Abstract
1. The rate of calcium release (Rrel) from the sarcoplasmic reticulum (SR) in voltage clamped segments of frog skeletal muscle fibres was calculated from myoplasmic free calcium transients (delta[Ca2+]) measured with the calcium indicator dye Antipyrylazo III. 2. During a 100-200 ms depolarizing pulse Rrel reached an early peak and then declined markedly. The time course and extent of decline of Rrel were independent of membrane potential over a range of potentials where release activation varied severalfold. 3. For test pulses applied shortly after relatively large or long conditioning pulses Rrel completely lacked the early peak. The peak gradually recovered as the interval between the conditioning and test pulses was increased to 1 s. 4. A latency was often observed before the start of recovery of the peak in Rrel. The latency appeared to be correlated with the time for delta[Ca2+] to fall below a certain level, indicating that recovery of the peak might represent reversal of a calcium-dependent process. It was therefore proposed that the rapid decline in Rrel during a pulse was due to calcium-dependent inactivation of the SR calcium release channels. 5. Inactivation continued to develop during the interval between a relatively large 20 ms conditioning pulse and a test pulse applied 20 ms later. This was as expected for calcium-dependent inactivation of SR calcium release because of the elevated [Ca2+] between the conditioning and test pulses. It was not as expected for external membrane potential-dependent inactivation. 6. Small steady elevations in [Ca2+] due to relatively small 200 ms conditioning pulses produced marked inactivation of Rrel, indicating an apparent dissociation constant for calcium-dependent inactivation only slightly above resting [Ca2+]. 7. All observations could be well simulated by a two-step model for inactivation in which myoplasmic free calcium equilibrates rapidly with a high-affinity calcium receptor on the release channel and then the calcium-receptor complex undergoes a slower conformational change to the inactivated state of the channel. 8. An alternative model in which calcium binds to a soluble receptor (e.g. free calmodulin) and then the calcium-receptor complex binds to and directly inactivates the channel was shown to be formally identical to the preceding model. Either model could closely simulate all observations.
Full text
PDF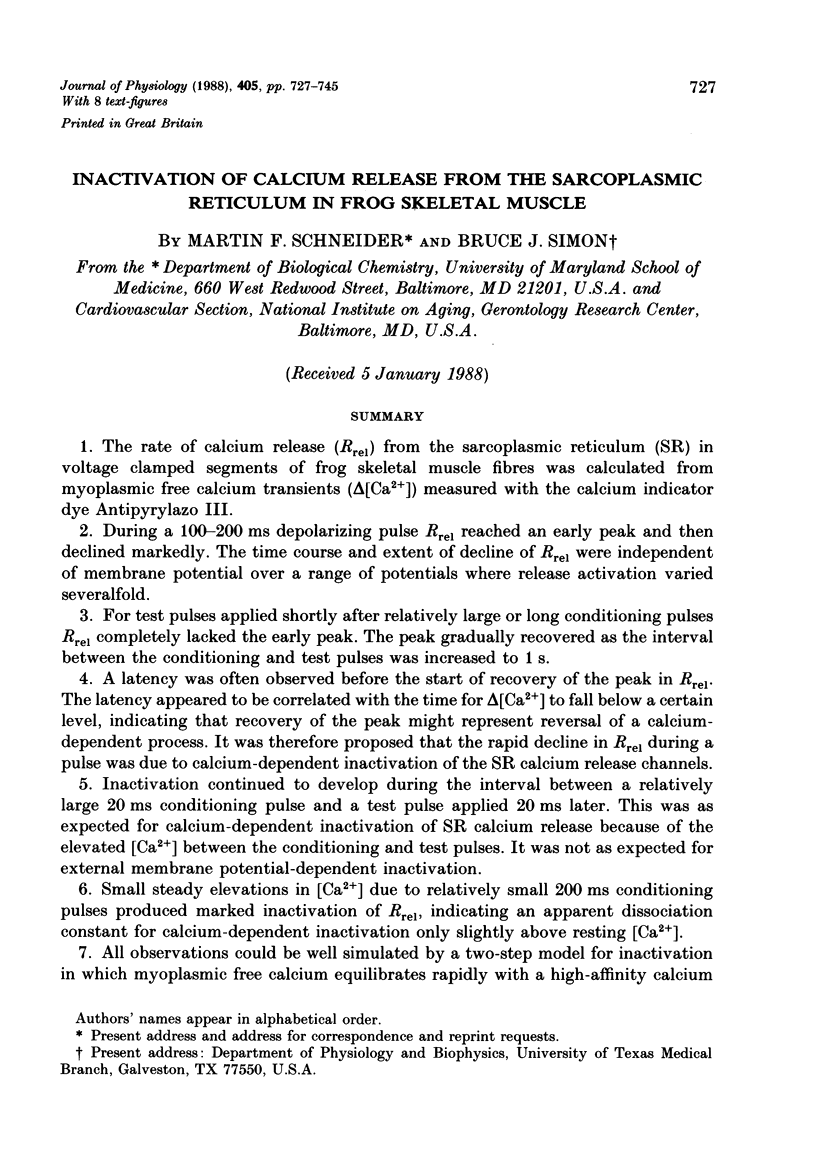
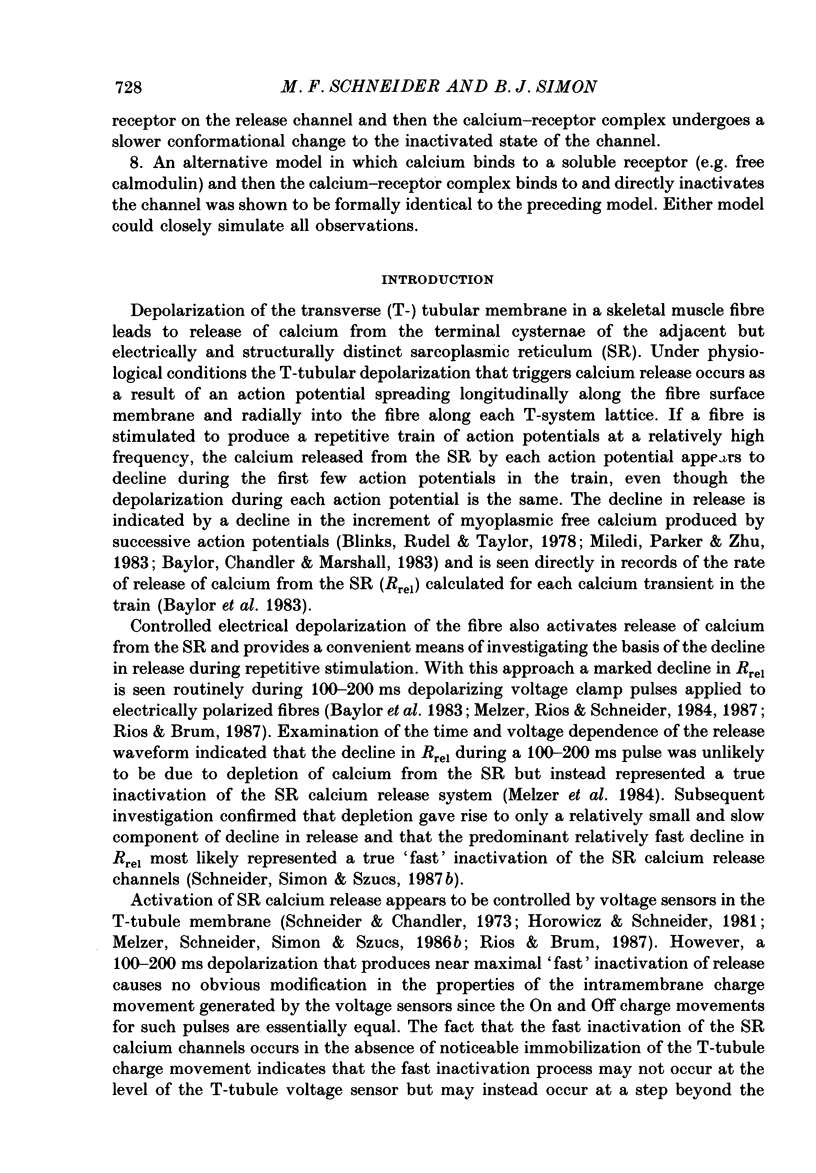
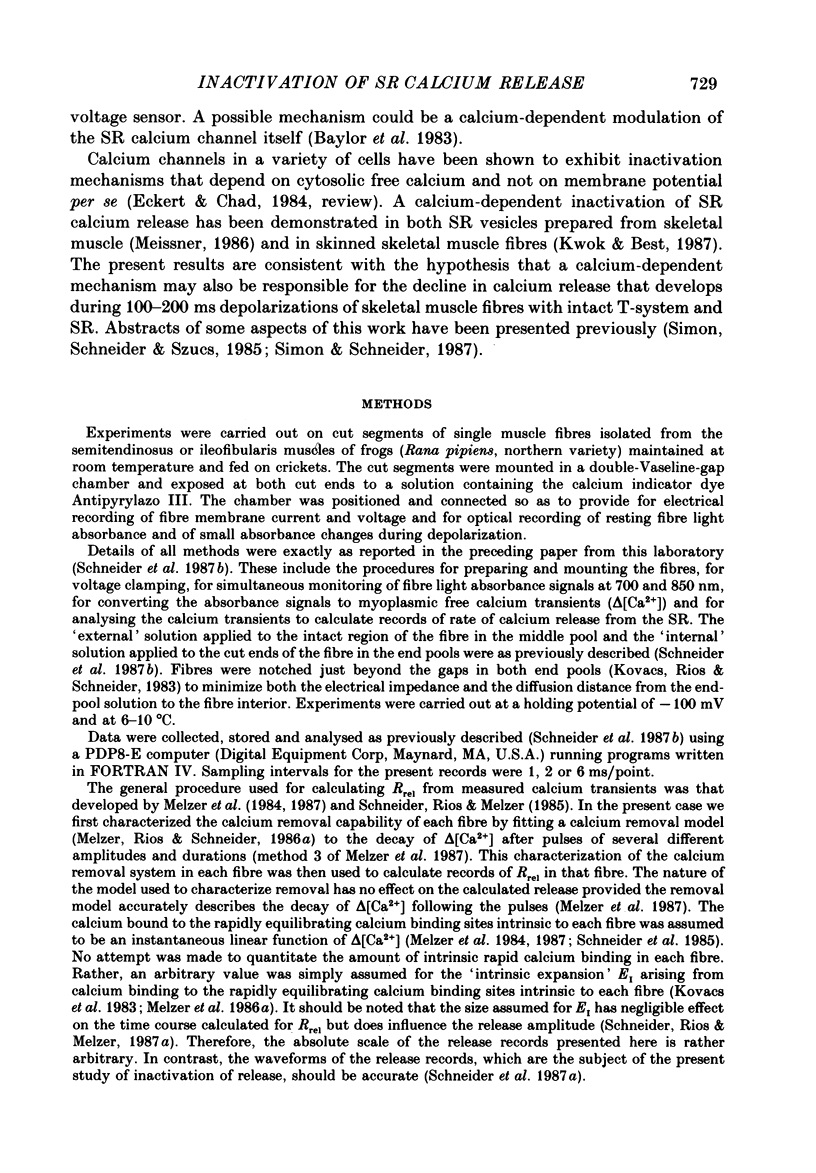
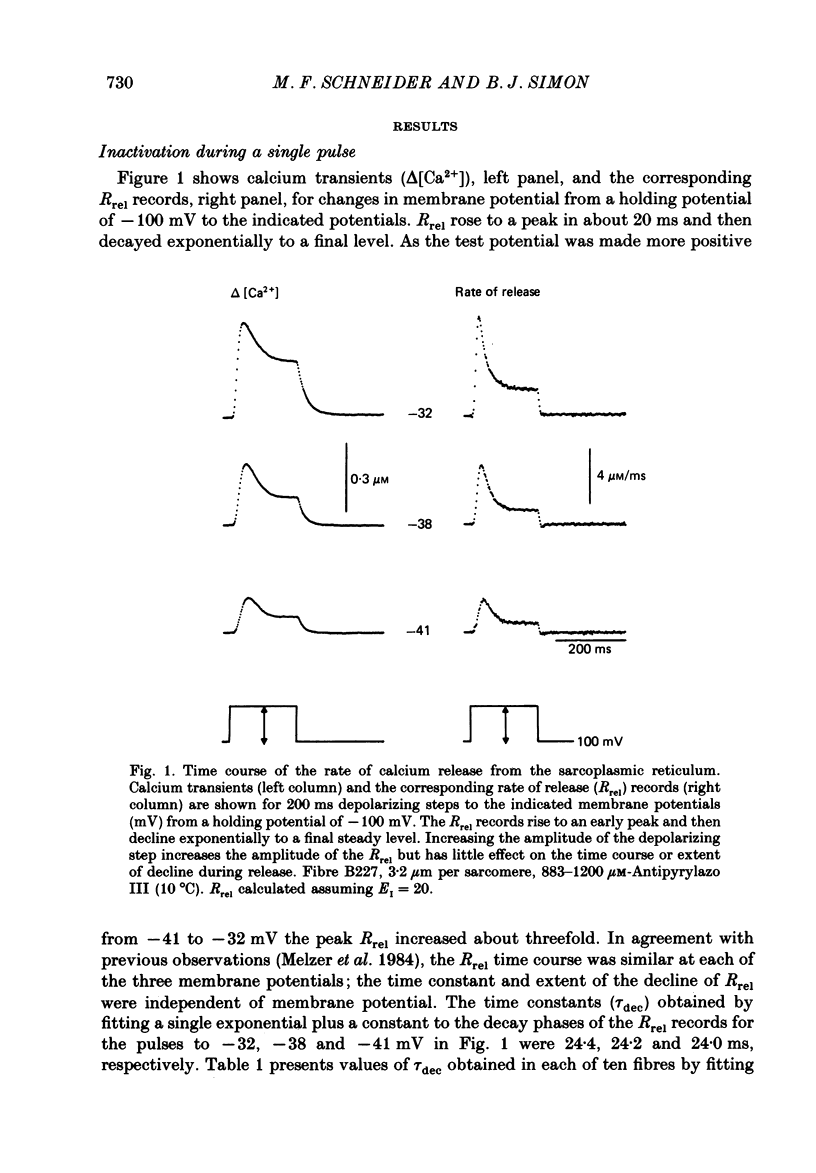
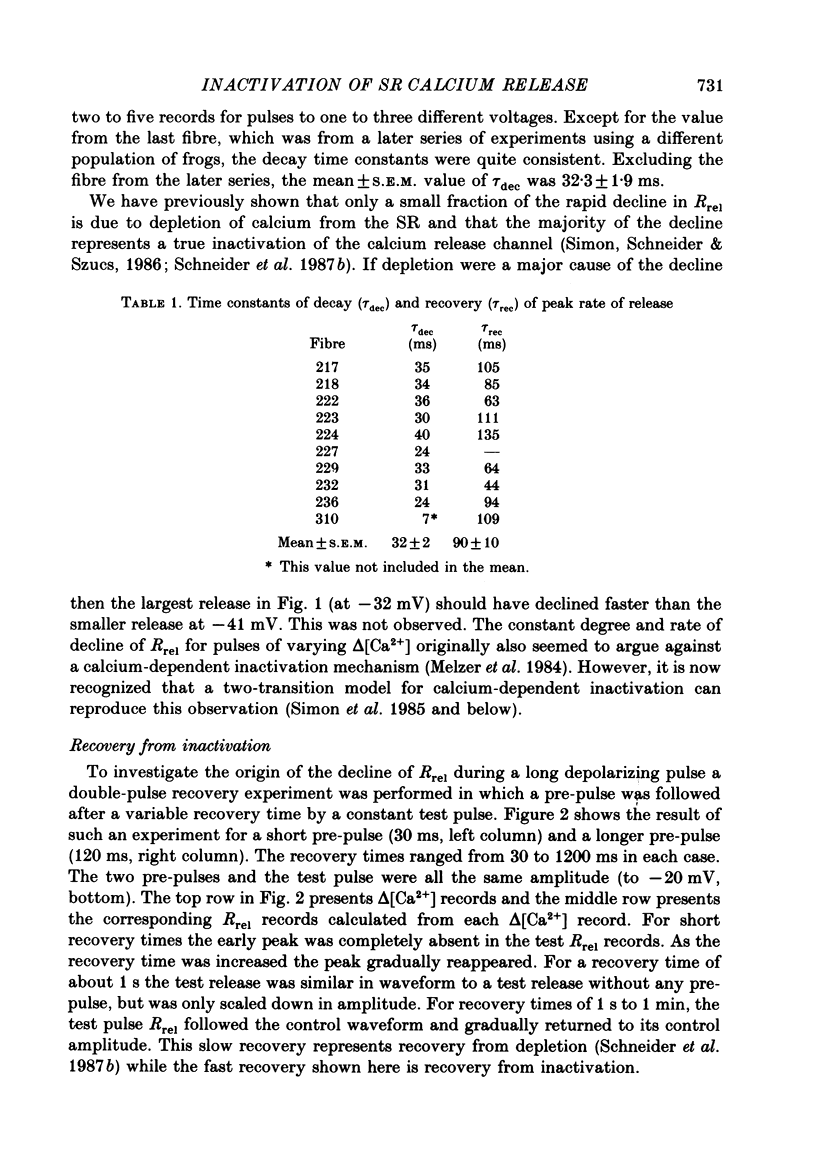
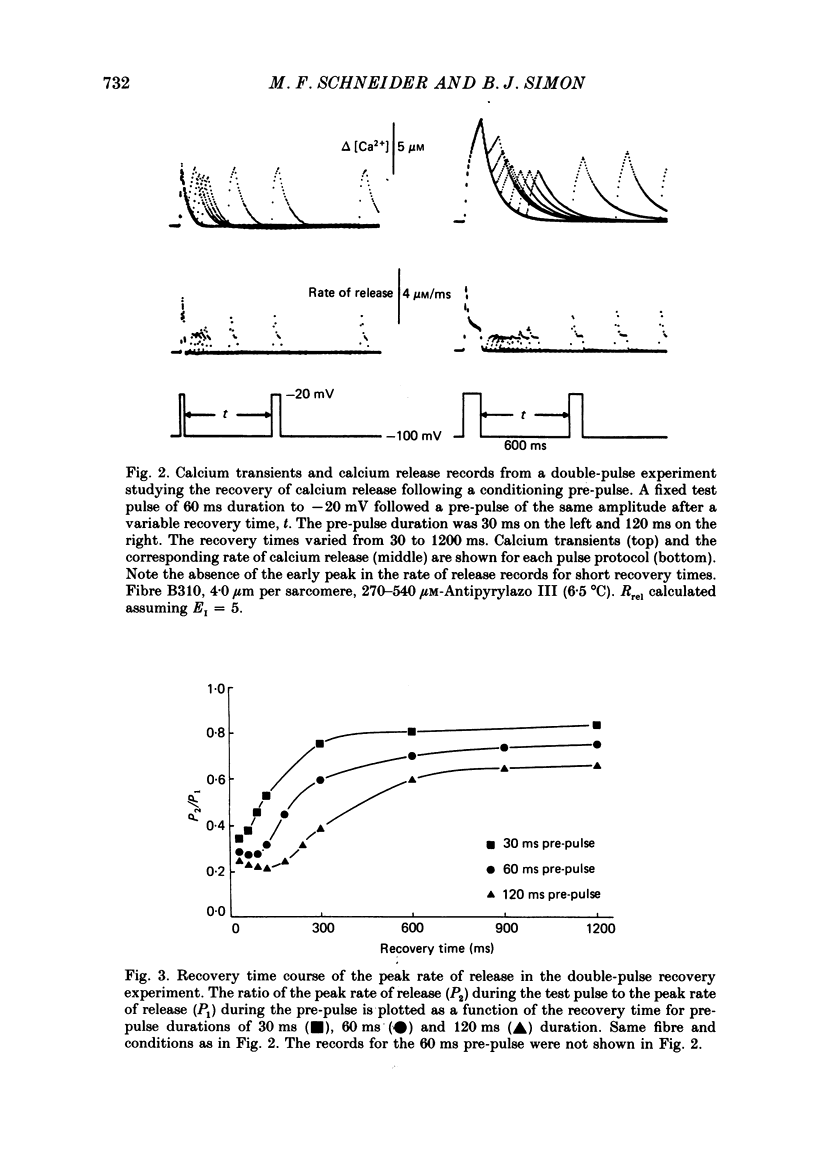
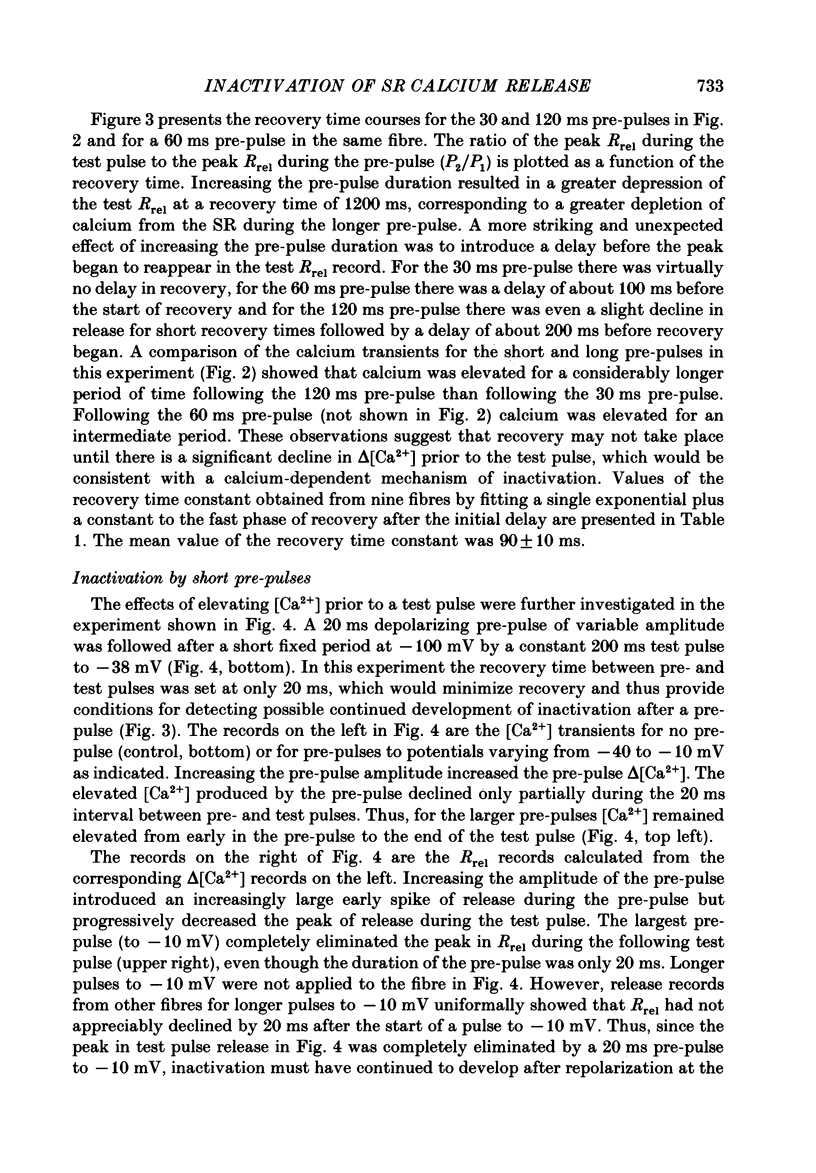
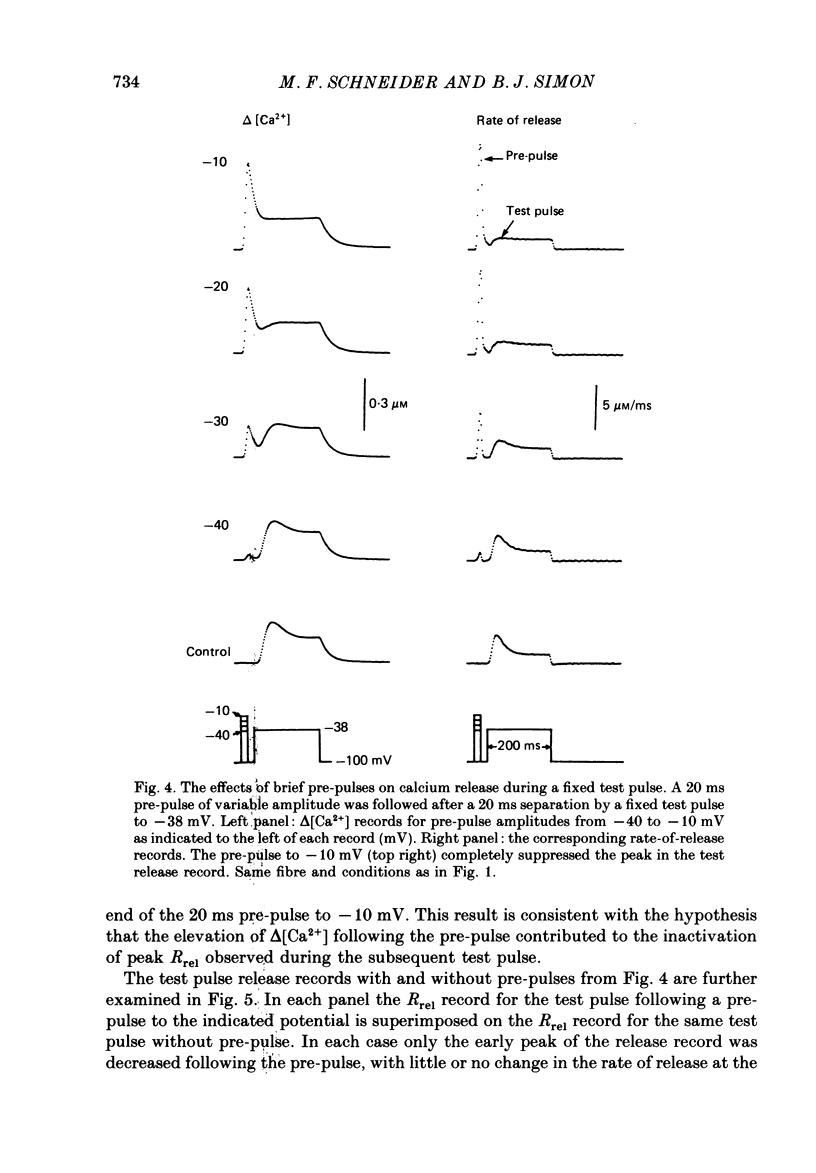
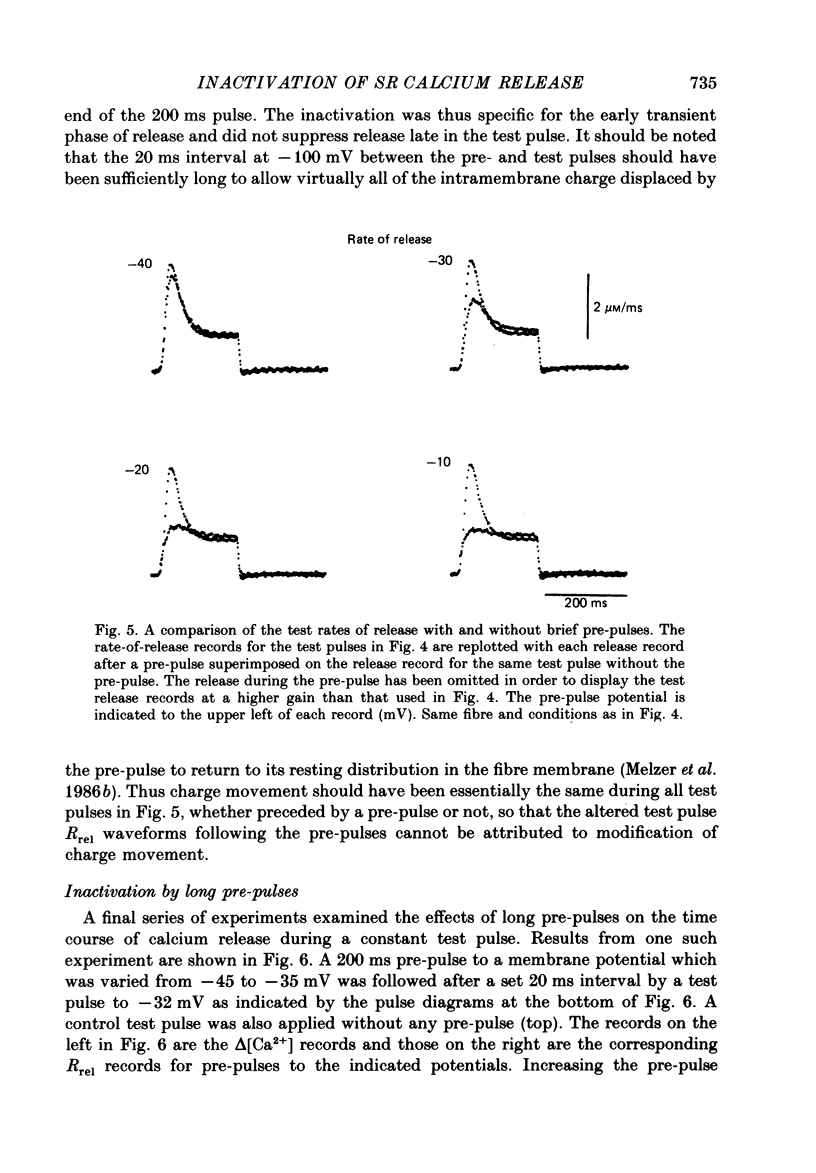
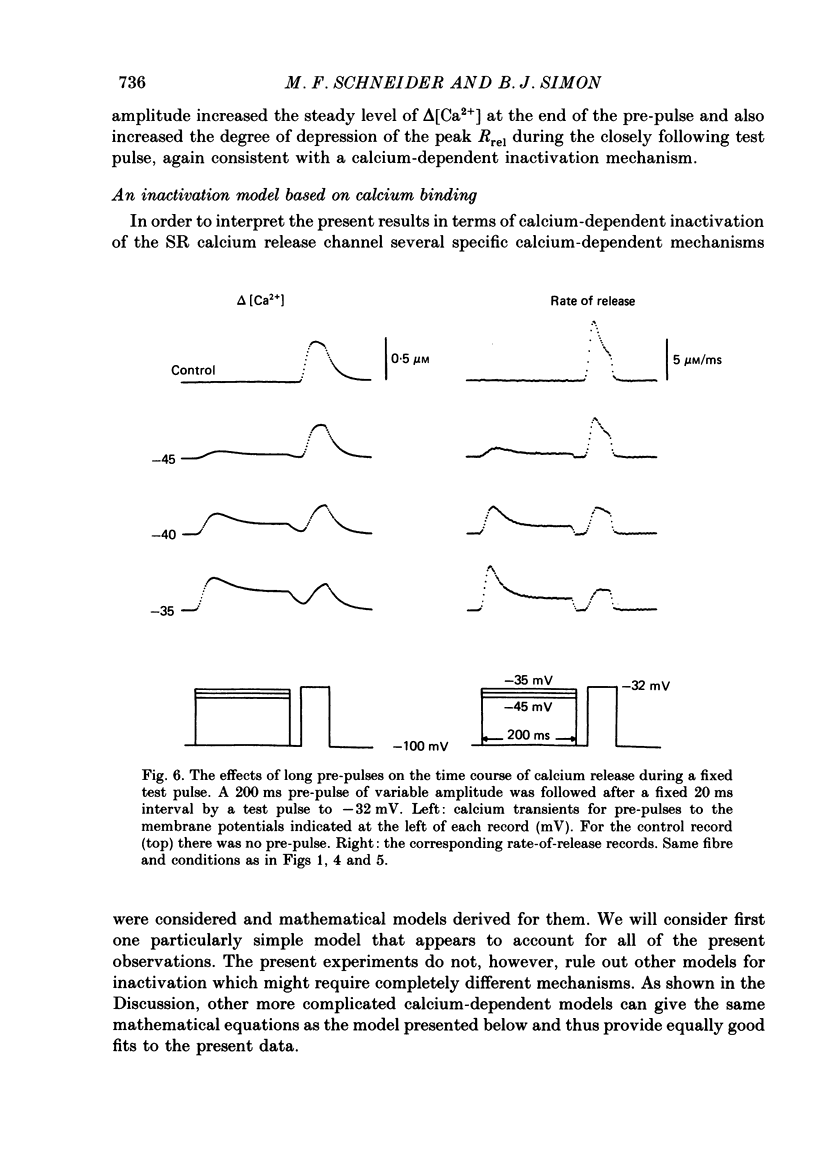
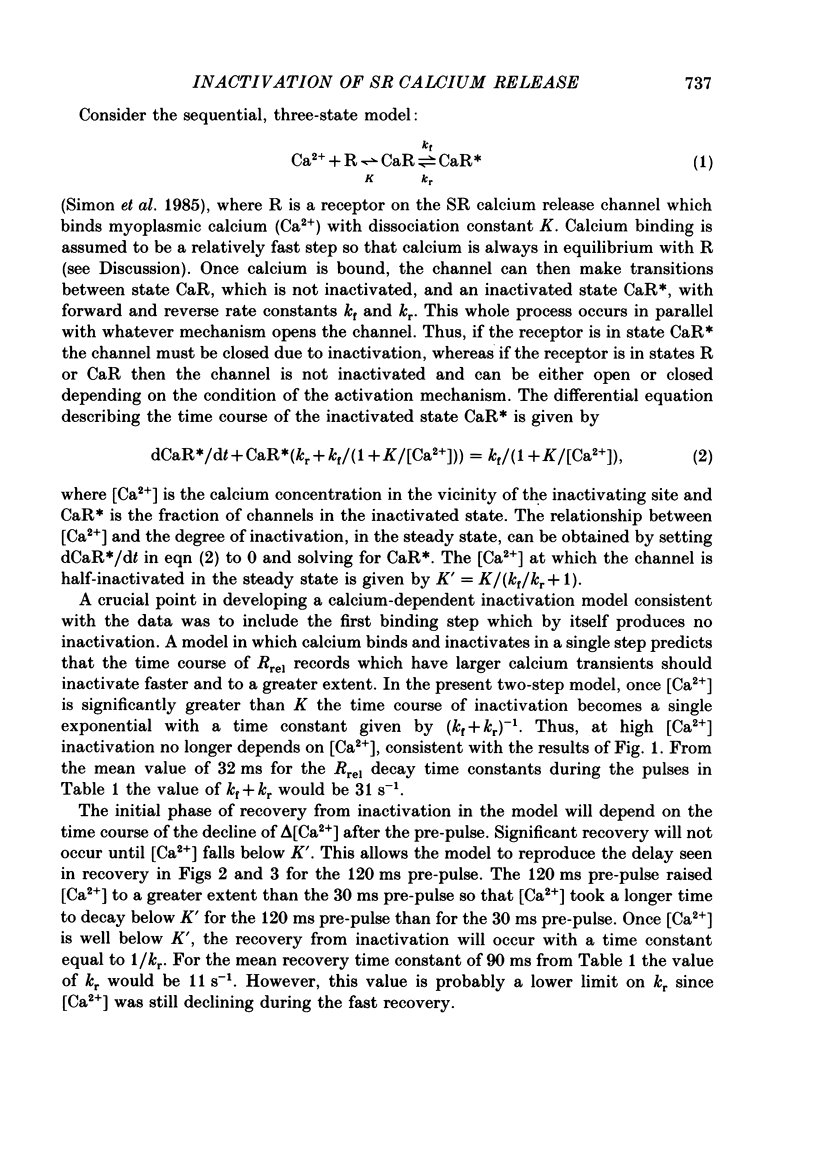
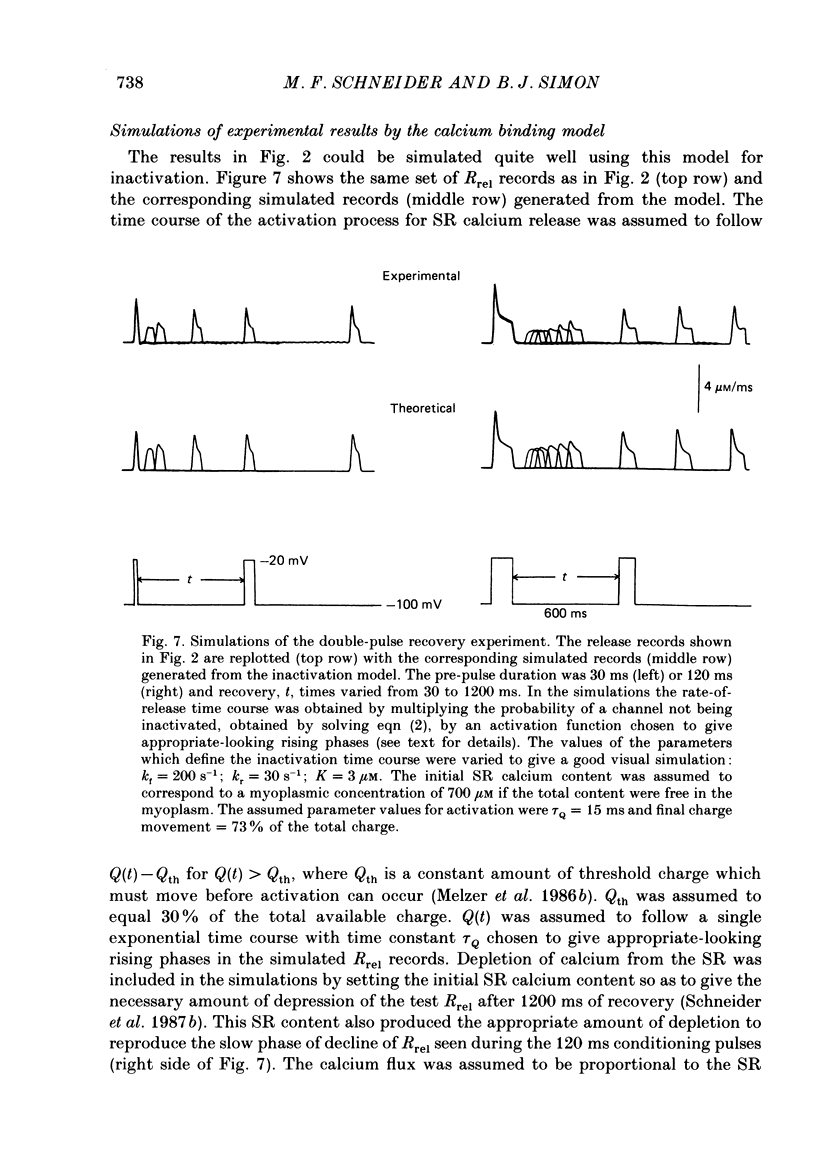
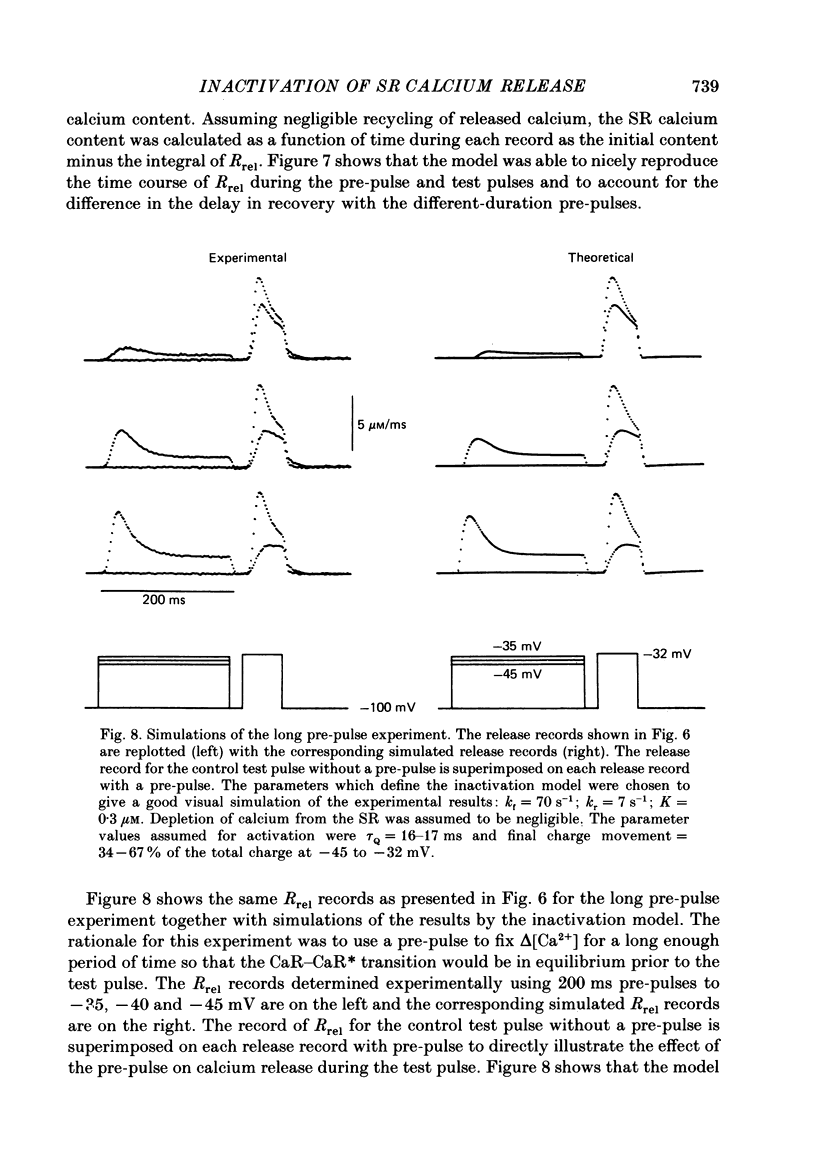
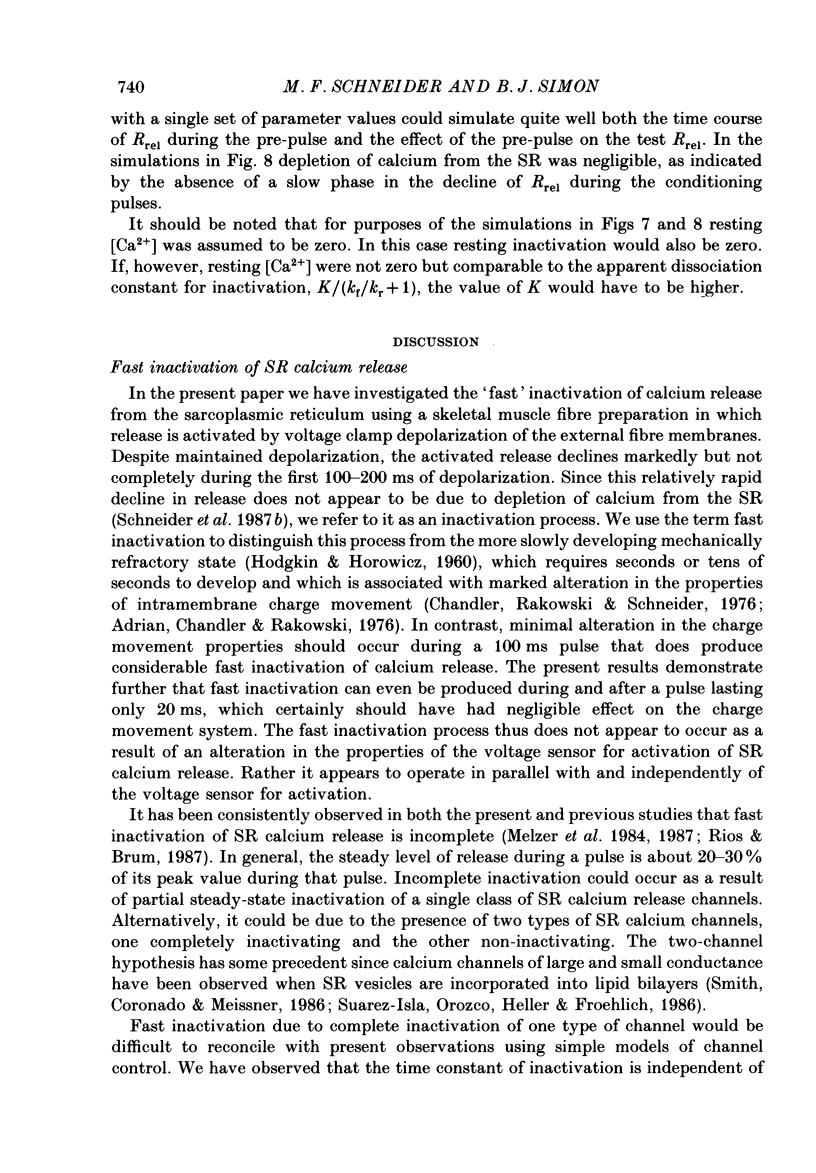
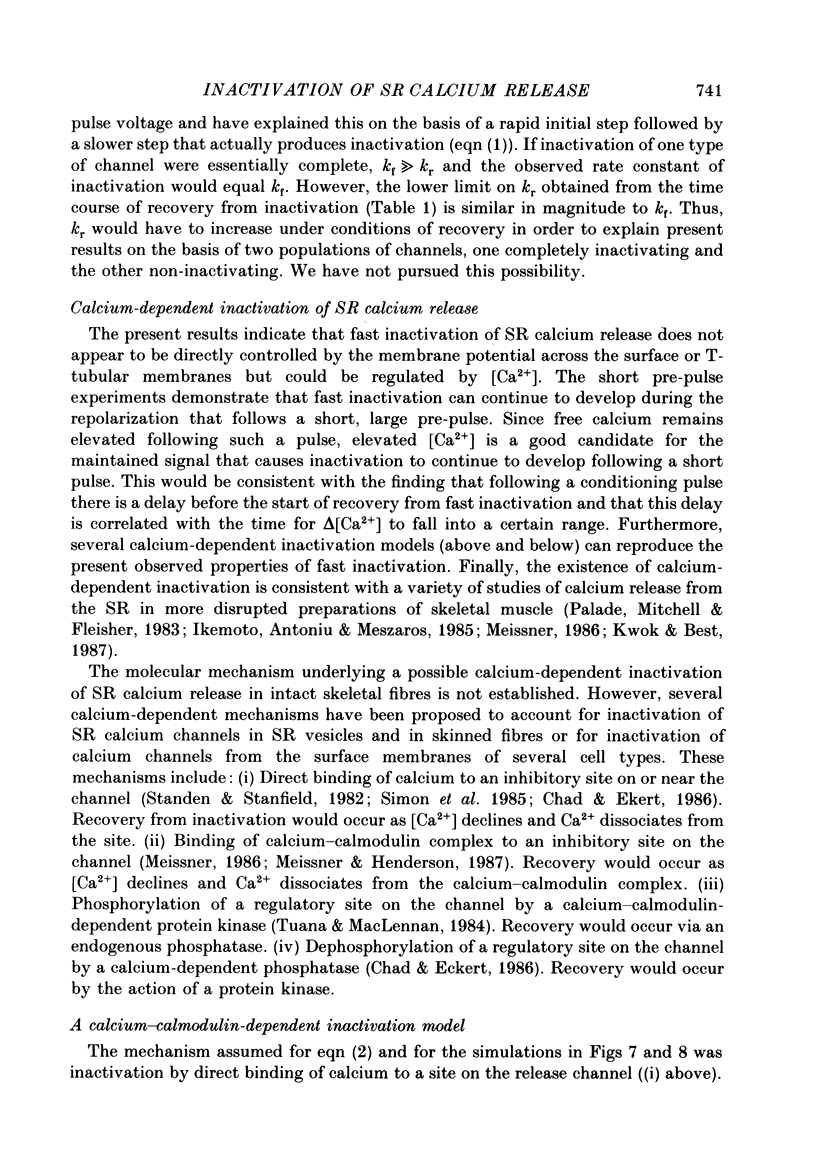
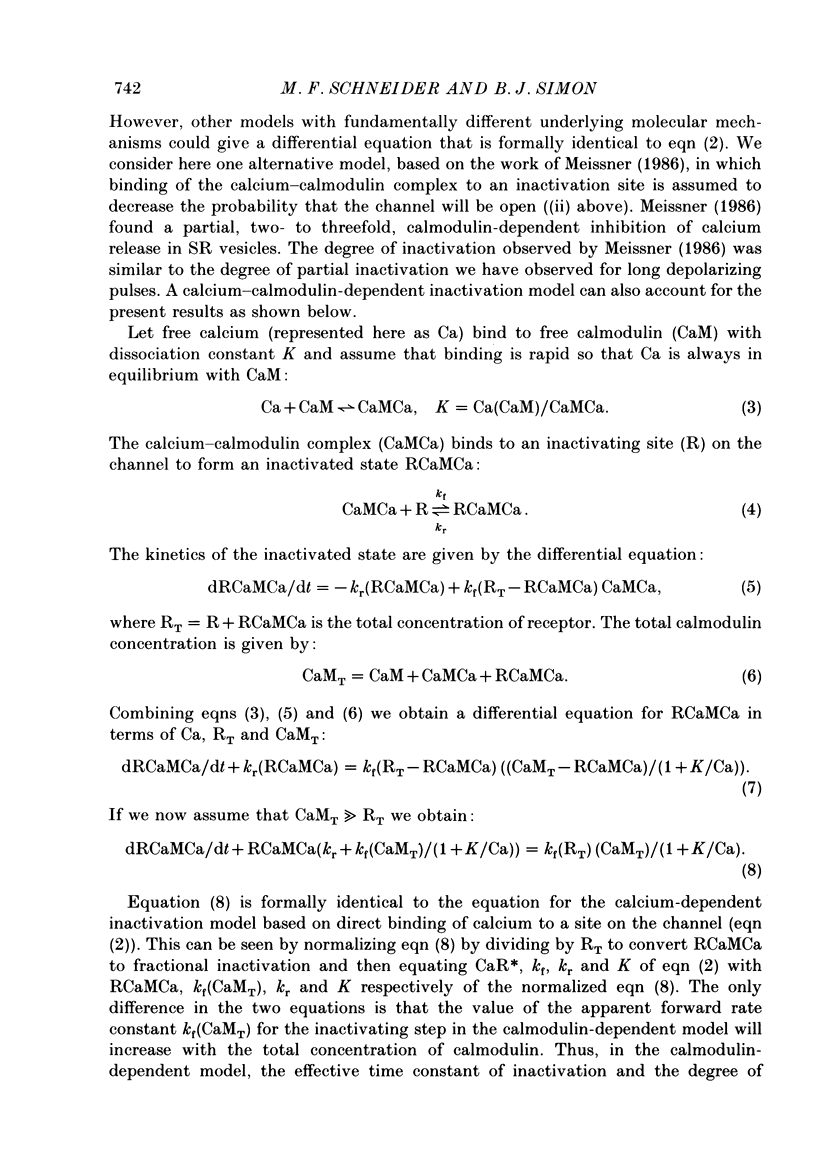
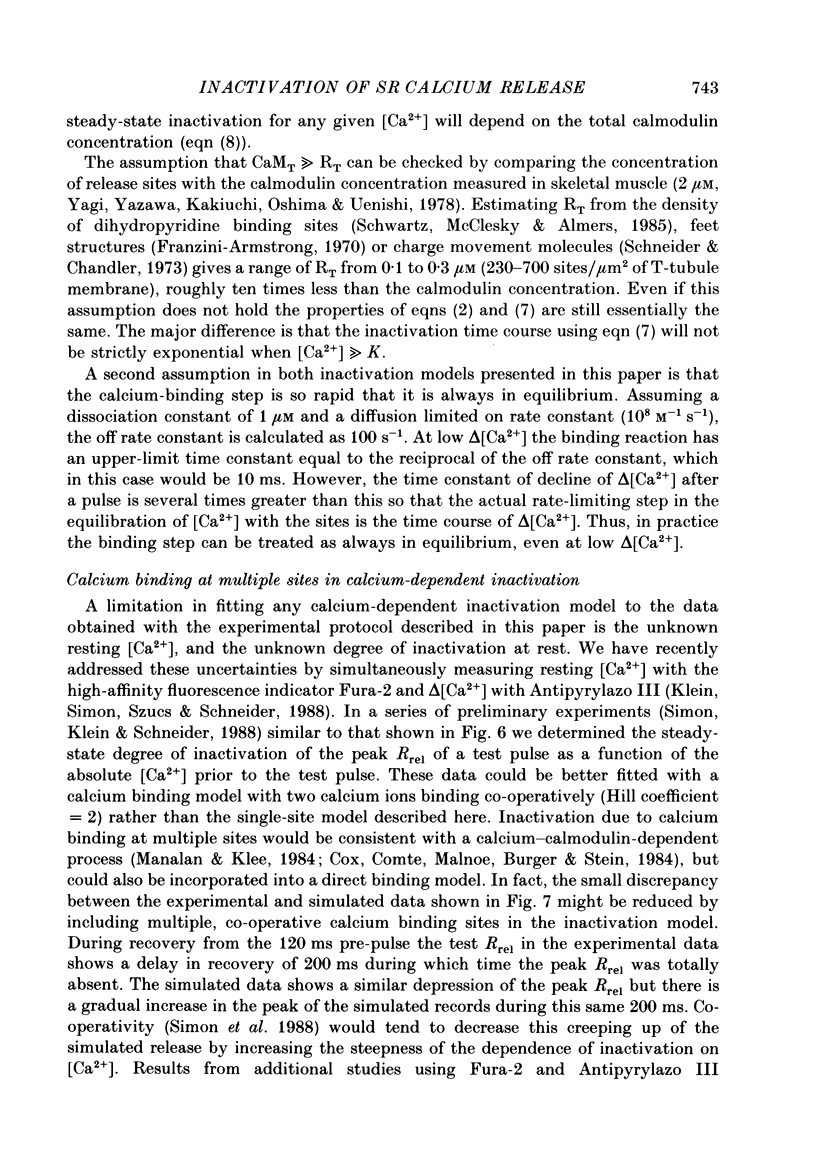
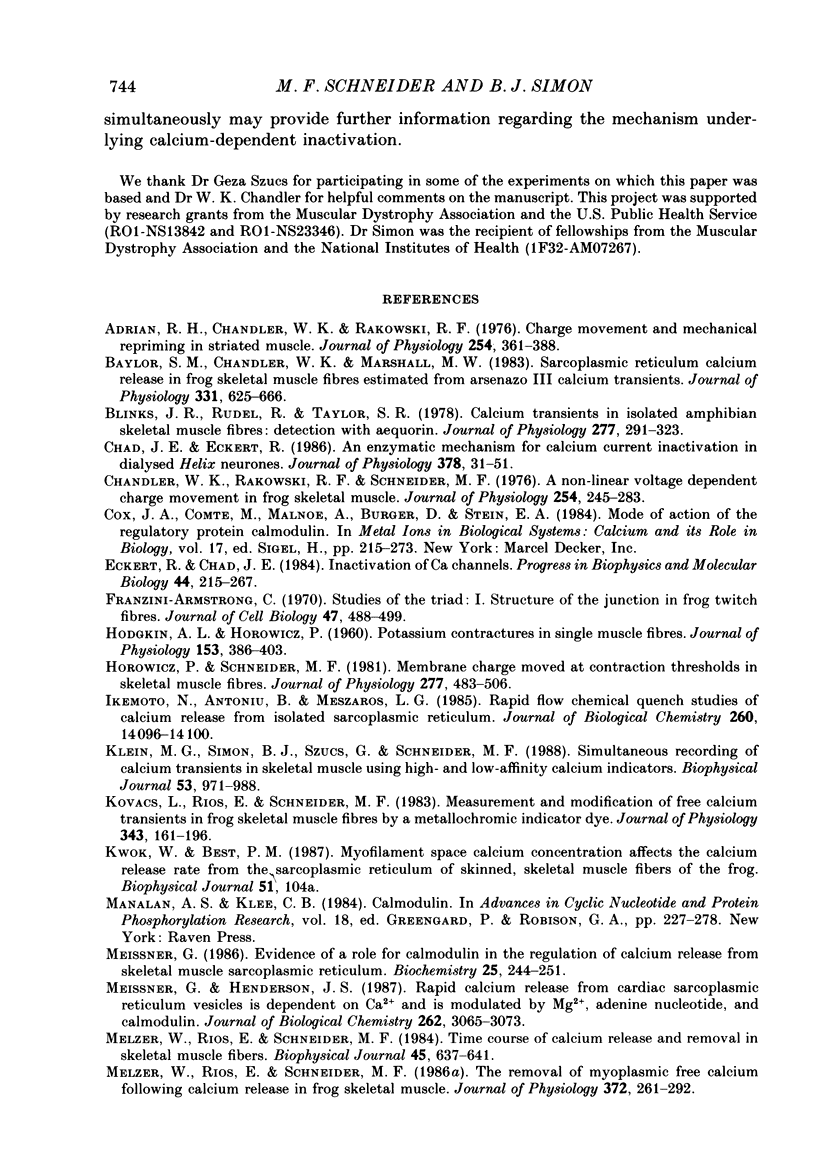
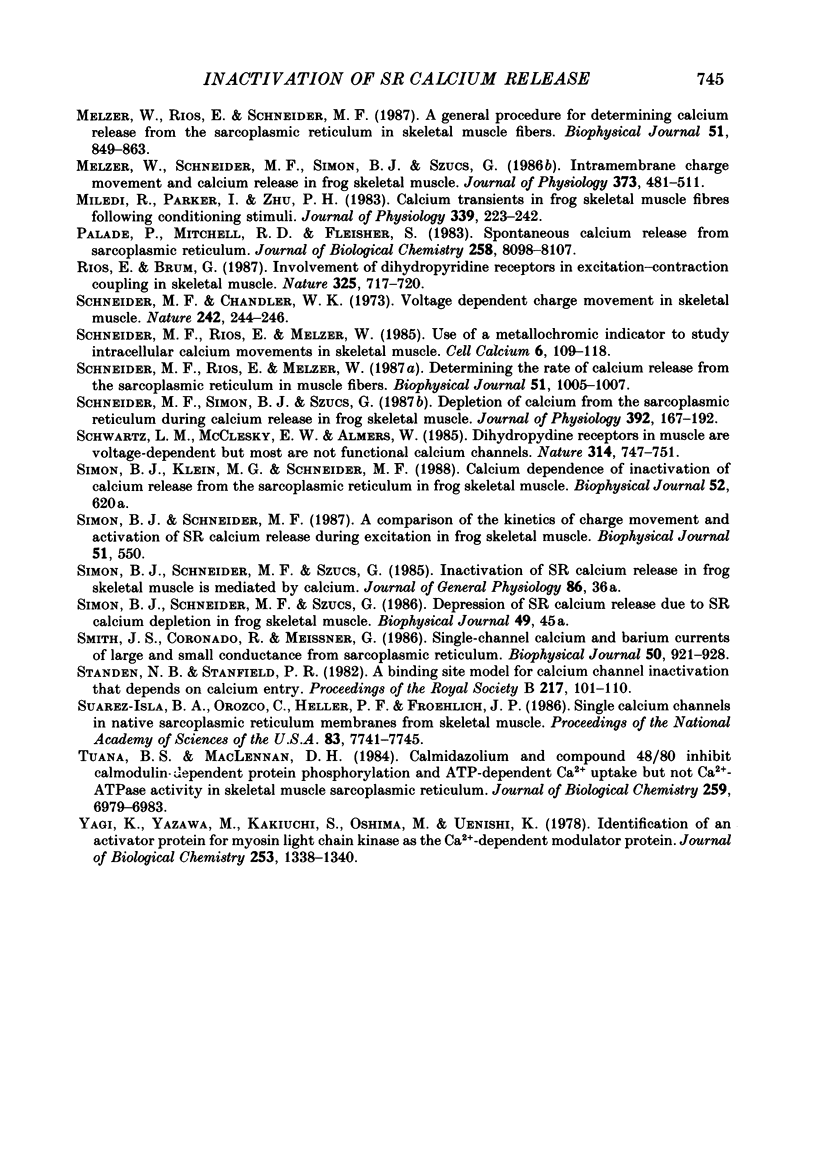
Selected References
These references are in PubMed. This may not be the complete list of references from this article.
- Adrian R. H., Chandler W. K., Rakowski R. F. Charge movement and mechanical repriming in skeletal muscle. J Physiol. 1976 Jan;254(2):361–388. doi: 10.1113/jphysiol.1976.sp011236. [DOI] [PMC free article] [PubMed] [Google Scholar]
- Baylor S. M., Chandler W. K., Marshall M. W. Sarcoplasmic reticulum calcium release in frog skeletal muscle fibres estimated from Arsenazo III calcium transients. J Physiol. 1983 Nov;344:625–666. doi: 10.1113/jphysiol.1983.sp014959. [DOI] [PMC free article] [PubMed] [Google Scholar]
- Blinks J. R., Rüdel R., Taylor S. R. Calcium transients in isolated amphibian skeletal muscle fibres: detection with aequorin. J Physiol. 1978 Apr;277:291–323. doi: 10.1113/jphysiol.1978.sp012273. [DOI] [PMC free article] [PubMed] [Google Scholar]
- Chad J. E., Eckert R. An enzymatic mechanism for calcium current inactivation in dialysed Helix neurones. J Physiol. 1986 Sep;378:31–51. doi: 10.1113/jphysiol.1986.sp016206. [DOI] [PMC free article] [PubMed] [Google Scholar]
- Chandler W. K., Rakowski R. F., Schneider M. F. A non-linear voltage dependent charge movement in frog skeletal muscle. J Physiol. 1976 Jan;254(2):245–283. doi: 10.1113/jphysiol.1976.sp011232. [DOI] [PMC free article] [PubMed] [Google Scholar]
- Eckert R., Chad J. E. Inactivation of Ca channels. Prog Biophys Mol Biol. 1984;44(3):215–267. doi: 10.1016/0079-6107(84)90009-9. [DOI] [PubMed] [Google Scholar]
- HODGKIN A. L., HOROWICZ P. Potassium contractures in single muscle fibres. J Physiol. 1960 Sep;153:386–403. doi: 10.1113/jphysiol.1960.sp006541. [DOI] [PMC free article] [PubMed] [Google Scholar]
- Ikemoto N., Antoniu B., Mészáros L. G. Rapid flow chemical quench studies of calcium release from isolated sarcoplasmic reticulum. J Biol Chem. 1985 Nov 15;260(26):14096–14100. [PubMed] [Google Scholar]
- Klein M. G., Simon B. J., Szucs G., Schneider M. F. Simultaneous recording of calcium transients in skeletal muscle using high- and low-affinity calcium indicators. Biophys J. 1988 Jun;53(6):971–988. doi: 10.1016/S0006-3495(88)83178-3. [DOI] [PMC free article] [PubMed] [Google Scholar]
- Kovacs L., Rios E., Schneider M. F. Measurement and modification of free calcium transients in frog skeletal muscle fibres by a metallochromic indicator dye. J Physiol. 1983 Oct;343:161–196. doi: 10.1113/jphysiol.1983.sp014887. [DOI] [PMC free article] [PubMed] [Google Scholar]
- Manalan A. S., Klee C. B. Calmodulin. Adv Cyclic Nucleotide Protein Phosphorylation Res. 1984;18:227–278. [PubMed] [Google Scholar]
- Meissner G. Evidence of a role for calmodulin in the regulation of calcium release from skeletal muscle sarcoplasmic reticulum. Biochemistry. 1986 Jan 14;25(1):244–251. doi: 10.1021/bi00349a034. [DOI] [PubMed] [Google Scholar]
- Meissner G., Henderson J. S. Rapid calcium release from cardiac sarcoplasmic reticulum vesicles is dependent on Ca2+ and is modulated by Mg2+, adenine nucleotide, and calmodulin. J Biol Chem. 1987 Mar 5;262(7):3065–3073. [PubMed] [Google Scholar]
- Melzer W., Rios E., Schneider M. F. A general procedure for determining the rate of calcium release from the sarcoplasmic reticulum in skeletal muscle fibers. Biophys J. 1987 Jun;51(6):849–863. doi: 10.1016/S0006-3495(87)83413-6. [DOI] [PMC free article] [PubMed] [Google Scholar]
- Melzer W., Rios E., Schneider M. F. Time course of calcium release and removal in skeletal muscle fibers. Biophys J. 1984 Mar;45(3):637–641. doi: 10.1016/S0006-3495(84)84203-4. [DOI] [PMC free article] [PubMed] [Google Scholar]
- Melzer W., Ríos E., Schneider M. F. The removal of myoplasmic free calcium following calcium release in frog skeletal muscle. J Physiol. 1986 Mar;372:261–292. doi: 10.1113/jphysiol.1986.sp016008. [DOI] [PMC free article] [PubMed] [Google Scholar]
- Melzer W., Schneider M. F., Simon B. J., Szucs G. Intramembrane charge movement and calcium release in frog skeletal muscle. J Physiol. 1986 Apr;373:481–511. doi: 10.1113/jphysiol.1986.sp016059. [DOI] [PMC free article] [PubMed] [Google Scholar]
- Miledi R., Parker I., Zhu P. H. Calcium transients in frog skeletal muscle fibres following conditioning stimuli. J Physiol. 1983 Jun;339:223–242. doi: 10.1113/jphysiol.1983.sp014713. [DOI] [PMC free article] [PubMed] [Google Scholar]
- Palade P., Mitchell R. D., Fleischer S. Spontaneous calcium release from sarcoplasmic reticulum. General description and effects of calcium. J Biol Chem. 1983 Jul 10;258(13):8098–8107. [PubMed] [Google Scholar]
- Rios E., Brum G. Involvement of dihydropyridine receptors in excitation-contraction coupling in skeletal muscle. Nature. 1987 Feb 19;325(6106):717–720. doi: 10.1038/325717a0. [DOI] [PubMed] [Google Scholar]
- Schneider M. F., Chandler W. K. Voltage dependent charge movement of skeletal muscle: a possible step in excitation-contraction coupling. Nature. 1973 Mar 23;242(5395):244–246. doi: 10.1038/242244a0. [DOI] [PubMed] [Google Scholar]
- Schneider M. F., Rios E., Melzer W. Use of a metallochromic indicator to study intracellular calcium movements in skeletal muscle. Cell Calcium. 1985 Apr;6(1-2):109–118. doi: 10.1016/0143-4160(85)90038-7. [DOI] [PubMed] [Google Scholar]
- Schneider M. F., Simon B. J., Szucs G. Depletion of calcium from the sarcoplasmic reticulum during calcium release in frog skeletal muscle. J Physiol. 1987 Nov;392:167–192. doi: 10.1113/jphysiol.1987.sp016775. [DOI] [PMC free article] [PubMed] [Google Scholar]
- Schwartz L. M., McCleskey E. W., Almers W. Dihydropyridine receptors in muscle are voltage-dependent but most are not functional calcium channels. 1985 Apr 25-May 1Nature. 314(6013):747–751. doi: 10.1038/314747a0. [DOI] [PubMed] [Google Scholar]
- Smith J. S., Coronado R., Meissner G. Single-channel calcium and barium currents of large and small conductance from sarcoplasmic reticulum. Biophys J. 1986 Nov;50(5):921–928. doi: 10.1016/S0006-3495(86)83533-0. [DOI] [PMC free article] [PubMed] [Google Scholar]
- Standen N. B., Stanfield P. R. A binding-site model for calcium channel inactivation that depends on calcium entry. Proc R Soc Lond B Biol Sci. 1982 Dec 22;217(1206):101–110. doi: 10.1098/rspb.1982.0097. [DOI] [PubMed] [Google Scholar]
- Suarez-Isla B. A., Orozco C., Heller P. F., Froehlich J. P. Single calcium channels in native sarcoplasmic reticulum membranes from skeletal muscle. Proc Natl Acad Sci U S A. 1986 Oct;83(20):7741–7745. doi: 10.1073/pnas.83.20.7741. [DOI] [PMC free article] [PubMed] [Google Scholar]
- Tuana B. S., MacLennan D. H. Calmidazolium and compound 48/80 inhibit calmodulin-dependent protein phosphorylation and ATP-dependent Ca2+ uptake but not Ca2+-ATPase activity in skeletal muscle sarcoplasmic reticulum. J Biol Chem. 1984 Jun 10;259(11):6979–6983. [PubMed] [Google Scholar]
- Yagi K., Yazawa M., Kakiuchi S., Ohshima M., Uenishi K. Identification of an activator protein for myosin light chain kinase as the Ca2+-dependent modulator protein. J Biol Chem. 1978 Mar 10;253(5):1338–1340. [PubMed] [Google Scholar]