Abstract
1. A single-microelectrode technique has been used to study the voltage dependence and the kinetics of inactivation and reactivation of a tetrodotoxin-resistant inward current (ICa) in single cells from bull-frog atrium. 2. In most cases the kinetics of both inactivation and reactivation can be well described as a single-exponential process. 3. Several different observations indicate that inactivation of ICa in these cells is controlled by both voltage-dependent and current-dependent processes, as has been demonstrated previously in heart (Kass & Sanguinetti, 1984; Lee, Marban & Tsien, 1985) and in other tissues (Hagiwara & Byerly, 1981; Tsien, 1983; Eckert & Chad, 1984). 4. Evidence in favour of a voltage-dependent inactivation mechanism included: (a) In paired-pulse measurements of steady-state inactivation ('f infinity') a 'conventional' steady-state f infinity vs. membrane potential (Vm) relationship was obtained in the range of membrane potentials from -60 to 0 mV. (b) Increasing [Ca2+]o from 2.5 to 7.5 mM, which resulted in a 2-3-fold increase in ICa, did not produce any significant increase in the amount of inactivation. (c) Using a 'gapped' double-pulse protocol non-monotonic U-shaped inactivation relationships were obtained, i.e. positive to approximately +20 mV some removal of inactivation occurred. However, f never approached a value near 1.00 at very depolarized potentials; it reached a maximum between 0.5 and 0.6. (d) In constant [Ca2+]o and at fixed Vm, the kinetics of ICa inactivation were independent of peak size of ICa. This was demonstrated by: (i) varying the holding potential (-90 to -30 mV), (ii) using paired-pulse 'recovery' protocols, and (iii) partial block by La3+ (1-10 microM) and Cd2+ (0.1 mM). (e) Influx of Ca2+ ions was not an obligatory prerequisite for development of inactivation. In all ionic conditions (Ca2+, Sr2+, Ba2+, Na+-free and Ca2+-free Ringer solutions) currents displayed inactivation phenomena, although the extent and kinetics of inactivation were dependent upon ionic conditions. Outward currents recorded above the reversal potential for ICa exhibited time- and voltage-dependent inactivation, and could be inactivated by brief depolarizing pre-pulses that produced no net inward current flow. Evidence against a possible role of the electrogenic Na+-Ca2+ exchanger in producing inactivation of these outward currents was obtained.(ABSTRACT TRUNCATED AT 400 WORDS)
Full text
PDF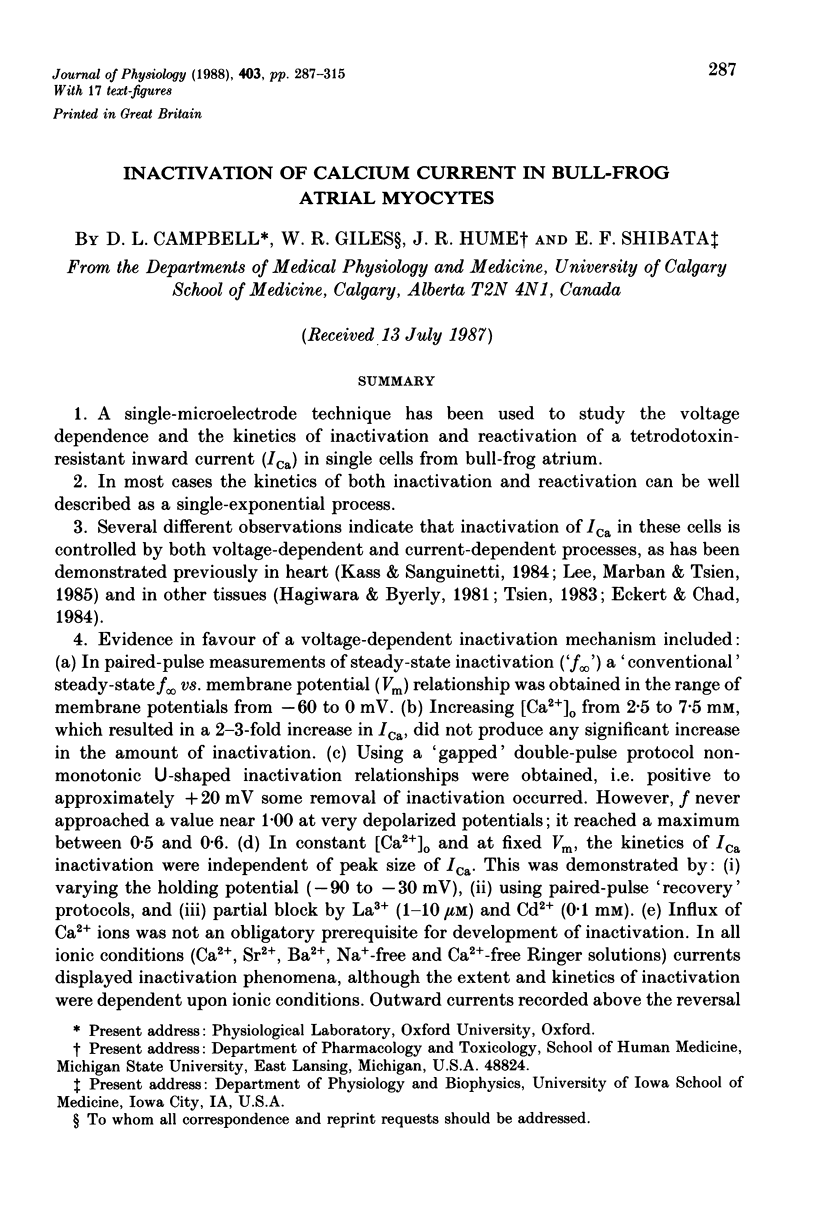
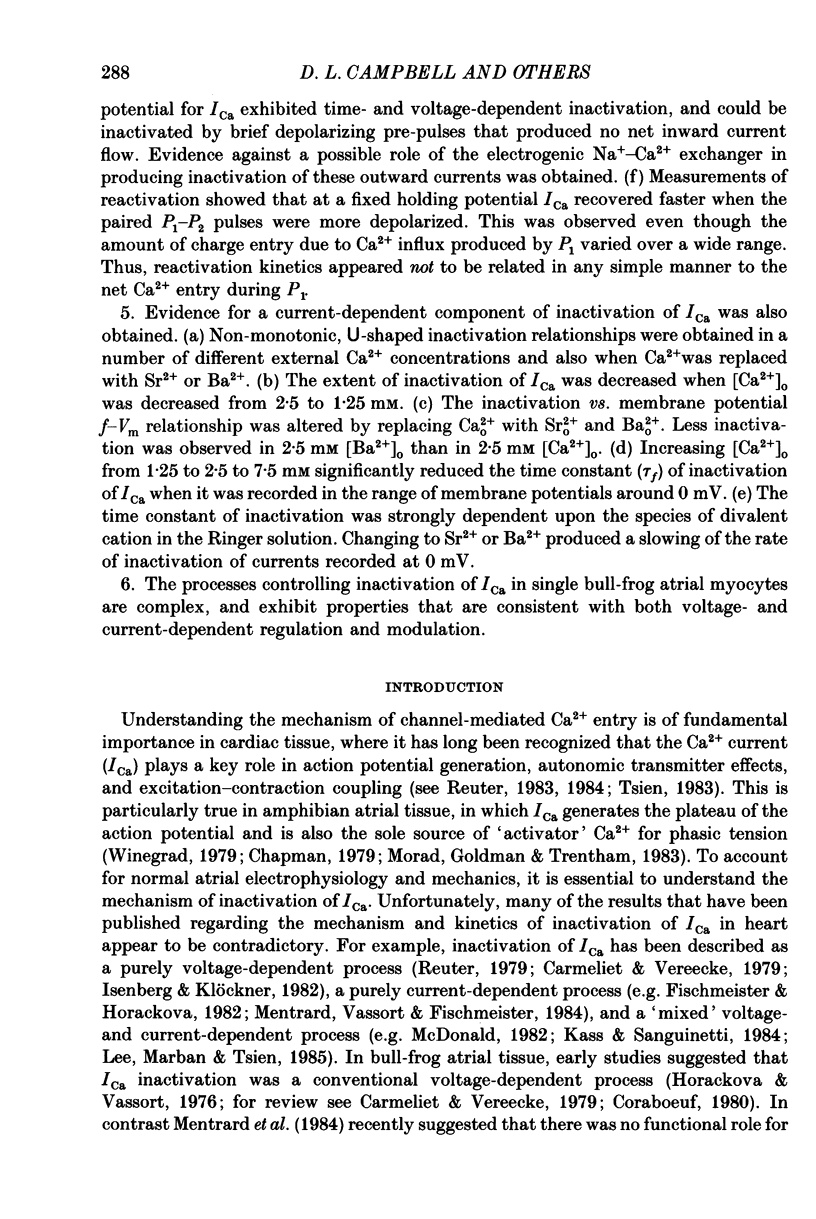
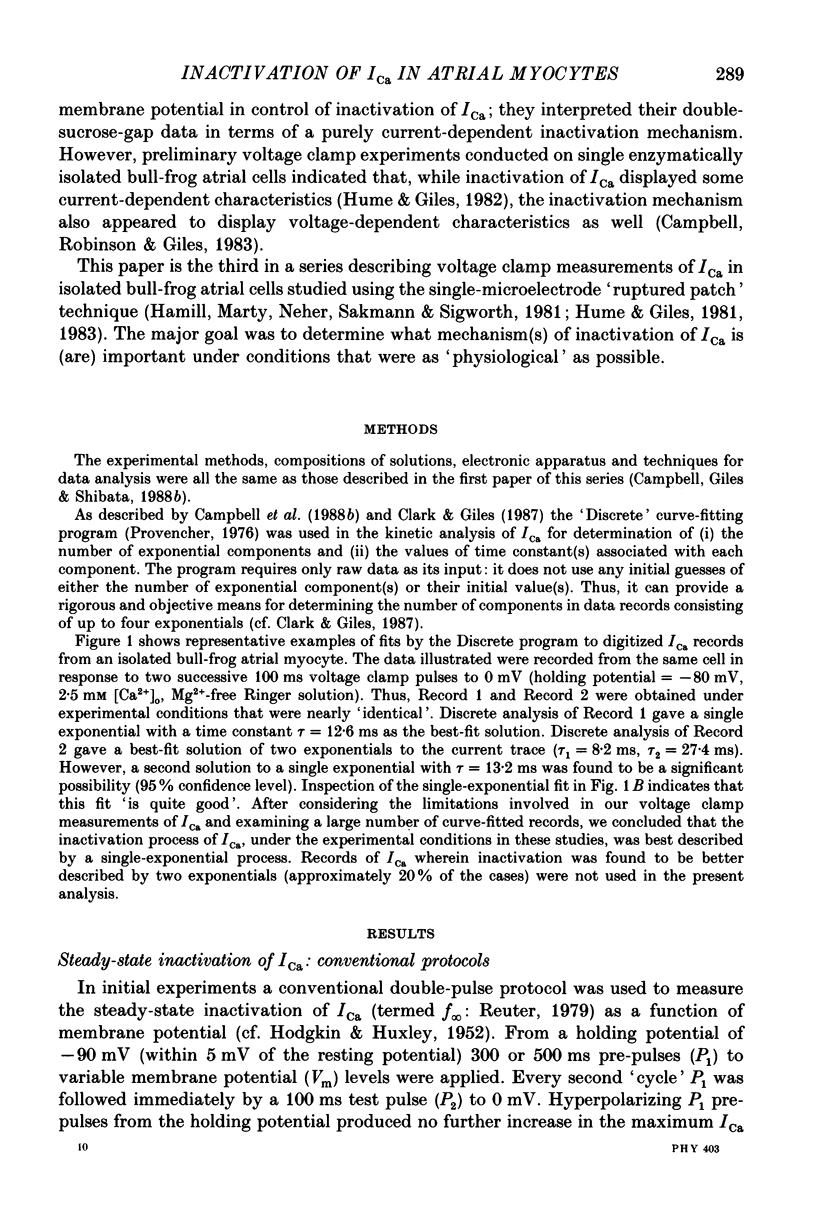
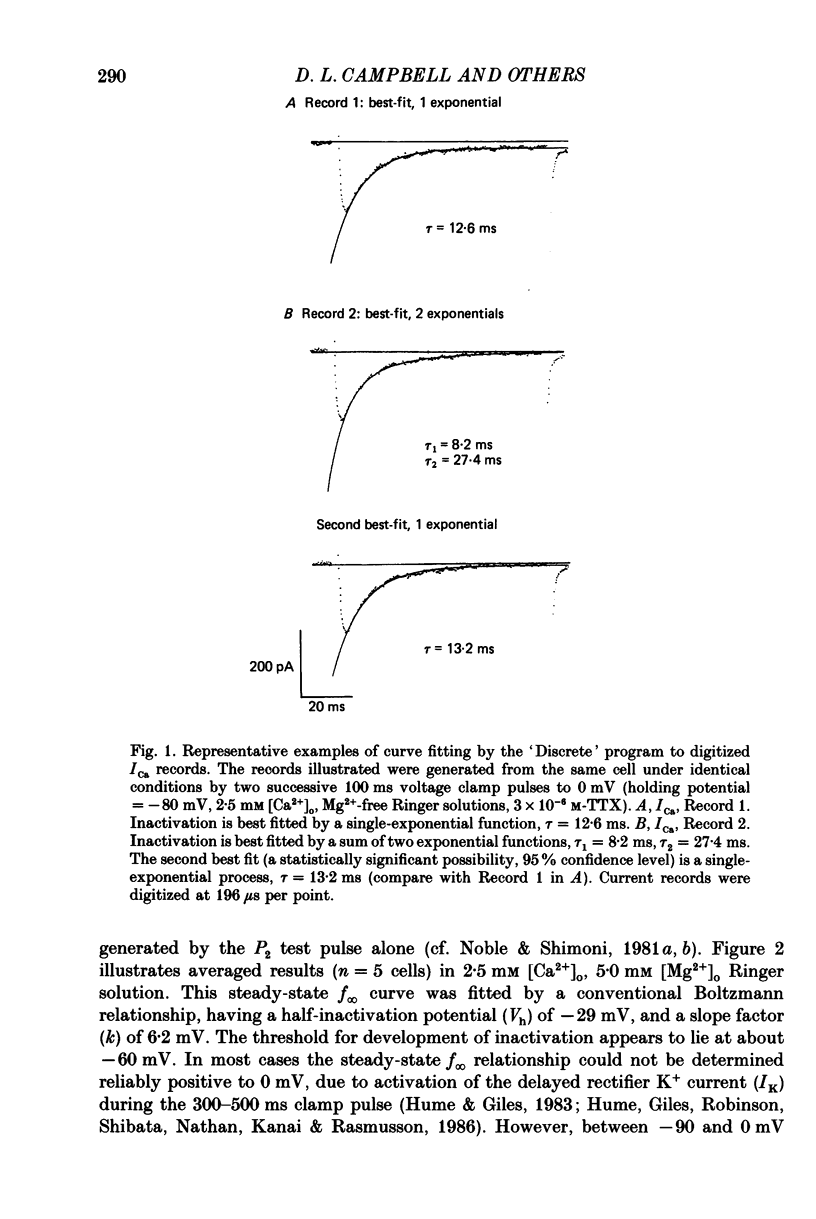
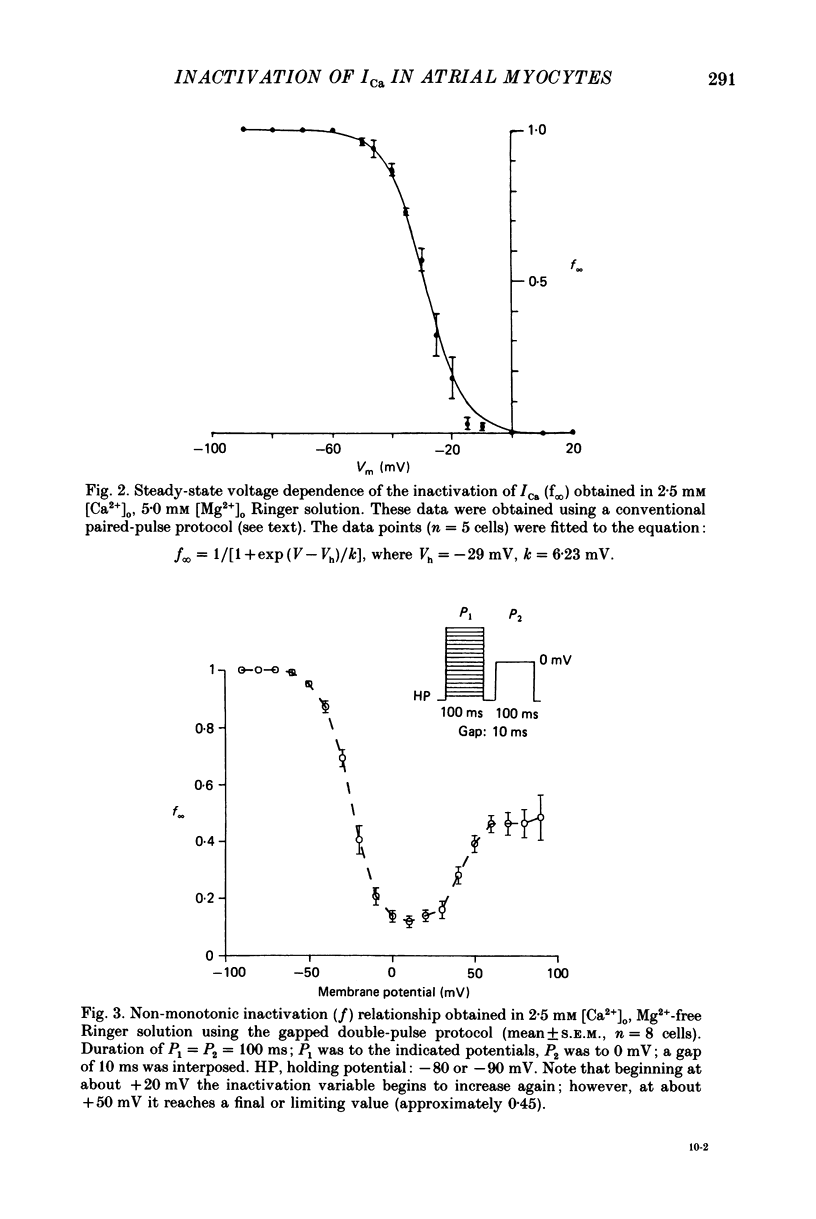
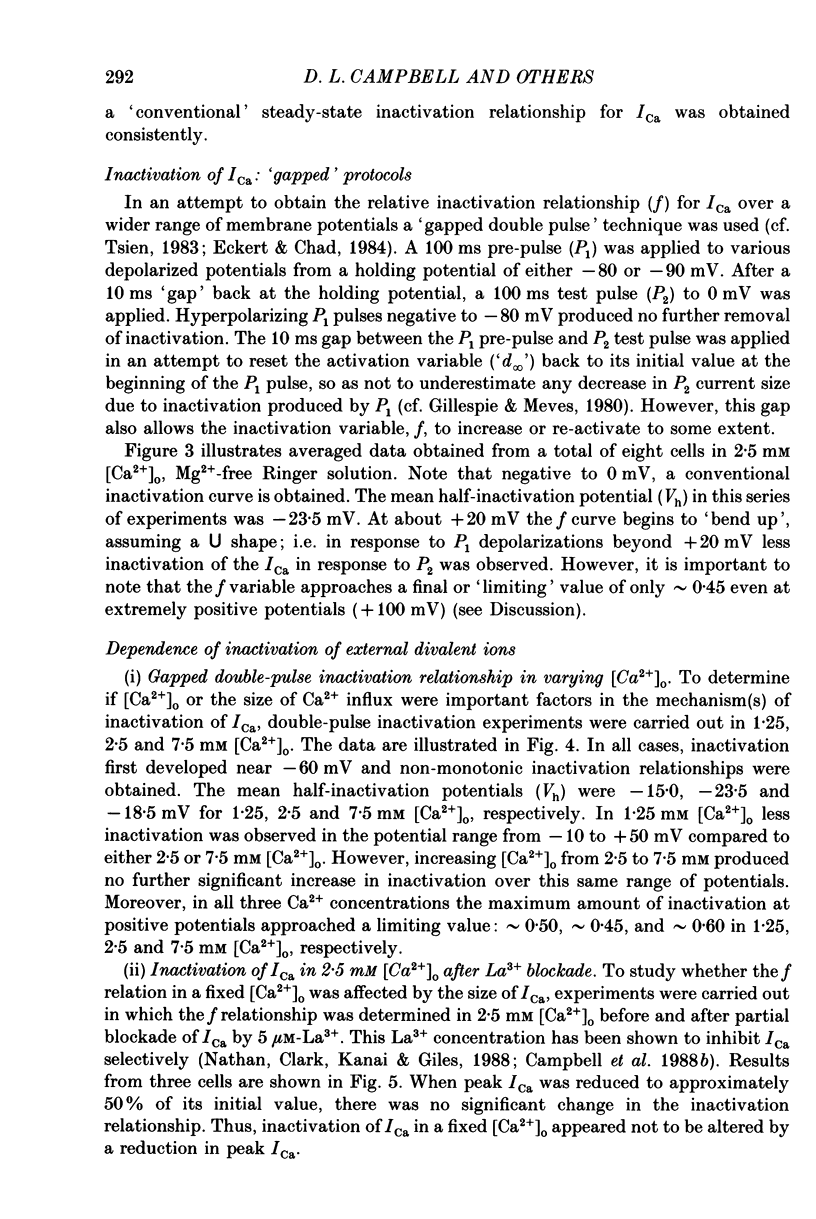
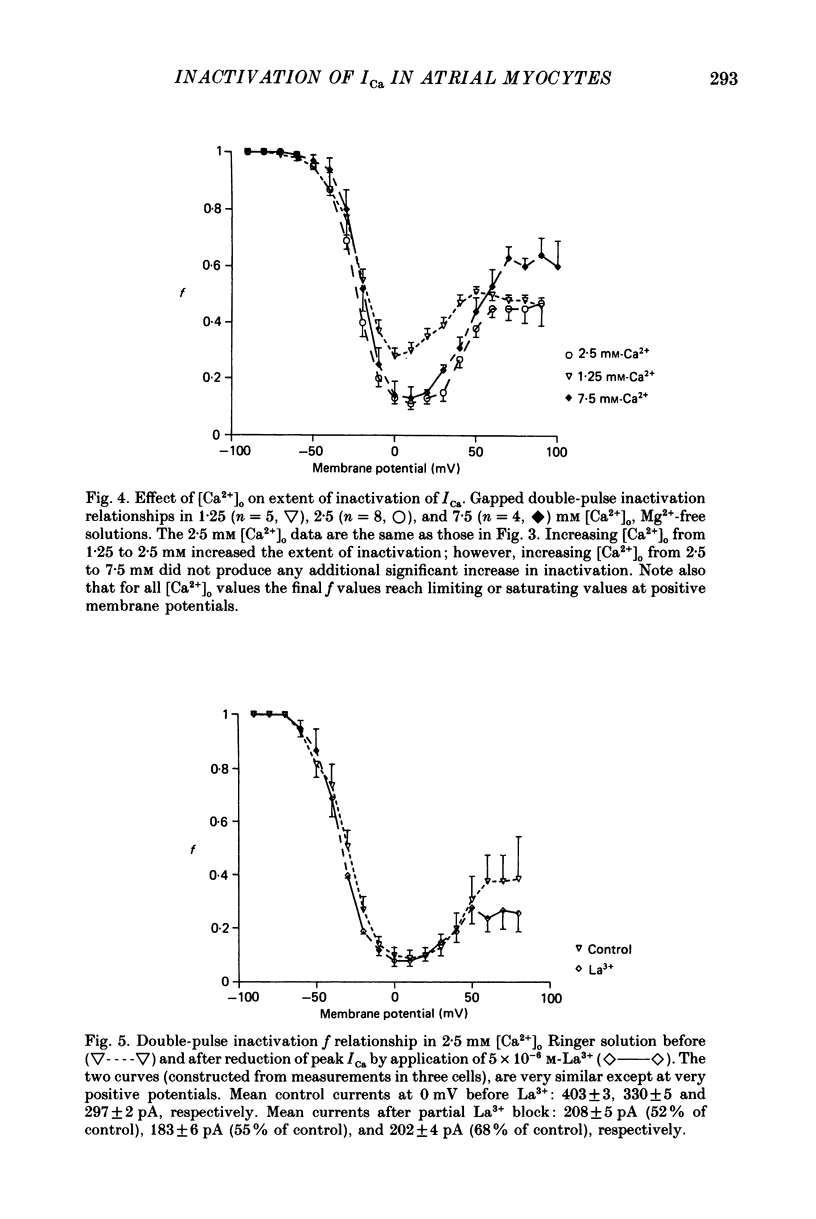
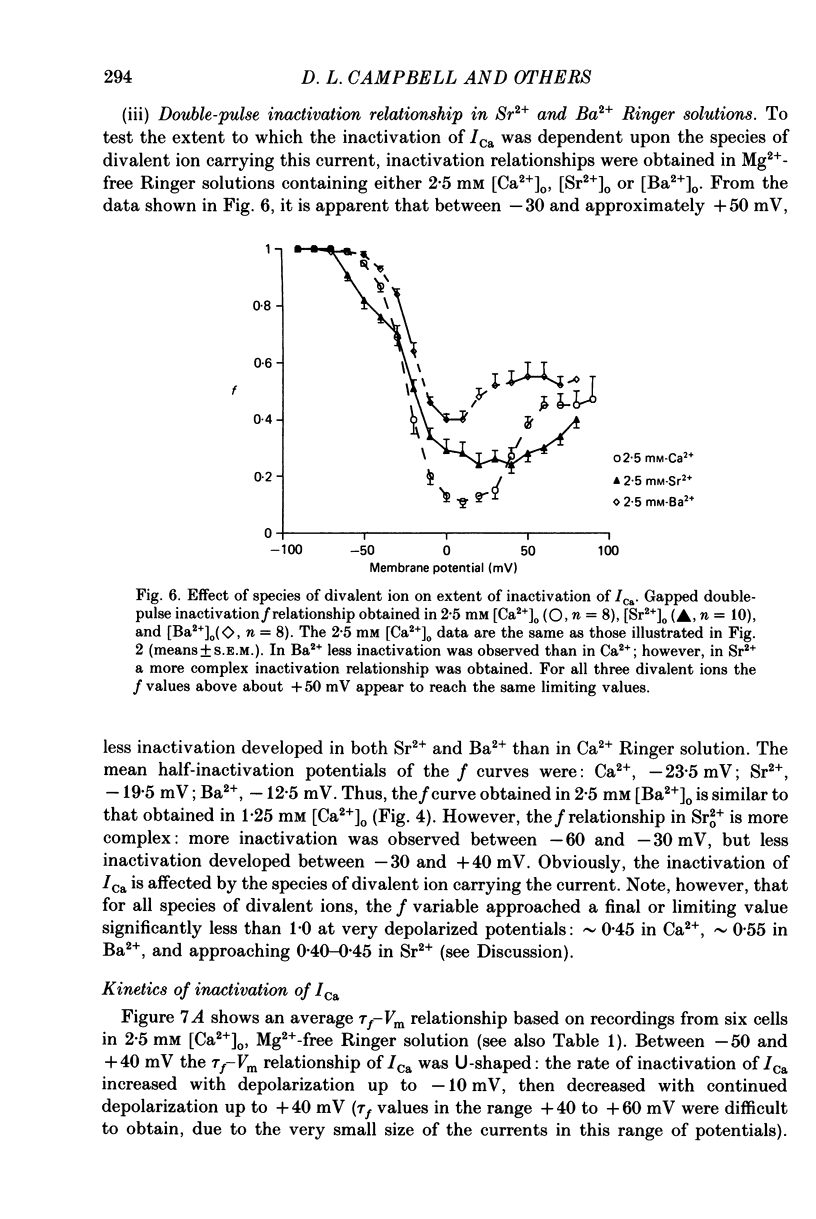
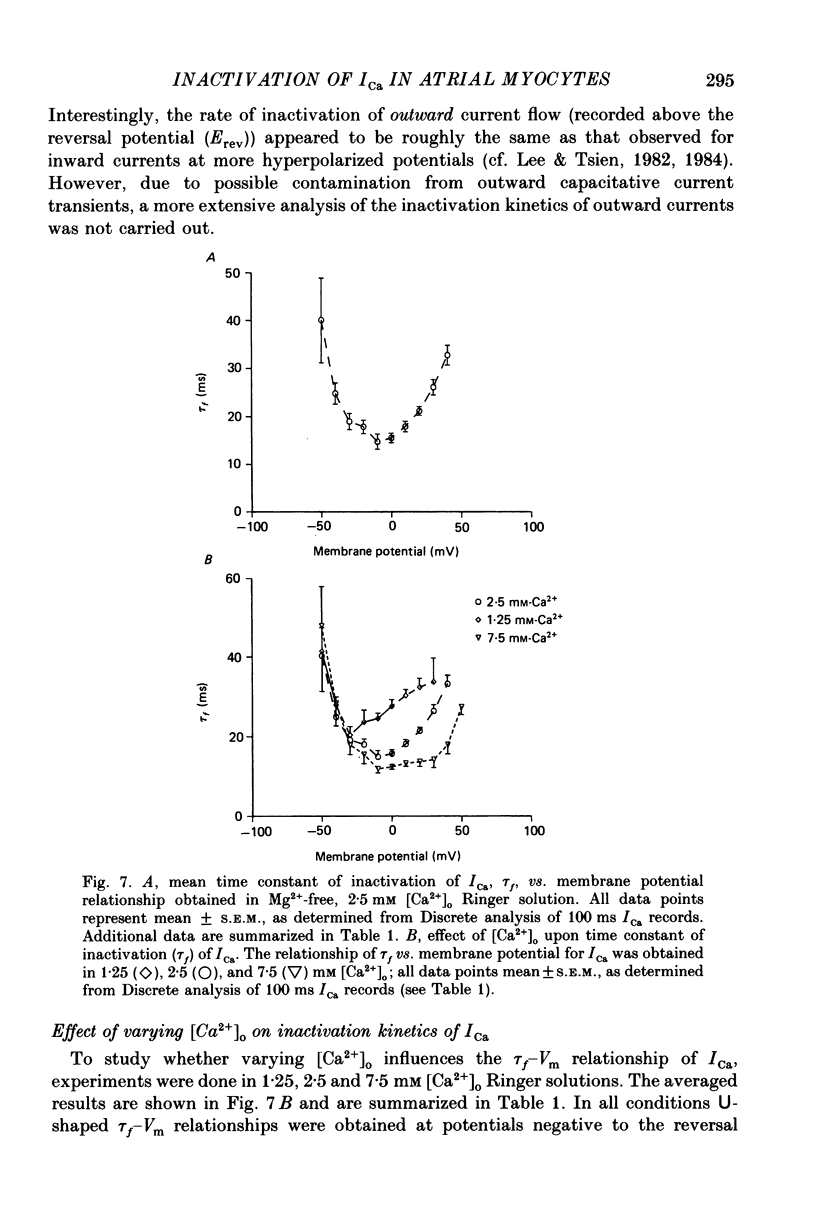
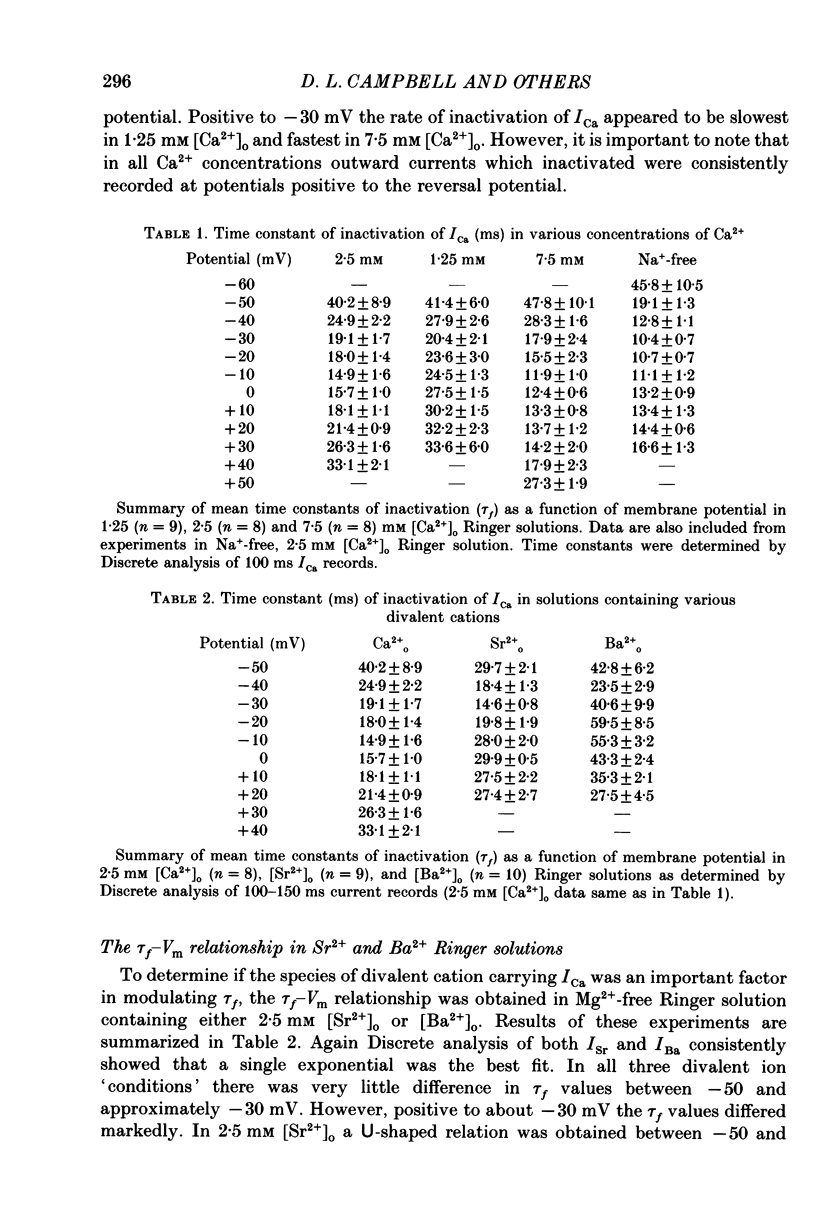
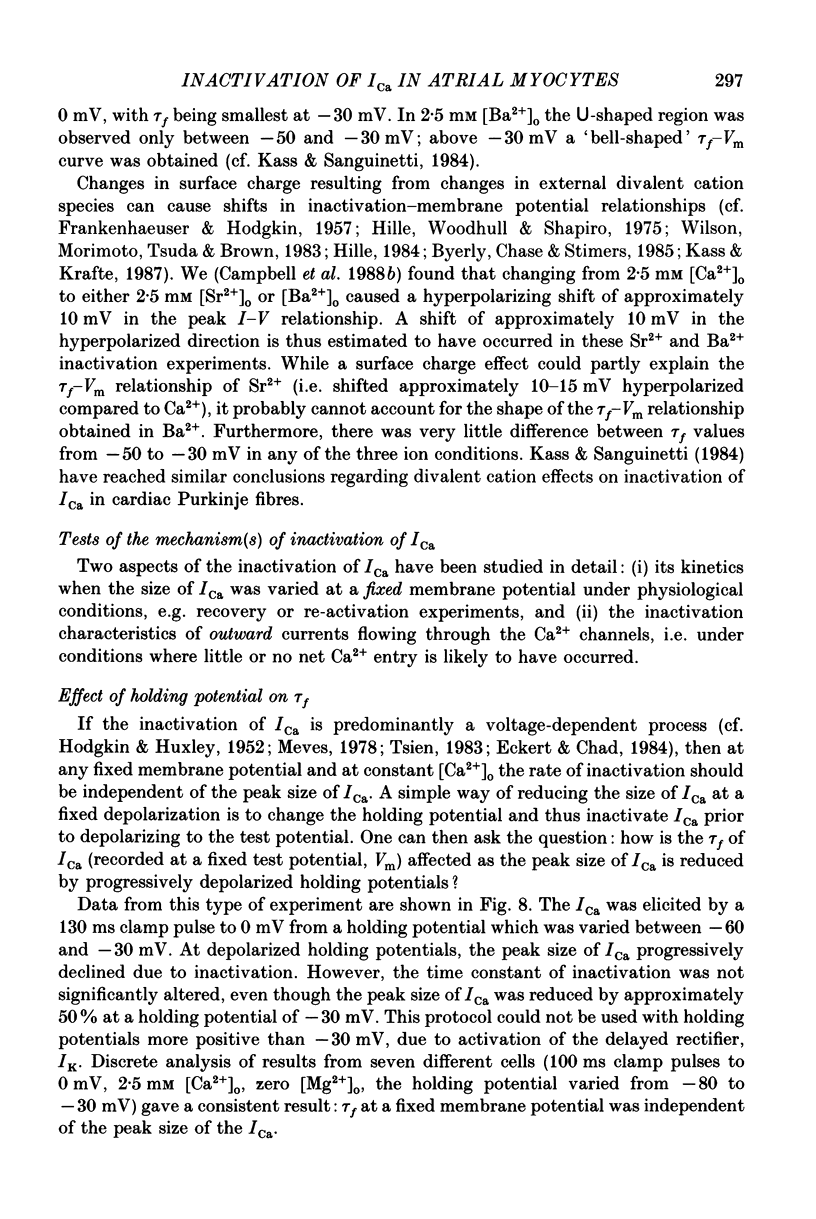
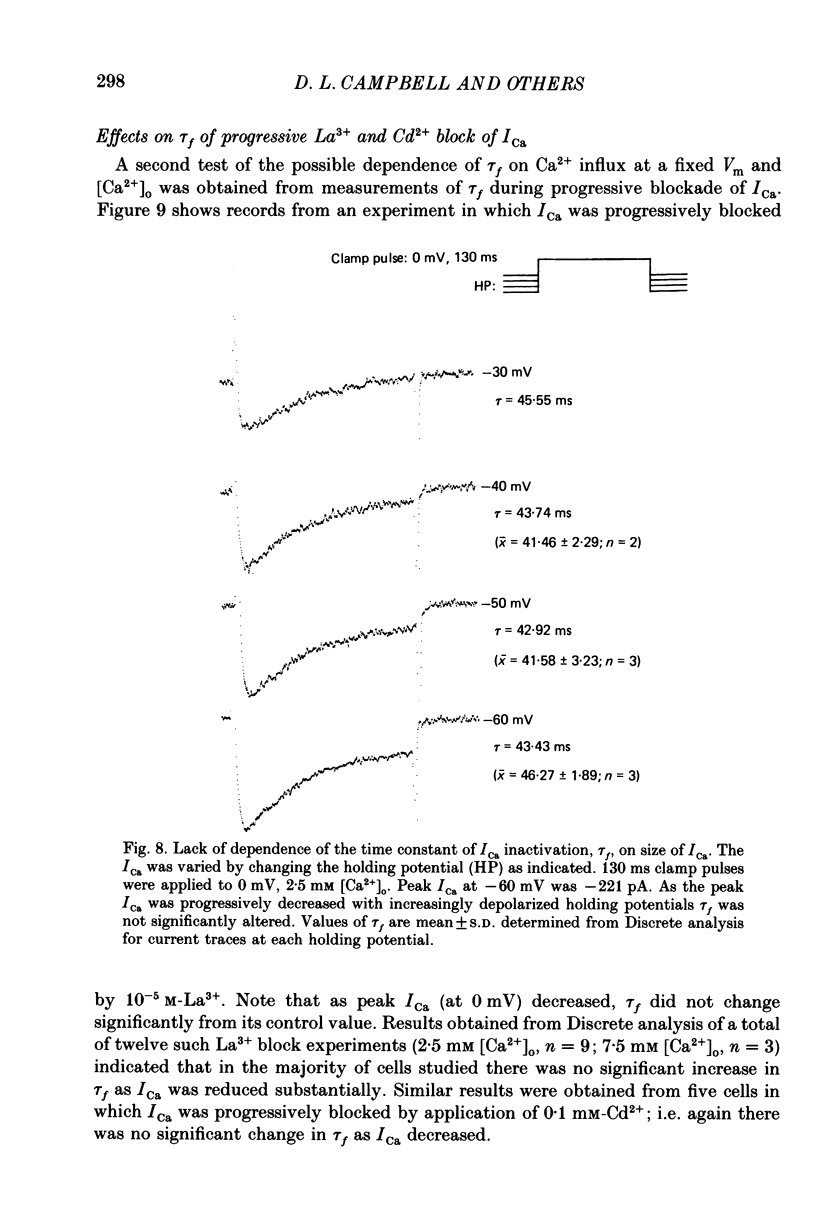
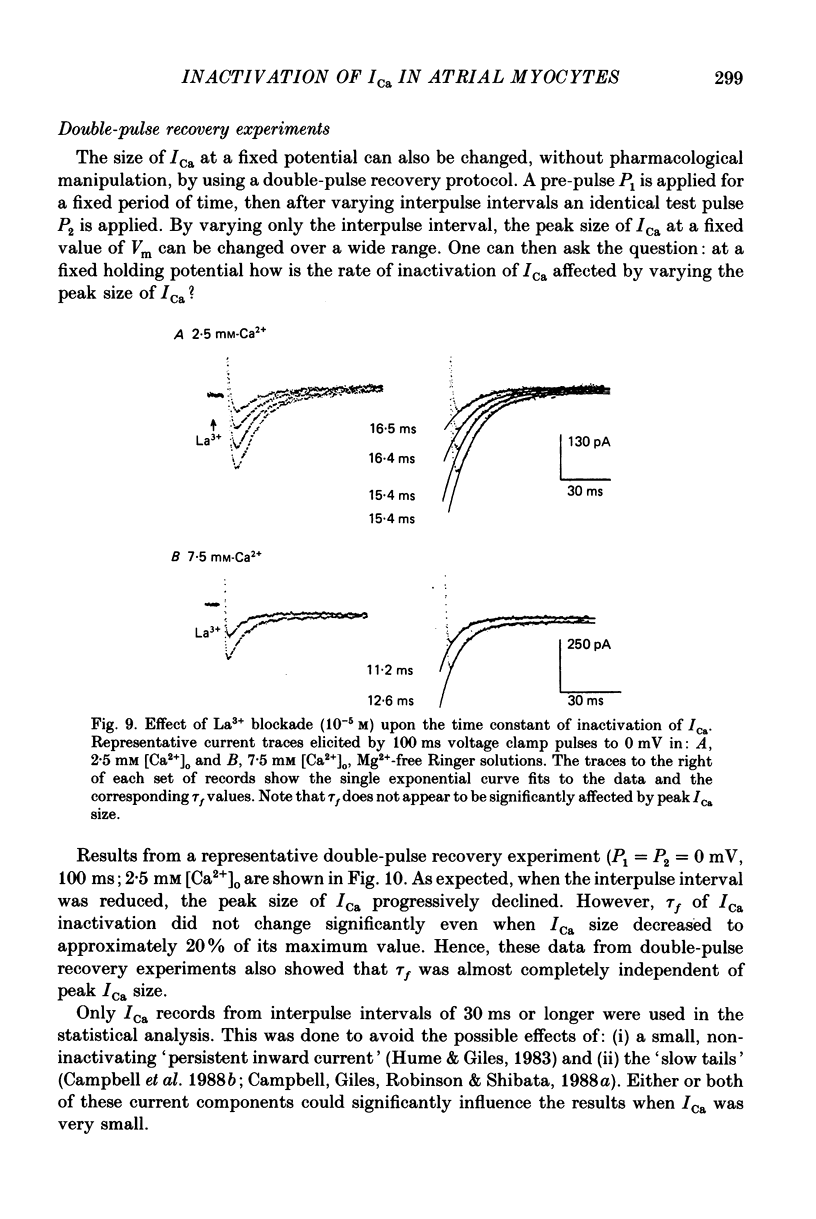
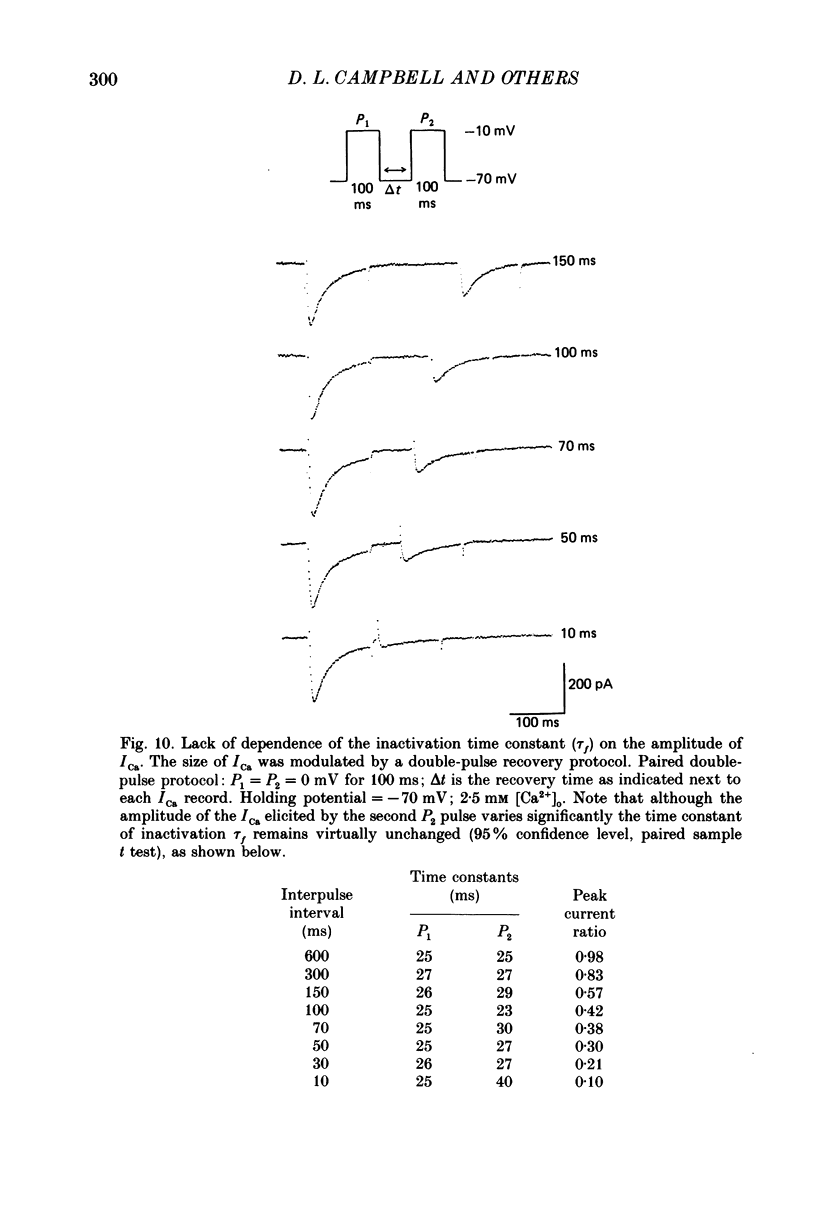
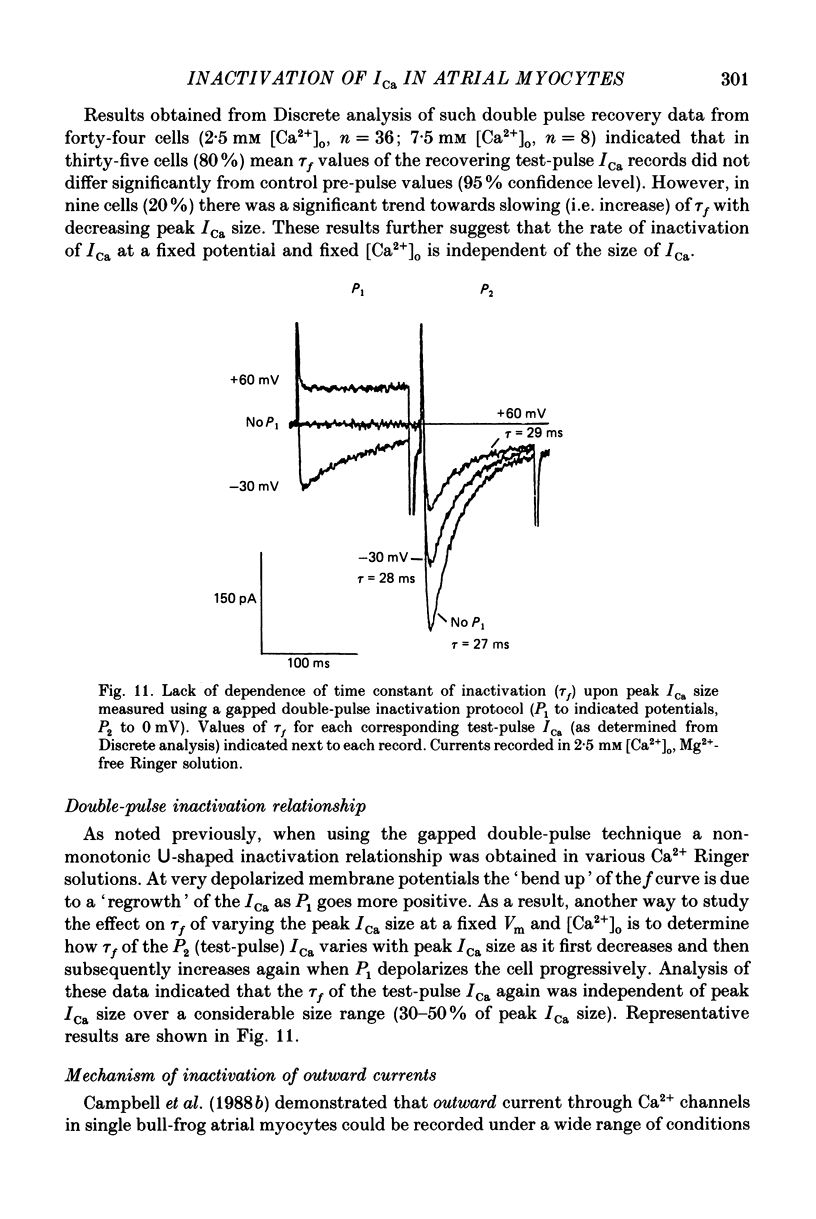
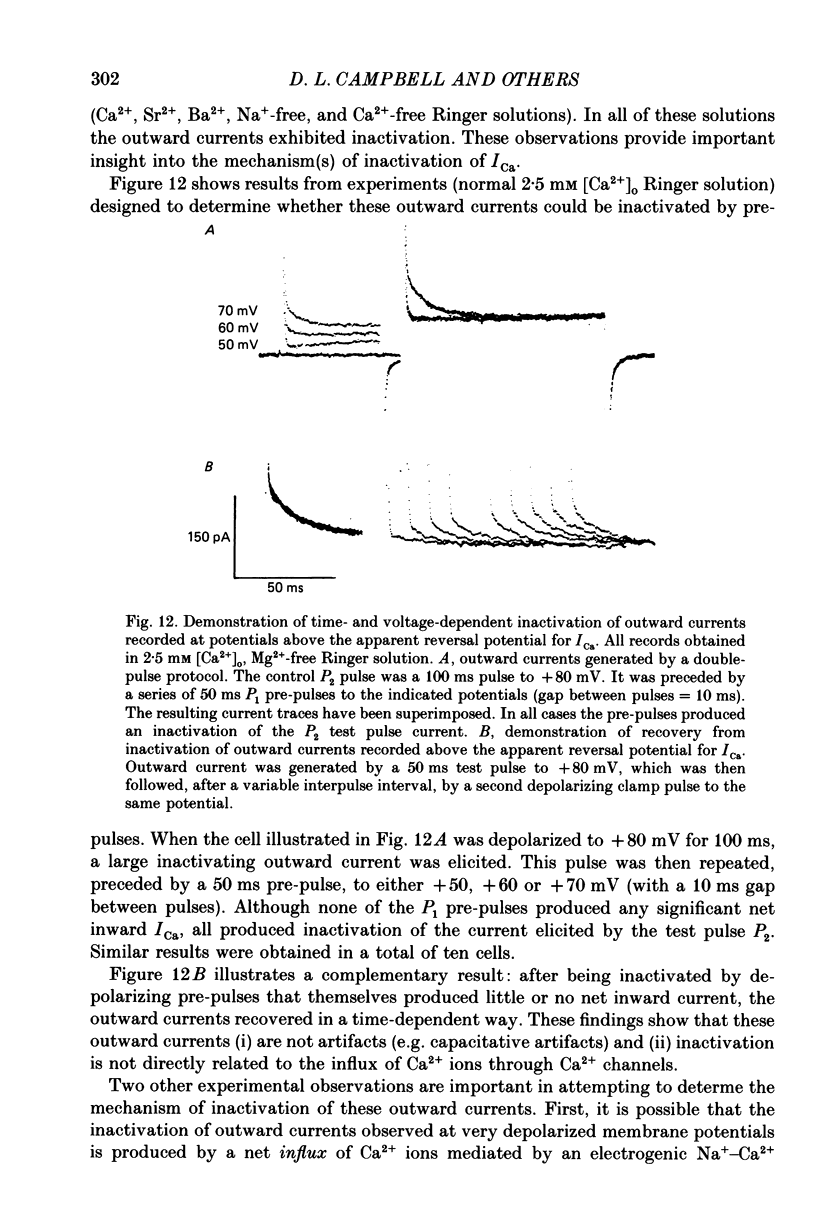
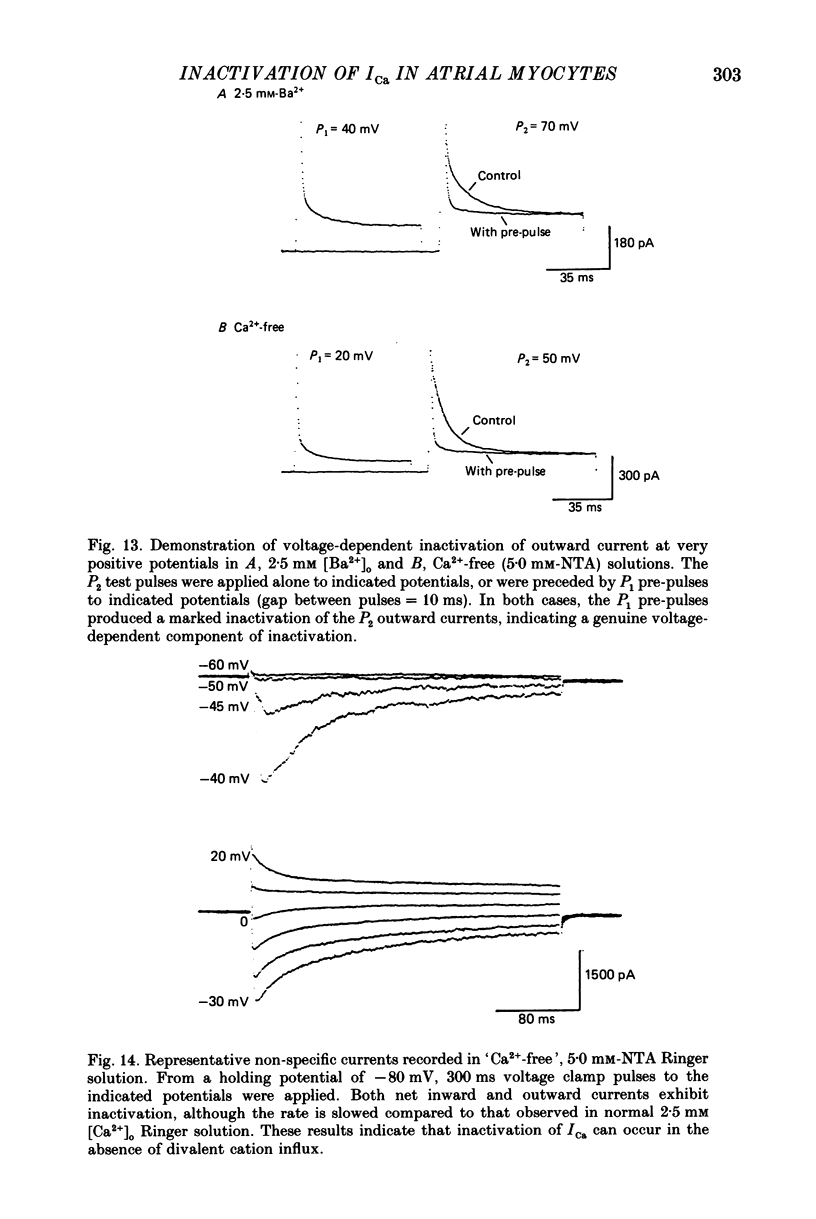
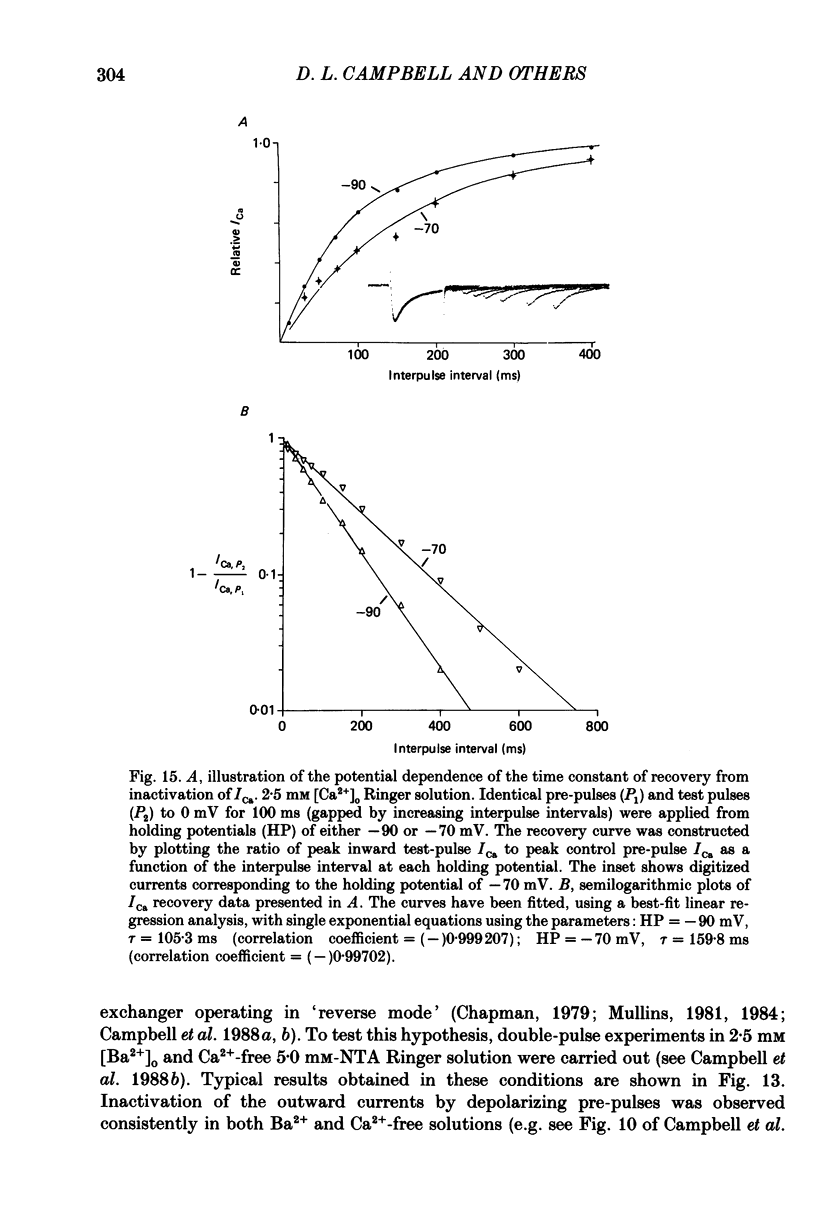
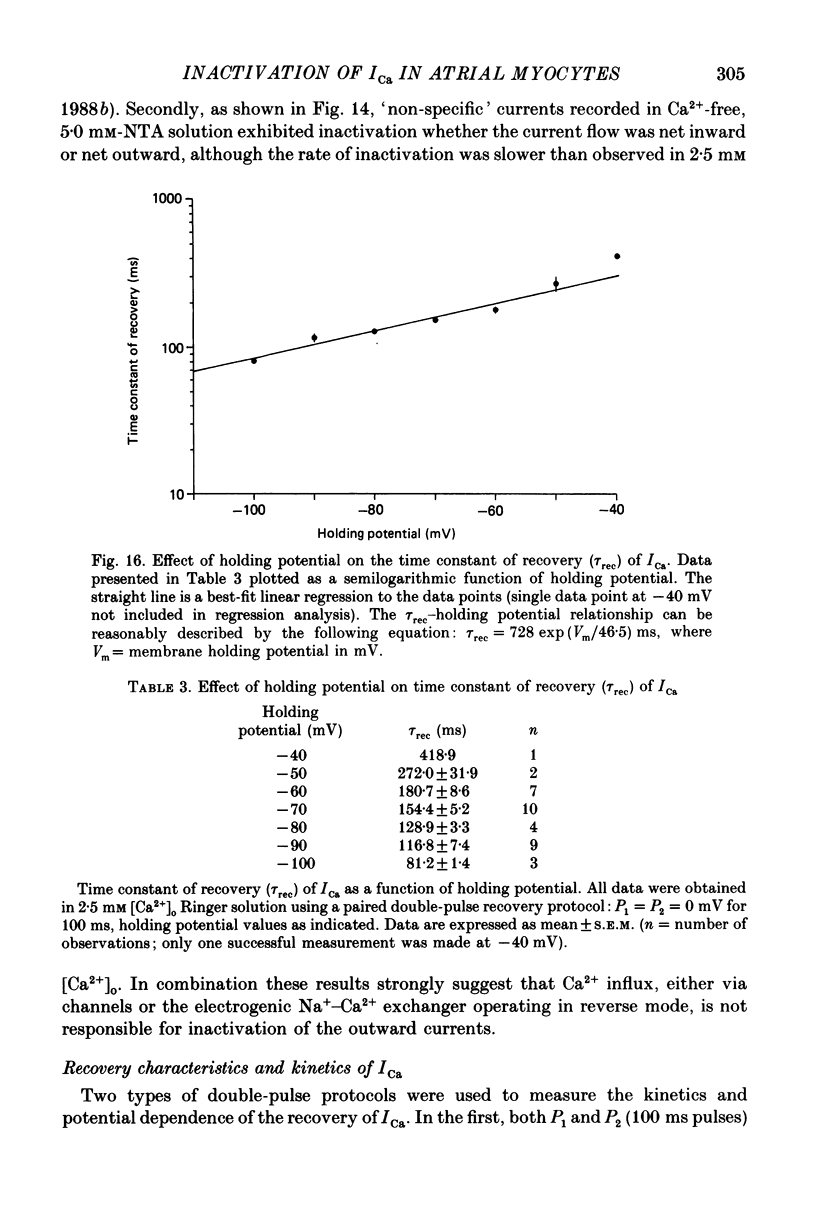
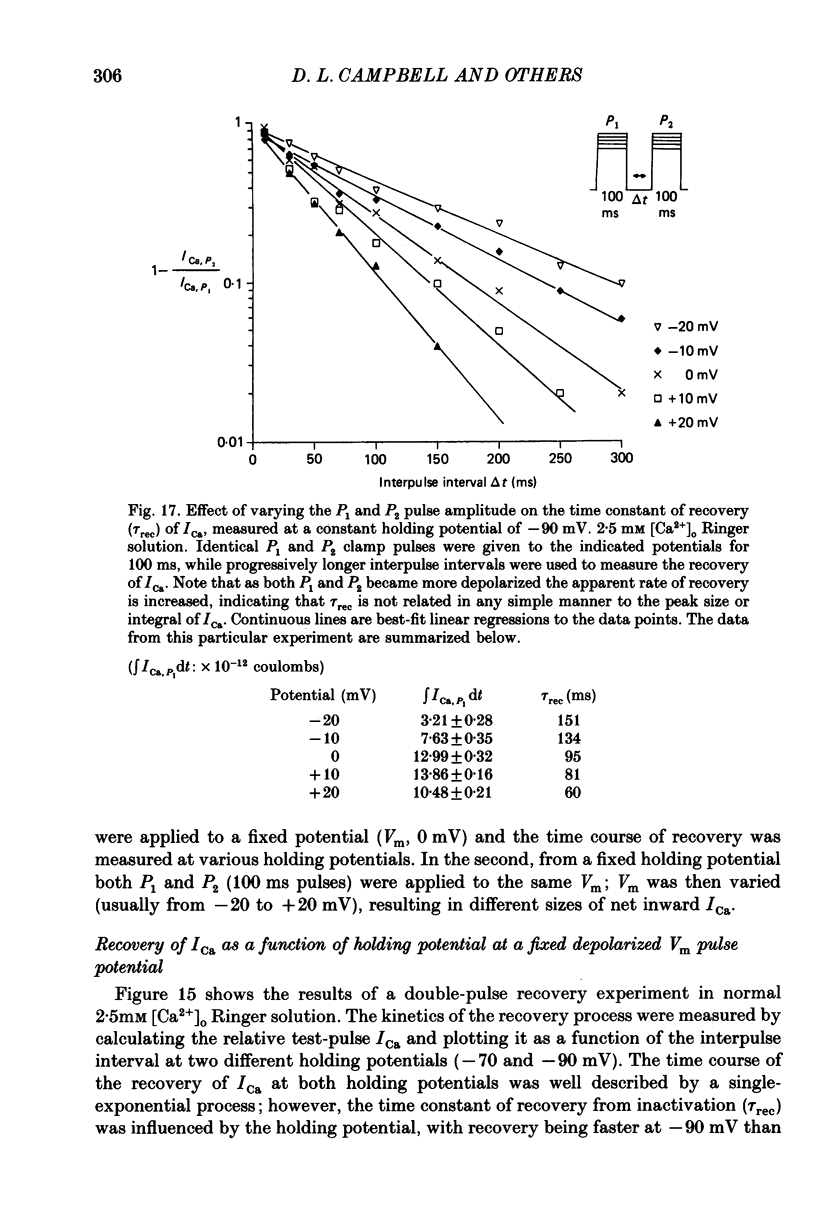
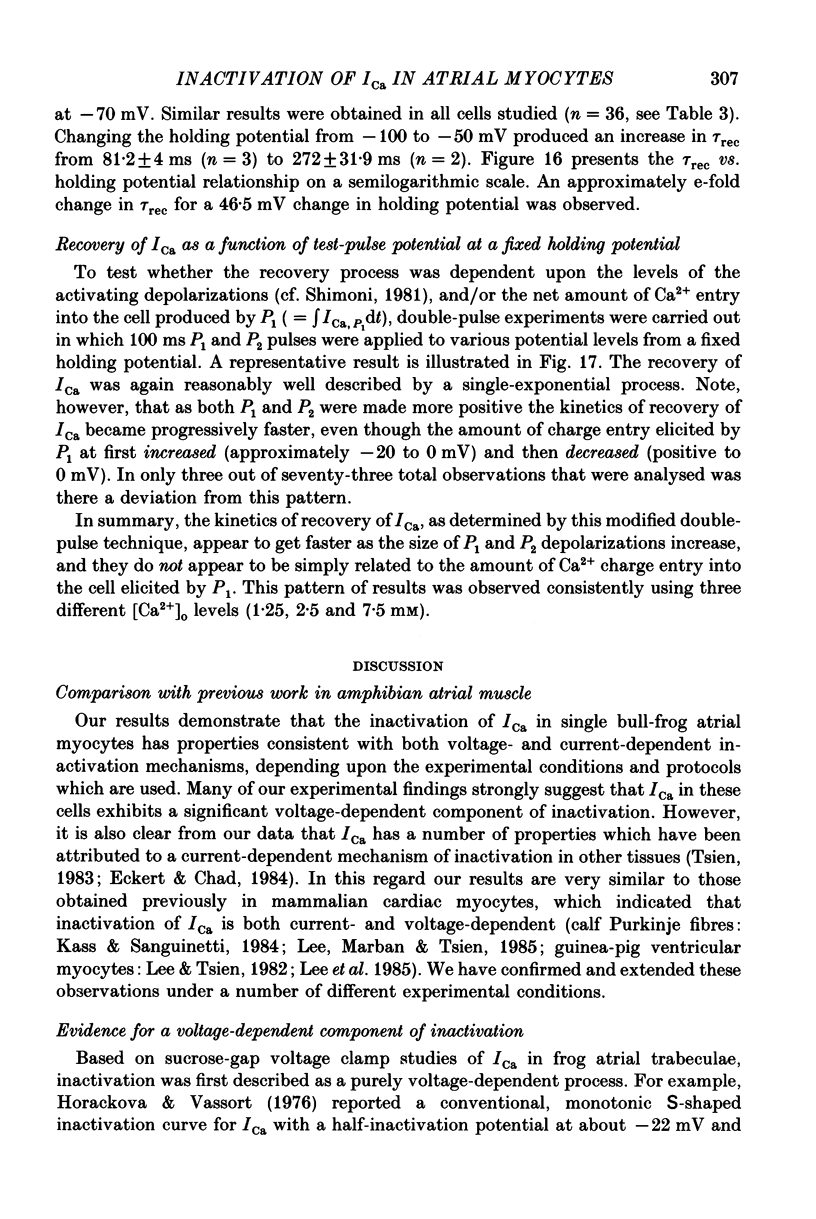
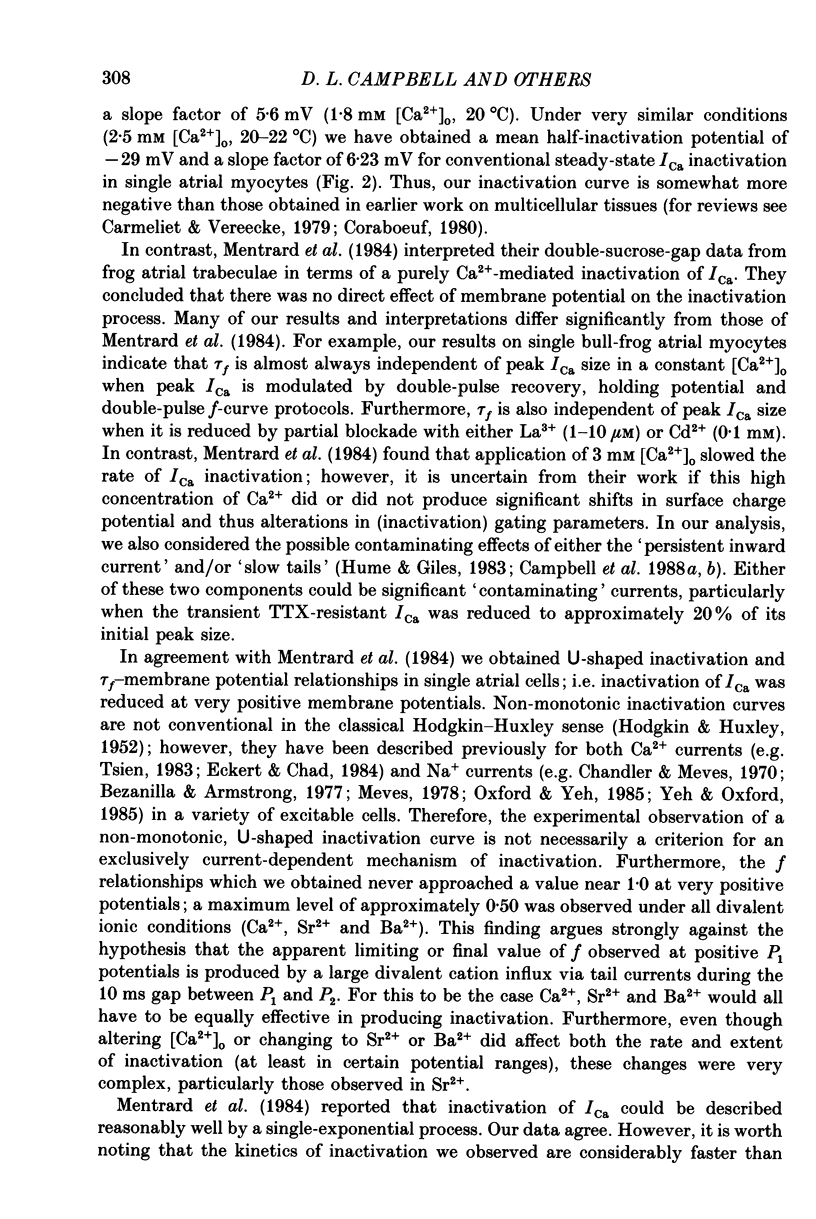
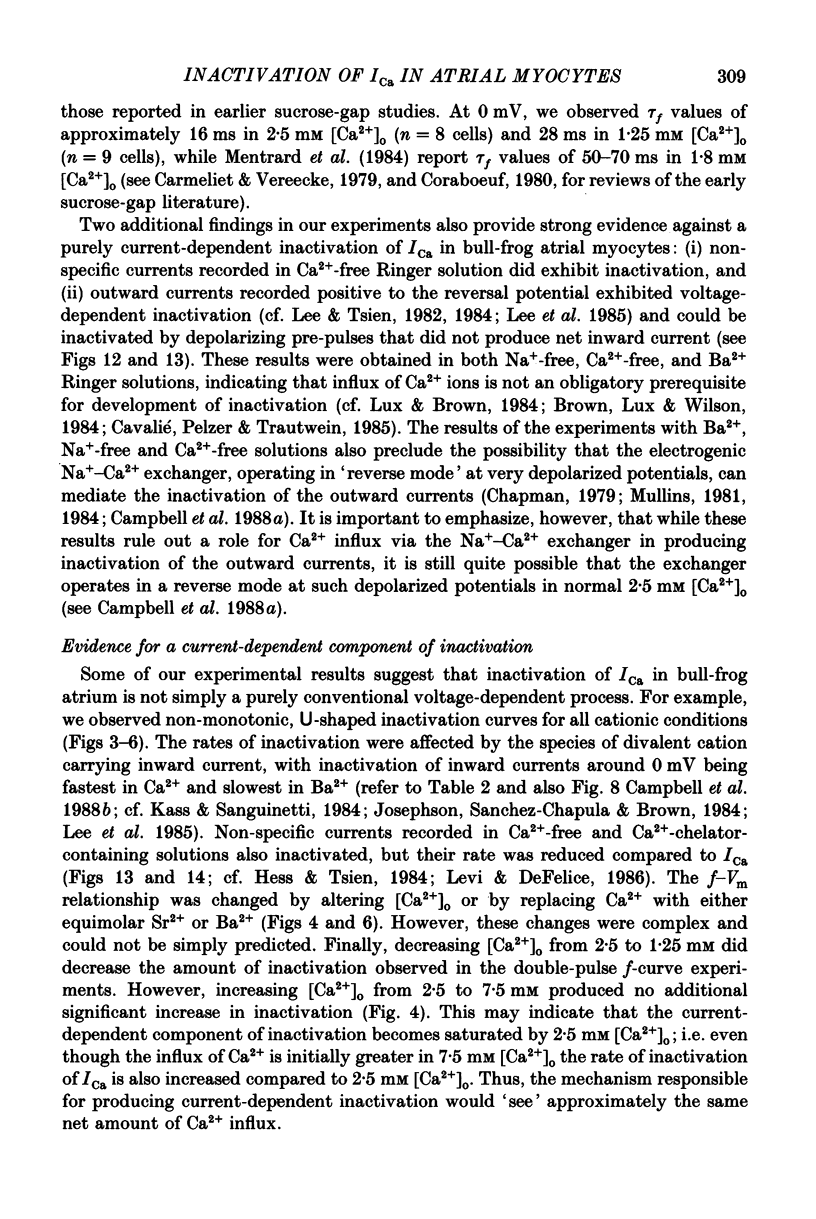
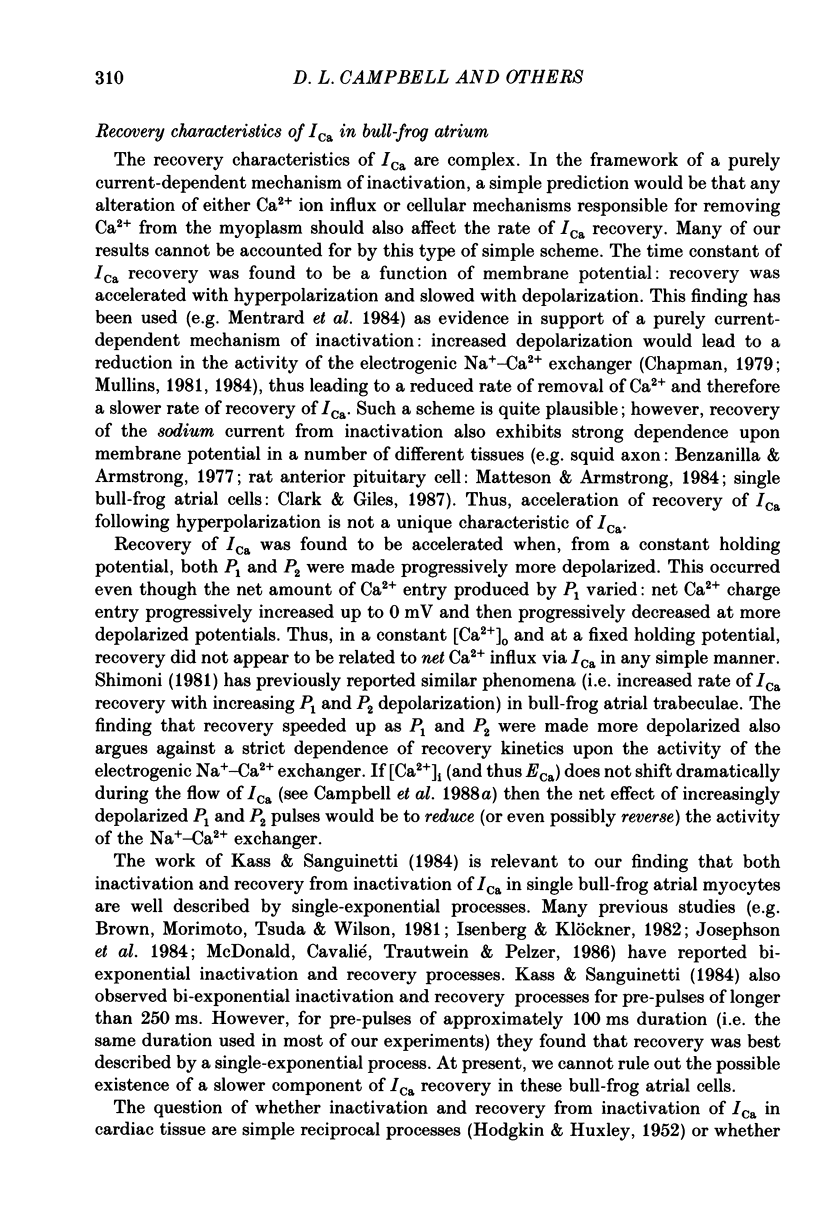
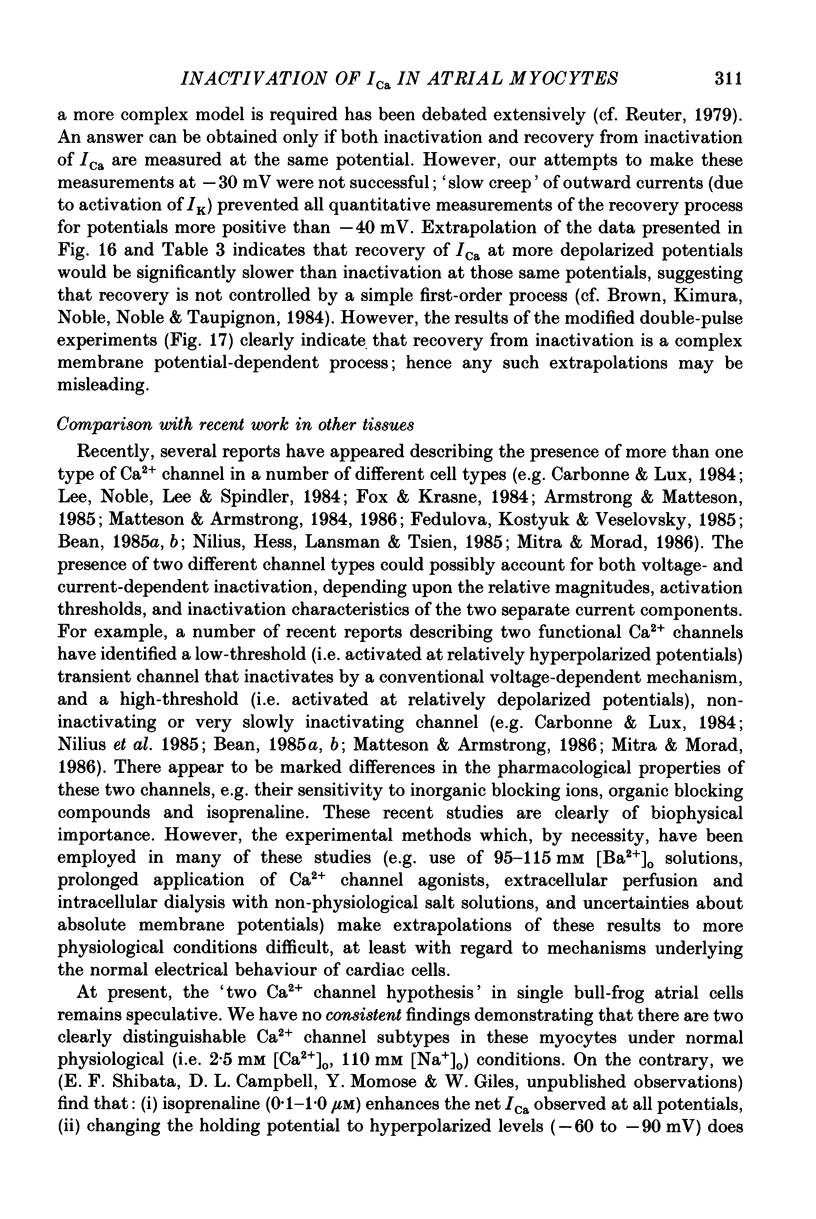
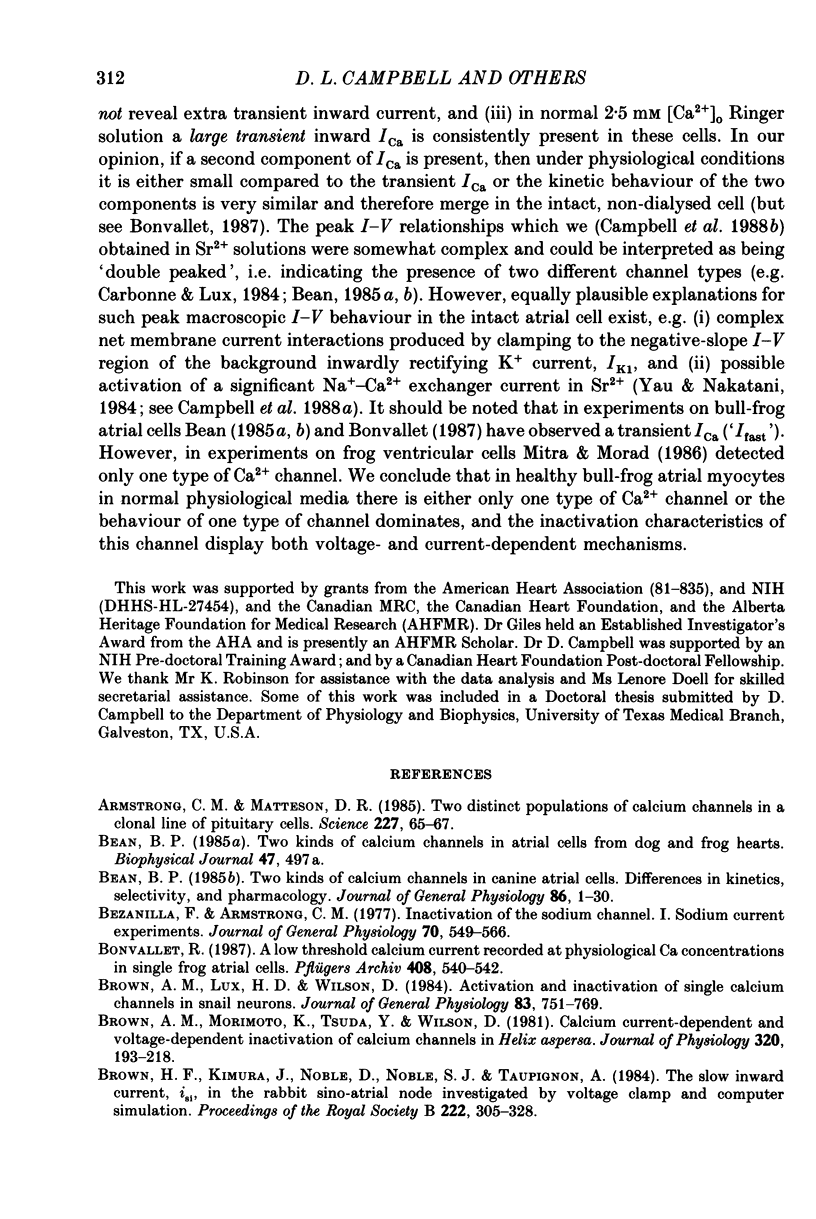
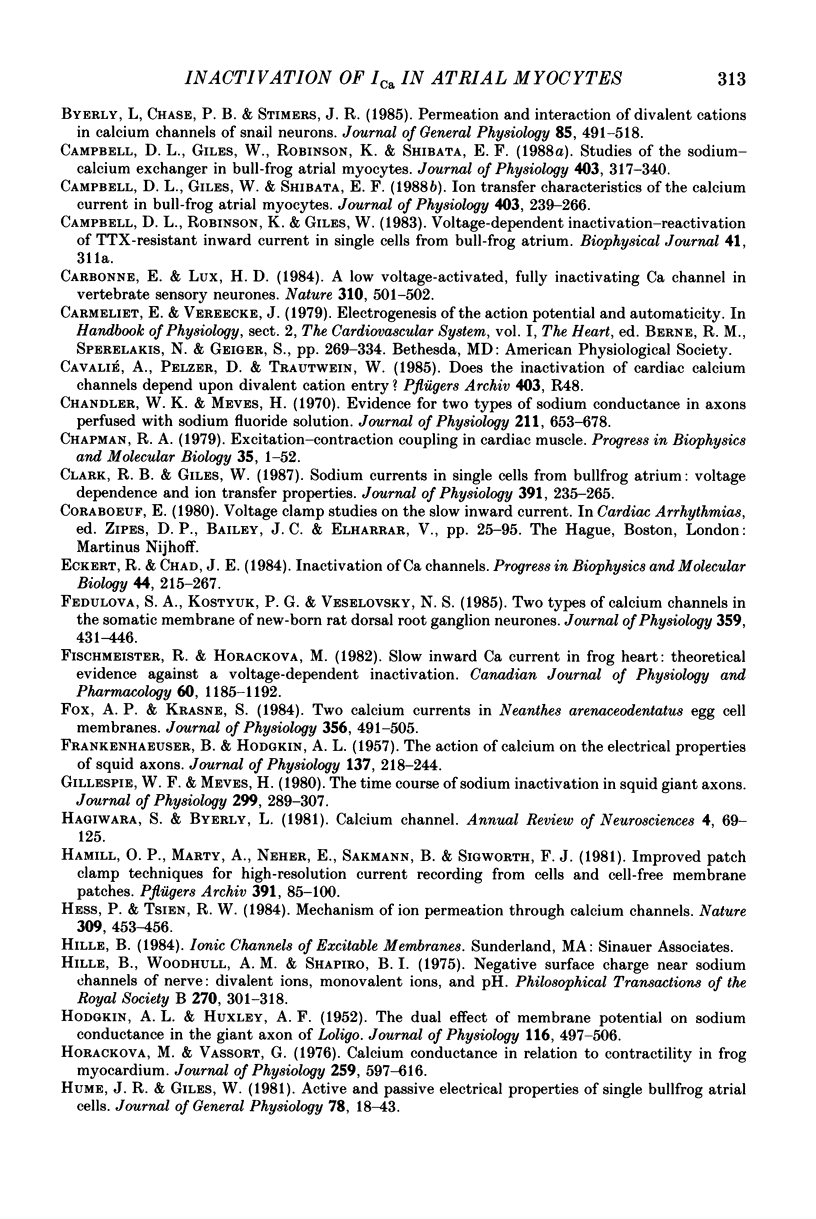
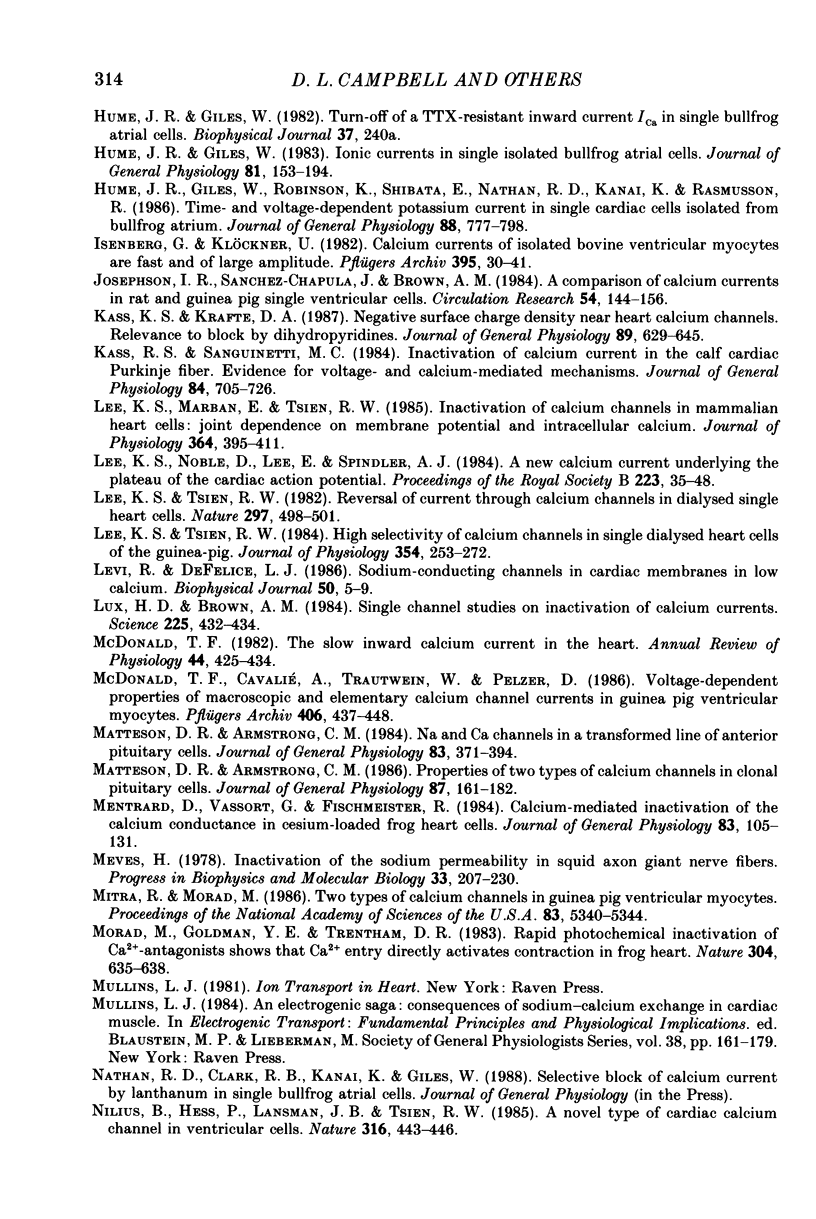
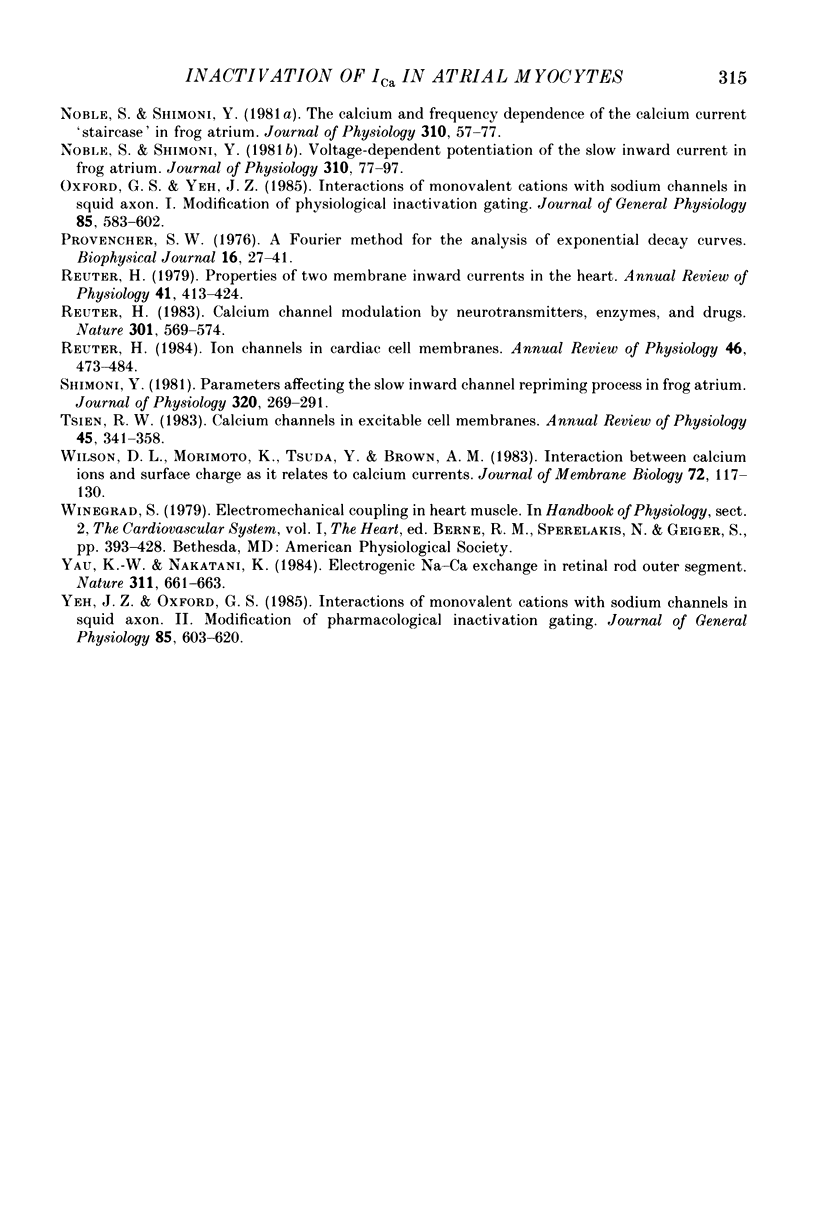
Selected References
These references are in PubMed. This may not be the complete list of references from this article.
- Armstrong C. M., Matteson D. R. Two distinct populations of calcium channels in a clonal line of pituitary cells. Science. 1985 Jan 4;227(4682):65–67. doi: 10.1126/science.2578071. [DOI] [PubMed] [Google Scholar]
- Bean B. P. Two kinds of calcium channels in canine atrial cells. Differences in kinetics, selectivity, and pharmacology. J Gen Physiol. 1985 Jul;86(1):1–30. doi: 10.1085/jgp.86.1.1. [DOI] [PMC free article] [PubMed] [Google Scholar]
- Bezanilla F., Armstrong C. M. Inactivation of the sodium channel. I. Sodium current experiments. J Gen Physiol. 1977 Nov;70(5):549–566. doi: 10.1085/jgp.70.5.549. [DOI] [PMC free article] [PubMed] [Google Scholar]
- Bonvallet R. A low threshold calcium current recorded at physiological Ca concentrations in single frog atrial cells. Pflugers Arch. 1987 May;408(5):540–542. doi: 10.1007/BF00585084. [DOI] [PubMed] [Google Scholar]
- Brown A. M., Lux H. D., Wilson D. L. Activation and inactivation of single calcium channels in snail neurons. J Gen Physiol. 1984 May;83(5):751–769. doi: 10.1085/jgp.83.5.751. [DOI] [PMC free article] [PubMed] [Google Scholar]
- Brown A. M., Morimoto K., Tsuda Y., wilson D. L. Calcium current-dependent and voltage-dependent inactivation of calcium channels in Helix aspersa. J Physiol. 1981 Nov;320:193–218. doi: 10.1113/jphysiol.1981.sp013944. [DOI] [PMC free article] [PubMed] [Google Scholar]
- Brown H. F., Kimura J., Noble D., Noble S. J., Taupignon A. The slow inward current, isi, in the rabbit sino-atrial node investigated by voltage clamp and computer simulation. Proc R Soc Lond B Biol Sci. 1984 Sep 22;222(1228):305–328. doi: 10.1098/rspb.1984.0066. [DOI] [PubMed] [Google Scholar]
- Byerly L., Chase P. B., Stimers J. R. Permeation and interaction of divalent cations in calcium channels of snail neurons. J Gen Physiol. 1985 Apr;85(4):491–518. doi: 10.1085/jgp.85.4.491. [DOI] [PMC free article] [PubMed] [Google Scholar]
- Campbell D. L., Giles W. R., Robinson K., Shibata E. F. Studies of the sodium-calcium exchanger in bull-frog atrial myocytes. J Physiol. 1988 Sep;403:317–340. doi: 10.1113/jphysiol.1988.sp017251. [DOI] [PMC free article] [PubMed] [Google Scholar]
- Campbell D. L., Giles W. R., Shibata E. F. Ion transfer characteristics of the calcium current in bull-frog atrial myocytes. J Physiol. 1988 Sep;403:239–266. doi: 10.1113/jphysiol.1988.sp017248. [DOI] [PMC free article] [PubMed] [Google Scholar]
- Carbone E., Lux H. D. A low voltage-activated, fully inactivating Ca channel in vertebrate sensory neurones. Nature. 1984 Aug 9;310(5977):501–502. doi: 10.1038/310501a0. [DOI] [PubMed] [Google Scholar]
- Chandler W. K., Meves H. Evidence for two types of sodium conductance in axons perfused with sodium fluoride solution. J Physiol. 1970 Dec;211(3):653–678. doi: 10.1113/jphysiol.1970.sp009298. [DOI] [PMC free article] [PubMed] [Google Scholar]
- Chapman R. A. Excitation-contraction coupling in cardiac muscle. Prog Biophys Mol Biol. 1979;35(1):1–52. doi: 10.1016/0079-6107(80)90002-4. [DOI] [PubMed] [Google Scholar]
- Clark R. B., Giles W. Sodium current in single cells from bullfrog atrium: voltage dependence and ion transfer properties. J Physiol. 1987 Oct;391:235–265. doi: 10.1113/jphysiol.1987.sp016736. [DOI] [PMC free article] [PubMed] [Google Scholar]
- Eckert R., Chad J. E. Inactivation of Ca channels. Prog Biophys Mol Biol. 1984;44(3):215–267. doi: 10.1016/0079-6107(84)90009-9. [DOI] [PubMed] [Google Scholar]
- FRANKENHAEUSER B., HODGKIN A. L. The action of calcium on the electrical properties of squid axons. J Physiol. 1957 Jul 11;137(2):218–244. doi: 10.1113/jphysiol.1957.sp005808. [DOI] [PMC free article] [PubMed] [Google Scholar]
- Fedulova S. A., Kostyuk P. G., Veselovsky N. S. Two types of calcium channels in the somatic membrane of new-born rat dorsal root ganglion neurones. J Physiol. 1985 Feb;359:431–446. doi: 10.1113/jphysiol.1985.sp015594. [DOI] [PMC free article] [PubMed] [Google Scholar]
- Fischmeister R., Horackova M. Slow inward Ca current in frog heart: theoretical evidence against a voltage-dependent inactivation. Can J Physiol Pharmacol. 1982 Sep;60(9):1185–1192. doi: 10.1139/y82-172. [DOI] [PubMed] [Google Scholar]
- Fox A. P., Krasne S. Two calcium currents in Neanthes arenaceodentatus egg cell membranes. J Physiol. 1984 Nov;356:491–505. doi: 10.1113/jphysiol.1984.sp015479. [DOI] [PMC free article] [PubMed] [Google Scholar]
- Gillespie J. I., Meves H. The time course of sodium inactivation in squid giant axons. J Physiol. 1980 Feb;299:289–307. doi: 10.1113/jphysiol.1980.sp013125. [DOI] [PMC free article] [PubMed] [Google Scholar]
- HODGKIN A. L., HUXLEY A. F. The dual effect of membrane potential on sodium conductance in the giant axon of Loligo. J Physiol. 1952 Apr;116(4):497–506. doi: 10.1113/jphysiol.1952.sp004719. [DOI] [PMC free article] [PubMed] [Google Scholar]
- Hagiwara S., Byerly L. Calcium channel. Annu Rev Neurosci. 1981;4:69–125. doi: 10.1146/annurev.ne.04.030181.000441. [DOI] [PubMed] [Google Scholar]
- Hamill O. P., Marty A., Neher E., Sakmann B., Sigworth F. J. Improved patch-clamp techniques for high-resolution current recording from cells and cell-free membrane patches. Pflugers Arch. 1981 Aug;391(2):85–100. doi: 10.1007/BF00656997. [DOI] [PubMed] [Google Scholar]
- Hess P., Tsien R. W. Mechanism of ion permeation through calcium channels. 1984 May 31-Jun 6Nature. 309(5967):453–456. doi: 10.1038/309453a0. [DOI] [PubMed] [Google Scholar]
- Hille B., Woodhull A. M., Shapiro B. I. Negative surface charge near sodium channels of nerve: divalent ions, monovalent ions, and pH. Philos Trans R Soc Lond B Biol Sci. 1975 Jun 10;270(908):301–318. doi: 10.1098/rstb.1975.0011. [DOI] [PubMed] [Google Scholar]
- Horackova M., Vassort G. Calcium conductance in relation to contractility in frog myocardium. J Physiol. 1976 Aug;259(3):597–616. doi: 10.1113/jphysiol.1976.sp011485. [DOI] [PMC free article] [PubMed] [Google Scholar]
- Hume J. R., Giles W. Ionic currents in single isolated bullfrog atrial cells. J Gen Physiol. 1983 Feb;81(2):153–194. doi: 10.1085/jgp.81.2.153. [DOI] [PMC free article] [PubMed] [Google Scholar]
- Hume J. R., Giles W., Robinson K., Shibata E. F., Nathan R. D., Kanai K., Rasmusson R. A time- and voltage-dependent K+ current in single cardiac cells from bullfrog atrium. J Gen Physiol. 1986 Dec;88(6):777–798. doi: 10.1085/jgp.88.6.777. [DOI] [PMC free article] [PubMed] [Google Scholar]
- Isenberg G., Klöckner U. Calcium currents of isolated bovine ventricular myocytes are fast and of large amplitude. Pflugers Arch. 1982 Oct;395(1):30–41. doi: 10.1007/BF00584965. [DOI] [PubMed] [Google Scholar]
- Josephson I. R., Sanchez-Chapula J., Brown A. M. A comparison of calcium currents in rat and guinea pig single ventricular cells. Circ Res. 1984 Feb;54(2):144–156. doi: 10.1161/01.res.54.2.144. [DOI] [PubMed] [Google Scholar]
- Kass R. S., Krafte D. S. Negative surface charge density near heart calcium channels. Relevance to block by dihydropyridines. J Gen Physiol. 1987 Apr;89(4):629–644. doi: 10.1085/jgp.89.4.629. [DOI] [PMC free article] [PubMed] [Google Scholar]
- Kass R. S., Sanguinetti M. C. Inactivation of calcium channel current in the calf cardiac Purkinje fiber. Evidence for voltage- and calcium-mediated mechanisms. J Gen Physiol. 1984 Nov;84(5):705–726. doi: 10.1085/jgp.84.5.705. [DOI] [PMC free article] [PubMed] [Google Scholar]
- Lee K. S., Marban E., Tsien R. W. Inactivation of calcium channels in mammalian heart cells: joint dependence on membrane potential and intracellular calcium. J Physiol. 1985 Jul;364:395–411. doi: 10.1113/jphysiol.1985.sp015752. [DOI] [PMC free article] [PubMed] [Google Scholar]
- Lee K. S., Noble D., Lee E., Spindler A. J. A new calcium current underlying the plateau of the cardiac action potential. Proc R Soc Lond B Biol Sci. 1984 Nov 22;223(1230):35–48. doi: 10.1098/rspb.1984.0081. [DOI] [PubMed] [Google Scholar]
- Lee K. S., Tsien R. W. High selectivity of calcium channels in single dialysed heart cells of the guinea-pig. J Physiol. 1984 Sep;354:253–272. doi: 10.1113/jphysiol.1984.sp015374. [DOI] [PMC free article] [PubMed] [Google Scholar]
- Lee K. S., Tsien R. W. Reversal of current through calcium channels in dialysed single heart cells. Nature. 1982 Jun 10;297(5866):498–501. doi: 10.1038/297498a0. [DOI] [PubMed] [Google Scholar]
- Levi R., DeFelice L. J. Sodium-conducting channels in cardiac membranes in low calcium. Biophys J. 1986 Jul;50(1):5–9. doi: 10.1016/S0006-3495(86)83433-6. [DOI] [PMC free article] [PubMed] [Google Scholar]
- Lux H. D., Brown A. M. Single channel studies on inactivation of calcium currents. Science. 1984 Jul 27;225(4660):432–434. doi: 10.1126/science.6330896. [DOI] [PubMed] [Google Scholar]
- Matteson D. R., Armstrong C. M. Na and Ca channels in a transformed line of anterior pituitary cells. J Gen Physiol. 1984 Mar;83(3):371–394. doi: 10.1085/jgp.83.3.371. [DOI] [PMC free article] [PubMed] [Google Scholar]
- Matteson D. R., Armstrong C. M. Properties of two types of calcium channels in clonal pituitary cells. J Gen Physiol. 1986 Jan;87(1):161–182. doi: 10.1085/jgp.87.1.161. [DOI] [PMC free article] [PubMed] [Google Scholar]
- McDonald T. F., Cavalié A., Trautwein W., Pelzer D. Voltage-dependent properties of macroscopic and elementary calcium channel currents in guinea pig ventricular myocytes. Pflugers Arch. 1986 May;406(5):437–448. doi: 10.1007/BF00583365. [DOI] [PubMed] [Google Scholar]
- McDonald T. F. The slow inward calcium current in the heart. Annu Rev Physiol. 1982;44:425–434. doi: 10.1146/annurev.ph.44.030182.002233. [DOI] [PubMed] [Google Scholar]
- Mentrard D., Vassort G., Fischmeister R. Calcium-mediated inactivation of the calcium conductance in cesium-loaded frog heart cells. J Gen Physiol. 1984 Jan;83(1):105–131. doi: 10.1085/jgp.83.1.105. [DOI] [PMC free article] [PubMed] [Google Scholar]
- Meves H. Inactivation of the sodium permeability in squid giant nerve fibres. Prog Biophys Mol Biol. 1978;33(2):207–230. doi: 10.1016/0079-6107(79)90029-4. [DOI] [PubMed] [Google Scholar]
- Mitra R., Morad M. Two types of calcium channels in guinea pig ventricular myocytes. Proc Natl Acad Sci U S A. 1986 Jul;83(14):5340–5344. doi: 10.1073/pnas.83.14.5340. [DOI] [PMC free article] [PubMed] [Google Scholar]
- Morad M., Goldman Y. E., Trentham D. R. Rapid photochemical inactivation of Ca2+-antagonists shows that Ca2+ entry directly activates contraction in frog heart. Nature. 1983 Aug 18;304(5927):635–638. doi: 10.1038/304635a0. [DOI] [PubMed] [Google Scholar]
- Mullins L. J. An electrogenic saga: consequences of sodium-calcium exchange in cardiac muscle. Soc Gen Physiol Ser. 1984;38:161–179. [PubMed] [Google Scholar]
- Nilius B., Hess P., Lansman J. B., Tsien R. W. A novel type of cardiac calcium channel in ventricular cells. Nature. 1985 Aug 1;316(6027):443–446. doi: 10.1038/316443a0. [DOI] [PubMed] [Google Scholar]
- Oxford G. S., Yeh J. Z. Interactions of monovalent cations with sodium channels in squid axon. I. Modification of physiological inactivation gating. J Gen Physiol. 1985 Apr;85(4):583–602. doi: 10.1085/jgp.85.4.583. [DOI] [PMC free article] [PubMed] [Google Scholar]
- Provencher S. W. A Fourier method for the analysis of exponential decay curves. Biophys J. 1976 Jan;16(1):27–41. doi: 10.1016/S0006-3495(76)85660-3. [DOI] [PMC free article] [PubMed] [Google Scholar]
- Reuter H. Calcium channel modulation by neurotransmitters, enzymes and drugs. Nature. 1983 Feb 17;301(5901):569–574. doi: 10.1038/301569a0. [DOI] [PubMed] [Google Scholar]
- Reuter H. Ion channels in cardiac cell membranes. Annu Rev Physiol. 1984;46:473–484. doi: 10.1146/annurev.ph.46.030184.002353. [DOI] [PubMed] [Google Scholar]
- Reuter H. Properties of two inward membrane currents in the heart. Annu Rev Physiol. 1979;41:413–424. doi: 10.1146/annurev.ph.41.030179.002213. [DOI] [PubMed] [Google Scholar]
- Shimoni Y. Parameters affecting the slow inward channel repriming process in frog atrium. J Physiol. 1981 Nov;320:269–291. doi: 10.1113/jphysiol.1981.sp013948. [DOI] [PMC free article] [PubMed] [Google Scholar]
- Tsien R. W. Calcium channels in excitable cell membranes. Annu Rev Physiol. 1983;45:341–358. doi: 10.1146/annurev.ph.45.030183.002013. [DOI] [PubMed] [Google Scholar]
- Wilson D. L., Morimoto K., Tsuda Y., Brown A. M. Interaction between calcium ions and surface charge as it relates to calcium currents. J Membr Biol. 1983;72(1-2):117–130. doi: 10.1007/BF01870319. [DOI] [PubMed] [Google Scholar]
- Yau K. W., Nakatani K. Electrogenic Na-Ca exchange in retinal rod outer segment. Nature. 1984 Oct 18;311(5987):661–663. doi: 10.1038/311661a0. [DOI] [PubMed] [Google Scholar]
- Yeh J. Z., Oxford G. S. Interactions of monovalent cations with sodium channels in squid axon. II. Modification of pharmacological inactivation gating. J Gen Physiol. 1985 Apr;85(4):603–620. doi: 10.1085/jgp.85.4.603. [DOI] [PMC free article] [PubMed] [Google Scholar]