Abstract
1. The turtle spinal cord produces three forms of the fictive scratch reflex in response to tactile stimulation of sites on the body surface. Common to all three forms is the rhythmic alternation of activity between hip protractor and hip retractor motoneurones. Hip protractor motoneurone activity is monitored via nerves innervating the hip protractor muscle puboischiofemoralis internus pars anteroventralis (VP-HP). Hip retractor activity is monitored via nerves innervating several monoarticular hip retractor muscles, one hip adductor muscle, and several biarticular hip retractor-knee flexor muscles (HR-KF). Each form is characterized by the timing of activity of motoneurones innervating femorotibialis (FT-KE), a monoarticular knee extensor muscle, within this alternating cycle (Robertson, Mortin, Keifer & Stein, 1985). In the present study, intracellular recordings revealed a corresponding regulation of synaptic drive to knee extensor motoneurones with respect to the synaptic drive to the motoneurones innervating antagonist muscles of the hip. These patterns of synaptic activation give rise to the distinct motor pattern underlying each form of the scratch reflex. 2. VP-HP, HR-KF and FT-KE motoneurones all exhibited phasic depolarizing and hyperpolarizing changes in membrane voltage during the production of the rhythmic motor patterns underlying each stratch form. Membrane depolarization is caused by synaptic excitation (EPSPs) and gives rise to motoneurone discharge. Hyperpolarization is primarily the result of postsynaptic inhibition (IPSPs) mediated by an increased conductance of chloride ions (Cl-) and ensures motor pool quiescence during antagonist activation. 3. VP-HP motoneurones depolarized during activation of the VP-HP motor pool and hyperpolarized during activation of the HR-KF motor pool. HR-KF motoneurones depolarized during activation of the HR-KF motor pool and hyperpolarized during activation of the VP-HP motor pool. In many cases, the amplitude of hyperpolarization was directly related to the intensity of the antagonist motor pool burst. During the rostral scratch, HR-KF motor pool activity was sometimes deleted, along with the depolarizing wave in HR-KF motoneurones and the hyperpolarizing wave in VP-HP motoneurones. The interneurones providing the synaptic drive to these antagonist motoneurones appear, therefore, to have reciprocal activation patterns. 4. FT-KE motoneurones depolarized during FT-KE motor pool activation and hyperpolarized during FT-KE motor pool quiescence. This alternation of opposing synaptic drive underlies the rhythmic activation of the FT-KE motor pool during all scratch forms.(ABSTRACT TRUNCATED AT 400 WORDS)
Full text
PDF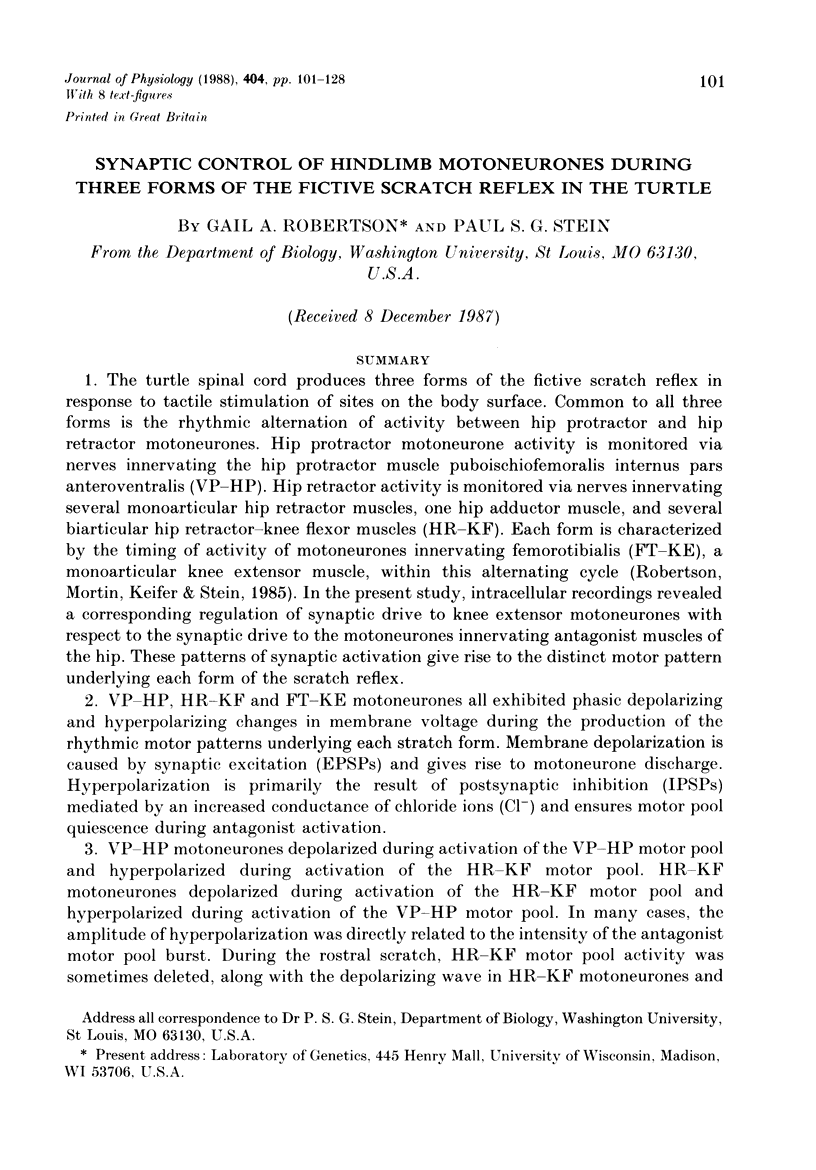
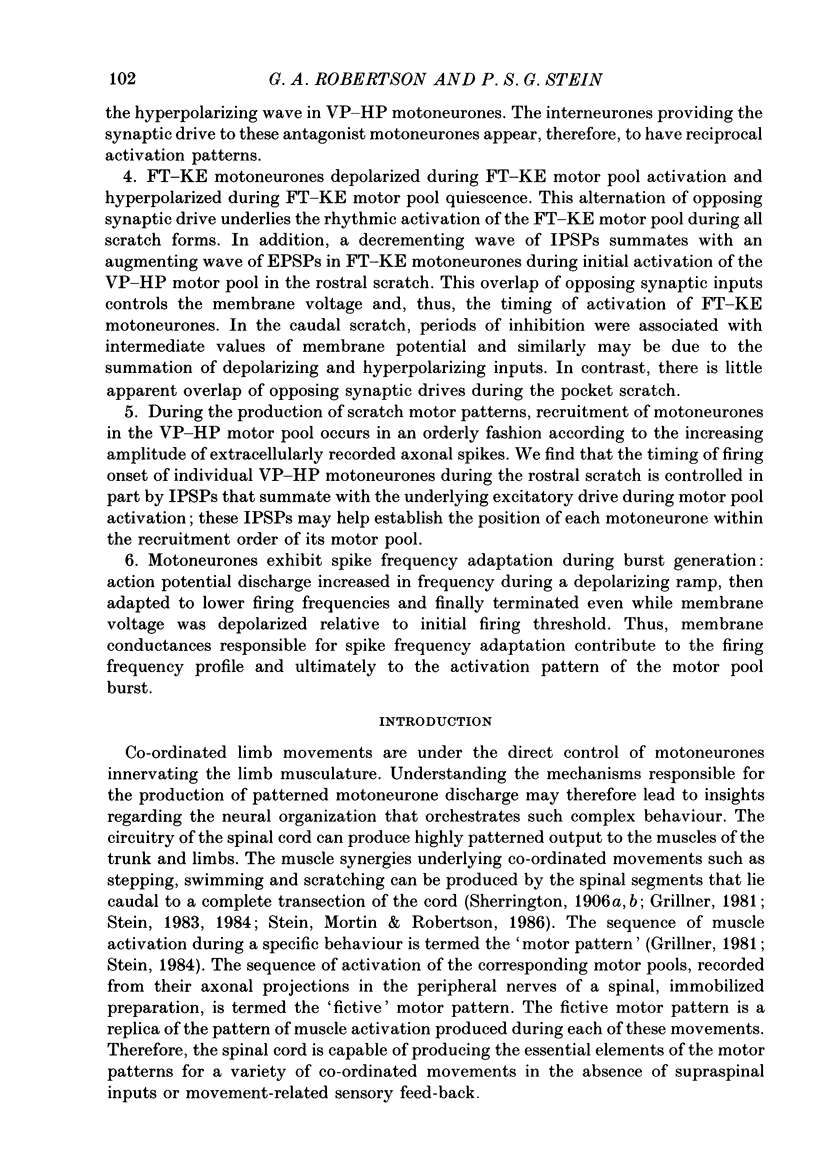
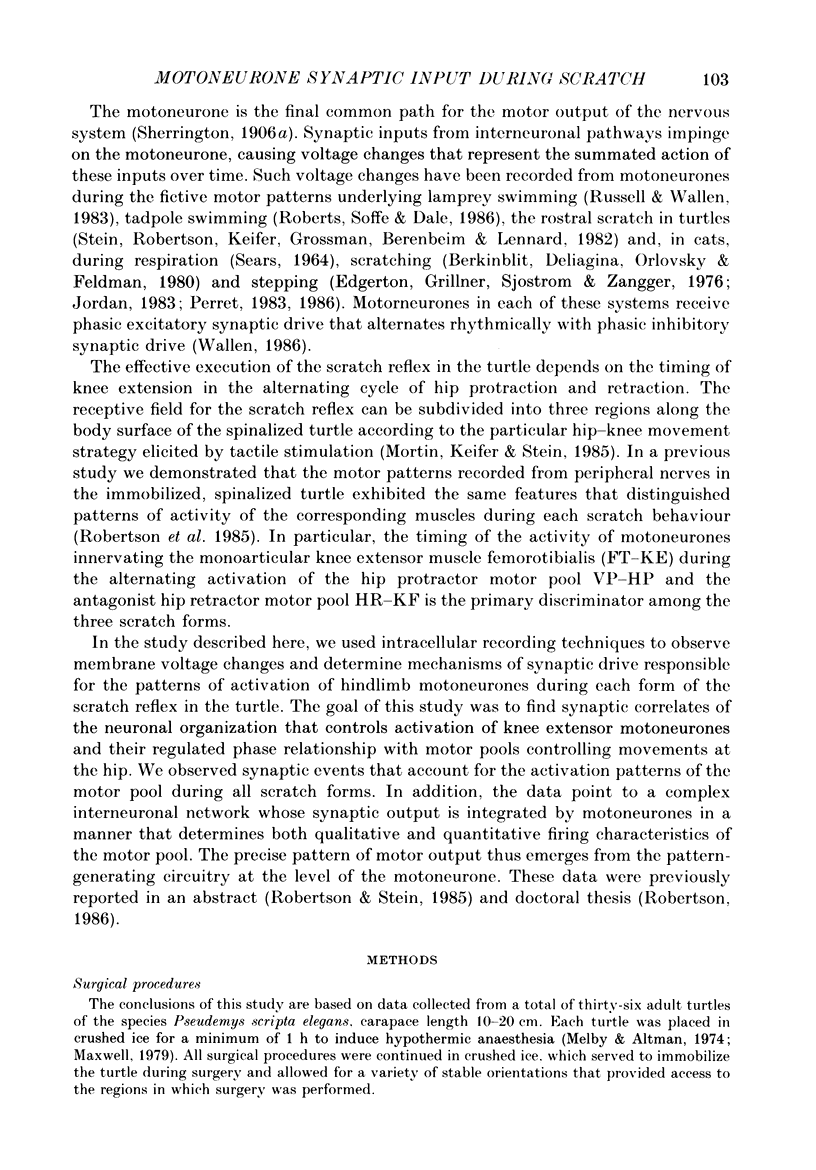
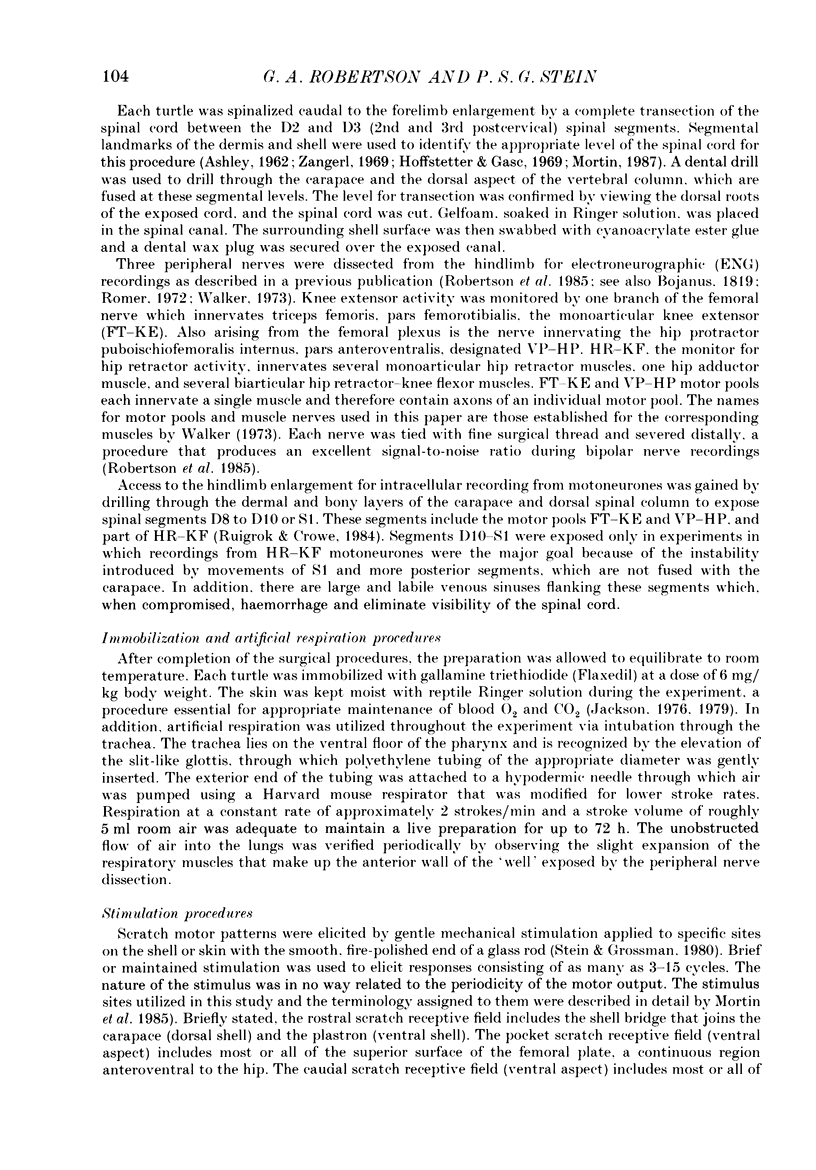
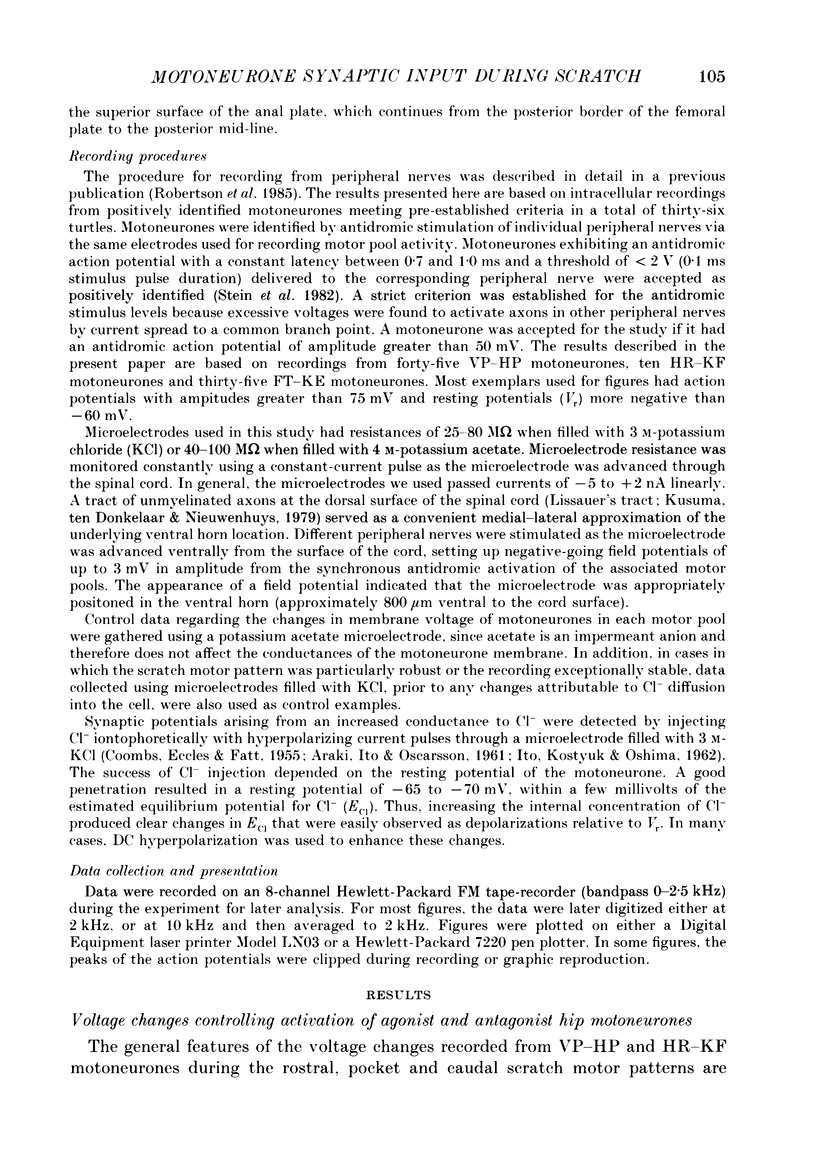
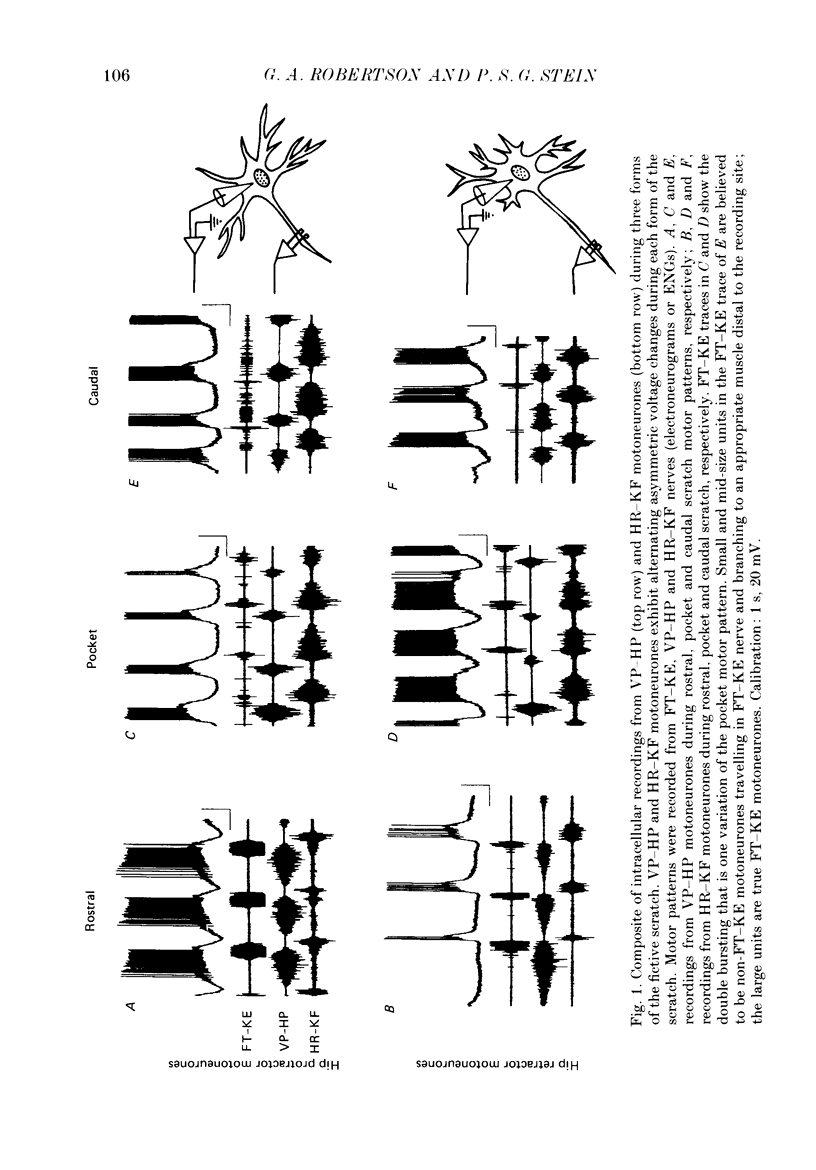
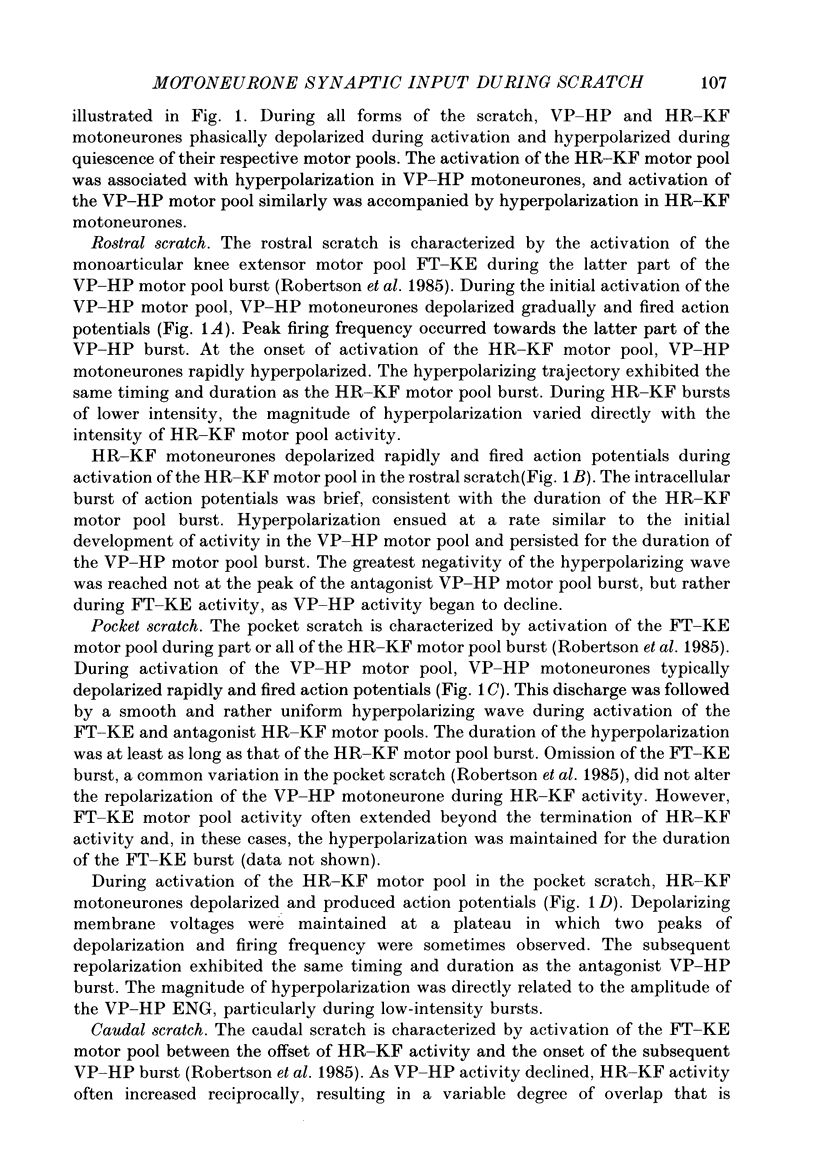
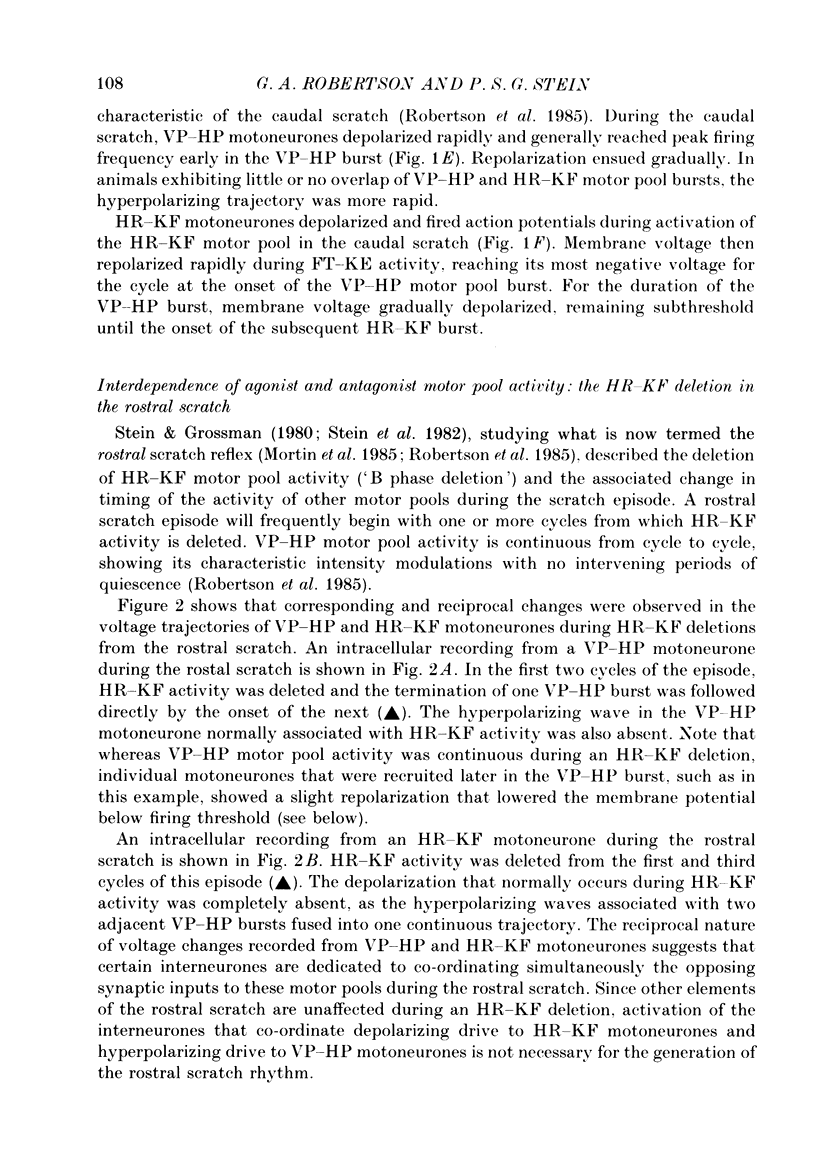
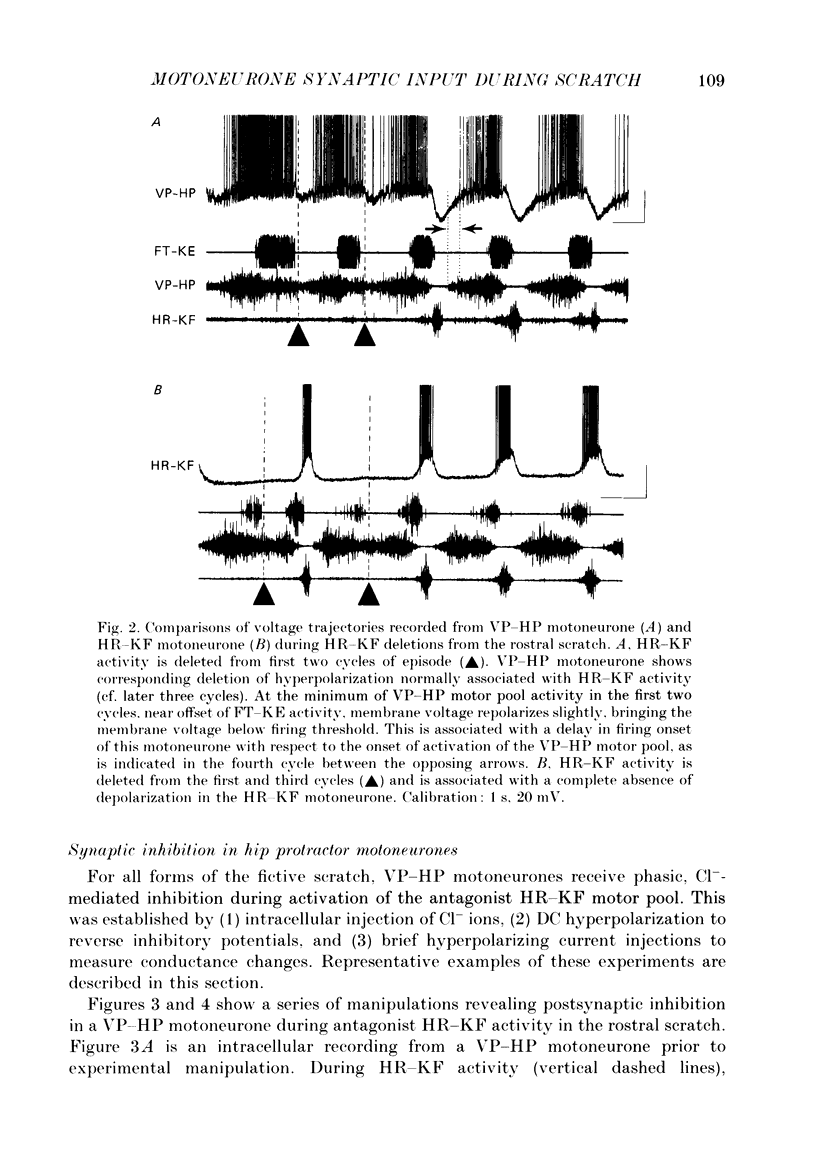
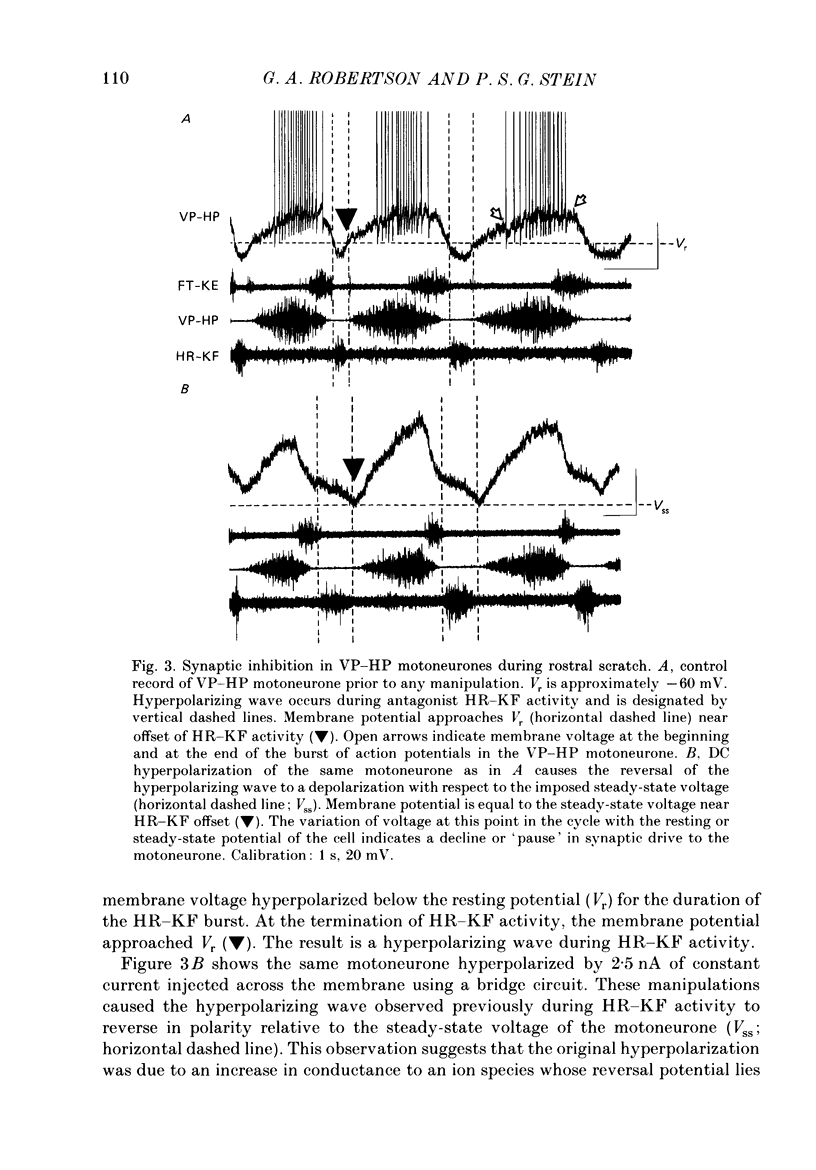
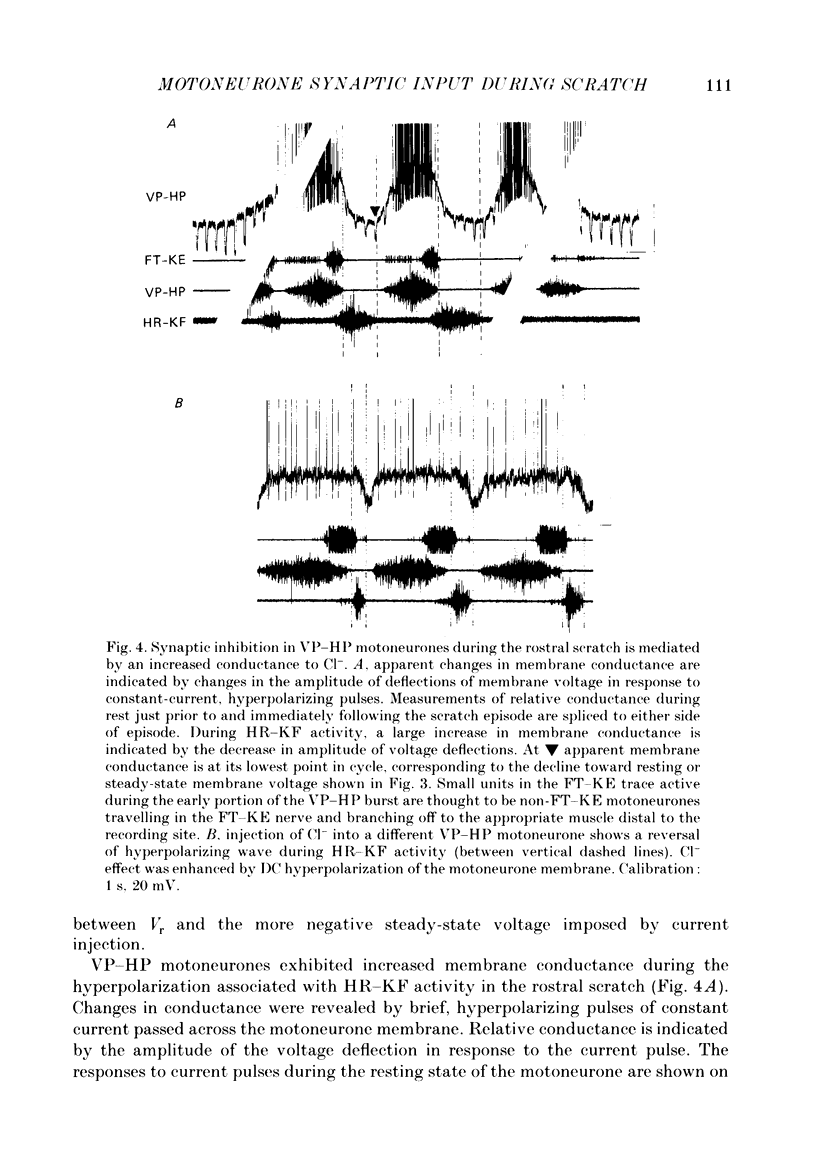
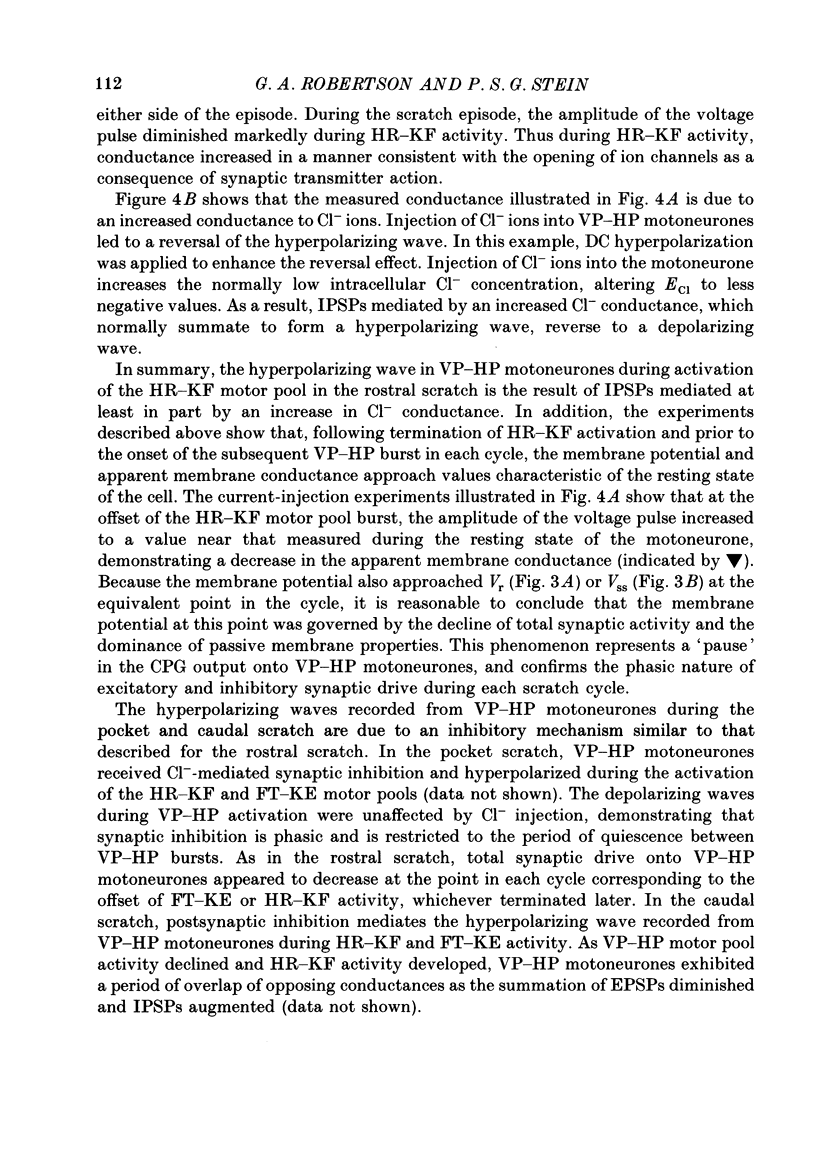
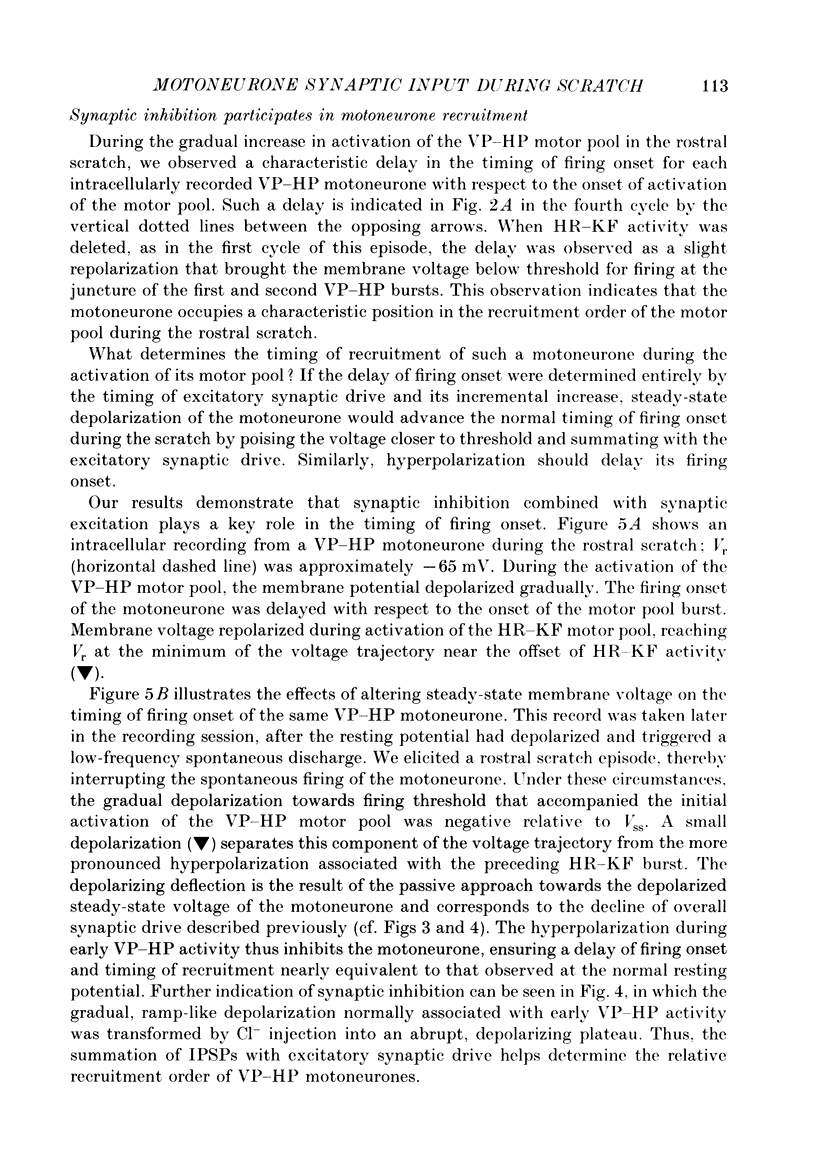
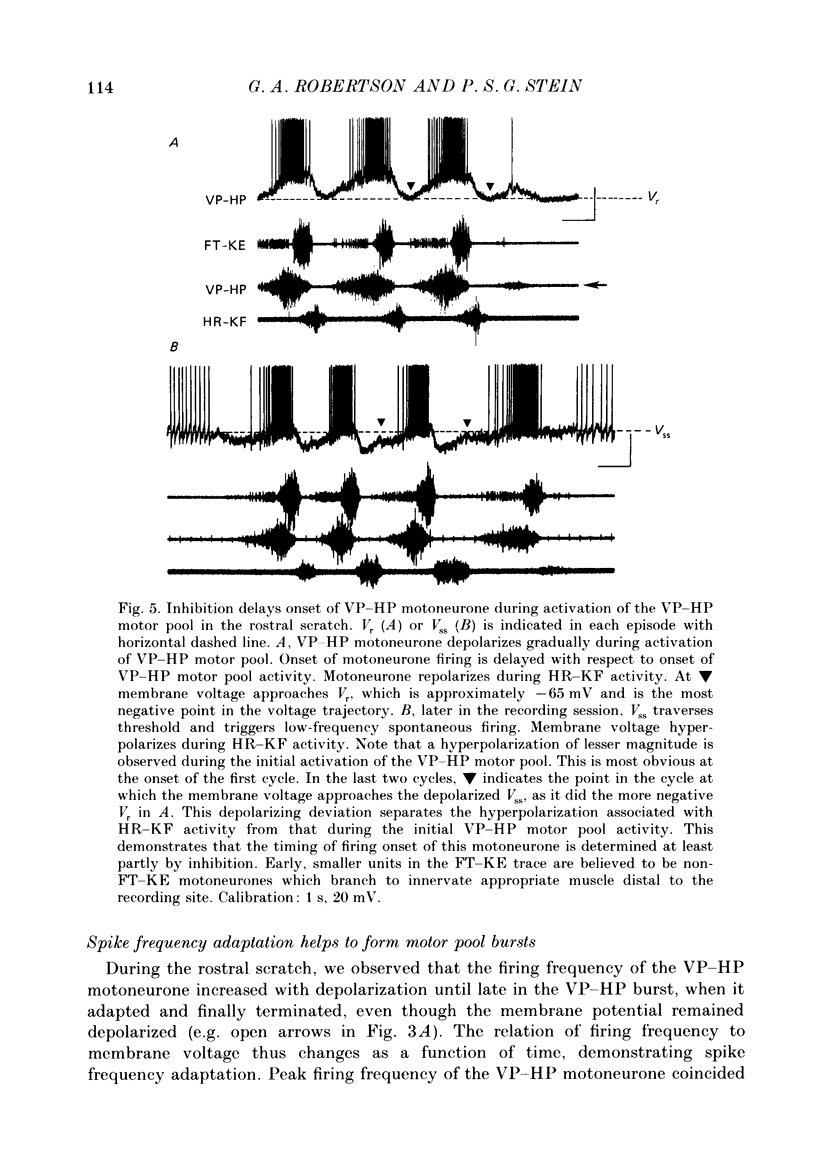
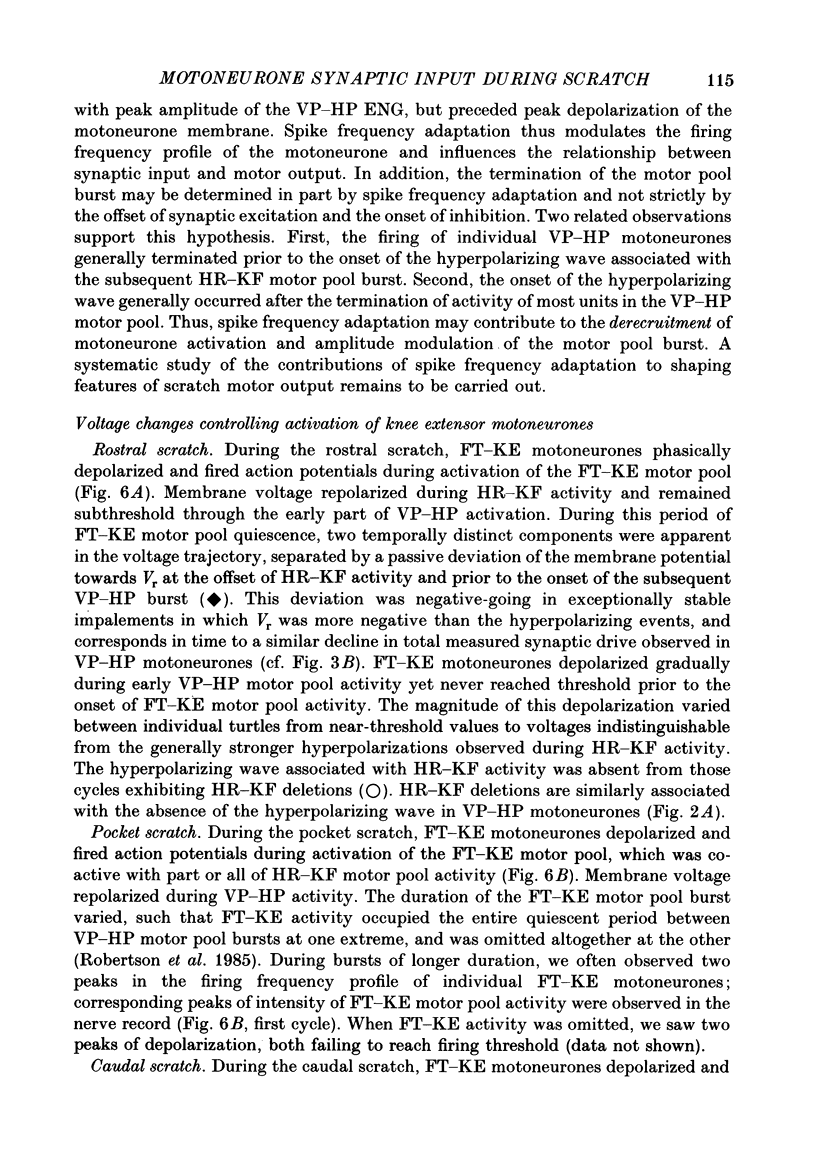
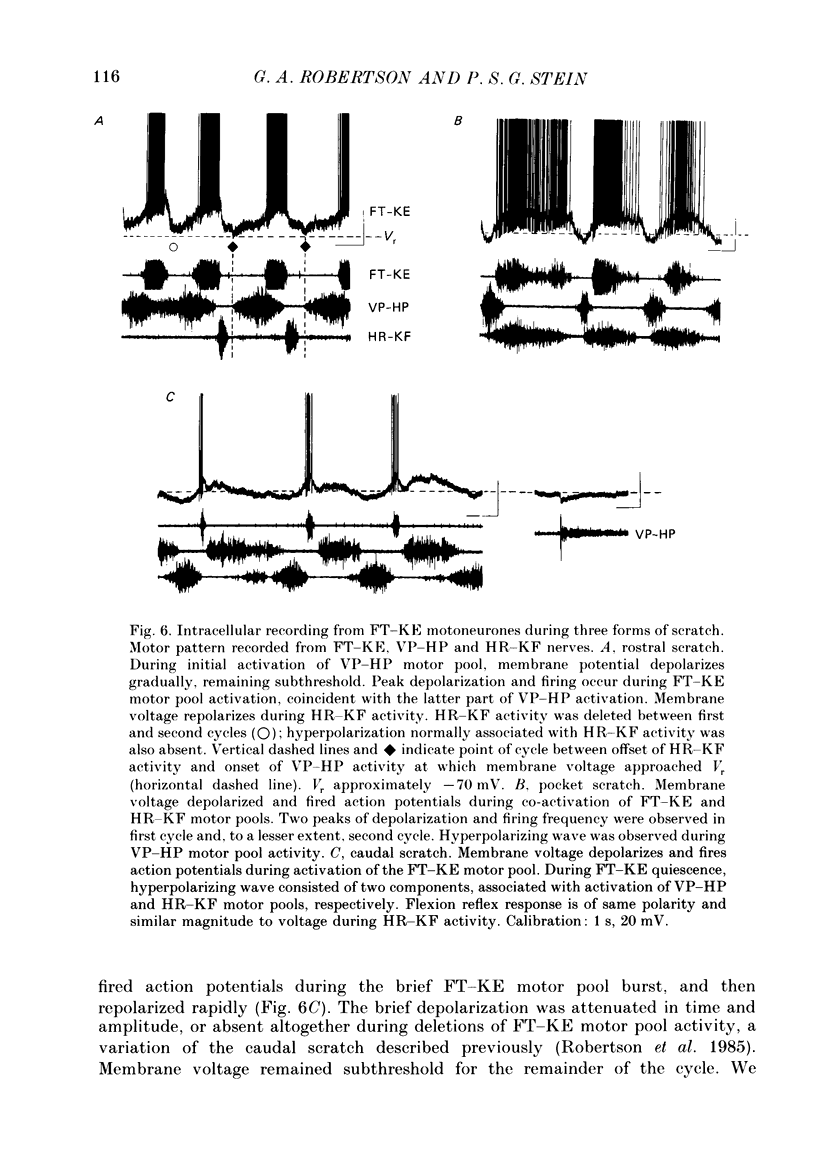
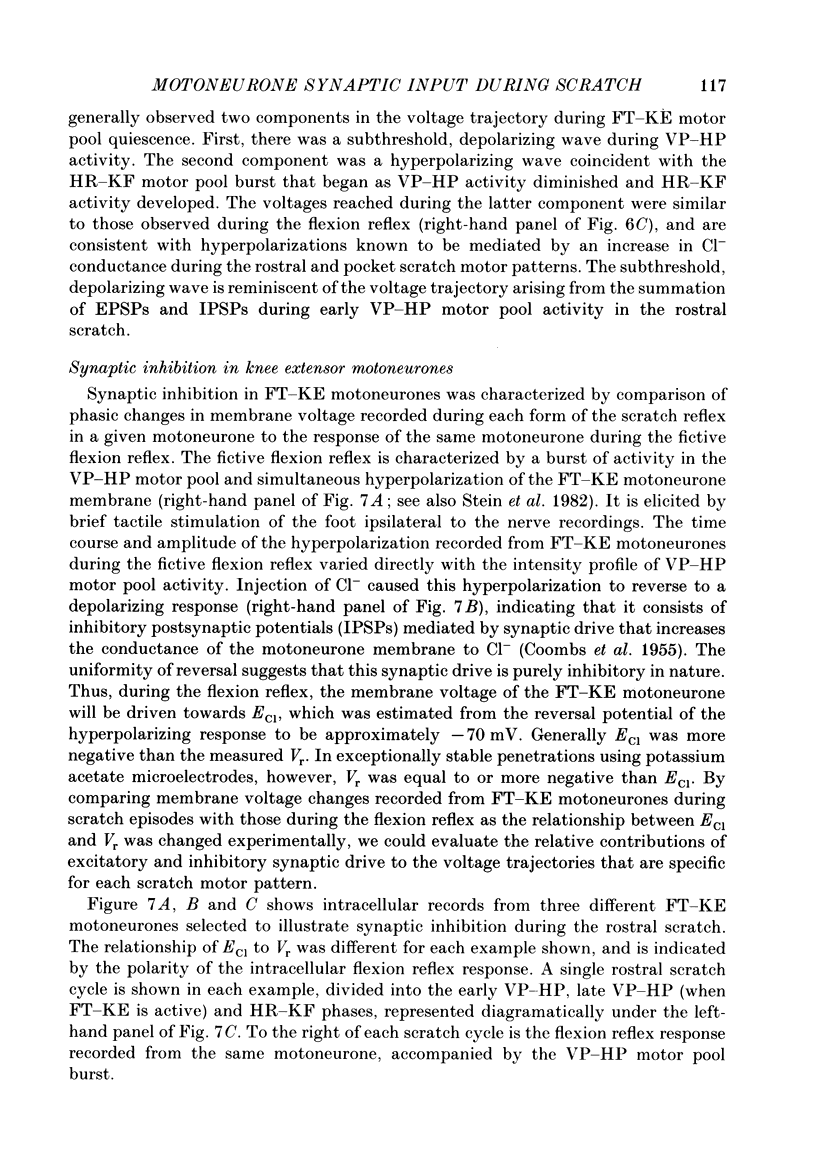
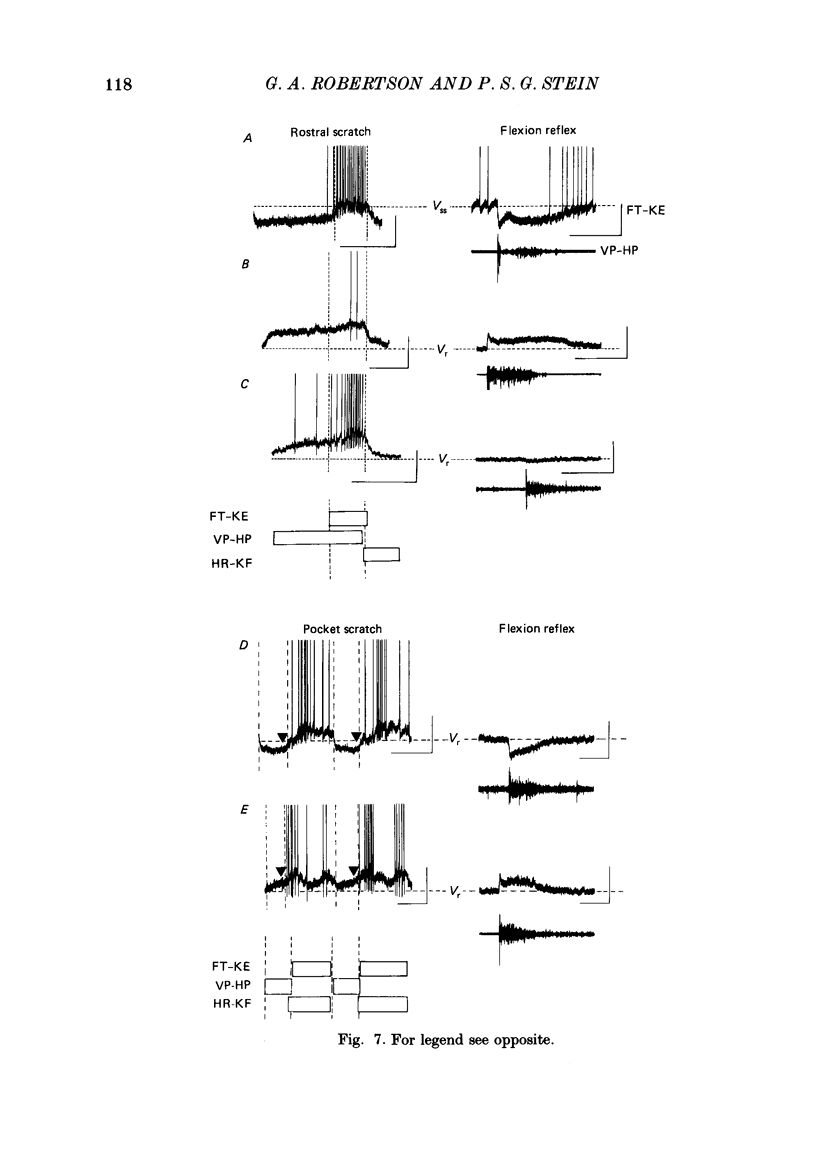
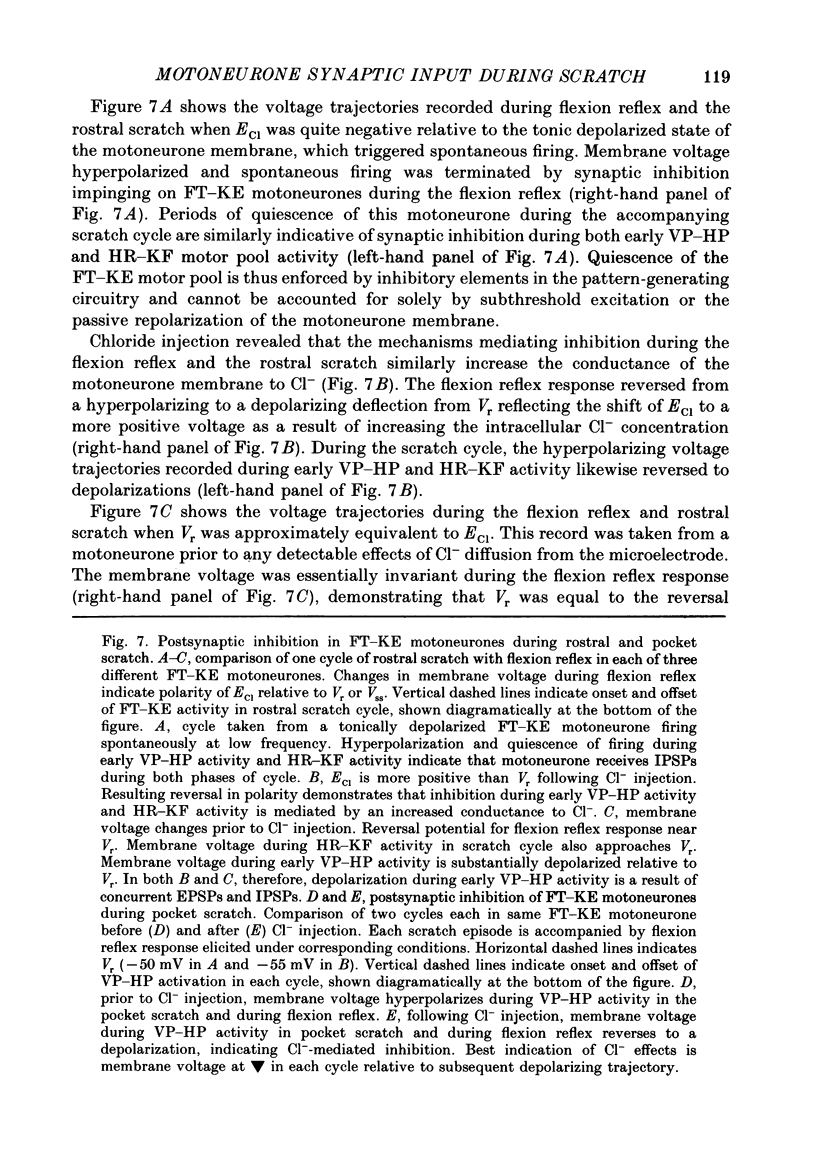
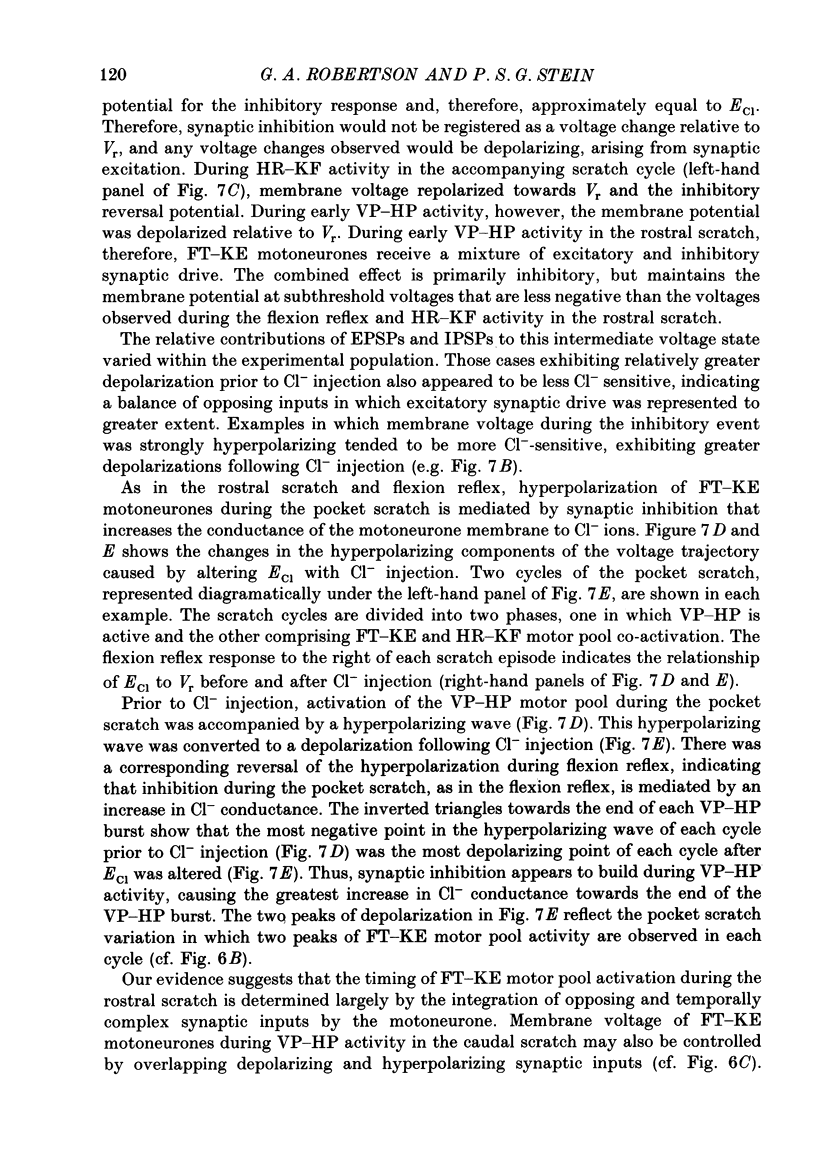
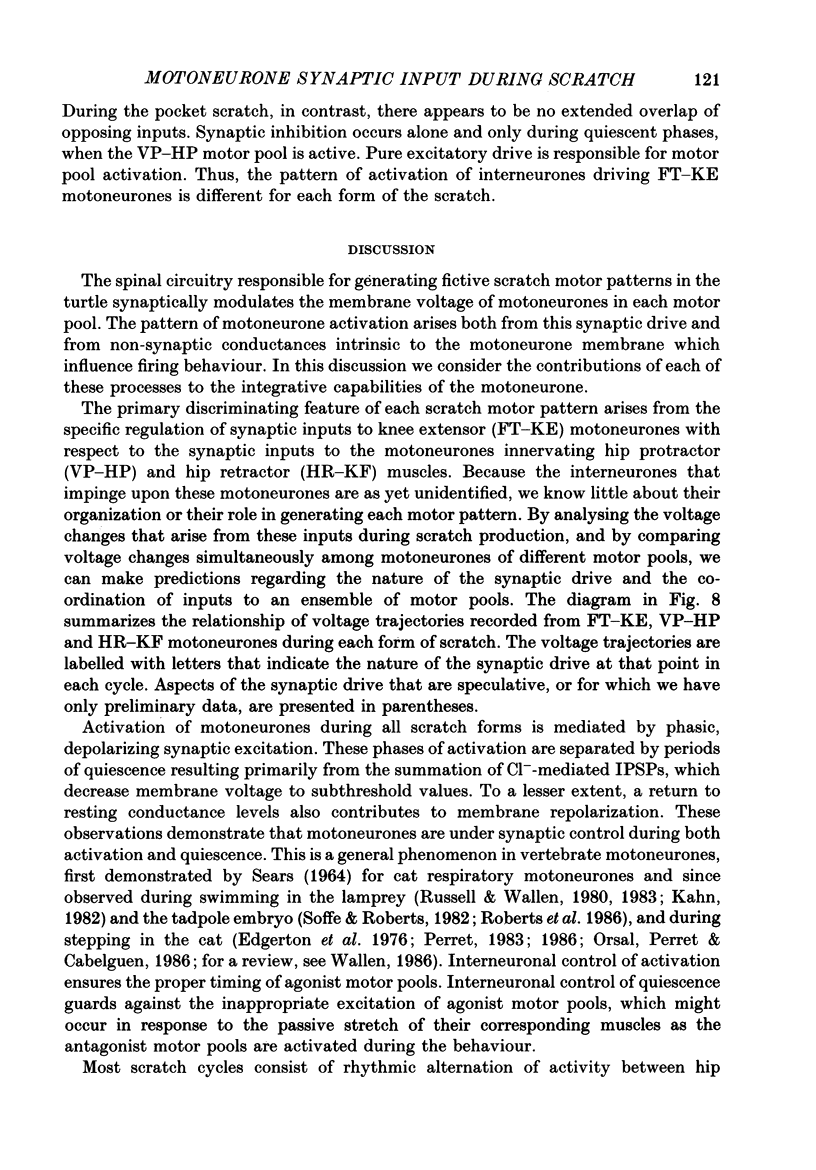
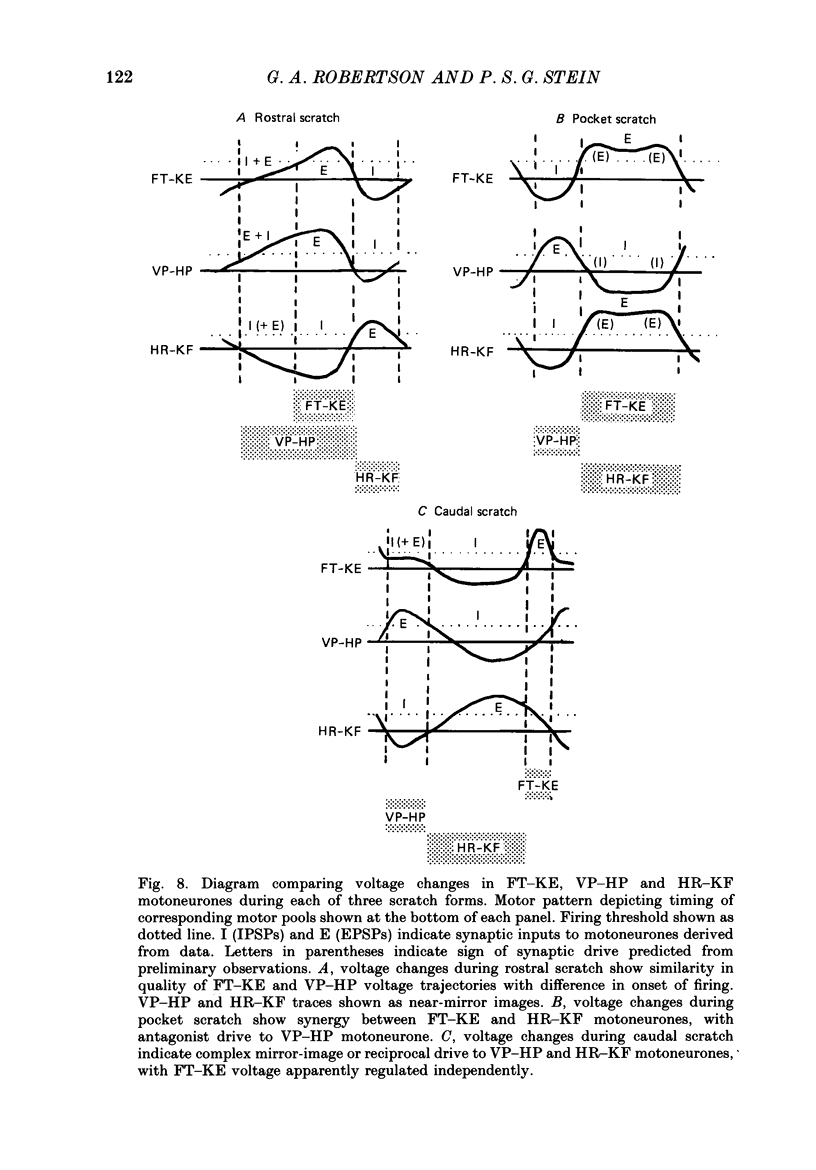
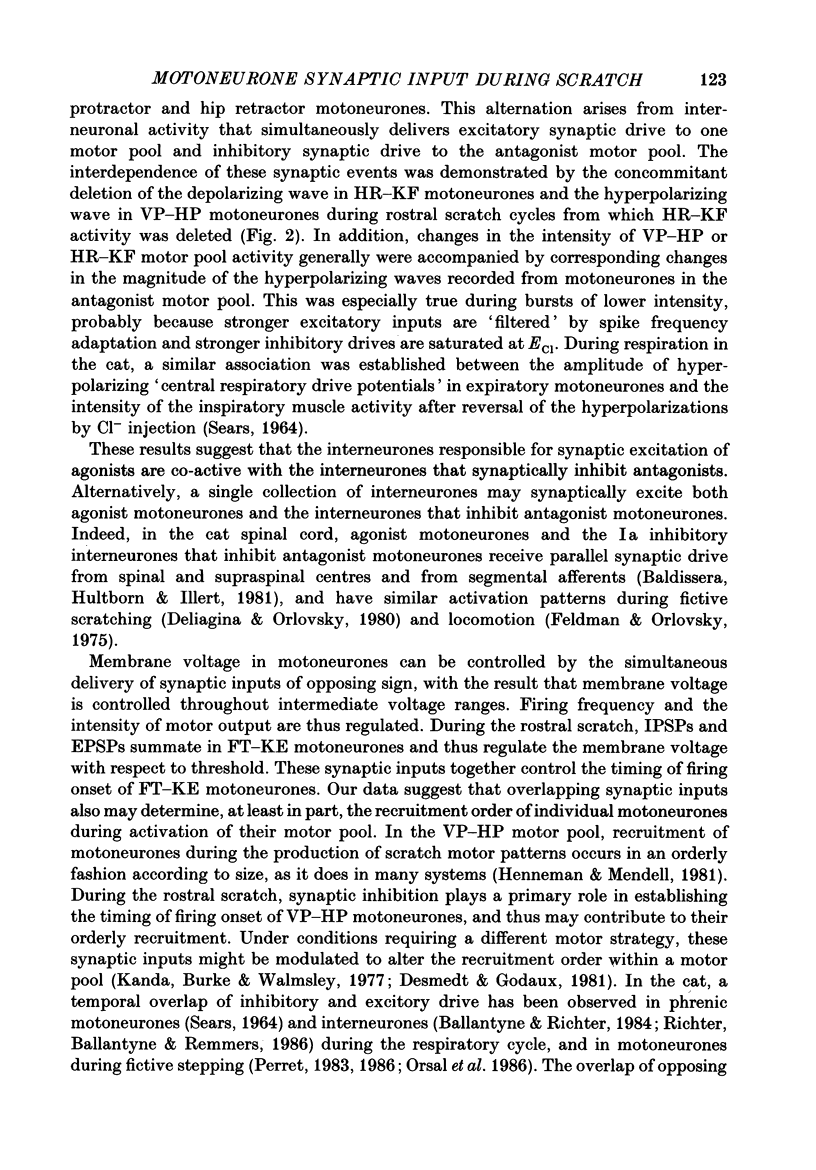
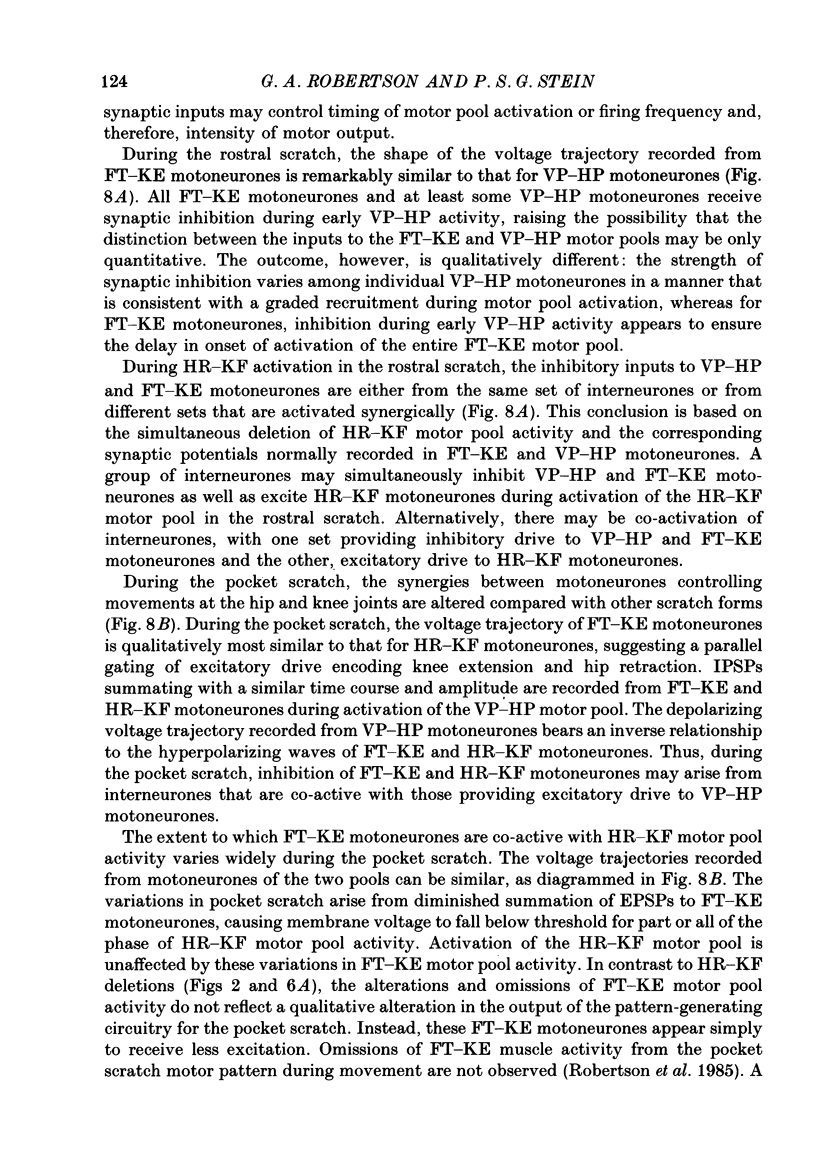
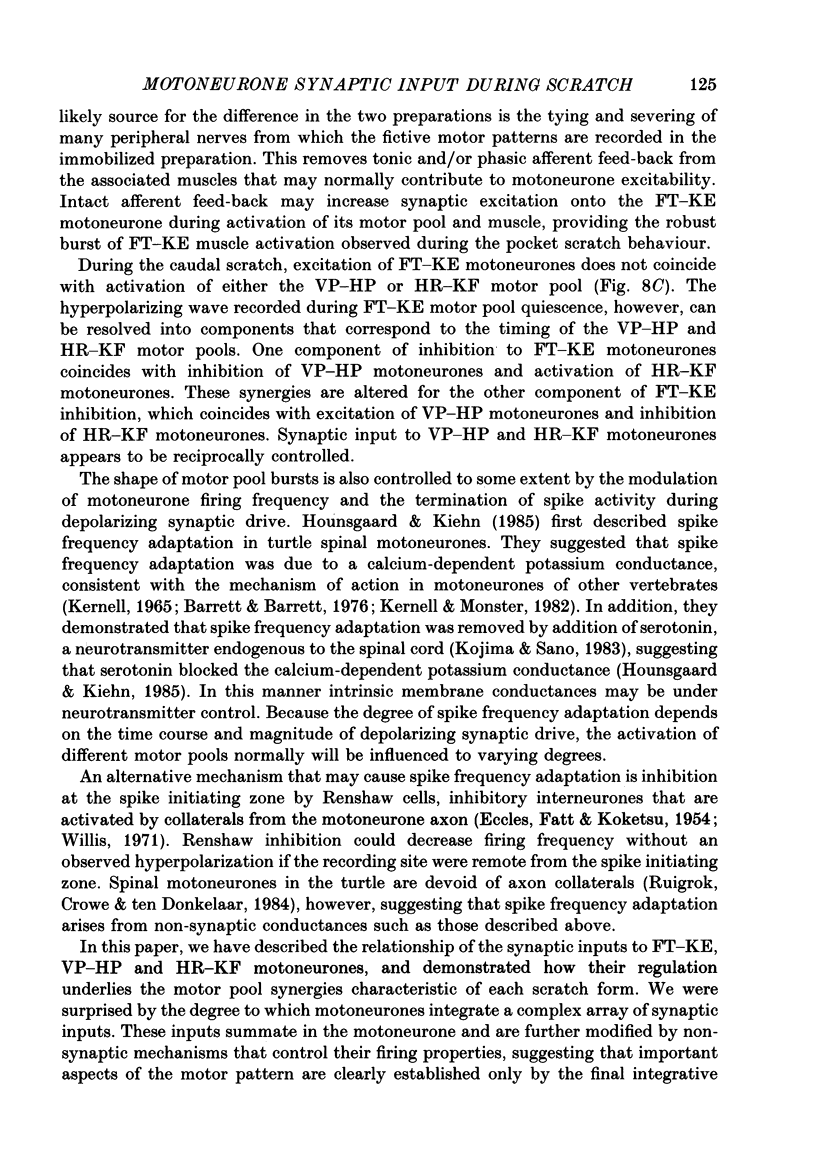
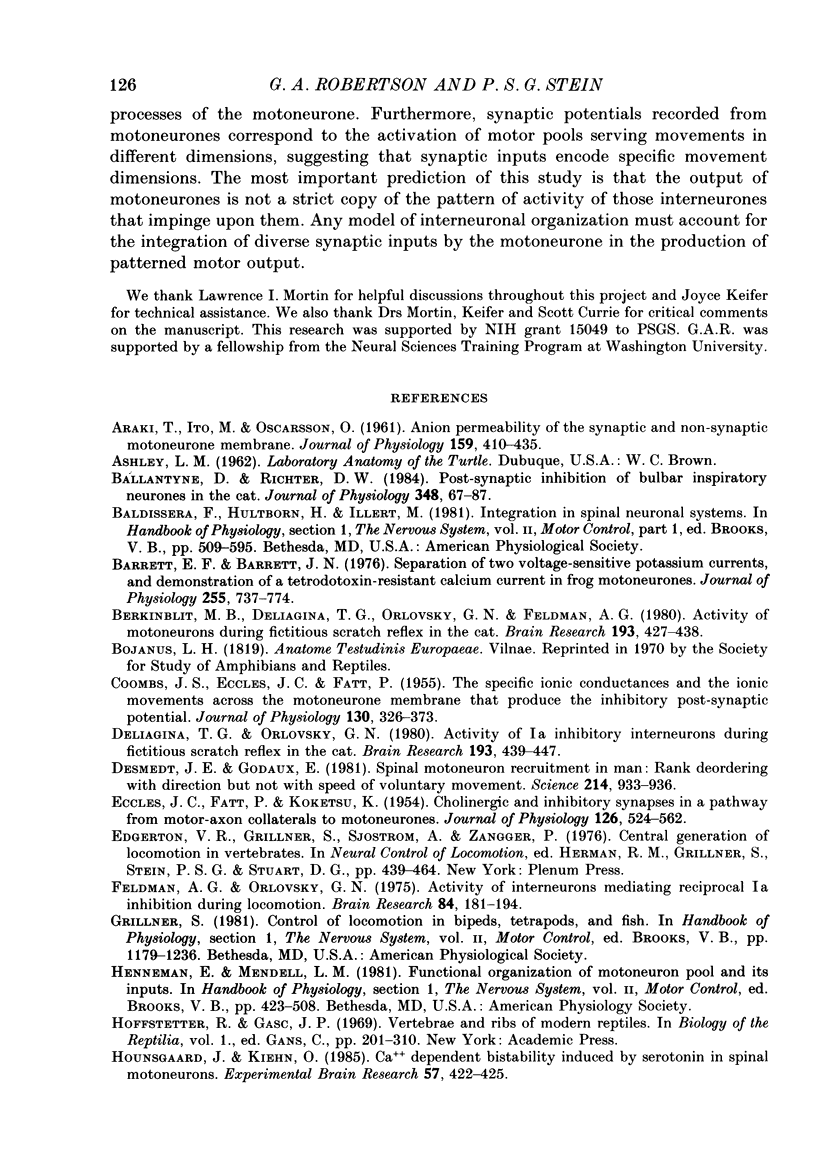
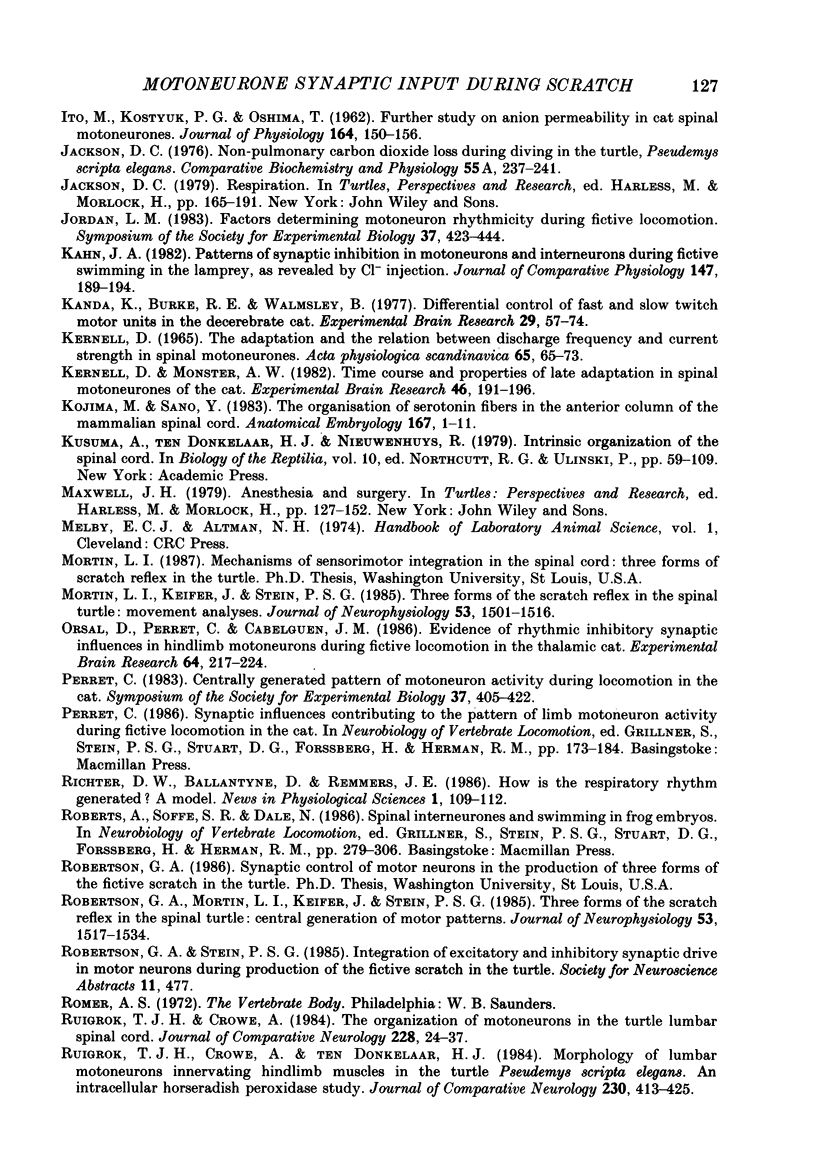
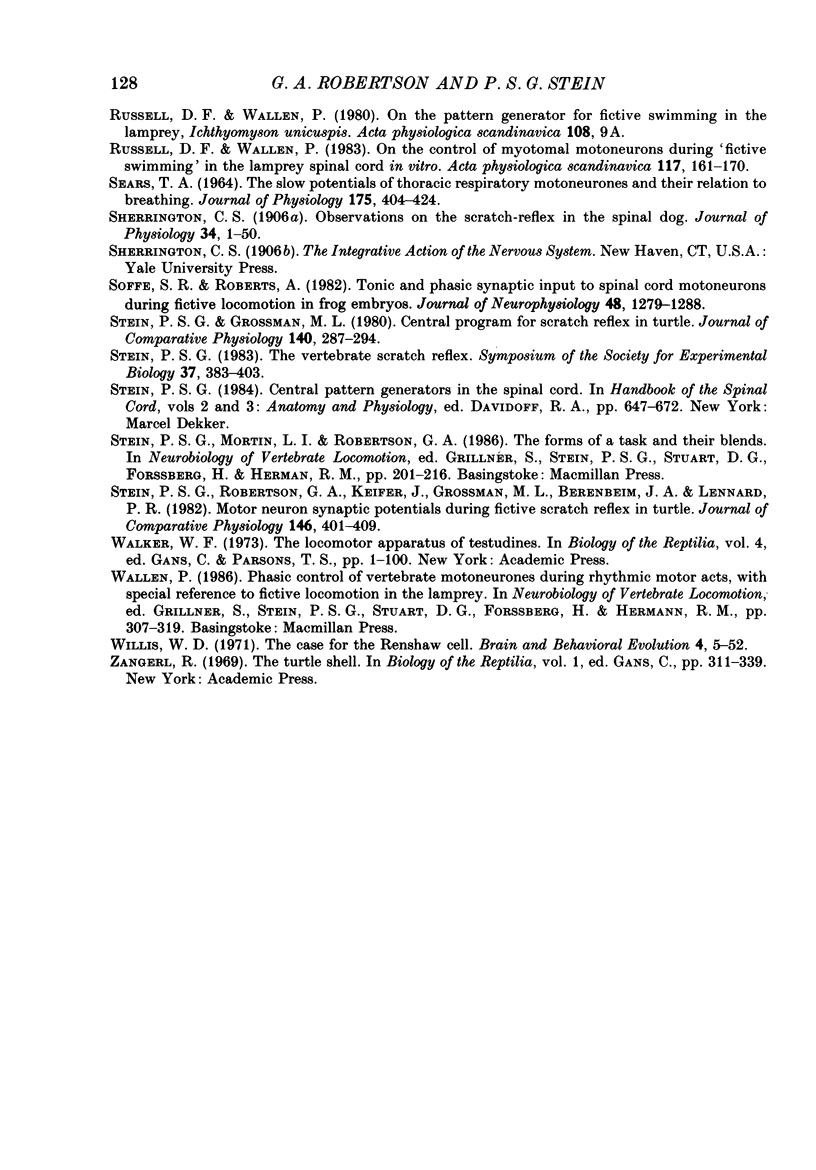
Selected References
These references are in PubMed. This may not be the complete list of references from this article.
- ARAKI T., ITO M., OSCARSSON O. Anion permeability of the synaptic and non-synaptic motoneurone membrane. J Physiol. 1961 Dec;159:410–435. doi: 10.1113/jphysiol.1961.sp006818. [DOI] [PMC free article] [PubMed] [Google Scholar]
- Ballantyne D., Richter D. W. Post-synaptic inhibition of bulbar inspiratory neurones in the cat. J Physiol. 1984 Mar;348:67–87. doi: 10.1113/jphysiol.1984.sp015100. [DOI] [PMC free article] [PubMed] [Google Scholar]
- Barrett E. F., Barret J. N. Separation of two voltage-sensitive potassium currents, and demonstration of a tetrodotoxin-resistant calcium current in frog motoneurones. J Physiol. 1976 Mar;255(3):737–774. doi: 10.1113/jphysiol.1976.sp011306. [DOI] [PMC free article] [PubMed] [Google Scholar]
- Berkinblit M. B., Deliagina T. G., Orlovsky G. N., Feldman A. G. Activity of motoneurons during fictitious scratch reflex in the cat. Brain Res. 1980 Jul 14;193(2):427–438. doi: 10.1016/0006-8993(80)90175-4. [DOI] [PubMed] [Google Scholar]
- COOMBS J. S., ECCLES J. C., FATT P. The specific ionic conductances and the ionic movements across the motoneuronal membrane that produce the inhibitory post-synaptic potential. J Physiol. 1955 Nov 28;130(2):326–374. doi: 10.1113/jphysiol.1955.sp005412. [DOI] [PMC free article] [PubMed] [Google Scholar]
- Deliagina T. G., Orlovsky G. N. Activity of Ia inhibitory interneurons during fictitious scratch reflex in the cat. Brain Res. 1980 Jul 14;193(2):439–447. doi: 10.1016/0006-8993(80)90176-6. [DOI] [PubMed] [Google Scholar]
- Desnedt H. E., Gidaux E. Spinal motoneuron recruitment in man: rank deordering with direction but not with speed of voluntary movement. Science. 1981 Nov 20;214(4523):933–936. doi: 10.1126/science.7302570. [DOI] [PubMed] [Google Scholar]
- ECCLES J. C., FATT P., KOKETSU K. Cholinergic and inhibitory synapses in a pathway from motor-axon collaterals to motoneurones. J Physiol. 1954 Dec 10;126(3):524–562. doi: 10.1113/jphysiol.1954.sp005226. [DOI] [PMC free article] [PubMed] [Google Scholar]
- Feldman A. G., Orlovsky G. N. Activity of interneurons mediating reciprocal 1a inhibition during locomotion. Brain Res. 1975 Feb 7;84(2):181–194. doi: 10.1016/0006-8993(75)90974-9. [DOI] [PubMed] [Google Scholar]
- Hounsgaard J., Kiehn O. Ca++ dependent bistability induced by serotonin in spinal motoneurons. Exp Brain Res. 1985;57(2):422–425. doi: 10.1007/BF00236551. [DOI] [PubMed] [Google Scholar]
- ITO M., KOSTYUK P. G., OSHIMA T. Further study on anion permeability of inhibitory post-synaptic membrane of cat motoneurones. J Physiol. 1962 Oct;164:150–156. doi: 10.1113/jphysiol.1962.sp007009. [DOI] [PMC free article] [PubMed] [Google Scholar]
- Jackson D. C. Non-pulmonary CO2 loss during diving in the turtle, Pseudemys scripta elegans. Comp Biochem Physiol A Comp Physiol. 1976;55(3):237–241. doi: 10.1016/0300-9629(76)90138-9. [DOI] [PubMed] [Google Scholar]
- Jordan L. M. Factors determining motoneuron rhythmicity during fictive locomotion. Symp Soc Exp Biol. 1983;37:423–444. [PubMed] [Google Scholar]
- Kanda K., Burke R. E., Walmsley B. Differential control of fast and slow twitch motor units in the decerebrate cat. Exp Brain Res. 1977 Aug 8;29(1):57–74. doi: 10.1007/BF00236875. [DOI] [PubMed] [Google Scholar]
- Kernell D., Monster A. W. Time course and properties of late adaptation in spinal motoneurones of the cat. Exp Brain Res. 1982;46(2):191–196. doi: 10.1007/BF00237176. [DOI] [PubMed] [Google Scholar]
- Kojima M., Sano Y. The organization of serotonin fibers in the anterior column of the mammalian spinal cord. An immunohistochemical study. Anat Embryol (Berl) 1983;167(1):1–11. doi: 10.1007/BF00304597. [DOI] [PubMed] [Google Scholar]
- Mortin L. I., Keifer J., Stein P. S. Three forms of the scratch reflex in the spinal turtle: movement analyses. J Neurophysiol. 1985 Jun;53(6):1501–1516. doi: 10.1152/jn.1985.53.6.1501. [DOI] [PubMed] [Google Scholar]
- Orsal D., Perret C., Cabelguen J. M. Evidence of rhythmic inhibitory synaptic influences in hindlimb motoneurons during fictive locomotion in the thalamic cat. Exp Brain Res. 1986;64(1):217–224. doi: 10.1007/BF00238216. [DOI] [PubMed] [Google Scholar]
- Perret C. Centrally generated pattern of motoneuron activity during locomotion in the cat. Symp Soc Exp Biol. 1983;37:405–422. [PubMed] [Google Scholar]
- Robertson G. A., Mortin L. I., Keifer J., Stein P. S. Three forms of the scratch reflex in the spinal turtle: central generation of motor patterns. J Neurophysiol. 1985 Jun;53(6):1517–1534. doi: 10.1152/jn.1985.53.6.1517. [DOI] [PubMed] [Google Scholar]
- Ruigrok T. J., Crowe A. The organization of motoneurons in the turtle lumbar spinal cord. J Comp Neurol. 1984 Sep 1;228(1):24–37. doi: 10.1002/cne.902280105. [DOI] [PubMed] [Google Scholar]
- Ruigrok T. J., Crowe A., ten Donkelaar H. J. Morphology of lumbar motoneurons innervating hindlimb muscles in the turtle Pseudemys scripta elegans: an intracellular horseradish peroxidase study. J Comp Neurol. 1984 Dec 10;230(3):413–425. doi: 10.1002/cne.902300309. [DOI] [PubMed] [Google Scholar]
- Russell D. F., Wallén P. On the control of myotomal motoneurones during "fictive swimming" in the lamprey spinal cord in vitro. Acta Physiol Scand. 1983 Feb;117(2):161–170. doi: 10.1111/j.1748-1716.1983.tb07193.x. [DOI] [PubMed] [Google Scholar]
- SEARS T. A. THE SLOW POTENTIALS OF THORACIC RESPIRATORY MOTONEURONES AND THEIR RELATION TO BREATHING. J Physiol. 1964 Dec;175:404–424. doi: 10.1113/jphysiol.1964.sp007524. [DOI] [PMC free article] [PubMed] [Google Scholar]
- Sherrington C. S. Observations on the scratch-reflex in the spinal dog. J Physiol. 1906 Mar 13;34(1-2):1–50. doi: 10.1113/jphysiol.1906.sp001139. [DOI] [PMC free article] [PubMed] [Google Scholar]
- Soffe S. R., Roberts A. Tonic and phasic synaptic input to spinal cord motoneurons during fictive locomotion in frog embryos. J Neurophysiol. 1982 Dec;48(6):1279–1288. doi: 10.1152/jn.1982.48.6.1279. [DOI] [PubMed] [Google Scholar]
- Stein P. S. The vertebrate scratch reflex. Symp Soc Exp Biol. 1983;37:383–403. [PubMed] [Google Scholar]
- Willis W. D. The case for the Renshaw cell. Brain Behav Evol. 1971;4(1):5–52. doi: 10.1159/000125422. [DOI] [PubMed] [Google Scholar]