Abstract
1. Intracellular recordings were made from antidromically identified sympathetic preganglionic neurones (SPNs) in transverse sections of thoraco-lumbar spinal cord from neonatal (12-22 day) rats. 2. Two types of hyperpolarizing (inhibitory) postsynaptic potentials (IPSPs) were recorded in the SPNs. The first type, which we have termed unitary IPSPs, were small, discrete IPSPs that occurred spontaneously and also following chemical or electrical stimulation applied to the spinal cord slices. The second type IPSP was a hyperpolarizing response evoked by either dorsal or ventral root stimulation. 3. Spontaneously occurring unitary IPSPs had an amplitude of 1 to 5 mV, and reversal potential of -60 to -75 mV; they were reversibly abolished by low Ca2+, tetrodotoxin (TTX) or strychnine but not by bicuculline and picrotoxin. 4. Pressure application of N-methyl-D-aspartate (NMDA), an excitatory amino SPNs; these were abolished by either strychnine or by the NMDA receptor antagonist D-2-amino-5-phosphonovalerate. Furthermore, electrical stimulation of dorsal rootlets elicited in several SPNs the discharge of strychnine-sensitive unitary IPSPs. 5. Electrical stimulation applied to dorsal or ventral rootlets elicited in nineteen and eight SPNs, respectively, an IPSP of larger amplitude (5 to 15 mV). The IPSP exhibited a reversal potential of -60 to 75 mV; it was changed to a depolarizing response in a low [Cl-]o solution, but was not significantly affected in a low [K+]o. Strychnine but not bicuculline or picrotoxin reversibly blocked the IPSPs in nearly all the SPNs. Additionally, hexamethonium and d-tubocurarine antagonized the IPSPs evoked by ventral but not by dorsal root stimulations. 6. Our results suggest that unitary and evoked IPSPs recorded in SPNs are due primarily to an increase of Cl- conductance by glycine or a glycine-like substance, released from interneurones, that can be activated by NMDA. Furthermore, IPSPs evoked by ventral root stimulation appear to represent a disynaptic event whereby nicotinic activation of a glycine-releasing interneurone results in a release of the inhibitory transmitter; this is then analogous to the Renshaw cell circuitry of the spinal motoneurones.
Full text
PDF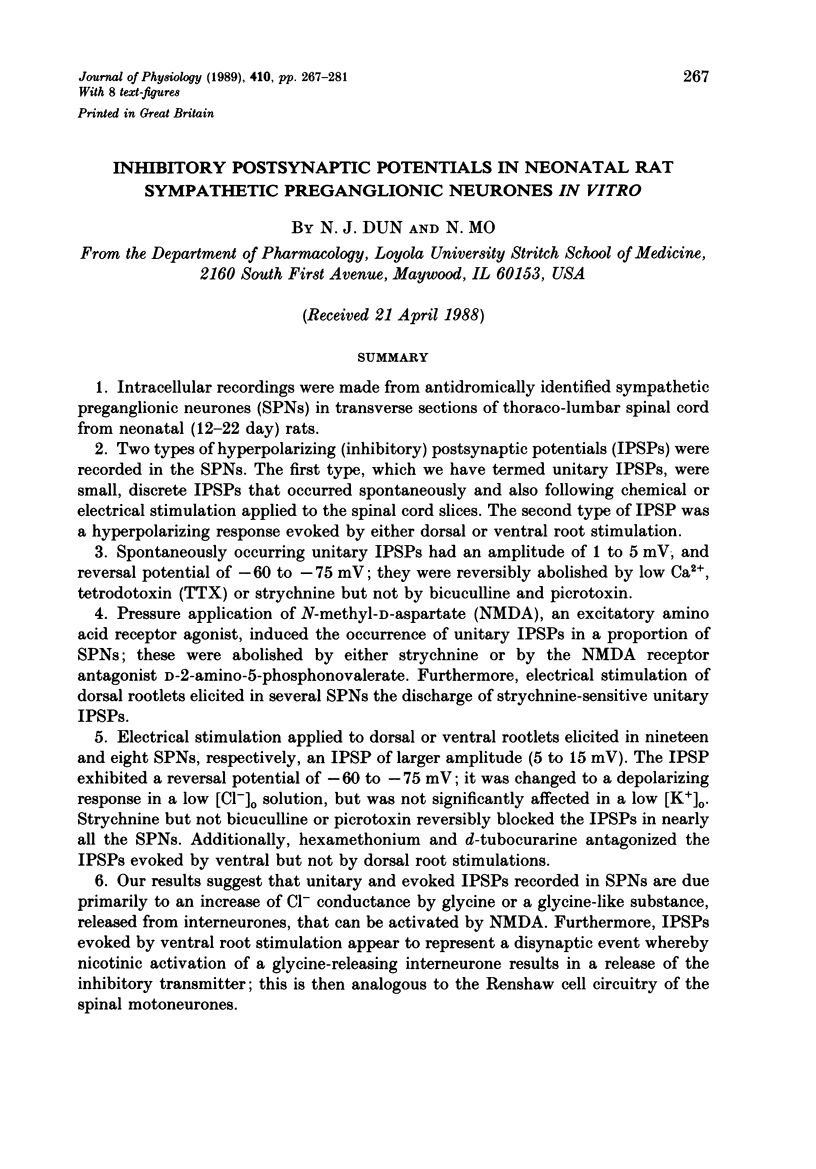
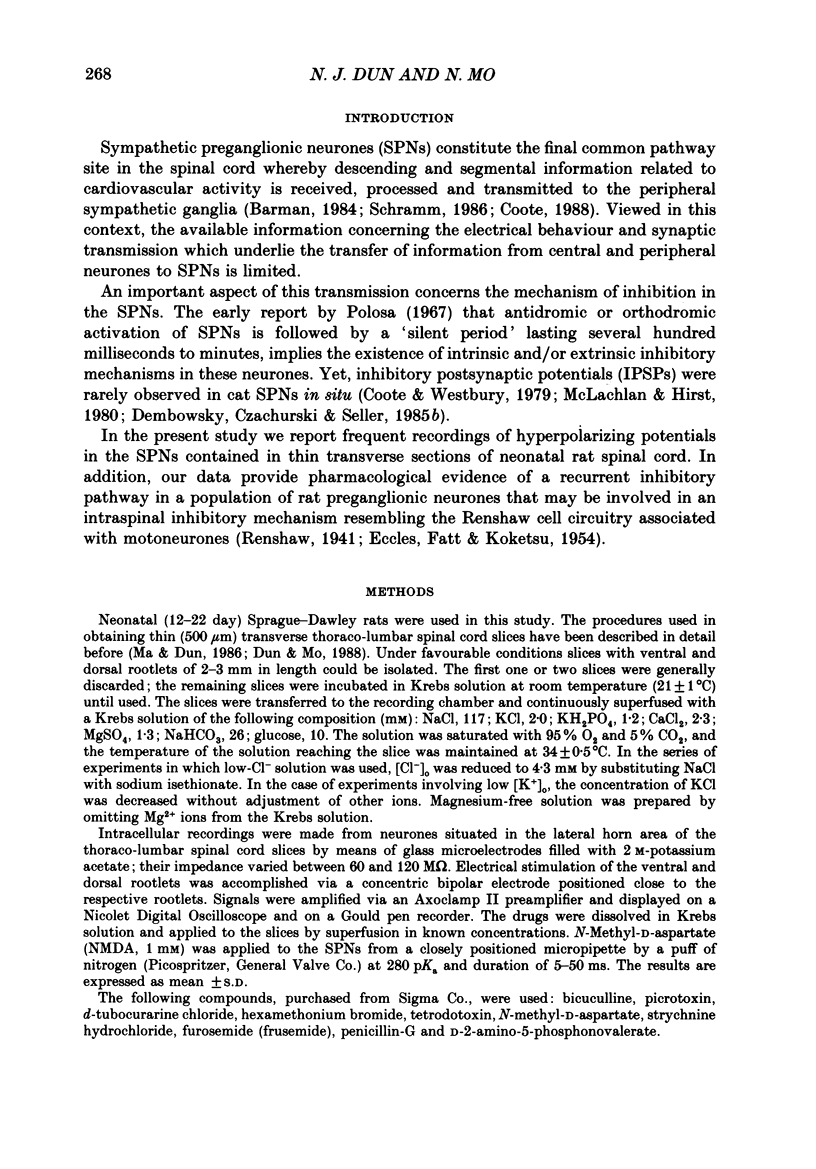
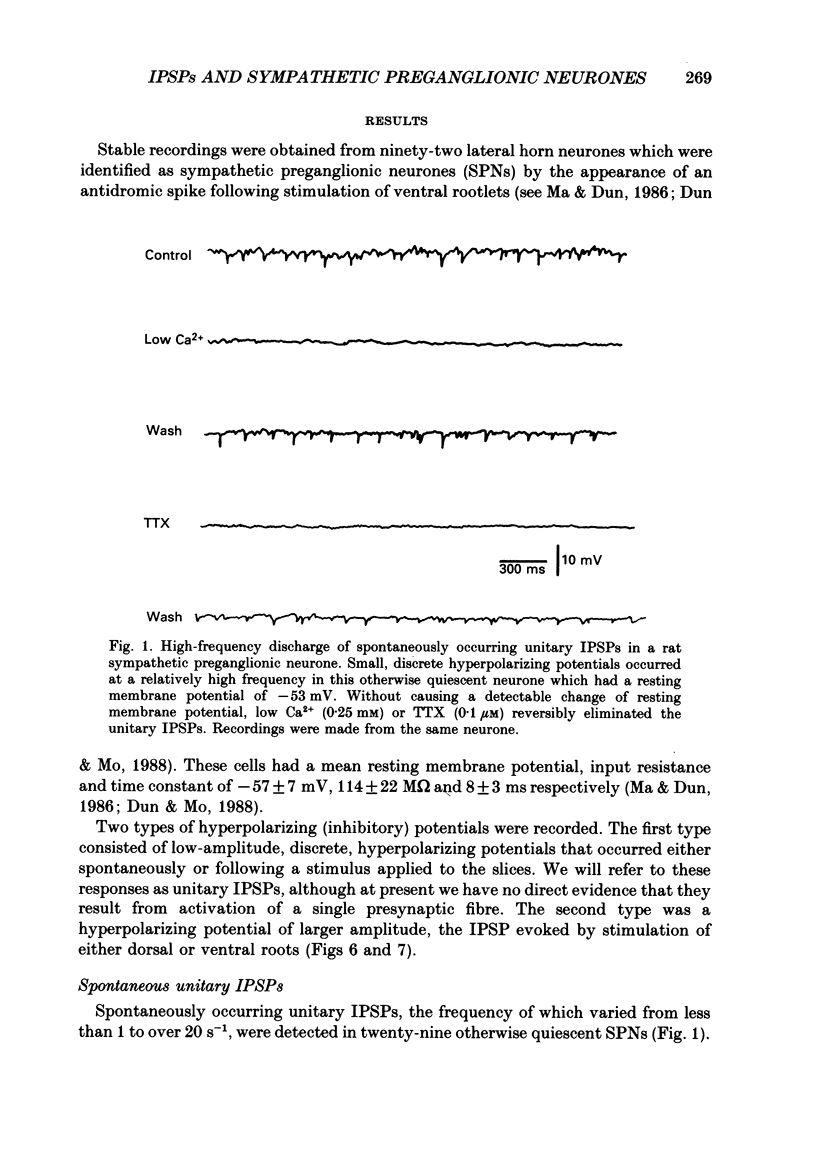
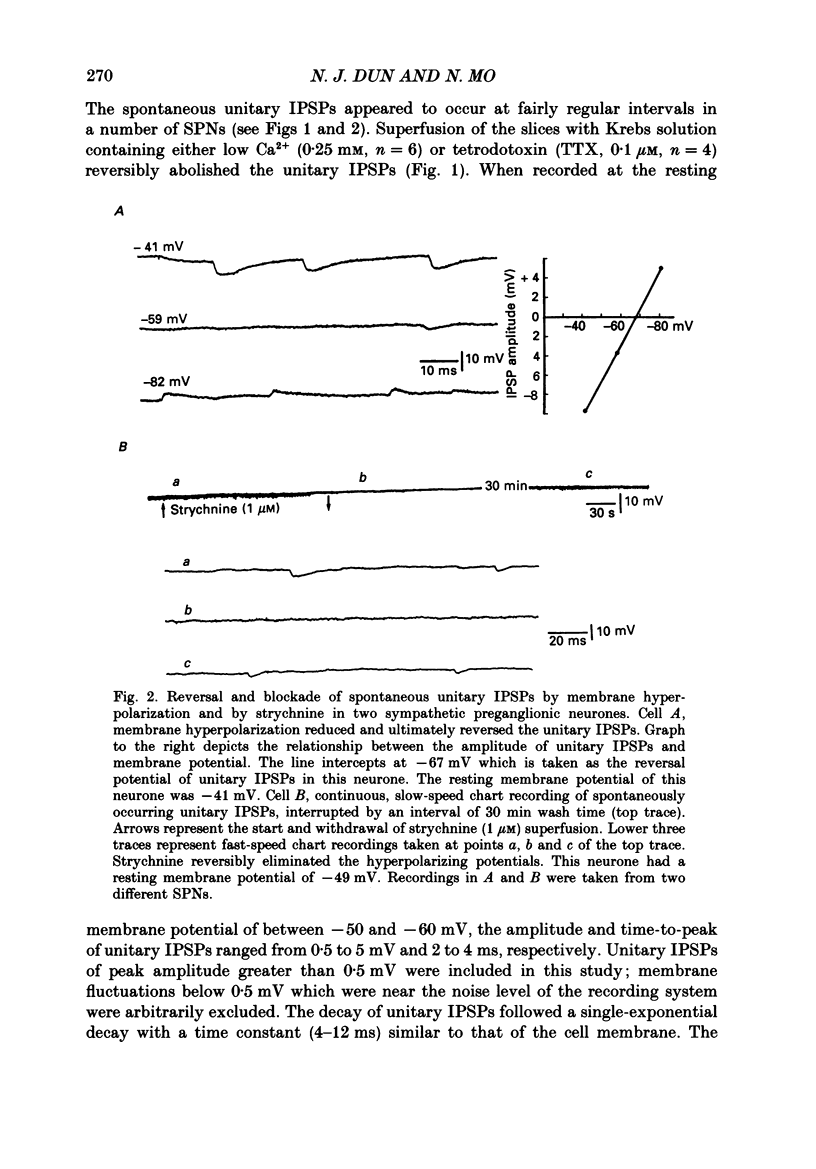
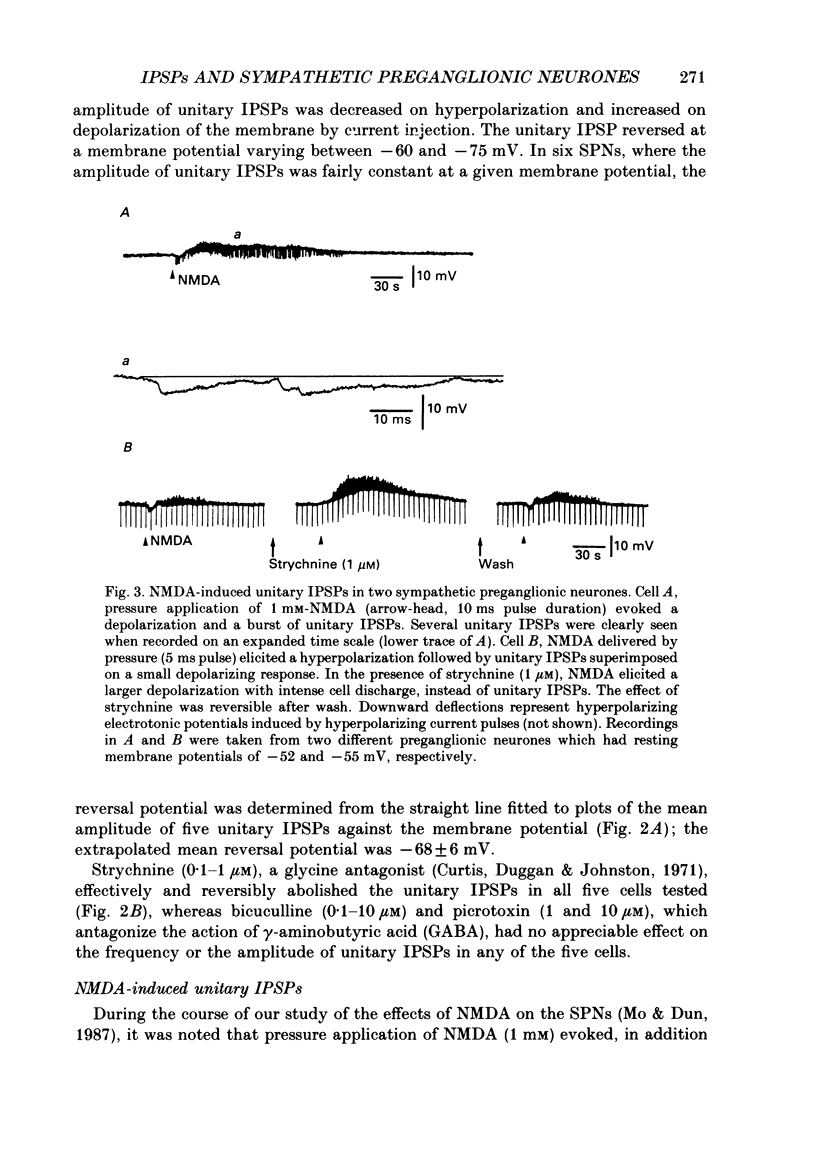
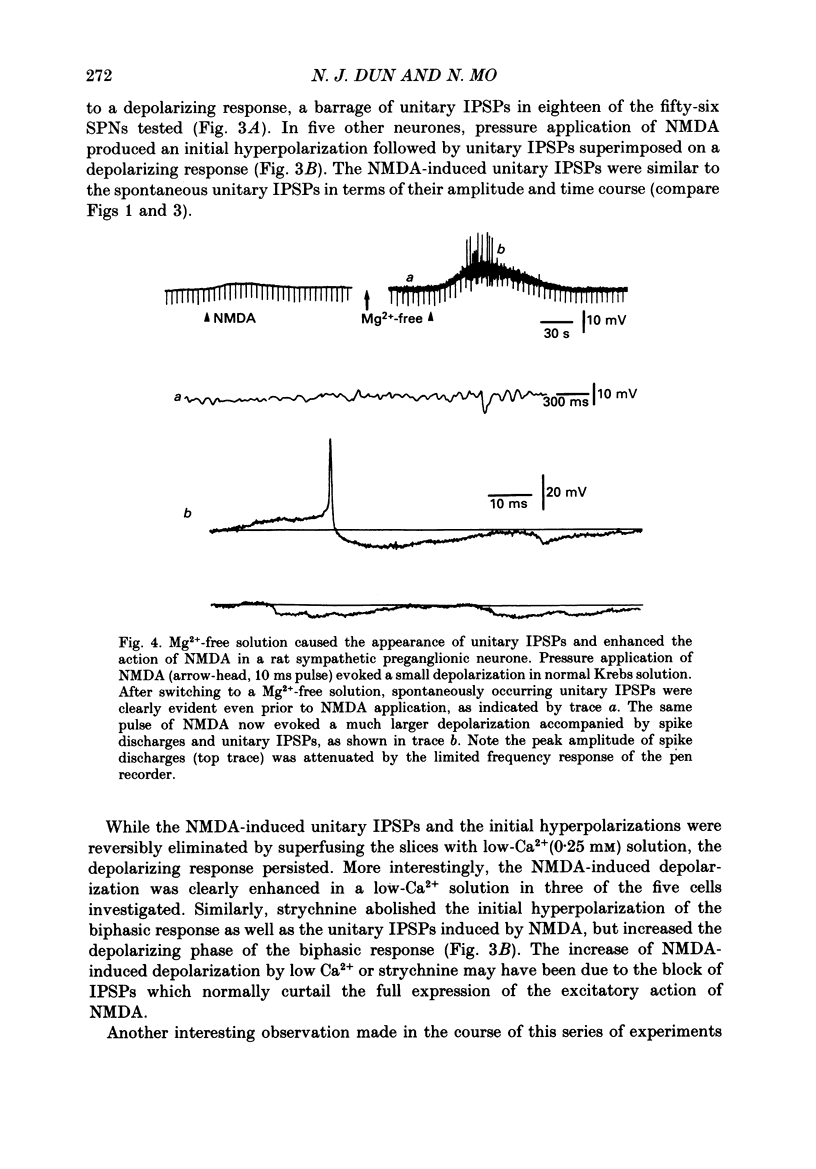
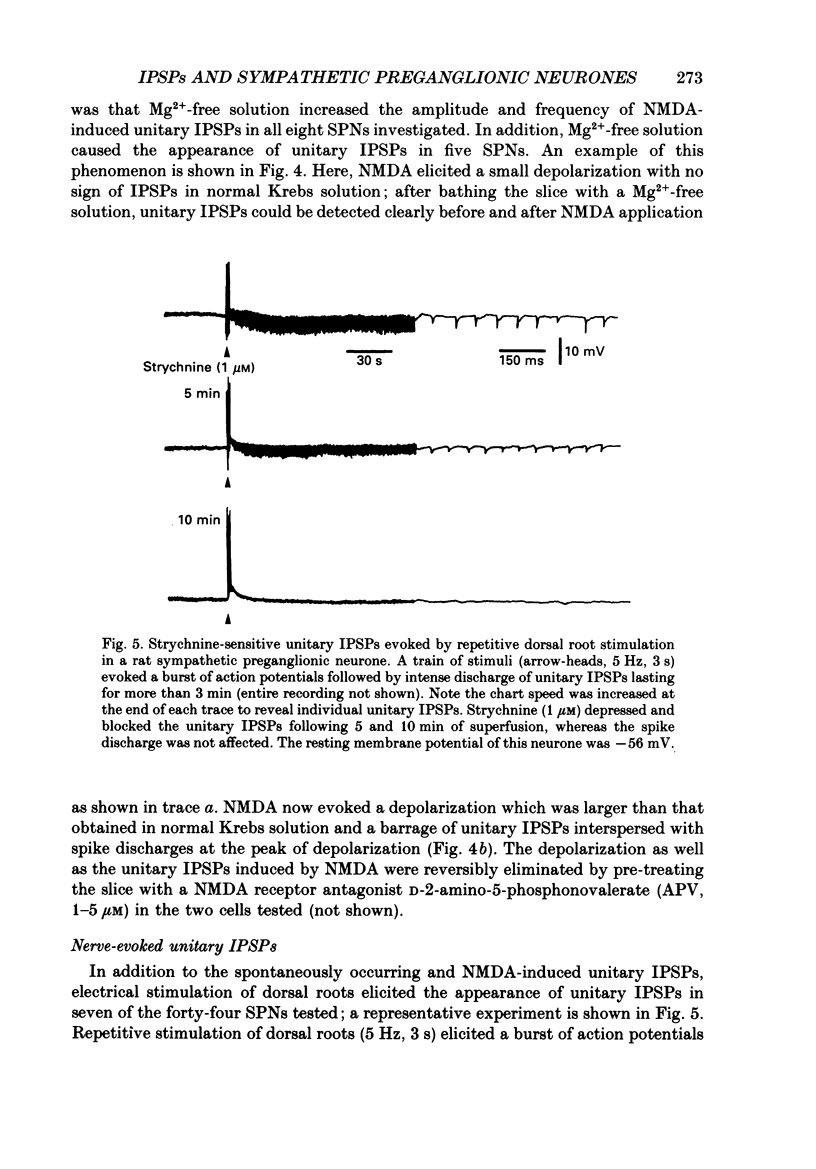
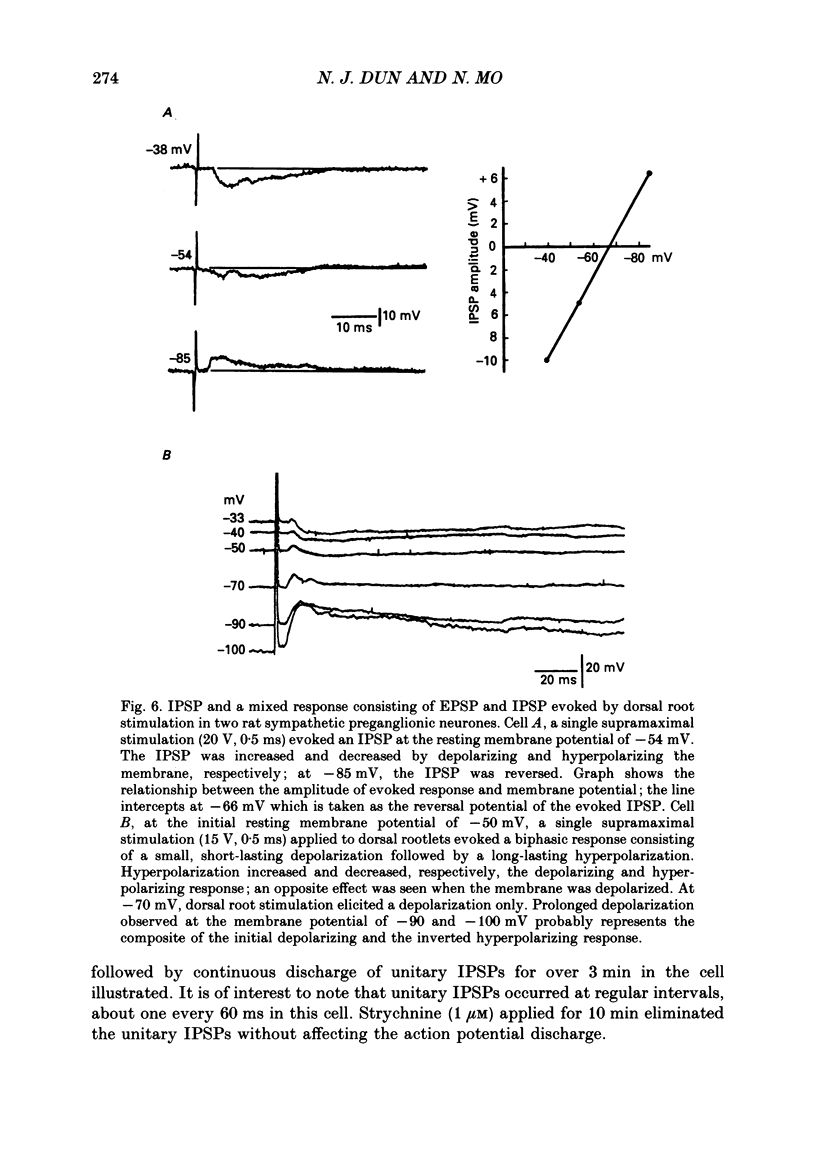
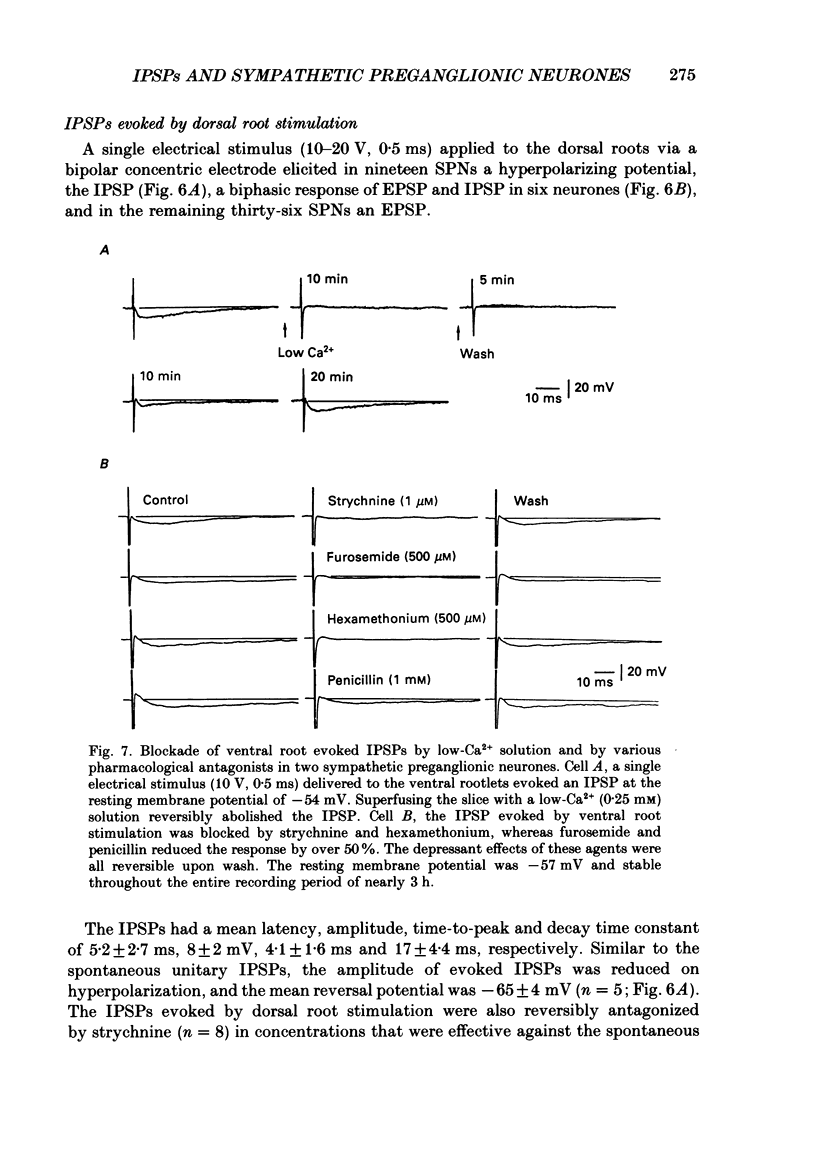
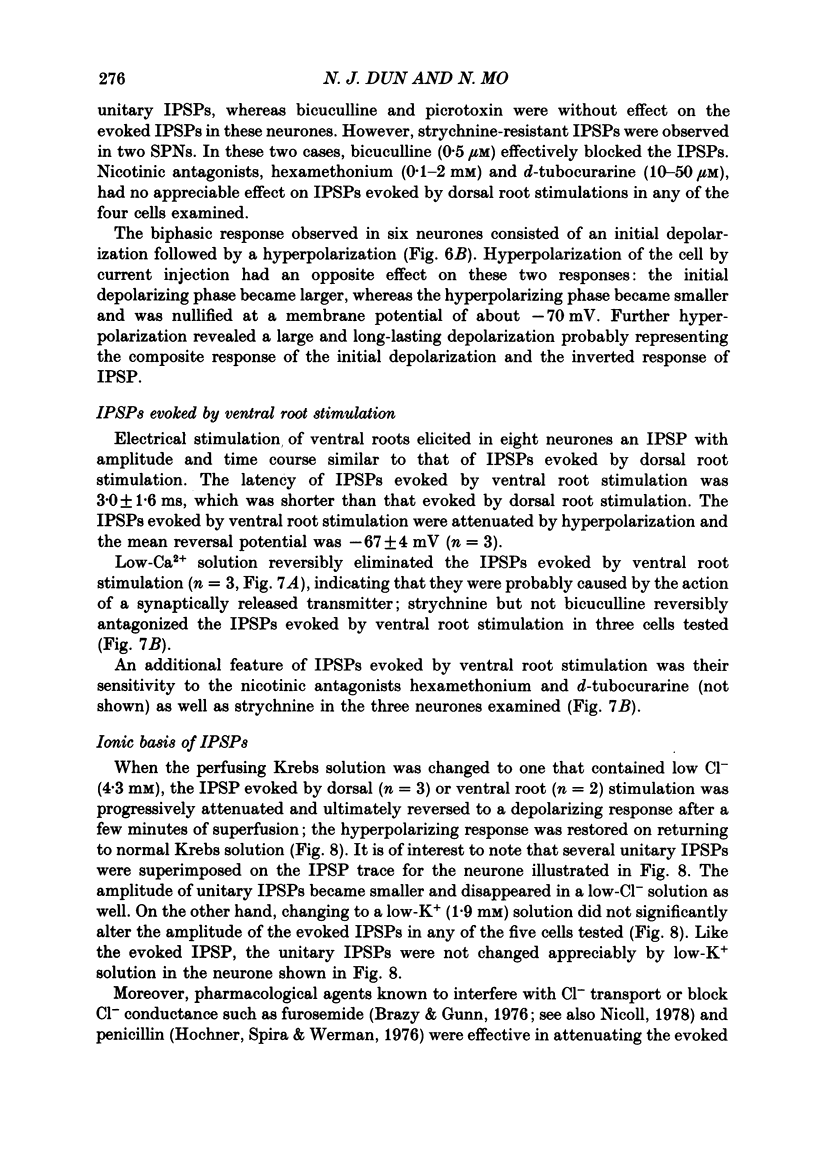
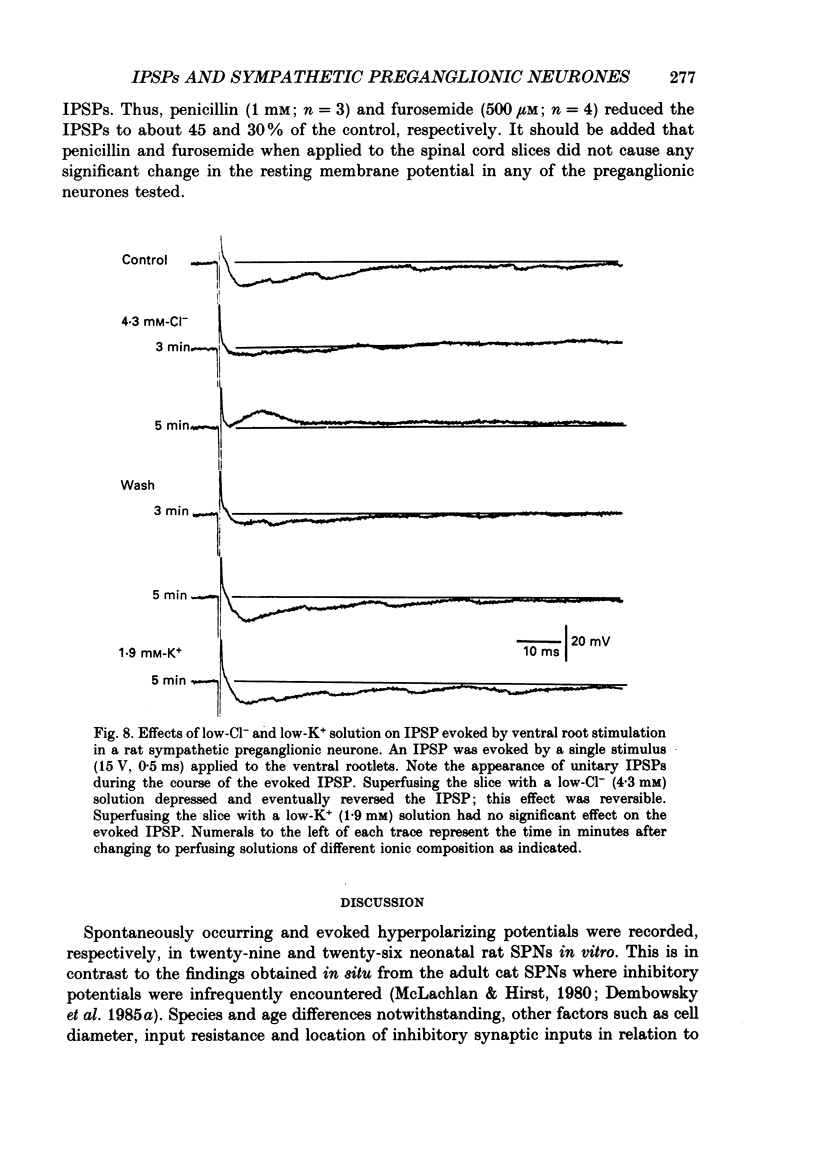
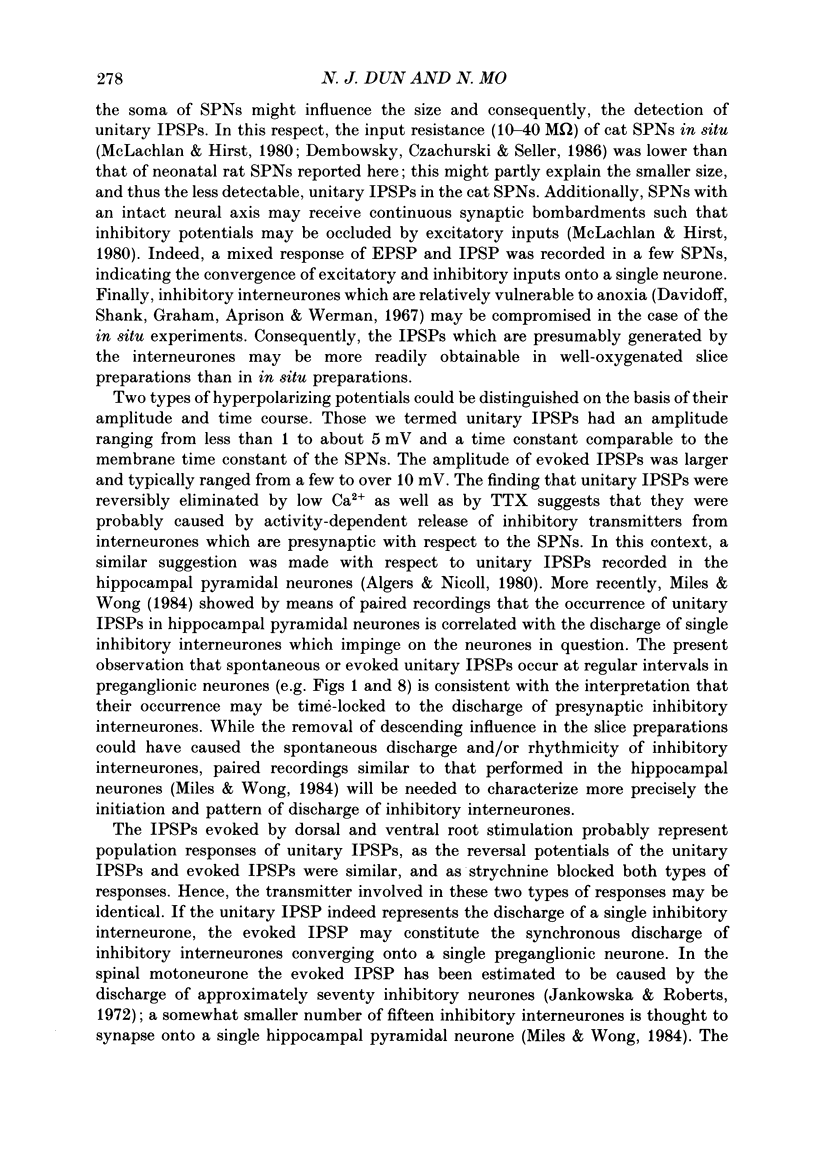
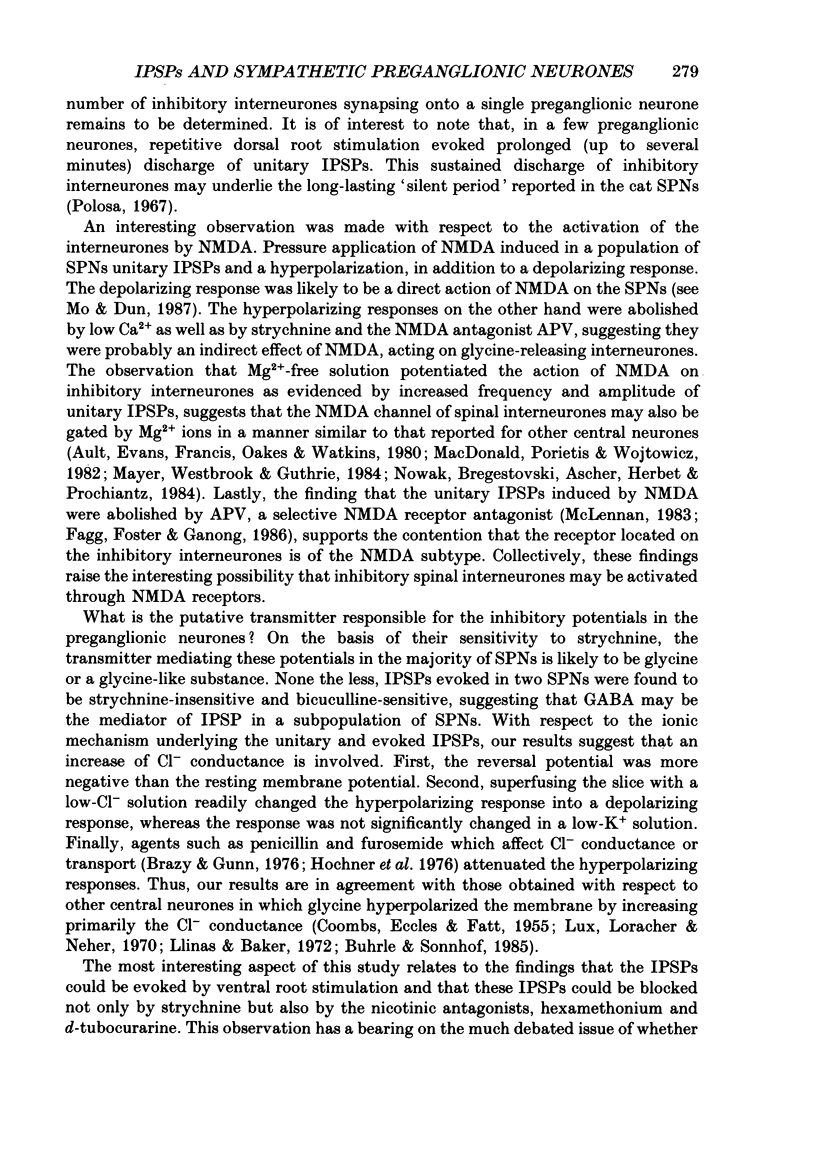
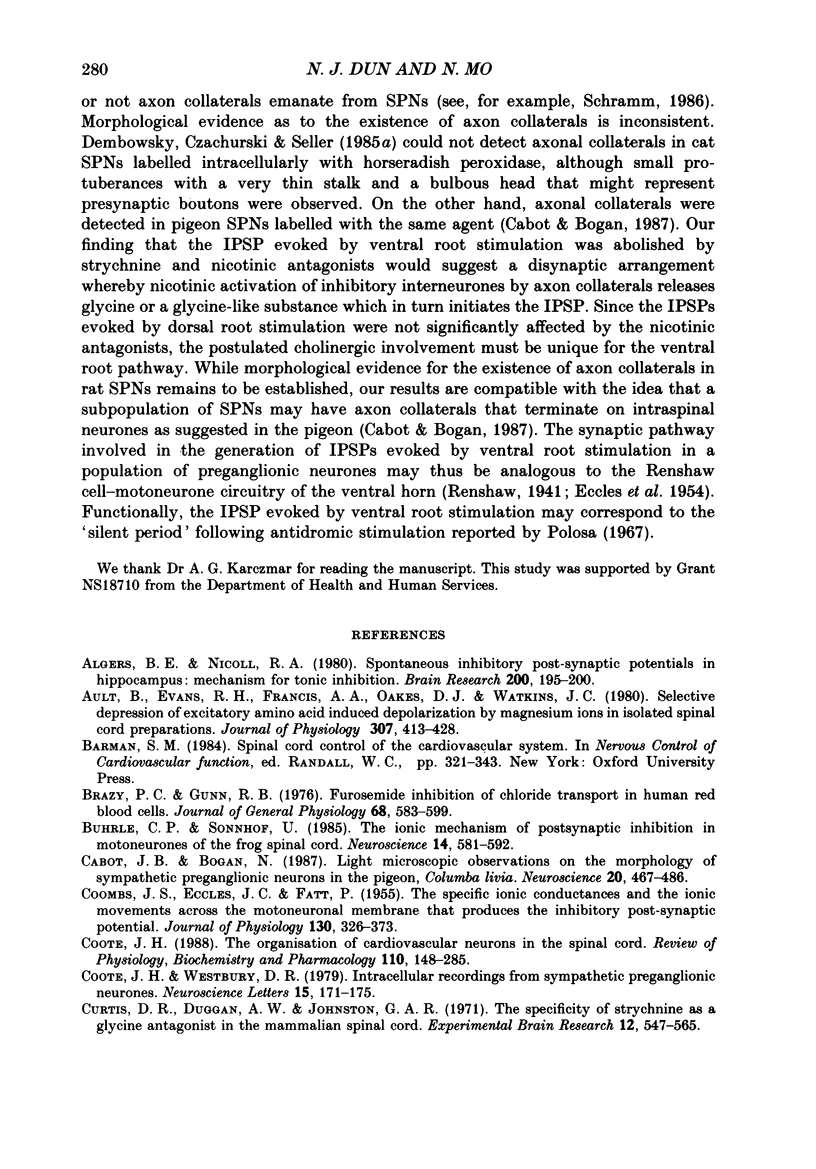
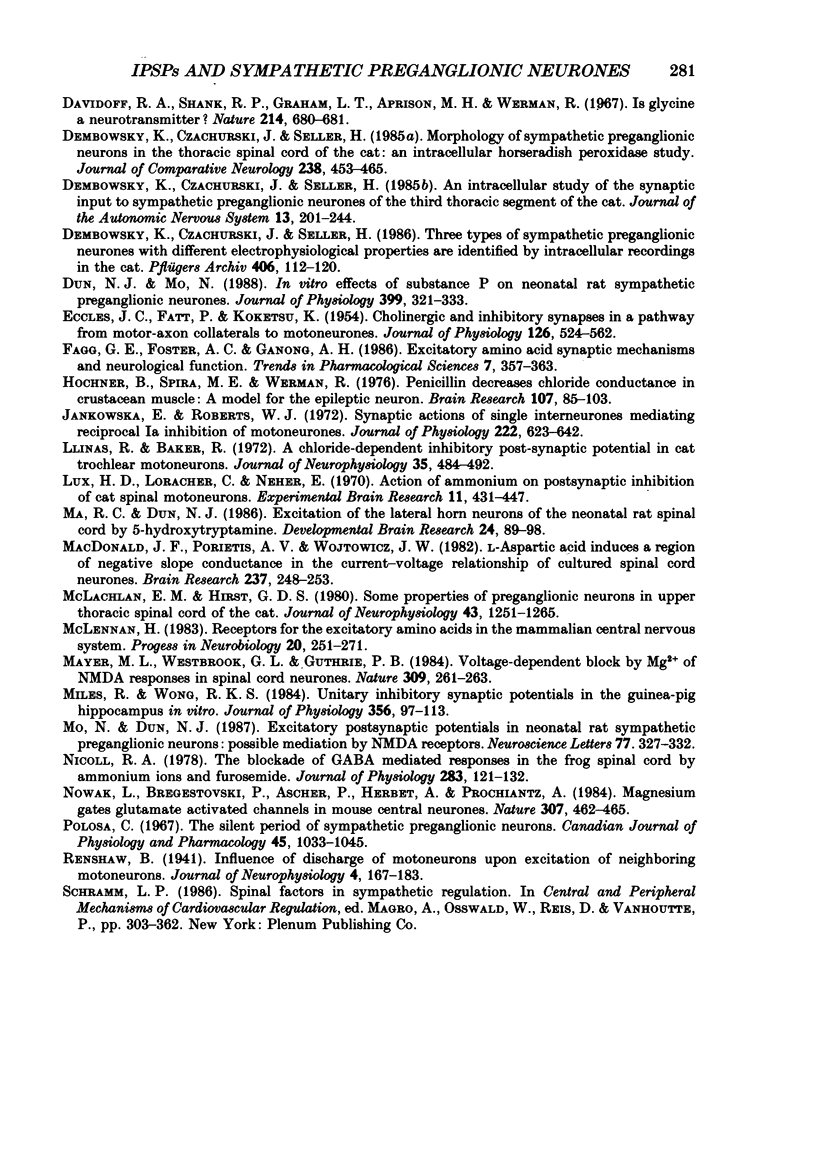
Selected References
These references are in PubMed. This may not be the complete list of references from this article.
- Alger B. E., Nicoll R. A. Spontaneous inhibitory post-synaptic potentials in hippocampus: mechanism for tonic inhibition. Brain Res. 1980 Oct 27;200(1):195–200. doi: 10.1016/0006-8993(80)91108-7. [DOI] [PubMed] [Google Scholar]
- Ault B., Evans R. H., Francis A. A., Oakes D. J., Watkins J. C. Selective depression of excitatory amino acid induced depolarizations by magnesium ions in isolated spinal cord preparations. J Physiol. 1980 Oct;307:413–428. doi: 10.1113/jphysiol.1980.sp013443. [DOI] [PMC free article] [PubMed] [Google Scholar]
- Brazy P. C., Gunn R. B. Furosemide inhibition of chloride transport in human red blood cells. J Gen Physiol. 1976 Dec;68(6):583–599. doi: 10.1085/jgp.68.6.583. [DOI] [PMC free article] [PubMed] [Google Scholar]
- Bührle C. P., Sonnhof U. The ionic mechanism of postsynaptic inhibition in motoneurones of the frog spinal cord. Neuroscience. 1985 Feb;14(2):581–592. doi: 10.1016/0306-4522(85)90312-4. [DOI] [PubMed] [Google Scholar]
- COOMBS J. S., ECCLES J. C., FATT P. The specific ionic conductances and the ionic movements across the motoneuronal membrane that produce the inhibitory post-synaptic potential. J Physiol. 1955 Nov 28;130(2):326–374. doi: 10.1113/jphysiol.1955.sp005412. [DOI] [PMC free article] [PubMed] [Google Scholar]
- Cabot J. B., Bogan N. Light microscopic observations on the morphology of sympathetic preganglionic neurons in the pigeon, Columba livia. Neuroscience. 1987 Feb;20(2):467–486. doi: 10.1016/0306-4522(87)90105-9. [DOI] [PubMed] [Google Scholar]
- Coote J. H. The organisation of cardiovascular neurons in the spinal cord. Rev Physiol Biochem Pharmacol. 1988;110:147–285. doi: 10.1007/BFb0027531. [DOI] [PubMed] [Google Scholar]
- Coote J. H., Westbury D. R. Intracellular recordings from sympathetic preganglionic neurones. Neurosci Lett. 1979 Dec;15(2-3):171–175. doi: 10.1016/0304-3940(79)96108-1. [DOI] [PubMed] [Google Scholar]
- Curtis D. R., Duggan A. W., Johnston G. A. The specificity of strychnine as a glycine antagonist in the mammalian spinal cord. Exp Brain Res. 1971 Jun 29;12(5):547–565. doi: 10.1007/BF00234248. [DOI] [PubMed] [Google Scholar]
- Davidoff R. A., Shank R. P., Graham L. T., Jr, Aprison M. H., Werman R. Association of glycine with spinal interneurones. Nature. 1967 May 13;214(5089):680–681. doi: 10.1038/214680a0. [DOI] [PubMed] [Google Scholar]
- Dembowsky K., Czachurski J., Seller H. An intracellular study of the synaptic input to sympathetic preganglionic neurones of the third thoracic segment of the cat. J Auton Nerv Syst. 1985 Jul;13(3):201–244. doi: 10.1016/0165-1838(85)90012-8. [DOI] [PubMed] [Google Scholar]
- Dembowsky K., Czachurski J., Seller H. Morphology of sympathetic preganglionic neurons in the thoracic spinal cord of the cat: an intracellular horseradish peroxidase study. J Comp Neurol. 1985 Aug 22;238(4):453–465. doi: 10.1002/cne.902380409. [DOI] [PubMed] [Google Scholar]
- Dembowsky K., Czachurski J., Seller H. Three types of sympathetic preganglionic neurones with different electrophysiological properties are identified by intracellular recordings in the cat. Pflugers Arch. 1986 Feb;406(2):112–120. doi: 10.1007/BF00586671. [DOI] [PubMed] [Google Scholar]
- Dun N. J., Mo N. In vitro effects of substance P on neonatal rat sympathetic preganglionic neurones. J Physiol. 1988 May;399:321–333. doi: 10.1113/jphysiol.1988.sp017083. [DOI] [PMC free article] [PubMed] [Google Scholar]
- ECCLES J. C., FATT P., KOKETSU K. Cholinergic and inhibitory synapses in a pathway from motor-axon collaterals to motoneurones. J Physiol. 1954 Dec 10;126(3):524–562. doi: 10.1113/jphysiol.1954.sp005226. [DOI] [PMC free article] [PubMed] [Google Scholar]
- Hochner B., Spira M. E., Werman R. Penicillin decreases chloride conductance in crustacean muscle: a model for the epileptic neuron. Brain Res. 1976 Apr 30;107(1):85–103. doi: 10.1016/0006-8993(76)90097-4. [DOI] [PubMed] [Google Scholar]
- Jankowska E., Roberts W. J. Synaptic actions of single interneurones mediating reciprocal Ia inhibition of motoneurones. J Physiol. 1972 May;222(3):623–642. doi: 10.1113/jphysiol.1972.sp009818. [DOI] [PMC free article] [PubMed] [Google Scholar]
- Llinas R., Baker R. A chloride-dependent inhibitory postsynaptic potential in cat trochlear motoneurons. J Neurophysiol. 1972 Jul;35(4):484–492. doi: 10.1152/jn.1972.35.4.484. [DOI] [PubMed] [Google Scholar]
- Lux H. D., Loracher C., Neher E. The action of ammonium on postsynaptic inhibition of cat spinal motoneurons. Exp Brain Res. 1970;11(5):431–447. doi: 10.1007/BF00233967. [DOI] [PubMed] [Google Scholar]
- Ma R. C., Dun N. J. Excitation of lateral horn neurons of the neonatal rat spinal cord by 5-hydroxytryptamine. Brain Res. 1986 Jan;389(1-2):89–98. doi: 10.1016/0165-3806(86)90176-8. [DOI] [PubMed] [Google Scholar]
- MacDonald J. F., Porietis A. V., Wojtowicz J. M. L-Aspartic acid induces a region of negative slope conductance in the current-voltage relationship of cultured spinal cord neurons. Brain Res. 1982 Apr 8;237(1):248–253. doi: 10.1016/0006-8993(82)90575-3. [DOI] [PubMed] [Google Scholar]
- Mayer M. L., Westbrook G. L., Guthrie P. B. Voltage-dependent block by Mg2+ of NMDA responses in spinal cord neurones. Nature. 1984 May 17;309(5965):261–263. doi: 10.1038/309261a0. [DOI] [PubMed] [Google Scholar]
- McLachlan E. M., Hirst G. D. Some properties of preganglionic neurons in upper thoracic spinal cord of the cat. J Neurophysiol. 1980 May;43(5):1251–1265. doi: 10.1152/jn.1980.43.5.1251. [DOI] [PubMed] [Google Scholar]
- McLennan H. Receptors for the excitatory amino acids in the mammalian central nervous system. Prog Neurobiol. 1983;20(3-4):251–271. doi: 10.1016/0301-0082(83)90004-7. [DOI] [PubMed] [Google Scholar]
- Miles R., Wong R. K. Unitary inhibitory synaptic potentials in the guinea-pig hippocampus in vitro. J Physiol. 1984 Nov;356:97–113. doi: 10.1113/jphysiol.1984.sp015455. [DOI] [PMC free article] [PubMed] [Google Scholar]
- Mo N., Dun N. J. Excitatory postsynaptic potentials in neonatal rat sympathetic preganglionic neurons: possible mediation by NMDA receptors. Neurosci Lett. 1987 Jun 26;77(3):327–332. doi: 10.1016/0304-3940(87)90522-2. [DOI] [PubMed] [Google Scholar]
- Nicoll R. A. The blockade of GABA mediated responses in the frog spinal cord by ammonium ions and furosemide. J Physiol. 1978 Oct;283:121–132. doi: 10.1113/jphysiol.1978.sp012491. [DOI] [PMC free article] [PubMed] [Google Scholar]
- Nowak L., Bregestovski P., Ascher P., Herbet A., Prochiantz A. Magnesium gates glutamate-activated channels in mouse central neurones. Nature. 1984 Feb 2;307(5950):462–465. doi: 10.1038/307462a0. [DOI] [PubMed] [Google Scholar]
- Polosa C. The silent period of sympathetic preganglionic neurons. Can J Physiol Pharmacol. 1967 Nov;45(6):1033–1045. doi: 10.1139/y67-121. [DOI] [PubMed] [Google Scholar]