Abstract
1. Experimental measurements and computer simulations have been used in attempts to identify an exchanger current (Iex) generated by an electrogenic Na+-Ca2+ exchanger in single cells from bull-frog atrium. 2. Voltage clamp measurements of an inward 'slow tail' current observed upon repolarization after depolarizing clamp pulses that elicit net inward Ca2+ currents (ICa) (see Campbell, Giles & Shibata, 1988c), show that these slow tails have a cationic dependence different from ICa. Slow tails are large and prominent in normal [Na+]o solutions containing either Ca2+ or Sr2+, but they are markedly reduced or absent in Ba2+, Ca2+-free, and Na+-free solutions. 3. Kinetic measurements on the slow tails show that they are not generated by deactivation of ICa, and suggest that they may be due to activation of Iex at negative potentials (-70 to -100 mV). 4. Computer simulations of the influx, buffering, and extrusion of Ca2+ provide further indirect evidence that the slow tails correspond to Iex. In addition, these calculations give insights into one plausible mechanism of Ca2+ homeostasis in frog atrium. When the Na+-Ca2+ exchanger formalism of Mullins (1979, 1981), as modified by DiFrancesco & Nobel (1985), is combined with equations for intracellular Ca2+ buffering by myoplasmic proteins (cf. Robertson, Johnson & Potter, 1981), slow inward tails are produced which are qualitatively similar to those recorded experimentally. 5. Comparisons of the size and time course of ICa with those of Iex suggest that Iex does not generate a physiologically significant current (or membrane potential change) during the plateau of the action potential. However, at potentials near the resting potential the inward current due to Iex may be significant. 6. Our theoretical results suggest that in the intact single atrial cell myoplasmic Ca2+-binding proteins (e.g. calmodulin and troponin) could be physiologically important modulators of the amplitude, polarity and kinetics of Iex. Hence, the specificity, capacity and kinetics of intracellular Ca2+ binding are essential components of any quantitative treatment of Iex in excitable tissue.
Full text
PDF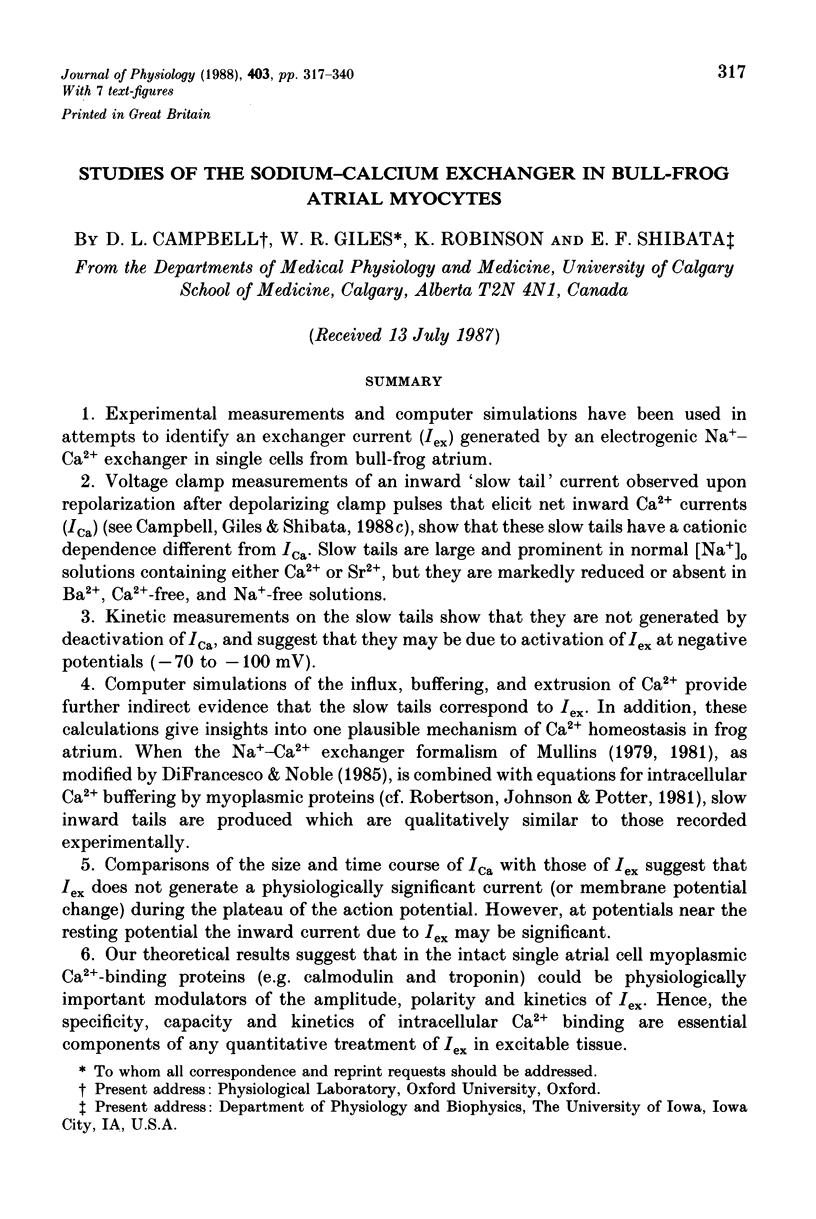
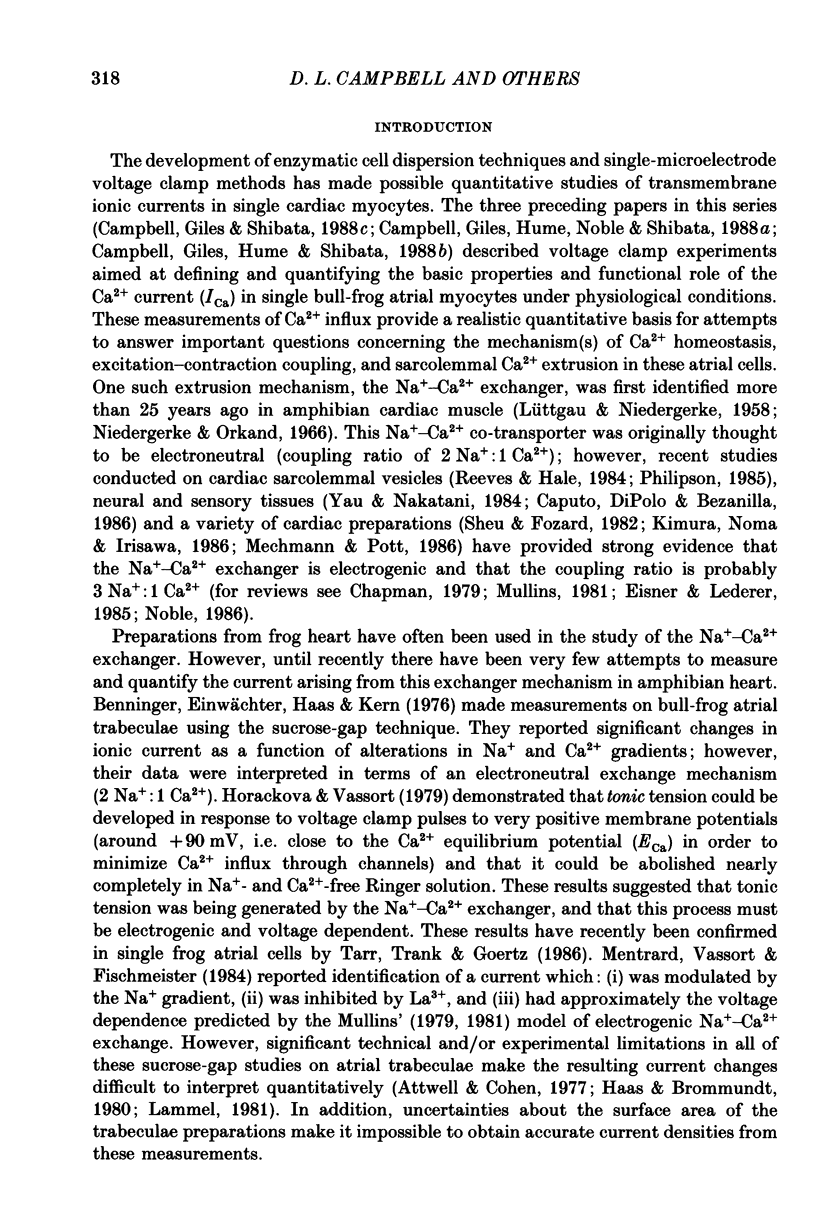
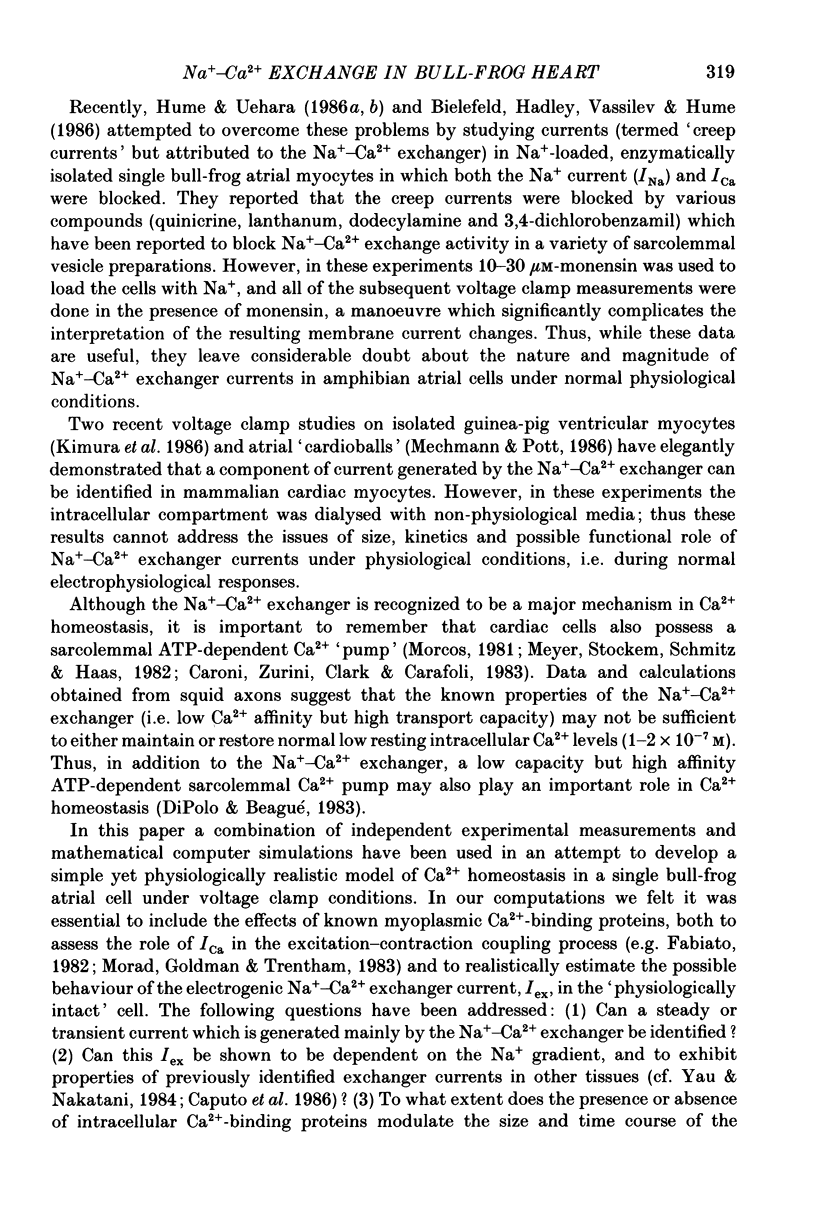
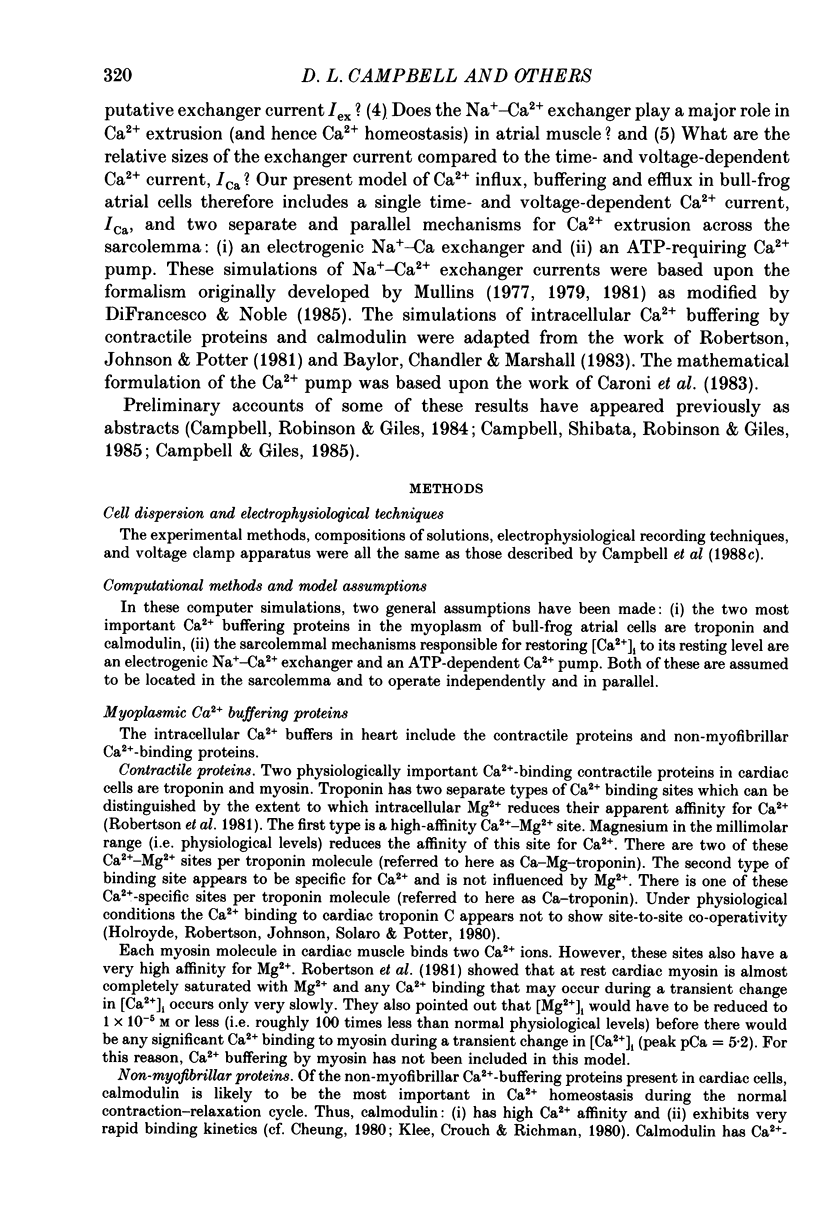
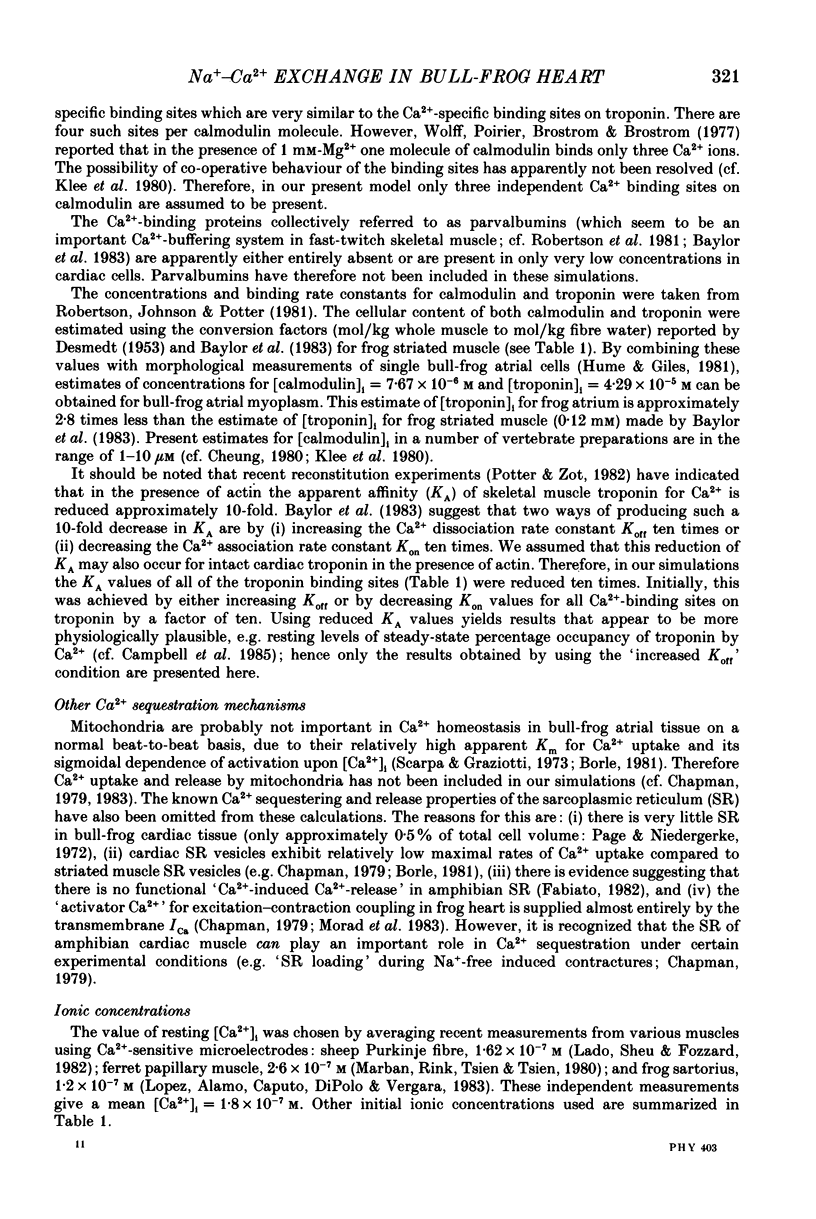
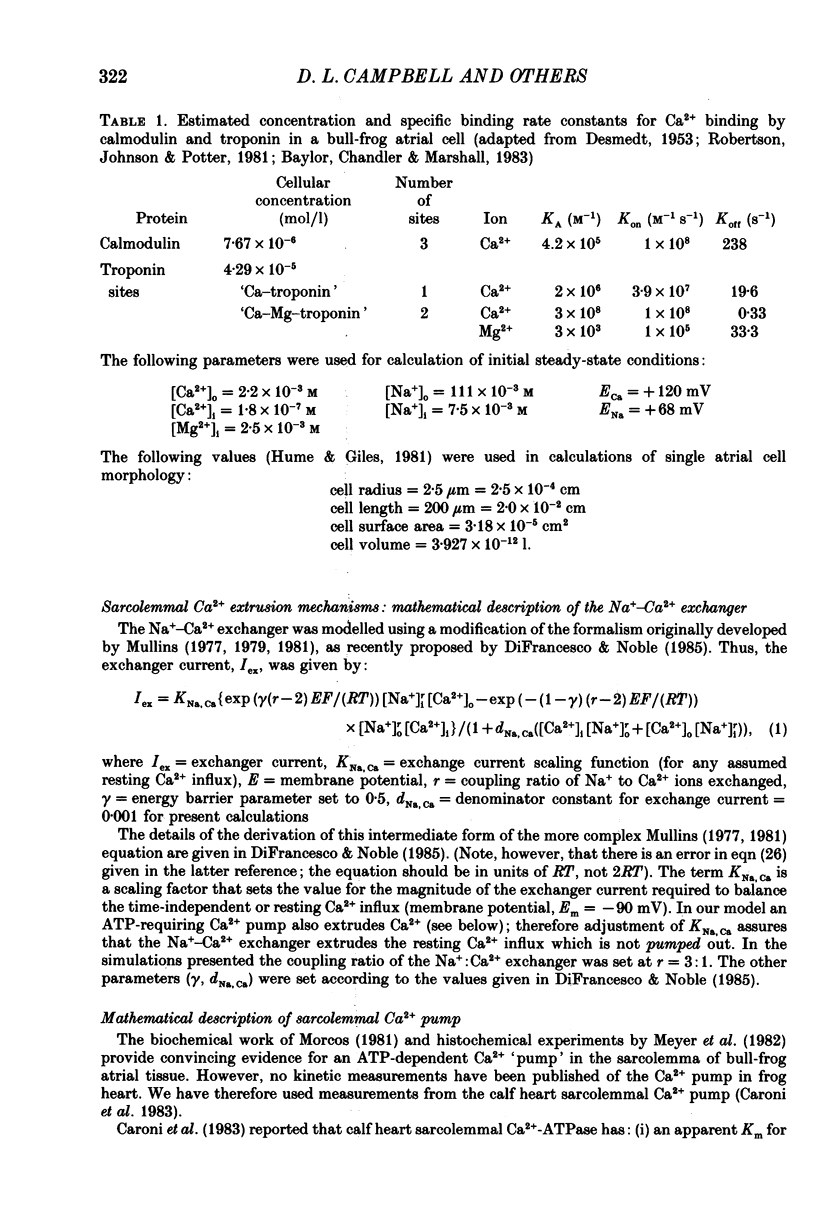
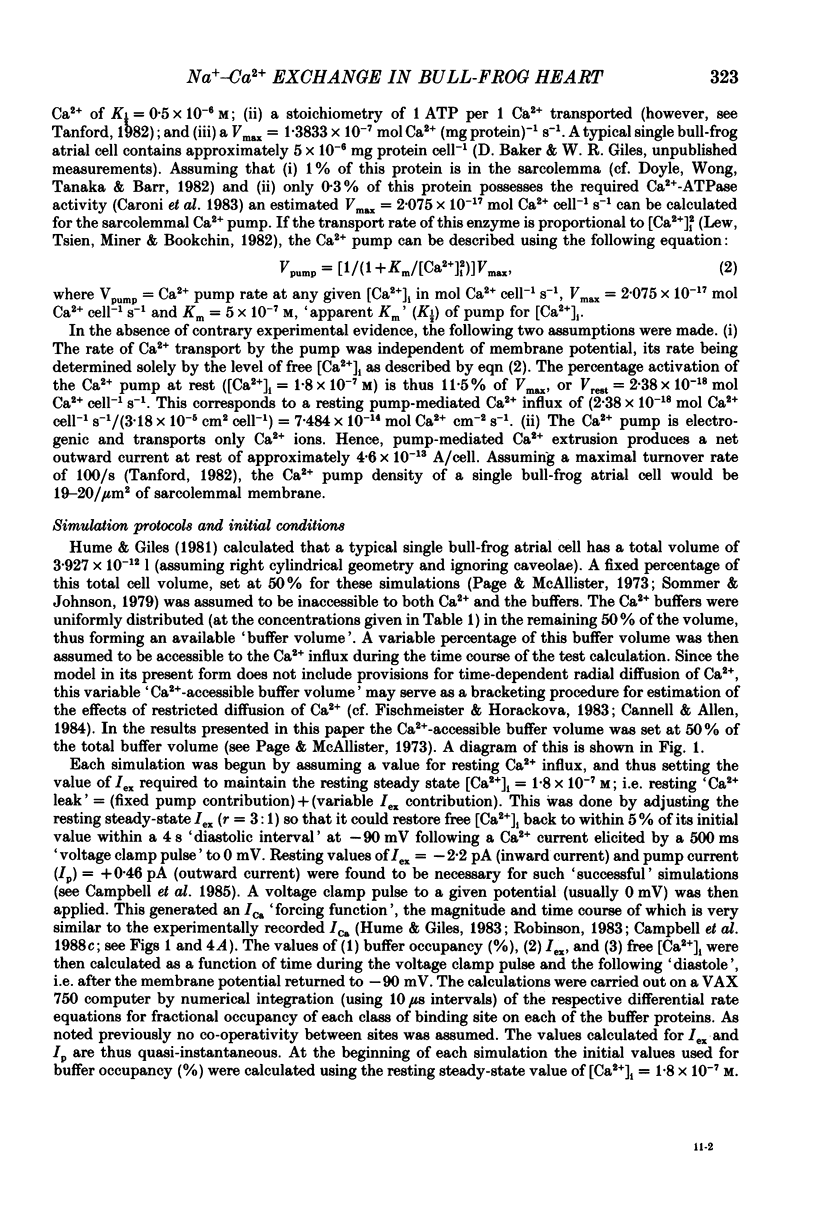
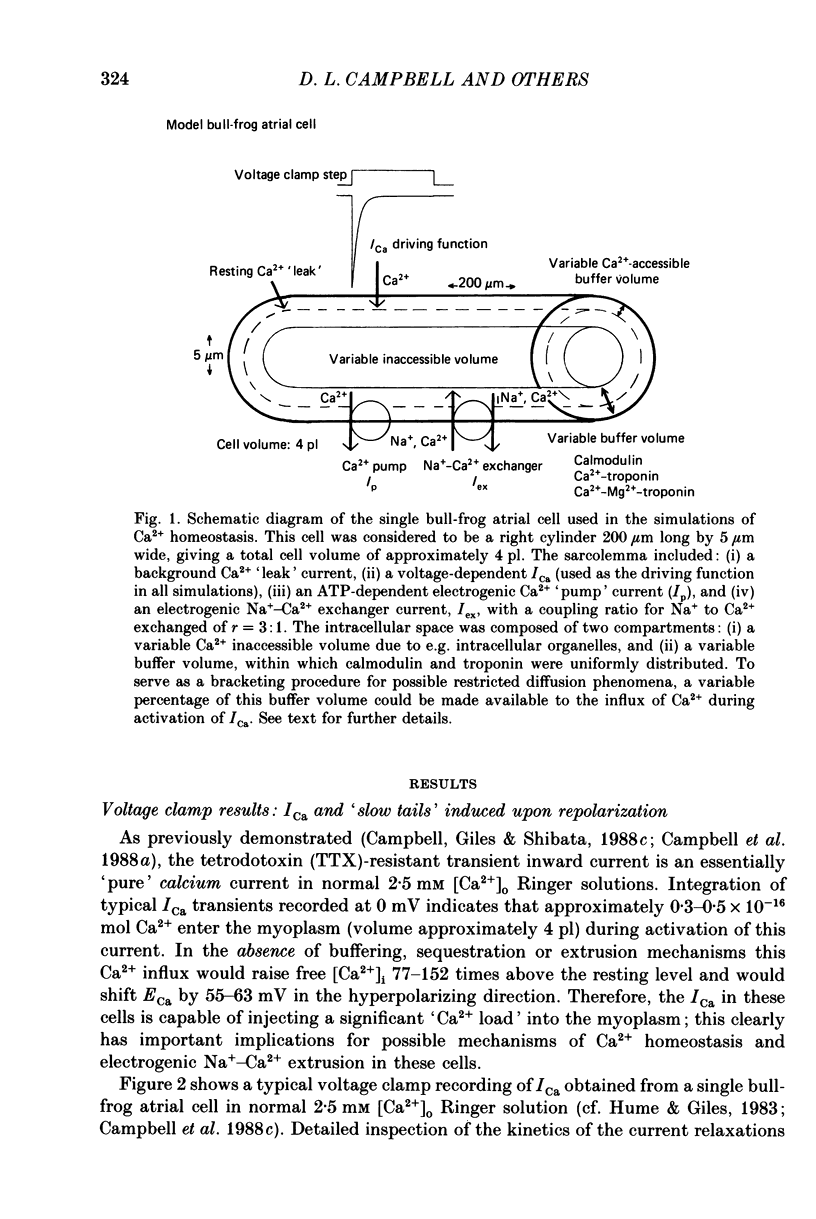
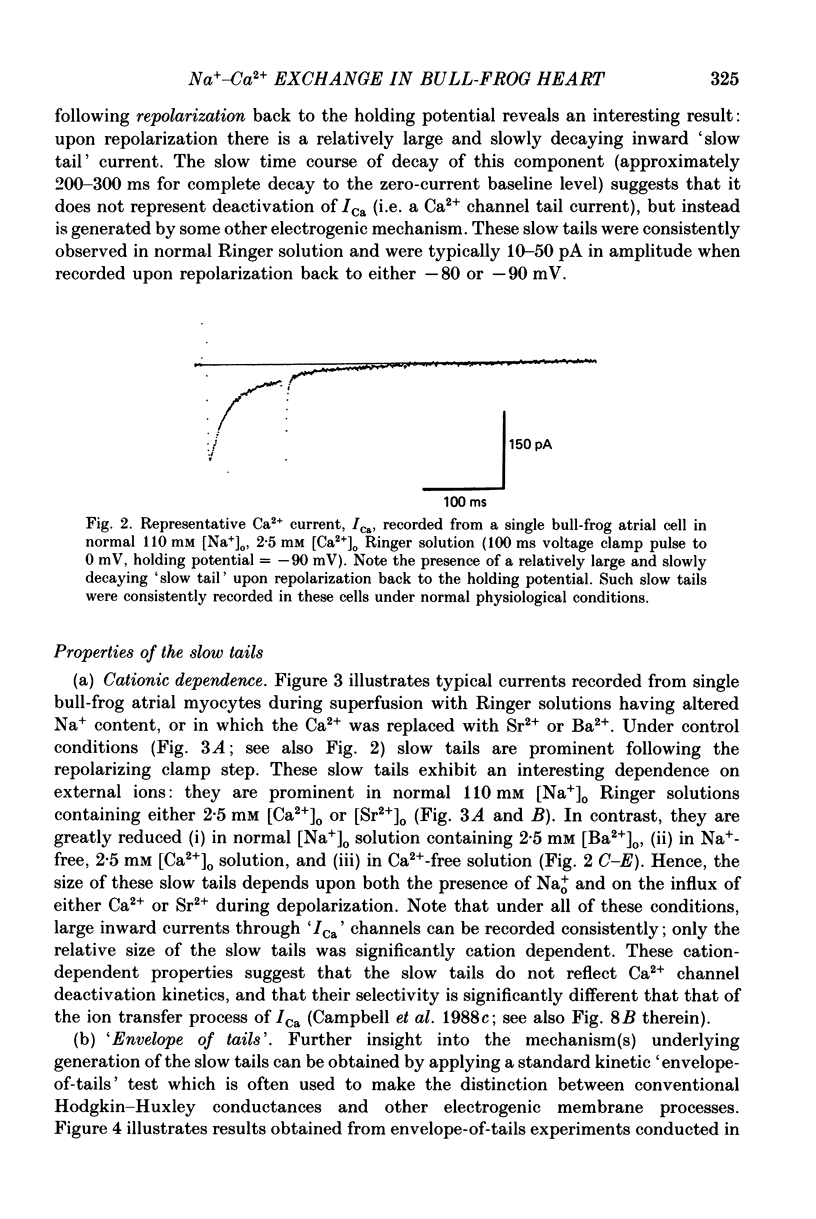
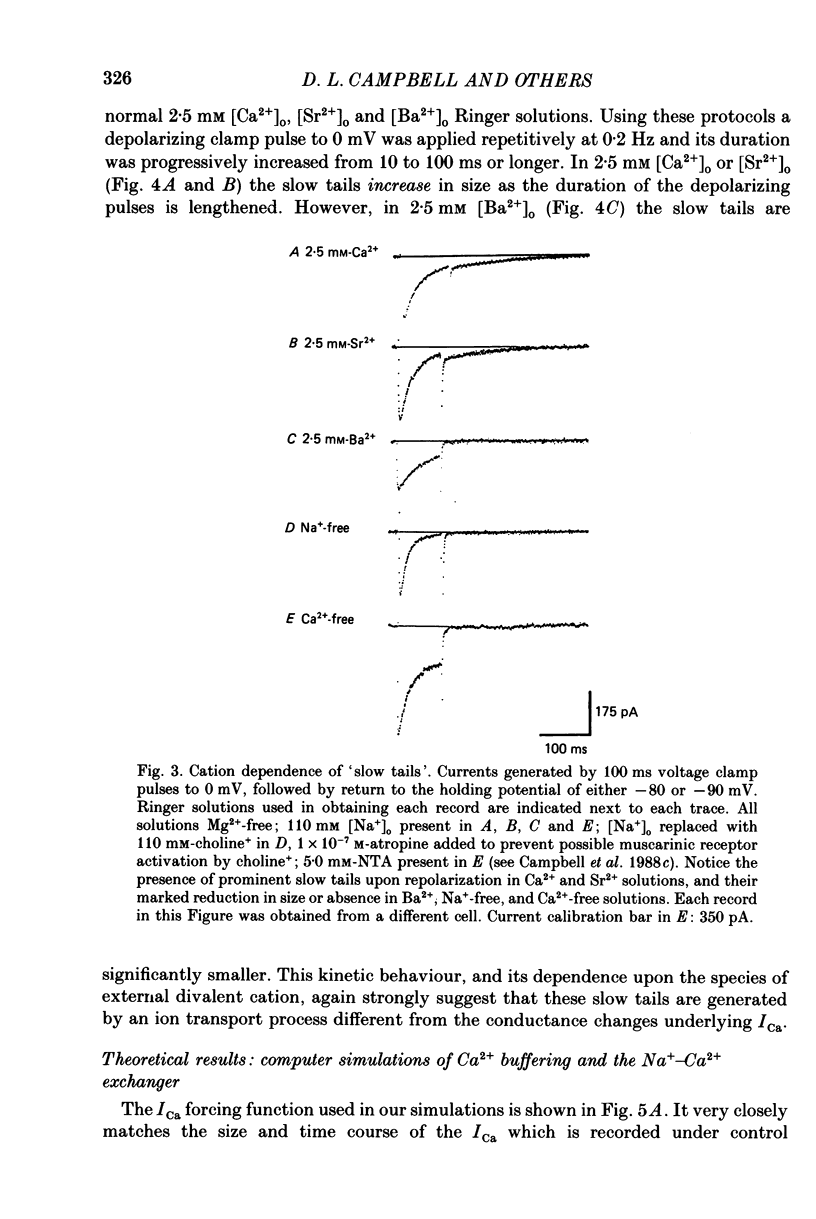
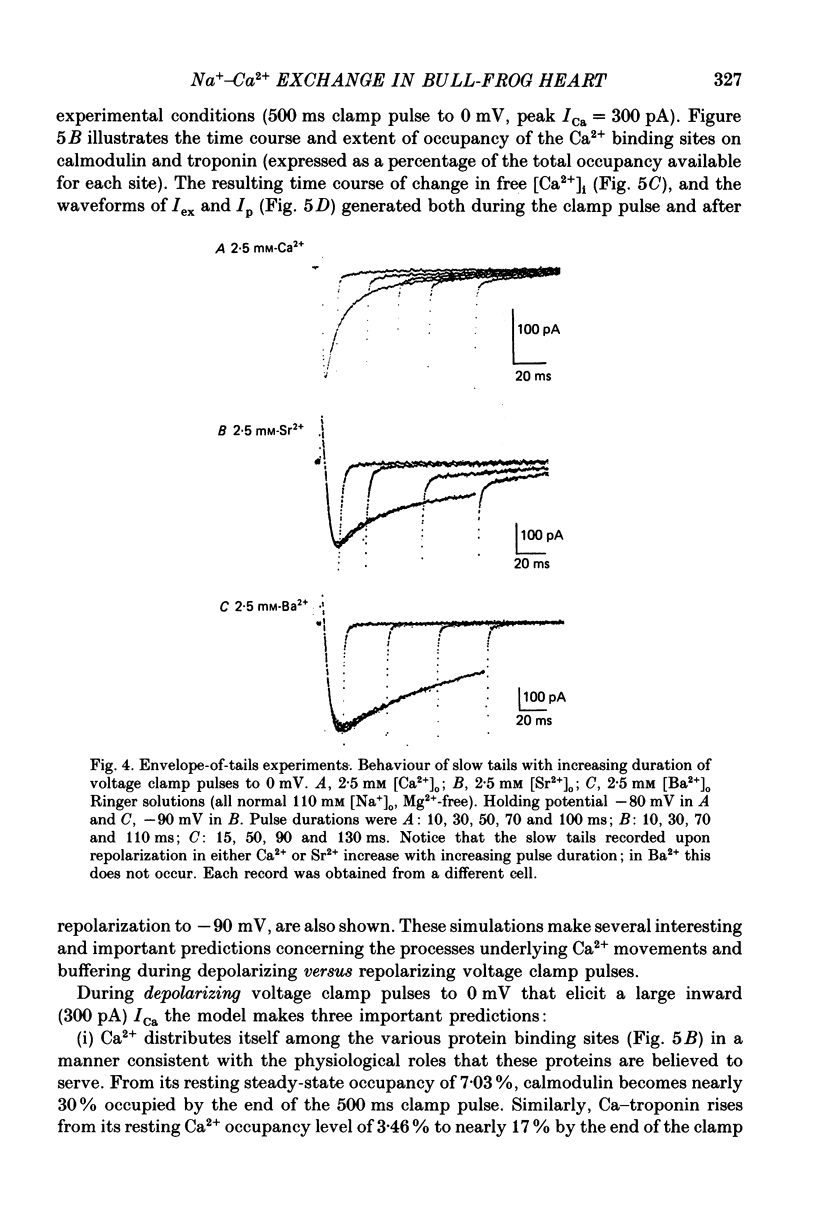
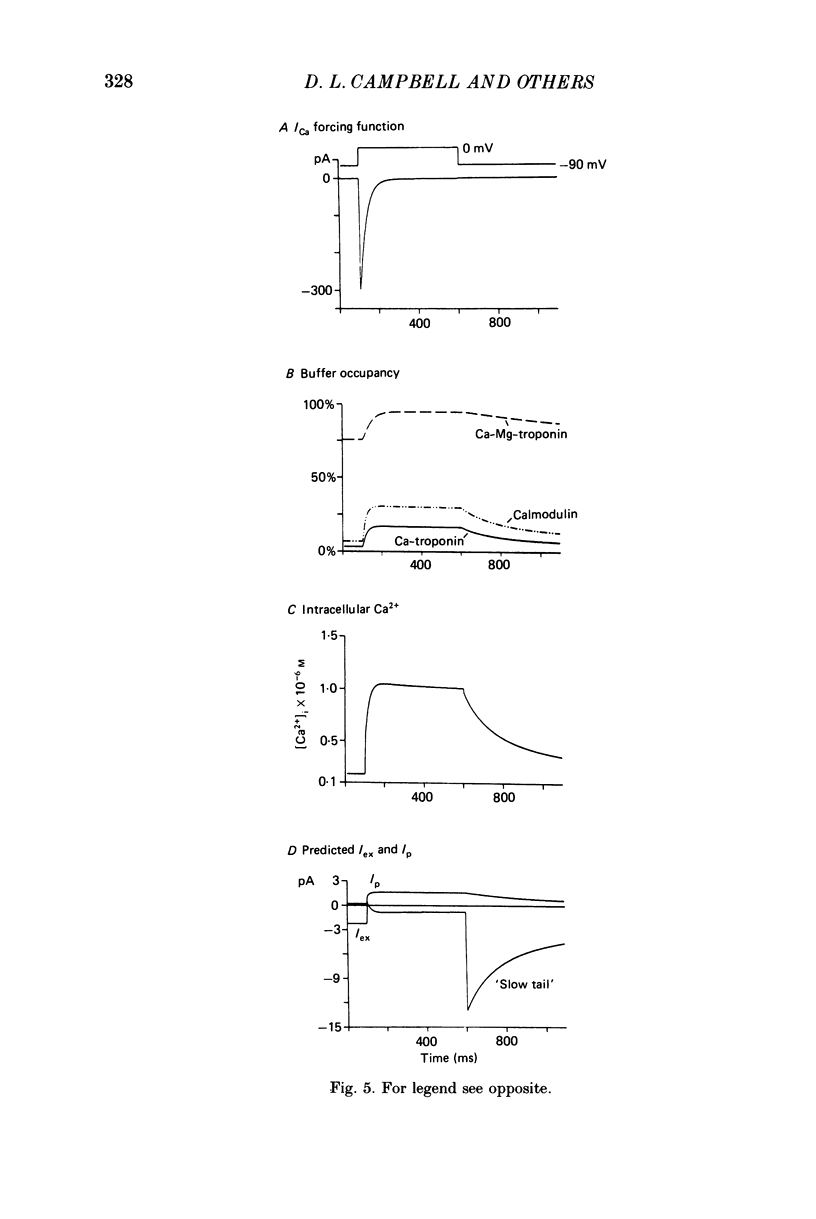
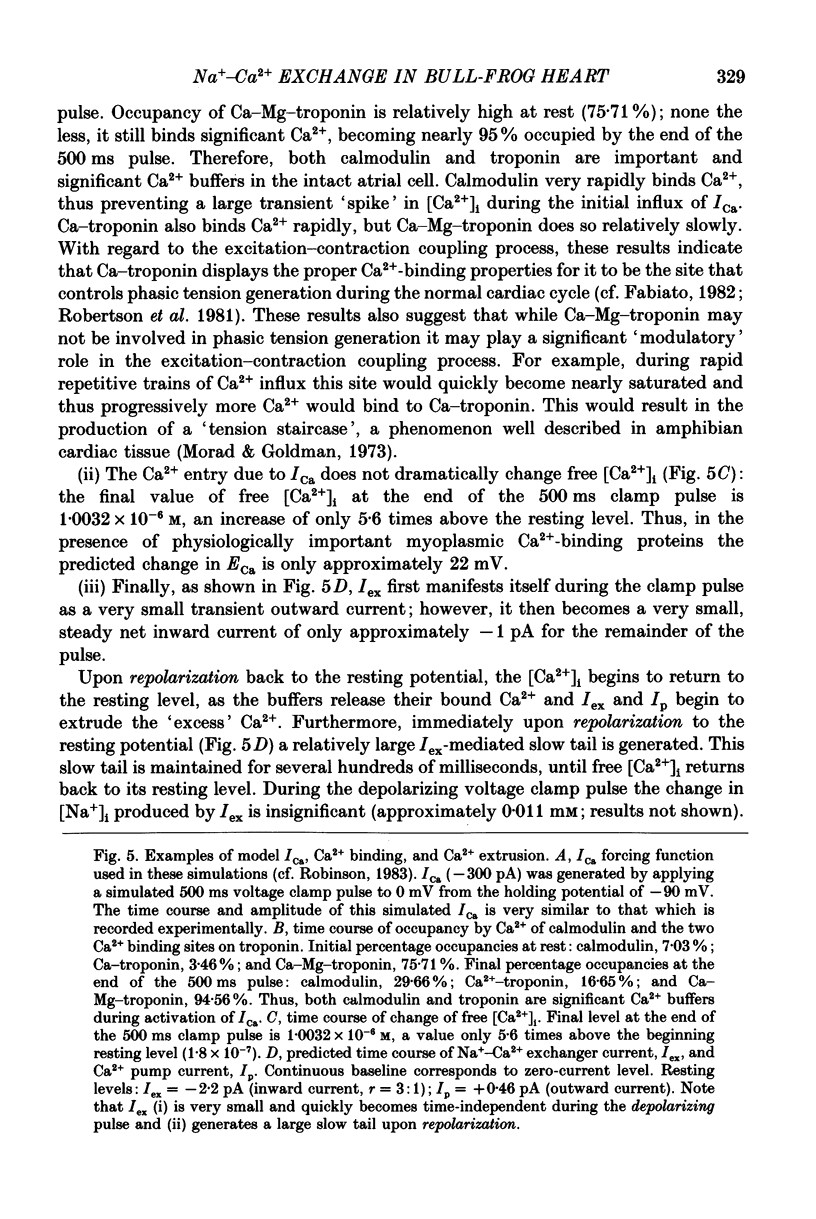
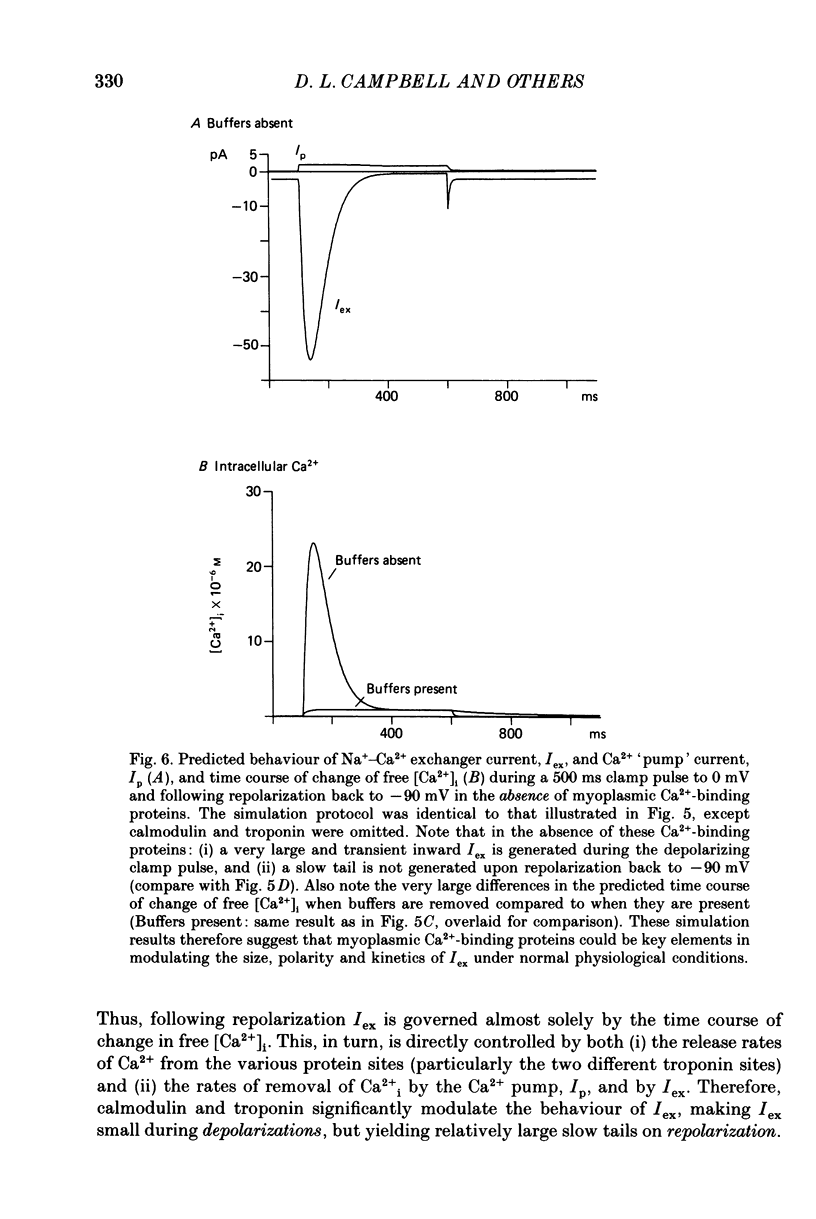
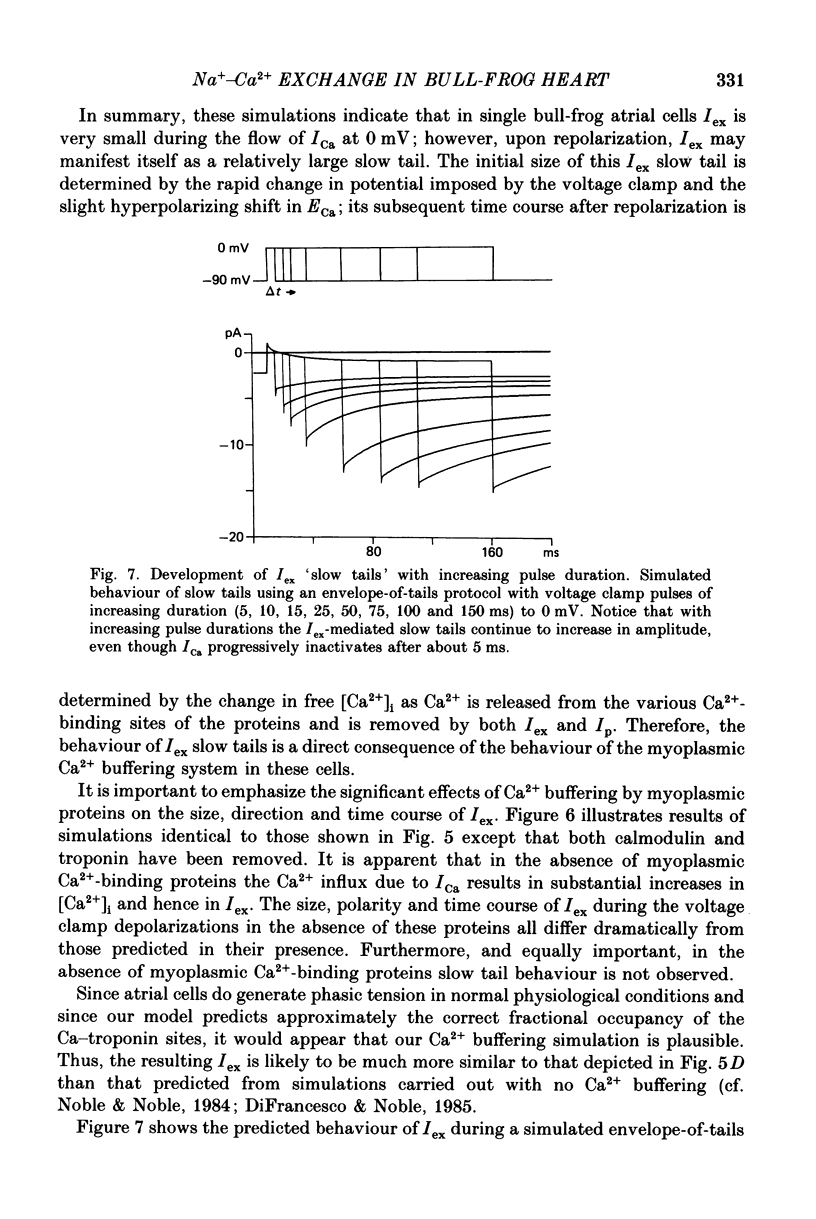
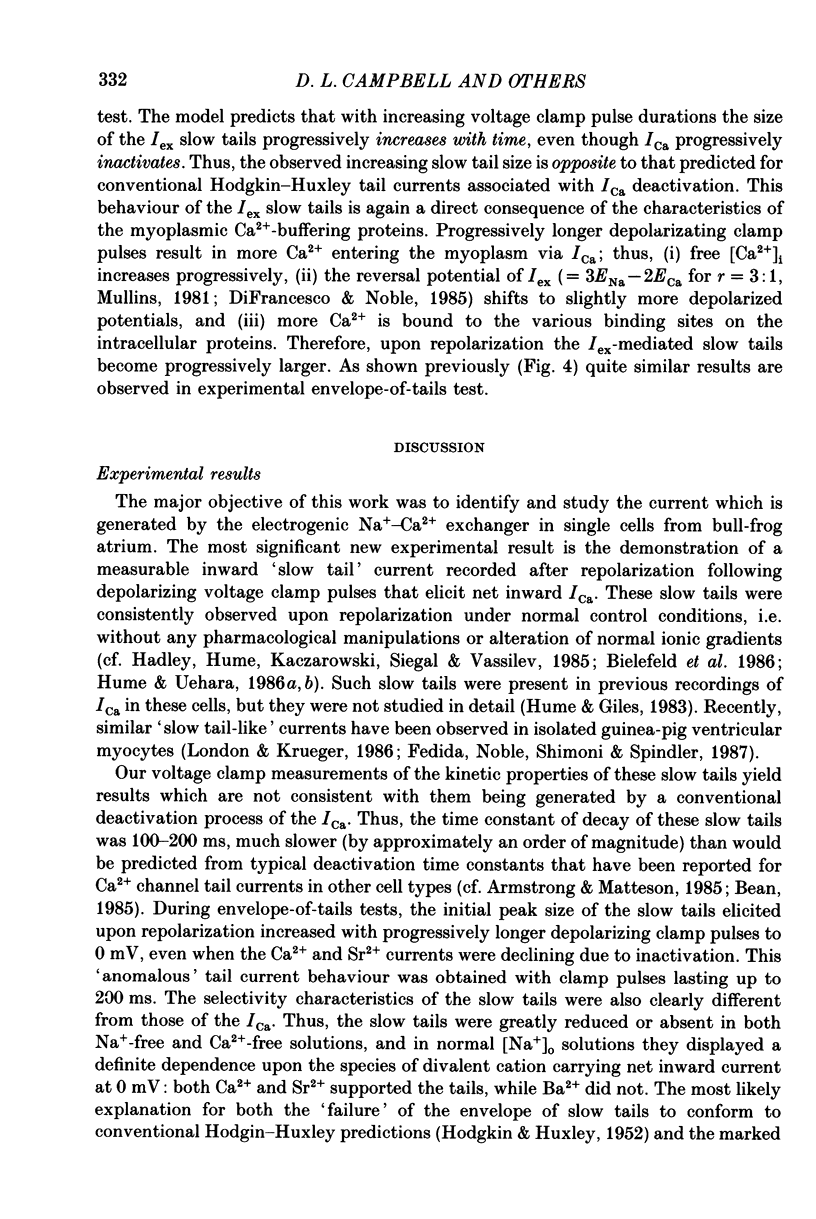
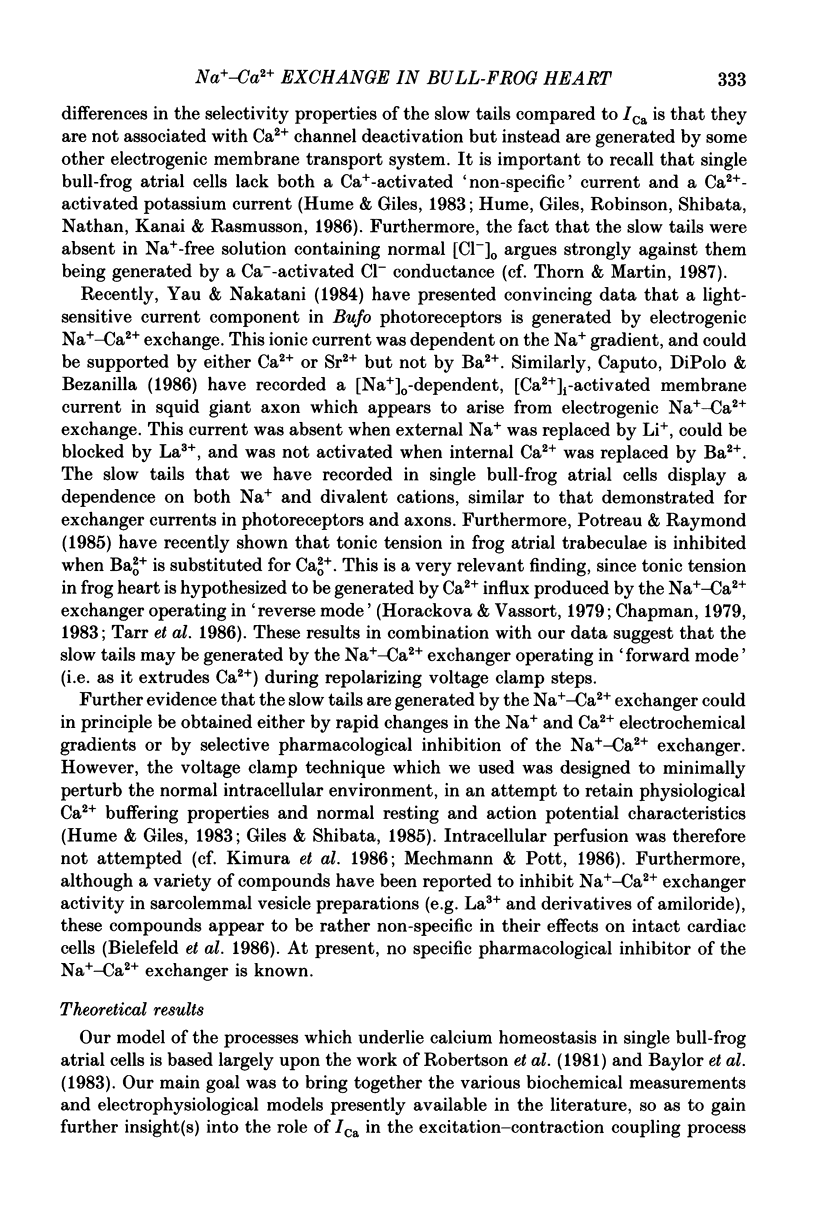
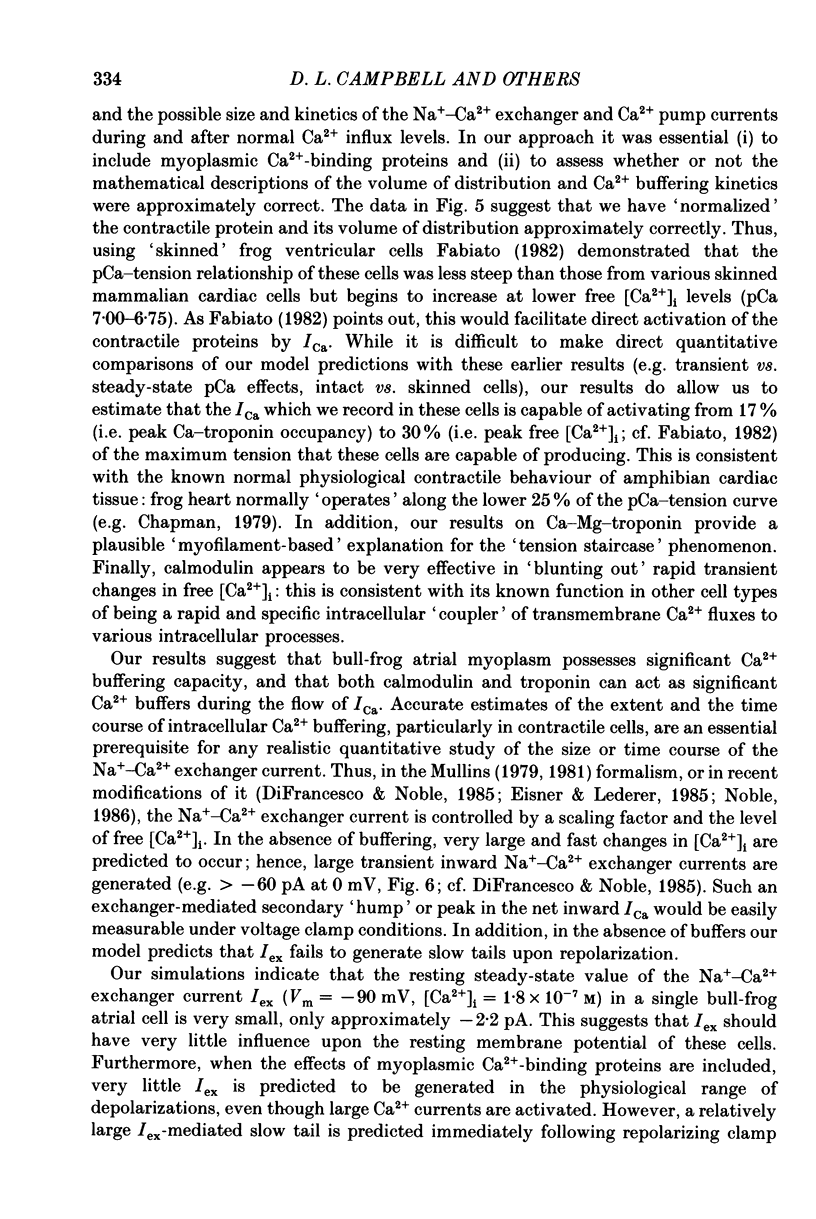
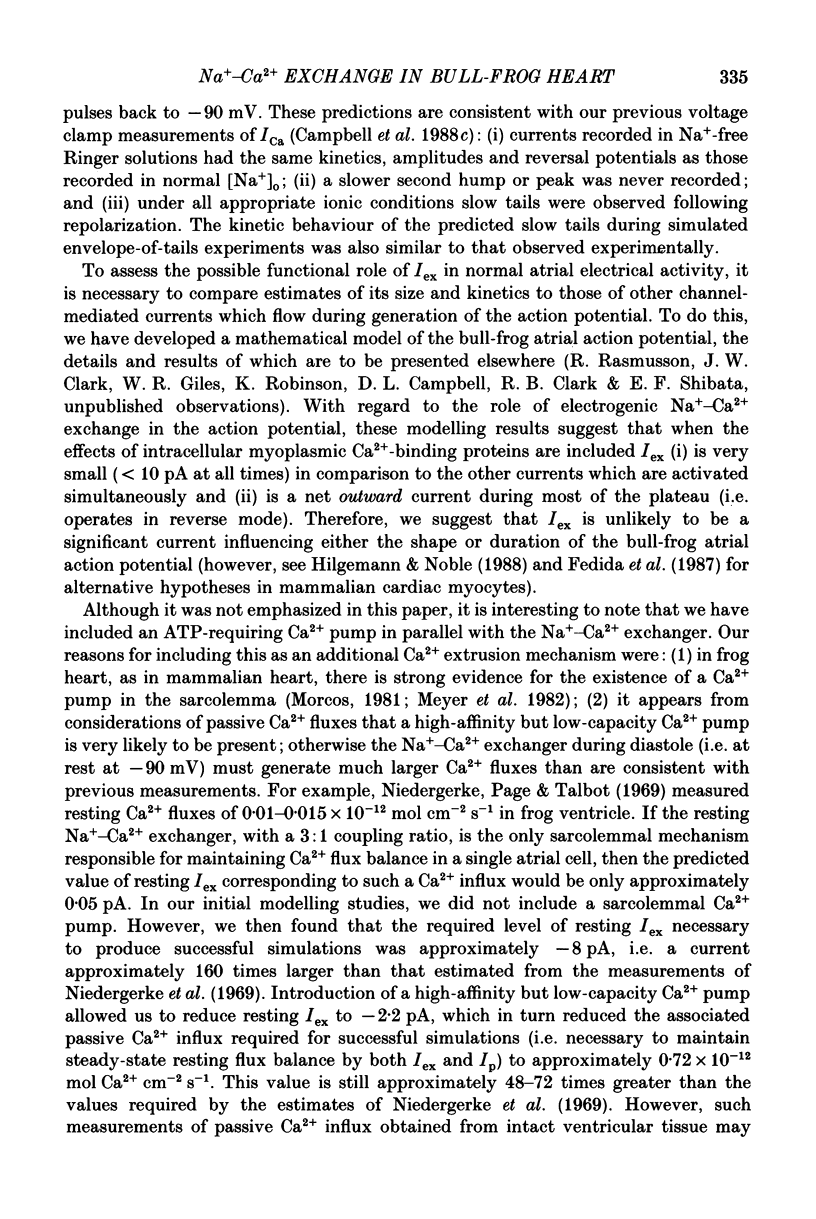
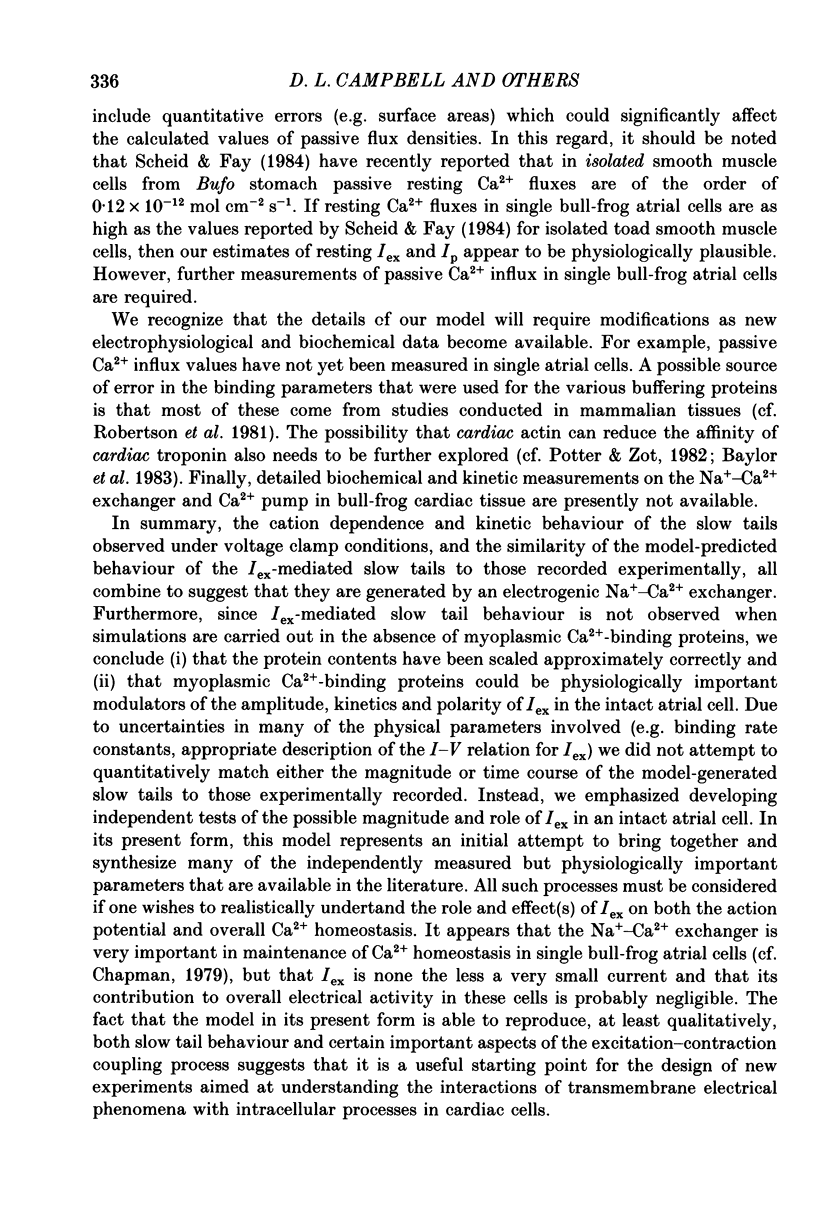
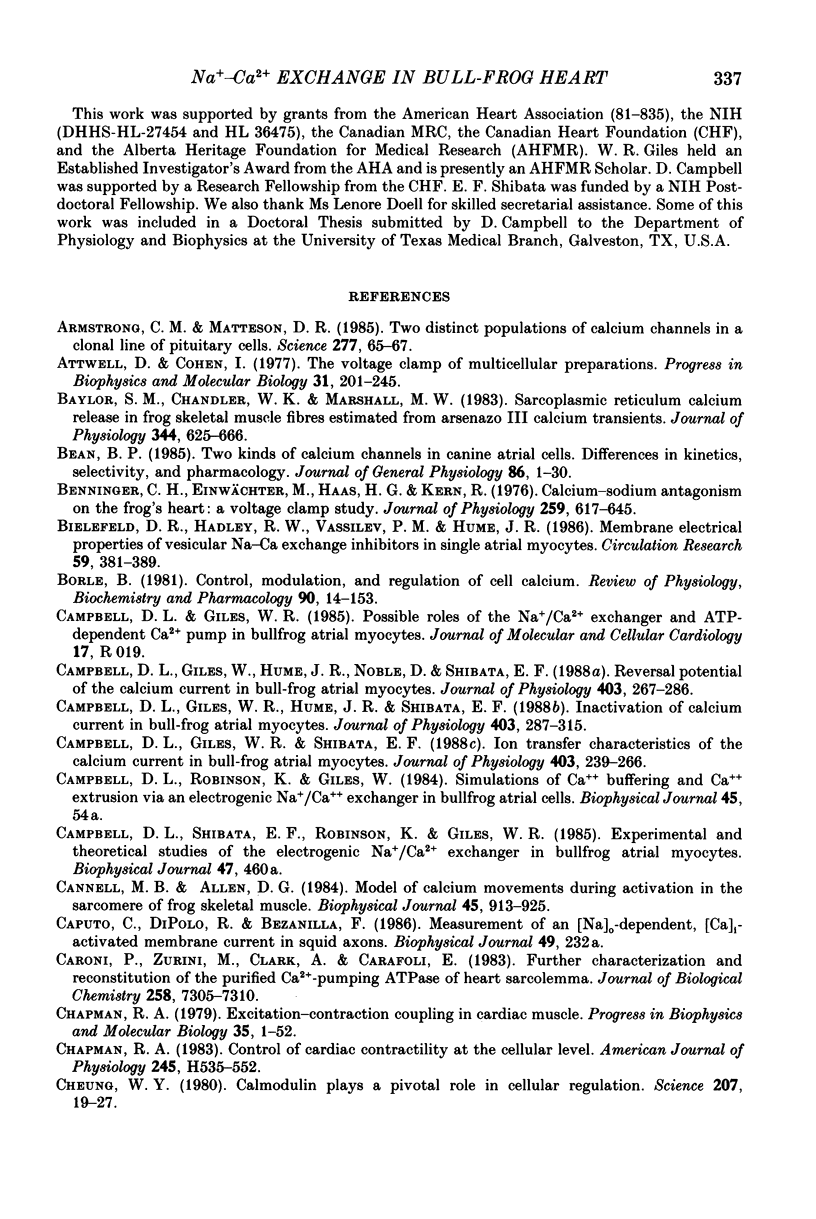
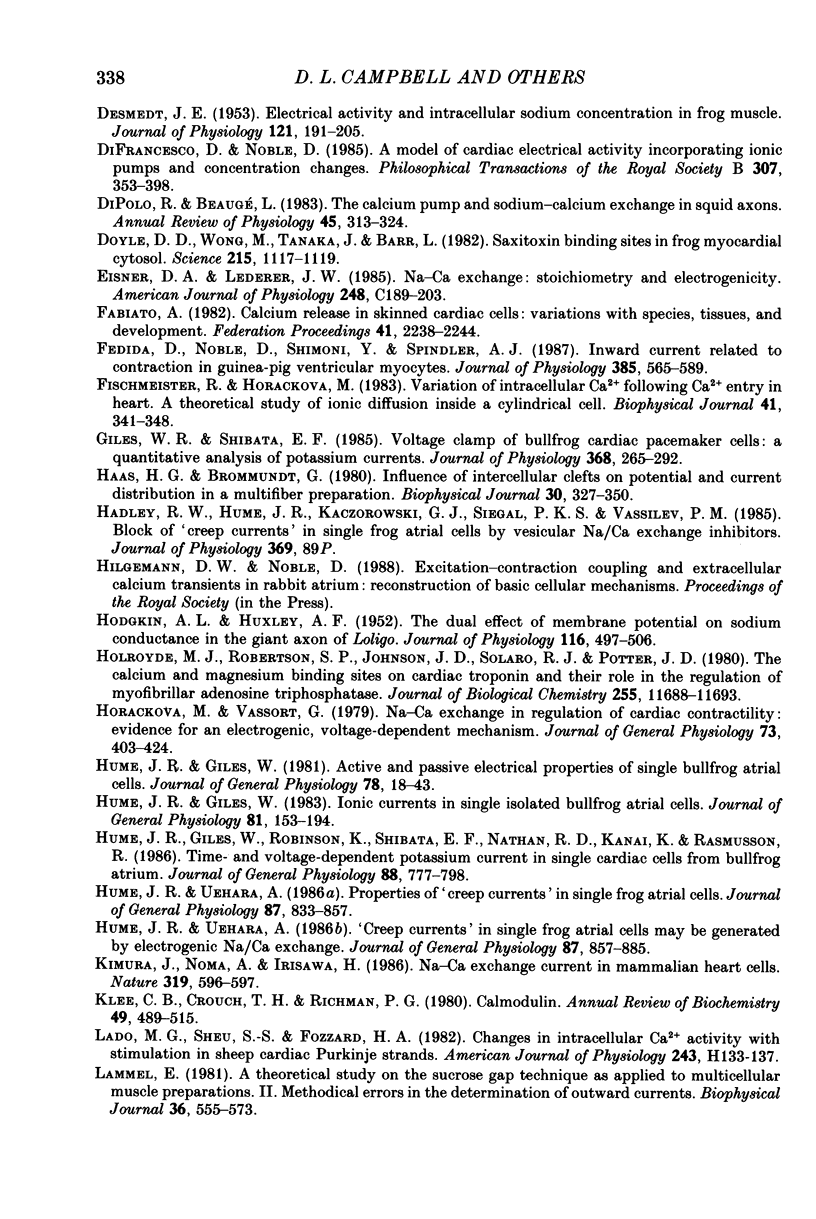
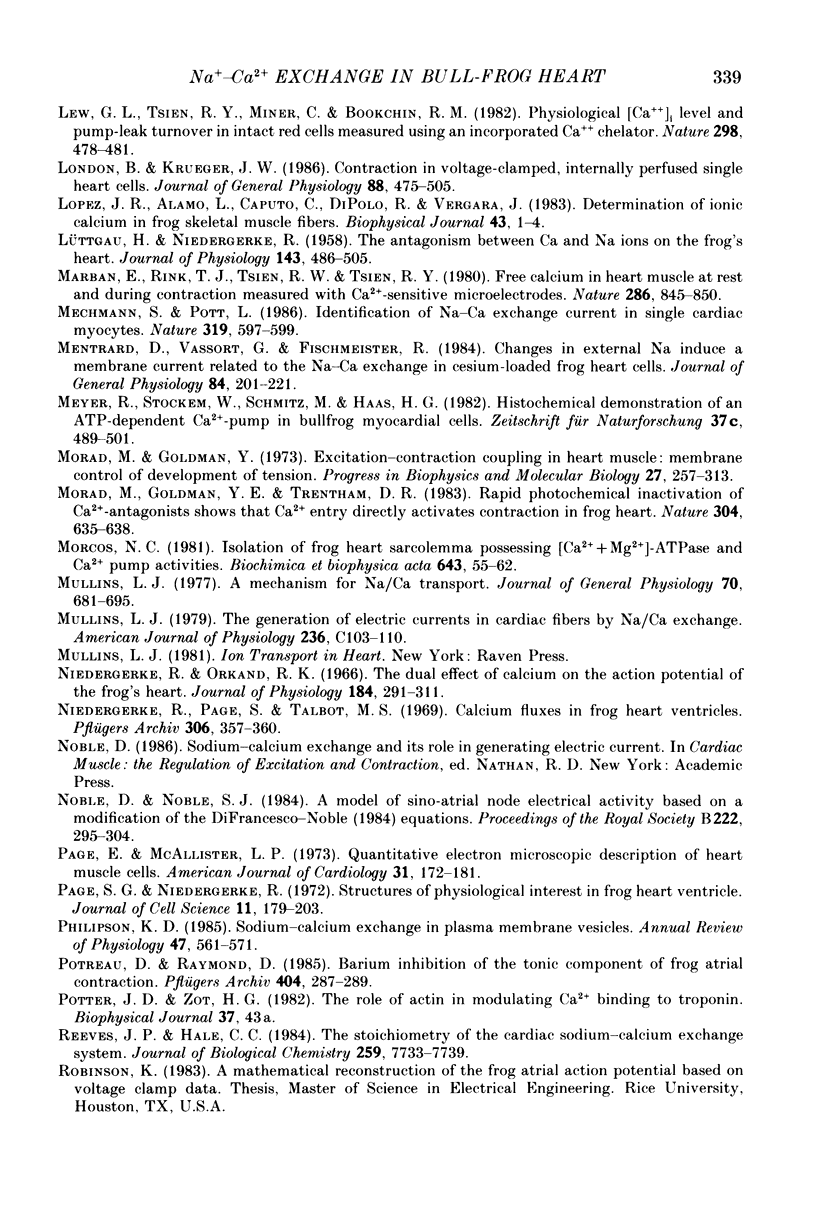
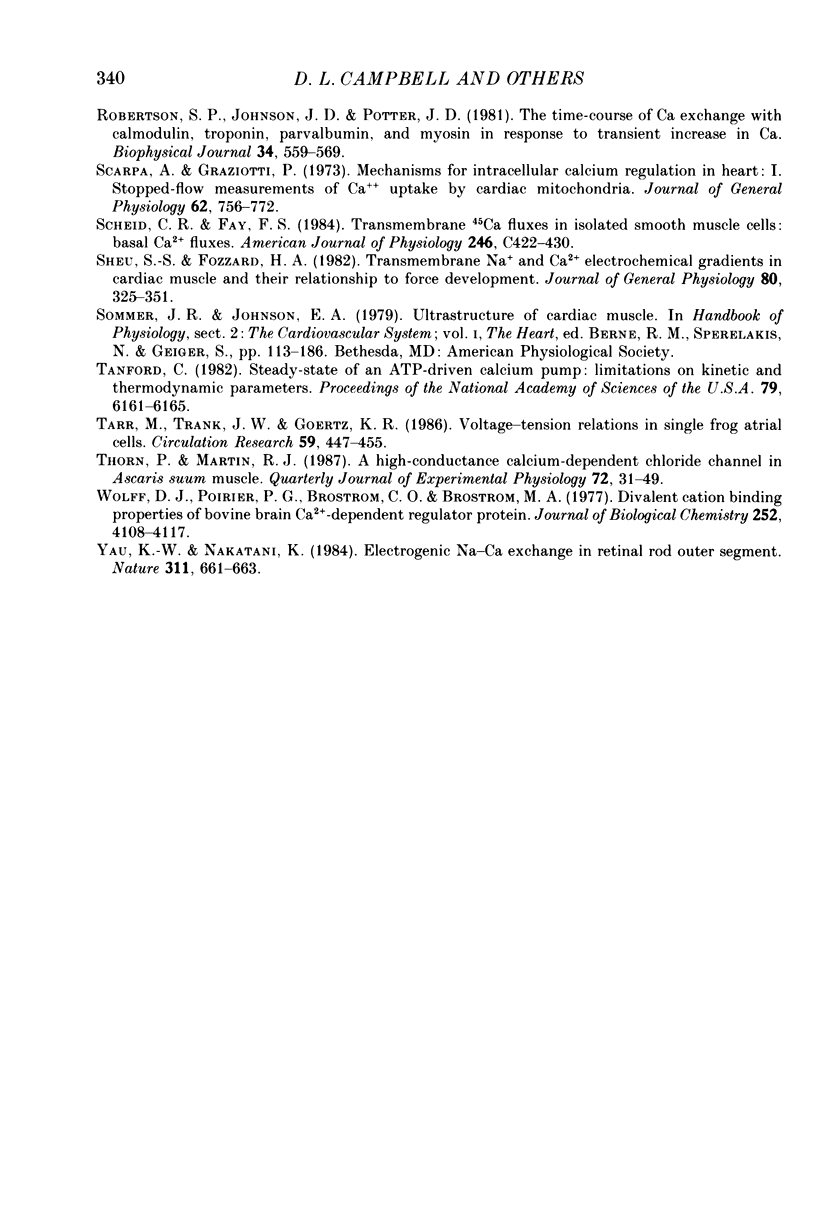
Selected References
These references are in PubMed. This may not be the complete list of references from this article.
- Armstrong C. M., Matteson D. R. Two distinct populations of calcium channels in a clonal line of pituitary cells. Science. 1985 Jan 4;227(4682):65–67. doi: 10.1126/science.2578071. [DOI] [PubMed] [Google Scholar]
- Attwell D., Cohen I. The voltage clamp of multicellular preparations. Prog Biophys Mol Biol. 1977;31(3):201–245. doi: 10.1016/0079-6107(78)90009-3. [DOI] [PubMed] [Google Scholar]
- Baylor S. M., Chandler W. K., Marshall M. W. Sarcoplasmic reticulum calcium release in frog skeletal muscle fibres estimated from Arsenazo III calcium transients. J Physiol. 1983 Nov;344:625–666. doi: 10.1113/jphysiol.1983.sp014959. [DOI] [PMC free article] [PubMed] [Google Scholar]
- Bean B. P. Two kinds of calcium channels in canine atrial cells. Differences in kinetics, selectivity, and pharmacology. J Gen Physiol. 1985 Jul;86(1):1–30. doi: 10.1085/jgp.86.1.1. [DOI] [PMC free article] [PubMed] [Google Scholar]
- Benninger C., Einwächter H. M., Haas H. G., Kern R. Calcium-sodium antagonism on the frog's heart: a voltage-clamp study. J Physiol. 1976 Aug;259(3):617–645. doi: 10.1113/jphysiol.1976.sp011486. [DOI] [PMC free article] [PubMed] [Google Scholar]
- Bielefeld D. R., Hadley R. W., Vassilev P. M., Hume J. R. Membrane electrical properties of vesicular Na-Ca exchange inhibitors in single atrial myocytes. Circ Res. 1986 Oct;59(4):381–389. doi: 10.1161/01.res.59.4.381. [DOI] [PubMed] [Google Scholar]
- Borle A. B. Control, Modulation, and regulation of cell calcium. Rev Physiol Biochem Pharmacol. 1981;90:13–153. doi: 10.1007/BFb0034078. [DOI] [PubMed] [Google Scholar]
- Campbell D. L., Giles W. R., Hume J. R., Noble D., Shibata E. F. Reversal potential of the calcium current in bull-frog atrial myocytes. J Physiol. 1988 Sep;403:267–286. doi: 10.1113/jphysiol.1988.sp017249. [DOI] [PMC free article] [PubMed] [Google Scholar]
- Campbell D. L., Giles W. R., Hume J. R., Shibata E. F. Inactivation of calcium current in bull-frog atrial myocytes. J Physiol. 1988 Sep;403:287–315. doi: 10.1113/jphysiol.1988.sp017250. [DOI] [PMC free article] [PubMed] [Google Scholar]
- Campbell D. L., Giles W. R., Shibata E. F. Ion transfer characteristics of the calcium current in bull-frog atrial myocytes. J Physiol. 1988 Sep;403:239–266. doi: 10.1113/jphysiol.1988.sp017248. [DOI] [PMC free article] [PubMed] [Google Scholar]
- Cannell M. B., Allen D. G. Model of calcium movements during activation in the sarcomere of frog skeletal muscle. Biophys J. 1984 May;45(5):913–925. doi: 10.1016/S0006-3495(84)84238-1. [DOI] [PMC free article] [PubMed] [Google Scholar]
- Caroni P., Zurini M., Clark A., Carafoli E. Further characterization and reconstitution of the purified Ca2+-pumping ATPase of heart sarcolemma. J Biol Chem. 1983 Jun 25;258(12):7305–7310. [PubMed] [Google Scholar]
- Chapman R. A. Control of cardiac contractility at the cellular level. Am J Physiol. 1983 Oct;245(4):H535–H552. doi: 10.1152/ajpheart.1983.245.4.H535. [DOI] [PubMed] [Google Scholar]
- Chapman R. A. Excitation-contraction coupling in cardiac muscle. Prog Biophys Mol Biol. 1979;35(1):1–52. doi: 10.1016/0079-6107(80)90002-4. [DOI] [PubMed] [Google Scholar]
- Cheung W. Y. Calmodulin plays a pivotal role in cellular regulation. Science. 1980 Jan 4;207(4426):19–27. doi: 10.1126/science.6243188. [DOI] [PubMed] [Google Scholar]
- DESMEDT J. E. Electrical activity and intracellular sodium concentration in frog muscle. J Physiol. 1953 Jul;121(1):191–205. doi: 10.1113/jphysiol.1953.sp004940. [DOI] [PMC free article] [PubMed] [Google Scholar]
- DiFrancesco D., Noble D. A model of cardiac electrical activity incorporating ionic pumps and concentration changes. Philos Trans R Soc Lond B Biol Sci. 1985 Jan 10;307(1133):353–398. doi: 10.1098/rstb.1985.0001. [DOI] [PubMed] [Google Scholar]
- DiPolo R., Beaugé L. The calcium pump and sodium-calcium exchange in squid axons. Annu Rev Physiol. 1983;45:313–324. doi: 10.1146/annurev.ph.45.030183.001525. [DOI] [PubMed] [Google Scholar]
- Doyle D. D., Wong M., Tanaka J., Barr L. Saxitoxin binding sites in frog-myocardial cytosol. Science. 1982 Feb 26;215(4536):1117–1119. doi: 10.1126/science.6278588. [DOI] [PubMed] [Google Scholar]
- Eisner D. A., Lederer W. J. Na-Ca exchange: stoichiometry and electrogenicity. Am J Physiol. 1985 Mar;248(3 Pt 1):C189–C202. doi: 10.1152/ajpcell.1985.248.3.C189. [DOI] [PubMed] [Google Scholar]
- Fabiato A. Calcium release in skinned cardiac cells: variations with species, tissues, and development. Fed Proc. 1982 May;41(7):2238–2244. [PubMed] [Google Scholar]
- Fedida D., Noble D., Shimoni Y., Spindler A. J. Inward current related to contraction in guinea-pig ventricular myocytes. J Physiol. 1987 Apr;385:565–589. doi: 10.1113/jphysiol.1987.sp016508. [DOI] [PMC free article] [PubMed] [Google Scholar]
- Fischmeister R., Horackova M. Variation of intracellular Ca2+ following Ca2+ current in heart. A theoretical study of ionic diffusion inside a cylindrical cell. Biophys J. 1983 Mar;41(3):341–348. doi: 10.1016/S0006-3495(83)84445-2. [DOI] [PMC free article] [PubMed] [Google Scholar]
- Giles W. R., Shibata E. F. Voltage clamp of bull-frog cardiac pace-maker cells: a quantitative analysis of potassium currents. J Physiol. 1985 Nov;368:265–292. doi: 10.1113/jphysiol.1985.sp015857. [DOI] [PMC free article] [PubMed] [Google Scholar]
- HODGKIN A. L., HUXLEY A. F. The dual effect of membrane potential on sodium conductance in the giant axon of Loligo. J Physiol. 1952 Apr;116(4):497–506. doi: 10.1113/jphysiol.1952.sp004719. [DOI] [PMC free article] [PubMed] [Google Scholar]
- Haas H. G., Brommundt G. Influence of intercellular clefts on potential and current distribution in a multifiber preparation. Biophys J. 1980 May;30(2):327–349. doi: 10.1016/S0006-3495(80)85098-3. [DOI] [PMC free article] [PubMed] [Google Scholar]
- Holroyde M. J., Robertson S. P., Johnson J. D., Solaro R. J., Potter J. D. The calcium and magnesium binding sites on cardiac troponin and their role in the regulation of myofibrillar adenosine triphosphatase. J Biol Chem. 1980 Dec 25;255(24):11688–11693. [PubMed] [Google Scholar]
- Horackova M., Vassort G. Sodium-calcium exchange in regulation of cardiac contractility. Evidence for an electrogenic, voltage-dependent mechanism. J Gen Physiol. 1979 Apr;73(4):403–424. doi: 10.1085/jgp.73.4.403. [DOI] [PMC free article] [PubMed] [Google Scholar]
- Hume J. R., Giles W. Ionic currents in single isolated bullfrog atrial cells. J Gen Physiol. 1983 Feb;81(2):153–194. doi: 10.1085/jgp.81.2.153. [DOI] [PMC free article] [PubMed] [Google Scholar]
- Hume J. R., Giles W., Robinson K., Shibata E. F., Nathan R. D., Kanai K., Rasmusson R. A time- and voltage-dependent K+ current in single cardiac cells from bullfrog atrium. J Gen Physiol. 1986 Dec;88(6):777–798. doi: 10.1085/jgp.88.6.777. [DOI] [PMC free article] [PubMed] [Google Scholar]
- Hume J. R., Uehara A. "Creep currents" in single frog atrial cells may be generated by electrogenic Na/Ca exchange. J Gen Physiol. 1986 Jun;87(6):857–884. doi: 10.1085/jgp.87.6.857. [DOI] [PMC free article] [PubMed] [Google Scholar]
- Kimura J., Noma A., Irisawa H. Na-Ca exchange current in mammalian heart cells. Nature. 1986 Feb 13;319(6054):596–597. doi: 10.1038/319596a0. [DOI] [PubMed] [Google Scholar]
- Klee C. B., Crouch T. H., Richman P. G. Calmodulin. Annu Rev Biochem. 1980;49:489–515. doi: 10.1146/annurev.bi.49.070180.002421. [DOI] [PubMed] [Google Scholar]
- LUTTGAU H. C., NIEDERGERKE R. The antagonism between Ca and Na ions on the frog's heart. J Physiol. 1958 Oct 31;143(3):486–505. doi: 10.1113/jphysiol.1958.sp006073. [DOI] [PMC free article] [PubMed] [Google Scholar]
- Lado M. G., Sheu S. S., Fozzard H. A. Changes in intracellular Ca2+ activity with stimulation in sheep cardiac Purkinje strands. Am J Physiol. 1982 Jul;243(1):H133–H137. doi: 10.1152/ajpheart.1982.243.1.H133. [DOI] [PubMed] [Google Scholar]
- Lammel E. A theoretical study on the sucrose gap technique as applied to multicellular muscle preparations. II. Methodical errors in the determination of outward currents. Biophys J. 1981 Dec;36(3):555–573. doi: 10.1016/S0006-3495(81)84752-2. [DOI] [PMC free article] [PubMed] [Google Scholar]
- Lew V. L., Tsien R. Y., Miner C., Bookchin R. M. Physiological [Ca2+]i level and pump-leak turnover in intact red cells measured using an incorporated Ca chelator. Nature. 1982 Jul 29;298(5873):478–481. doi: 10.1038/298478a0. [DOI] [PubMed] [Google Scholar]
- London B., Krueger J. W. Contraction in voltage-clamped, internally perfused single heart cells. J Gen Physiol. 1986 Oct;88(4):475–505. doi: 10.1085/jgp.88.4.475. [DOI] [PMC free article] [PubMed] [Google Scholar]
- López J. R., Alamo L., Caputo C., DiPolo R., Vergara S. Determination of ionic calcium in frog skeletal muscle fibers. Biophys J. 1983 Jul;43(1):1–4. doi: 10.1016/S0006-3495(83)84316-1. [DOI] [PMC free article] [PubMed] [Google Scholar]
- Marban E., Rink T. J., Tsien R. W., Tsien R. Y. Free calcium in heart muscle at rest and during contraction measured with Ca2+ -sensitive microelectrodes. Nature. 1980 Aug 28;286(5776):845–850. doi: 10.1038/286845a0. [DOI] [PubMed] [Google Scholar]
- Mechmann S., Pott L. Identification of Na-Ca exchange current in single cardiac myocytes. Nature. 1986 Feb 13;319(6054):597–599. doi: 10.1038/319597a0. [DOI] [PubMed] [Google Scholar]
- Mentrard D., Vassort G., Fischmeister R. Changes in external Na induce a membrane current related to the Na-Ca exchange in cesium-loaded frog heart cells. J Gen Physiol. 1984 Aug;84(2):201–220. doi: 10.1085/jgp.84.2.201. [DOI] [PMC free article] [PubMed] [Google Scholar]
- Meyer R., Stockem W., Schmitz M., Haas H. G. Histochemical demonstration of an ATP-dependent Ca2+-pump in bullfrog myocardial cells. Z Naturforsch C. 1982 May-Jun;37(5-6):489–501. doi: 10.1515/znc-1982-5-622. [DOI] [PubMed] [Google Scholar]
- Morad M., Goldman Y. E., Trentham D. R. Rapid photochemical inactivation of Ca2+-antagonists shows that Ca2+ entry directly activates contraction in frog heart. Nature. 1983 Aug 18;304(5927):635–638. doi: 10.1038/304635a0. [DOI] [PubMed] [Google Scholar]
- Morcos N. C. Isolation of frog heart sarcolemma possessing (Ca2+ + Mg2+)-ATPase and Ca2+ pump activities. Biochim Biophys Acta. 1981 Apr 22;643(1):55–62. doi: 10.1016/0005-2736(81)90218-2. [DOI] [PubMed] [Google Scholar]
- Mullins L. J. A mechanism for Na/Ca transport. J Gen Physiol. 1977 Dec;70(6):681–695. doi: 10.1085/jgp.70.6.681. [DOI] [PMC free article] [PubMed] [Google Scholar]
- Niedergerke R., Orkand R. K. The dual effect of calcium on the action potential of the frog's heart. J Physiol. 1966 May;184(2):291–311. doi: 10.1113/jphysiol.1966.sp007916. [DOI] [PMC free article] [PubMed] [Google Scholar]
- Niedergerke R., Page S., Talbot M. S. Calcium fluxes in frog heart ventricles. Pflugers Arch. 1969;306(4):357–360. doi: 10.1007/BF00589161. [DOI] [PubMed] [Google Scholar]
- Noble D., Noble S. J. A model of sino-atrial node electrical activity based on a modification of the DiFrancesco-Noble (1984) equations. Proc R Soc Lond B Biol Sci. 1984 Sep 22;222(1228):295–304. doi: 10.1098/rspb.1984.0065. [DOI] [PubMed] [Google Scholar]
- Page E., McCallister L. P. Quantitative electron microscopic description of heart muscle cells. Application to normal, hypertrophied and thyroxin-stimulated hearts. Am J Cardiol. 1973 Feb;31(2):172–181. doi: 10.1016/0002-9149(73)91030-8. [DOI] [PubMed] [Google Scholar]
- Page S. G., Niedergerke R. Structures of physiological interest in the frog heart ventricle. J Cell Sci. 1972 Jul;11(1):179–203. doi: 10.1242/jcs.11.1.179. [DOI] [PubMed] [Google Scholar]
- Philipson K. D. Sodium-calcium exchange in plasma membrane vesicles. Annu Rev Physiol. 1985;47:561–571. doi: 10.1146/annurev.ph.47.030185.003021. [DOI] [PubMed] [Google Scholar]
- Potreau D., Raymond G. Barium inhibition of the tonic component of frog atrial contraction. Pflugers Arch. 1985 Jul;404(3):287–289. doi: 10.1007/BF00581253. [DOI] [PubMed] [Google Scholar]
- Reeves J. P., Hale C. C. The stoichiometry of the cardiac sodium-calcium exchange system. J Biol Chem. 1984 Jun 25;259(12):7733–7739. [PubMed] [Google Scholar]
- Robertson S. P., Johnson J. D., Potter J. D. The time-course of Ca2+ exchange with calmodulin, troponin, parvalbumin, and myosin in response to transient increases in Ca2+. Biophys J. 1981 Jun;34(3):559–569. doi: 10.1016/S0006-3495(81)84868-0. [DOI] [PMC free article] [PubMed] [Google Scholar]
- Scarpa A., Graziotti P. Mechanisms for intracellular calcium regulation in heart. I. Stopped-flow measurements of Ca++ uptake by cardiac mitochondria. J Gen Physiol. 1973 Dec;62(6):756–772. doi: 10.1085/jgp.62.6.756. [DOI] [PMC free article] [PubMed] [Google Scholar]
- Scheid C. R., Fay F. S. Transmembrane 45Ca fluxes in isolated smooth muscle cells: basal Ca2+ fluxes. Am J Physiol. 1984 May;246(5 Pt 1):C422–C430. doi: 10.1152/ajpcell.1984.246.5.C422. [DOI] [PubMed] [Google Scholar]
- Sheu S. S., Fozzard H. A. Transmembrane Na+ and Ca2+ electrochemical gradients in cardiac muscle and their relationship to force development. J Gen Physiol. 1982 Sep;80(3):325–351. doi: 10.1085/jgp.80.3.325. [DOI] [PMC free article] [PubMed] [Google Scholar]
- Tanford C. Steady state of an ATP-driven calcium pump: limitations on kinetic and thermodynamic parameters. Proc Natl Acad Sci U S A. 1982 Oct;79(20):6161–6165. doi: 10.1073/pnas.79.20.6161. [DOI] [PMC free article] [PubMed] [Google Scholar]
- Tarr M., Trank J. W., Goertz K. K. Voltage-tension relations in single frog atrial cardiac cells. Circ Res. 1986 Oct;59(4):447–455. doi: 10.1161/01.res.59.4.447. [DOI] [PubMed] [Google Scholar]
- Thorn P., Martin R. J. A high-conductance calcium-dependent chloride channel in Ascaris suum muscle. Q J Exp Physiol. 1987 Jan;72(1):31–49. doi: 10.1113/expphysiol.1987.sp003053. [DOI] [PubMed] [Google Scholar]
- Wolff D. J., Poirier P. G., Brostrom C. O., Brostrom M. A. Divalent cation binding properties of bovine brain Ca2+-dependent regulator protein. J Biol Chem. 1977 Jun 25;252(12):4108–4117. [PubMed] [Google Scholar]
- Yau K. W., Nakatani K. Electrogenic Na-Ca exchange in retinal rod outer segment. Nature. 1984 Oct 18;311(5987):661–663. doi: 10.1038/311661a0. [DOI] [PubMed] [Google Scholar]