Abstract
1. Inner hair cells were mechanically isolated from the apical, low-frequency region of the guinea-pig cochlea and maintained by superfusion with tissue-culture medium. Membrane currents were studied under voltage clamp, using the whole-cell recording mode of the patch-clamp technique. 2. The cells were studied mostly at 35-38 degrees C to obtain realistic kinetics of the currents, relevant to the functioning of these cells in vivo. 3. Isolated inner hair cells had resting potentials of about -65 mV. Depolarizing voltage steps from a holding potential of about -80 mV resulted in large time- and voltage-dependent outward currents. Hyperpolarizing voltage steps from the same holding potential only showed a small leakage conductance of 0.5-2.5 nS. 4. On repolarization to different membrane potentials, the tail currents reversed around -75 mV. This indicates that the outward currents were mainly carried by potassium ions. 5. Pharmacological dissection of the currents provided evidence for two different potassium conductances. The largest conductance had extremely fast kinetics. Its principal time constant of activation was about 0.15-0.35 ms, the faster values being obtained for larger depolarizations. This fast potassium conductance was blocked by 25 mM-tetraethylammonium chloride in the bath. 6. A smaller, slow potassium conductance, with principal time constants of activation of 2-10 ms (speeding up with depolarization), was blocked by 10-15 mM-4-aminopyridine in the patch pipette. 7. Both potassium conductances were activated over the membrane potential range of about -60 to -20 mV. This is approximately the same as the range of the receptor potential measured in vivo. Therefore these conductances should influence the properties of the receptor potential in inner hair cells. 8. Current injection experiments showed two main effects of the potassium conductances: (a) a non-linearity in the voltage-current relationships; (b) a strongly damped oscillation of the membrane potential in response to a large step of outward current. This oscillatory behaviour is caused by the fast potassium conductance.
Full text
PDF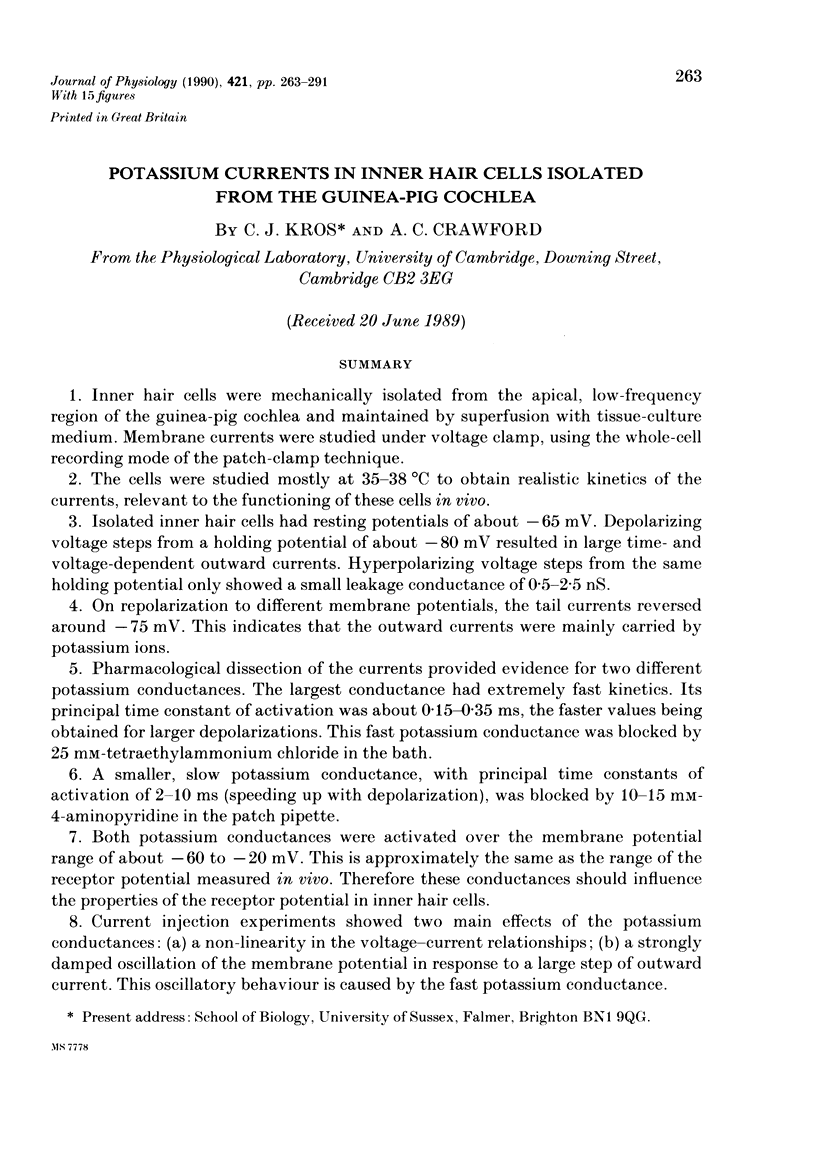
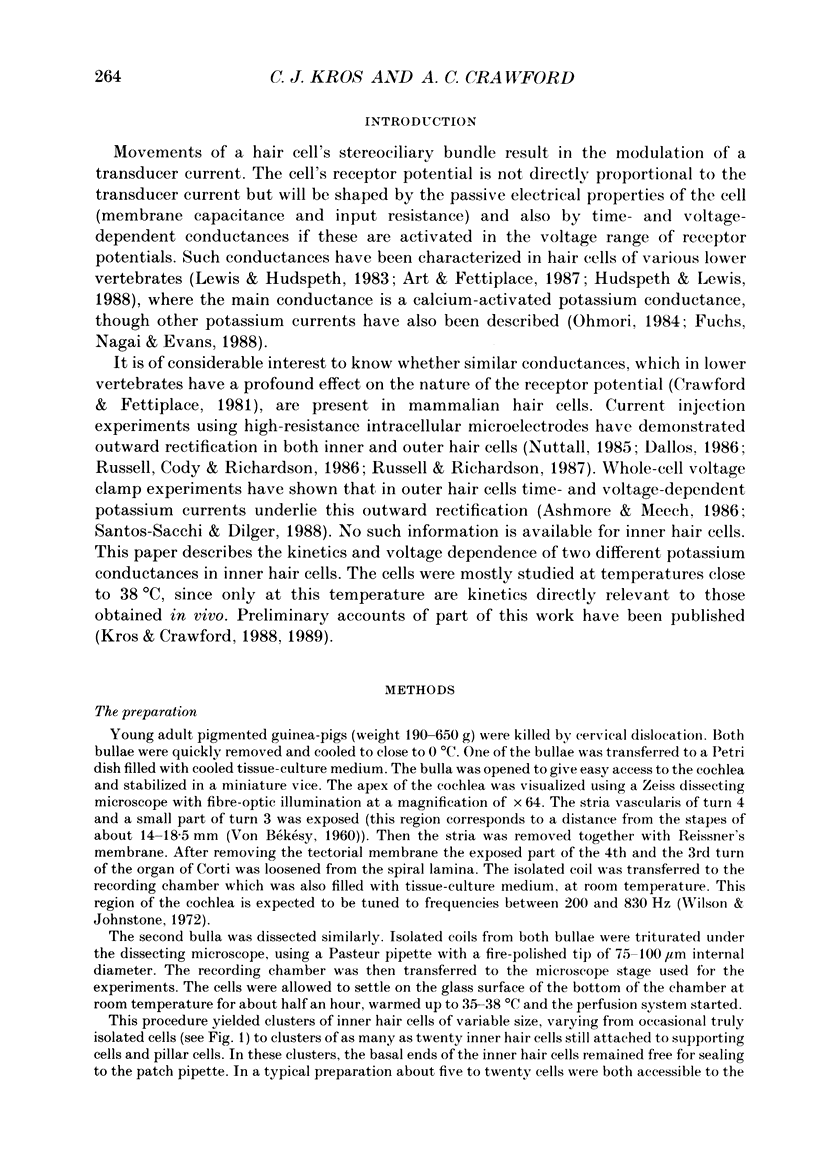
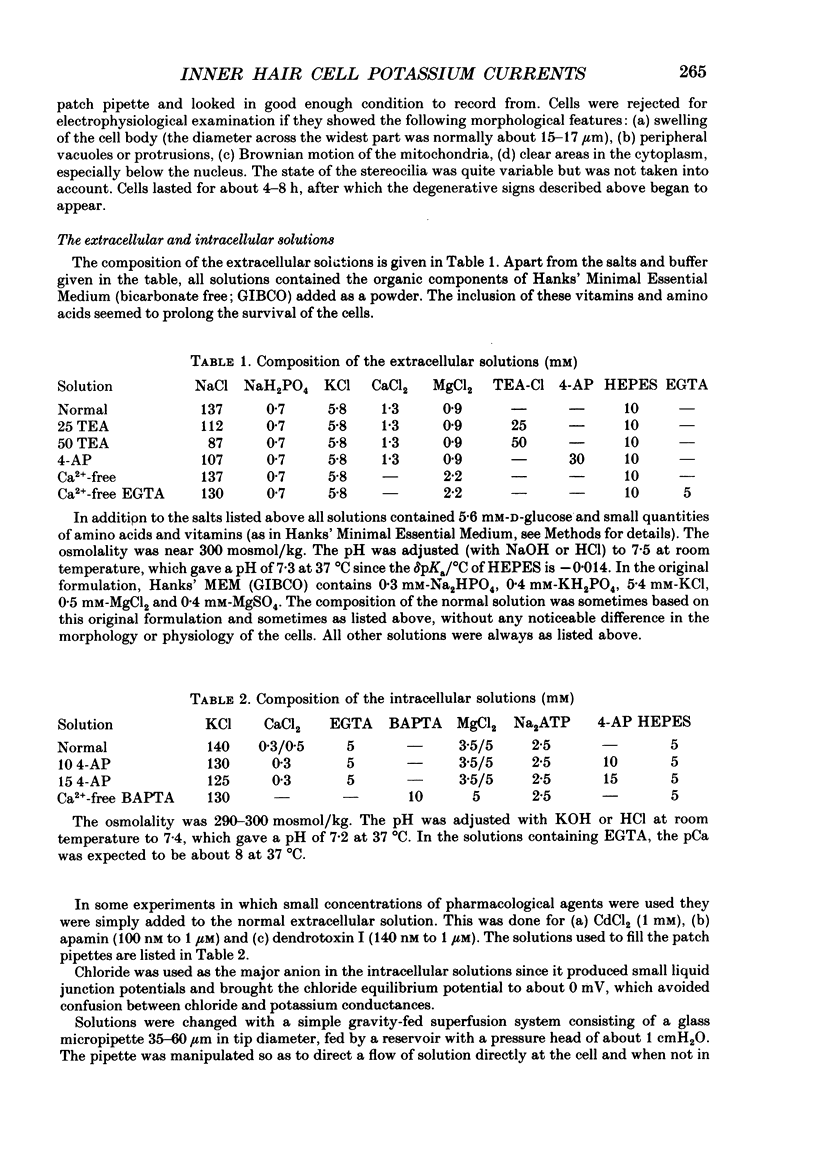
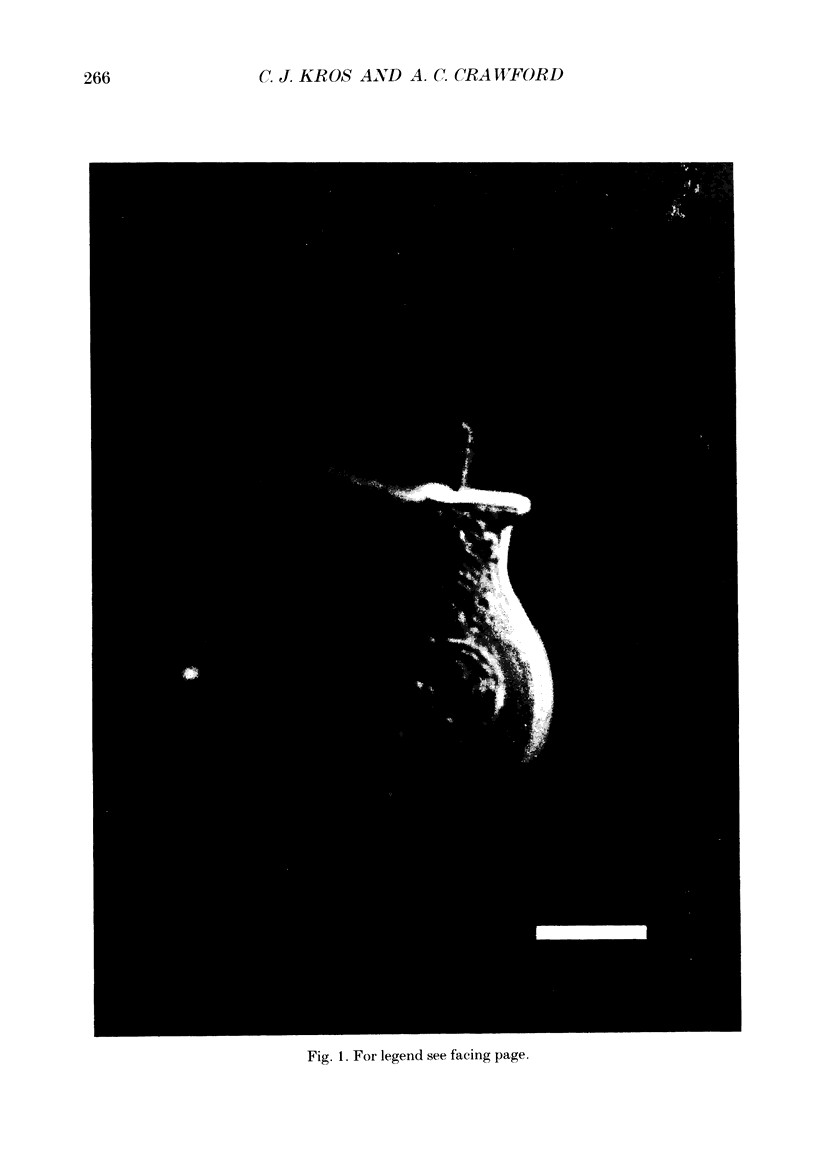
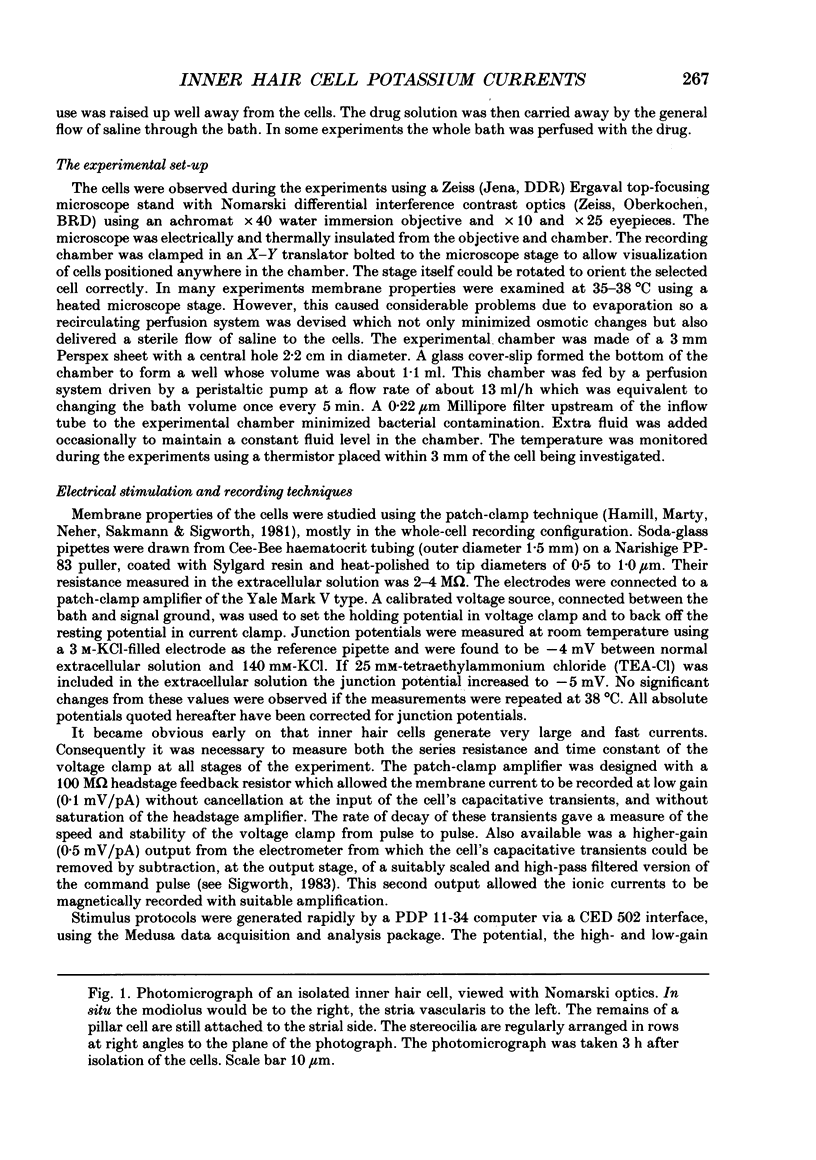
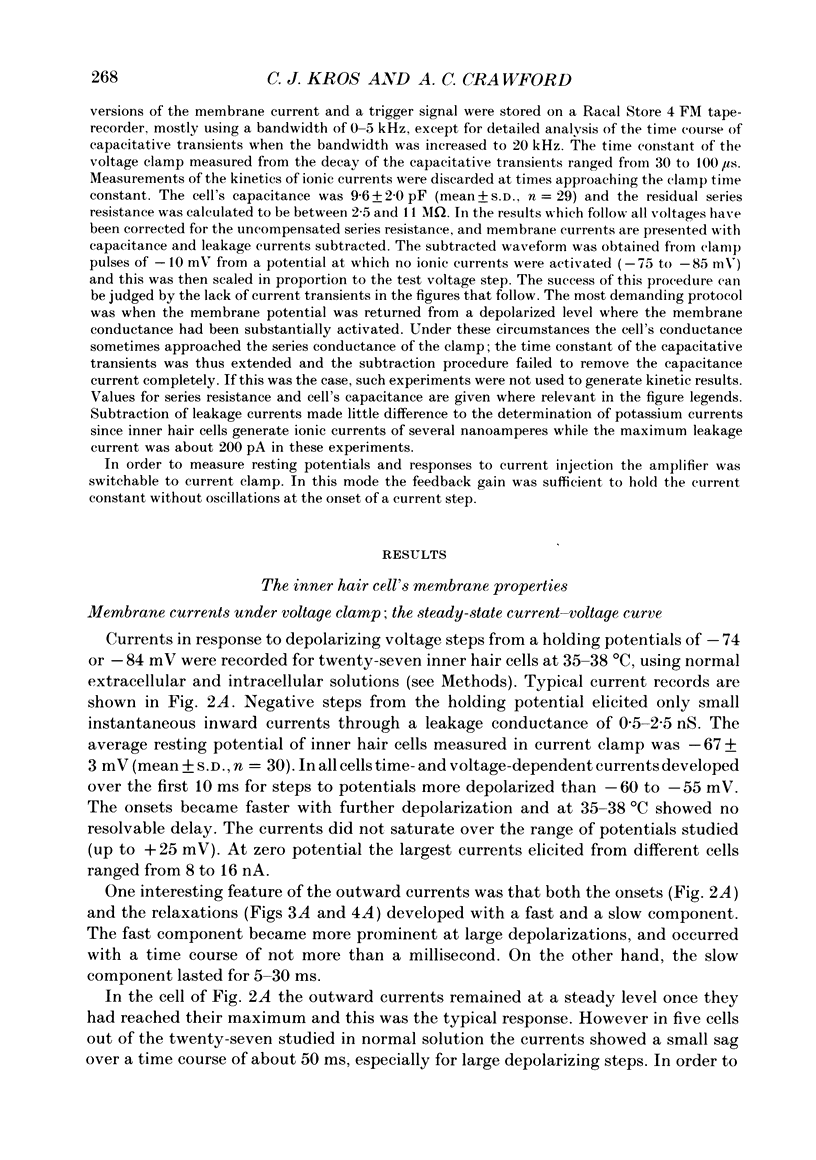
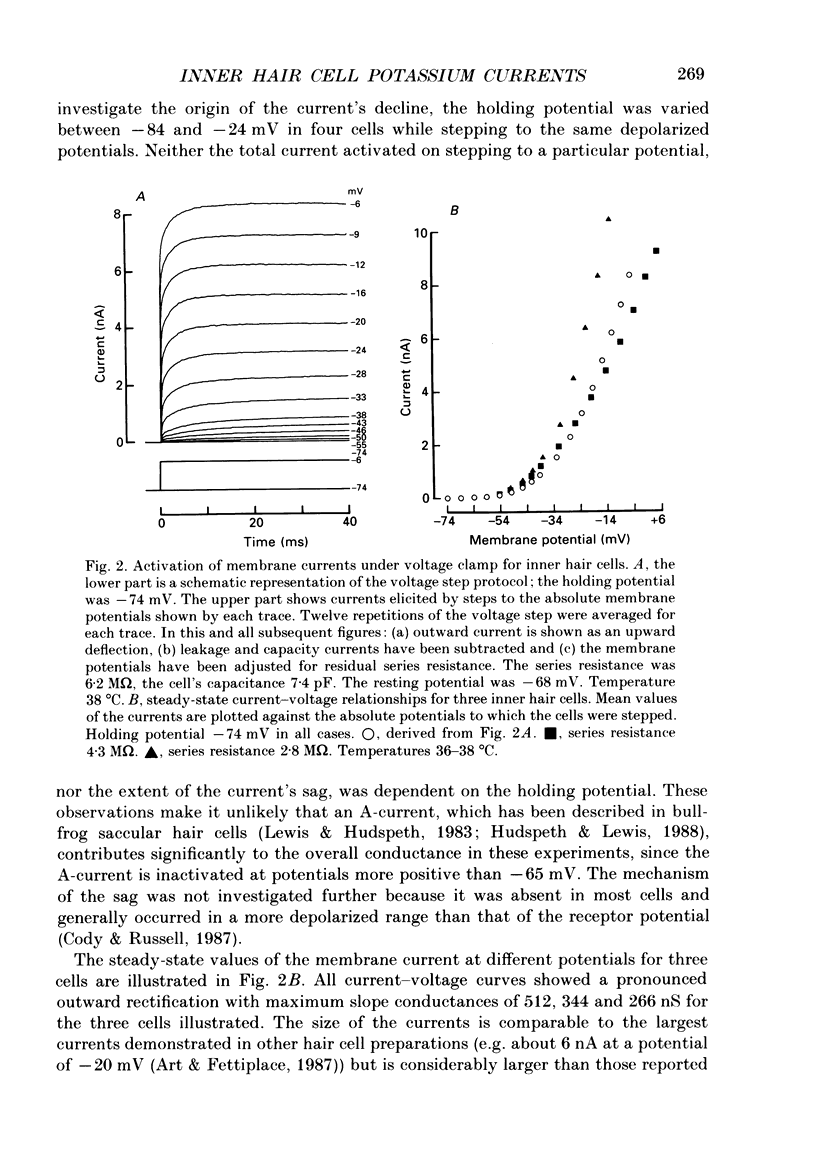
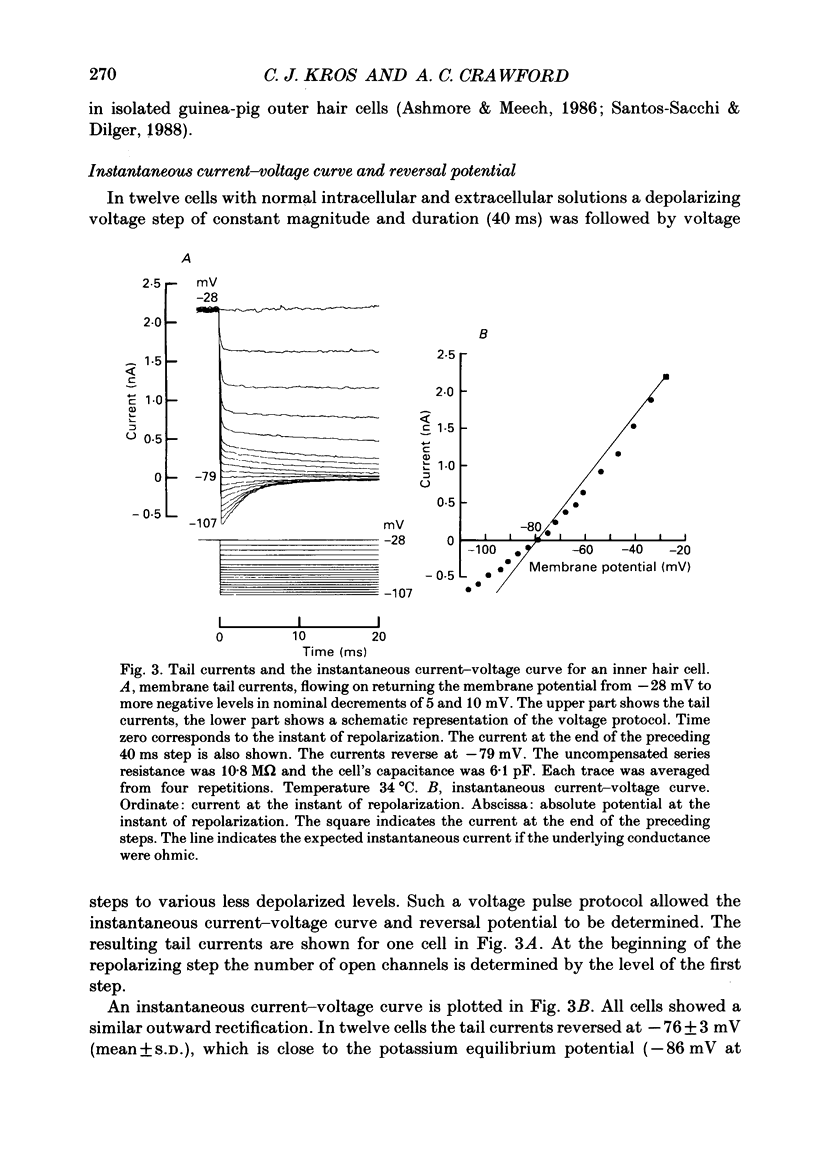
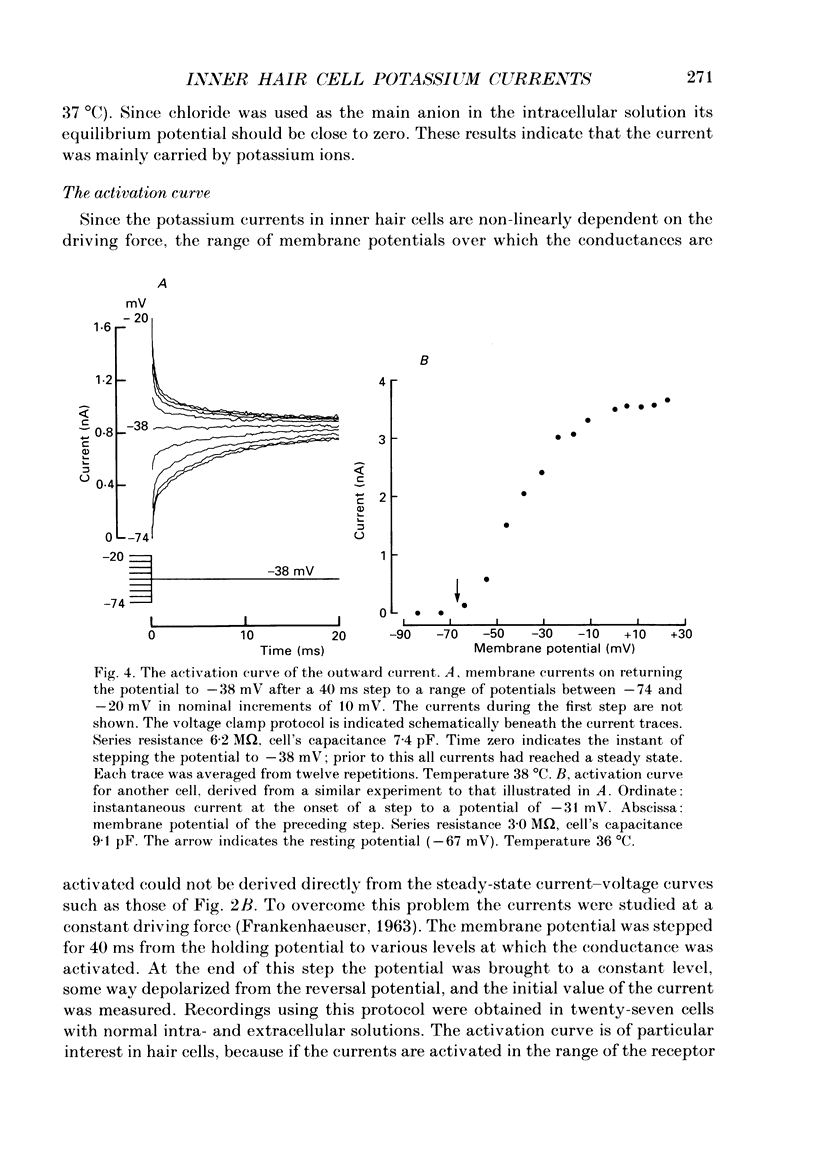
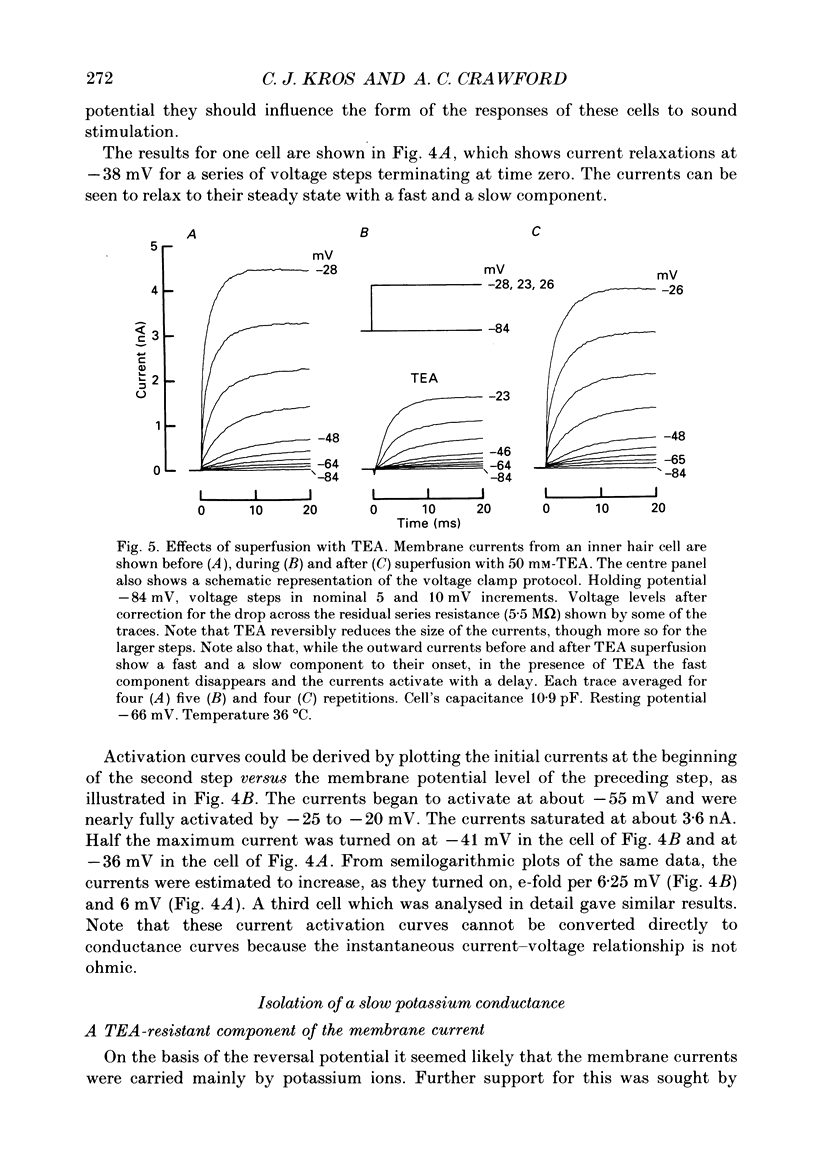
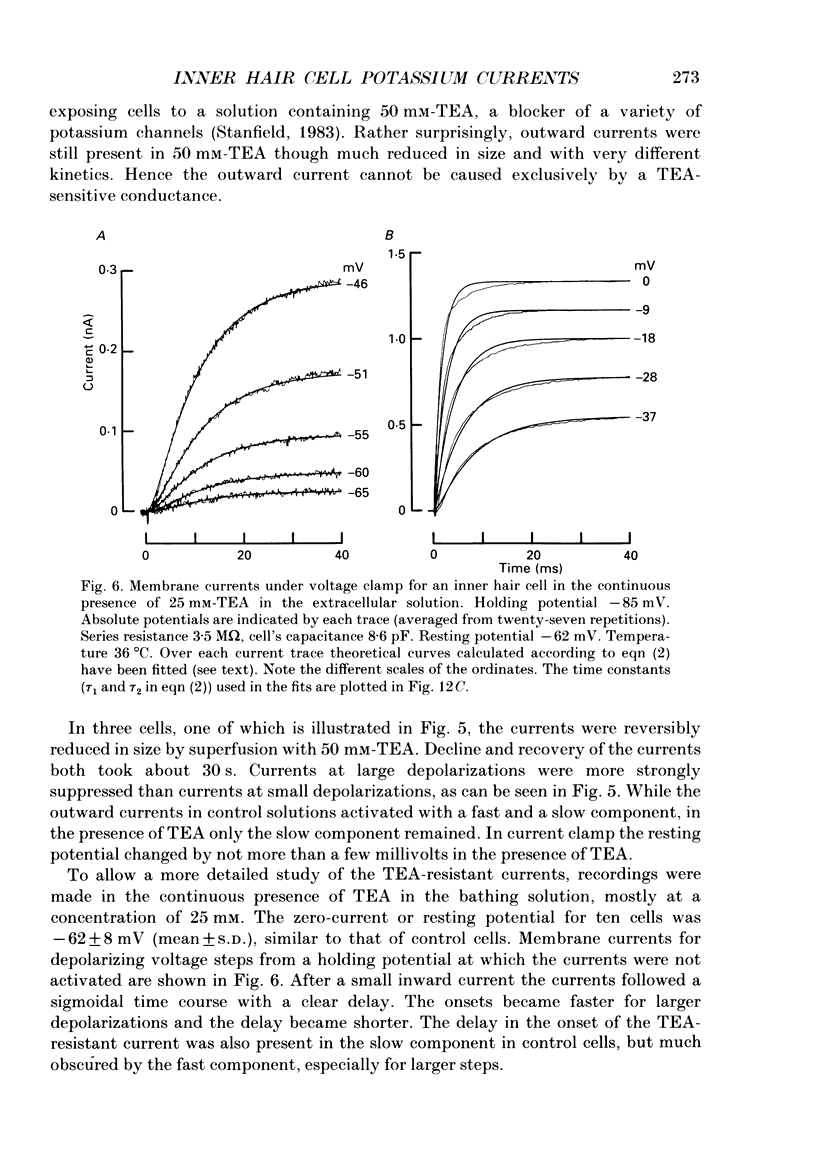
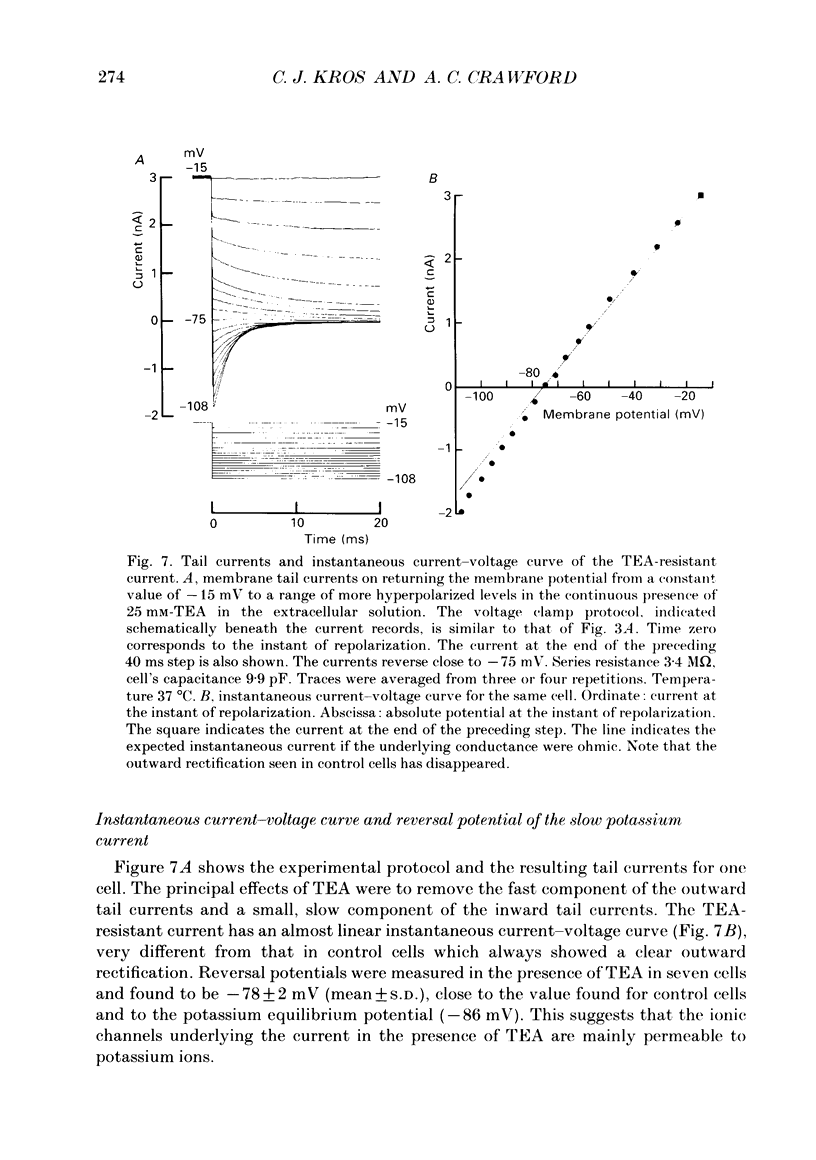
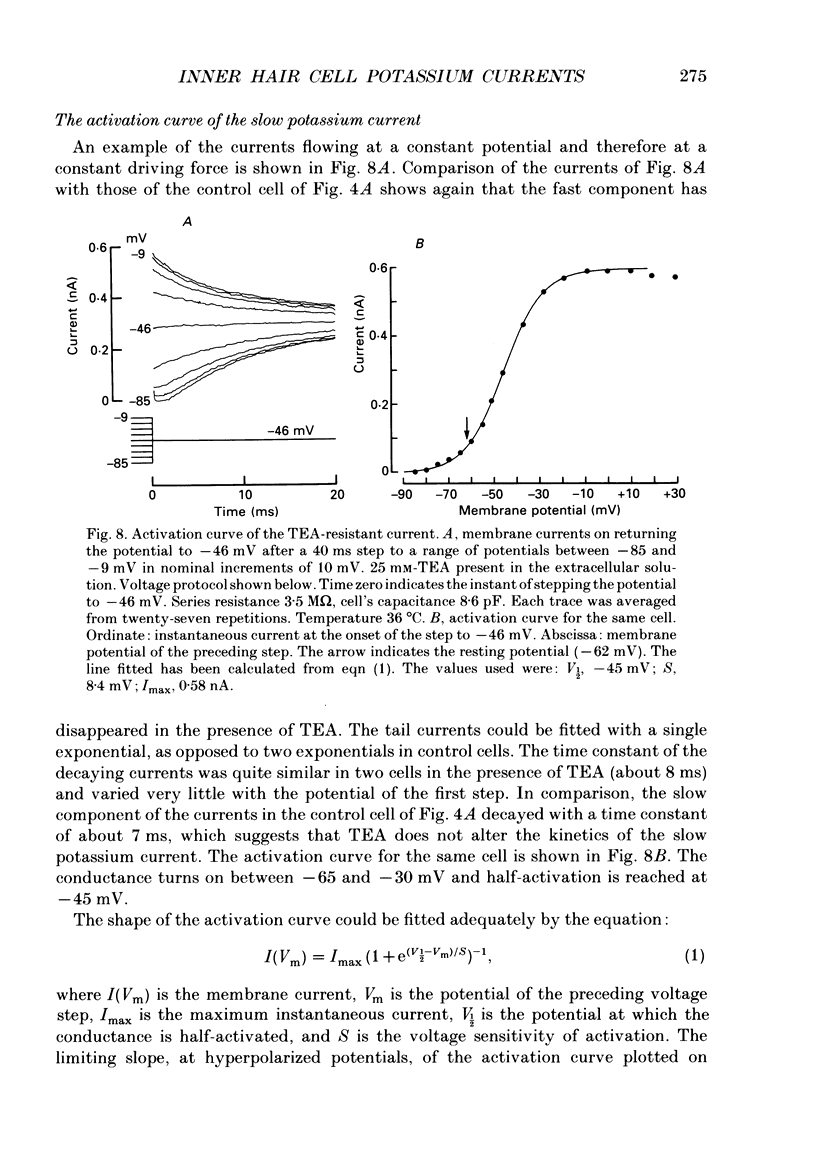
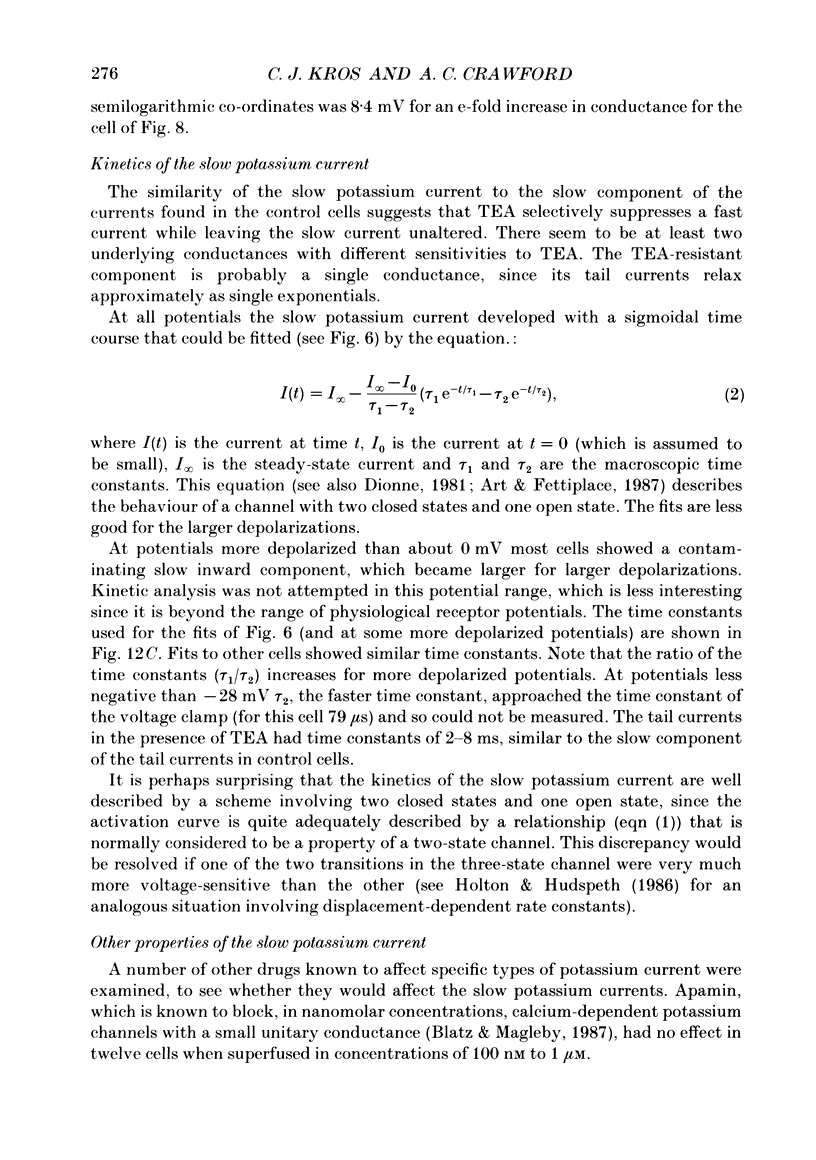
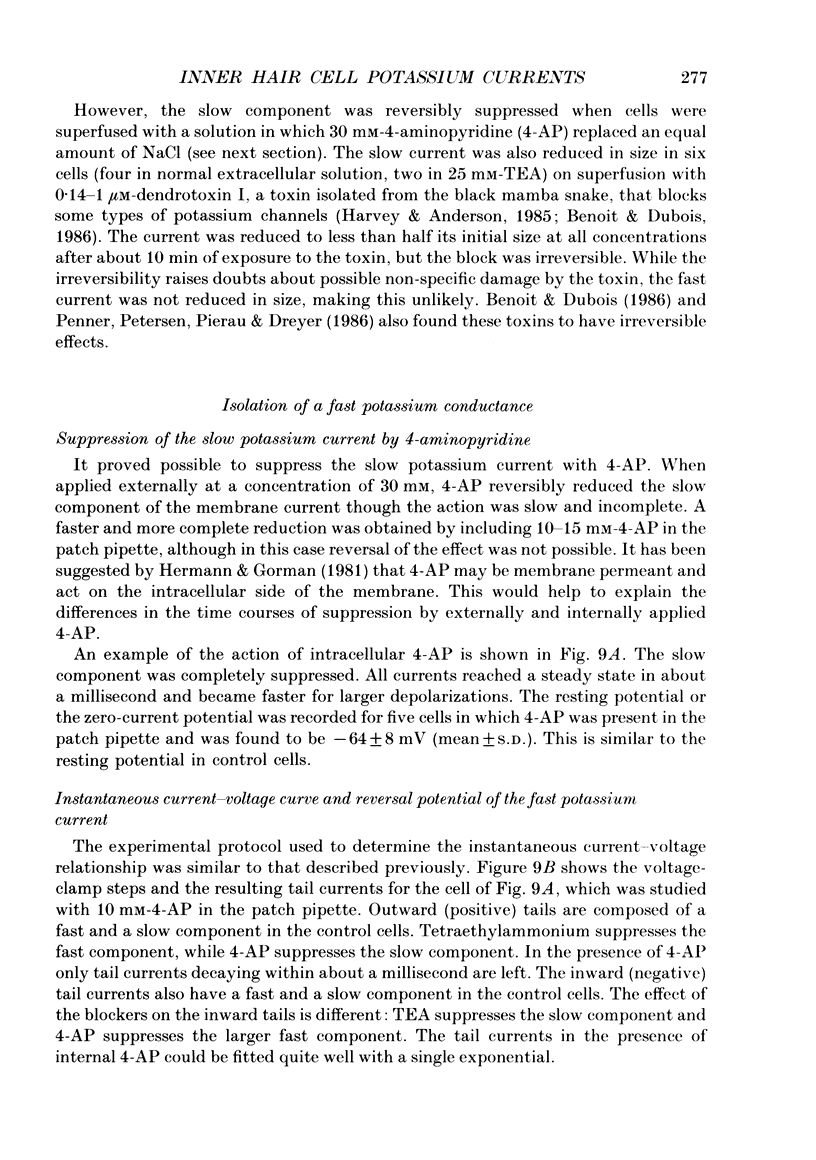
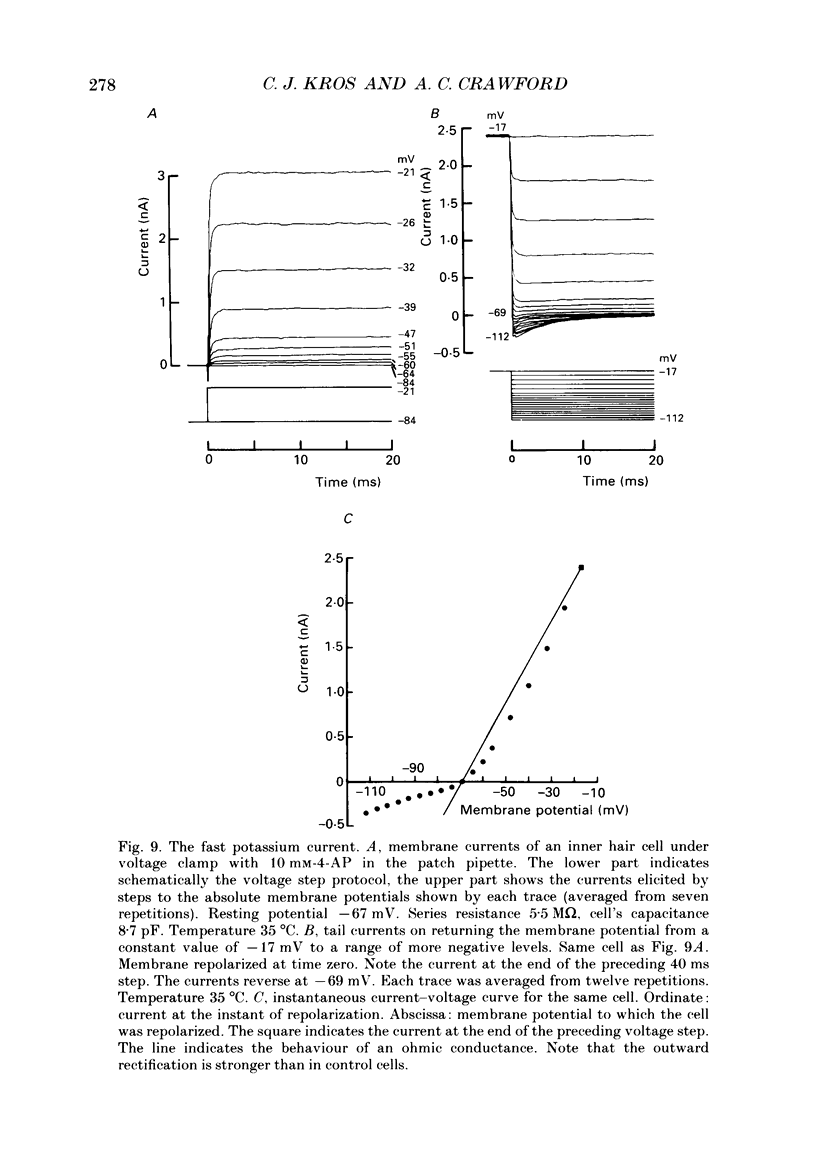
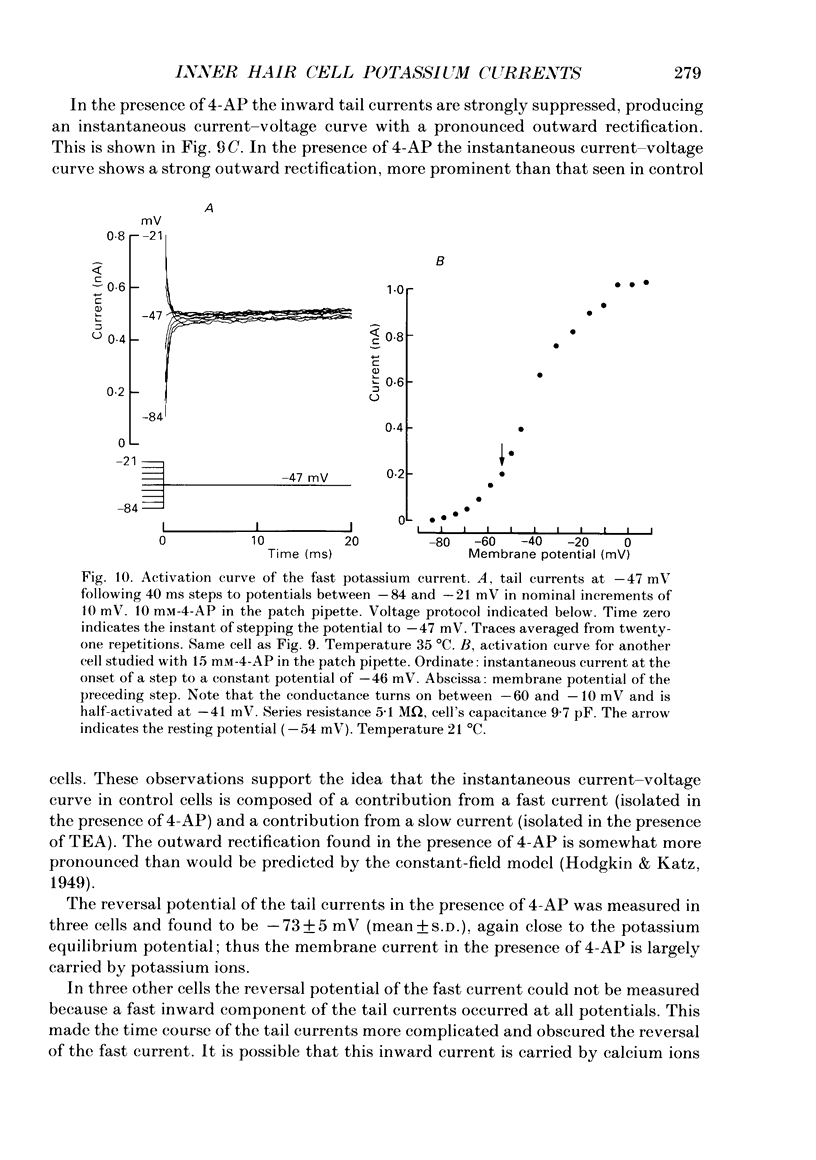
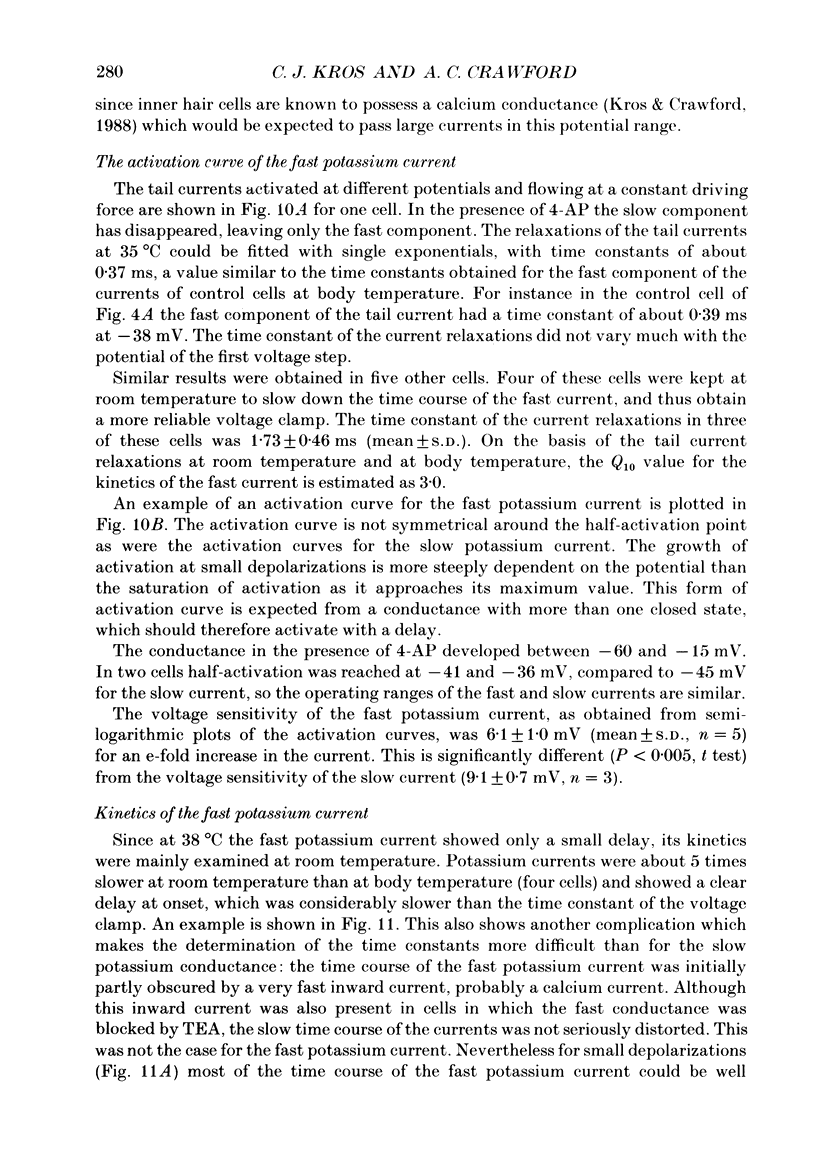
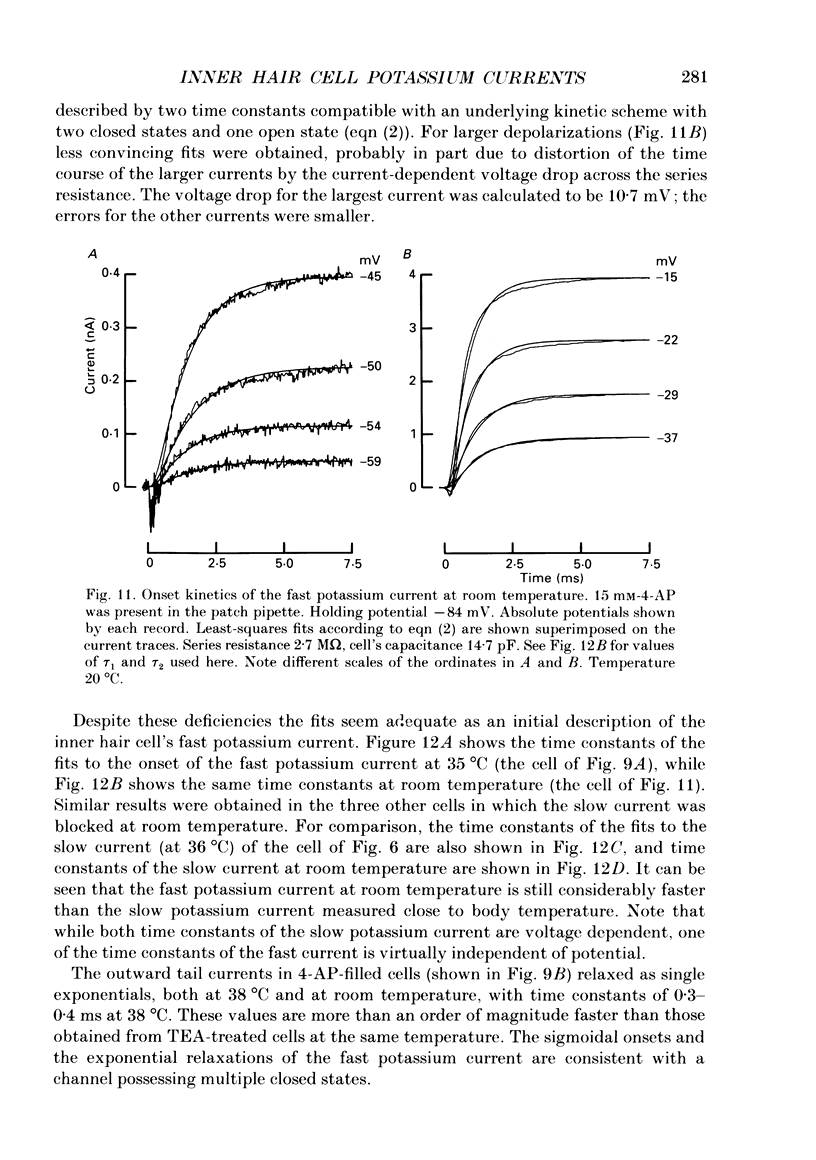
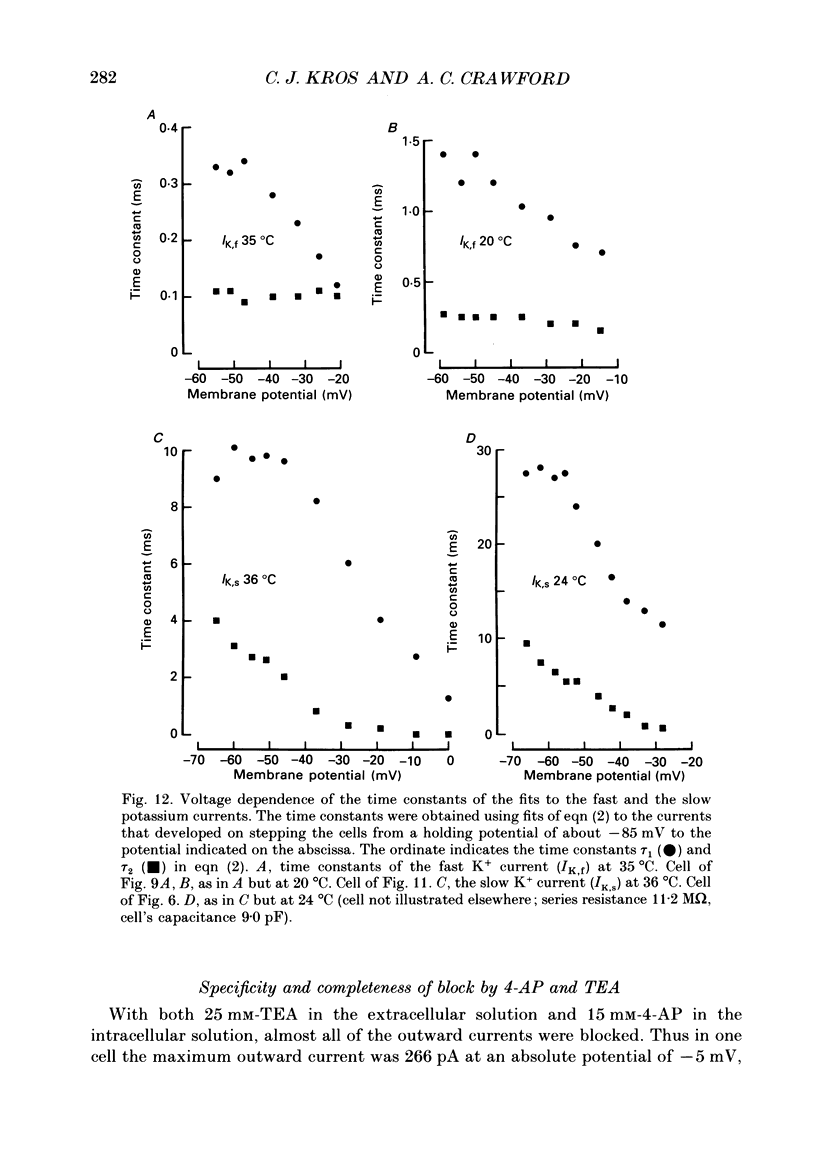
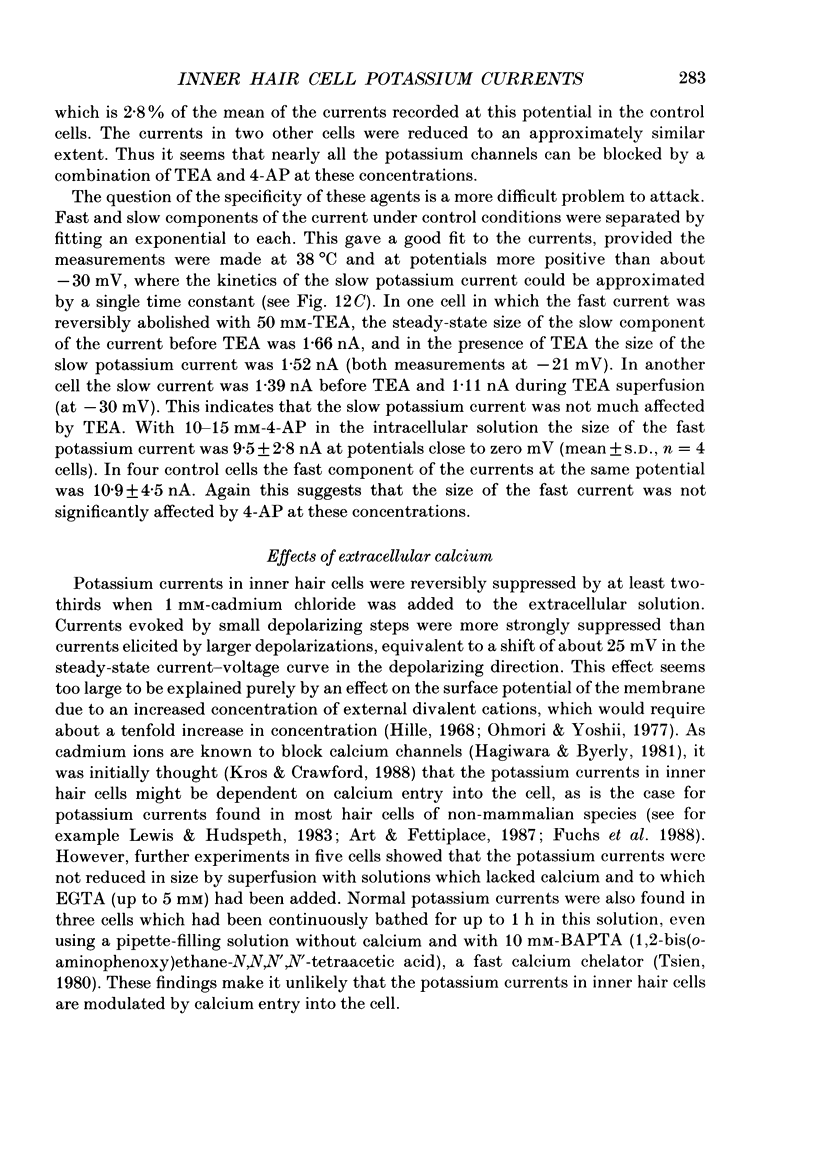
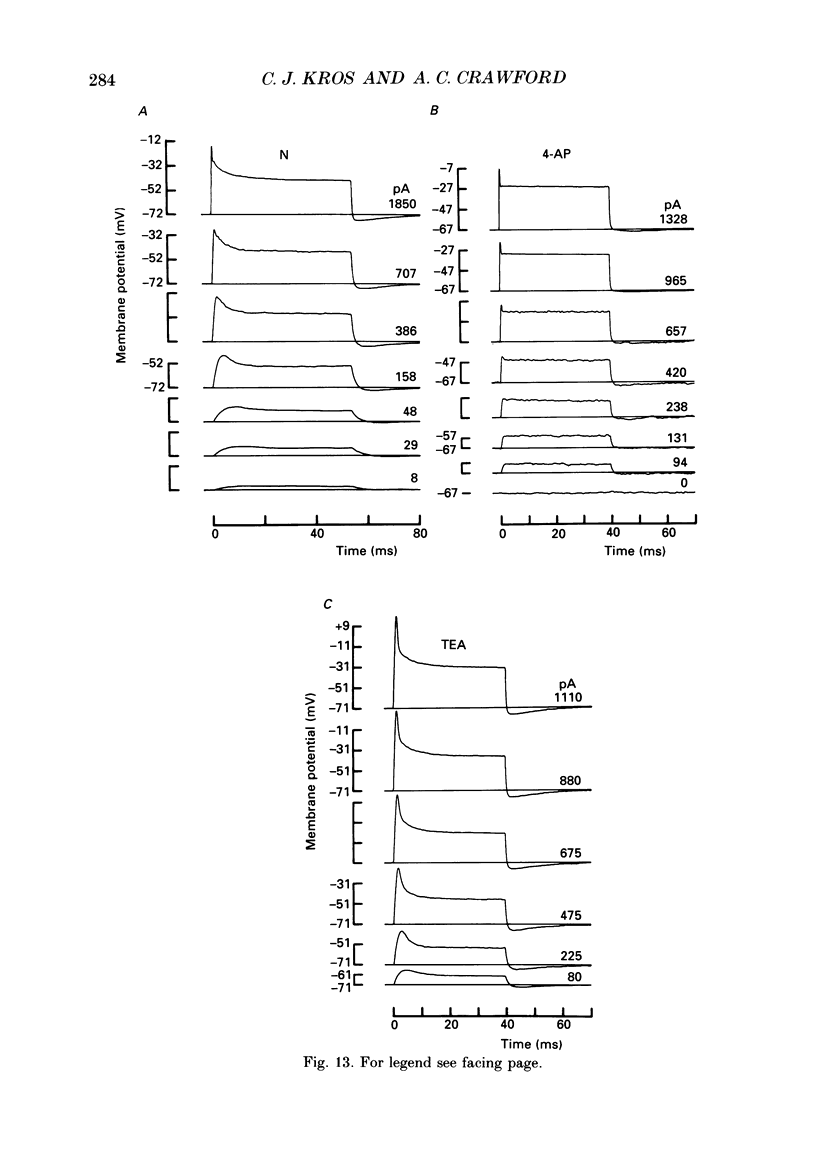
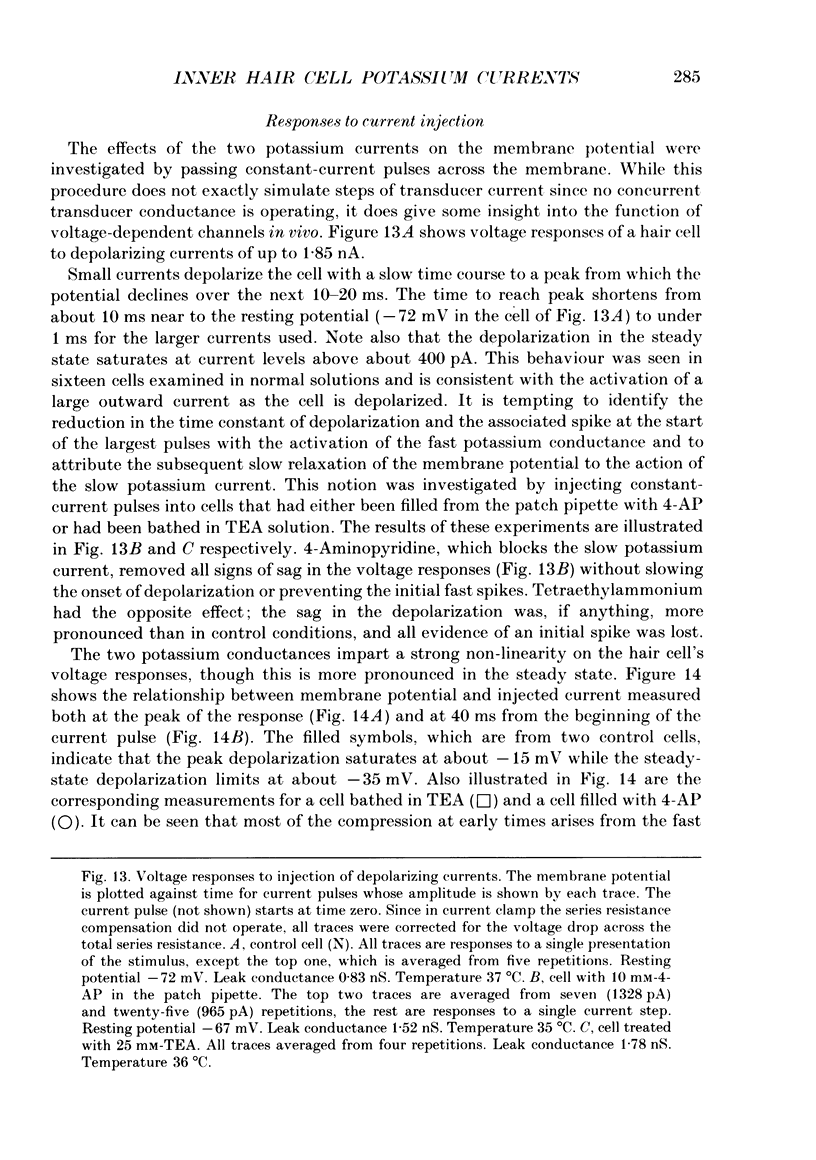
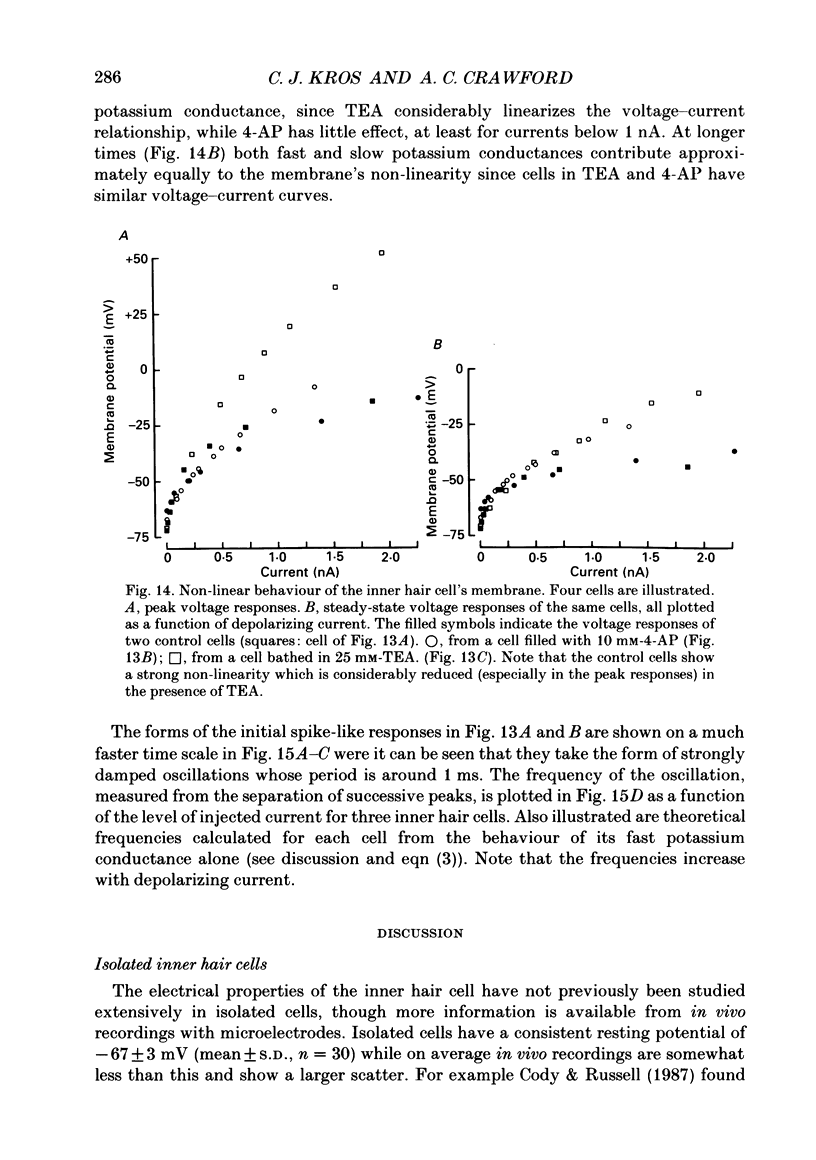
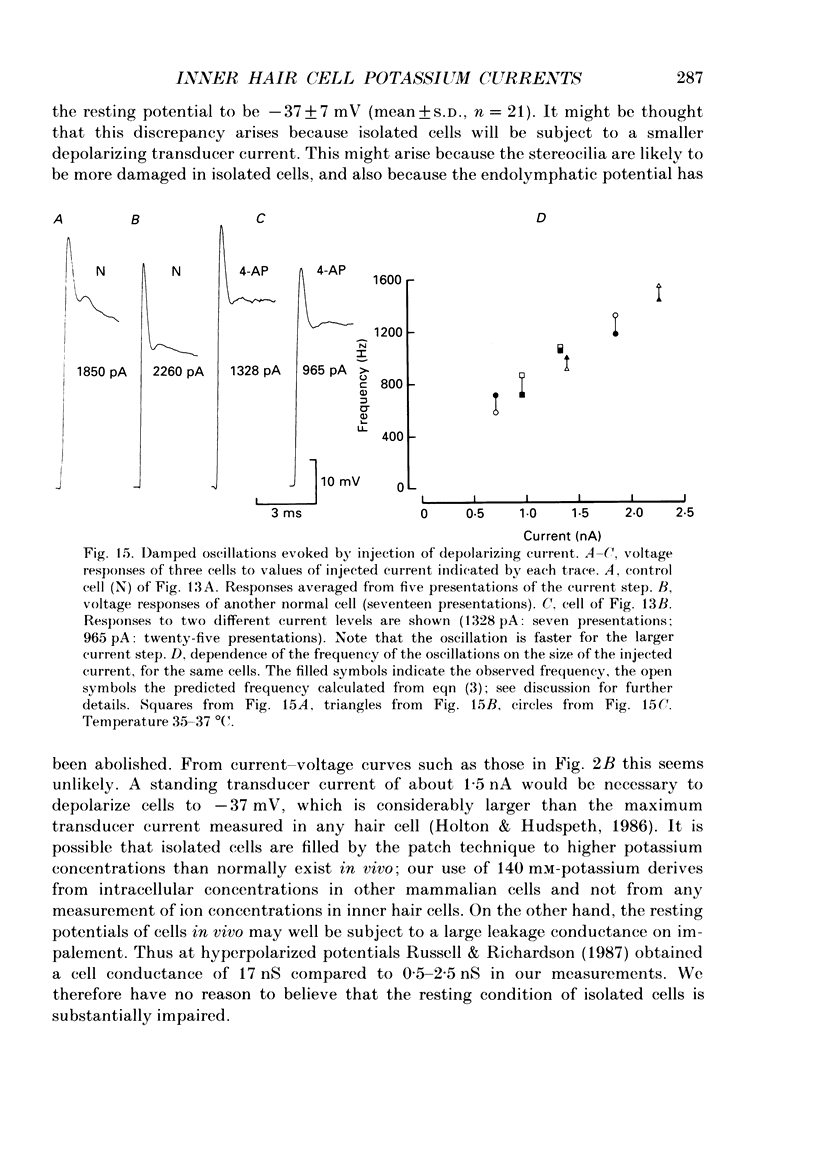
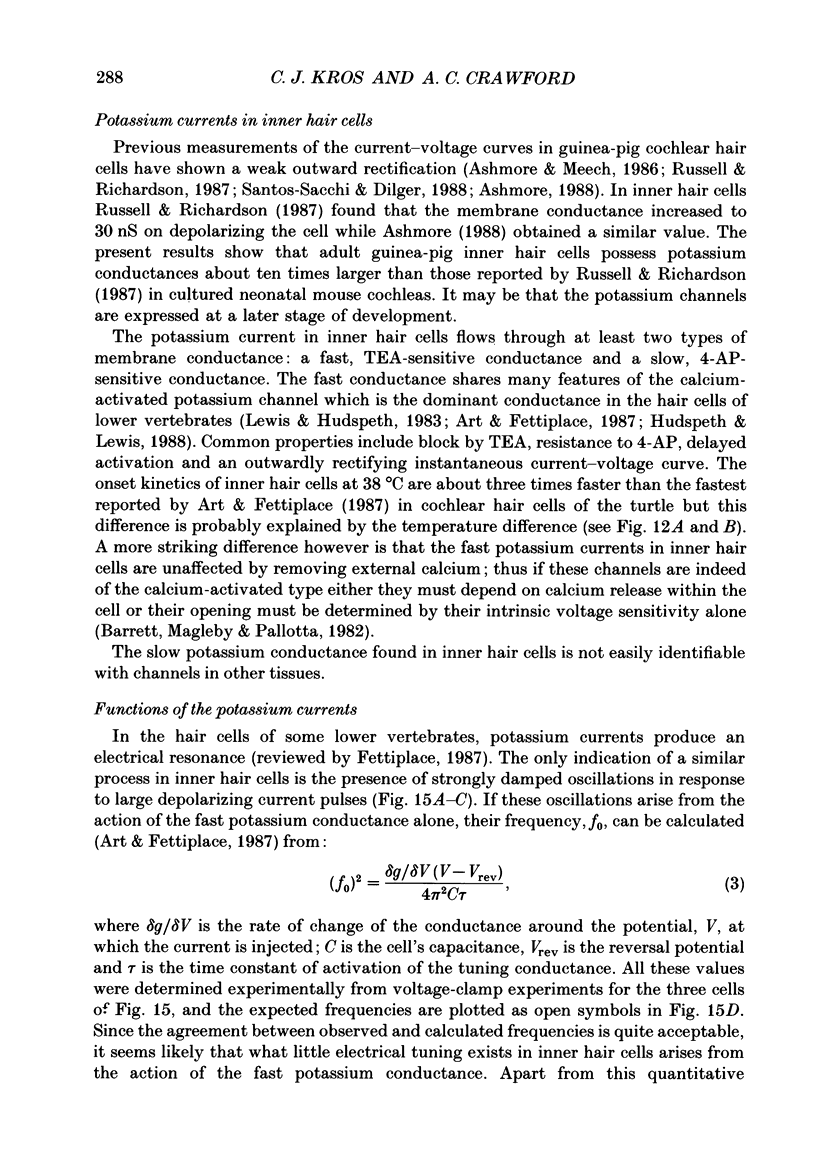
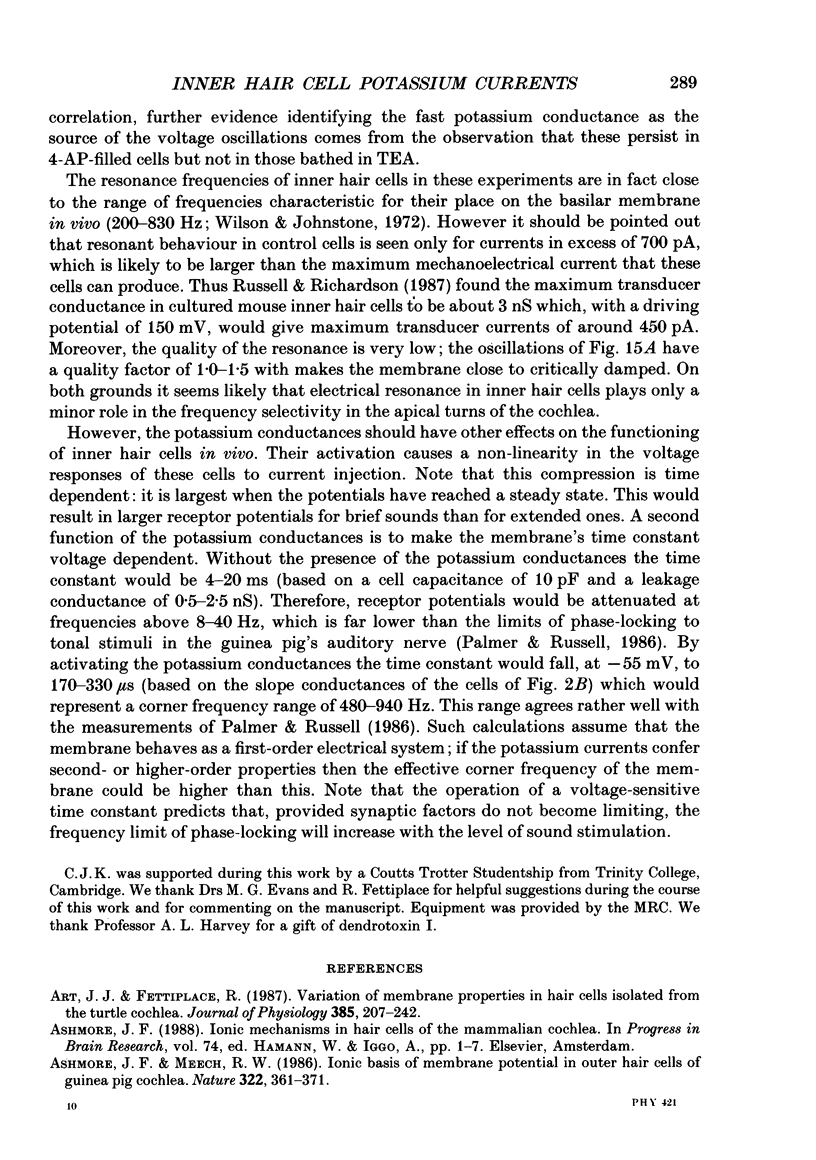
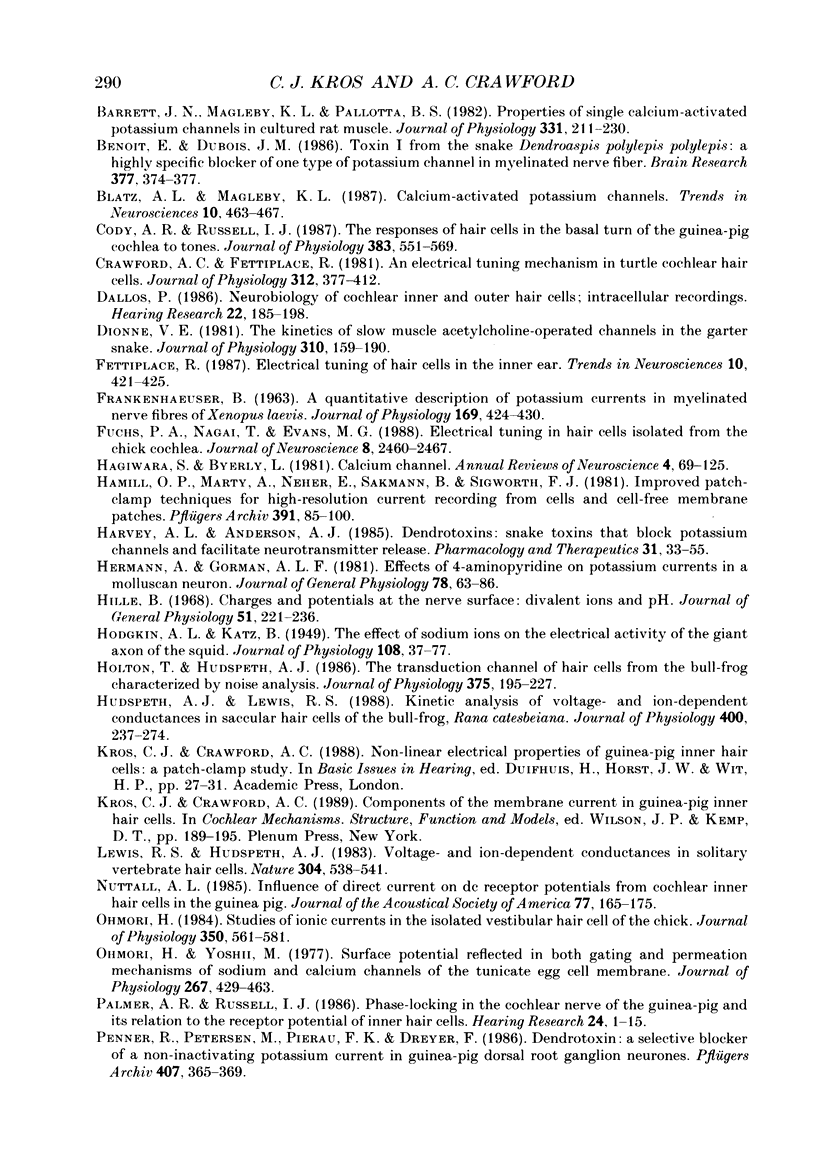
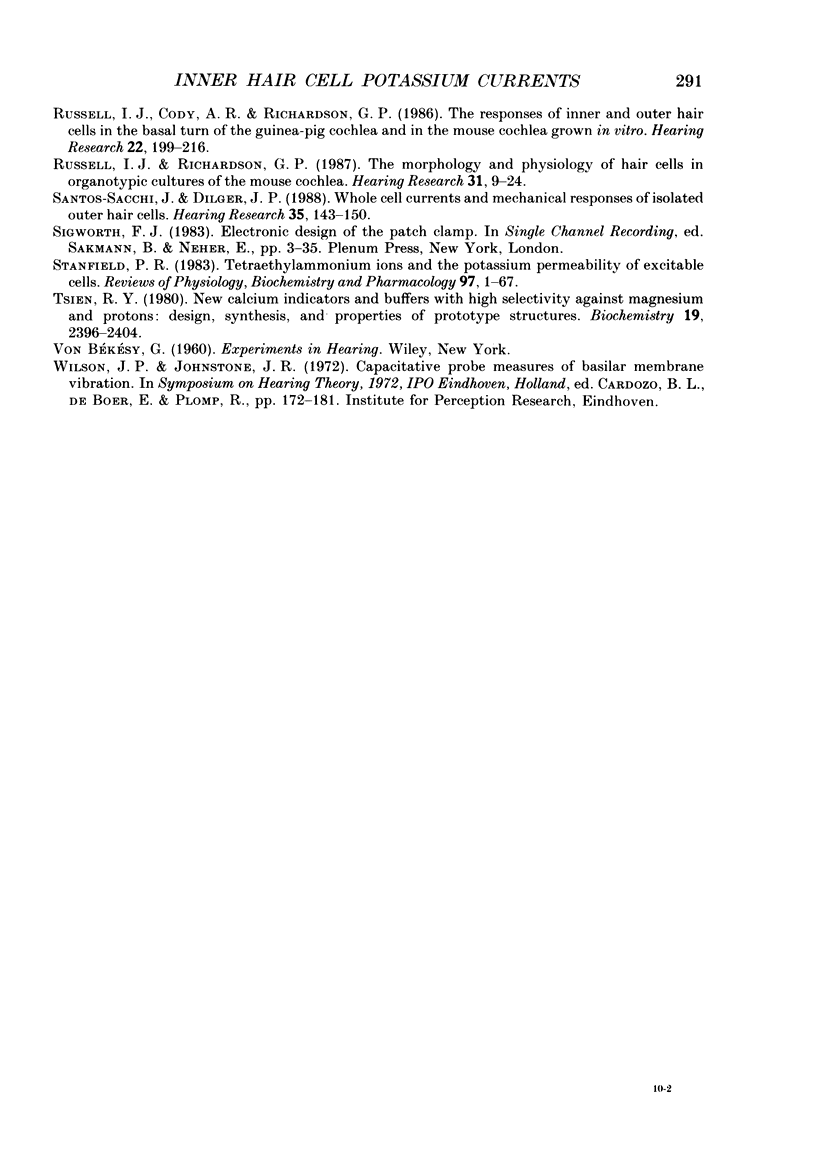
Images in this article
Selected References
These references are in PubMed. This may not be the complete list of references from this article.
- Art J. J., Fettiplace R. Variation of membrane properties in hair cells isolated from the turtle cochlea. J Physiol. 1987 Apr;385:207–242. doi: 10.1113/jphysiol.1987.sp016492. [DOI] [PMC free article] [PubMed] [Google Scholar]
- Ashmore J. F., Meech R. W. Ionic basis of membrane potential in outer hair cells of guinea pig cochlea. Nature. 1986 Jul 24;322(6077):368–371. doi: 10.1038/322368a0. [DOI] [PubMed] [Google Scholar]
- Barrett J. N., Magleby K. L., Pallotta B. S. Properties of single calcium-activated potassium channels in cultured rat muscle. J Physiol. 1982 Oct;331:211–230. doi: 10.1113/jphysiol.1982.sp014370. [DOI] [PMC free article] [PubMed] [Google Scholar]
- Benoit E., Dubois J. M. Toxin I from the snake Dendroaspis polylepis polylepis: a highly specific blocker of one type of potassium channel in myelinated nerve fiber. Brain Res. 1986 Jul 9;377(2):374–377. doi: 10.1016/0006-8993(86)90884-x. [DOI] [PubMed] [Google Scholar]
- Cody A. R., Russell I. J. The response of hair cells in the basal turn of the guinea-pig cochlea to tones. J Physiol. 1987 Feb;383:551–569. doi: 10.1113/jphysiol.1987.sp016428. [DOI] [PMC free article] [PubMed] [Google Scholar]
- Crawford A. C., Fettiplace R. An electrical tuning mechanism in turtle cochlear hair cells. J Physiol. 1981 Mar;312:377–412. doi: 10.1113/jphysiol.1981.sp013634. [DOI] [PMC free article] [PubMed] [Google Scholar]
- Dallos P. Neurobiology of cochlear inner and outer hair cells: intracellular recordings. Hear Res. 1986;22:185–198. doi: 10.1016/0378-5955(86)90095-x. [DOI] [PubMed] [Google Scholar]
- Dionne V. E. The kinetics of slow muscle acetylcholine-operated channels in the garter snake. J Physiol. 1981 Jan;310:159–190. doi: 10.1113/jphysiol.1981.sp013542. [DOI] [PMC free article] [PubMed] [Google Scholar]
- FRANKENHAEUSER B. A QUANTITATIVE DESCRIPTION OF POTASSIUM CURRENTS IN MYELINATED NERVE FIBRES OF XENOPUS LAEVIS. J Physiol. 1963 Nov;169:424–430. doi: 10.1113/jphysiol.1963.sp007268. [DOI] [PMC free article] [PubMed] [Google Scholar]
- Fuchs P. A., Nagai T., Evans M. G. Electrical tuning in hair cells isolated from the chick cochlea. J Neurosci. 1988 Jul;8(7):2460–2467. doi: 10.1523/JNEUROSCI.08-07-02460.1988. [DOI] [PMC free article] [PubMed] [Google Scholar]
- HODGKIN A. L., KATZ B. The effect of sodium ions on the electrical activity of giant axon of the squid. J Physiol. 1949 Mar 1;108(1):37–77. doi: 10.1113/jphysiol.1949.sp004310. [DOI] [PMC free article] [PubMed] [Google Scholar]
- Hagiwara S., Byerly L. Calcium channel. Annu Rev Neurosci. 1981;4:69–125. doi: 10.1146/annurev.ne.04.030181.000441. [DOI] [PubMed] [Google Scholar]
- Hamill O. P., Marty A., Neher E., Sakmann B., Sigworth F. J. Improved patch-clamp techniques for high-resolution current recording from cells and cell-free membrane patches. Pflugers Arch. 1981 Aug;391(2):85–100. doi: 10.1007/BF00656997. [DOI] [PubMed] [Google Scholar]
- Harvey A. L., Anderson A. J. Dendrotoxins: snake toxins that block potassium channels and facilitate neurotransmitter release. Pharmacol Ther. 1985;31(1-2):33–55. doi: 10.1016/0163-7258(85)90036-1. [DOI] [PubMed] [Google Scholar]
- Hermann A., Gorman A. L. Effects of 4-aminopyridine on potassium currents in a molluscan neuron. J Gen Physiol. 1981 Jul;78(1):63–86. doi: 10.1085/jgp.78.1.63. [DOI] [PMC free article] [PubMed] [Google Scholar]
- Hille B. Charges and potentials at the nerve surface. Divalent ions and pH. J Gen Physiol. 1968 Feb;51(2):221–236. doi: 10.1085/jgp.51.2.221. [DOI] [PMC free article] [PubMed] [Google Scholar]
- Holton T., Hudspeth A. J. The transduction channel of hair cells from the bull-frog characterized by noise analysis. J Physiol. 1986 Jun;375:195–227. doi: 10.1113/jphysiol.1986.sp016113. [DOI] [PMC free article] [PubMed] [Google Scholar]
- Hudspeth A. J., Lewis R. S. Kinetic analysis of voltage- and ion-dependent conductances in saccular hair cells of the bull-frog, Rana catesbeiana. J Physiol. 1988 Jun;400:237–274. doi: 10.1113/jphysiol.1988.sp017119. [DOI] [PMC free article] [PubMed] [Google Scholar]
- Lewis R. S., Hudspeth A. J. Voltage- and ion-dependent conductances in solitary vertebrate hair cells. Nature. 1983 Aug 11;304(5926):538–541. doi: 10.1038/304538a0. [DOI] [PubMed] [Google Scholar]
- Nuttall A. L. Influence of direct current on dc receptor potentials from cochlear inner hair cells in the guinea pig. J Acoust Soc Am. 1985 Jan;77(1):165–175. doi: 10.1121/1.392282. [DOI] [PubMed] [Google Scholar]
- Ohmori H. Studies of ionic currents in the isolated vestibular hair cell of the chick. J Physiol. 1984 May;350:561–581. doi: 10.1113/jphysiol.1984.sp015218. [DOI] [PMC free article] [PubMed] [Google Scholar]
- Ohmori H., Yoshii M. Surface potential reflected in both gating and permeation mechanisms of sodium and calcium channels of the tunicate egg cell membrane. J Physiol. 1977 May;267(2):429–463. doi: 10.1113/jphysiol.1977.sp011821. [DOI] [PMC free article] [PubMed] [Google Scholar]
- Palmer A. R., Russell I. J. Phase-locking in the cochlear nerve of the guinea-pig and its relation to the receptor potential of inner hair-cells. Hear Res. 1986;24(1):1–15. doi: 10.1016/0378-5955(86)90002-x. [DOI] [PubMed] [Google Scholar]
- Penner R., Petersen M., Pierau F. K., Dreyer F. Dendrotoxin: a selective blocker of a non-inactivating potassium current in guinea-pig dorsal root ganglion neurones. Pflugers Arch. 1986 Oct;407(4):365–369. doi: 10.1007/BF00652619. [DOI] [PubMed] [Google Scholar]
- Russell I. J., Cody A. R., Richardson G. P. The responses of inner and outer hair cells in the basal turn of the guinea-pig cochlea and in the mouse cochlea grown in vitro. Hear Res. 1986;22:199–216. doi: 10.1016/0378-5955(86)90096-1. [DOI] [PubMed] [Google Scholar]
- Russell I. J., Richardson G. P. The morphology and physiology of hair cells in organotypic cultures of the mouse cochlea. Hear Res. 1987 Nov;31(1):9–24. doi: 10.1016/0378-5955(87)90210-3. [DOI] [PubMed] [Google Scholar]
- Santos-Sacchi J., Dilger J. P. Whole cell currents and mechanical responses of isolated outer hair cells. Hear Res. 1988 Sep 15;35(2-3):143–150. doi: 10.1016/0378-5955(88)90113-x. [DOI] [PubMed] [Google Scholar]
- Stanfield P. R. Tetraethylammonium ions and the potassium permeability of excitable cells. Rev Physiol Biochem Pharmacol. 1983;97:1–67. doi: 10.1007/BFb0035345. [DOI] [PubMed] [Google Scholar]
- Tsien R. Y. New calcium indicators and buffers with high selectivity against magnesium and protons: design, synthesis, and properties of prototype structures. Biochemistry. 1980 May 27;19(11):2396–2404. doi: 10.1021/bi00552a018. [DOI] [PubMed] [Google Scholar]