Abstract
1. Rat dentate granule neurones in hippocampal slices were voltage-clamped at 21-23 degrees C using CsCl-filled microelectrodes. The perfusate contained TTX and K+ channel blockers to isolate pharmacologically inward Ca2+ currents. 2. From hyperpolarized holding potentials of -65 to -85 mV, depolarizing test potentials to between -50 and -40 mV elicited a transient (100-200 ms) low-threshold (TLT) current which was also elicited from more depolarized holding potentials following hyperpolarizing voltage steps of -40 mV or greater. 3. Larger depolarizing steps from a hyperpolarized holding potential triggered a large (2-6 nA), transient high-threshold (THT) inward current, rapidly peaking and decaying over 500 ms, followed by a sustained inward current component. 4. At depolarized holding potentials (-50 to -20 mV), the THT current was apparently inactivated and a sustained high-threshold (SHT) inward current was evident during depolarizing voltage steps of 10 mV or more. 5. From hyperpolarized holding potentials with depolarizing voltage steps of 10-30 mV, most neurones demonstrated a small-amplitude, sustained low-threshold (SLT) inward current with similar characteristics to the SHT current. 6. Zero-Ca2+ perfusate or high concentrations of Ca2+ channel blockers (Cd2+, Mn2+ or Ni2+) diminished or abolished all inward currents. 7. Repetitive voltage step activation of each current at 0.5 Hz reduced the large THT current to less than 25% of an unconditioned control current, reduced the SHT current by 50%, but had little effect on the TLT current. 8. A low concentration of Cd2+ (50 microM) blocked the THT and SHT currents with little effect on the TLT current. Nimodipine (1 microM) attenuated the SHT current. Ni2+ (100 microM) selectively attenuated the TLT current. 9. In low-Ca2+ perfusate, high concentrations of Ca2+ (10-15 mM), focally applied to different parts of the neurone, increased the THT current when applied to the dendrites, the SHT current when applied to the soma and the TLT current at all locations. Conversely, in regular perfusate, Cd2+ (1-5 mM), focally applied to the dendrites decreased the THT current and somatic applications decreased the SHT current. The TLT current was diminished regardless of the site of Cd2+ application. 10. These results suggest the existence of three different Ca2+ currents in dentate granule cells separable by their activation and inactivation characteristics, pharmacology and site of initiation.
Full text
PDF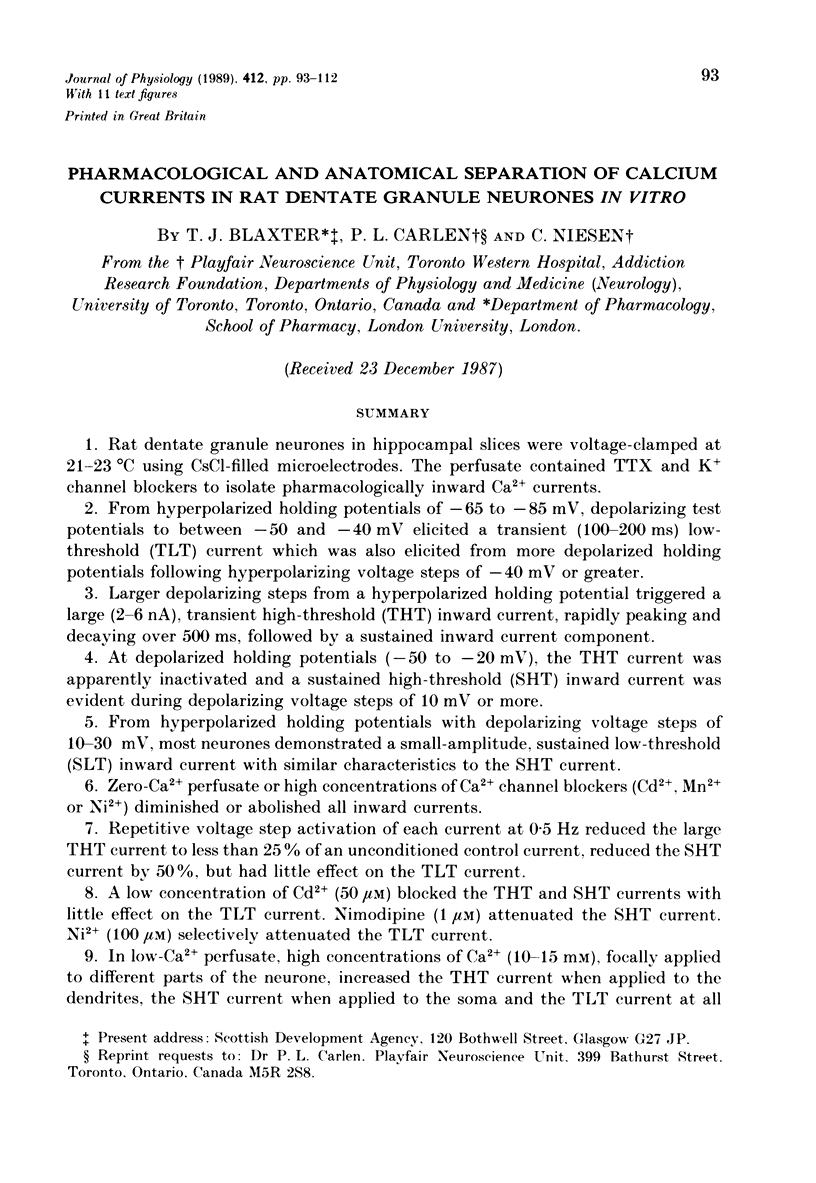
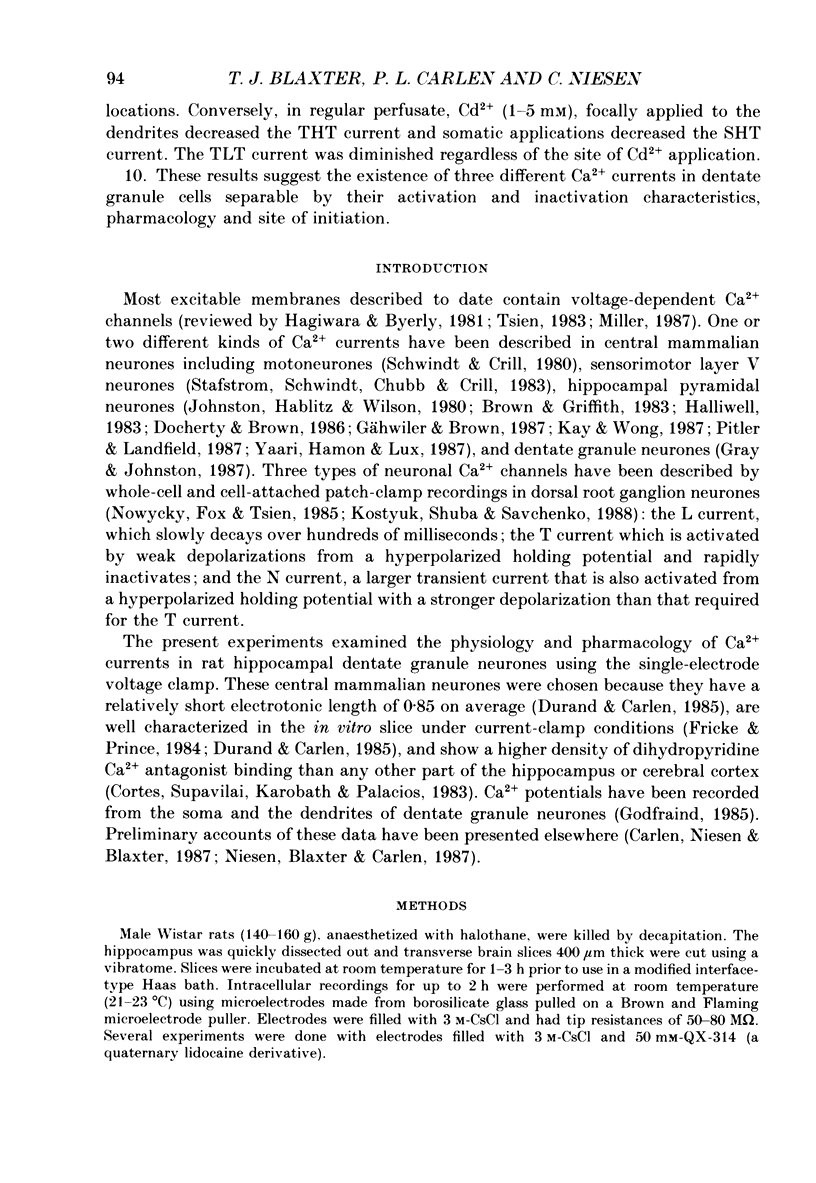
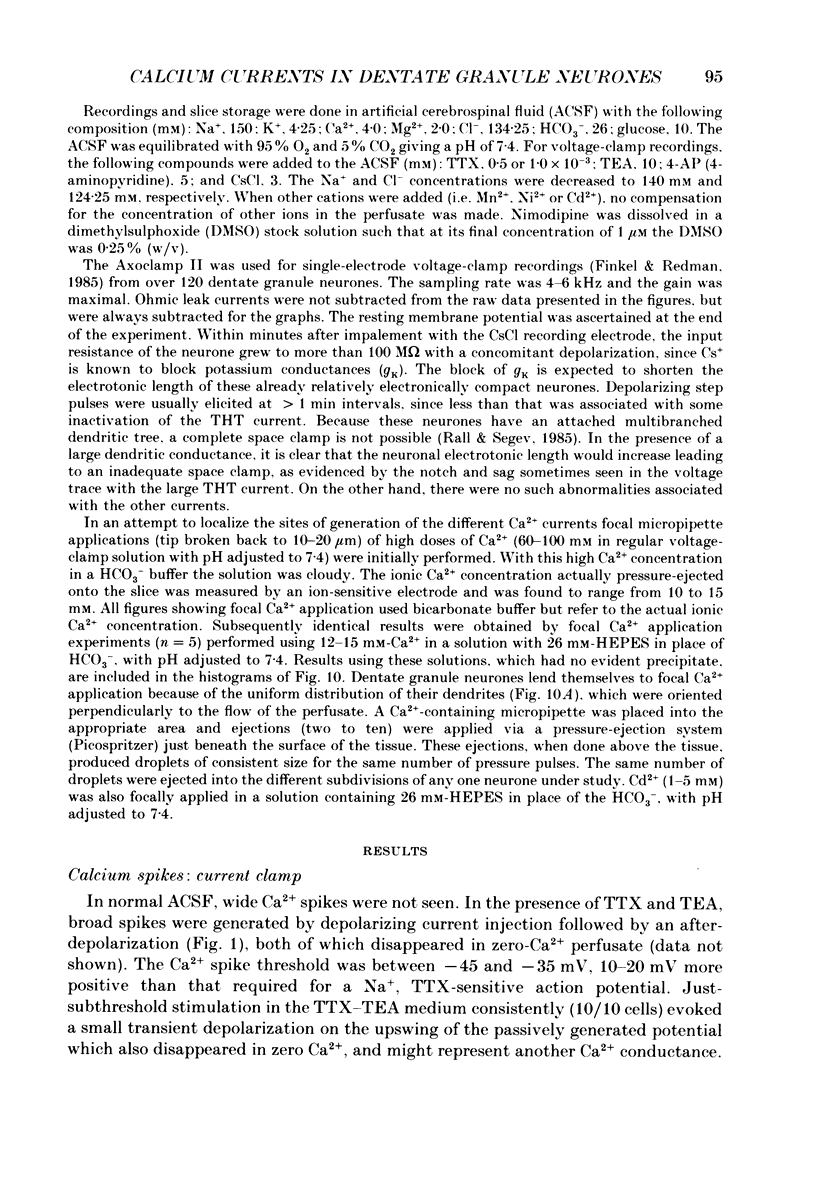
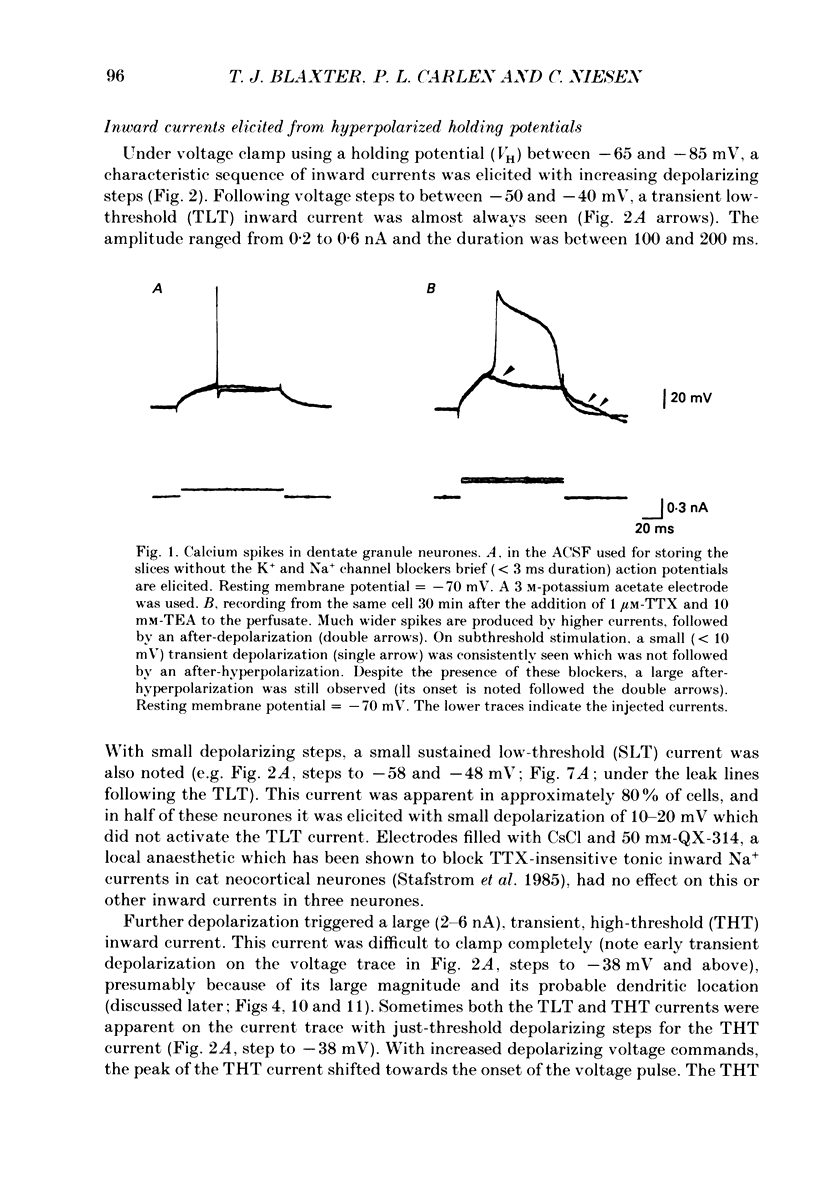
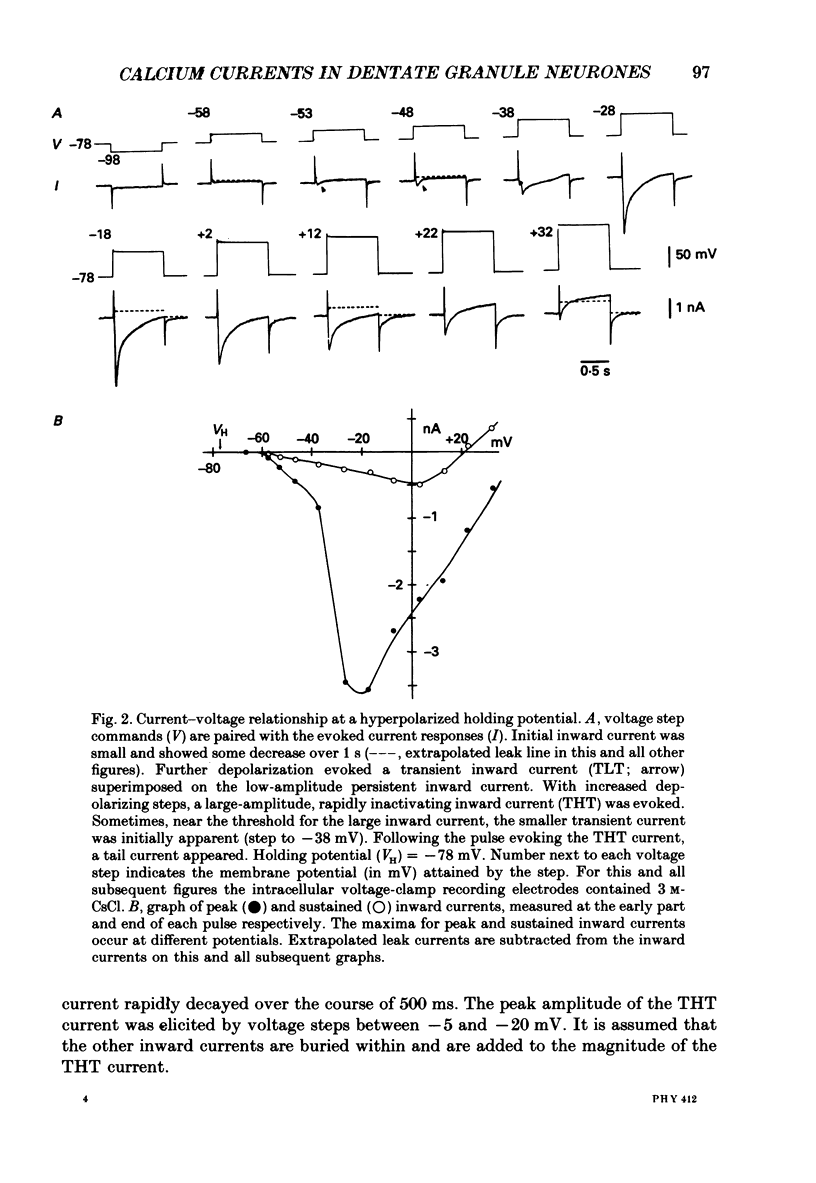
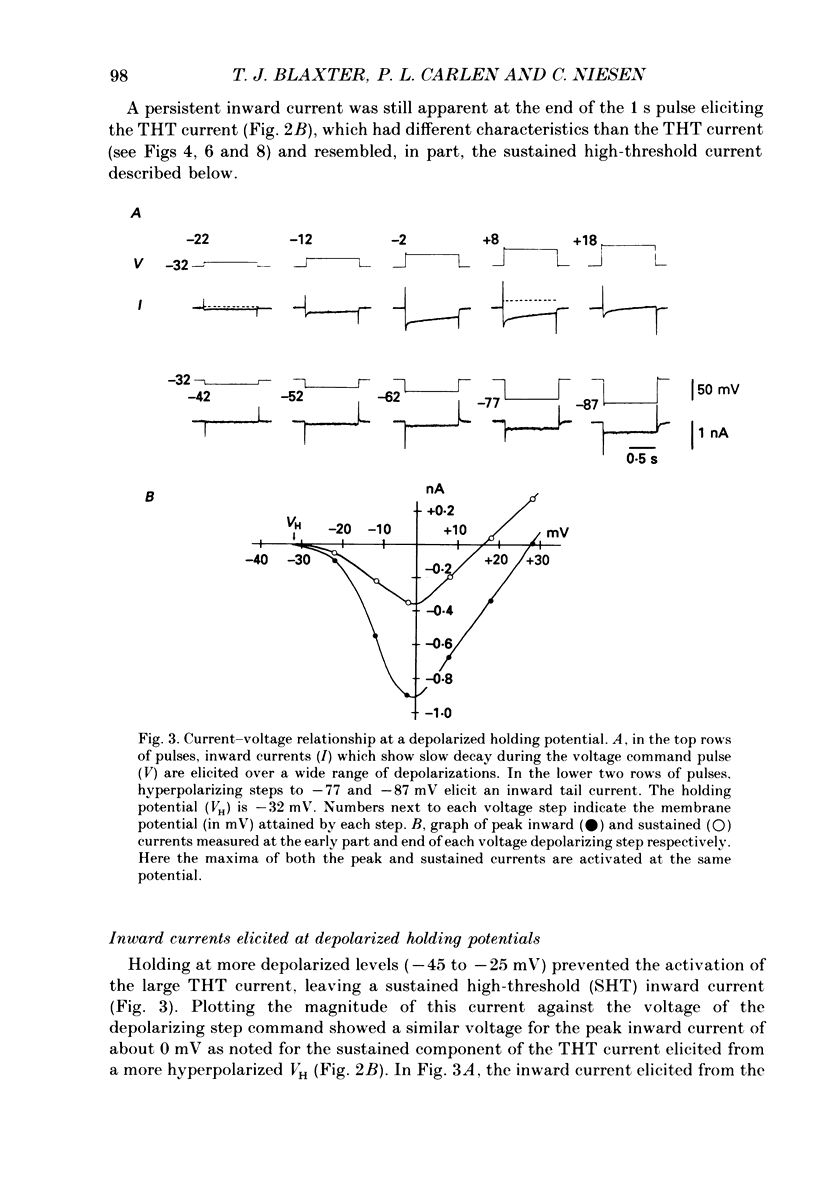
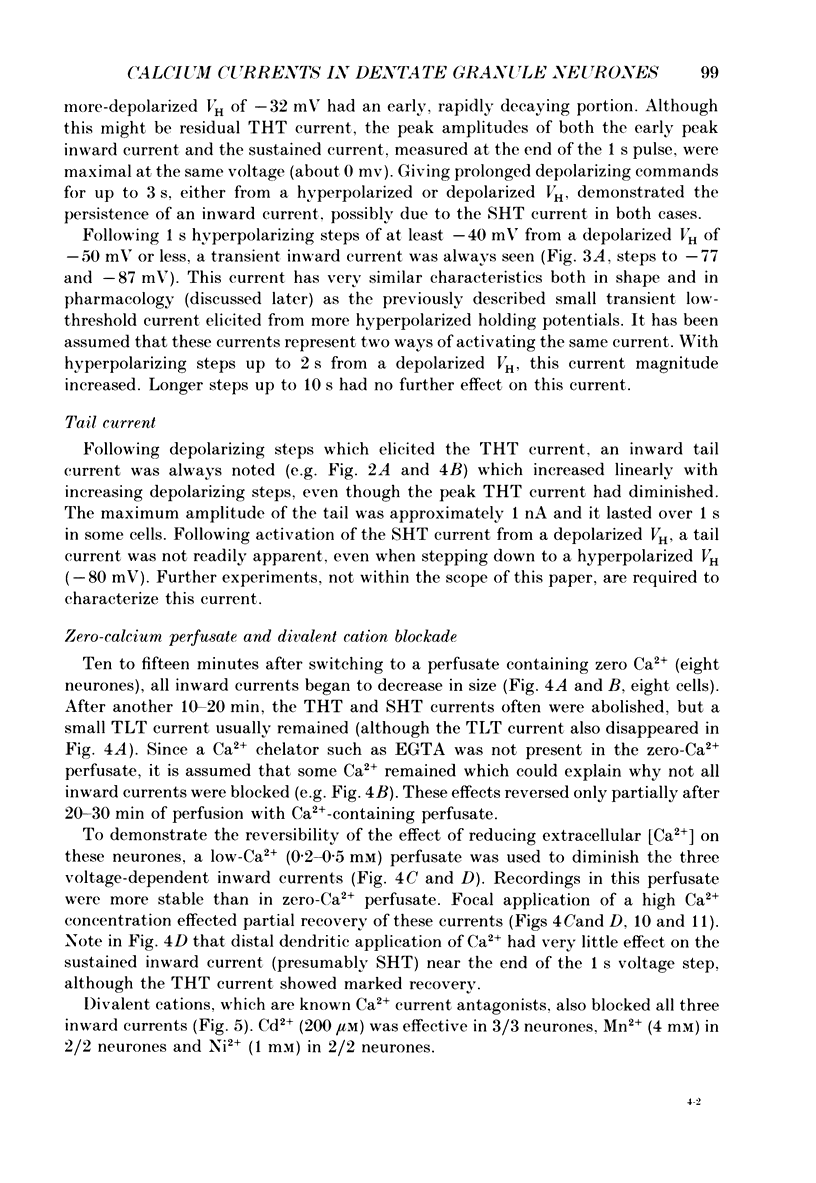
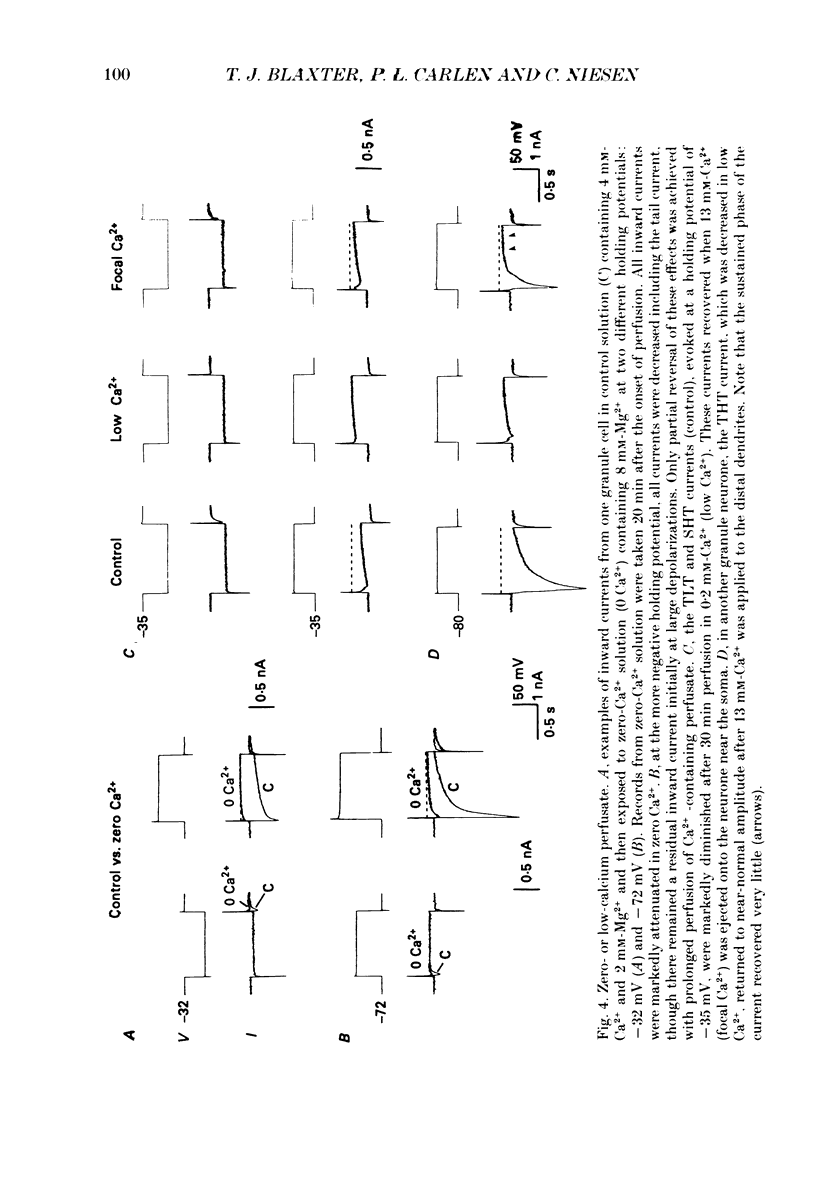
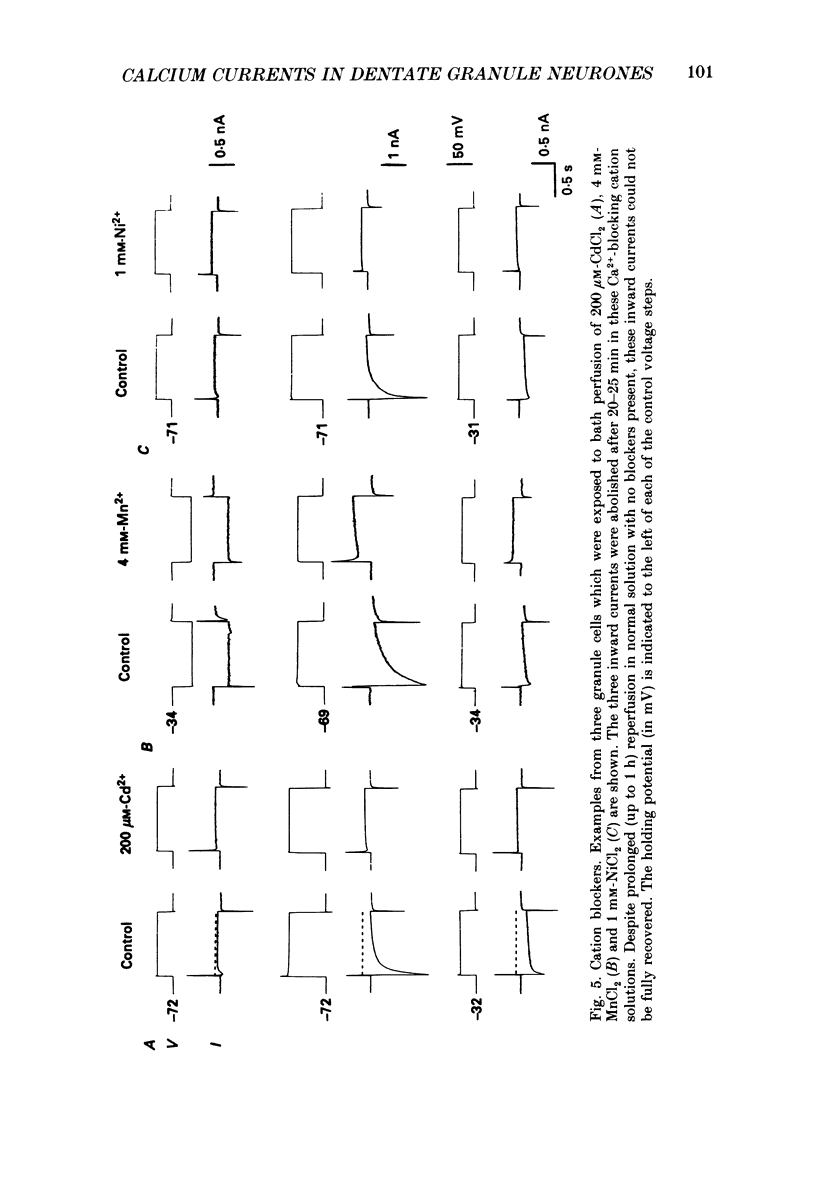
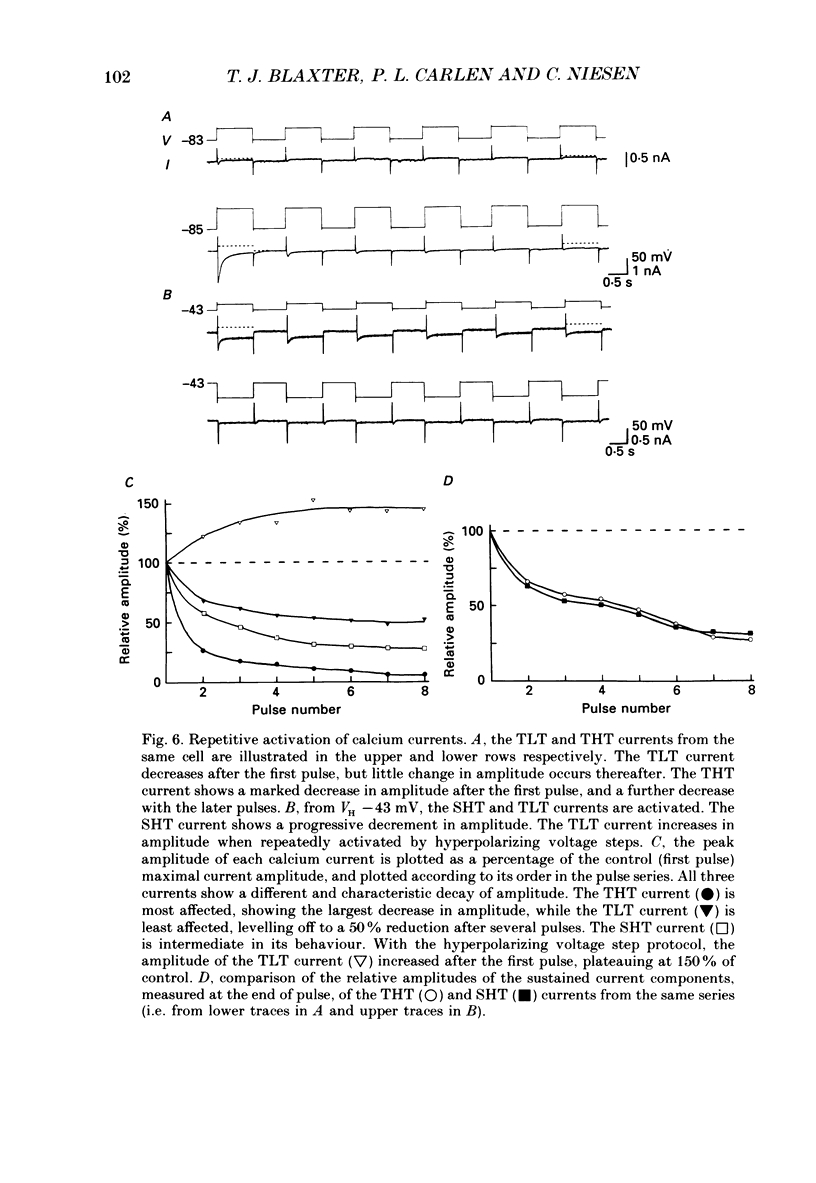
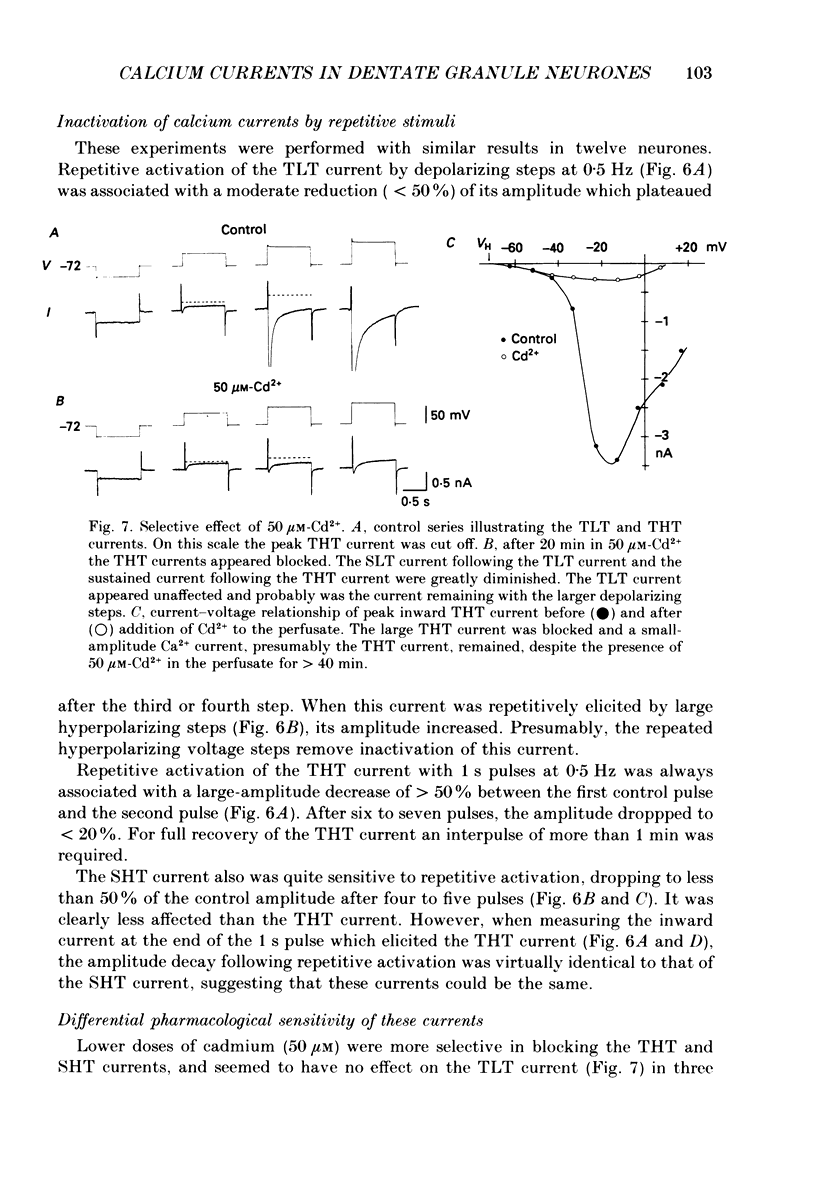
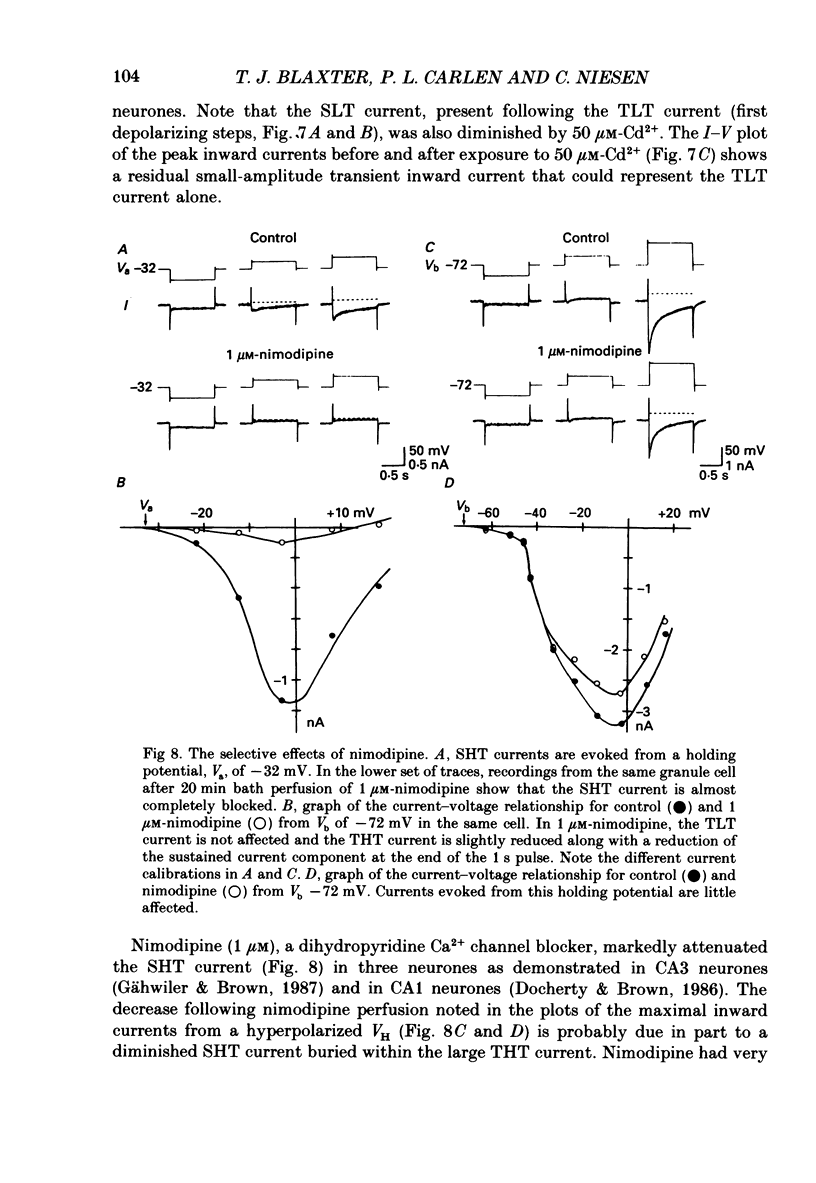
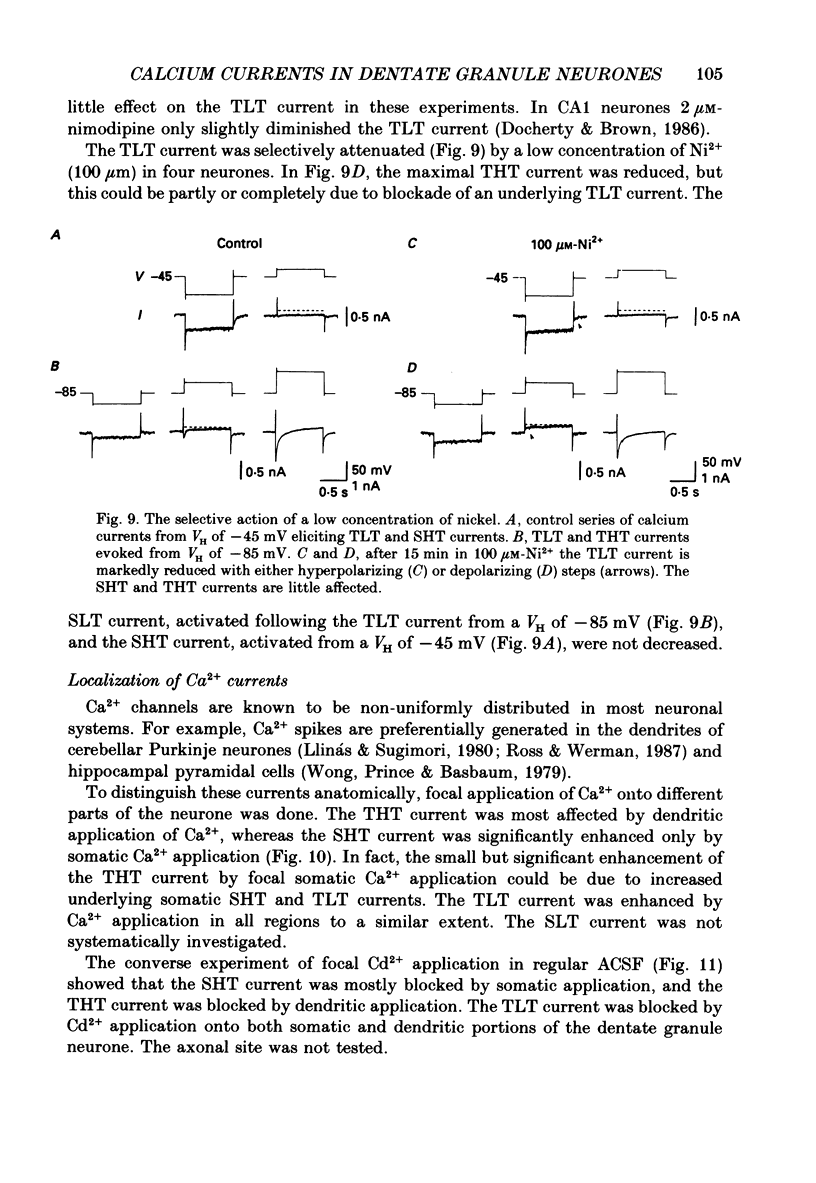
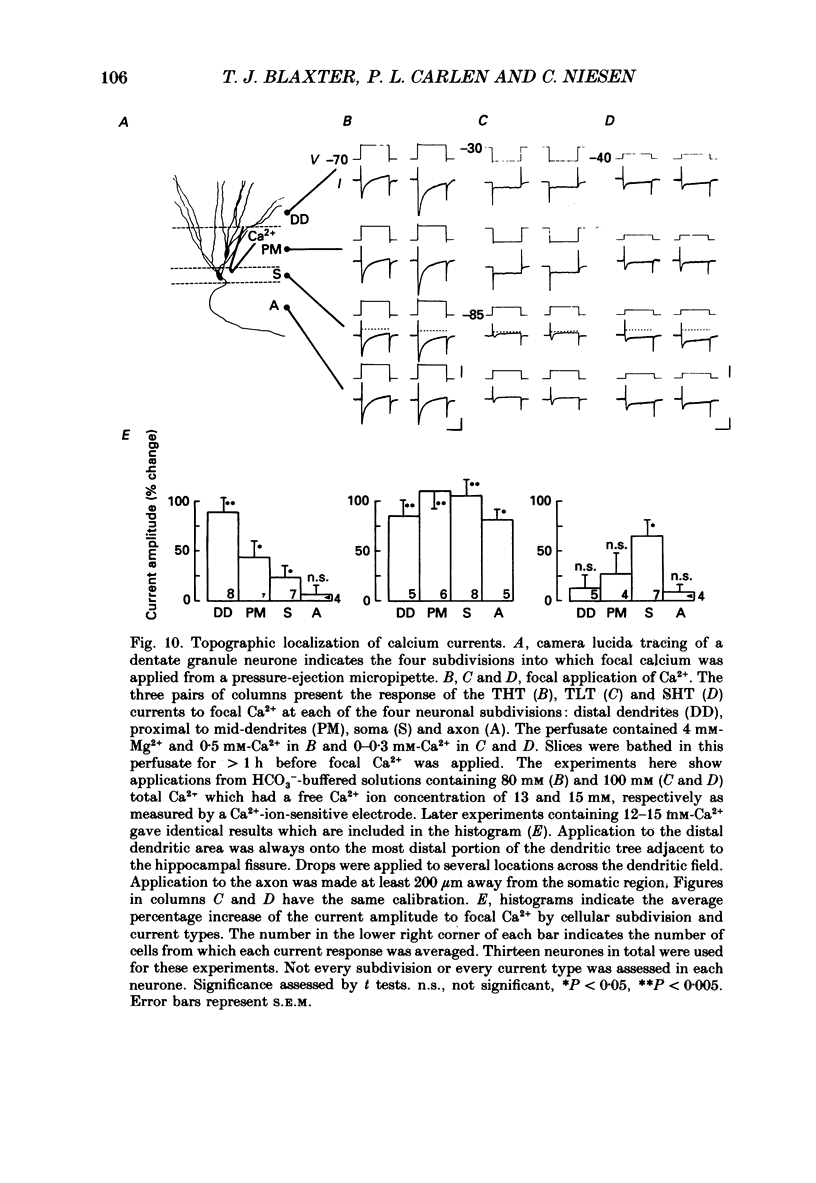
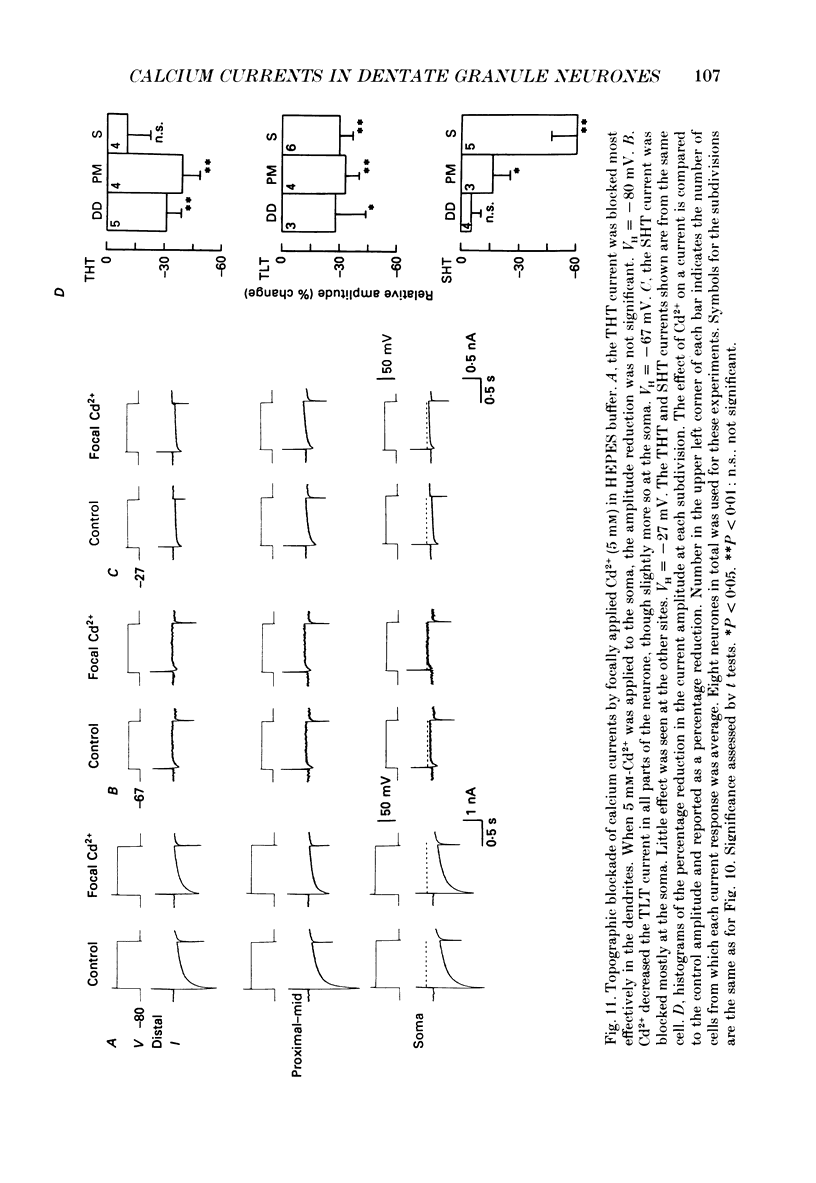
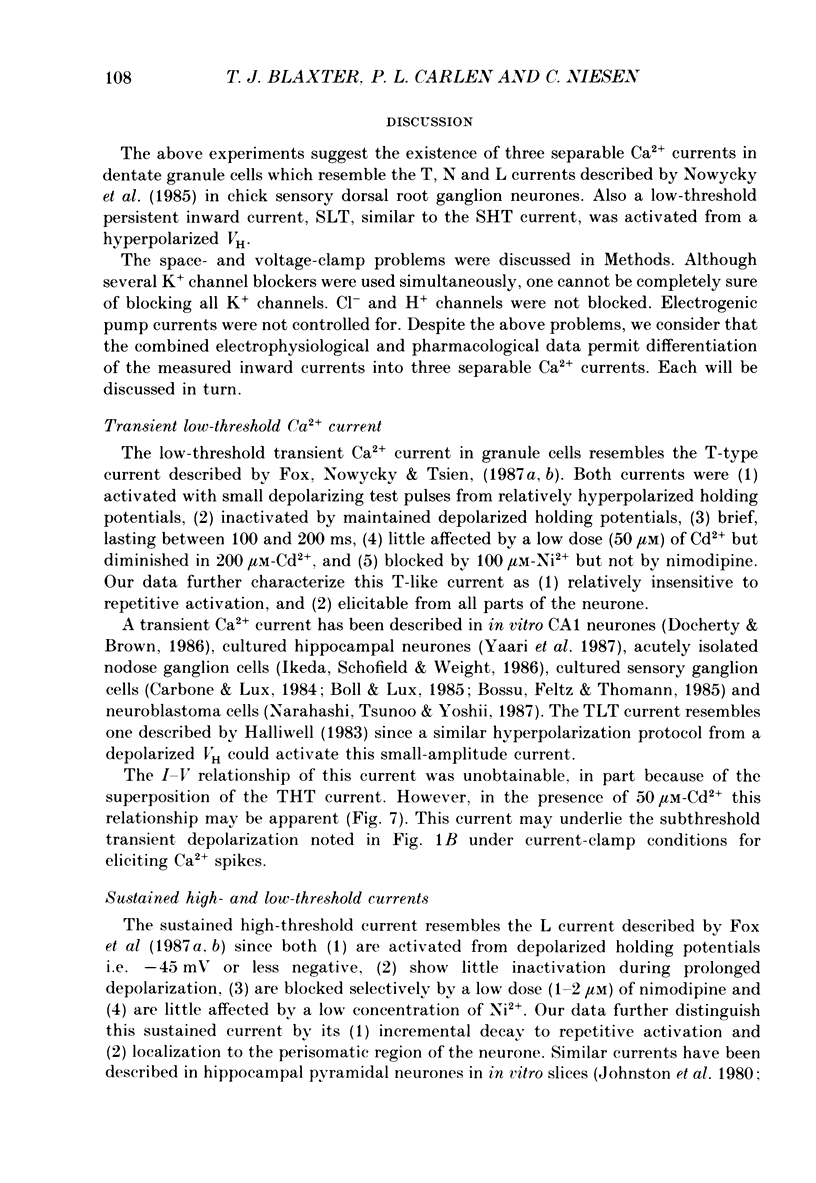
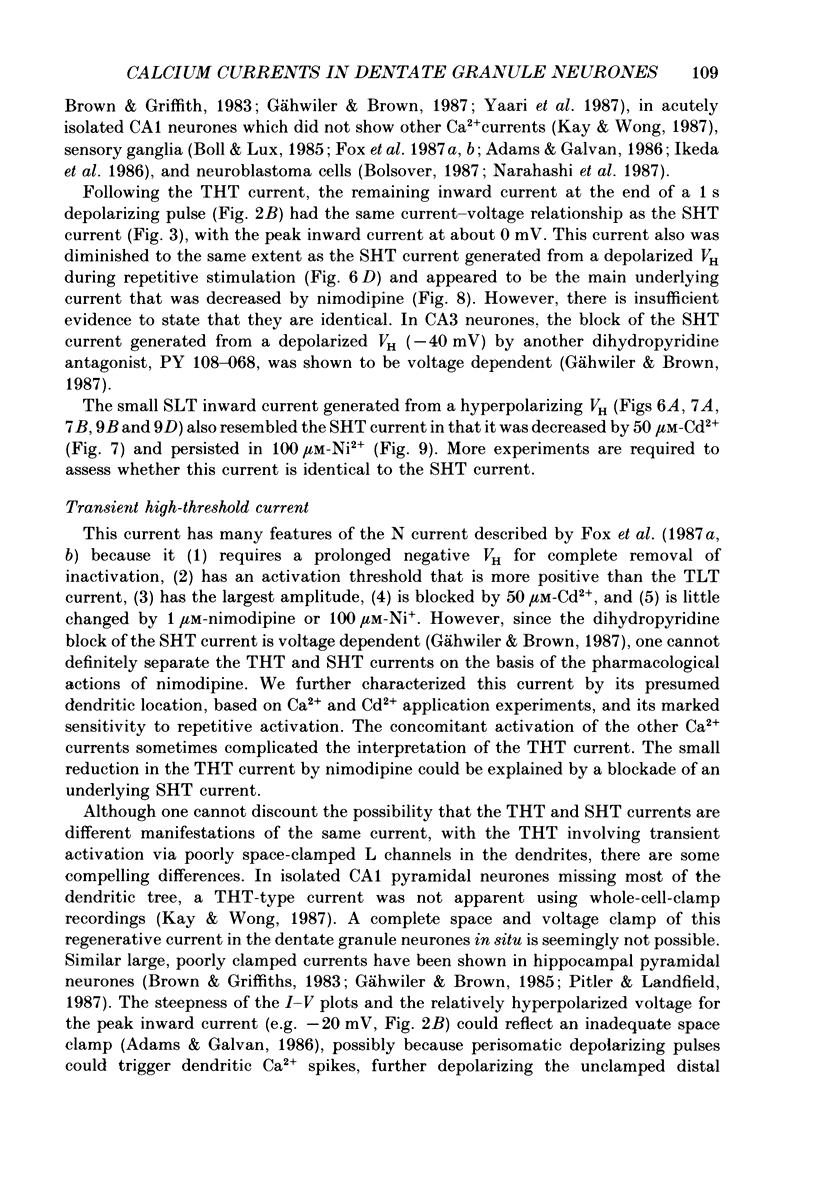
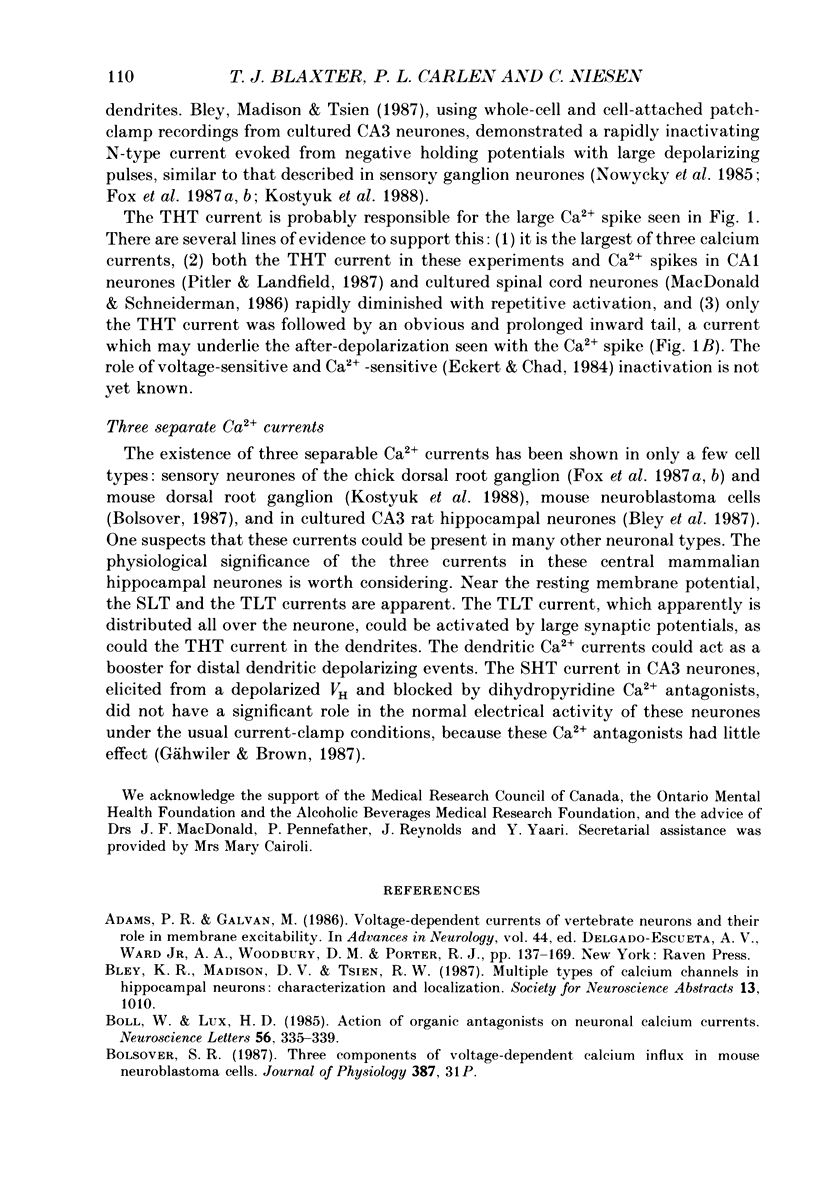
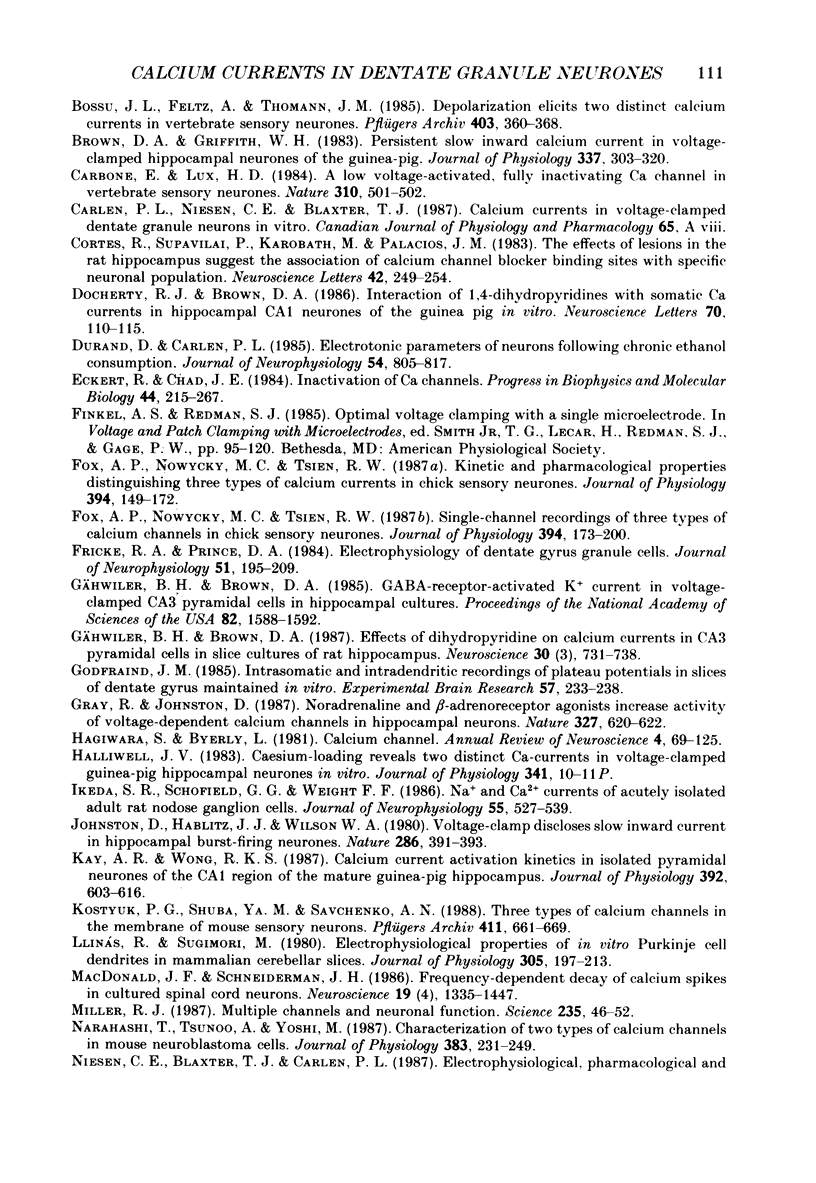
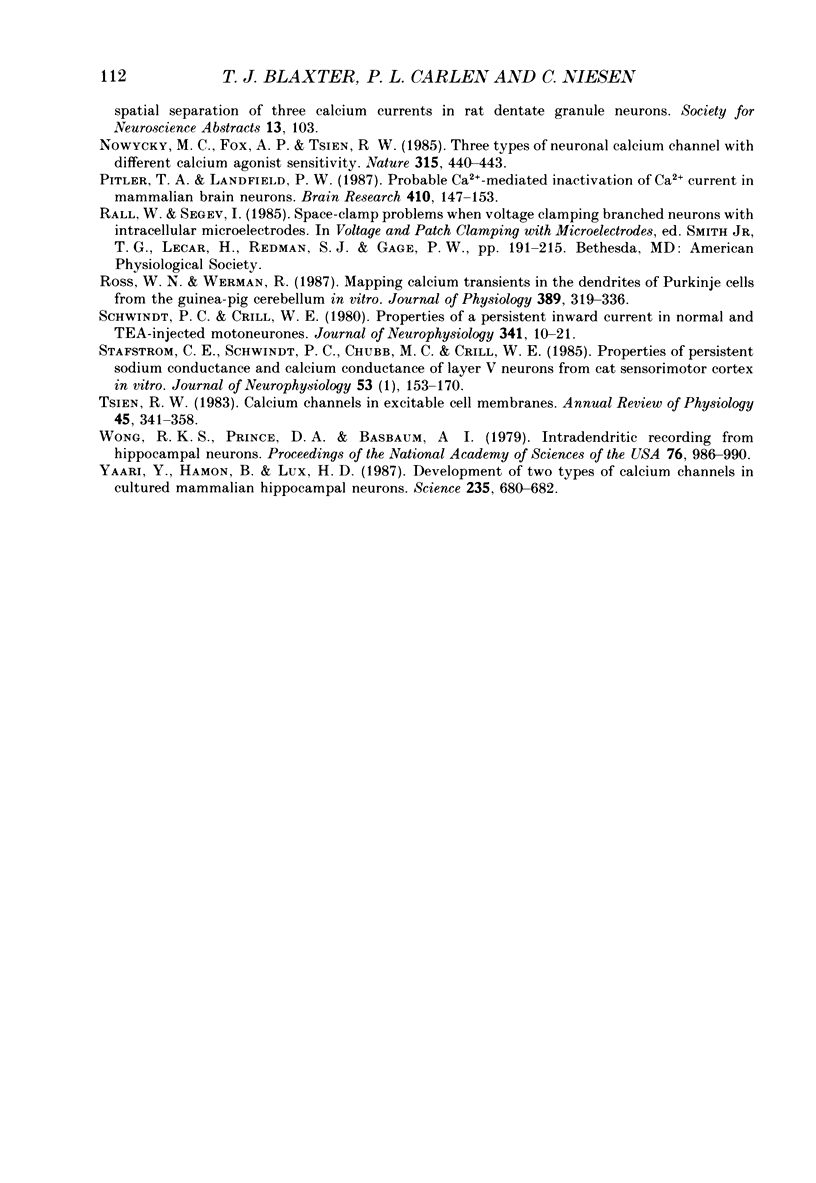
Selected References
These references are in PubMed. This may not be the complete list of references from this article.
- Adams P. R., Galvan M. Voltage-dependent currents of vertebrate neurons and their role in membrane excitability. Adv Neurol. 1986;44:137–170. [PubMed] [Google Scholar]
- Boll W., Lux H. D. Action of organic antagonists on neuronal calcium currents. Neurosci Lett. 1985 May 23;56(3):335–339. doi: 10.1016/0304-3940(85)90265-4. [DOI] [PubMed] [Google Scholar]
- Bossu J. L., Feltz A., Thomann J. M. Depolarization elicits two distinct calcium currents in vertebrate sensory neurones. Pflugers Arch. 1985 Apr;403(4):360–368. doi: 10.1007/BF00589247. [DOI] [PubMed] [Google Scholar]
- Brown D. A., Griffith W. H. Persistent slow inward calcium current in voltage-clamped hippocampal neurones of the guinea-pig. J Physiol. 1983 Apr;337:303–320. doi: 10.1113/jphysiol.1983.sp014625. [DOI] [PMC free article] [PubMed] [Google Scholar]
- Carbone E., Lux H. D. A low voltage-activated, fully inactivating Ca channel in vertebrate sensory neurones. Nature. 1984 Aug 9;310(5977):501–502. doi: 10.1038/310501a0. [DOI] [PubMed] [Google Scholar]
- Cortes R., Supavilai P., Karobath M., Palacios J. M. The effects of lesions in the rat hippocampus suggest the association of calcium channel blocker binding sites with specific neuronal population. Neurosci Lett. 1983 Dec 11;42(3):249–254. doi: 10.1016/0304-3940(83)90270-7. [DOI] [PubMed] [Google Scholar]
- Docherty R. J., Brown D. A. Interaction of 1,4-dihydropyridines with somatic Ca currents in hippocampal CA1 neurones of the guinea pig in vitro. Neurosci Lett. 1986 Sep 25;70(1):110–115. doi: 10.1016/0304-3940(86)90447-7. [DOI] [PubMed] [Google Scholar]
- Durand D., Carlen P. Electrotonic parameters of neurons following chronic ethanol consumption. J Neurophysiol. 1985 Oct;54(4):807–817. doi: 10.1152/jn.1985.54.4.807. [DOI] [PubMed] [Google Scholar]
- Eckert R., Chad J. E. Inactivation of Ca channels. Prog Biophys Mol Biol. 1984;44(3):215–267. doi: 10.1016/0079-6107(84)90009-9. [DOI] [PubMed] [Google Scholar]
- Fox A. P., Nowycky M. C., Tsien R. W. Kinetic and pharmacological properties distinguishing three types of calcium currents in chick sensory neurones. J Physiol. 1987 Dec;394:149–172. doi: 10.1113/jphysiol.1987.sp016864. [DOI] [PMC free article] [PubMed] [Google Scholar]
- Fox A. P., Nowycky M. C., Tsien R. W. Single-channel recordings of three types of calcium channels in chick sensory neurones. J Physiol. 1987 Dec;394:173–200. doi: 10.1113/jphysiol.1987.sp016865. [DOI] [PMC free article] [PubMed] [Google Scholar]
- Fricke R. A., Prince D. A. Electrophysiology of dentate gyrus granule cells. J Neurophysiol. 1984 Feb;51(2):195–209. doi: 10.1152/jn.1984.51.2.195. [DOI] [PubMed] [Google Scholar]
- Godfraind J. M. Intrasomatic and intradendritic recordings of plateau potentials in slices of the dentate gyrus maintained in vitro. Exp Brain Res. 1985;57(2):233–238. doi: 10.1007/BF00236528. [DOI] [PubMed] [Google Scholar]
- Gray R., Johnston D. Noradrenaline and beta-adrenoceptor agonists increase activity of voltage-dependent calcium channels in hippocampal neurons. Nature. 1987 Jun 18;327(6123):620–622. doi: 10.1038/327620a0. [DOI] [PubMed] [Google Scholar]
- Gähwiler B. H., Brown D. A. Effects of dihydropyridines on calcium currents in CA3 pyramidal cells in slice cultures of rat hippocampus. Neuroscience. 1987 Mar;20(3):731–738. doi: 10.1016/0306-4522(87)90236-3. [DOI] [PubMed] [Google Scholar]
- Hagiwara S., Byerly L. Calcium channel. Annu Rev Neurosci. 1981;4:69–125. doi: 10.1146/annurev.ne.04.030181.000441. [DOI] [PubMed] [Google Scholar]
- Ikeda S. R., Schofield G. G., Weight F. F. Na+ and Ca2+ currents of acutely isolated adult rat nodose ganglion cells. J Neurophysiol. 1986 Mar;55(3):527–539. doi: 10.1152/jn.1986.55.3.527. [DOI] [PubMed] [Google Scholar]
- Johnston D., Hablitz J. J., Wilson W. A. Voltage clamp discloses slow inward current in hippocampal burst-firing neurones. Nature. 1980 Jul 24;286(5771):391–393. doi: 10.1038/286391a0. [DOI] [PubMed] [Google Scholar]
- Kay A. R., Wong R. K. Calcium current activation kinetics in isolated pyramidal neurones of the Ca1 region of the mature guinea-pig hippocampus. J Physiol. 1987 Nov;392:603–616. doi: 10.1113/jphysiol.1987.sp016799. [DOI] [PMC free article] [PubMed] [Google Scholar]
- Kostyuk P. G., Shuba YaM, Savchenko A. N. Three types of calcium channels in the membrane of mouse sensory neurons. Pflugers Arch. 1988 Jun;411(6):661–669. doi: 10.1007/BF00580863. [DOI] [PubMed] [Google Scholar]
- Llinás R., Sugimori M. Electrophysiological properties of in vitro Purkinje cell dendrites in mammalian cerebellar slices. J Physiol. 1980 Aug;305:197–213. doi: 10.1113/jphysiol.1980.sp013358. [DOI] [PMC free article] [PubMed] [Google Scholar]
- MacDonald J. F., Schneiderman J. H. Frequency-dependent decay of calcium spikes in cultured spinal cord neurons. Neuroscience. 1986 Dec;19(4):1335–1347. doi: 10.1016/0306-4522(86)90147-8. [DOI] [PubMed] [Google Scholar]
- Miller R. J. Multiple calcium channels and neuronal function. Science. 1987 Jan 2;235(4784):46–52. doi: 10.1126/science.2432656. [DOI] [PubMed] [Google Scholar]
- Narahashi T., Tsunoo A., Yoshii M. Characterization of two types of calcium channels in mouse neuroblastoma cells. J Physiol. 1987 Feb;383:231–249. doi: 10.1113/jphysiol.1987.sp016406. [DOI] [PMC free article] [PubMed] [Google Scholar]
- Pitler T. A., Landfield P. W. Probable Ca2+-mediated inactivation of Ca2+ currents in mammalian brain neurons. Brain Res. 1987 Apr 28;410(1):147–153. doi: 10.1016/s0006-8993(87)80037-9. [DOI] [PubMed] [Google Scholar]
- Ross W. N., Werman R. Mapping calcium transients in the dendrites of Purkinje cells from the guinea-pig cerebellum in vitro. J Physiol. 1987 Aug;389:319–336. doi: 10.1113/jphysiol.1987.sp016659. [DOI] [PMC free article] [PubMed] [Google Scholar]
- Stafstrom C. E., Schwindt P. C., Chubb M. C., Crill W. E. Properties of persistent sodium conductance and calcium conductance of layer V neurons from cat sensorimotor cortex in vitro. J Neurophysiol. 1985 Jan;53(1):153–170. doi: 10.1152/jn.1985.53.1.153. [DOI] [PubMed] [Google Scholar]
- Tsien R. W. Calcium channels in excitable cell membranes. Annu Rev Physiol. 1983;45:341–358. doi: 10.1146/annurev.ph.45.030183.002013. [DOI] [PubMed] [Google Scholar]
- Wong R. K., Prince D. A., Basbaum A. I. Intradendritic recordings from hippocampal neurons. Proc Natl Acad Sci U S A. 1979 Feb;76(2):986–990. doi: 10.1073/pnas.76.2.986. [DOI] [PMC free article] [PubMed] [Google Scholar]
- Yaari Y., Hamon B., Lux H. D. Development of two types of calcium channels in cultured mammalian hippocampal neurons. Science. 1987 Feb 6;235(4789):680–682. doi: 10.1126/science.2433765. [DOI] [PubMed] [Google Scholar]