Abstract
1. We have used double-barrelled ion-sensitive microelectrodes to measure the intracellular pH, pHi, the intracellular Na+ activity, aiNa, and the membrane potential in identified glial cells of the central nervous system of the leech Hirudo medicinalis to study the effect of CO2-HCO3-. 2. When a HEPES-buffered saline was exchanged for a saline buffered with 2% CO2 + 11 mM-HCO3-, keeping the pH constant at 7.4, the mean steady-state pHi of the glial cells increased from 6.85 +/- 0.06 to 7.18 +/- 0.13 (mean +/- S.D., n = 25). 3. This CO2-HCO3- -dependent alkalinization was inhibited in the absence of external Na+ (exchanged by N-methyl-D-glucamine), but was unaffected by the inhibitor of Na+-H+ exchange, amiloride (2 mM). 4. The aiNa of the glial cells increased by 2-4 mM from a mean steady state of 7.2 +/- 2 mM (mean +/- S.D., n = 6) upon introduction of CO2-HCO3- -buffered saline. This CO2-HCO3- -dependent rise in aiNa increased to about double when the pHi had been decreased by acid loading the cells (addition and subsequent removal of NH4+). 5. The CO2-HCO3- -dependent increases of pHi and aiNa were inhibited by the stilbene 4,4-diisothiocyanostilbene-2,2'-disulphonic acid (DIDS, 0.5-1.0 mM). 6. Removal of external Cl- and depletion of intracellular Cl- did not inhibit the CO2-HCO3- -dependent alkalinization. 7. The CO2-HCO3- -dependent alkalinization was unaffected by inhibitors of the carbonic anhydrase, acetazolamide (0.2 mM) or ethoxzolamide (2 microM). 8. The membrane potential became more negative by 3-20 mV upon addition of CO2-HCO3-. This hyperpolarization was even further enlarged in the presence of Ba2+ (which reduces the K+ permeability) or at increased external K+ concentration (which depolarizes the membrane and brings the membrane potential to the K+ equilibrium potential). The CO2-HCO3- -induced membrane hyperpolarization was inhibited in Na+-free saline and in the presence of DIDS. Ouabain (0.5 mM) sometimes reduced, but never abolished, the hyperpolarization. 9. The stoichiometry of the co-transport is suggested to be 2 HCO3-:1 Na+ with an equilibrium potential of -90 mV calculated for this coupling ratio in the steady state. 10. It is concluded that in the presence of CO2-HCO3- an inwardly directed electrogenic Na+-HCO3- co-transport is stimulated across the glial membrane, which greatly determines the pHi and thereby affects the intracellular buffering power of the glial cells.
Full text
PDF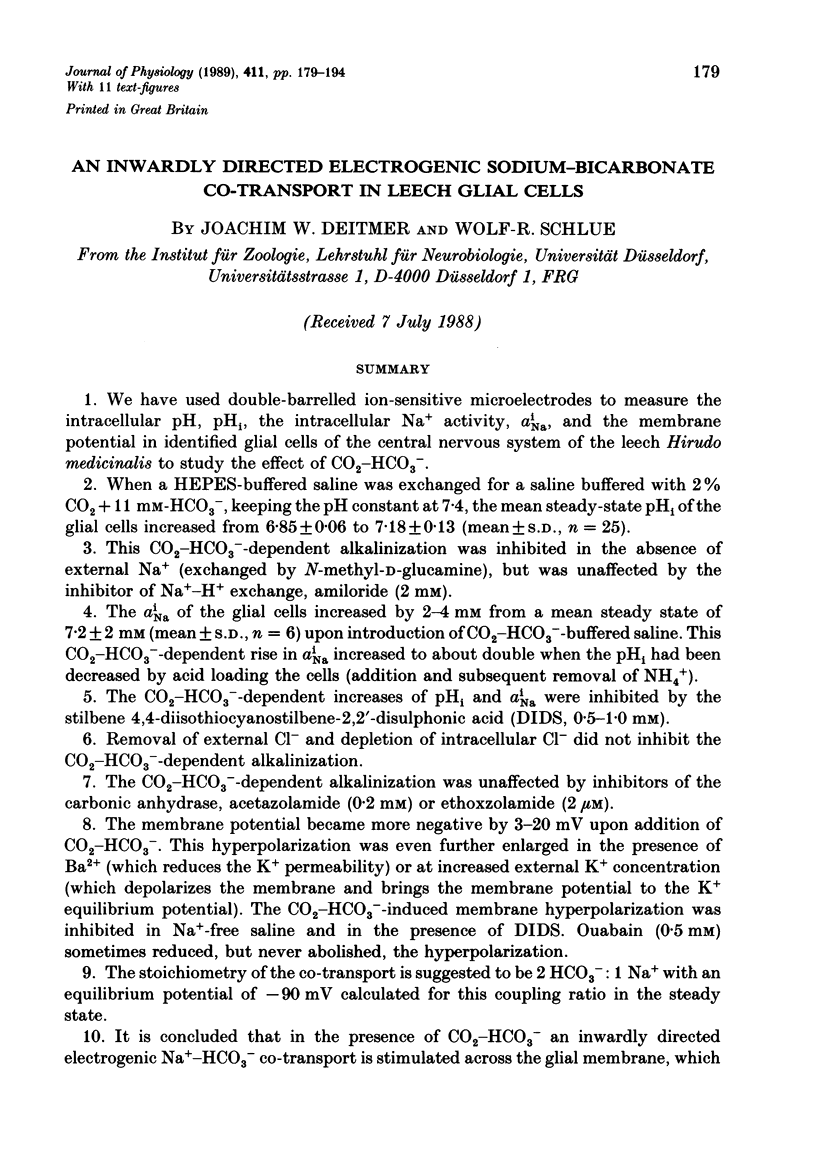
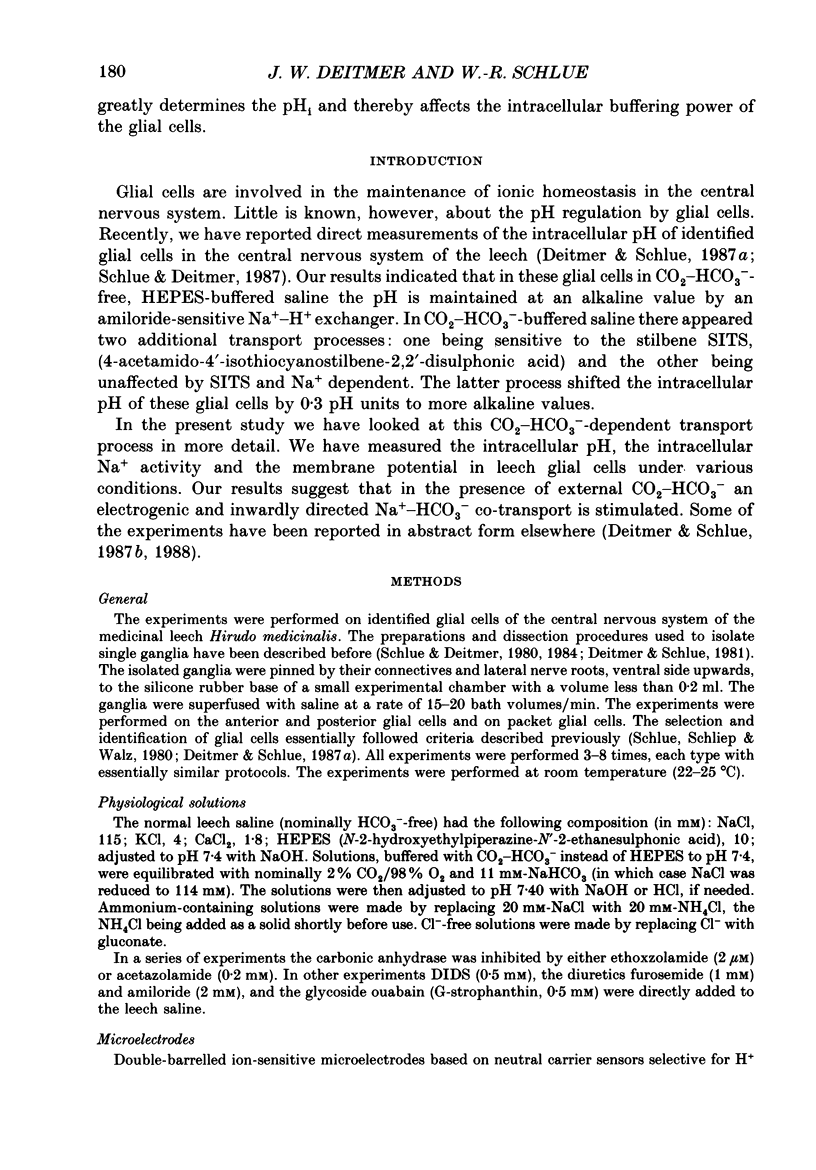
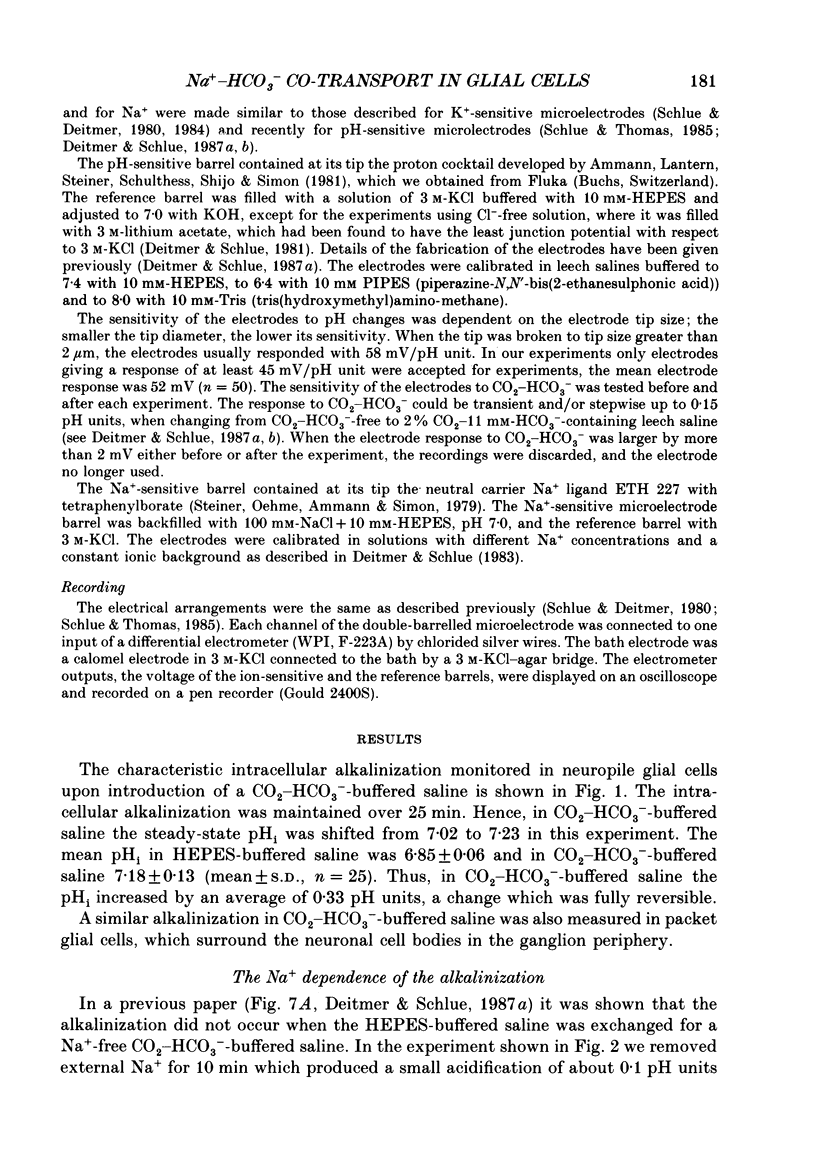
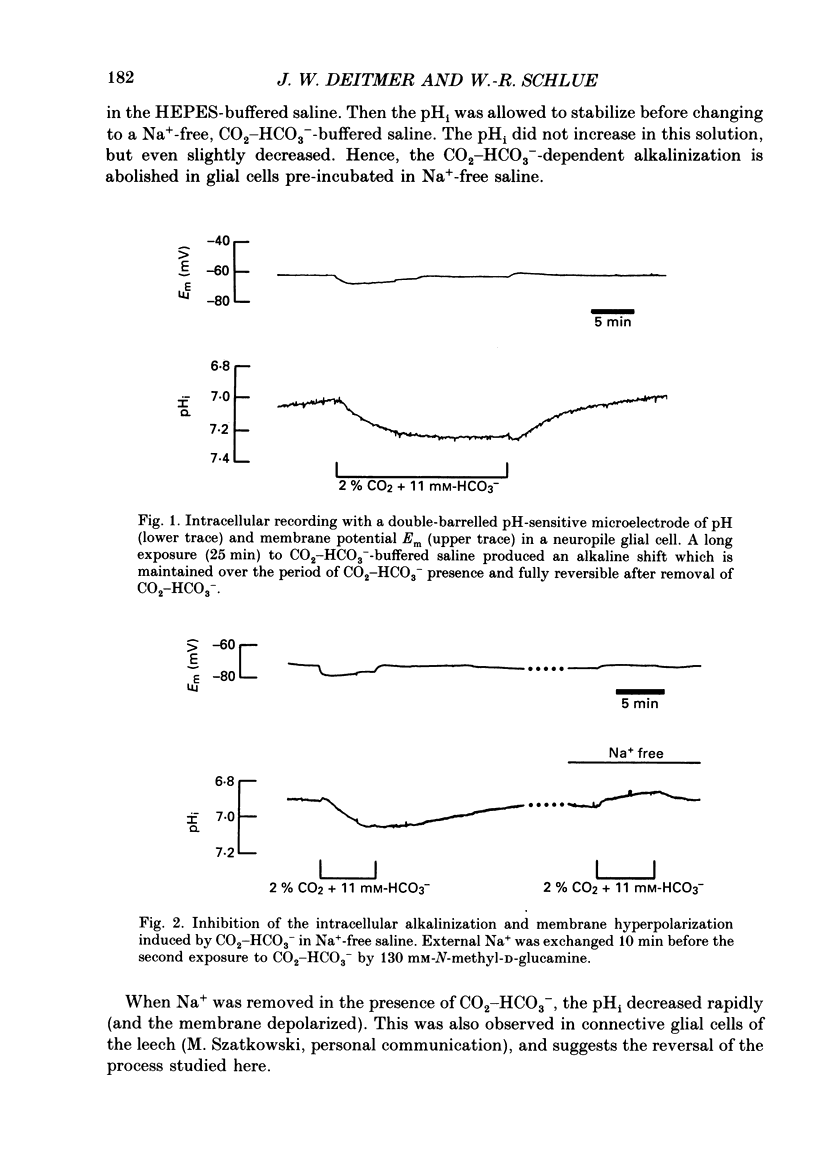
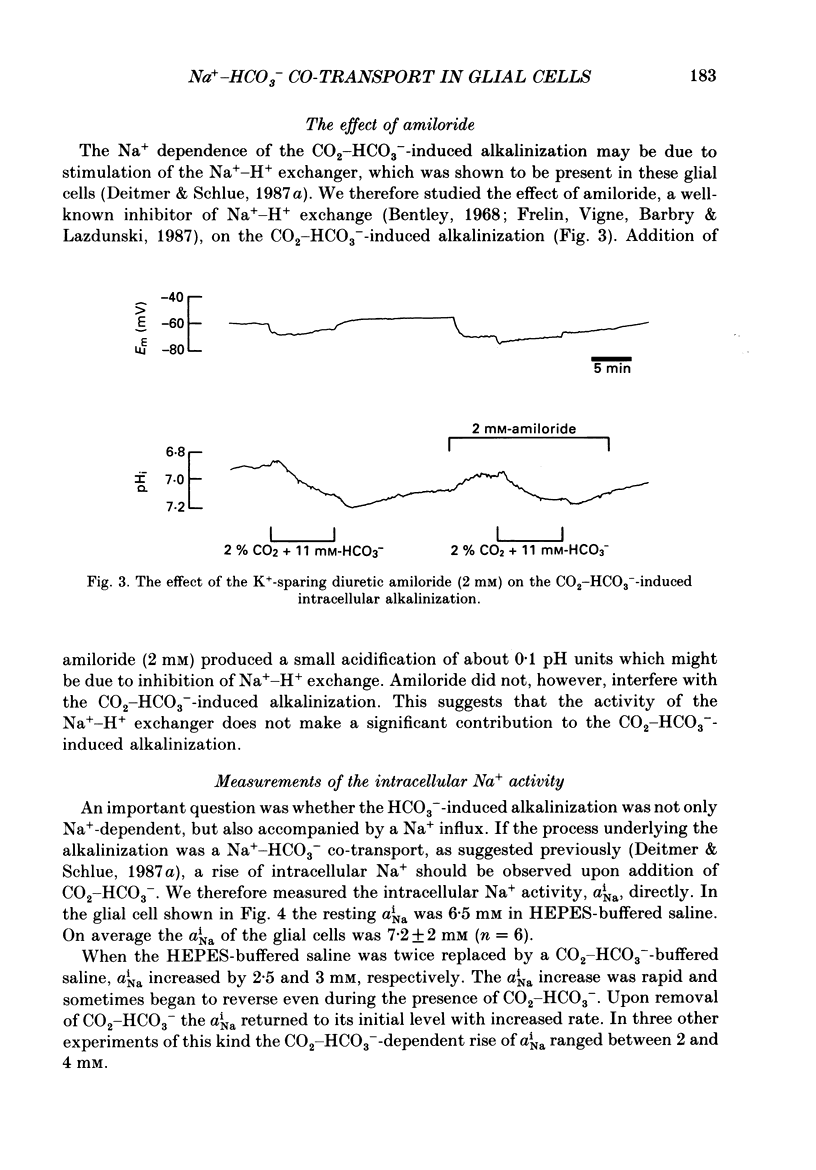
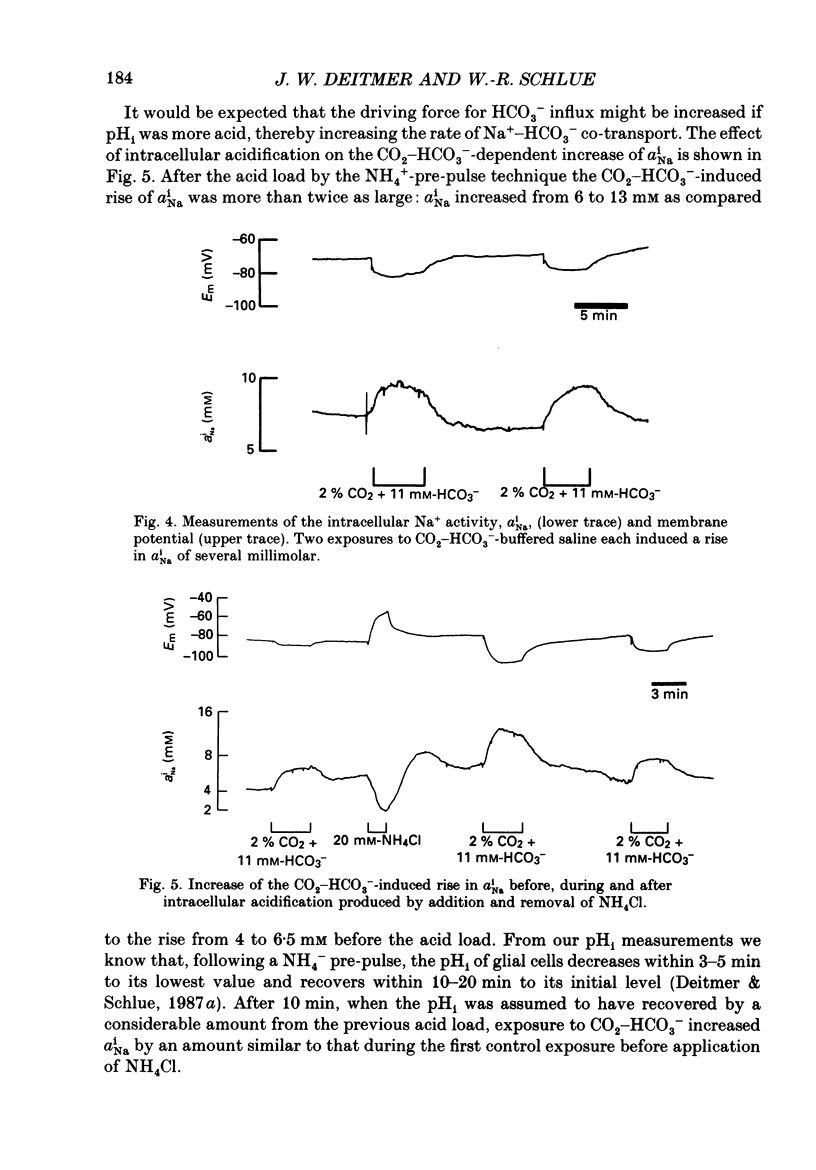
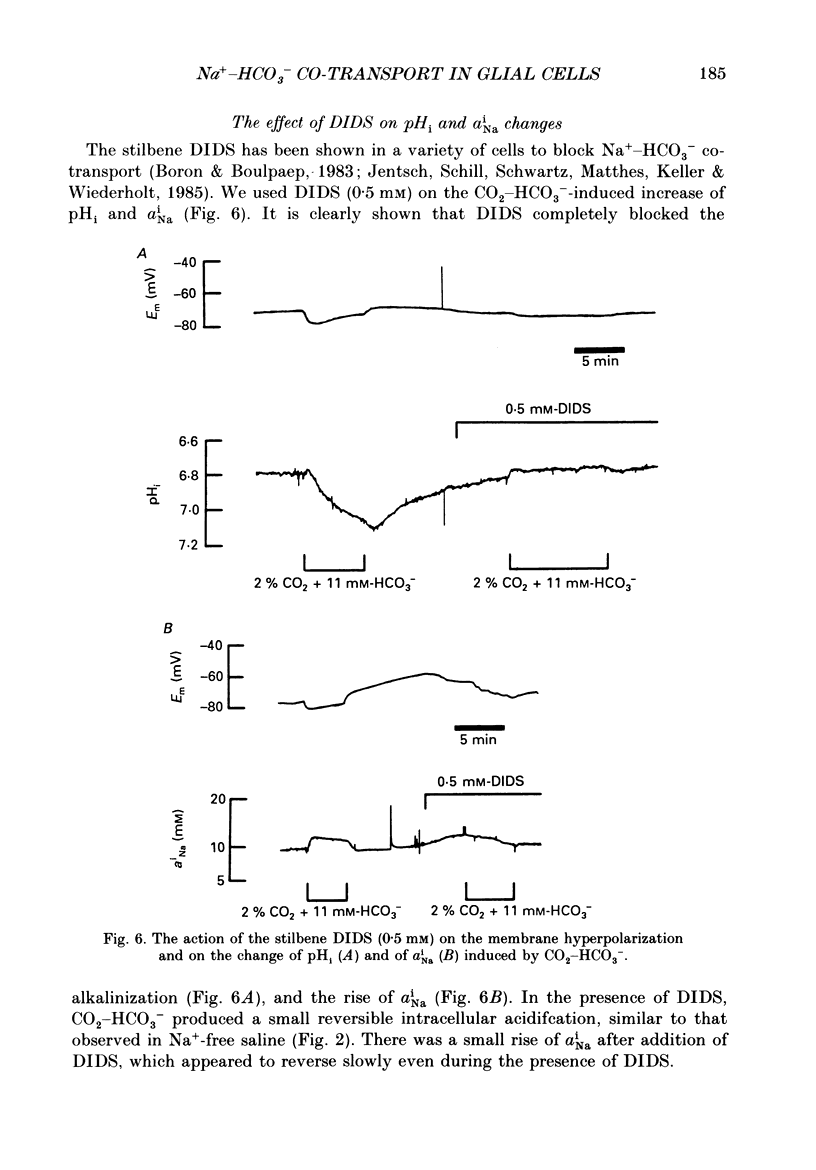
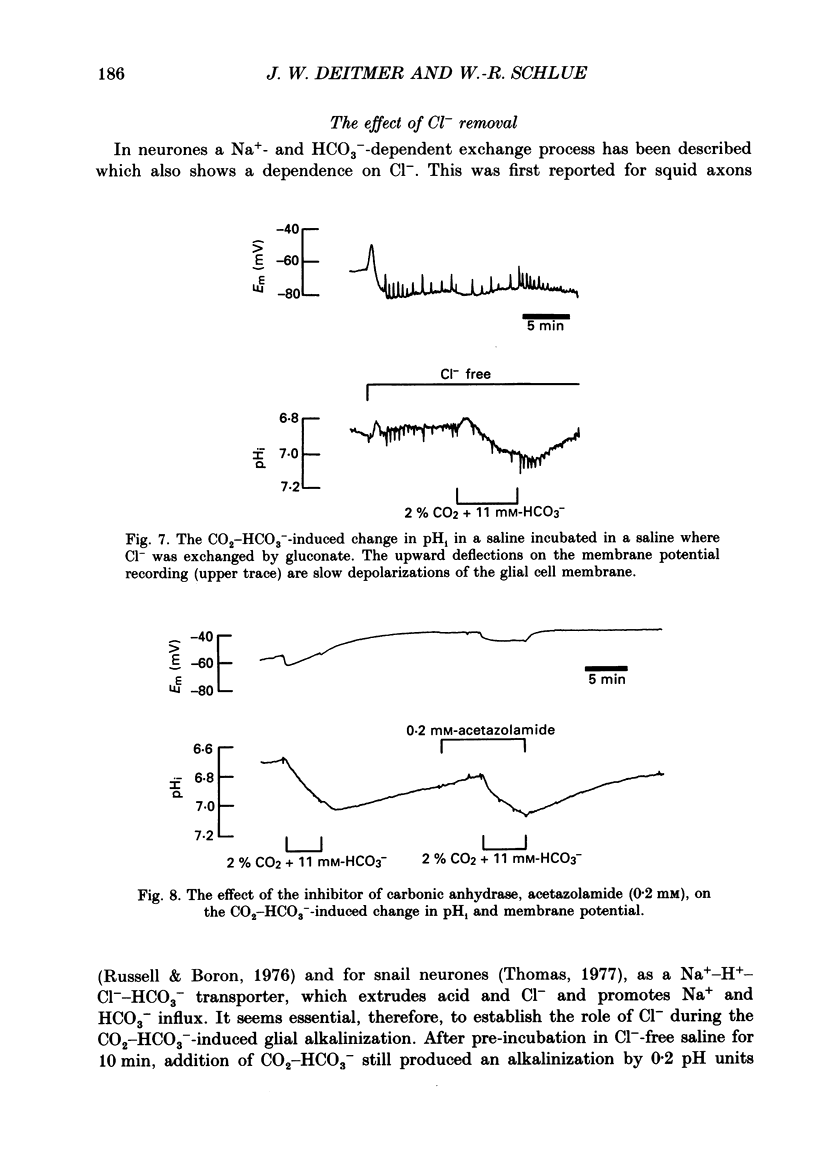
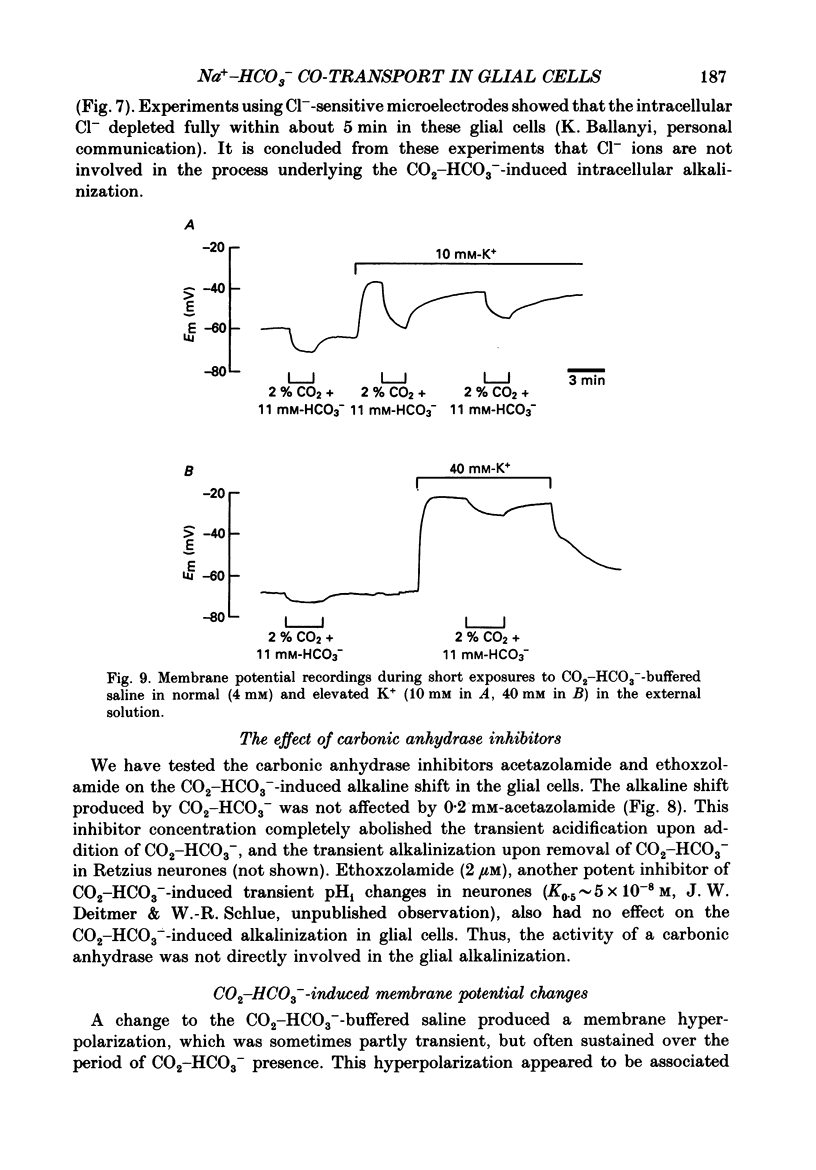
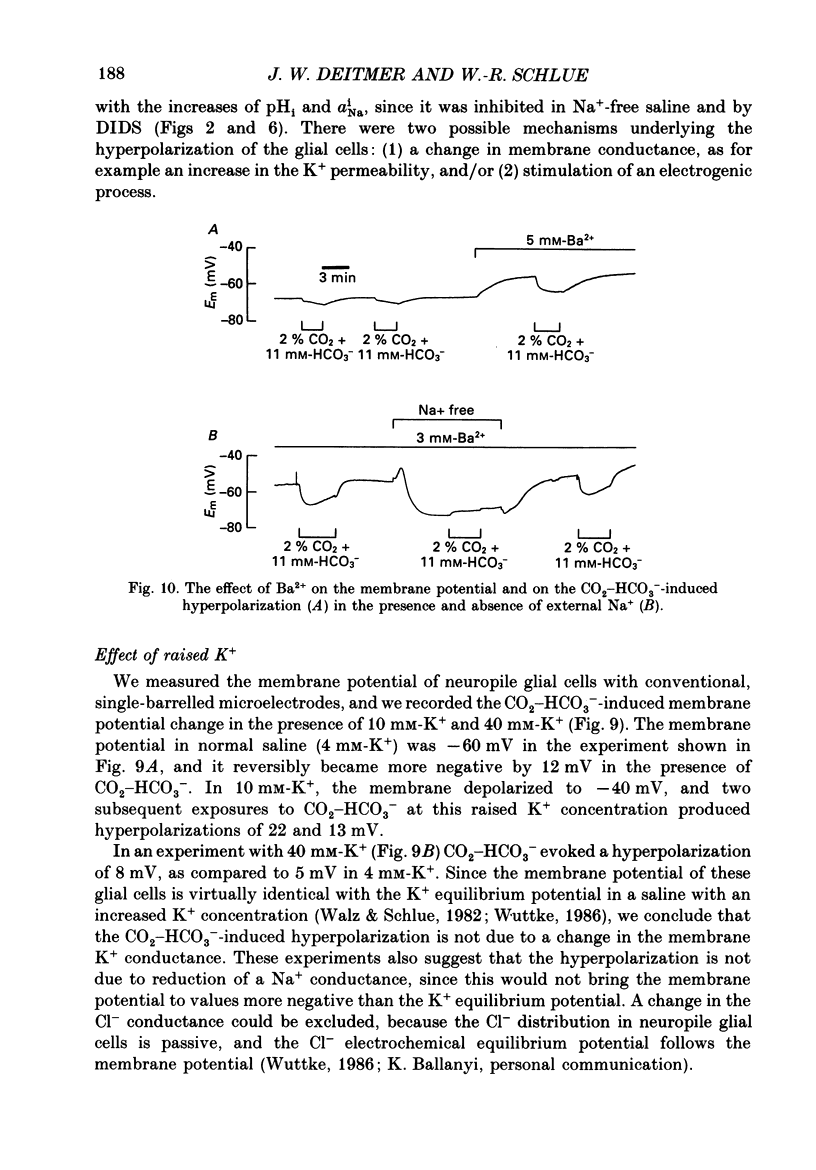
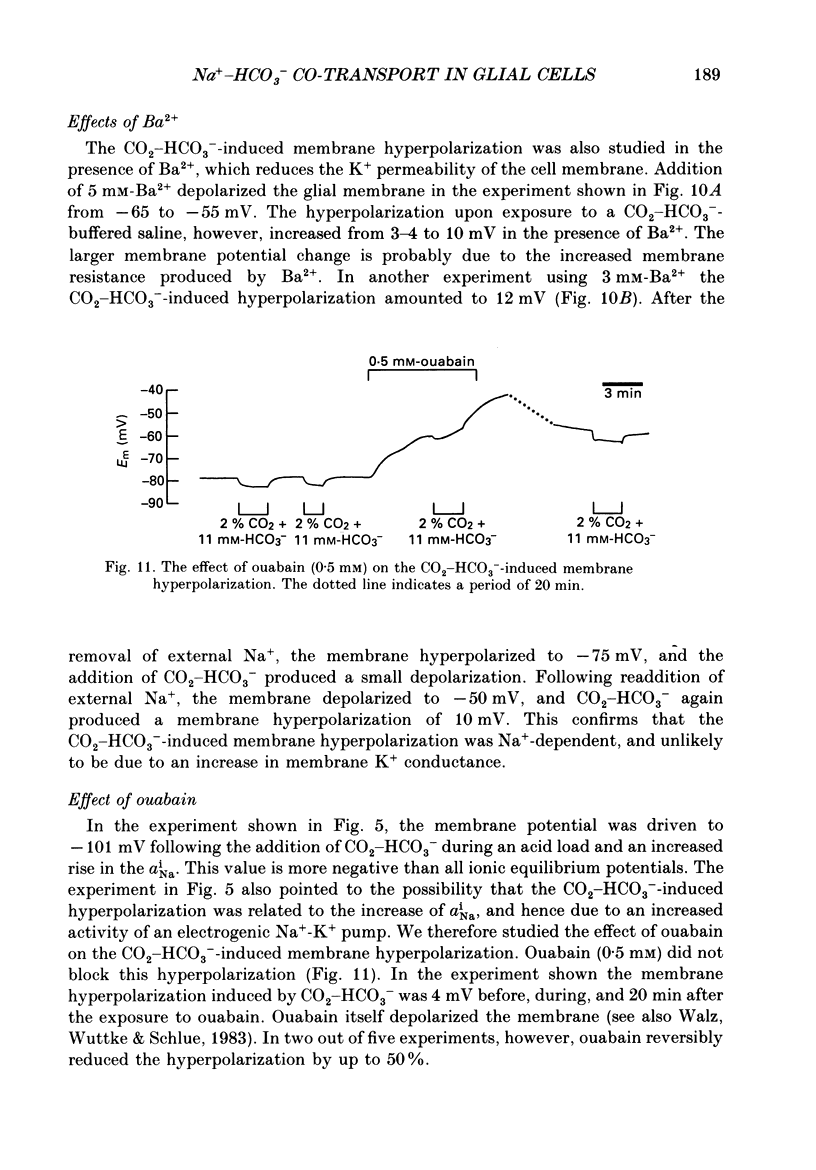
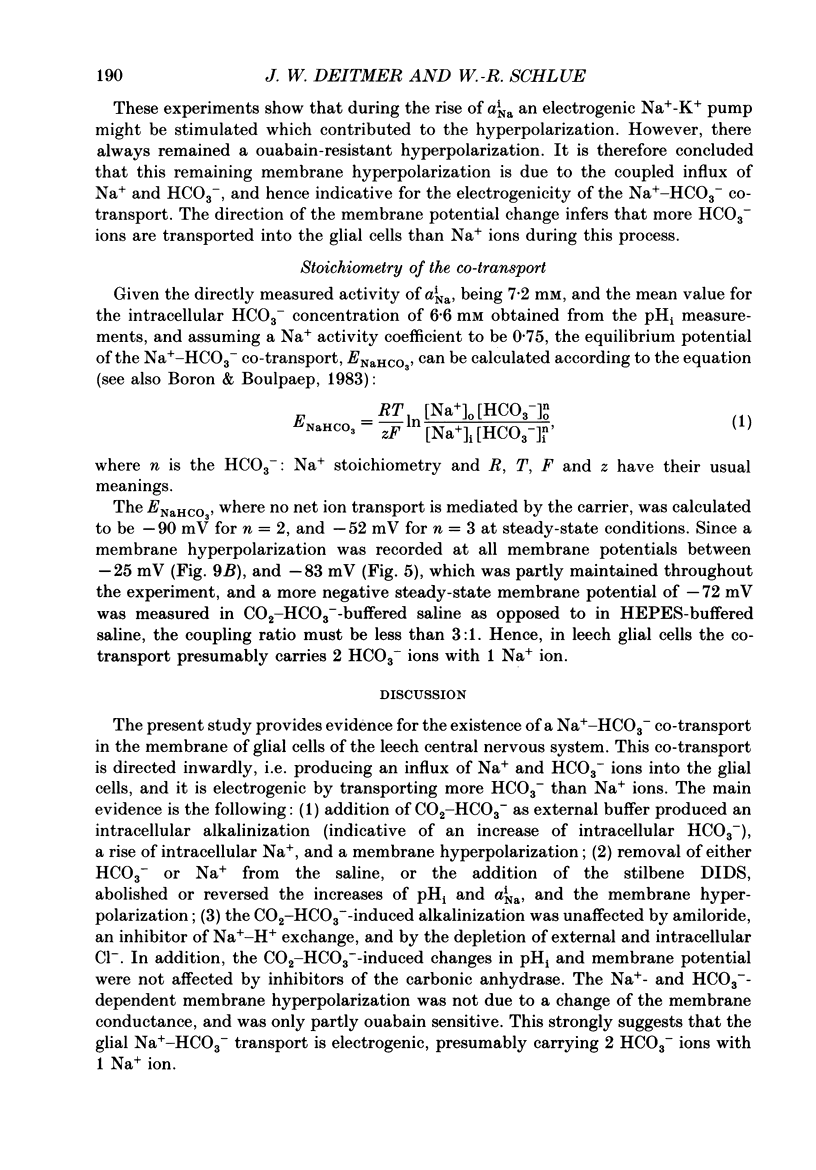
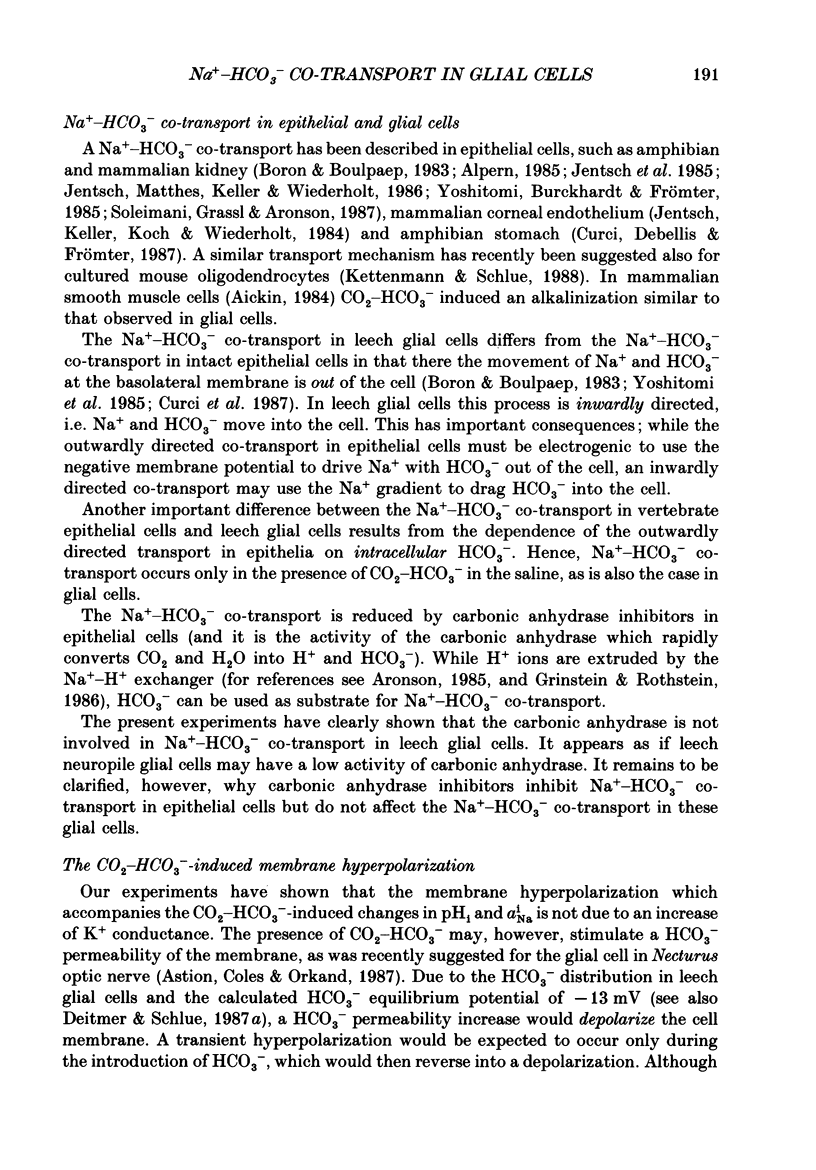
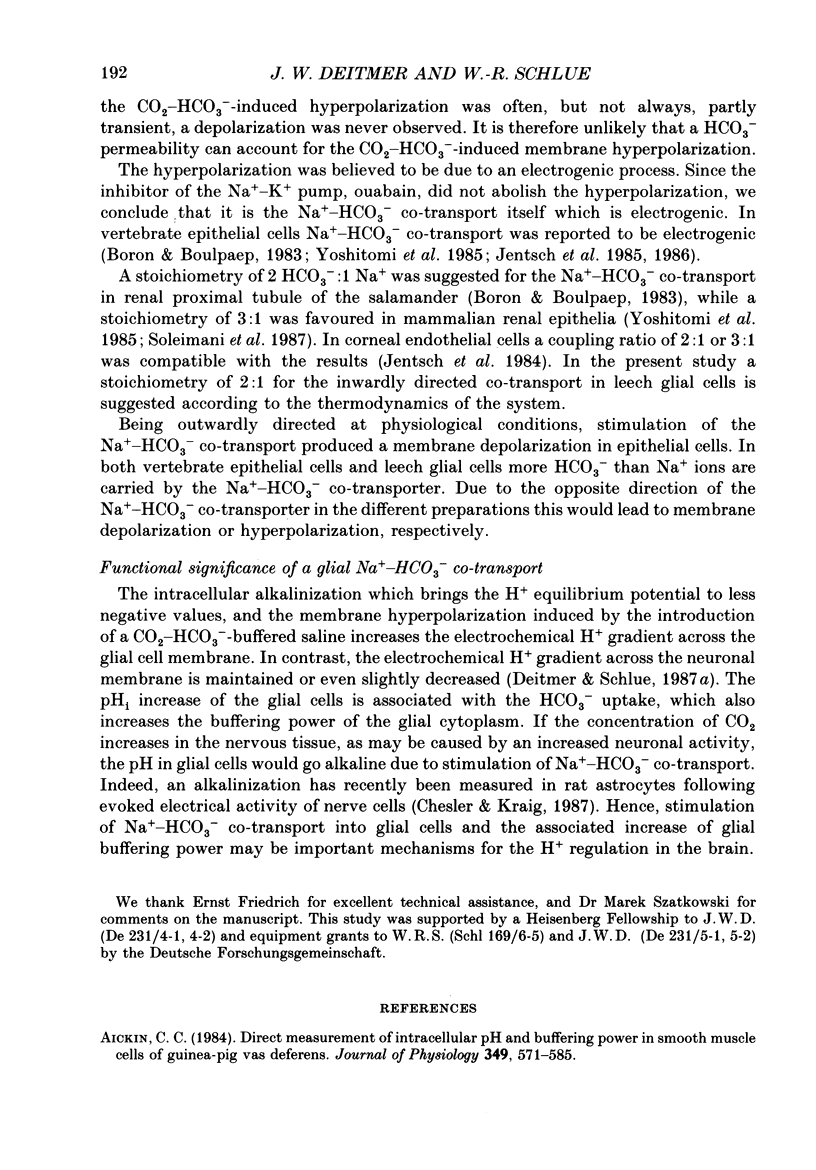
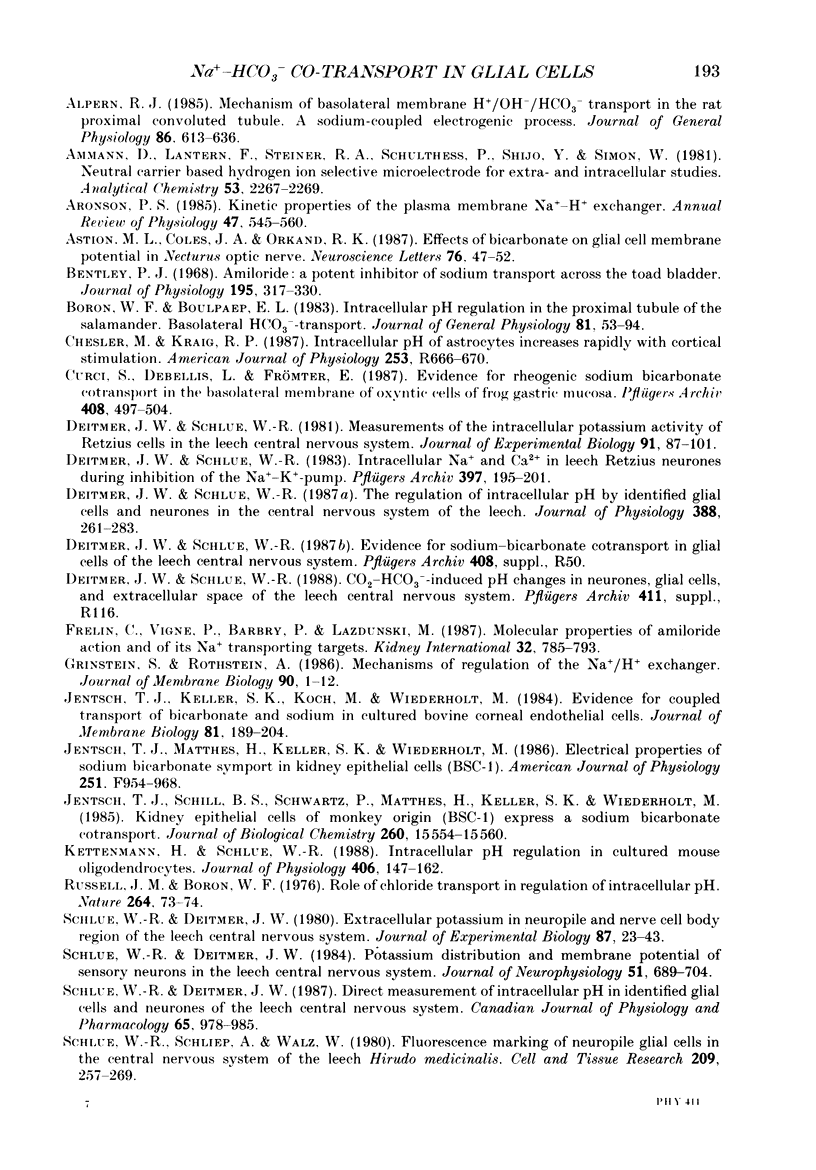
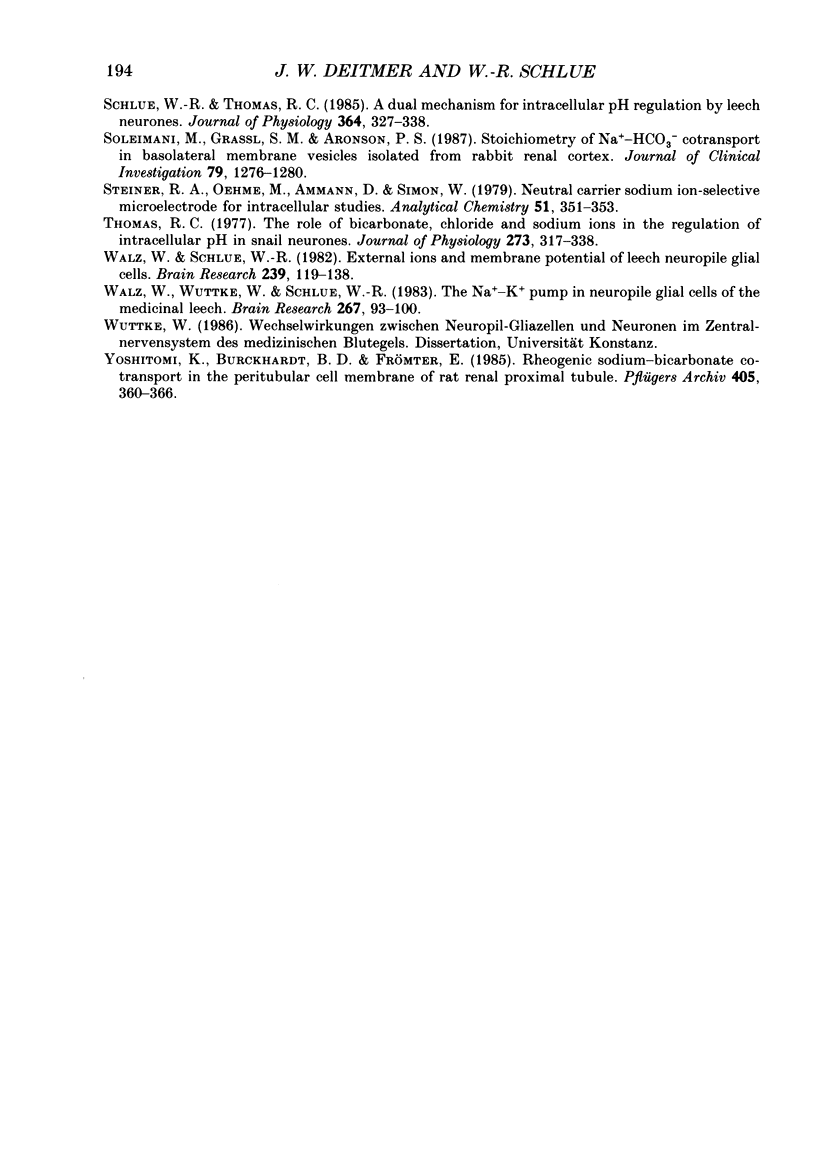
Selected References
These references are in PubMed. This may not be the complete list of references from this article.
- Aickin C. C. Direct measurement of intracellular pH and buffering power in smooth muscle cells of guinea-pig vas deferens. J Physiol. 1984 Apr;349:571–585. doi: 10.1113/jphysiol.1984.sp015174. [DOI] [PMC free article] [PubMed] [Google Scholar]
- Alpern R. J. Mechanism of basolateral membrane H+/OH-/HCO-3 transport in the rat proximal convoluted tubule. A sodium-coupled electrogenic process. J Gen Physiol. 1985 Nov;86(5):613–636. doi: 10.1085/jgp.86.5.613. [DOI] [PMC free article] [PubMed] [Google Scholar]
- Ammann D., Lanter F., Steiner R. A., Schulthess P., Shijo Y., Simon W. Neutral carrier based hydrogen ion selective microelectrode for extra- and intracellular studies. Anal Chem. 1981 Dec;53(14):2267–2269. doi: 10.1021/ac00237a031. [DOI] [PubMed] [Google Scholar]
- Aronson P. S. Kinetic properties of the plasma membrane Na+-H+ exchanger. Annu Rev Physiol. 1985;47:545–560. doi: 10.1146/annurev.ph.47.030185.002553. [DOI] [PubMed] [Google Scholar]
- Astion M. L., Coles J. A., Orkand R. K. Effects of bicarbonate on glial cell membrane potential in Necturus optic nerve. Neurosci Lett. 1987 Apr 23;76(1):47–52. doi: 10.1016/0304-3940(87)90190-x. [DOI] [PubMed] [Google Scholar]
- Bentley P. J. Amiloride: a potent inhibitor of sodium transport across the toad bladder. J Physiol. 1968 Mar;195(2):317–330. doi: 10.1113/jphysiol.1968.sp008460. [DOI] [PMC free article] [PubMed] [Google Scholar]
- Boron W. F., Boulpaep E. L. Intracellular pH regulation in the renal proximal tubule of the salamander. Basolateral HCO3- transport. J Gen Physiol. 1983 Jan;81(1):53–94. doi: 10.1085/jgp.81.1.53. [DOI] [PMC free article] [PubMed] [Google Scholar]
- Chesler M., Kraig R. P. Intracellular pH of astrocytes increases rapidly with cortical stimulation. Am J Physiol. 1987 Oct;253(4 Pt 2):R666–R670. doi: 10.1152/ajpregu.1987.253.4.R666. [DOI] [PMC free article] [PubMed] [Google Scholar]
- Curci S., Debellis L., Frömter E. Evidence for rheogenic sodium bicarbonate cotransport in the basolateral membrane of oxyntic cells of frog gastric fundus. Pflugers Arch. 1987 May;408(5):497–504. doi: 10.1007/BF00585075. [DOI] [PubMed] [Google Scholar]
- Deitmer J. W., Schlue W. R. Intracellular Na+ and Ca2+ in leech Retzius neurones during inhibition of the Na+-K+ pump. Pflugers Arch. 1983 May;397(3):195–201. doi: 10.1007/BF00584357. [DOI] [PubMed] [Google Scholar]
- Deitmer J. W., Schlue W. R. The regulation of intracellular pH by identified glial cells and neurones in the central nervous system of the leech. J Physiol. 1987 Jul;388:261–283. doi: 10.1113/jphysiol.1987.sp016614. [DOI] [PMC free article] [PubMed] [Google Scholar]
- Frelin C., Vigne P., Barbry P., Lazdunski M. Molecular properties of amiloride action and of its Na+ transporting targets. Kidney Int. 1987 Dec;32(6):785–793. doi: 10.1038/ki.1987.277. [DOI] [PubMed] [Google Scholar]
- Grinstein S., Rothstein A. Mechanisms of regulation of the Na+/H+ exchanger. J Membr Biol. 1986;90(1):1–12. doi: 10.1007/BF01869680. [DOI] [PubMed] [Google Scholar]
- Jentsch T. J., Keller S. K., Koch M., Wiederholt M. Evidence for coupled transport of bicarbonate and sodium in cultured bovine corneal endothelial cells. J Membr Biol. 1984;81(3):189–204. doi: 10.1007/BF01868713. [DOI] [PubMed] [Google Scholar]
- Jentsch T. J., Matthes H., Keller S. K., Wiederholt M. Electrical properties of sodium bicarbonate symport in kidney epithelial cells (BSC-1). Am J Physiol. 1986 Dec;251(6 Pt 2):F954–F968. doi: 10.1152/ajprenal.1986.251.6.F954. [DOI] [PubMed] [Google Scholar]
- Jentsch T. J., Schill B. S., Schwartz P., Matthes H., Keller S. K., Wiederholt M. Kidney epithelial cells of monkey origin (BSC-1) express a sodium bicarbonate cotransport. Characterization by 22Na+ flux measurements. J Biol Chem. 1985 Dec 15;260(29):15554–15560. [PubMed] [Google Scholar]
- Kettenmann H., Schlue W. R. Intracellular pH regulation in cultured mouse oligodendrocytes. J Physiol. 1988 Dec;406:147–162. doi: 10.1113/jphysiol.1988.sp017373. [DOI] [PMC free article] [PubMed] [Google Scholar]
- Russell J. M., Boron W. F. Role of choloride transport in regulation of intracellular pH. Nature. 1976 Nov 4;264(5581):73–74. doi: 10.1038/264073a0. [DOI] [PubMed] [Google Scholar]
- Schlue W. R., Deitmer J. W. Direct measurement of intracellular pH in identified glial cells and neurones of the leech central nervous system. Can J Physiol Pharmacol. 1987 May;65(5):978–985. doi: 10.1139/y87-155. [DOI] [PubMed] [Google Scholar]
- Schlue W. R., Deitmer J. W. Extracellular potassium in neuropile and nerve cell body region of the leech central nervous system. J Exp Biol. 1980 Aug;87:23–43. doi: 10.1242/jeb.87.1.23. [DOI] [PubMed] [Google Scholar]
- Schlue W. R., Deitmer J. W. Potassium distribution and membrane potential of sensory neurons in the leech nervous system. J Neurophysiol. 1984 Apr;51(4):689–704. doi: 10.1152/jn.1984.51.4.689. [DOI] [PubMed] [Google Scholar]
- Schlue W. R., Schliep A., Walz W. Fluorescence marking of neuropile glial cells in the central nervous system of the leech Hirudo medicinalis. Cell Tissue Res. 1980;209(2):257–269. doi: 10.1007/BF00237630. [DOI] [PubMed] [Google Scholar]
- Schlue W. R., Thomas R. C. A dual mechanism for intracellular pH regulation by leech neurones. J Physiol. 1985 Jul;364:327–338. doi: 10.1113/jphysiol.1985.sp015748. [DOI] [PMC free article] [PubMed] [Google Scholar]
- Soleimani M., Grassi S. M., Aronson P. S. Stoichiometry of Na+-HCO-3 cotransport in basolateral membrane vesicles isolated from rabbit renal cortex. J Clin Invest. 1987 Apr;79(4):1276–1280. doi: 10.1172/JCI112948. [DOI] [PMC free article] [PubMed] [Google Scholar]
- Thomas R. C. The role of bicarbonate, chloride and sodium ions in the regulation of intracellular pH in snail neurones. J Physiol. 1977 Dec;273(1):317–338. doi: 10.1113/jphysiol.1977.sp012096. [DOI] [PMC free article] [PubMed] [Google Scholar]
- Walz W., Schlue W. R. External ions and membrane potential of leech neuropile glial cells. Brain Res. 1982 May 6;239(1):119–138. doi: 10.1016/0006-8993(82)90837-x. [DOI] [PubMed] [Google Scholar]
- Walz W., Wuttke W., Schlue W. R. The Na+-K+ pump in neuropile glial cells of the medicinal leech. Brain Res. 1983 May 9;267(1):93–100. doi: 10.1016/0006-8993(83)91042-9. [DOI] [PubMed] [Google Scholar]
- Yoshitomi K., Burckhardt B. C., Frömter E. Rheogenic sodium-bicarbonate cotransport in the peritubular cell membrane of rat renal proximal tubule. Pflugers Arch. 1985 Dec;405(4):360–366. doi: 10.1007/BF00595689. [DOI] [PubMed] [Google Scholar]