Abstract
1. Current mediated by GABAA receptors was examined in pyramidal cells acutely dissociated from the hippocampus of mature guinea-pigs. Current responses were measured using whole-cell voltage-clamp recordings. An internal perfusion technique was used to change the intracellular contents during recording. 2. Application of GABA (100-300 microM) by short duration pressure pulses produced outward current responses at a holding potential of -10 mV. When recordings were made with intracellular solutions which did not contain Mg-ATP, GABA responses progressively decreased to less than 10% of their initial values after 10 min. This 'run-down' of the GABA response could not be accounted for by desensitization since the rate of run-down was not dependent upon agonist application. 3. The run-down of the GABAA response was reversed when Mg2+ (4 mM) and ATP (2 mM) were introduced into the intracellular perfusate. In addition to the presence of Mg-ATP, buffering of Ca2+ in the intracellular solution to low levels (approximately 10(-8) M) was also necessary to stabilize the GABAA response. 4. The role of a phosphorylation process in regulating the GABAA receptor was tested. After the GABA response stabilized, introduction of alkaline phosphatase (100 micrograms/ml) to the intracellular perfusate caused a complete run-down of the GABA response. 5. Stable GABA responses were obtained when ATP was replaced by ATP-gamma-S (adenosine 5'-O-(thiotriphosphate), an analogue of ATP that donates a thiophosphate group resulting in a product that is more resistant to hydrolysis. Following such treatment GABA responses declined more slowly after the introduction of intracellular alkaline phosphatase. 6. Run-down of GABA responses accelerated when intracellular Ca2+ concentration ([Ca2+]i) was elevated to about 5 x 10(-4) M. The run-down caused by elevated [Ca2+]i could be stopped and reversed by reducing [Ca2+]i to about 10(-8) M. 7. The introduction of ATP-gamma-S to the intracellular medium retarded the run-down of GABA responses caused by elevation of [Ca2+]i. 8. N-(6-Aminohexyl)-5-chloro-1-naphthalenesulphonamide (W-7), a calmodulin inhibitor, reduced the rate of run-down induced by elevated [Ca2+]i. 9. These results suggest that the function of the GABAA receptor is maintained by phosphorylation of the receptor or some closely associated regulatory molecule. Elevation of [Ca2+]i destabilizes the function of the GABAA receptor, probably by activating a Ca2+/calmodulin-dependent phosphatase.
Full text
PDF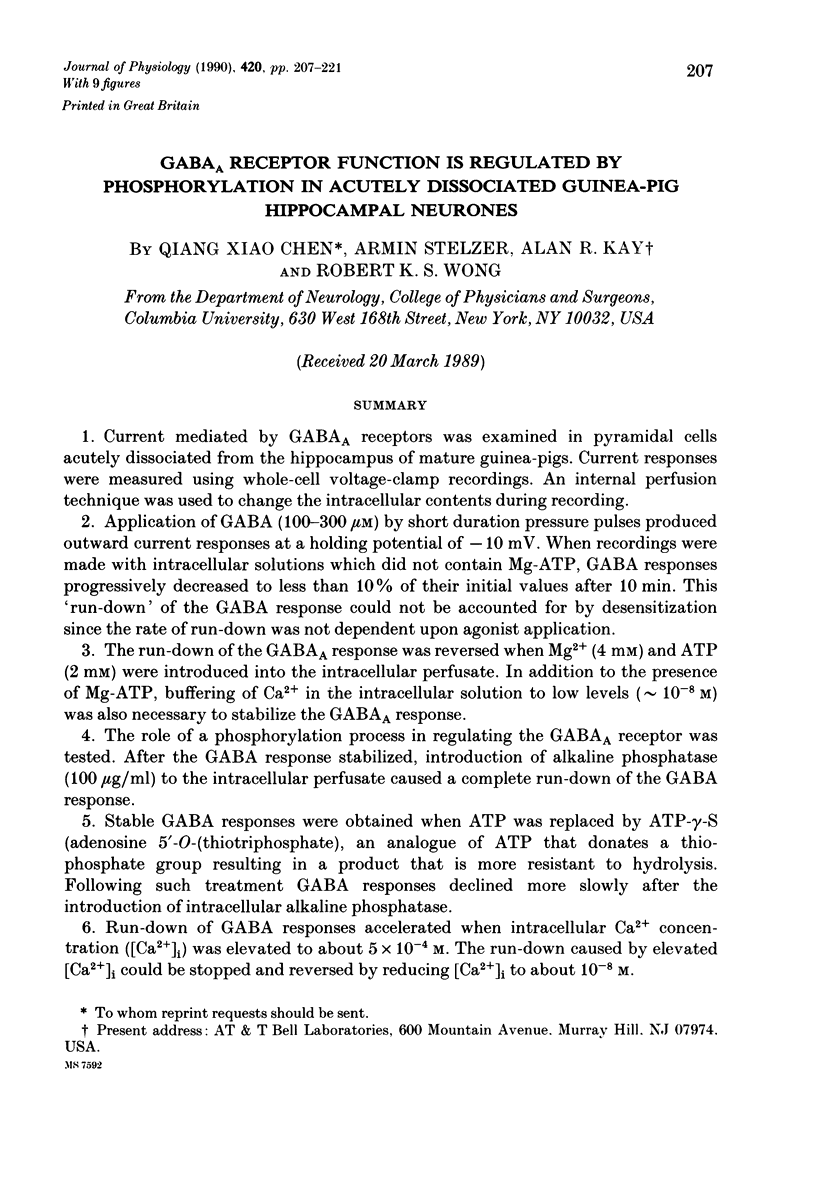
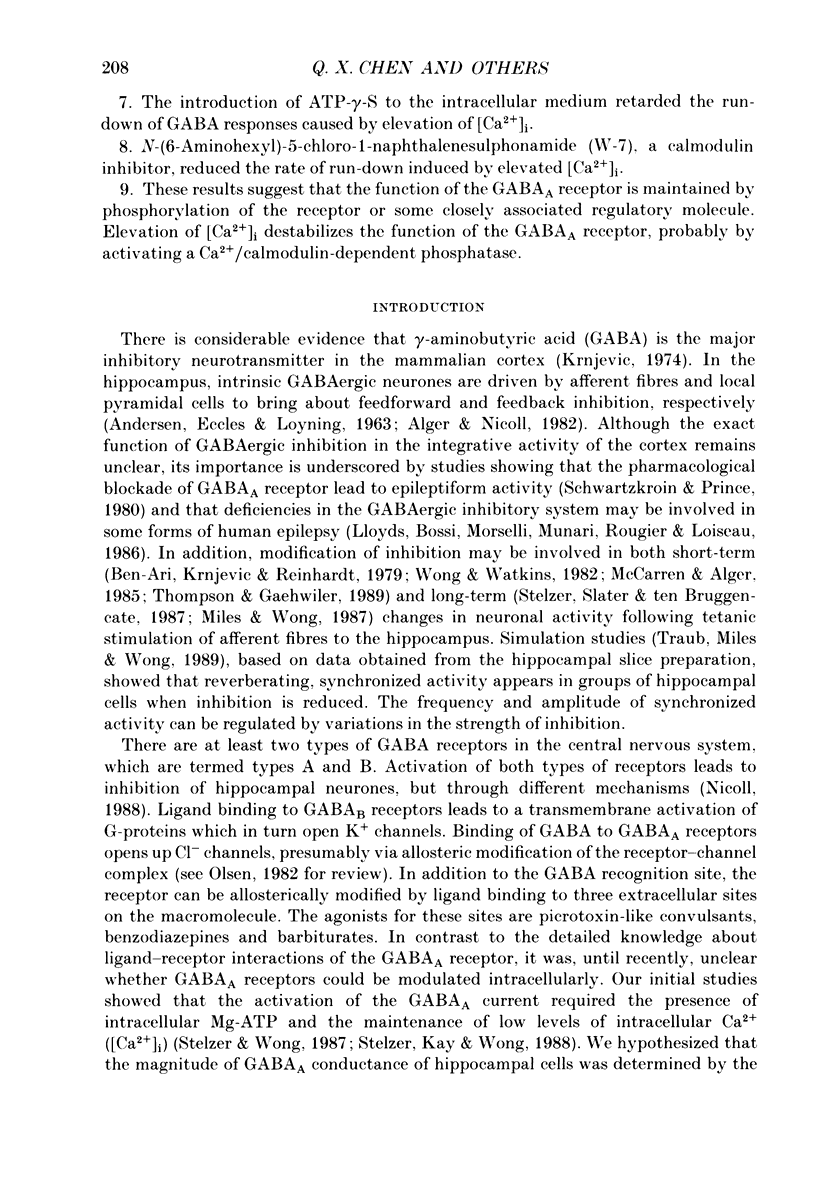
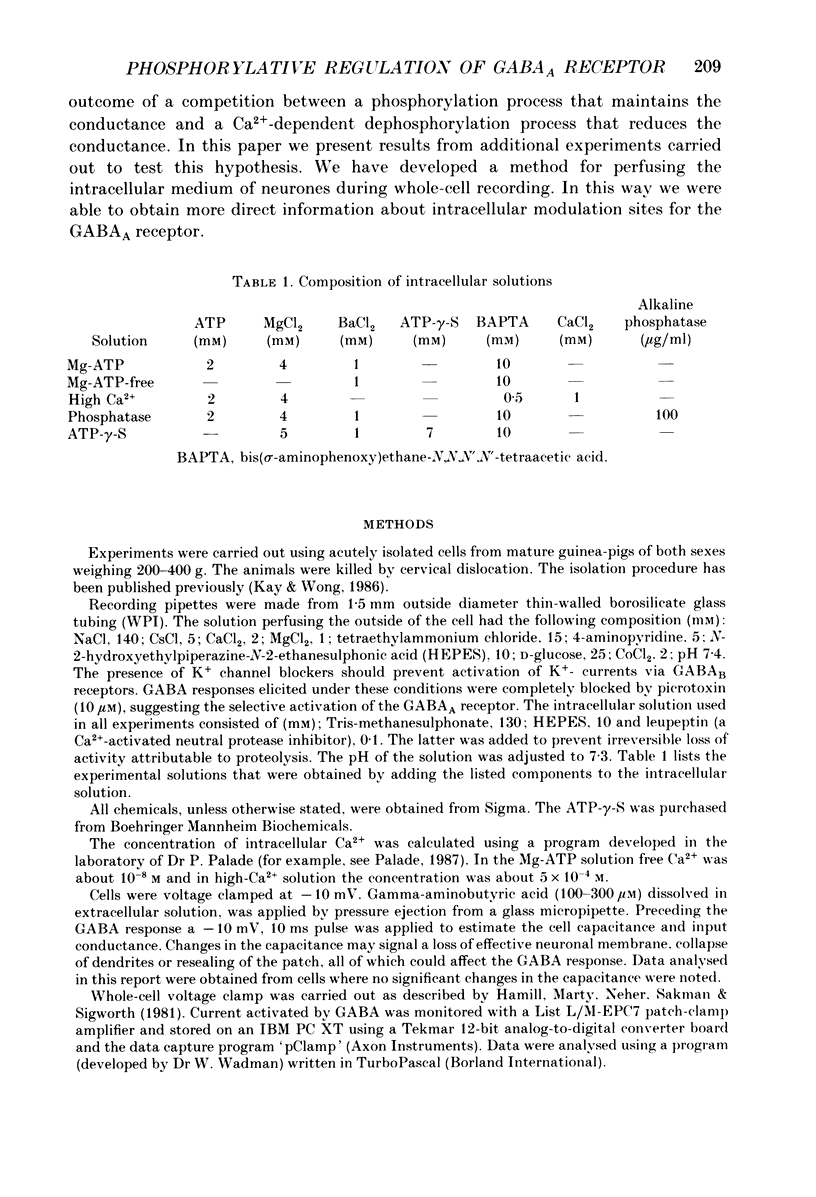
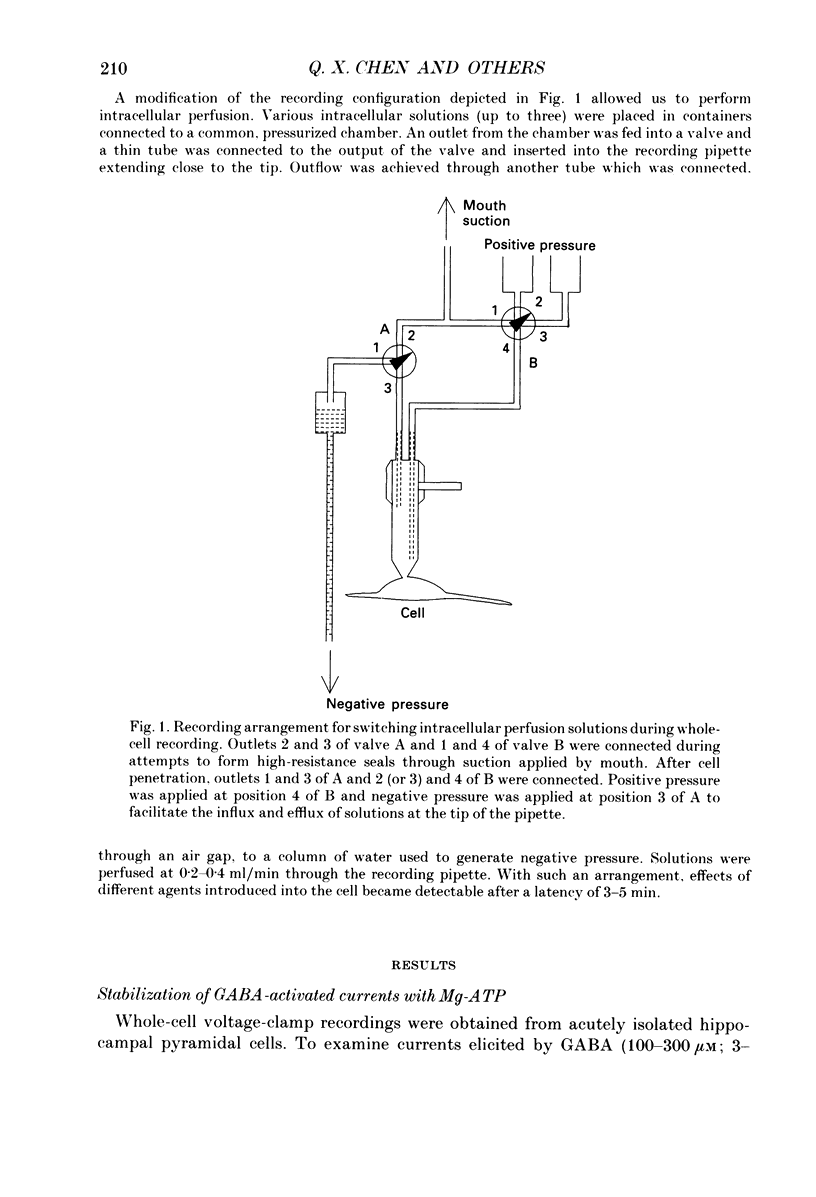
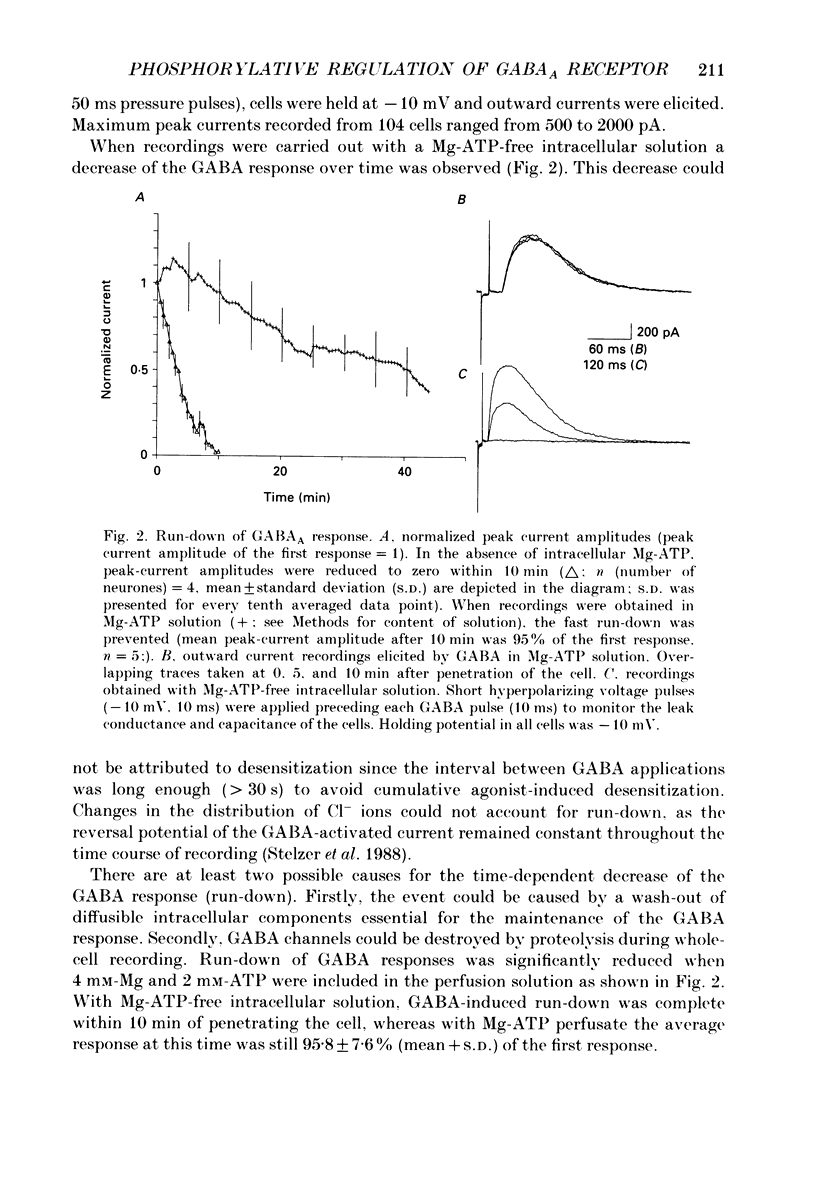
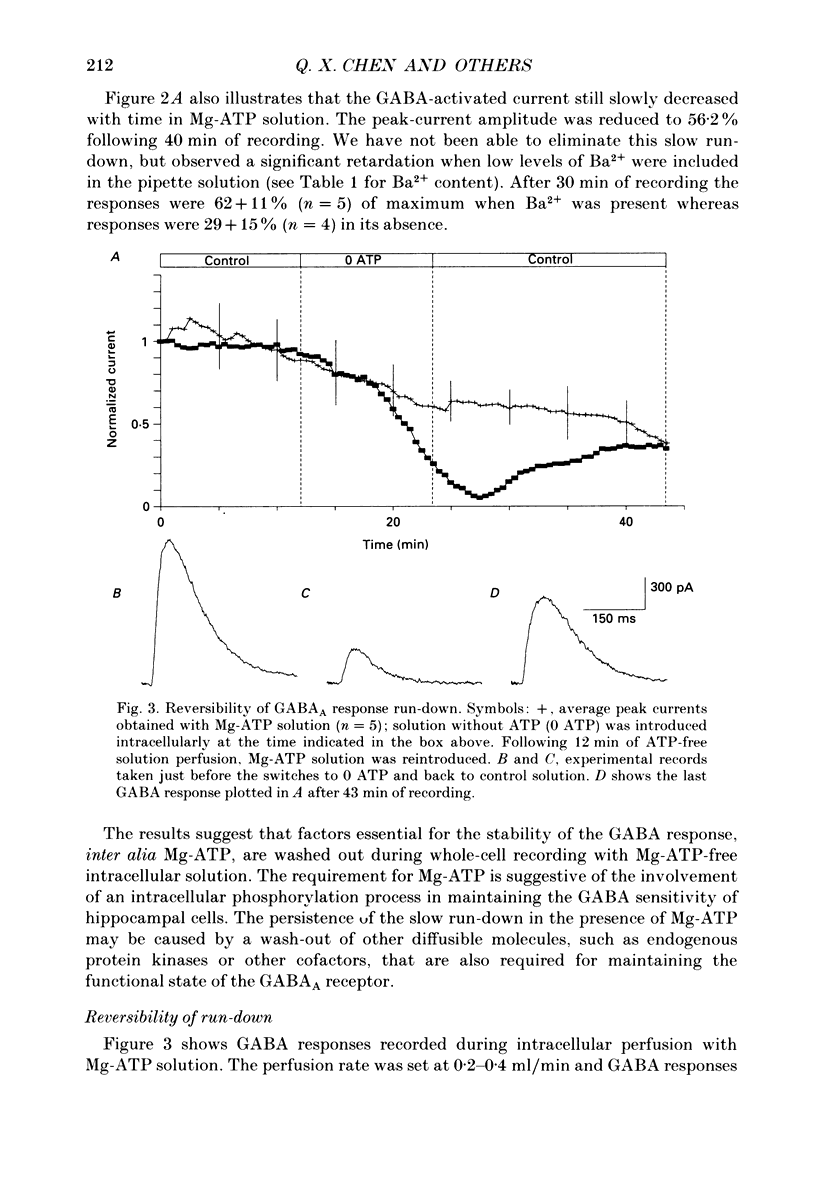
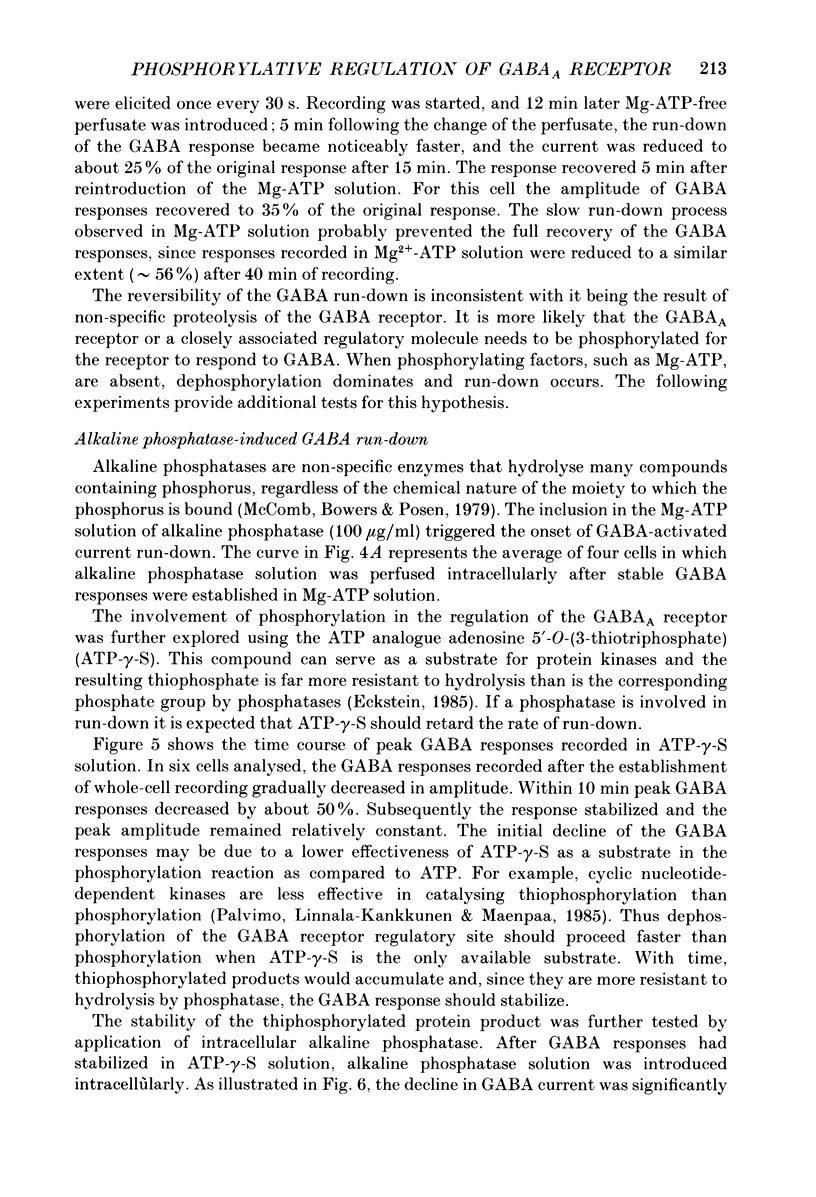
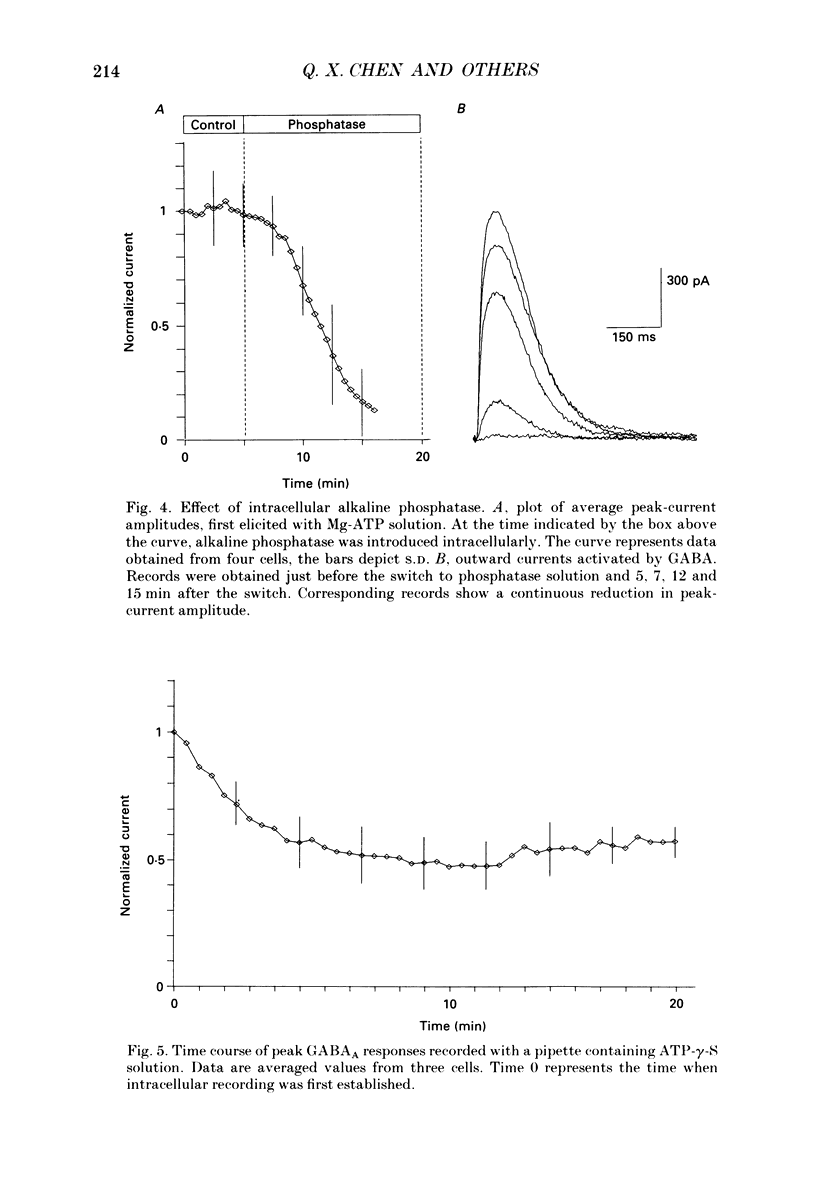
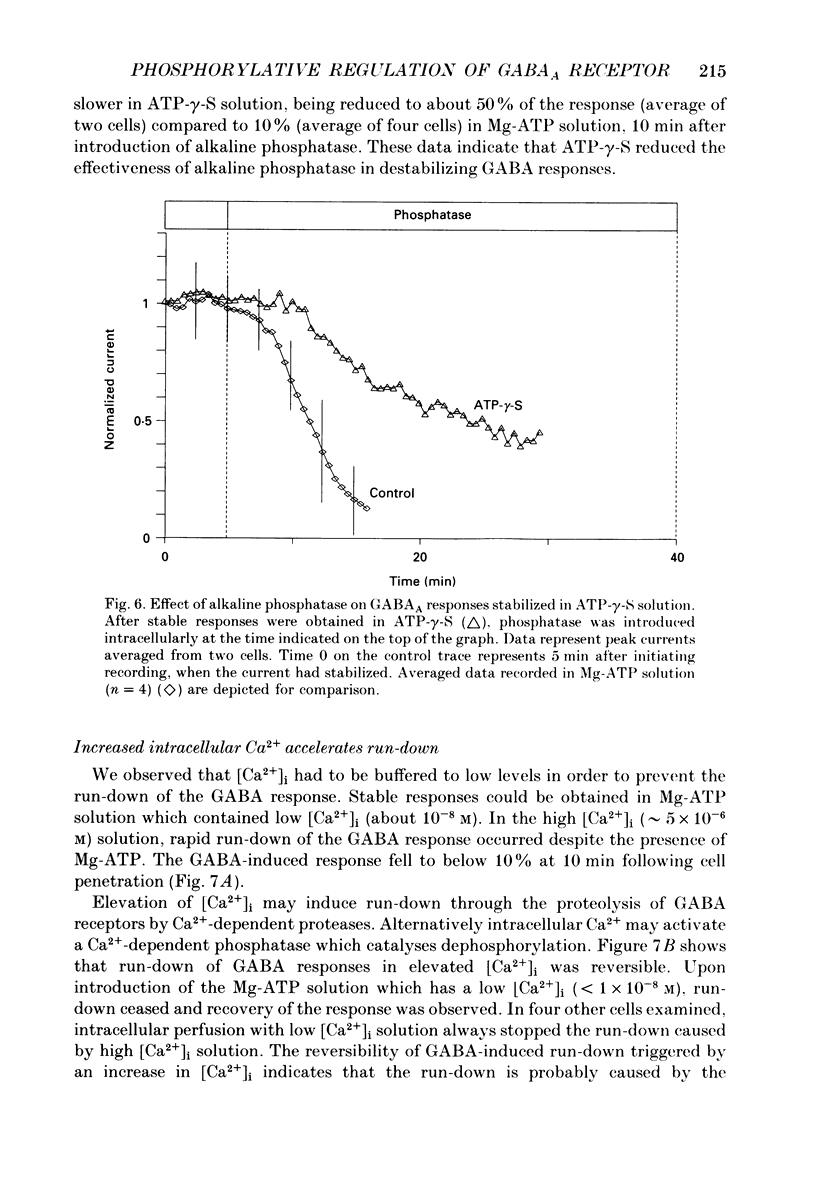
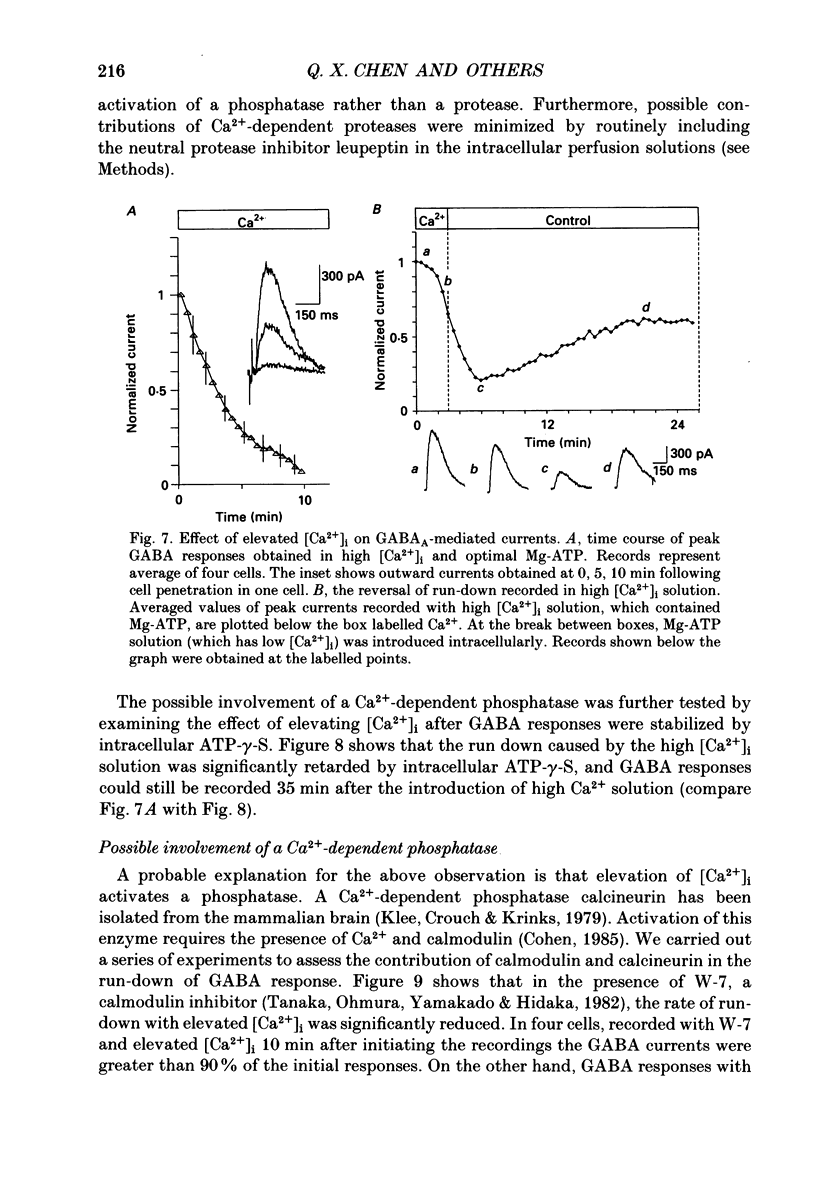
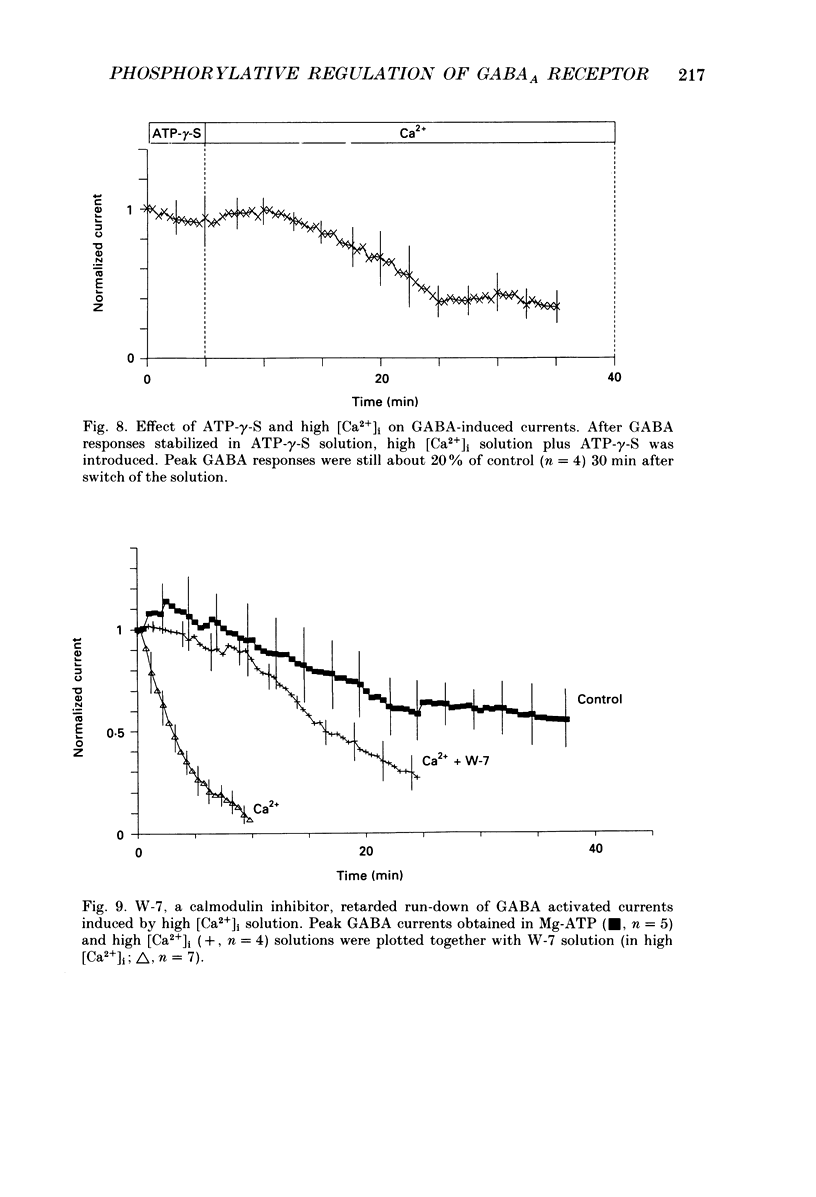
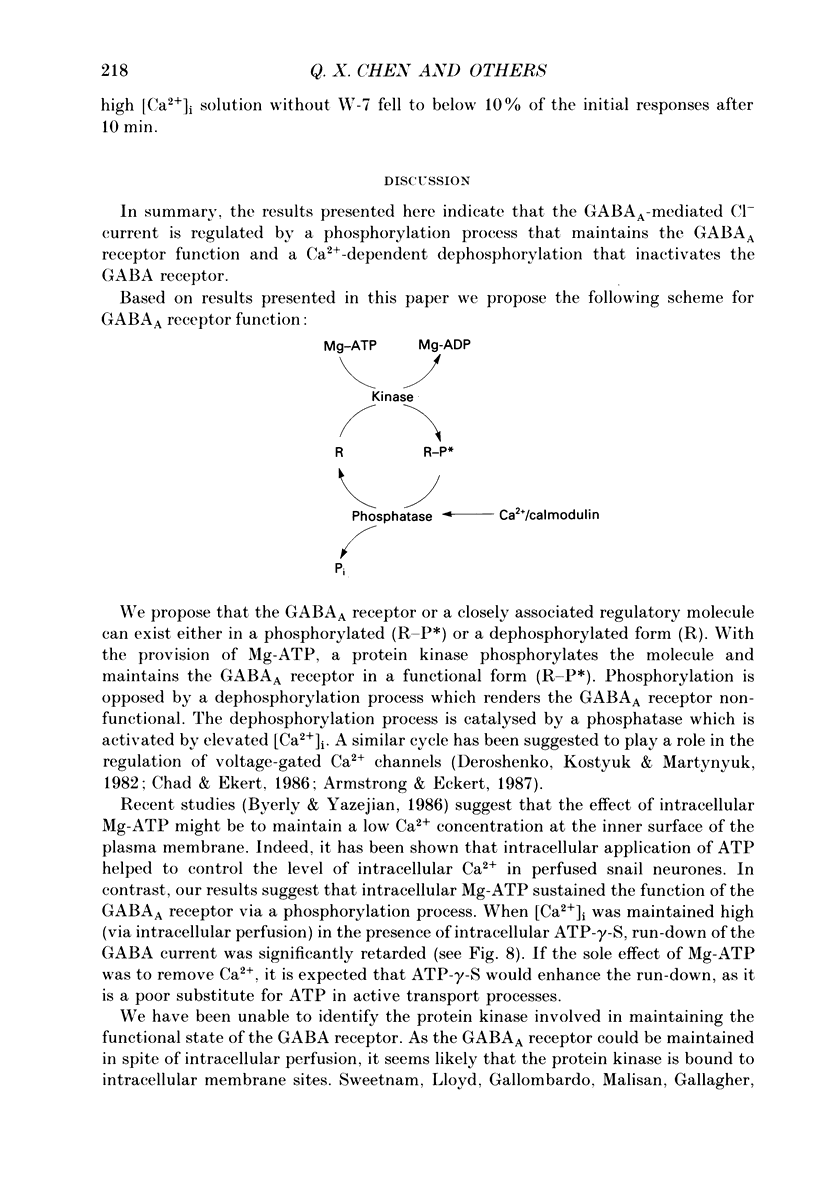
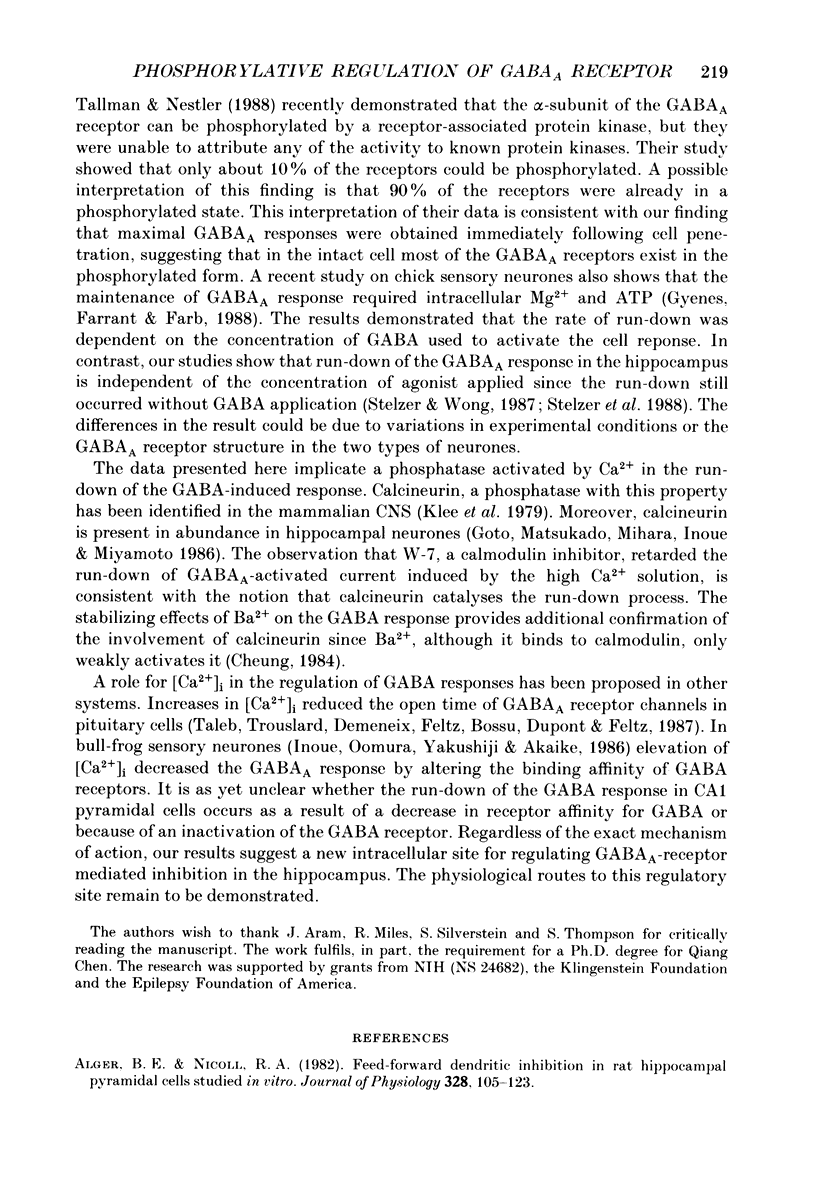
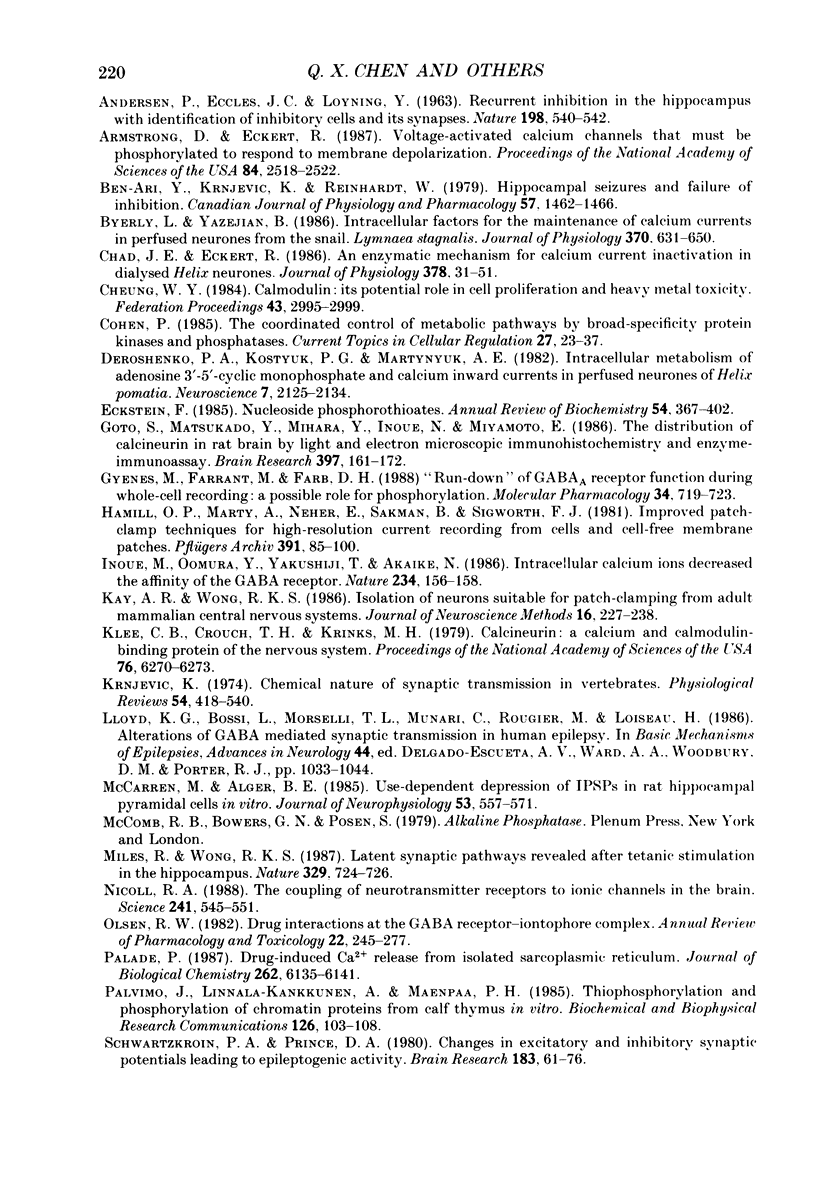
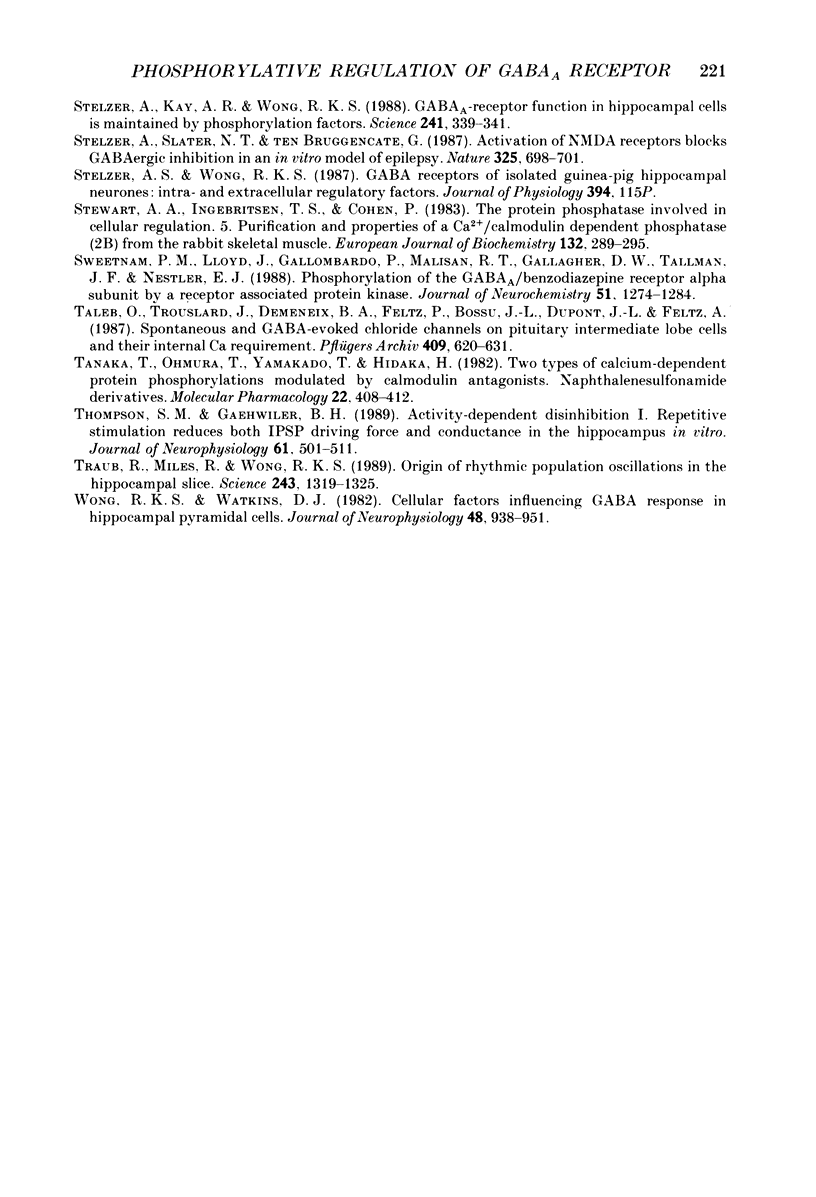
Selected References
These references are in PubMed. This may not be the complete list of references from this article.
- ANDERSEN P., ECCLES J. C., LOYNING Y. Recurrent inhibition in the hippocampus with identification of the inhibitory cell and its synapses. Nature. 1963 May 11;198:540–542. doi: 10.1038/198540a0. [DOI] [PubMed] [Google Scholar]
- Alger B. E., Nicoll R. A. Feed-forward dendritic inhibition in rat hippocampal pyramidal cells studied in vitro. J Physiol. 1982 Jul;328:105–123. doi: 10.1113/jphysiol.1982.sp014255. [DOI] [PMC free article] [PubMed] [Google Scholar]
- Armstrong D., Eckert R. Voltage-activated calcium channels that must be phosphorylated to respond to membrane depolarization. Proc Natl Acad Sci U S A. 1987 Apr;84(8):2518–2522. doi: 10.1073/pnas.84.8.2518. [DOI] [PMC free article] [PubMed] [Google Scholar]
- Byerly L., Yazejian B. Intracellular factors for the maintenance of calcium currents in perfused neurones from the snail, Lymnaea stagnalis. J Physiol. 1986 Jan;370:631–650. doi: 10.1113/jphysiol.1986.sp015955. [DOI] [PMC free article] [PubMed] [Google Scholar]
- Chad J. E., Eckert R. An enzymatic mechanism for calcium current inactivation in dialysed Helix neurones. J Physiol. 1986 Sep;378:31–51. doi: 10.1113/jphysiol.1986.sp016206. [DOI] [PMC free article] [PubMed] [Google Scholar]
- Cheung W. Y. Calmodulin: its potential role in cell proliferation and heavy metal toxicity. Fed Proc. 1984 Dec;43(15):2995–2999. [PubMed] [Google Scholar]
- Cohen P. The coordinated control of metabolic pathways by broad-specificity protein kinases and phosphatases. Curr Top Cell Regul. 1985;27:23–37. doi: 10.1016/b978-0-12-152827-0.50010-4. [DOI] [PubMed] [Google Scholar]
- Doroshenko P. A., Kostyuk P. G., Martynyuk A. E. Intracellular metabolism of adenosine 3',5'-cyclic monophosphate and calcium inward current in perfused neurones of Helix pomatia. Neuroscience. 1982;7(9):2125–2134. doi: 10.1016/0306-4522(82)90124-5. [DOI] [PubMed] [Google Scholar]
- Eckstein F. Nucleoside phosphorothioates. Annu Rev Biochem. 1985;54:367–402. doi: 10.1146/annurev.bi.54.070185.002055. [DOI] [PubMed] [Google Scholar]
- Goto S., Matsukado Y., Mihara Y., Inoue N., Miyamoto E. The distribution of calcineurin in rat brain by light and electron microscopic immunohistochemistry and enzyme-immunoassay. Brain Res. 1986 Nov 5;397(1):161–172. doi: 10.1016/0006-8993(86)91381-8. [DOI] [PubMed] [Google Scholar]
- Gyenes M., Farrant M., Farb D. H. "Run-down" of gamma-aminobutyric acidA receptor function during whole-cell recording: a possible role for phosphorylation. Mol Pharmacol. 1988 Dec;34(6):719–723. [PubMed] [Google Scholar]
- Hamill O. P., Marty A., Neher E., Sakmann B., Sigworth F. J. Improved patch-clamp techniques for high-resolution current recording from cells and cell-free membrane patches. Pflugers Arch. 1981 Aug;391(2):85–100. doi: 10.1007/BF00656997. [DOI] [PubMed] [Google Scholar]
- Inoue M., Oomura Y., Yakushiji T., Akaike N. Intracellular calcium ions decrease the affinity of the GABA receptor. Nature. 1986 Nov 13;324(6093):156–158. doi: 10.1038/324156a0. [DOI] [PubMed] [Google Scholar]
- Kay A. R., Wong R. K. Isolation of neurons suitable for patch-clamping from adult mammalian central nervous systems. J Neurosci Methods. 1986 May;16(3):227–238. doi: 10.1016/0165-0270(86)90040-3. [DOI] [PubMed] [Google Scholar]
- Klee C. B., Crouch T. H., Krinks M. H. Calcineurin: a calcium- and calmodulin-binding protein of the nervous system. Proc Natl Acad Sci U S A. 1979 Dec;76(12):6270–6273. doi: 10.1073/pnas.76.12.6270. [DOI] [PMC free article] [PubMed] [Google Scholar]
- Lloyd K. G., Bossi L., Morselli P. L., Munari C., Rougier M., Loiseau H. Alterations of GABA-mediated synaptic transmission in human epilepsy. Adv Neurol. 1986;44:1033–1044. [PubMed] [Google Scholar]
- McCarren M., Alger B. E. Use-dependent depression of IPSPs in rat hippocampal pyramidal cells in vitro. J Neurophysiol. 1985 Feb;53(2):557–571. doi: 10.1152/jn.1985.53.2.557. [DOI] [PubMed] [Google Scholar]
- Miles R., Wong R. K. Latent synaptic pathways revealed after tetanic stimulation in the hippocampus. Nature. 1987 Oct 22;329(6141):724–726. doi: 10.1038/329724a0. [DOI] [PubMed] [Google Scholar]
- Nicoll R. A. The coupling of neurotransmitter receptors to ion channels in the brain. Science. 1988 Jul 29;241(4865):545–551. doi: 10.1126/science.2456612. [DOI] [PubMed] [Google Scholar]
- Olsen R. W. Drug interactions at the GABA receptor-ionophore complex. Annu Rev Pharmacol Toxicol. 1982;22:245–277. doi: 10.1146/annurev.pa.22.040182.001333. [DOI] [PubMed] [Google Scholar]
- Palade P. Drug-induced Ca2+ release from isolated sarcoplasmic reticulum. I. Use of pyrophosphate to study caffeine-induced Ca2+ release. J Biol Chem. 1987 May 5;262(13):6135–6141. [PubMed] [Google Scholar]
- Palvimo J., Linnala-Kankkunen A., Mäenpä P. H. Thiophosphorylation and phosphorylation of chromatin proteins from calf thymus in vitro. Biochem Biophys Res Commun. 1985 Jan 16;126(1):103–108. doi: 10.1016/0006-291x(85)90577-7. [DOI] [PubMed] [Google Scholar]
- Schwartzkroin P. A., Prince D. A. Changes in excitatory and inhibitory synaptic potentials leading to epileptogenic activity. Brain Res. 1980 Feb 3;183(1):61–76. doi: 10.1016/0006-8993(80)90119-5. [DOI] [PubMed] [Google Scholar]
- Stelzer A., Kay A. R., Wong R. K. GABAA-receptor function in hippocampal cells is maintained by phosphorylation factors. Science. 1988 Jul 15;241(4863):339–341. doi: 10.1126/science.2455347. [DOI] [PubMed] [Google Scholar]
- Stewart A. A., Ingebritsen T. S., Cohen P. The protein phosphatases involved in cellular regulation. 5. Purification and properties of a Ca2+/calmodulin-dependent protein phosphatase (2B) from rabbit skeletal muscle. Eur J Biochem. 1983 May 2;132(2):289–295. doi: 10.1111/j.1432-1033.1983.tb07361.x. [DOI] [PubMed] [Google Scholar]
- Sweetnam P. M., Lloyd J., Gallombardo P., Malison R. T., Gallager D. W., Tallman J. F., Nestler E. J. Phosphorylation of the GABAa/benzodiazepine receptor alpha subunit by a receptor-associated protein kinase. J Neurochem. 1988 Oct;51(4):1274–1284. doi: 10.1111/j.1471-4159.1988.tb03097.x. [DOI] [PubMed] [Google Scholar]
- Taleb O., Trouslard J., Demeneix B. A., Feltz P., Bossu J. L., Dupont J. L., Feltz A. Spontaneous and GABA-evoked chloride channels on pituitary intermediate lobe cells and their internal Ca requirements. Pflugers Arch. 1987 Aug;409(6):620–631. doi: 10.1007/BF00584663. [DOI] [PubMed] [Google Scholar]
- Tanaka T., Ohmura T., Yamakado T., Hidaka H. Two types of calcium-dependent protein phosphorylations modulated by calmodulin antagonists. Naphthalenesulfonamide derivatives. Mol Pharmacol. 1982 Sep;22(2):408–412. [PubMed] [Google Scholar]
- Thompson S. M., Gähwiler B. H. Activity-dependent disinhibition. I. Repetitive stimulation reduces IPSP driving force and conductance in the hippocampus in vitro. J Neurophysiol. 1989 Mar;61(3):501–511. doi: 10.1152/jn.1989.61.3.501. [DOI] [PubMed] [Google Scholar]
- Traub R. D., Miles R., Wong R. K. Model of the origin of rhythmic population oscillations in the hippocampal slice. Science. 1989 Mar 10;243(4896):1319–1325. doi: 10.1126/science.2646715. [DOI] [PubMed] [Google Scholar]
- Wong R. K., Watkins D. J. Cellular factors influencing GABA response in hippocampal pyramidal cells. J Neurophysiol. 1982 Oct;48(4):938–951. doi: 10.1152/jn.1982.48.4.938. [DOI] [PubMed] [Google Scholar]