined using disopyramide analogues. Biophys J. 1987 Jan;51(1):123–135. doi: 10.1016/S0006-3495(87)83317-9. [DOI] [PMC free article] [PubMed] [Google Scholar]