Abstract
1. We have employed a paired-tracer isotope dilution technique in a perfused rat hindlimb preparation to obtain information on the kinetics of transport across the sarcolemmal membranes of acidic, neutral and basic amino acids. 2. We have defined the characteristics of the saturable transport of amino acids normally regarded as paradigm substrates for the A, ASC, L, y+(basic) and the dicarboxylic amino acid transport systems. Their maximal transport capacities (Vmax, nmol min-1 (g muscle)-1 and substrate concentrations for half-maximal transport (Km, mM) of representative amino acid substrates are as follows: 2-aminoisobutyrate (AIB), Vmax = 15 +/- 7, Km = 1.26 +/- 0.6; alanine, Vmax = 332 +/- 53, Km = 3.9 +/- 0.9; serine, Vmax = 410 +/- 61, Km 3.4 +/- 0.5; leucine, Vmax = 2800 +/- 420, Km = 20 +/- 2; lysine, Vmax = 136 +/- 46, Km = 2.1 +/- 1.3; glutamate, Vmax = 86 +/- 6, Km = 1.05 +/- 0.05; proline, Vmax = 196 +/- 48, Km = 4.1 +/- 0.6. 3. Glycine uptake was faster than expected on the basis of diffusion but was not saturable and showed uptake that could be best described by a first-order rate constant of 0.07 +/- 0.003 min-1. 4. We have attempted to discriminate kinetically between possible routes of entry for an amino acid on the basis of competitive and non-competitive interaction between substrates potentially sharing common routes. On this basis, the major routes of alanine entry appear to be via the ASC and L systems with the A system playing a quantitatively minor role. Glutamate and aspartate appear to be transported exclusively by a dicarboxylate amino acid carrier. The branched-chain amino acids (BCAA) and the aromatic amino acid, phenylalanine, are almost equivalent substrates for an L-like system. 5. Insulin had no detectable effect on the uptake of paradigm substrates for ASC, L, y+, the dicarboxylic amino acid or glycine transport systems. 6. Transport of serine and lysine was Na+ dependent. Lysine transport apparently occurred with a stoichiometry of 2 Na+: 1 lysine. With the exception of alanine, whose transport was partially Na+ dependent, all other amino acids examined in the present study were transported in a Na+-independent manner.
Full text
PDF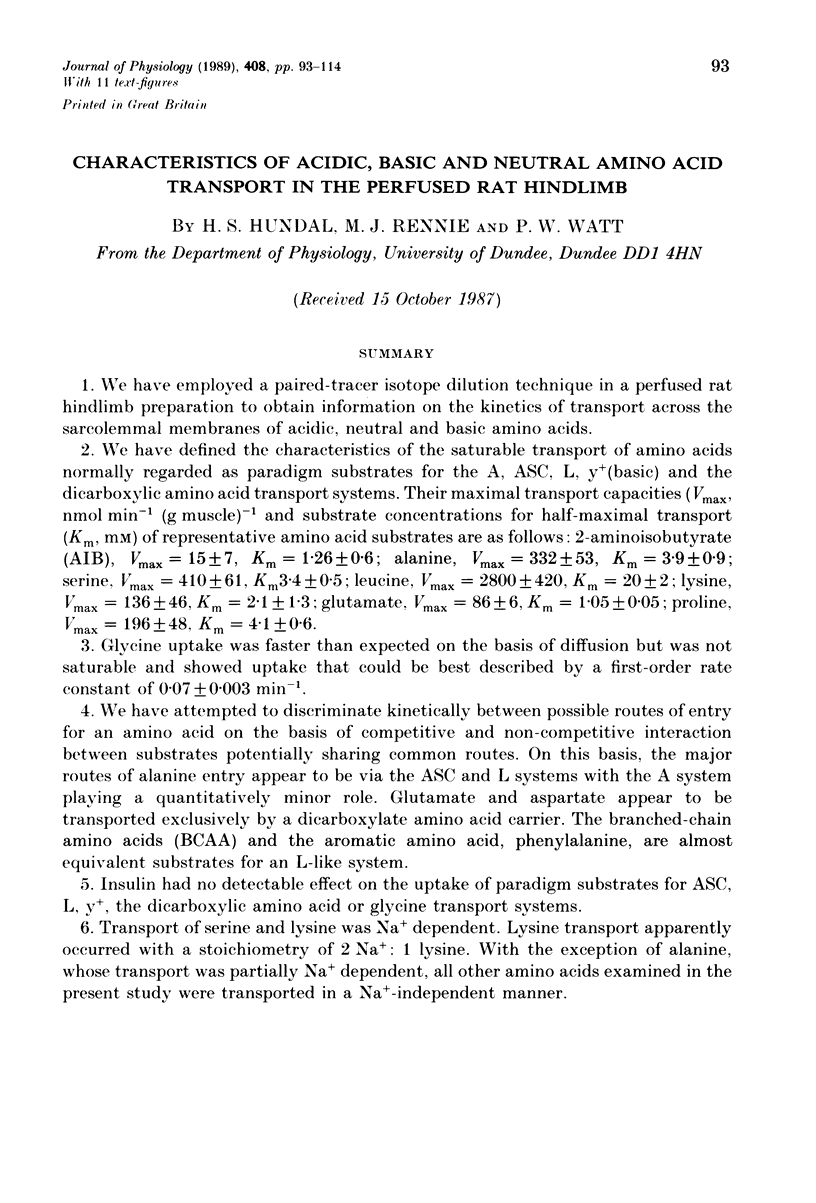
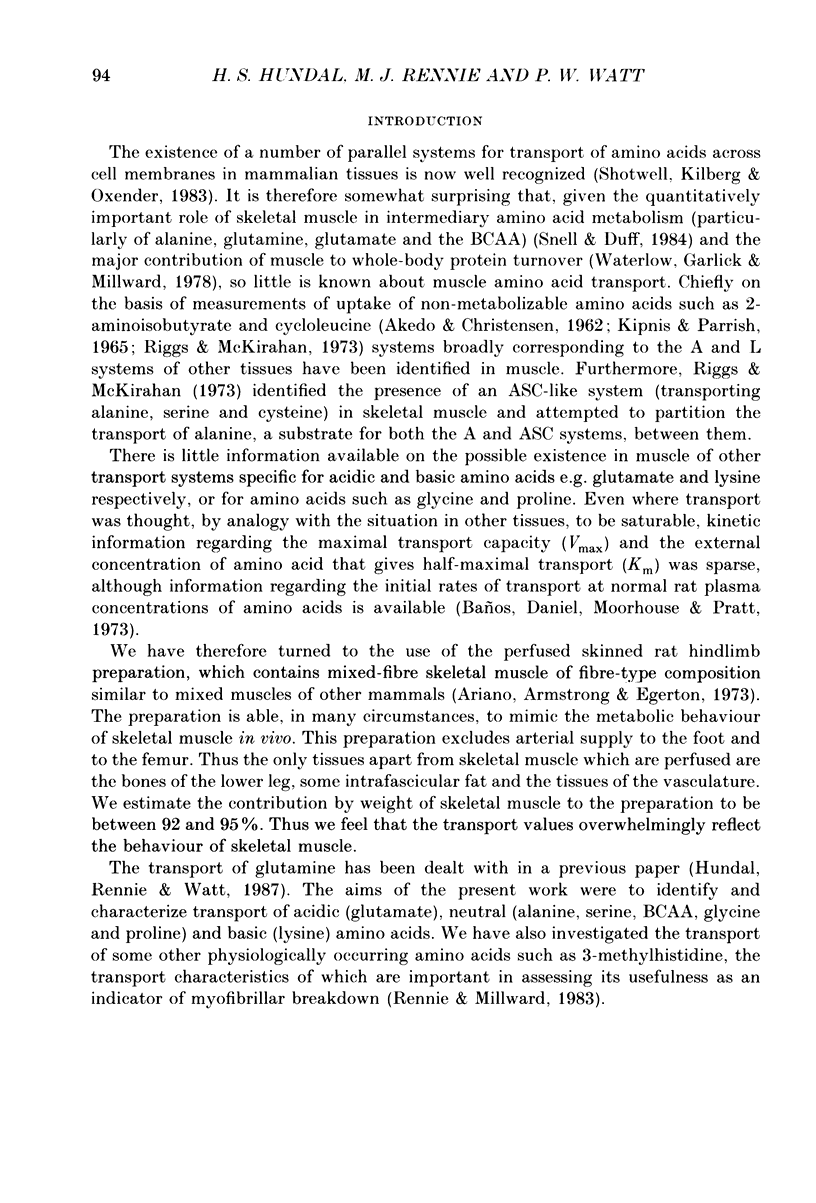
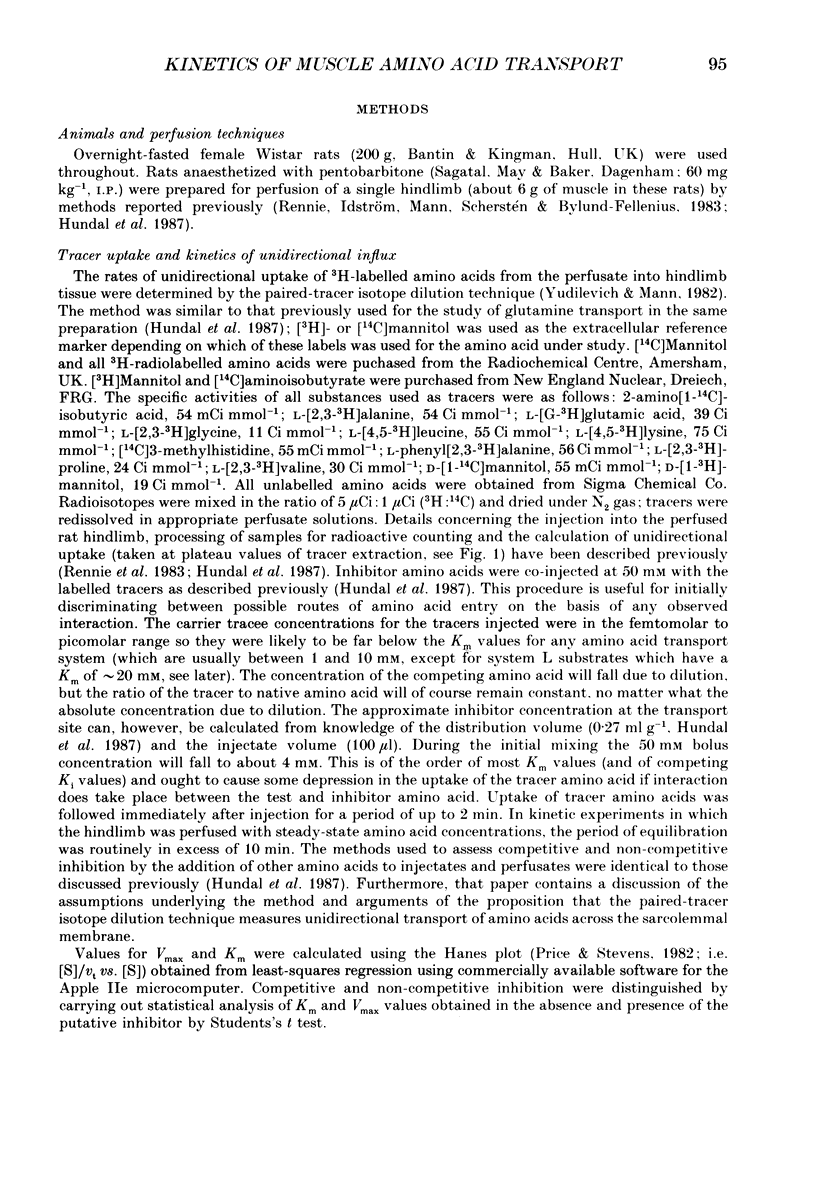
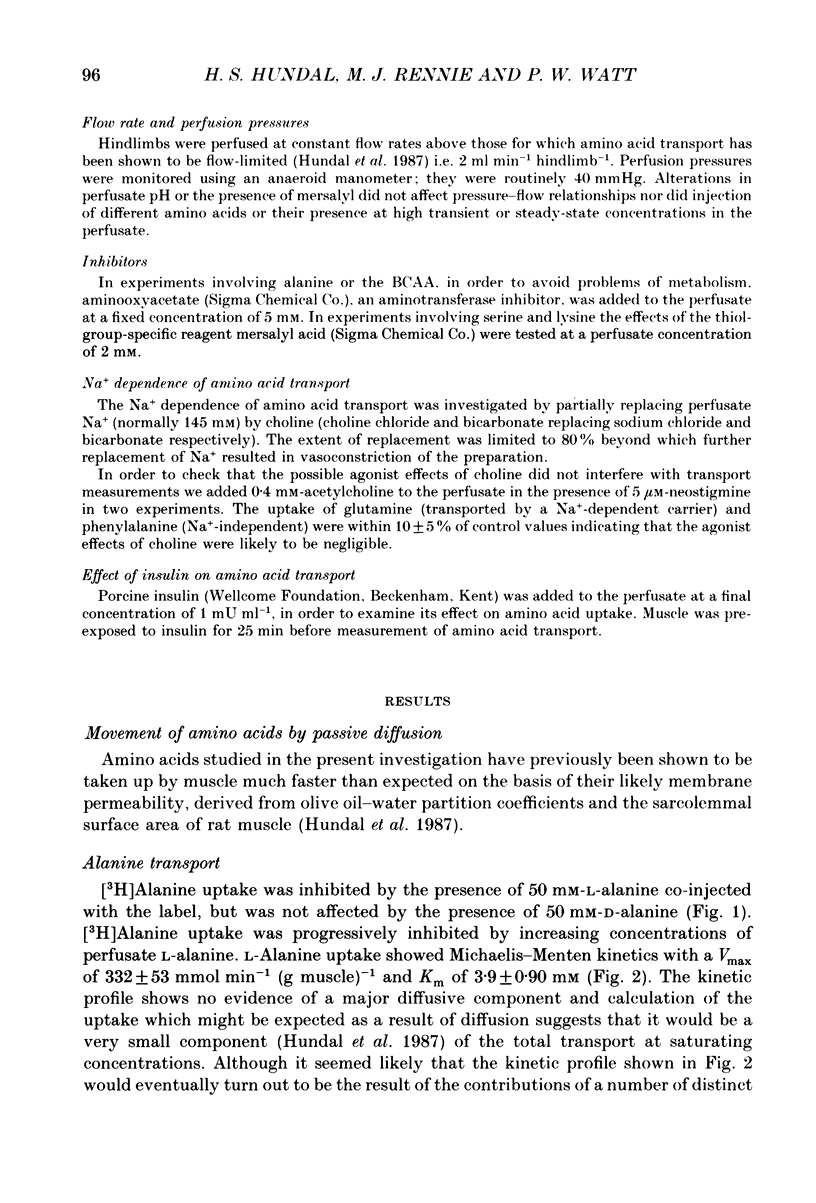
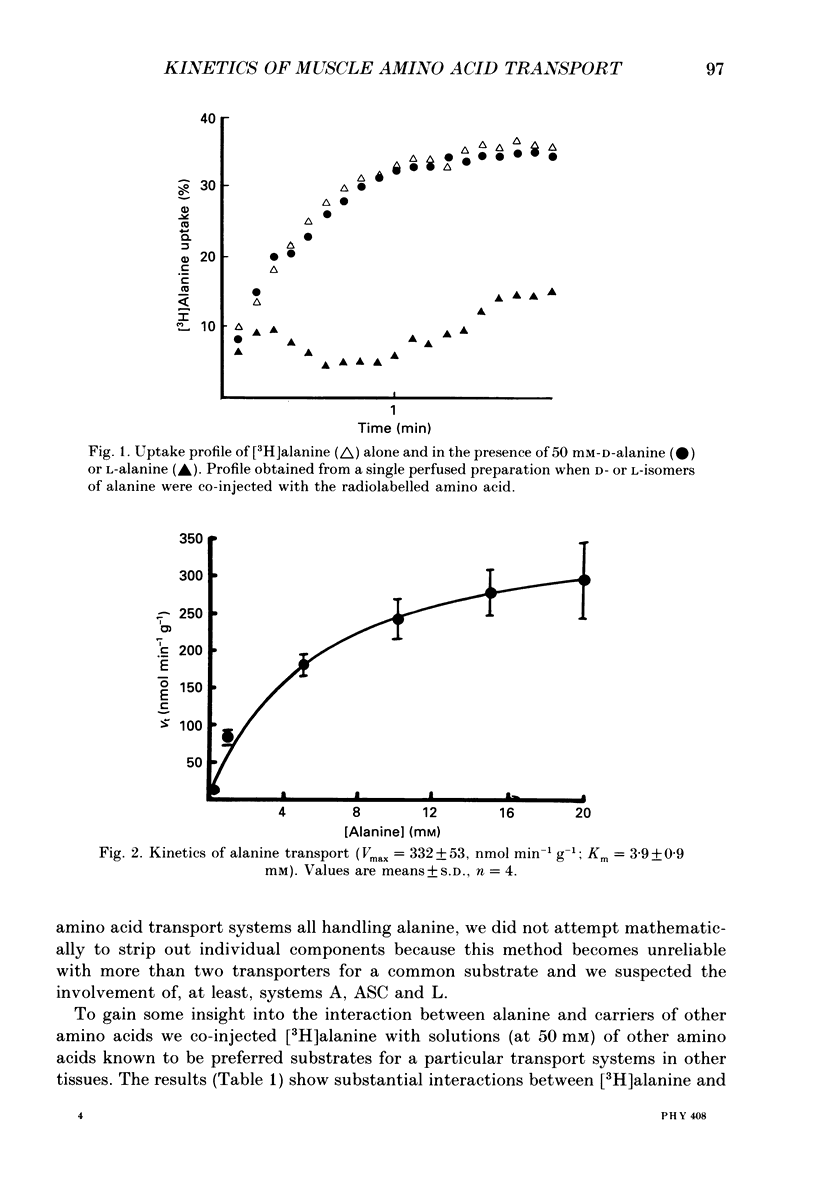
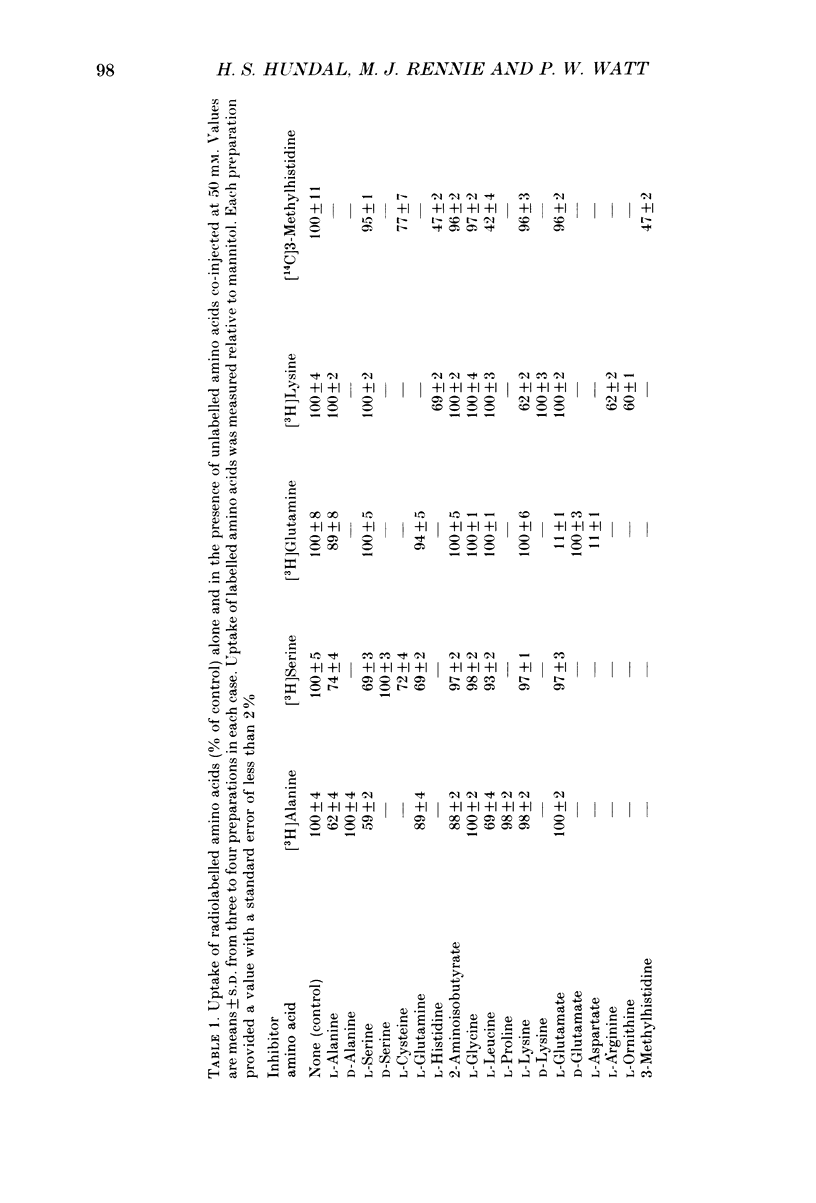
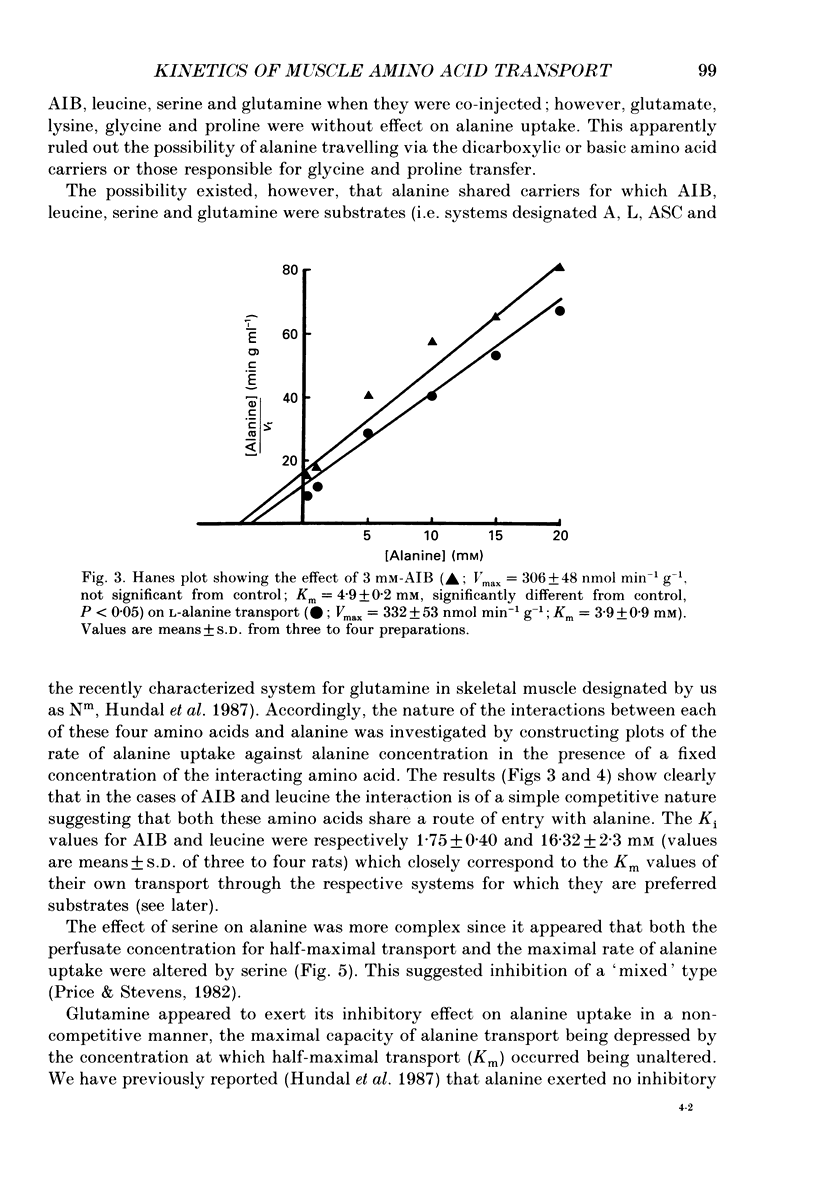
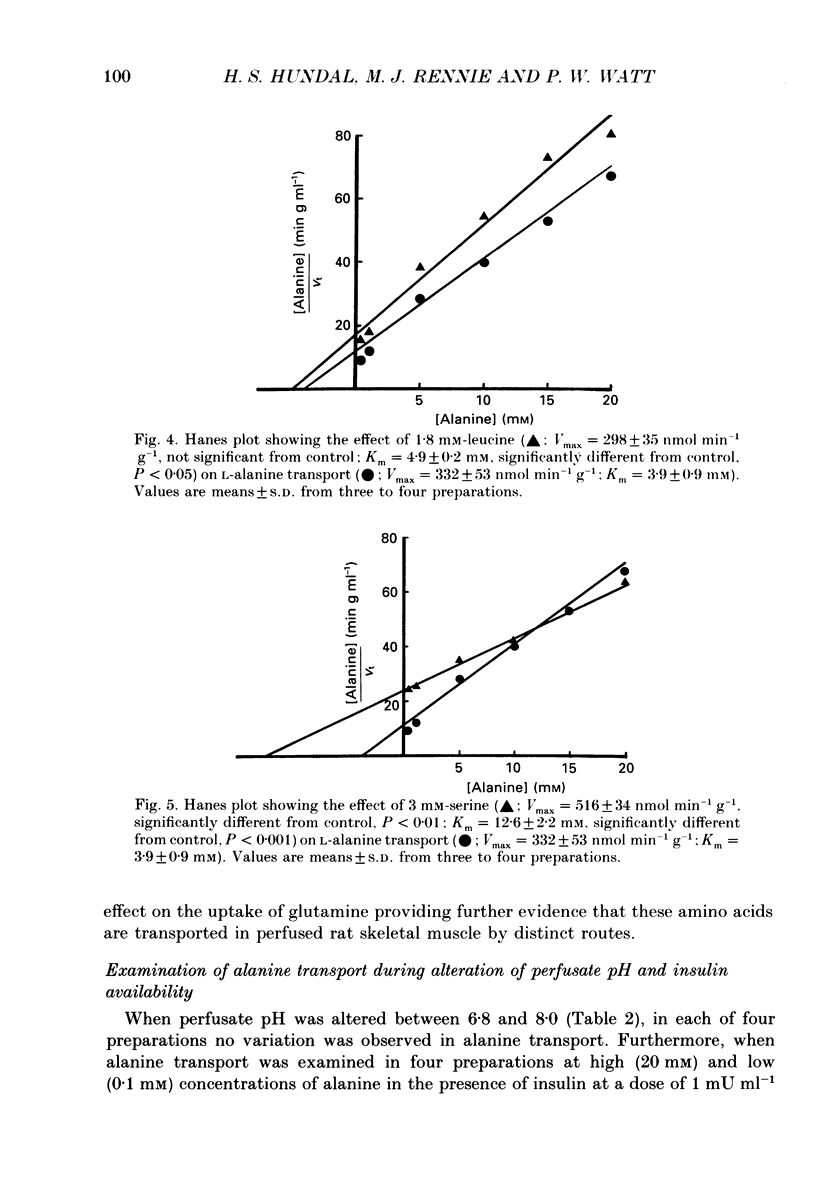
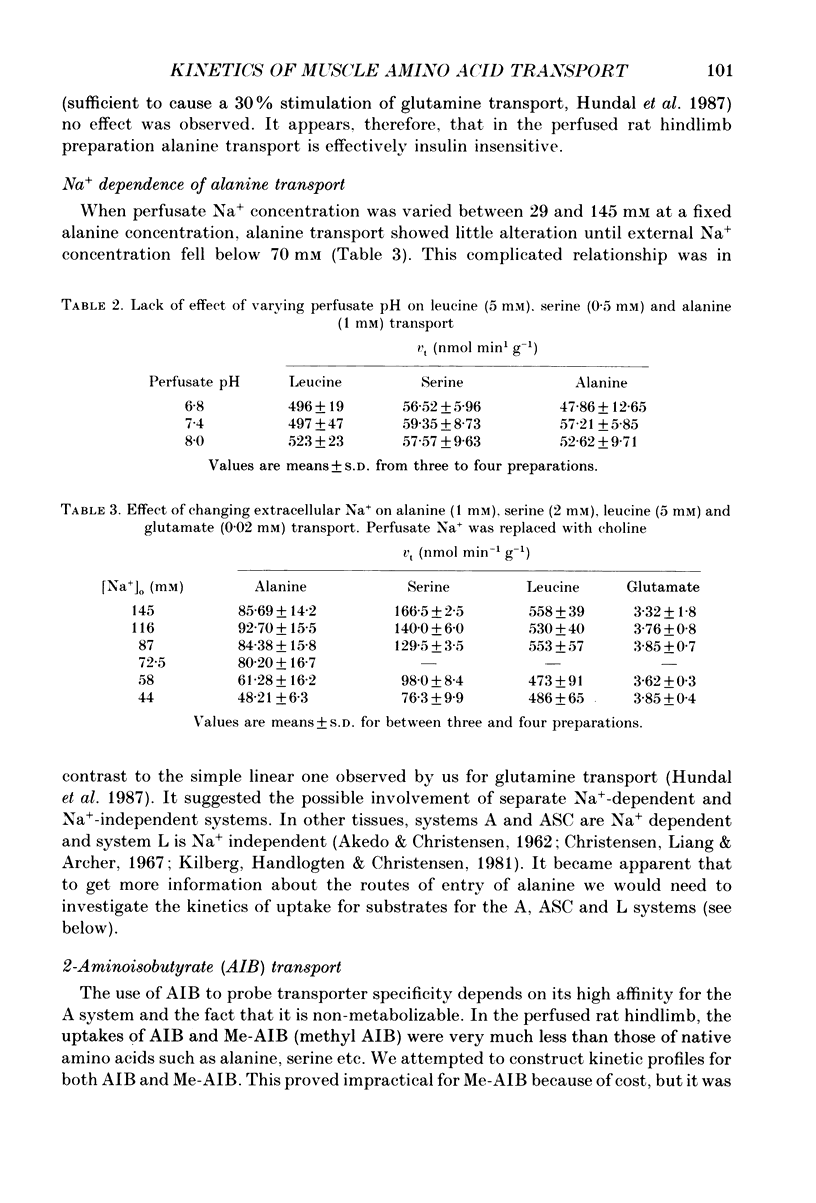
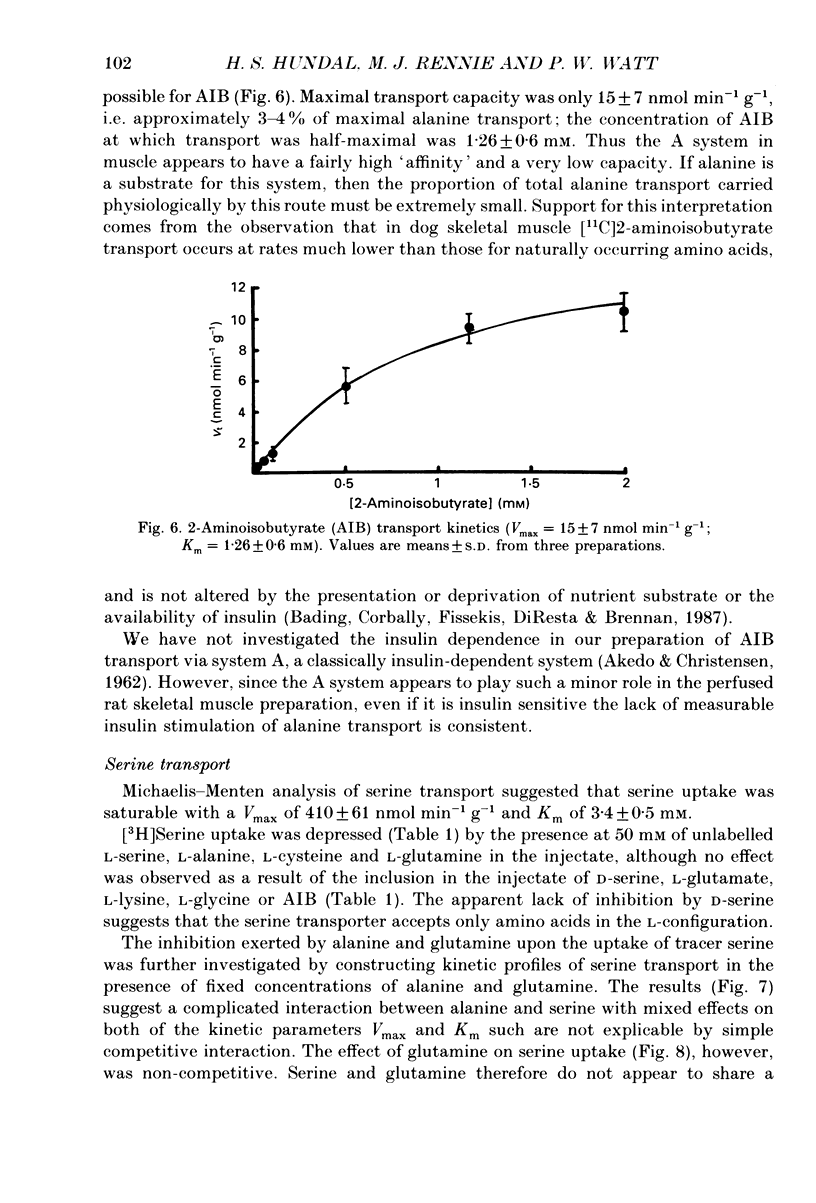
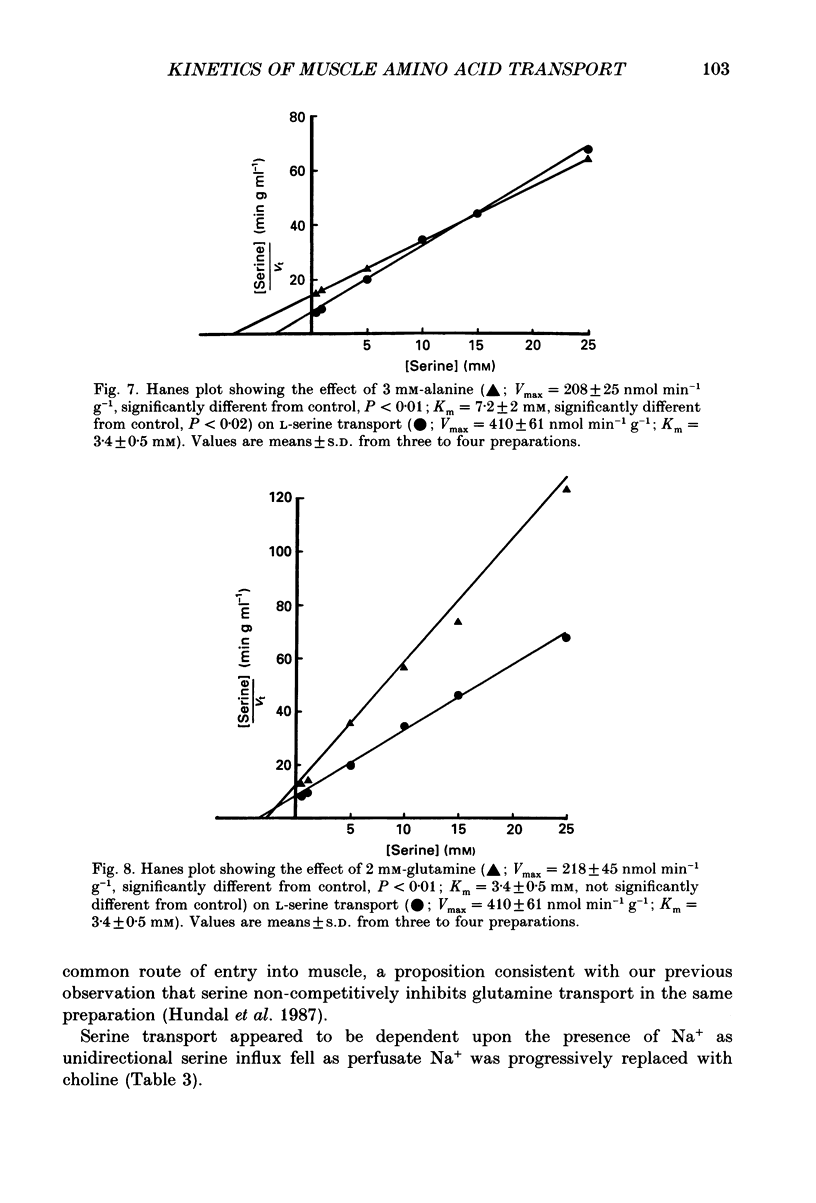
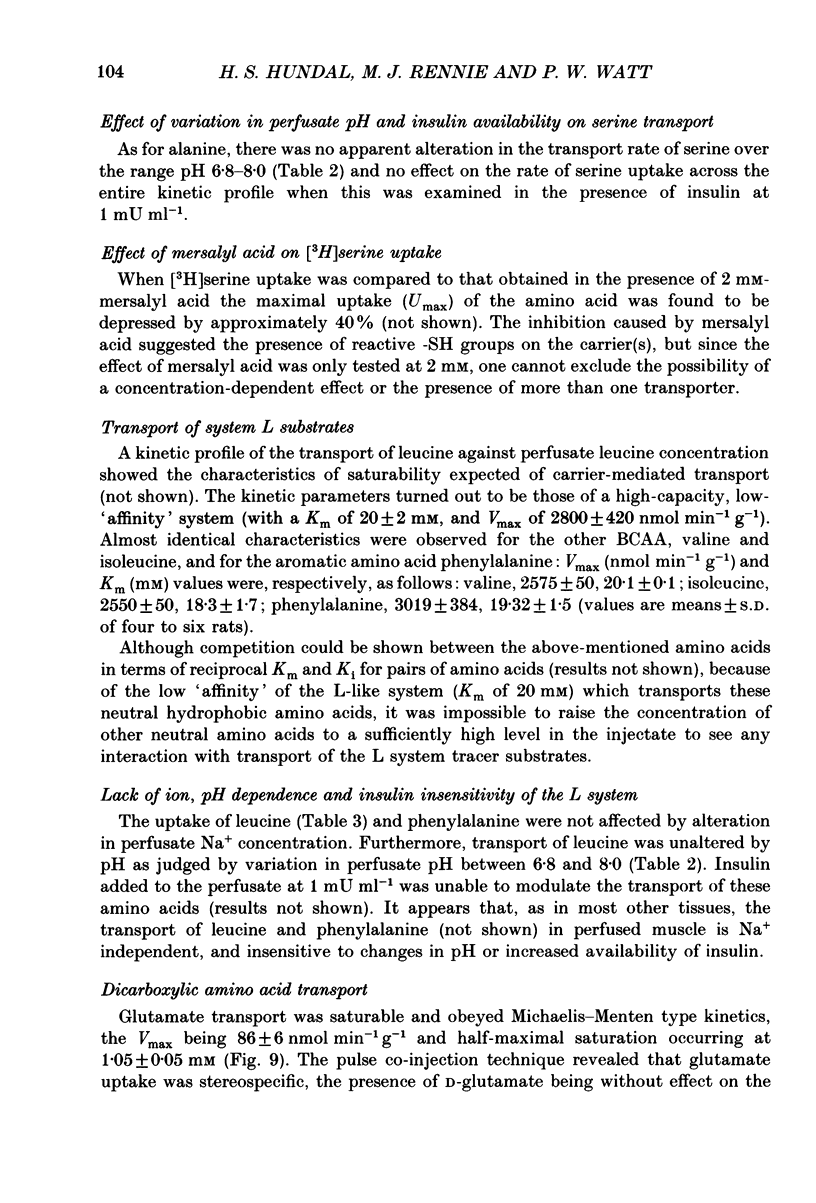
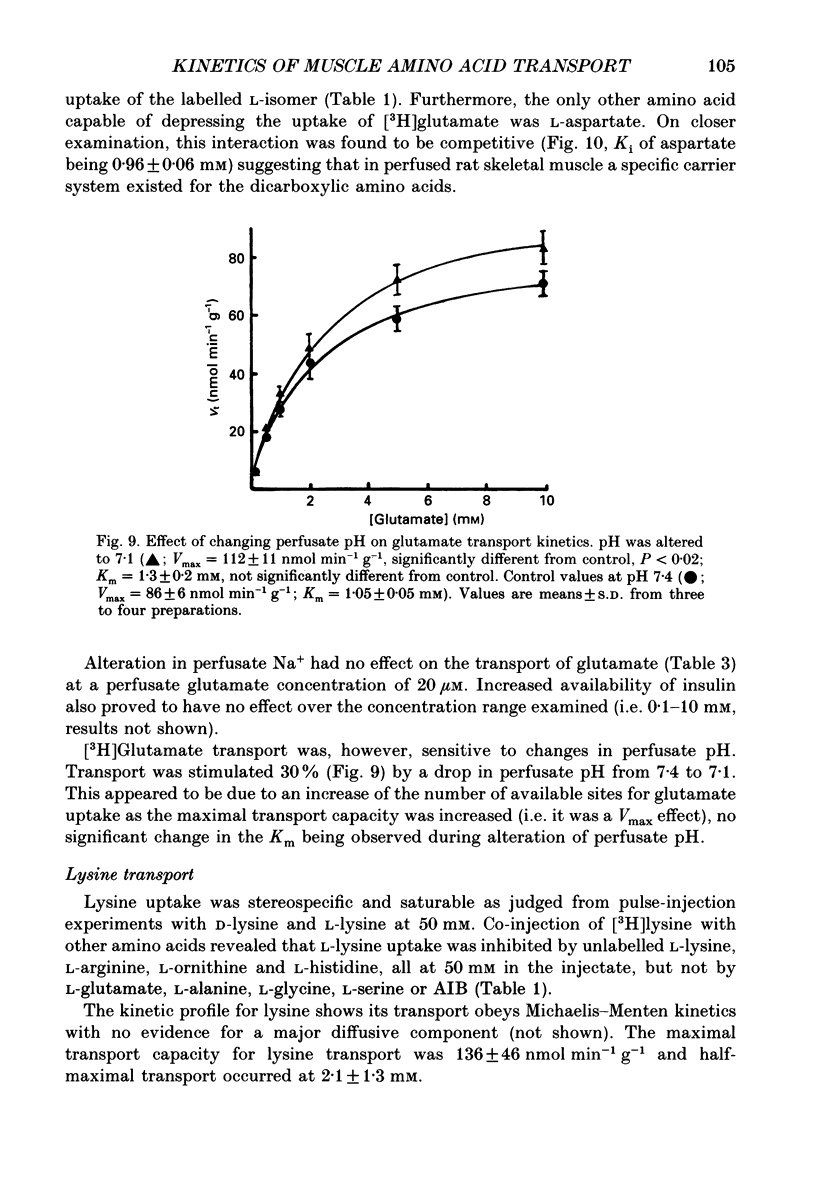
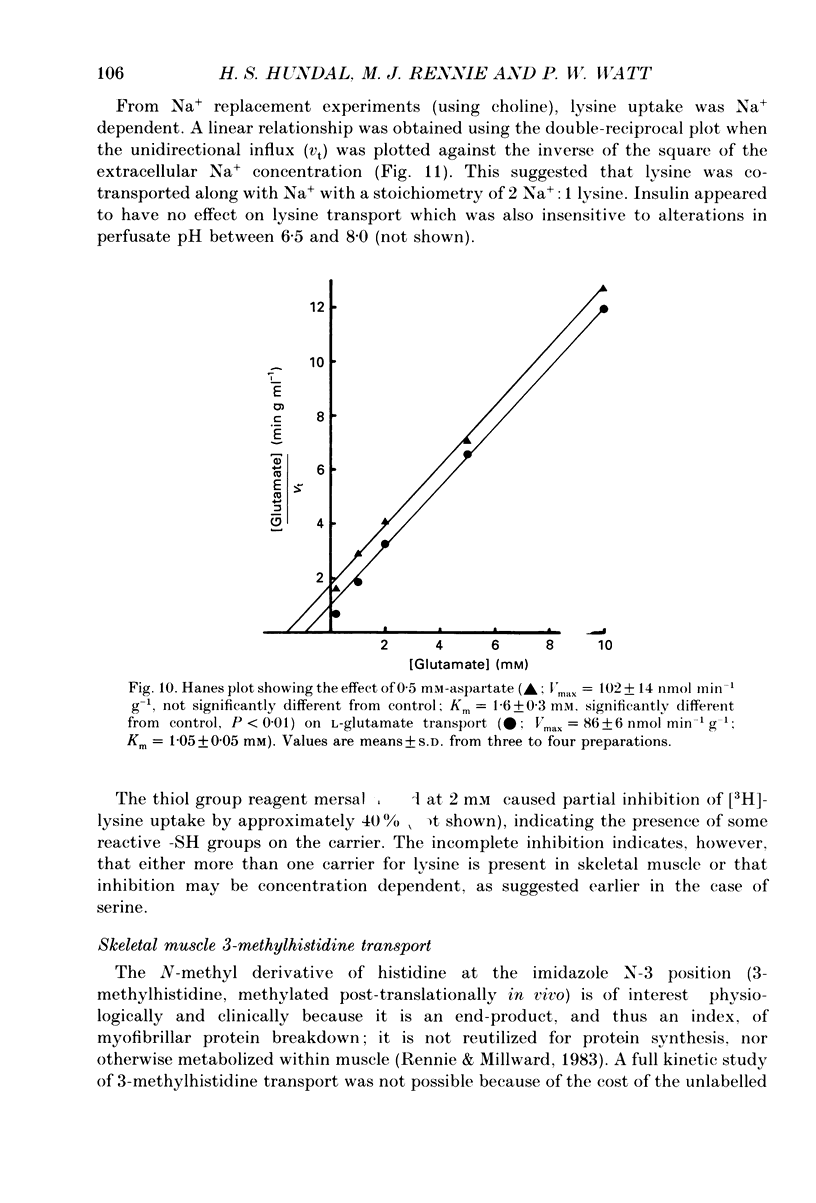
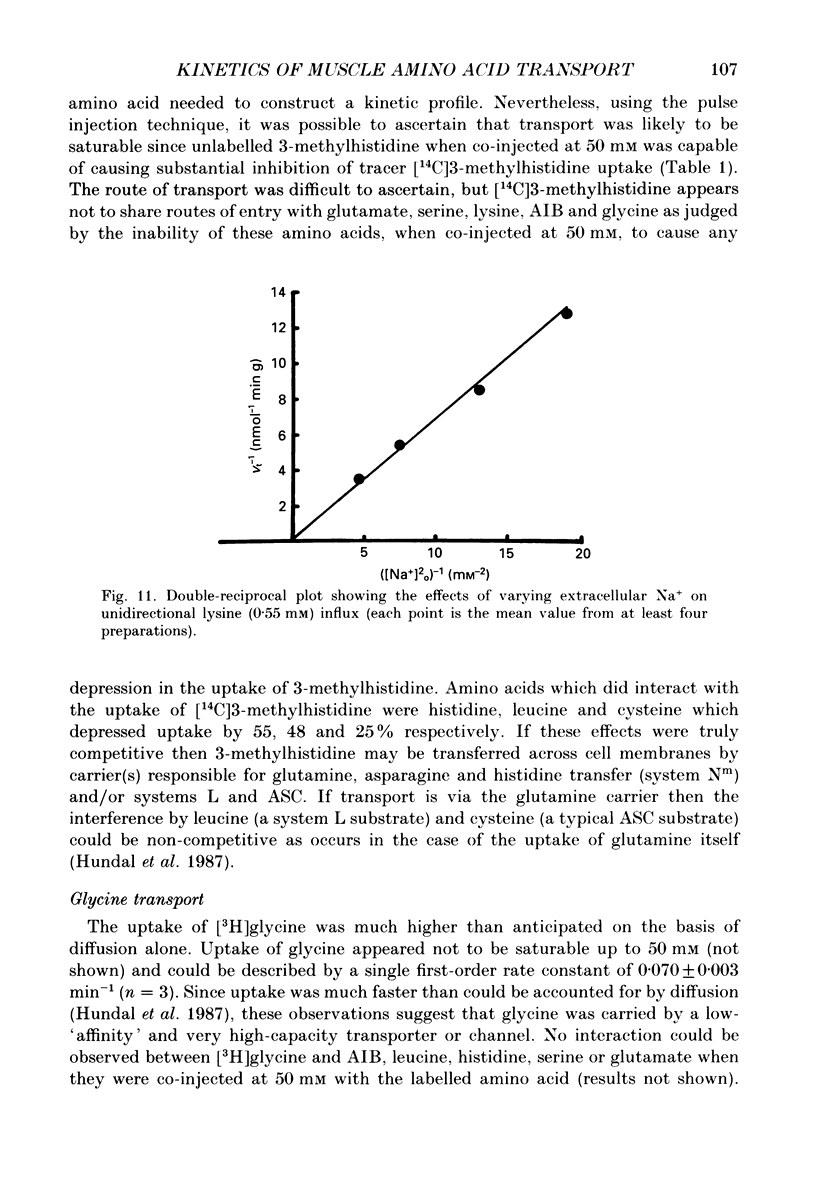
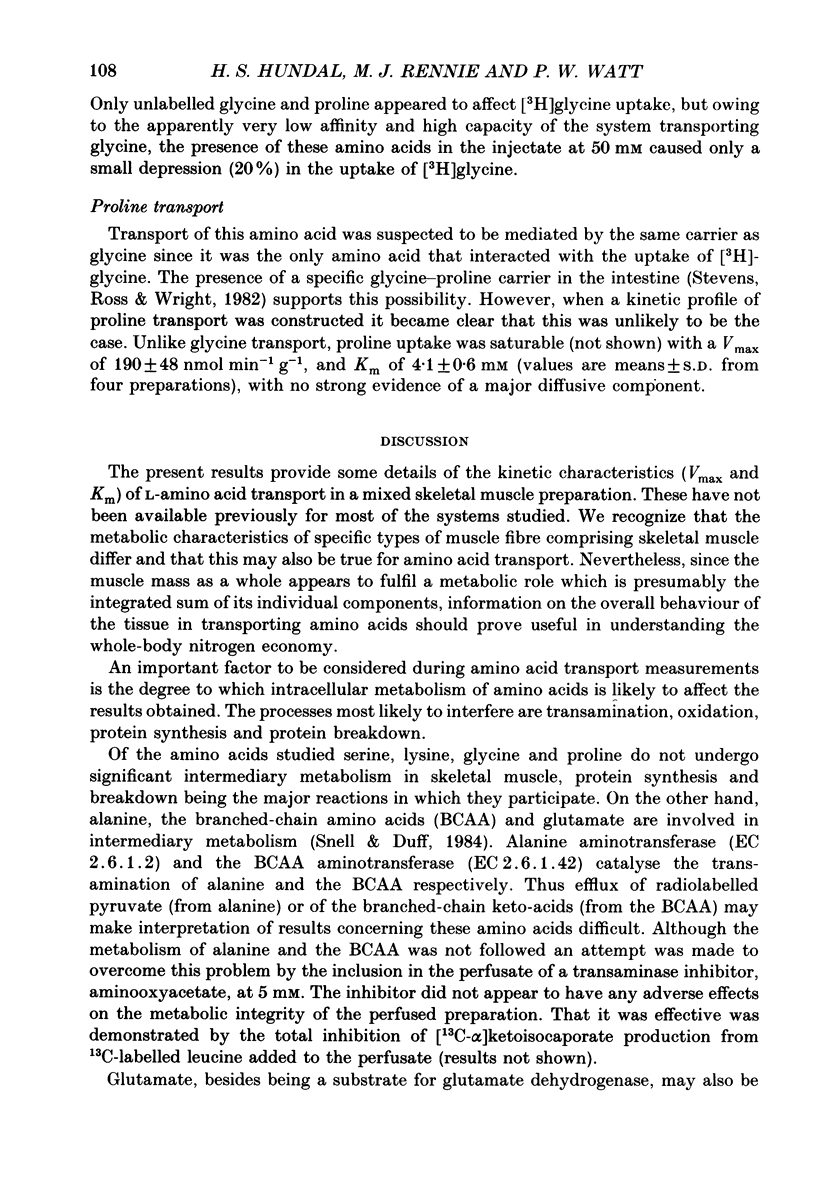
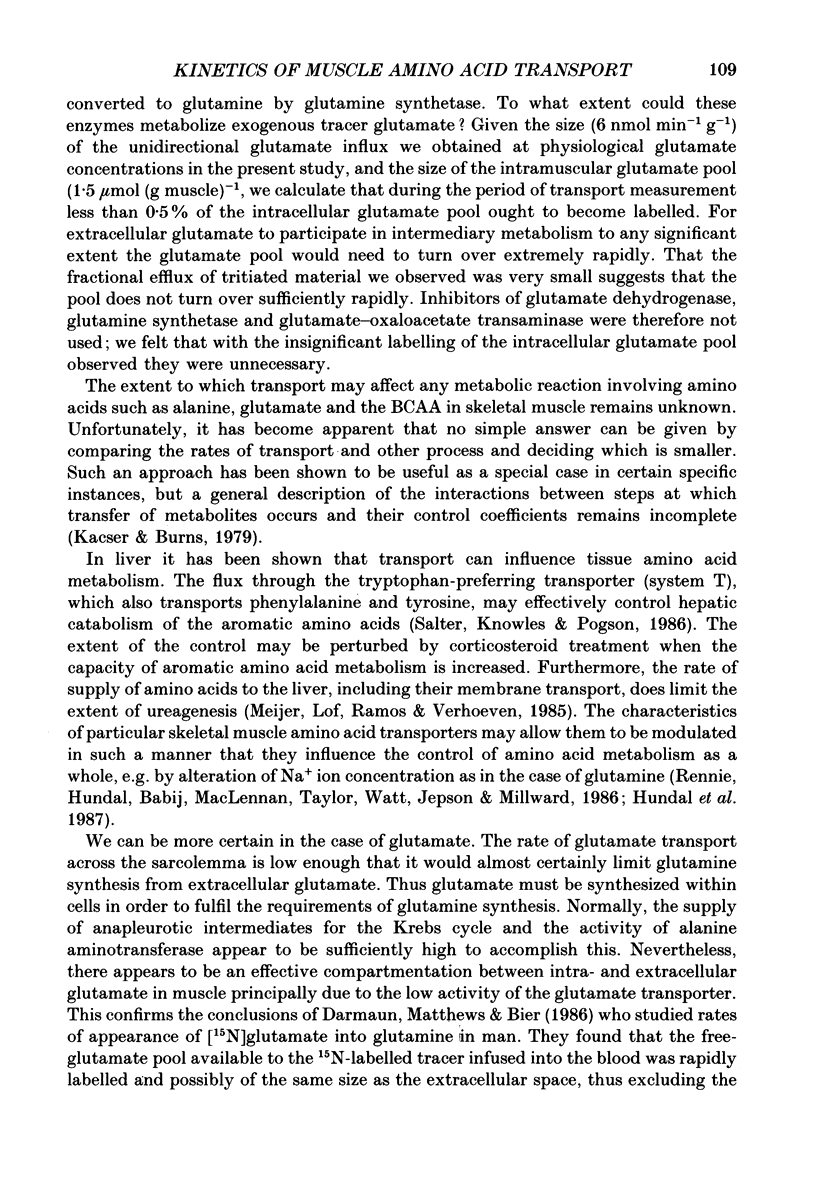
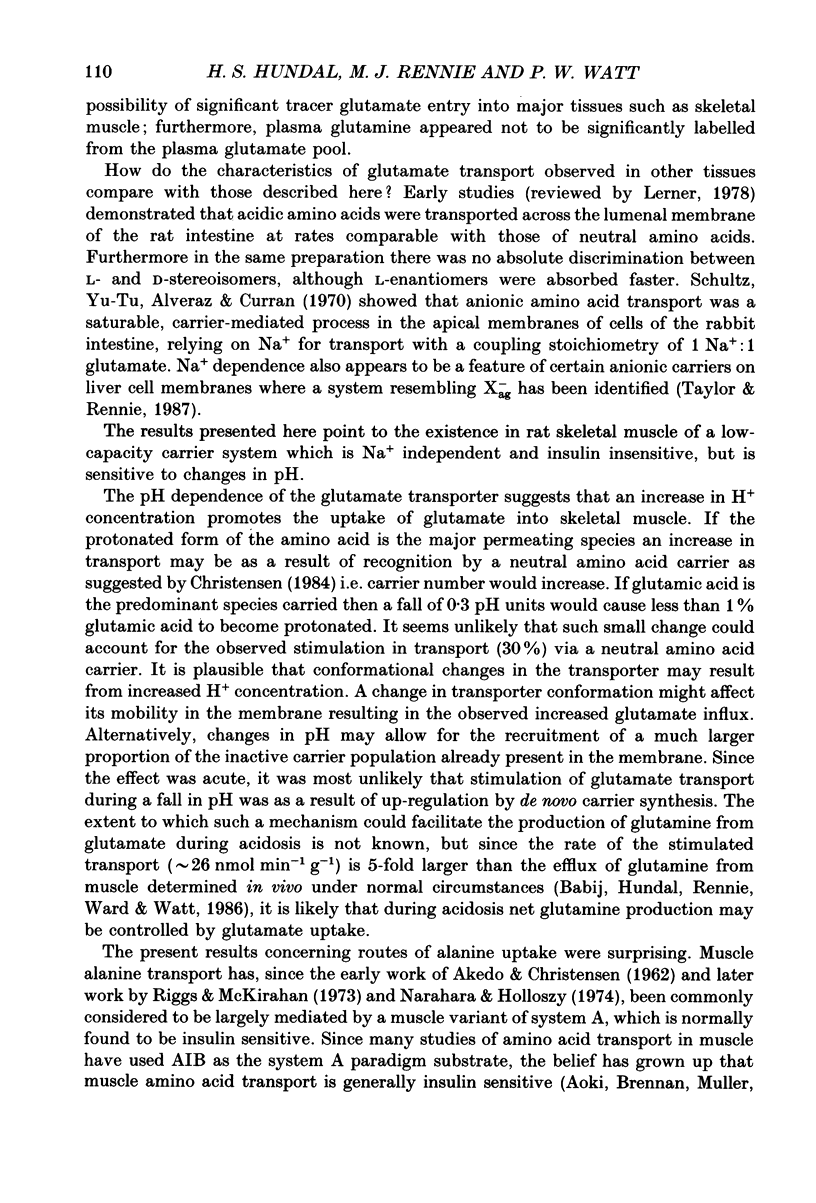
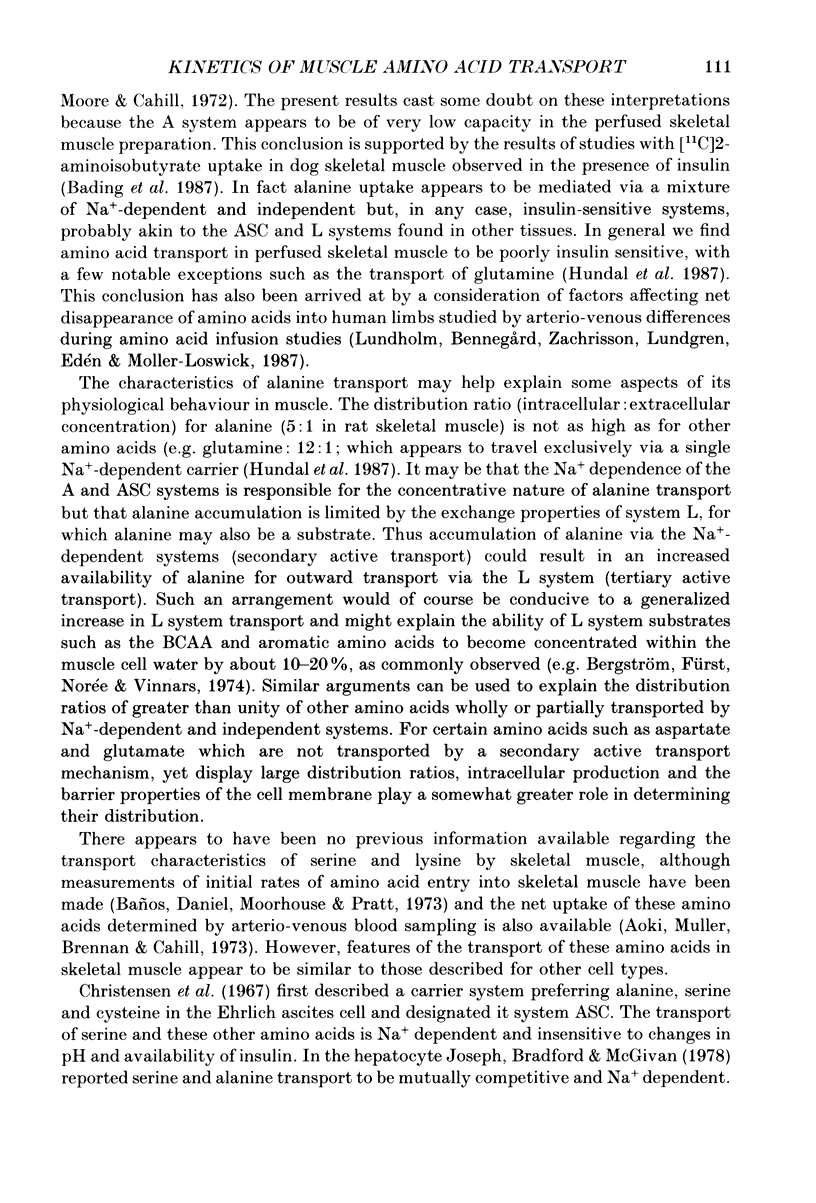
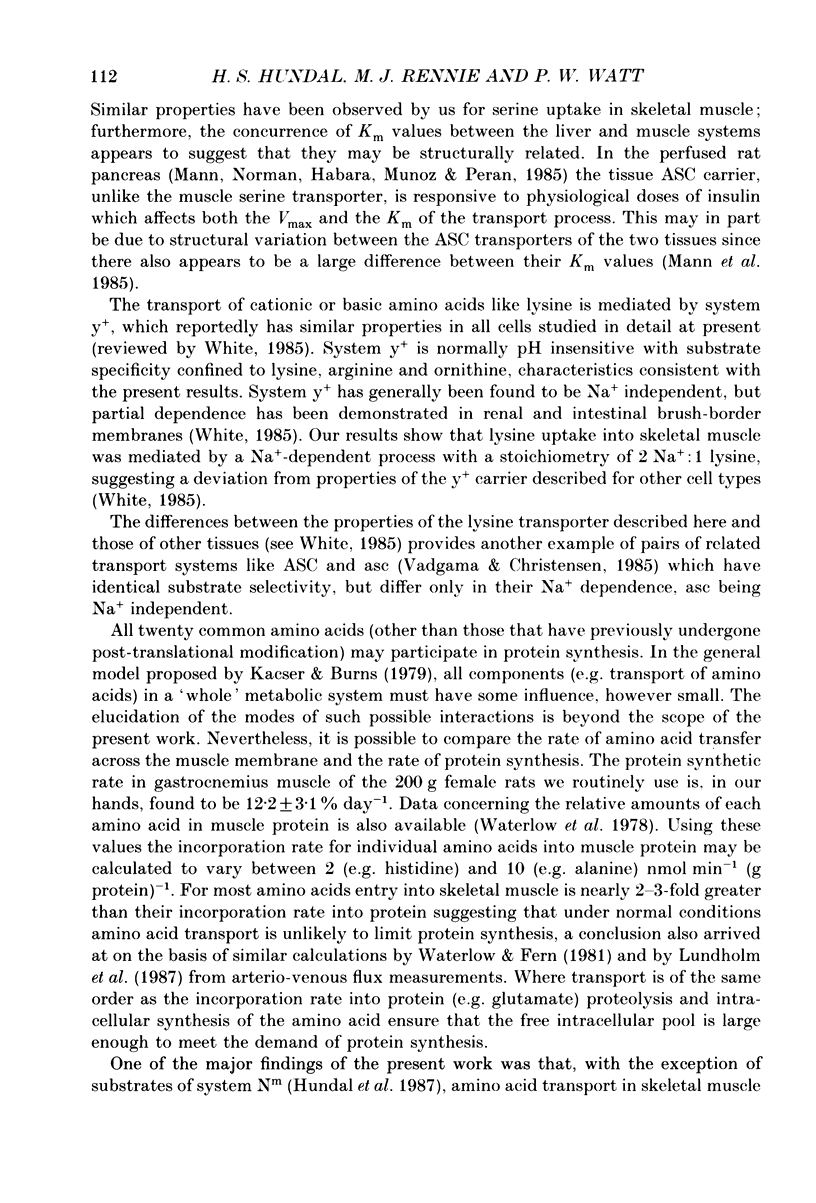
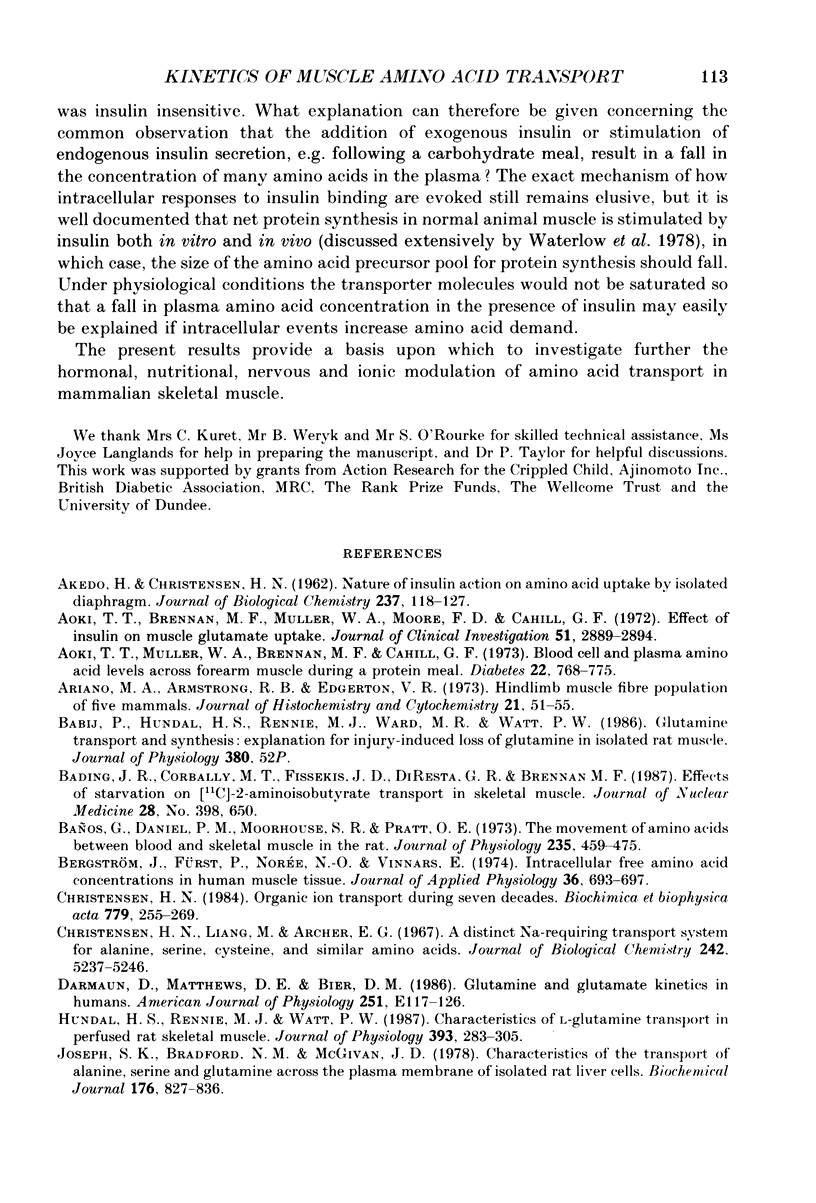
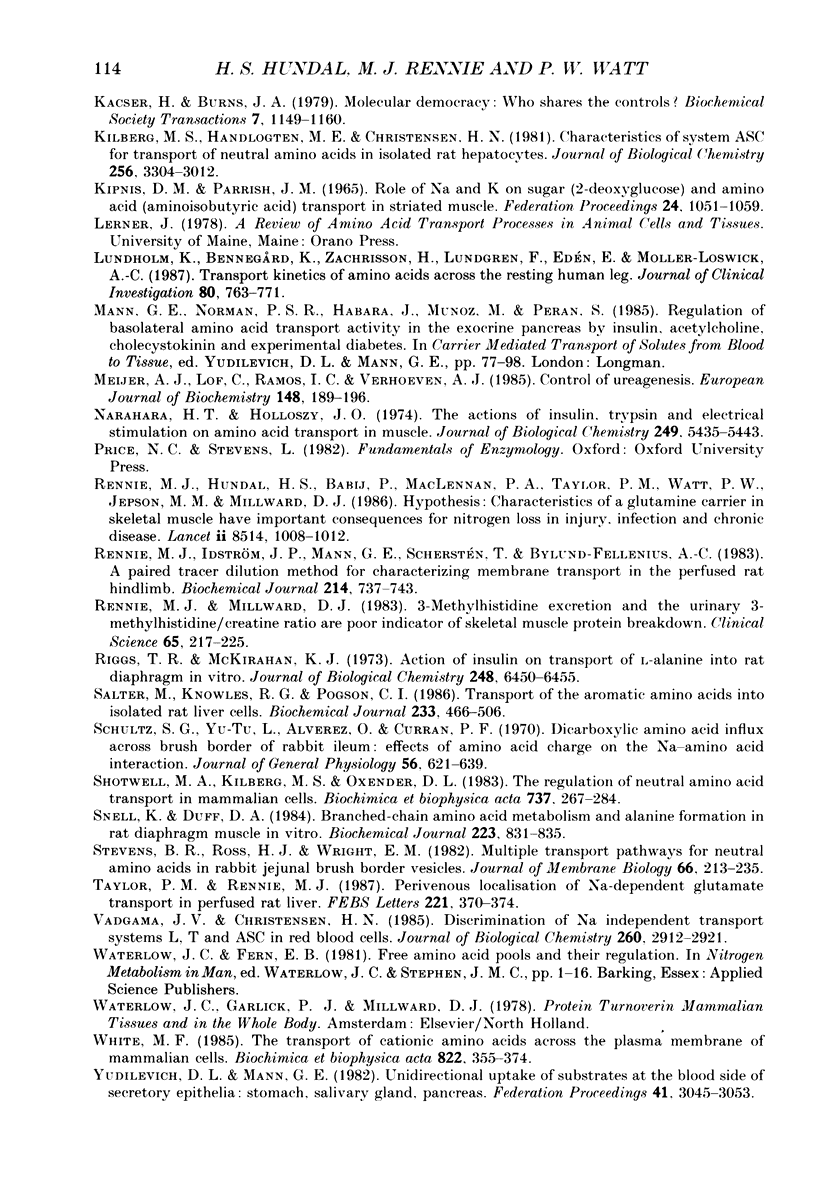
Selected References
These references are in PubMed. This may not be the complete list of references from this article.
- AKEDO H., CHRISTENSEN H. N. Nature of insulin action on amino acid uptake by the isolated diaphragm. J Biol Chem. 1962 Jan;237:118–122. [PubMed] [Google Scholar]
- Aoki T. T., Brennan M. F., Müller W. A., Moore F. D., Cahill G. F., Jr Effect of insulin on muscle glutamate uptake. Whole blood versus plasma glutamate analysis. J Clin Invest. 1972 Nov;51(11):2889–2894. doi: 10.1172/JCI107112. [DOI] [PMC free article] [PubMed] [Google Scholar]
- Aoki T. T., Muller W. A., Brennan M. F., Cahill G. F., Jr Blood cell and plasma amino acid levels across forearm muscle during a protein meal. Diabetes. 1973 Oct;22(10):768–775. doi: 10.2337/diab.22.10.768. [DOI] [PubMed] [Google Scholar]
- Ariano M. A., Armstrong R. B., Edgerton V. R. Hindlimb muscle fiber populations of five mammals. J Histochem Cytochem. 1973 Jan;21(1):51–55. doi: 10.1177/21.1.51. [DOI] [PubMed] [Google Scholar]
- Baños G., Daniel P. M., Moorhouse S. R., Pratt O. E. The movement of amino acids between blood and skeletal muscle in the rat. J Physiol. 1973 Dec;235(2):459–475. doi: 10.1113/jphysiol.1973.sp010397. [DOI] [PMC free article] [PubMed] [Google Scholar]
- Bergström J., Fürst P., Norée L. O., Vinnars E. Intracellular free amino acid concentration in human muscle tissue. J Appl Physiol. 1974 Jun;36(6):693–697. doi: 10.1152/jappl.1974.36.6.693. [DOI] [PubMed] [Google Scholar]
- Christensen H. N., Liang M., Archer E. G. A distinct Na+-requiring transport system for alanine, serine, cysteine, and similar amino acids. J Biol Chem. 1967 Nov 25;242(22):5237–5246. [PubMed] [Google Scholar]
- Christensen H. N. Organic ion transport during seven decades. The amino acids. Biochim Biophys Acta. 1984 Sep 3;779(3):255–269. doi: 10.1016/0304-4157(84)90012-1. [DOI] [PubMed] [Google Scholar]
- Hundal H. S., Rennie M. J., Watt P. W. Characteristics of L-glutamine transport in perfused rat skeletal muscle. J Physiol. 1987 Dec;393:283–305. doi: 10.1113/jphysiol.1987.sp016824. [DOI] [PMC free article] [PubMed] [Google Scholar]
- Joseph S. K., Bradford N. M., McGivan J. D. Characteristics of the transport of alanine, serine and glutamine across the plasma membrane of isolated rat liver cells. Biochem J. 1978 Dec 15;176(3):827–836. doi: 10.1042/bj1760827. [DOI] [PMC free article] [PubMed] [Google Scholar]
- Kacser H., Burns J. A. MOlecular democracy: who shares the controls? Biochem Soc Trans. 1979 Oct;7(5):1149–1160. doi: 10.1042/bst0071149. [DOI] [PubMed] [Google Scholar]
- Kilberg M. S., Handlogten M. E., Christensen H. N. Characteristics of system ASC for transport of neutral amino acids in the isolated rat hepatocyte. J Biol Chem. 1981 Apr 10;256(7):3304–3312. [PubMed] [Google Scholar]
- Kipnis D. M., Parrish J. E. Role of Na+ and K+ on sugar (2-deoxyglucose) and amino acid (alpha-aminoisobutyric acid) transport in striated muscle. Fed Proc. 1965 Sep-Oct;24(5):1051–1059. [PubMed] [Google Scholar]
- Lundholm K., Bennegård K., Zachrisson H., Lundgren F., Edén E., Möller-Loswick A. C. Transport kinetics of amino acids across the resting human leg. J Clin Invest. 1987 Sep;80(3):763–771. doi: 10.1172/JCI113132. [DOI] [PMC free article] [PubMed] [Google Scholar]
- Meijer A. J., Lof C., Ramos I. C., Verhoeven A. J. Control of ureogenesis. Eur J Biochem. 1985 Apr 1;148(1):189–196. doi: 10.1111/j.1432-1033.1985.tb08824.x. [DOI] [PubMed] [Google Scholar]
- Narahara H. T., Holloszy J. O. The actions of insulin, trypsin, and electrical stimulation on amino acid transport in muscle. J Biol Chem. 1974 Sep 10;249(17):5435–5443. [PubMed] [Google Scholar]
- Rennie M. J., Hundal H. S., Babij P., MacLennan P., Taylor P. M., Watt P. W., Jepson M. M., Millward D. J. Characteristics of a glutamine carrier in skeletal muscle have important consequences for nitrogen loss in injury, infection, and chronic disease. Lancet. 1986 Nov 1;2(8514):1008–1012. doi: 10.1016/s0140-6736(86)92617-6. [DOI] [PubMed] [Google Scholar]
- Rennie M. J., Idström J. P., Mann G. E., Scherstén T., Bylund-Fellenius A. C. A paired-tracer dilution method for characterizing membrane transport in the perfused rat hindlimb. Effects of insulin, feeding and fasting on the kinetics of sugar transport. Biochem J. 1983 Sep 15;214(3):737–743. doi: 10.1042/bj2140737. [DOI] [PMC free article] [PubMed] [Google Scholar]
- Rennie M. J., Millward D. J. 3-Methylhistidine excretion and the urinary 3-methylhistidine/creatinine ratio are poor indicators of skeletal muscle protein breakdown. Clin Sci (Lond) 1983 Sep;65(3):217–225. doi: 10.1042/cs0650217. [DOI] [PubMed] [Google Scholar]
- Riggs T. R., McKirahan K. J. Action of insulin on transport of L-alanine into rat diaphragm in vitro. Evidence that the hormone affects only one neutral amino acid transport system. J Biol Chem. 1973 Sep 25;248(18):6450–6455. [PubMed] [Google Scholar]
- Salter M., Knowles R. G., Pogson C. I. Transport of the aromatic amino acids into isolated rat liver cells. Properties of uptake by two distinct systems. Biochem J. 1986 Jan 15;233(2):499–506. doi: 10.1042/bj2330499. [DOI] [PMC free article] [PubMed] [Google Scholar]
- Schultz S. G., Alvarez O. O., Curran P. F., Yu-Tu L. Dicarboxylic amino acid influx across brush border of rabbit ileum. Effects of amino acid charge on the sodium-amino acid interaction. J Gen Physiol. 1970 Nov;56(5):621–639. doi: 10.1085/jgp.56.5.621. [DOI] [PMC free article] [PubMed] [Google Scholar]
- Shotwell M. A., Kilberg M. S., Oxender D. L. The regulation of neutral amino acid transport in mammalian cells. Biochim Biophys Acta. 1983 May 24;737(2):267–284. doi: 10.1016/0304-4157(83)90003-5. [DOI] [PubMed] [Google Scholar]
- Snell K., Duff D. A. Branched-chain amino acid metabolism and alanine formation in rat diaphragm muscle in vitro. Effects of dichloroacetate. Biochem J. 1984 Nov 1;223(3):831–835. doi: 10.1042/bj2230831. [DOI] [PMC free article] [PubMed] [Google Scholar]
- Stevens B. R., Ross H. J., Wright E. M. Multiple transport pathways for neutral amino acids in rabbit jejunal brush border vesicles. J Membr Biol. 1982;66(3):213–225. doi: 10.1007/BF01868496. [DOI] [PubMed] [Google Scholar]
- Taylor P. M., Rennie M. J. Perivenous localisation of Na-dependent glutamate transport in perfused rat liver. FEBS Lett. 1987 Sep 14;221(2):370–374. doi: 10.1016/0014-5793(87)80958-4. [DOI] [PubMed] [Google Scholar]
- Vadgama J. V., Christensen H. N. Discrimination of Na+-independent transport systems L, T, and asc in erythrocytes. Na+ independence of the latter a consequence of cell maturation? J Biol Chem. 1985 Mar 10;260(5):2912–2921. [PubMed] [Google Scholar]
- White M. F. The transport of cationic amino acids across the plasma membrane of mammalian cells. Biochim Biophys Acta. 1985 Dec 9;822(3-4):355–374. doi: 10.1016/0304-4157(85)90015-2. [DOI] [PubMed] [Google Scholar]
- Yudilevich D. L., Mann G. E. Unidirectional uptake of substrates at the blood side of secretory epithelia: stomach, salivary gland, pancreas. Fed Proc. 1982 Dec;41(14):3045–3053. [PubMed] [Google Scholar]