Abstract
1. The effects of varied levels (25-300 mM) of ionic strength on mechanical properties and ATP hydrolysis rate of chemically skinned guinea-pig taenia coli fibres were investigated. 2. The tension development following activation by calcium (pCa 4.8), and relaxation following removal of calcium (pCa 9), were slower in 25 mM compared to 150 mM ionic strength. In fibres activated by thiophosphorylation of myosin light chains, by exposure to ATP-gamma-S, the tension development was rapid and independent of ionic strength. 3. The maximal shortening velocity (Vmax) was obtained from force-velocity relations determined by the quick-release method. The rate of ATP hydrolysis (JATP) was determined by measurement of pyruvate released from phosphoenolpyruvate (PEP). In order to obtain maximal Vmax and JATP at a Mg-ATP concentration of 1 mM, an ATP regenerating system was required. In thiophosphorylated fibres 2 mM-phosphocreatine (PCr) or 3.2 mM-PEP were adequate for maximal Vmax and JATP respectively. In calcium-activated fibres 5 mM-PCr was required for maximal Vmax. 4. The isometric force of thiophosphorylated fibres showed a biphasic dependence on ionic strength with a maximum at 90 mM. Vmax was essentially unchanged between 50 and 200 mM ionic strength. At 25 mM ionic strength, isometric force and Vmax were decreased by, respectively, about 15 and 25%. At 250 mM ionic strength, isometric force and Vmax were decreased by, respectively, 47 and 33%. 5. Vmax decreased with decreasing [Mg-ATP]. At [Mg-ATP] less than 0.1 mM there was no difference in Vmax between 35 and 150 mM ionic strength. At 250 mM ionic strength Vmax was lower than that at 150 mM at all [Mg-ATP]. 6. JATP during contraction in thiophosphorylated fibres at 35, 150 and 250 mM ionic strength was respectively, 0.62, 0.98 and 0.93 mumol g-1 min-1. The energetic tension cost (JATP/force) increased with ionic strength. 7. The force response to a quick stretch was investigated in the relaxed, contracted and rigor states at 25, 150 and 250 mM ionic strength. Stiffness in the relaxed state increased with speed of stretch and was higher the lower the ionic strength. In the contracted and rigor states, stiffness was also affected by ionic strength, but the relative effect in the contracted state was small.(ABSTRACT TRUNCATED AT 400 WORDS)
Full text
PDF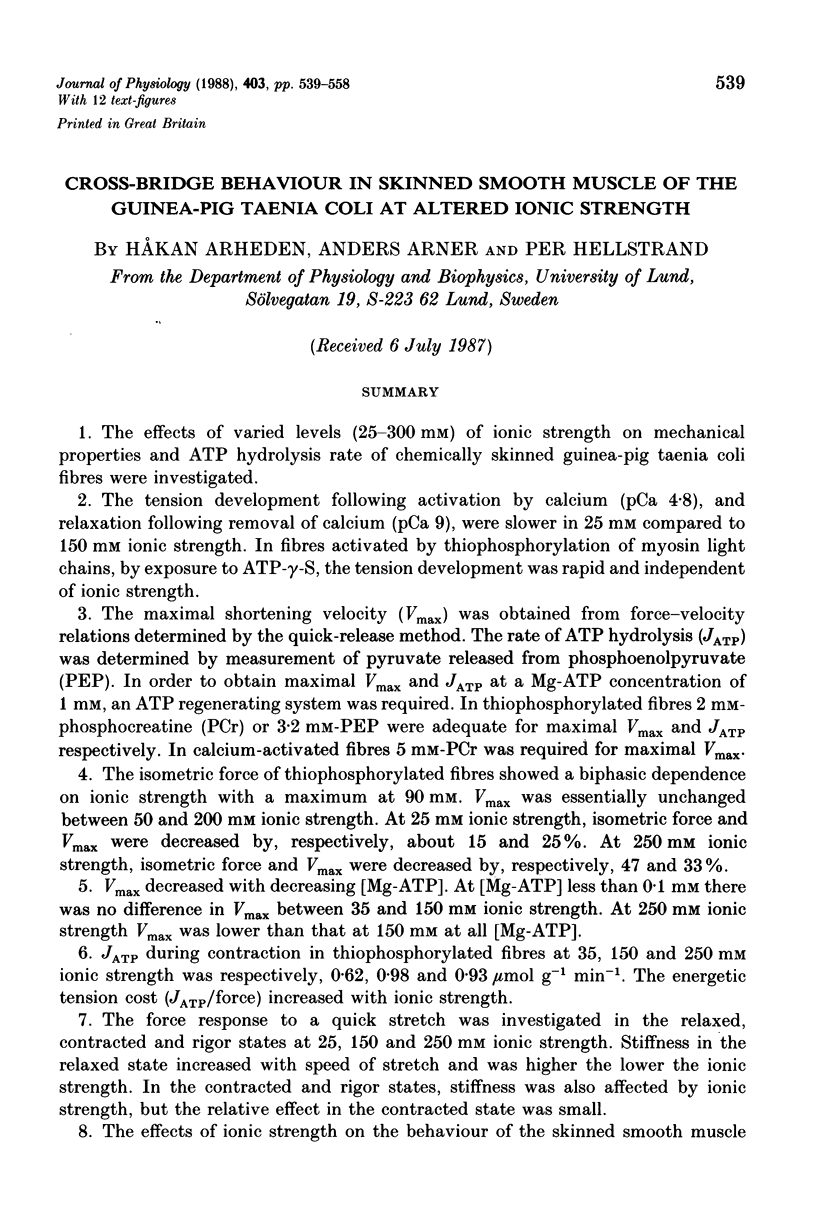
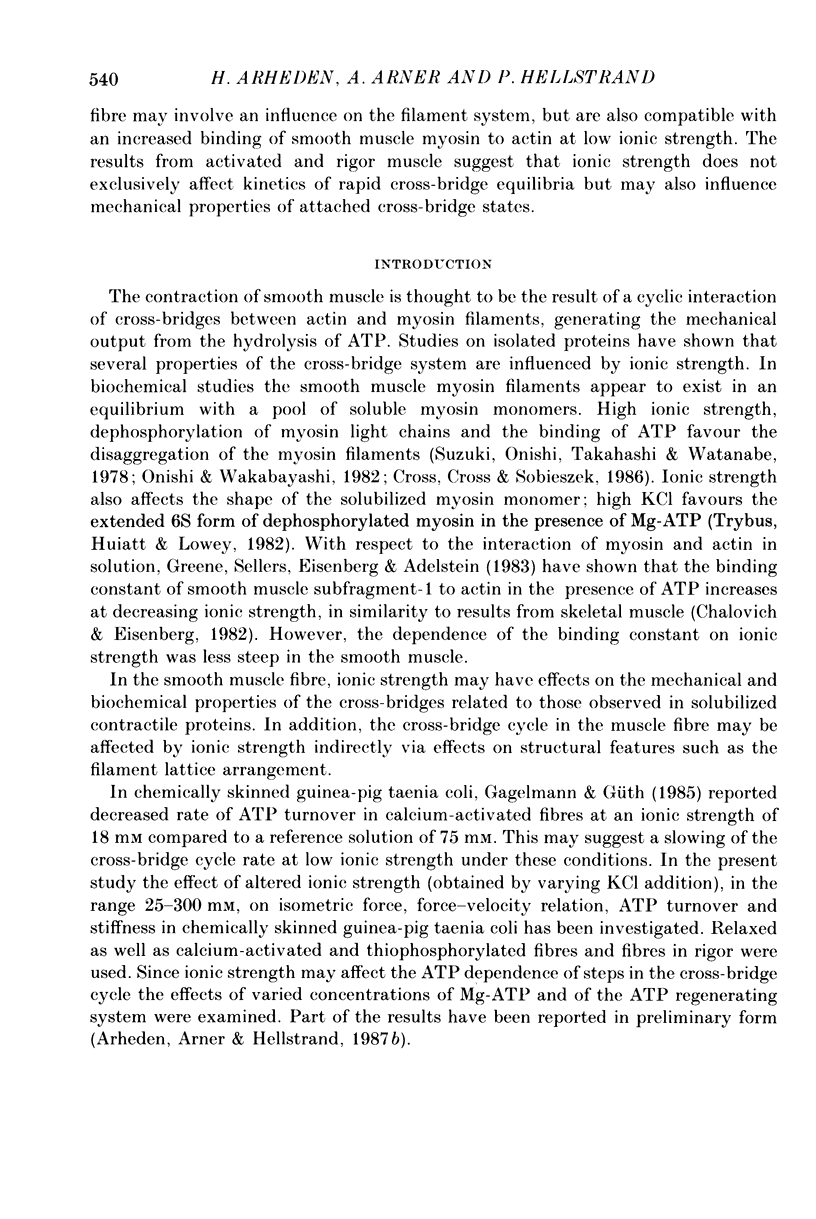
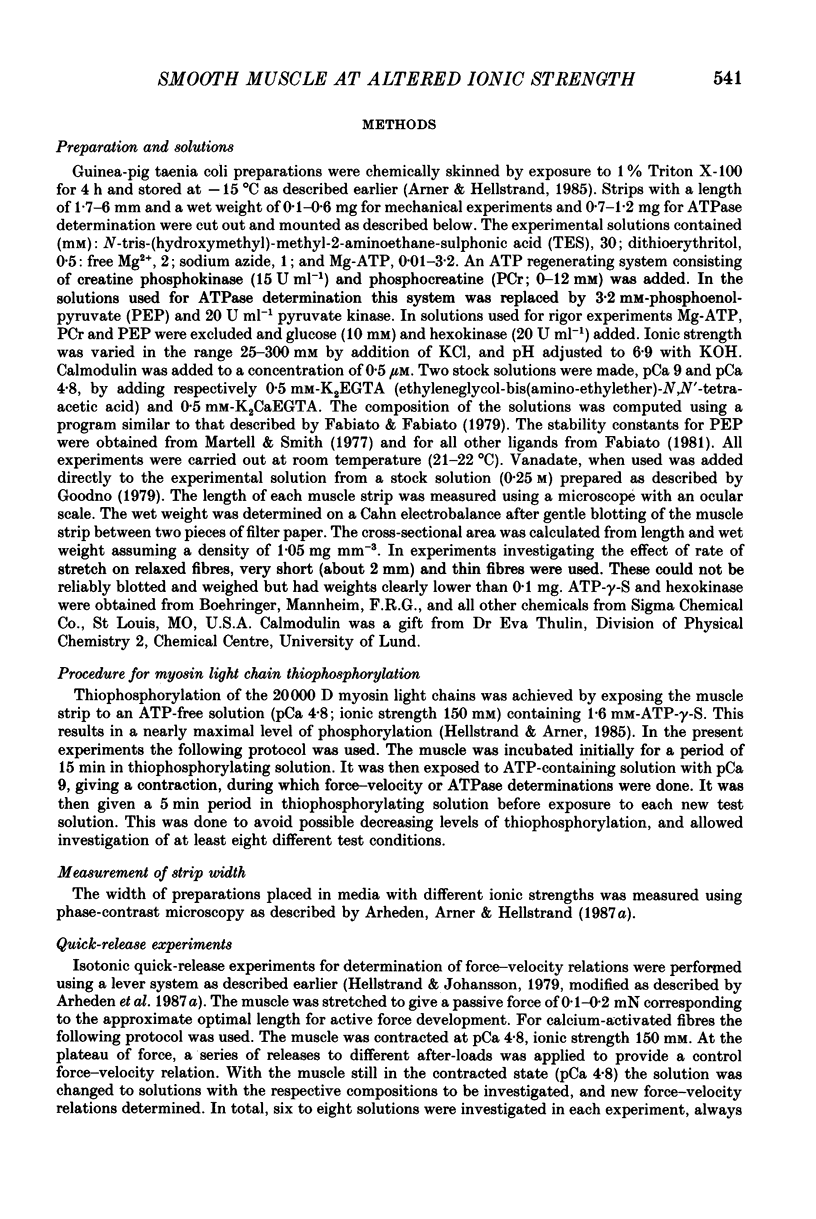
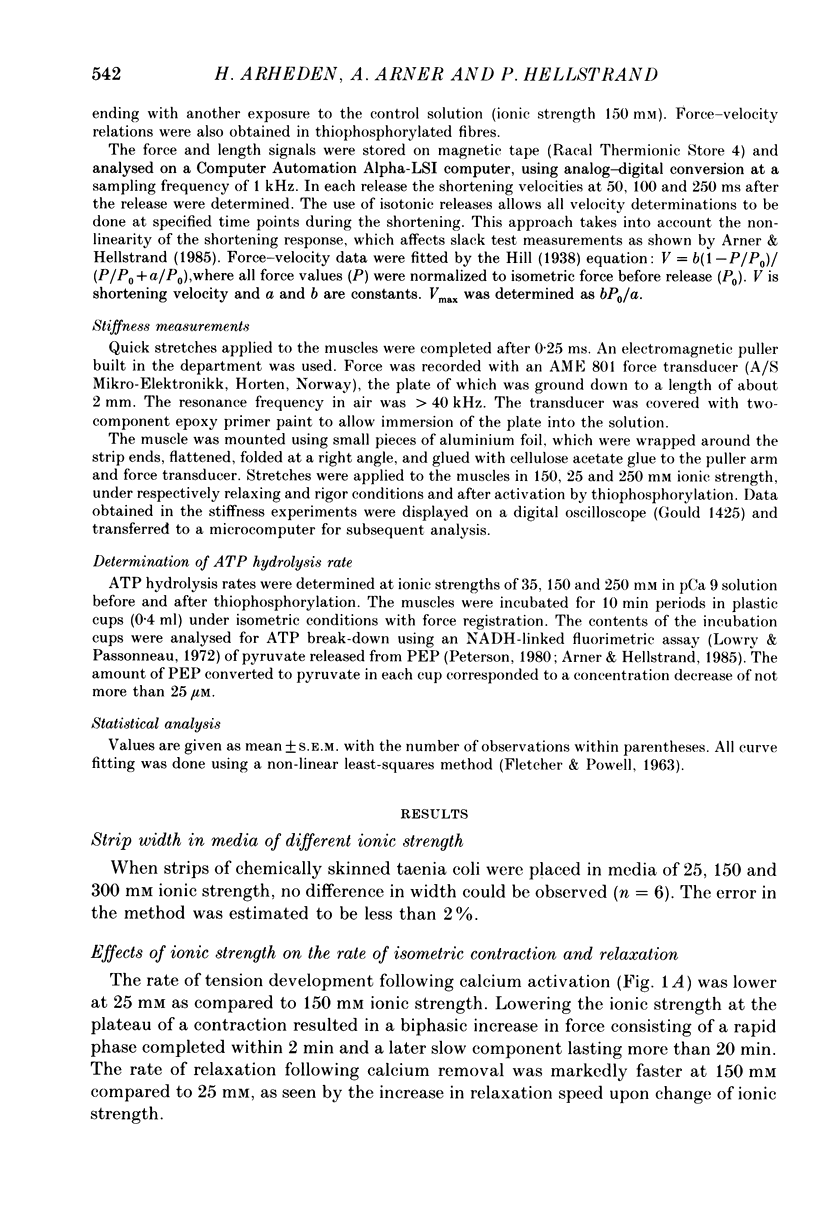
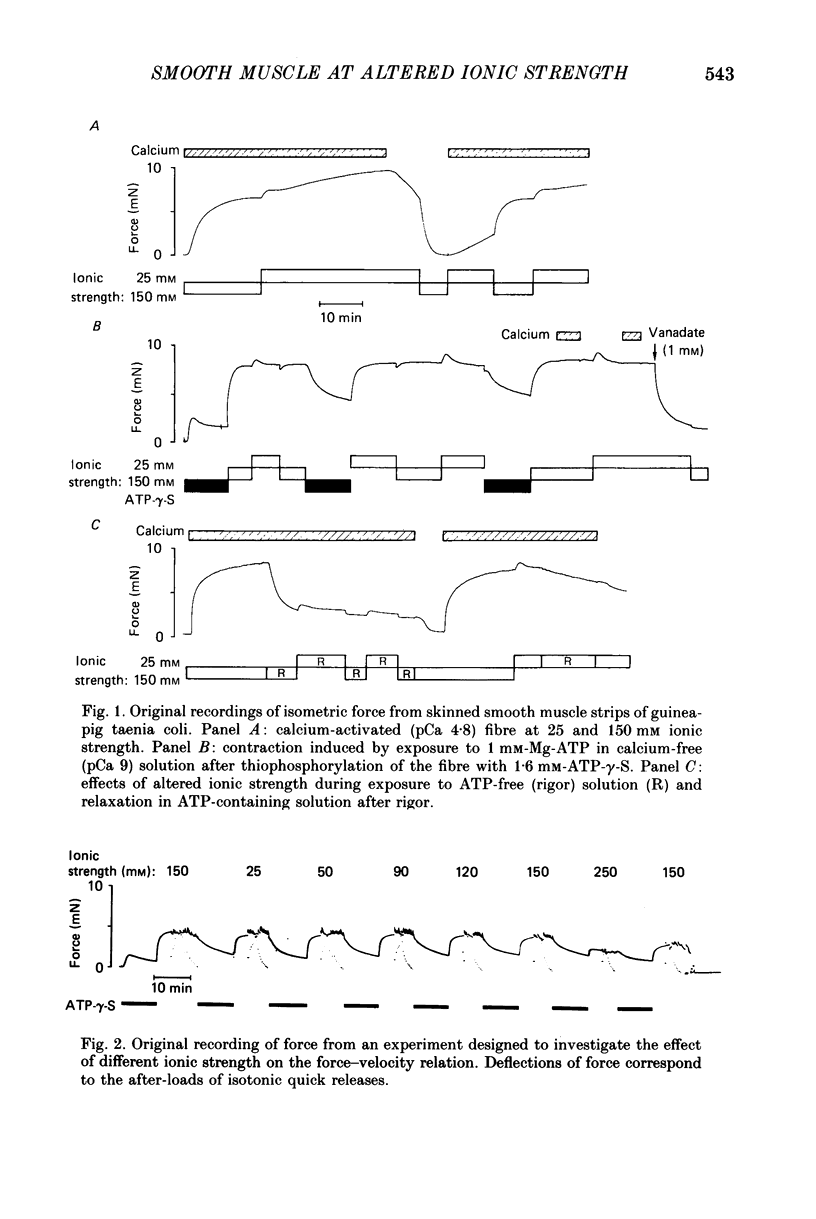
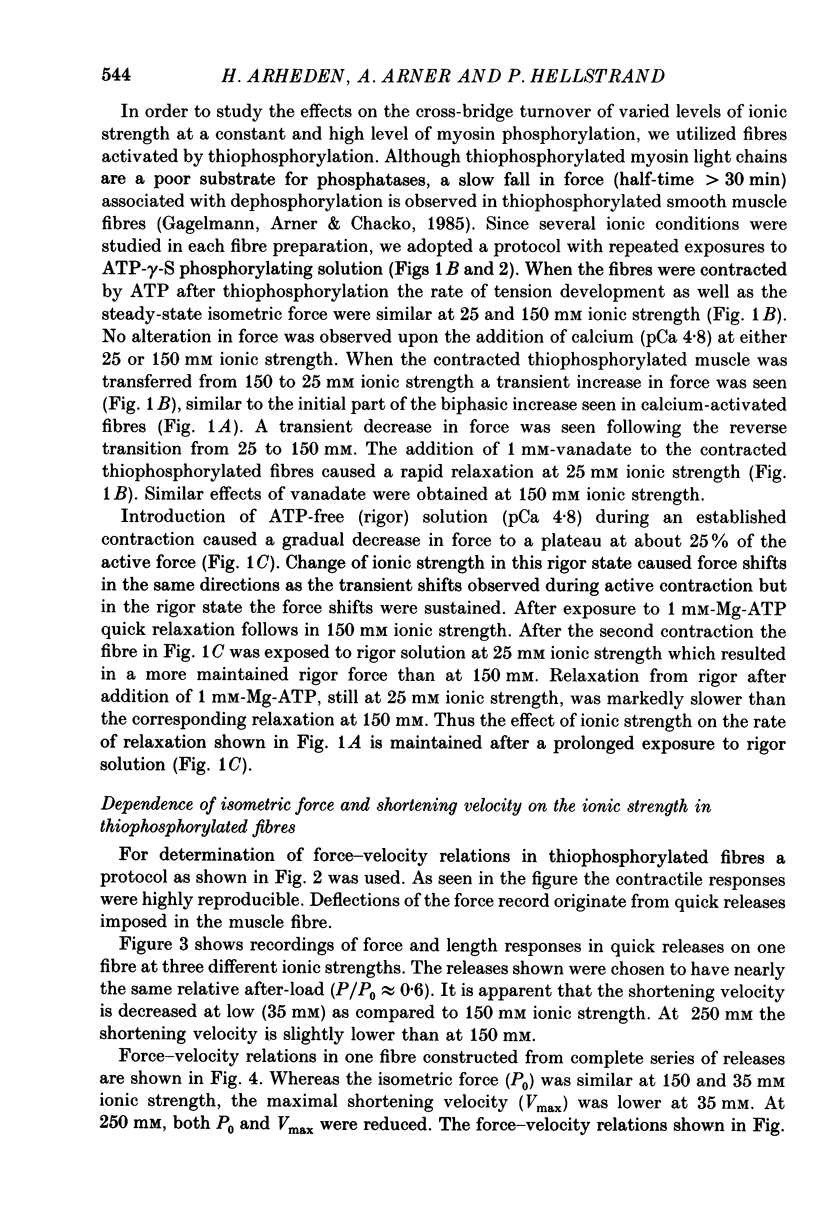
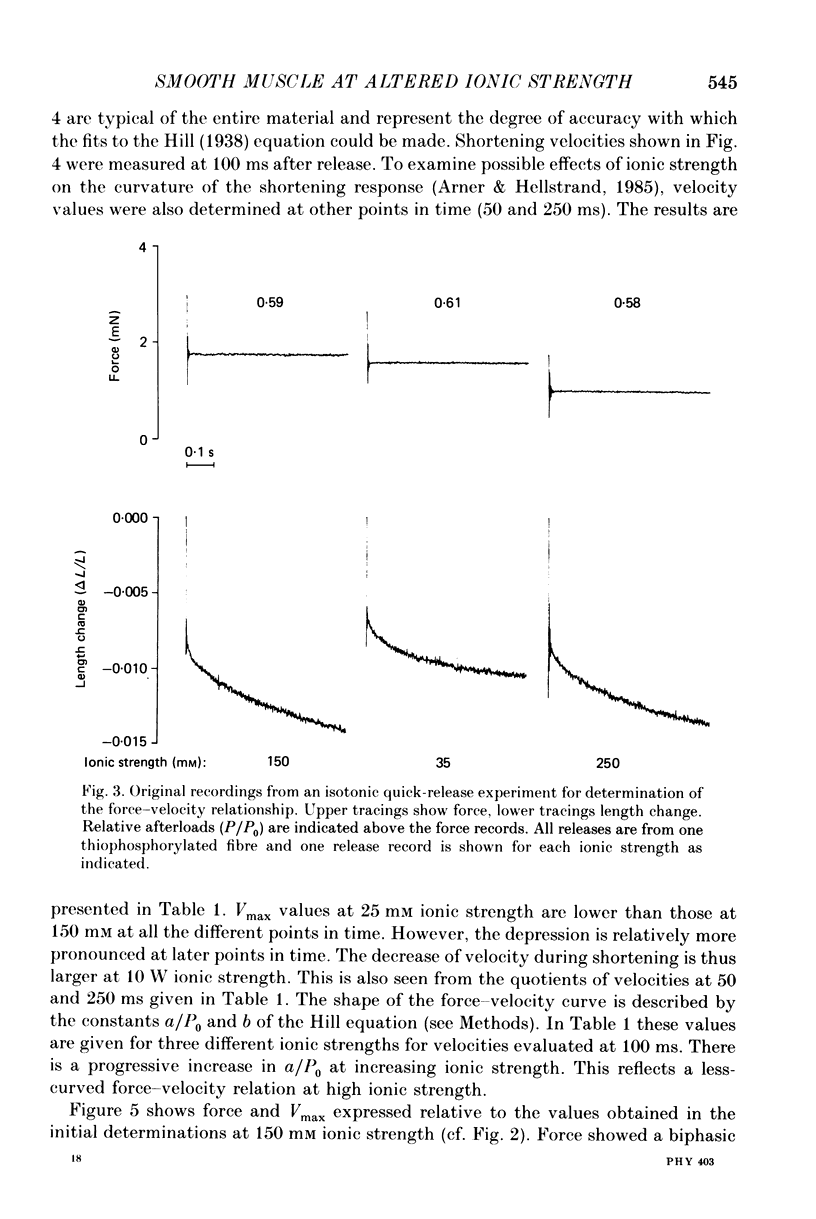
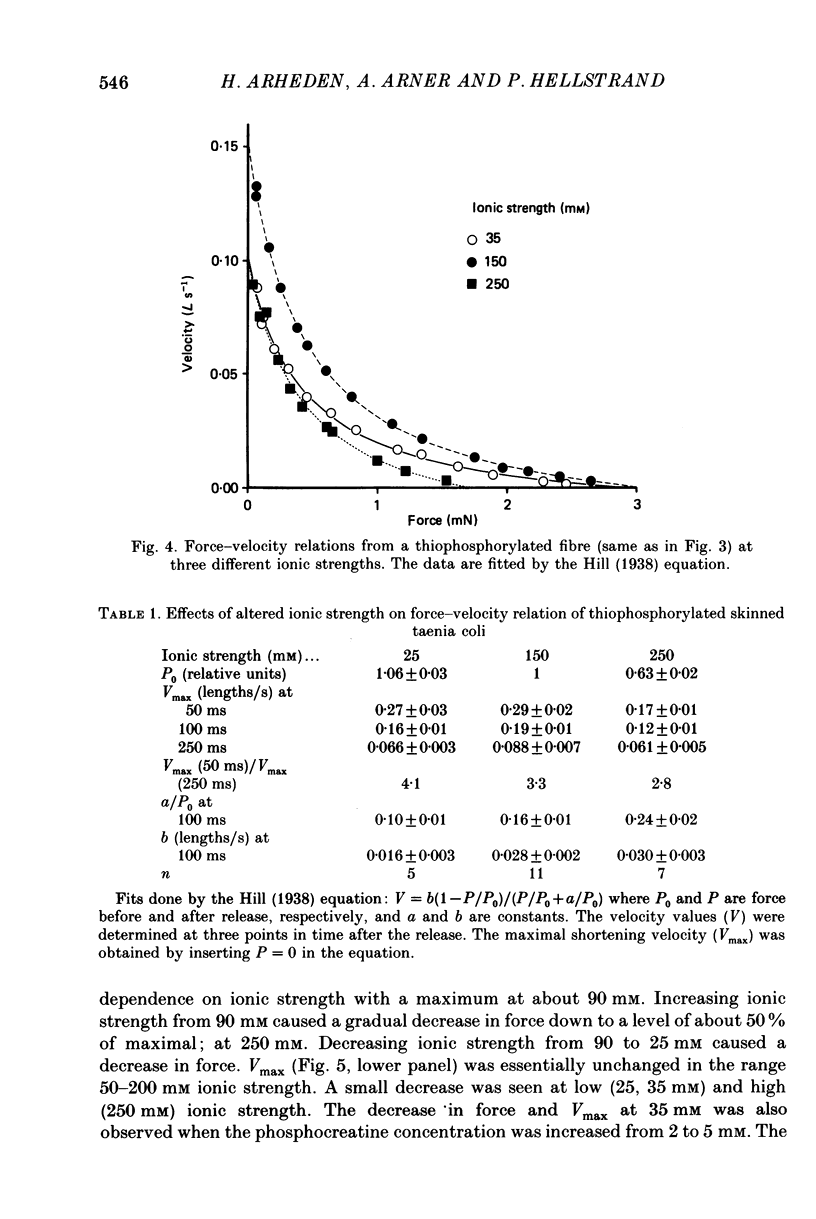
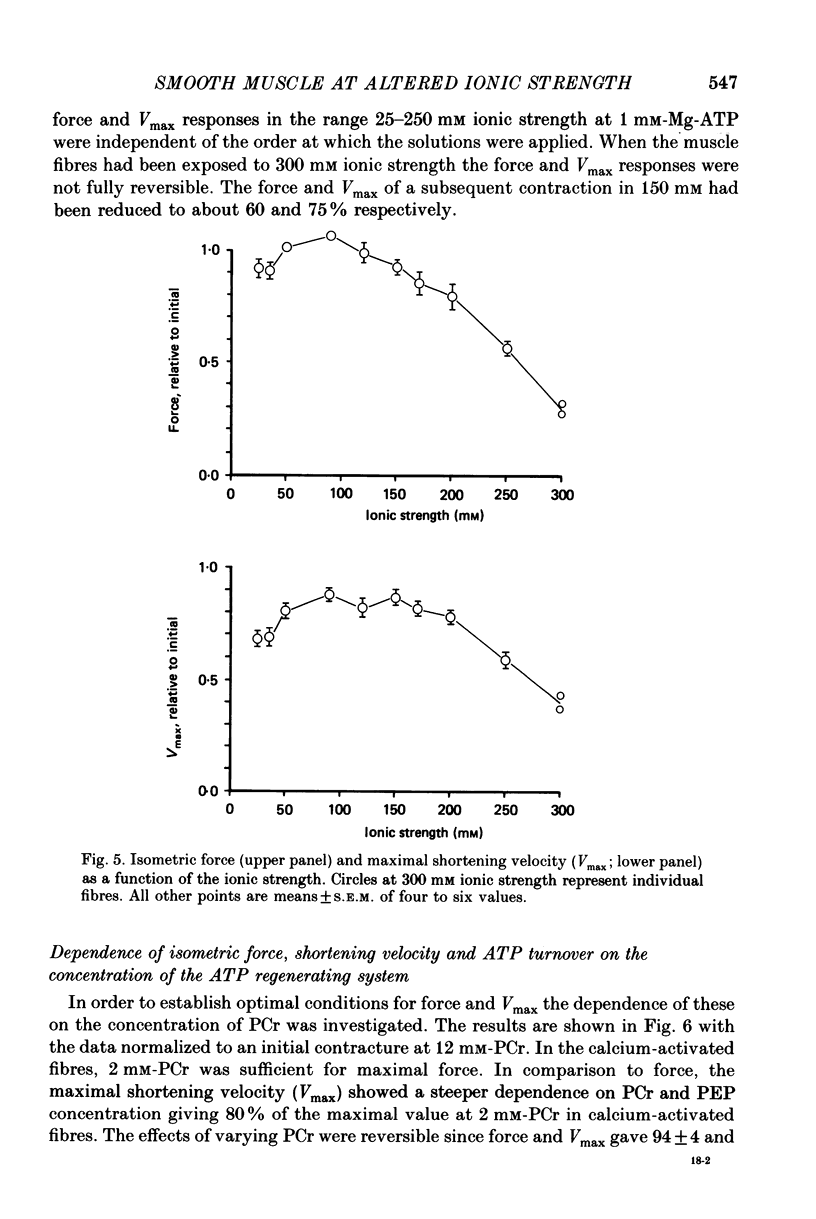
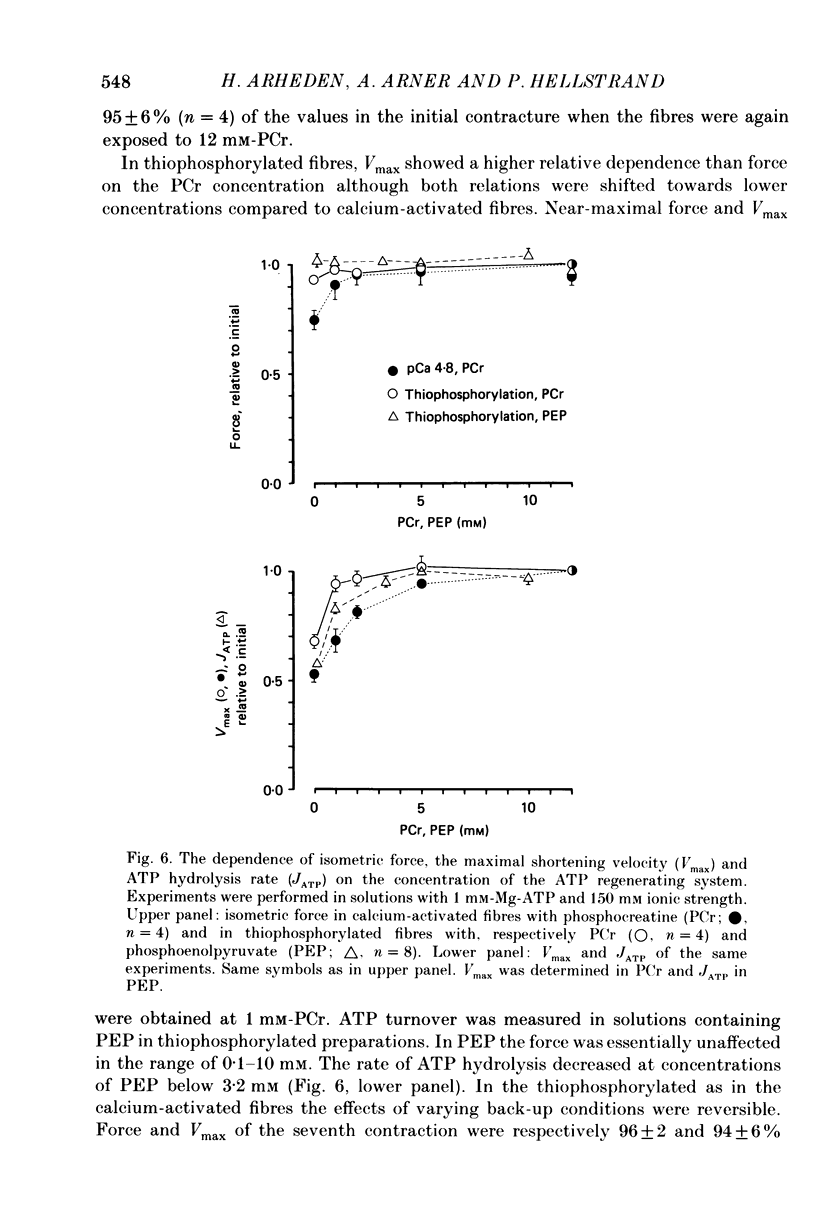
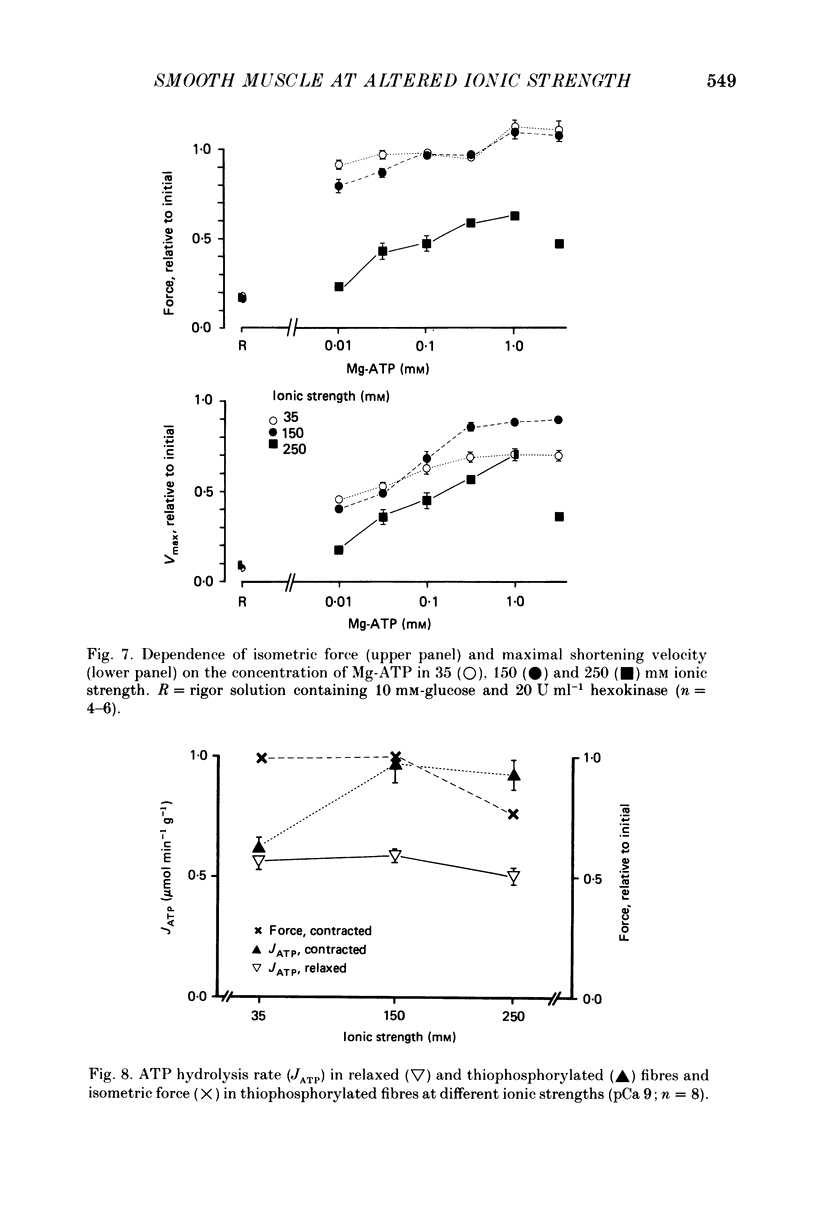
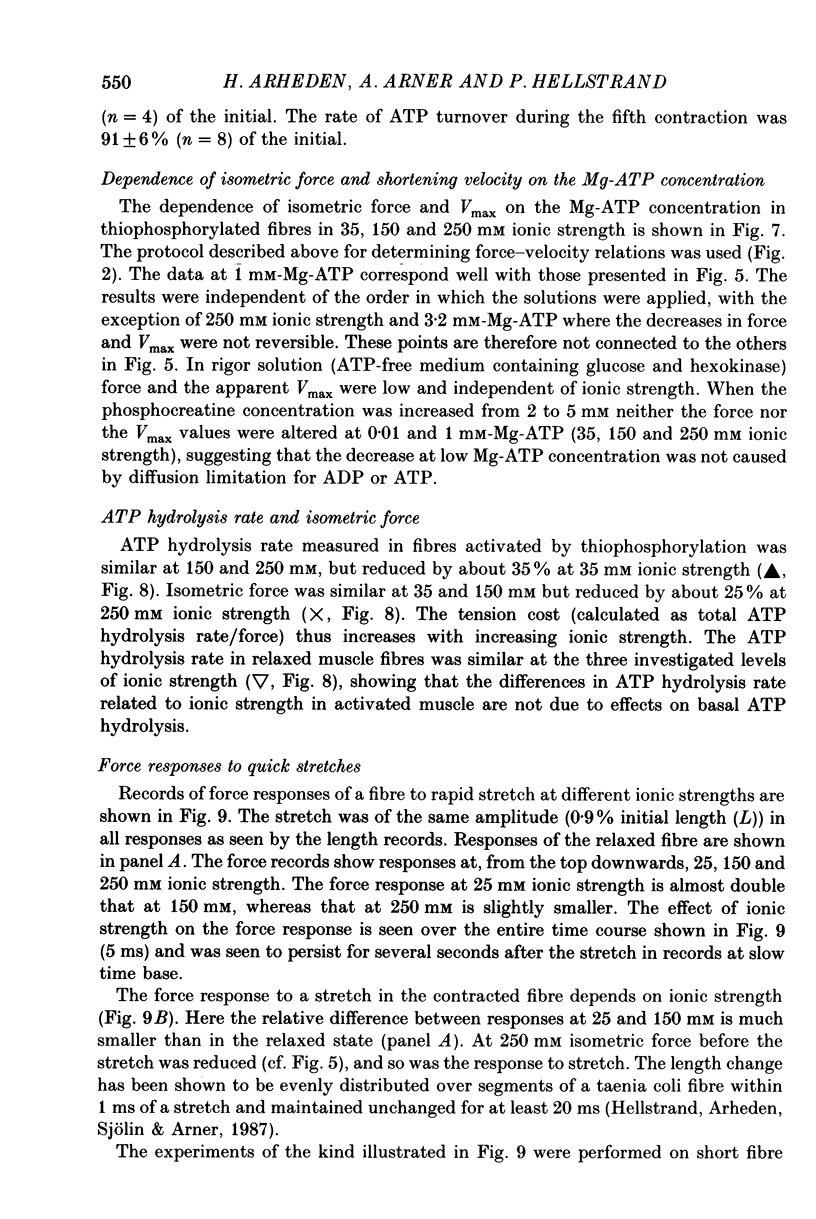
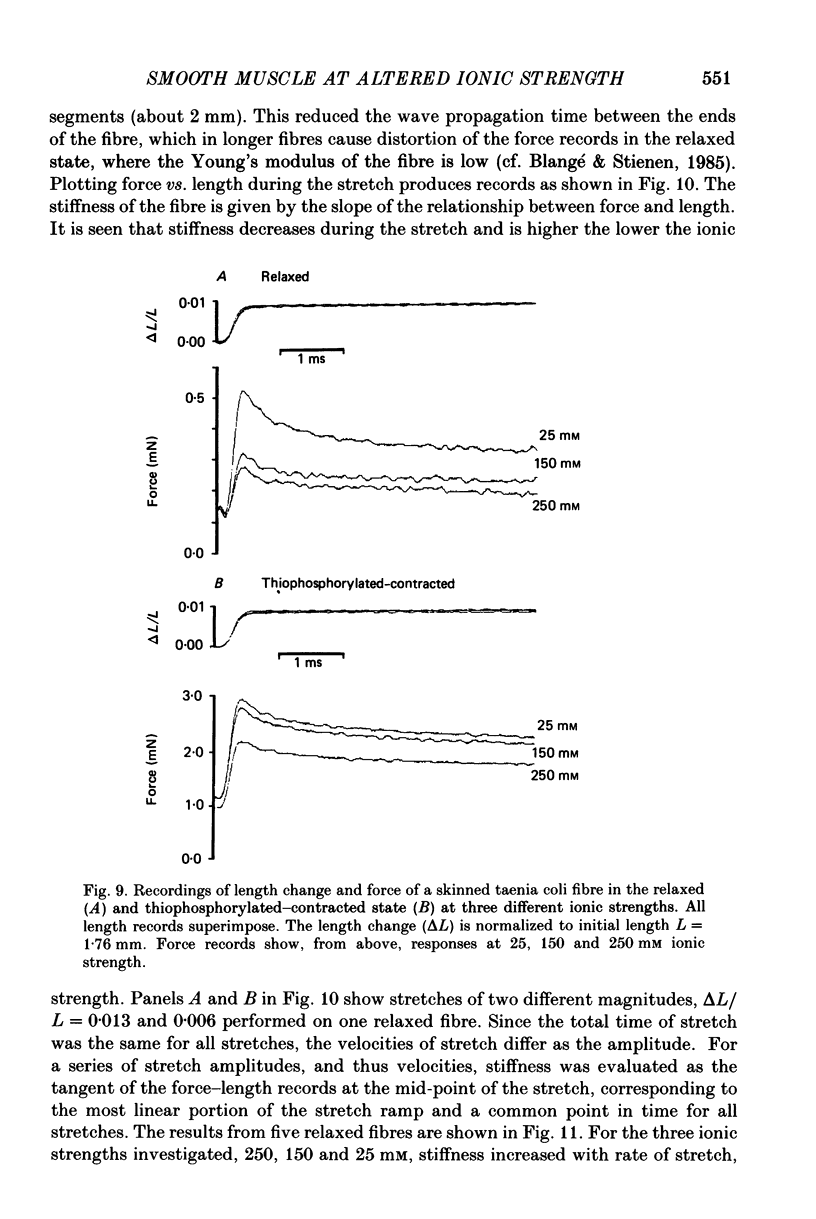
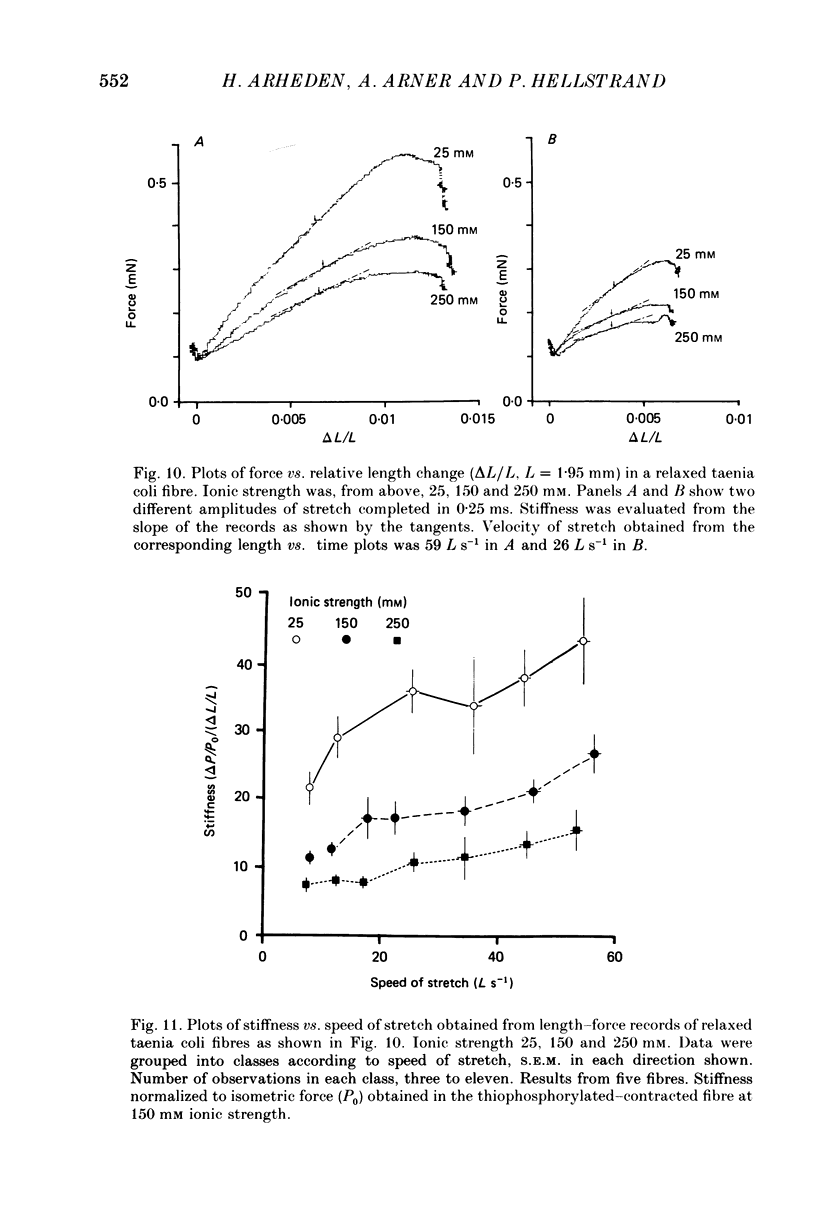
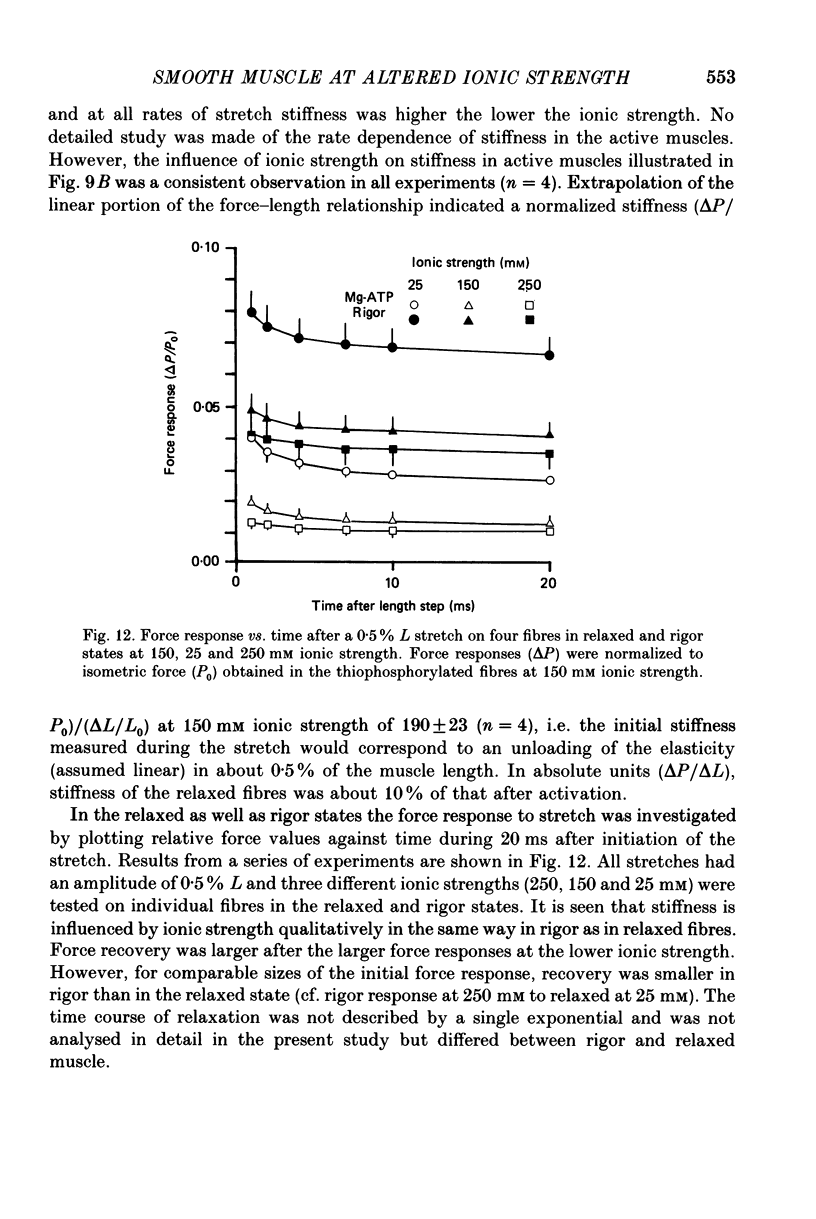
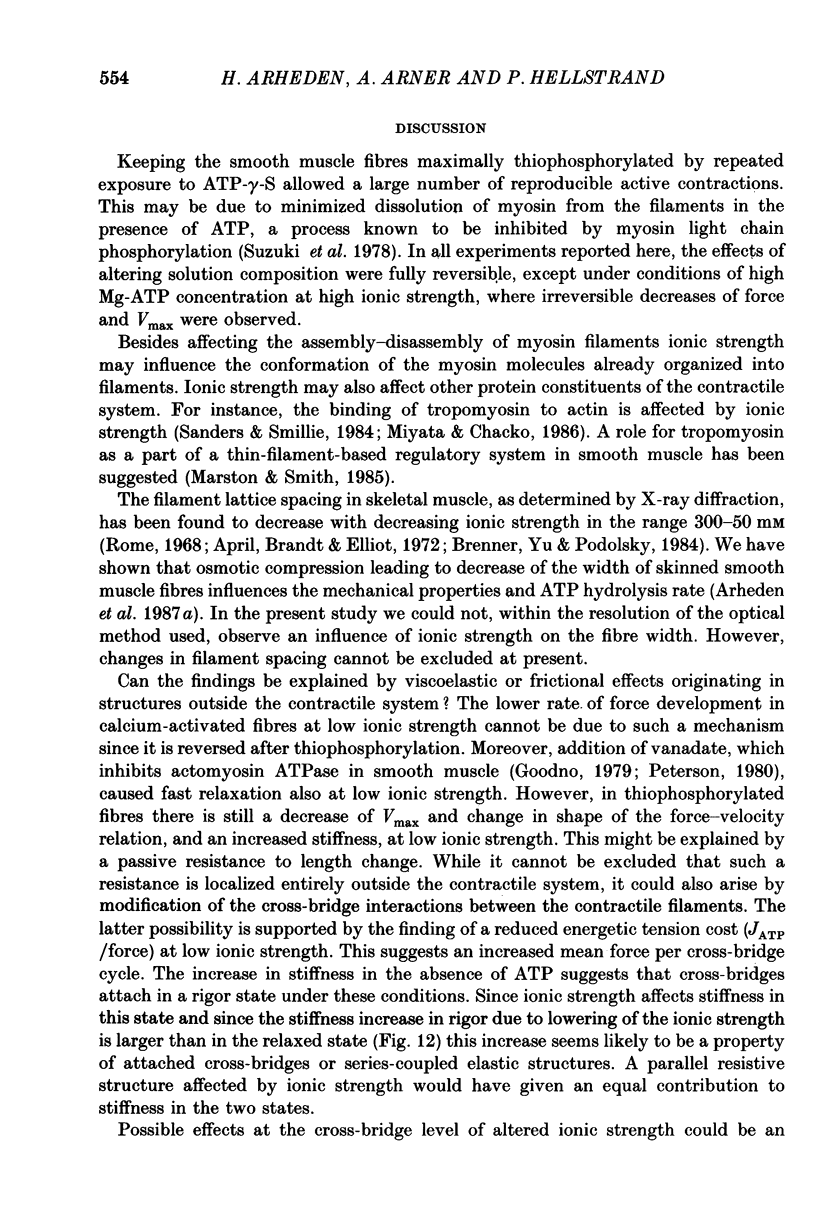
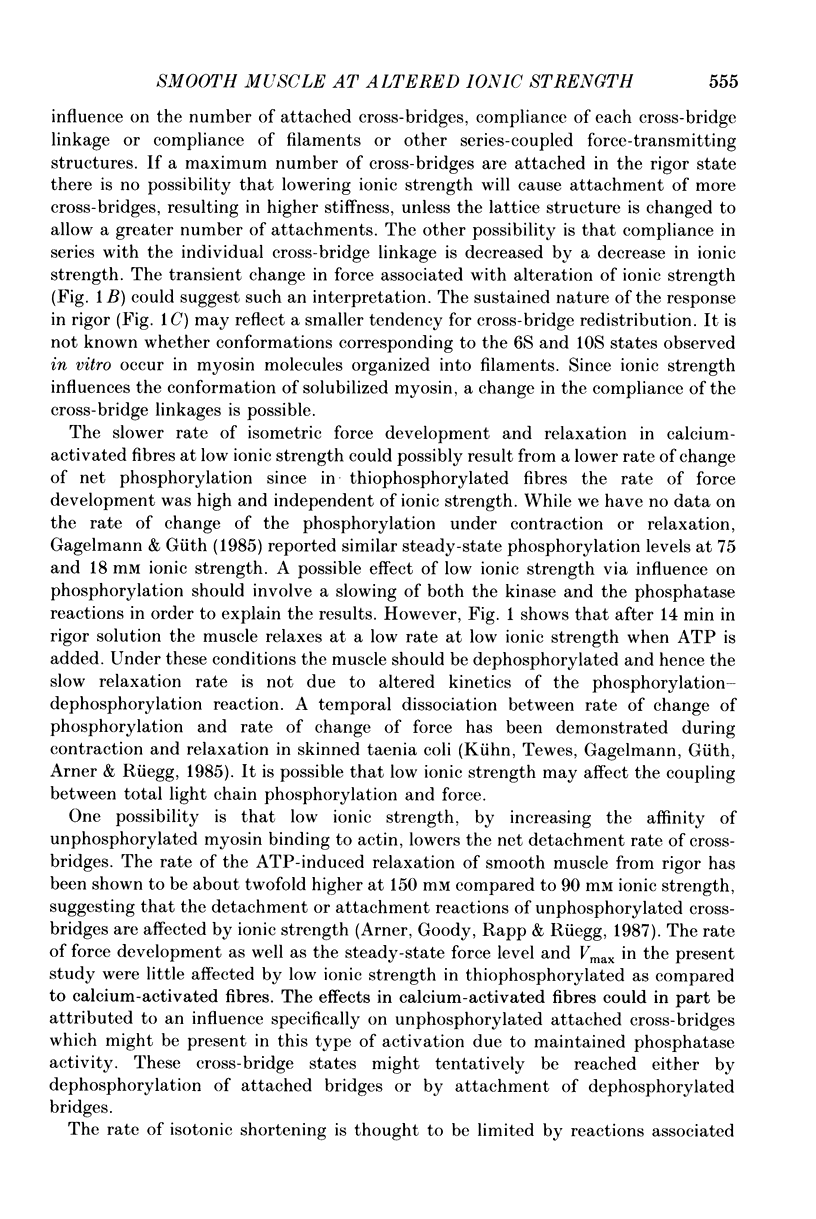
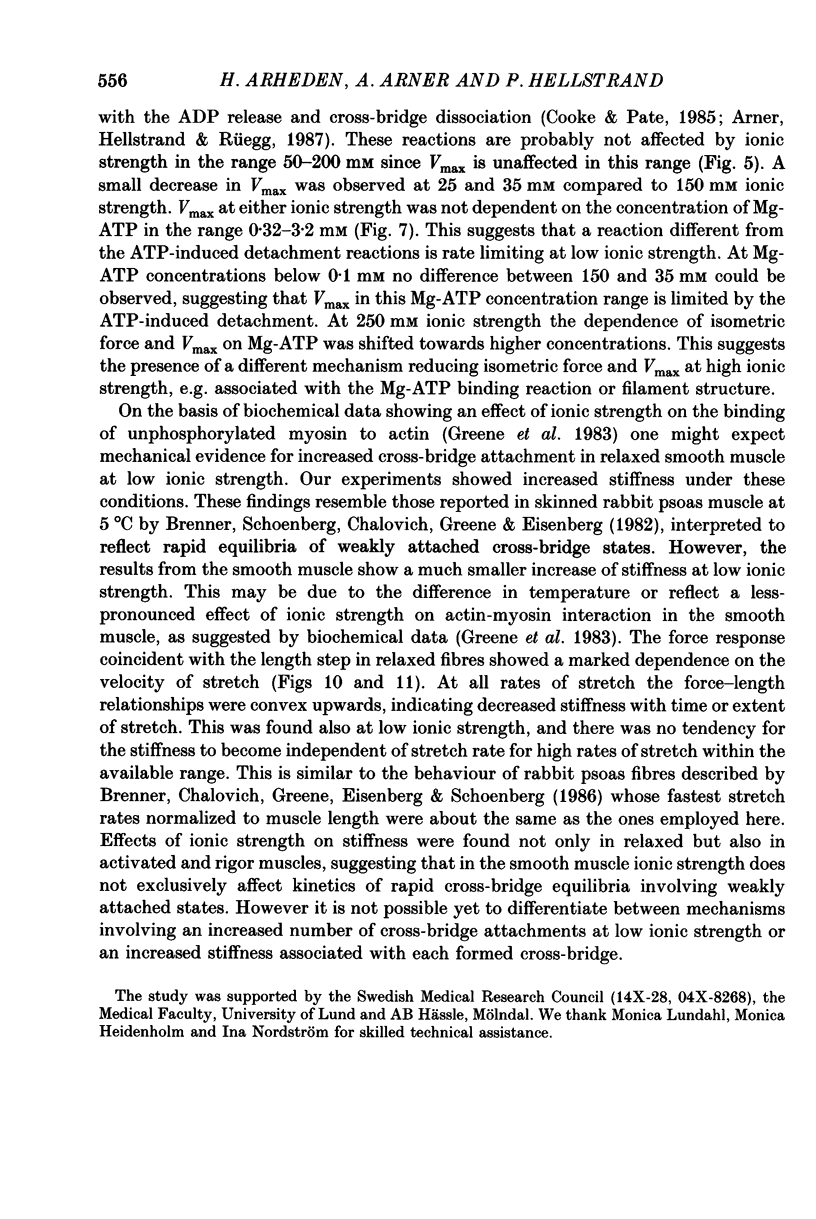
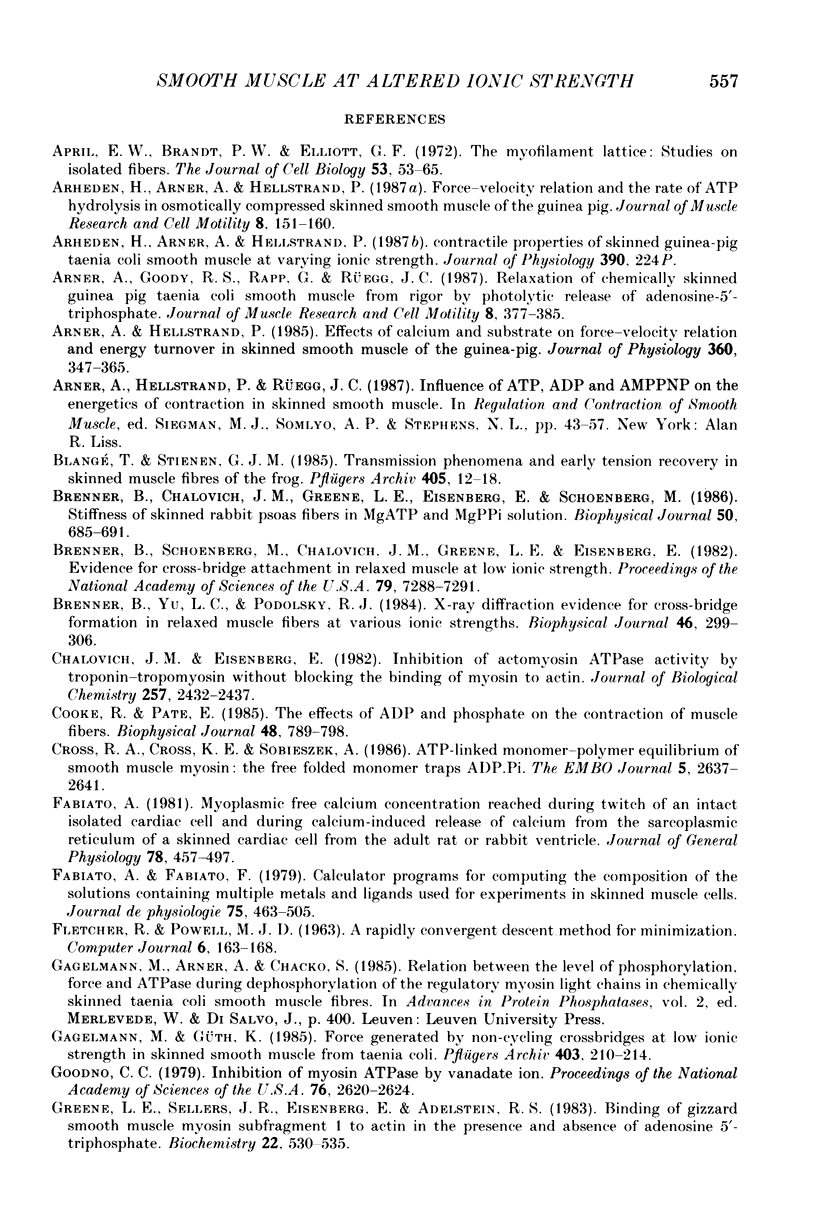
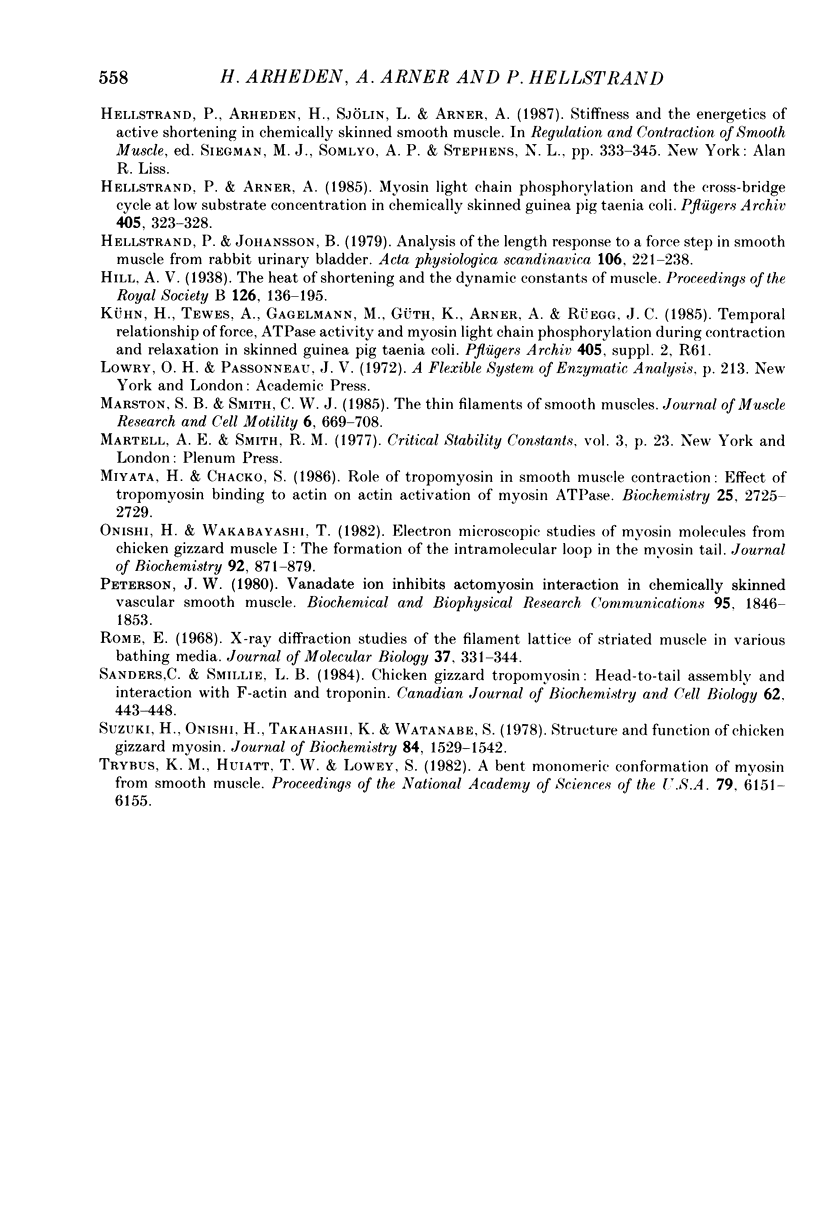
Selected References
These references are in PubMed. This may not be the complete list of references from this article.
- April E. W., Brandt P. W., Elliott G. F. The myofilament lattice: studies on isolated fibers. II. The effects of osmotic strength, ionic concentration, and pH upon the unit-cell volume. J Cell Biol. 1972 Apr;53(1):53–65. doi: 10.1083/jcb.53.1.53. [DOI] [PMC free article] [PubMed] [Google Scholar]
- Arheden H., Arner A., Hellstrand P. Force-velocity relation and rate of ATP hydrolysis in osmotically compressed skinned smooth muscle of the guinea pig. J Muscle Res Cell Motil. 1987 Apr;8(2):151–160. doi: 10.1007/BF01753991. [DOI] [PubMed] [Google Scholar]
- Arner A., Goody R. S., Rapp G., Rüegg J. C. Relaxation of chemically skinned guinea pig taenia coli smooth muscle from rigor by photolytic release of adenosine-5'-triphosphate. J Muscle Res Cell Motil. 1987 Oct;8(5):377–385. doi: 10.1007/BF01578427. [DOI] [PubMed] [Google Scholar]
- Arner A., Hellstrand P. Effects of calcium and substrate on force-velocity relation and energy turnover in skinned smooth muscle of the guinea-pig. J Physiol. 1985 Mar;360:347–365. doi: 10.1113/jphysiol.1985.sp015621. [DOI] [PMC free article] [PubMed] [Google Scholar]
- Arner A., Hellstrand P., Rüegg J. C. Influence of ATP, ADP and AMPPNP on the energetics of contraction in skinned smooth muscle. Prog Clin Biol Res. 1987;245:43–57. [PubMed] [Google Scholar]
- Blangé T., Stienen G. J. Transmission phenomena and early tension recovery in skinned muscle fibres of the frog. Pflugers Arch. 1985 Sep;405(1):12–18. doi: 10.1007/BF00591091. [DOI] [PubMed] [Google Scholar]
- Brenner B., Chalovich J. M., Greene L. E., Eisenberg E., Schoenberg M. Stiffness of skinned rabbit psoas fibers in MgATP and MgPPi solution. Biophys J. 1986 Oct;50(4):685–691. doi: 10.1016/S0006-3495(86)83509-3. [DOI] [PMC free article] [PubMed] [Google Scholar]
- Brenner B., Schoenberg M., Chalovich J. M., Greene L. E., Eisenberg E. Evidence for cross-bridge attachment in relaxed muscle at low ionic strength. Proc Natl Acad Sci U S A. 1982 Dec;79(23):7288–7291. doi: 10.1073/pnas.79.23.7288. [DOI] [PMC free article] [PubMed] [Google Scholar]
- Brenner B., Yu L. C., Podolsky R. J. X-ray diffraction evidence for cross-bridge formation in relaxed muscle fibers at various ionic strengths. Biophys J. 1984 Sep;46(3):299–306. doi: 10.1016/S0006-3495(84)84026-6. [DOI] [PMC free article] [PubMed] [Google Scholar]
- Chalovich J. M., Eisenberg E. Inhibition of actomyosin ATPase activity by troponin-tropomyosin without blocking the binding of myosin to actin. J Biol Chem. 1982 Mar 10;257(5):2432–2437. [PMC free article] [PubMed] [Google Scholar]
- Cooke R., Pate E. The effects of ADP and phosphate on the contraction of muscle fibers. Biophys J. 1985 Nov;48(5):789–798. doi: 10.1016/S0006-3495(85)83837-6. [DOI] [PMC free article] [PubMed] [Google Scholar]
- Cross R. A., Cross K. E., Sobieszek A. ATP-linked monomer-polymer equilibrium of smooth muscle myosin: the free folded monomer traps ADP.Pi. EMBO J. 1986 Oct;5(10):2637–2641. doi: 10.1002/j.1460-2075.1986.tb04545.x. [DOI] [PMC free article] [PubMed] [Google Scholar]
- Fabiato A., Fabiato F. Calculator programs for computing the composition of the solutions containing multiple metals and ligands used for experiments in skinned muscle cells. J Physiol (Paris) 1979;75(5):463–505. [PubMed] [Google Scholar]
- Fabiato A. Myoplasmic free calcium concentration reached during the twitch of an intact isolated cardiac cell and during calcium-induced release of calcium from the sarcoplasmic reticulum of a skinned cardiac cell from the adult rat or rabbit ventricle. J Gen Physiol. 1981 Nov;78(5):457–497. doi: 10.1085/jgp.78.5.457. [DOI] [PMC free article] [PubMed] [Google Scholar]
- Gagelmann M., Güth K. Force generated by non-cycling crossbridges at low ionic strength in skinned smooth muscle from Taenia coli. Pflugers Arch. 1985 Feb;403(2):210–214. doi: 10.1007/BF00584102. [DOI] [PubMed] [Google Scholar]
- Goodno C. C. Inhibition of myosin ATPase by vanadate ion. Proc Natl Acad Sci U S A. 1979 Jun;76(6):2620–2624. doi: 10.1073/pnas.76.6.2620. [DOI] [PMC free article] [PubMed] [Google Scholar]
- Greene L. E., Sellers J. R., Eisenberg E., Adelstein R. S. Binding of gizzard smooth muscle myosin subfragment 1 to actin in the presence and absence of adenosine 5'-triphosphate. Biochemistry. 1983 Feb 1;22(3):530–535. doi: 10.1021/bi00272a002. [DOI] [PubMed] [Google Scholar]
- Hellstrand P., Arheden H., Sjölin L., Arner A. Stiffness and the energetics of active shortening in chemically skinned smooth muscle. Prog Clin Biol Res. 1987;245:333–345. [PubMed] [Google Scholar]
- Hellstrand P., Arner A. Myosin light chain phosphorylation and the cross-bridge cycle at low substrate concentration in chemically skinned guinea pig Taenia coli. Pflugers Arch. 1985 Dec;405(4):323–328. doi: 10.1007/BF00595684. [DOI] [PubMed] [Google Scholar]
- Hellstrand P., Johansson B. Analysis of the length response to a force step in smooth muscle from rabbit urinary bladder. Acta Physiol Scand. 1979 Jun;106(2):221–238. doi: 10.1111/j.1748-1716.1979.tb06392.x. [DOI] [PubMed] [Google Scholar]
- Marston S. B., Smith C. W. The thin filaments of smooth muscles. J Muscle Res Cell Motil. 1985 Dec;6(6):669–708. doi: 10.1007/BF00712237. [DOI] [PubMed] [Google Scholar]
- Miyata H., Chacko S. Role of tropomyosin in smooth muscle contraction: effect of tropomyosin binding to actin on actin activation of myosin ATPase. Biochemistry. 1986 May 6;25(9):2725–2729. doi: 10.1021/bi00357a067. [DOI] [PubMed] [Google Scholar]
- Onishi H., Wakabayashi T. Electron microscopic studies of myosin molecules from chicken gizzard muscle I: the formation of the intramolecular loop in the myosin tail. J Biochem. 1982 Sep;92(3):871–879. doi: 10.1093/oxfordjournals.jbchem.a134001. [DOI] [PubMed] [Google Scholar]
- Peterson J. W. Vanadate ion inhibits actomyosin interaction in chemically skinned vascular smooth muscle. Biochem Biophys Res Commun. 1980 Aug 29;95(4):1846–1853. doi: 10.1016/s0006-291x(80)80114-8. [DOI] [PubMed] [Google Scholar]
- Rome E. X-ray diffraction studies of the filament lattice of striated muscle in various bathing media. J Mol Biol. 1968 Oct 28;37(2):331–344. doi: 10.1016/0022-2836(68)90272-6. [DOI] [PubMed] [Google Scholar]
- Sanders C., Smillie L. B. Chicken gizzard tropomyosin: head-to-tail assembly and interaction with F-actin and troponin. Can J Biochem Cell Biol. 1984 Jun;62(6):443–448. doi: 10.1139/o84-060. [DOI] [PubMed] [Google Scholar]
- Suzuki H., Onishi H., Takahashi K., Watanabe S. Structure and function of chicken gizzard myosin. J Biochem. 1978 Dec;84(6):1529–1542. doi: 10.1093/oxfordjournals.jbchem.a132278. [DOI] [PubMed] [Google Scholar]
- Trybus K. M., Huiatt T. W., Lowey S. A bent monomeric conformation of myosin from smooth muscle. Proc Natl Acad Sci U S A. 1982 Oct;79(20):6151–6155. doi: 10.1073/pnas.79.20.6151. [DOI] [PMC free article] [PubMed] [Google Scholar]