Abstract
1. Calcium current (ICa) was recorded in single rat heart cells at two periods during development: (1) at 2-7 days post-partum (neonatal), and (2) at 6-8 weeks (adult). 2. We measured both transient and steady-state components of ICa and could describe ICa in terms of the steady-state activation (d infinity) and inactivation (f infinity) parameters, the channel reversal potential (Echannel) and a relative conductance parameter, gr. 3. In adult single cells, the application of ryanodine (10 microM), an agent known to alter the function of the sarcoplasmic reticulum (SR), abolished contraction rapidly and increased ICa. Ryanodine also produced a 13 mV shift in f infinity towards more positive potentials and altered its slope, while producing a small increase in gr but no effect on d infinity. In neonatal single cells, ryanodine (10 microM) had no significant effect on contraction, ICa, d infinity, f infinity, or gr. Caffeine (10 mM), a less specific agent widely used to investigate sarcoplasmic reticulum function, had actions similar to those of ryanodine. 4. In adult myocytes, when EGTA (10 or 20 mM) or bis(o-aminophenoxy)ethane-N,N,N',N'-tetraacetic acid (BAPTA, 10 mM) were included in the pipette solution, contractions were rapidly abolished, while a small (4 mV) shift of f infinity to more positive potentials was seen. A large additional shift of f infinity was observed when ryanodine (10 microM) was added to the superfusion solution in the continued presence of EGTA or BAPTA. The alterations of ICa in EGTA (or BAPTA) plus ryanodine were the same as those seen in ryanodine alone. In neonatal cells, in contrast, when EGTA or BAPTA were included in the pipette solution we observed only a small effect on f infinity and the application of ryanodine had no effect. 5. Electron micrographs of our preparations show that the dissociated adult cells have sharp sarcolemmal borders, fully developed sarcomeres with T-tubules and sarcoplasmic reticulum membranes. In contrast, the neonatal cells that we use have few of these intracellular structures. Our observations in these preparations are consistent with the work of others (e.g. Penefsky, 1974; Hirakow & Gotoh, 1975; Ishikawa & Yamada, 1975; Legato, 1975; Hoerter, Mazet & Vassort, 1981). 6. Our data suggest that fully developed sarcoplasmic reticulum in rat heart cells can affect ICa.(ABSTRACT TRUNCATED AT 400 WORDS)
Full text
PDF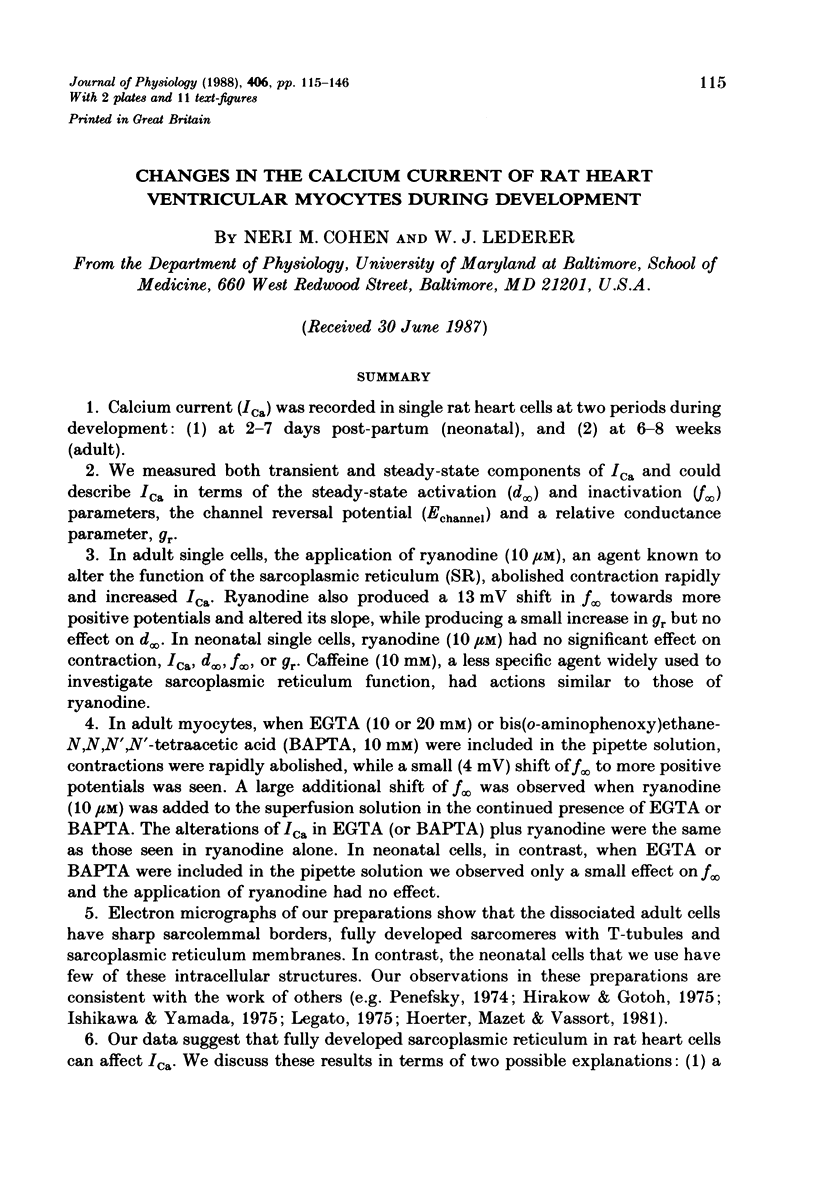
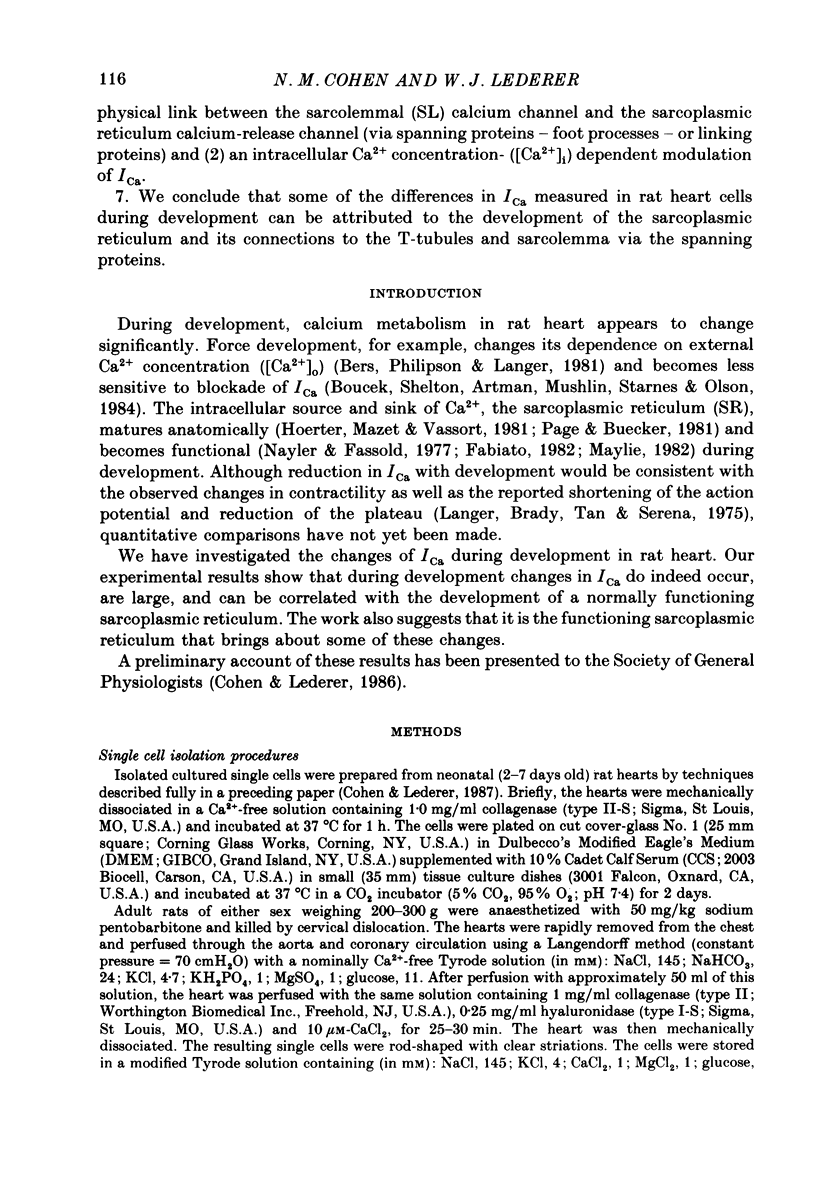
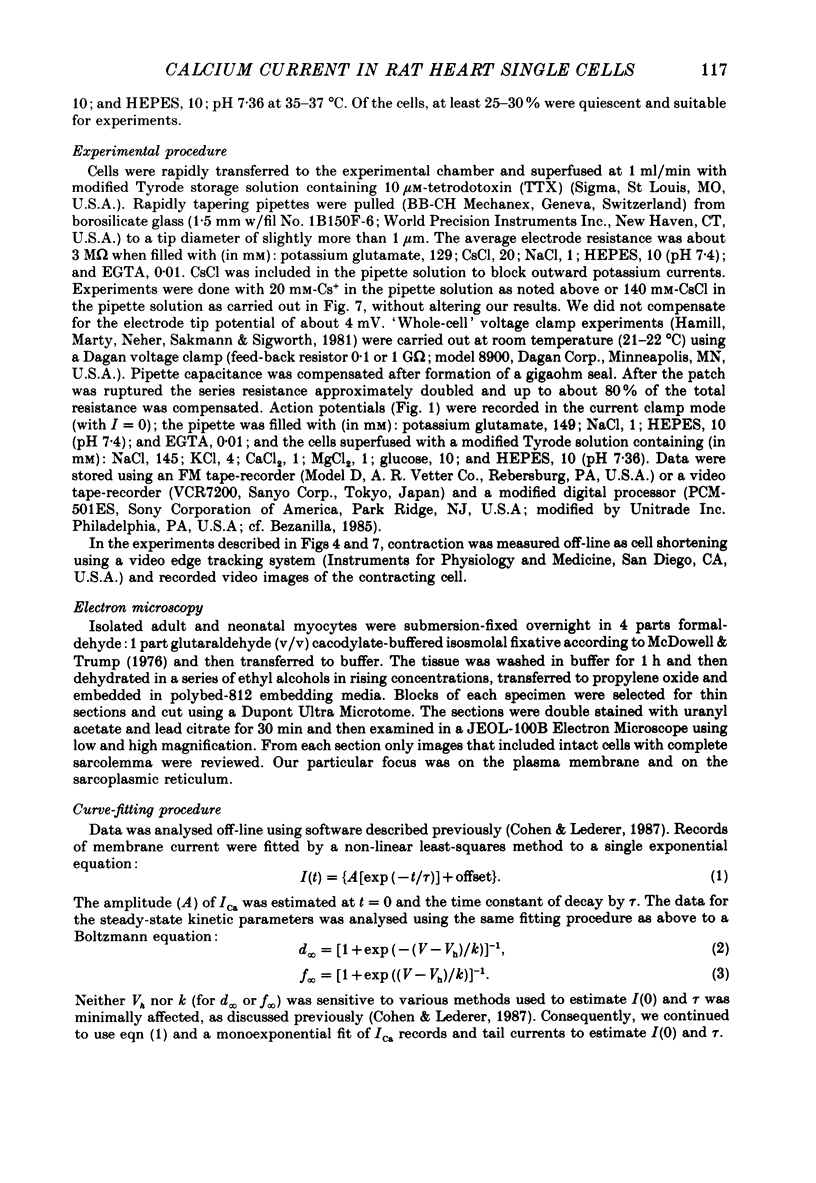
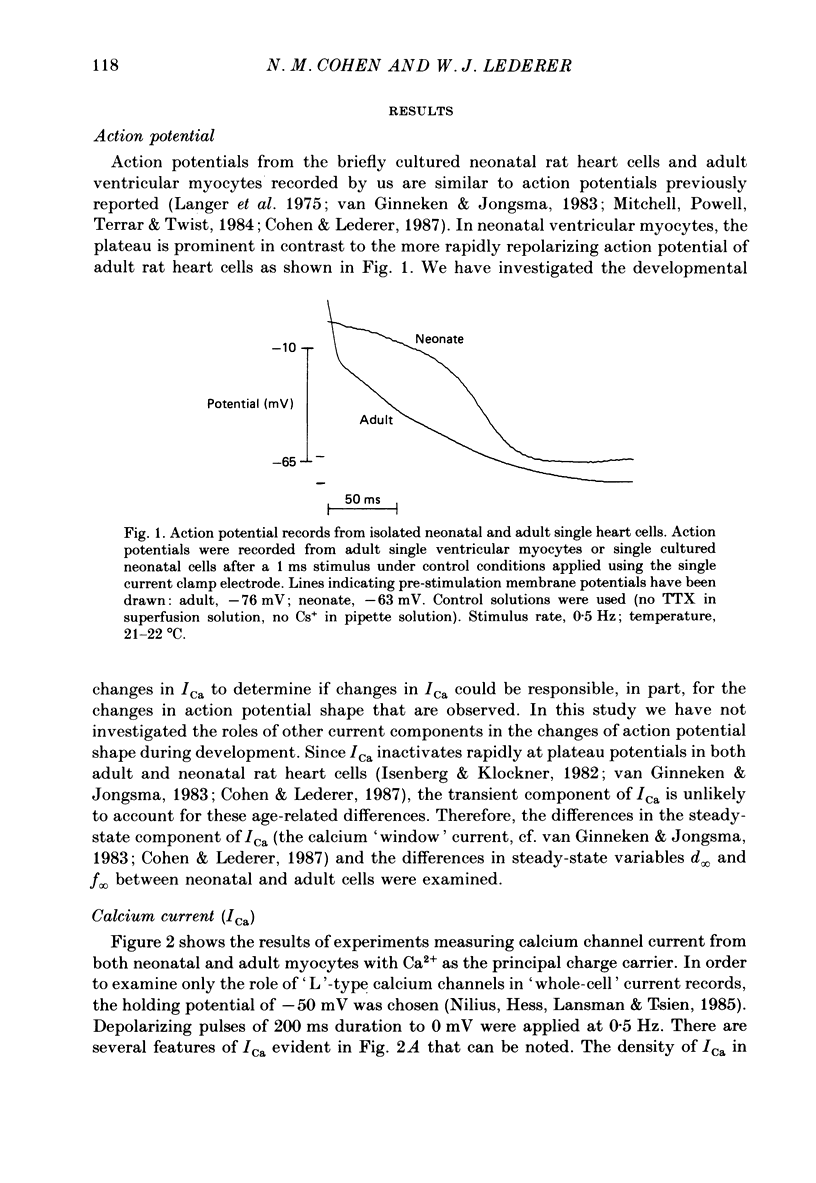
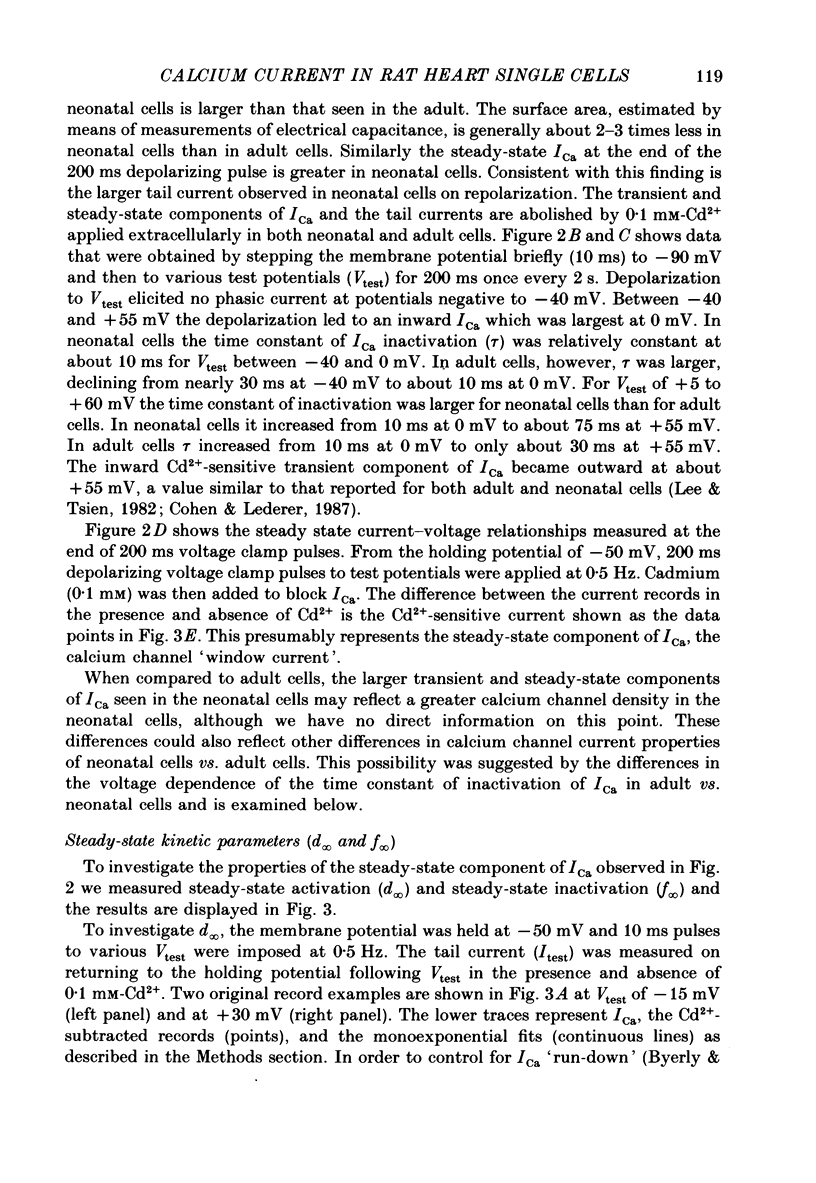
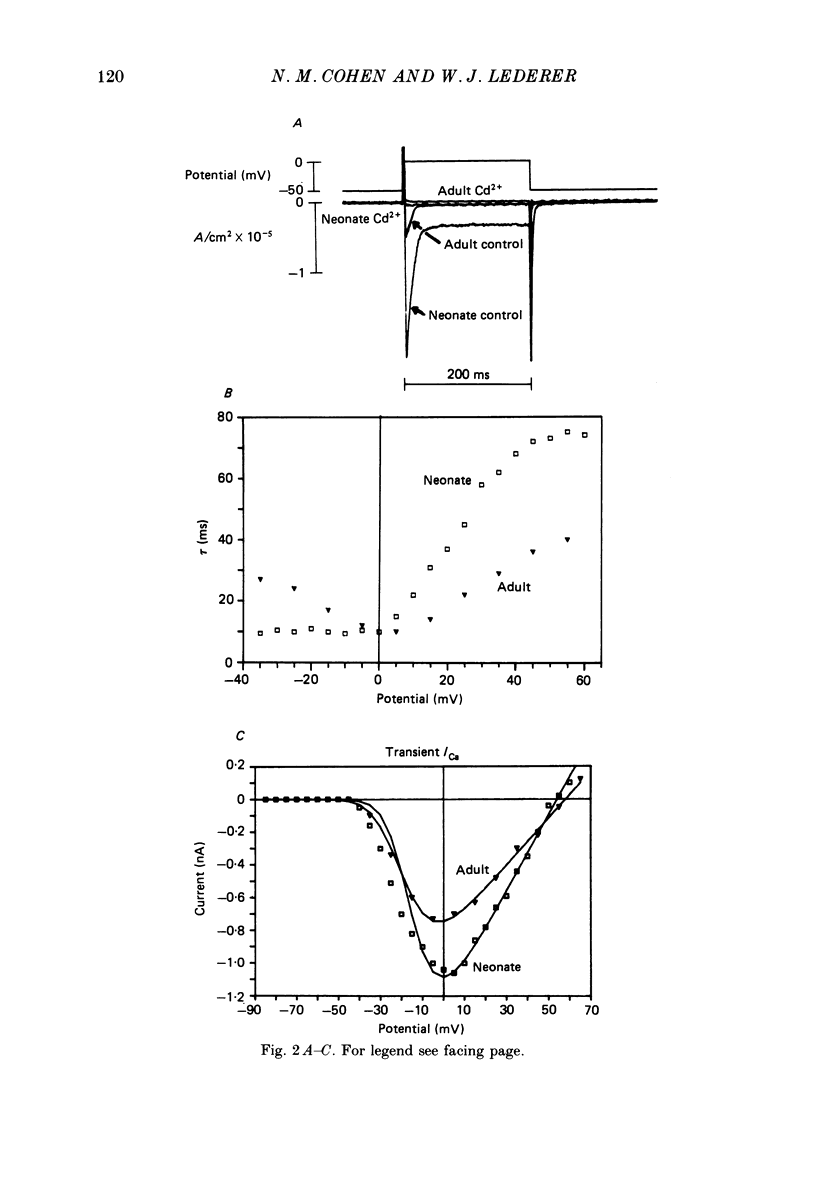
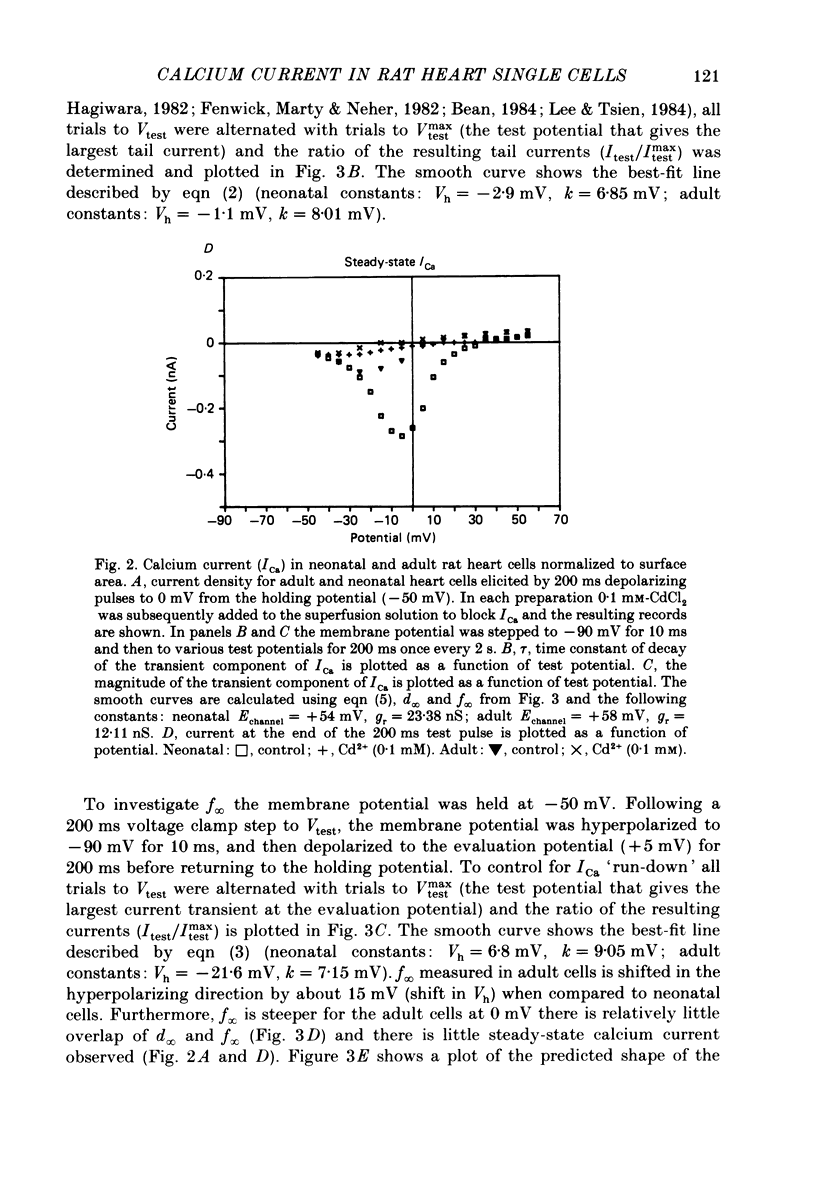
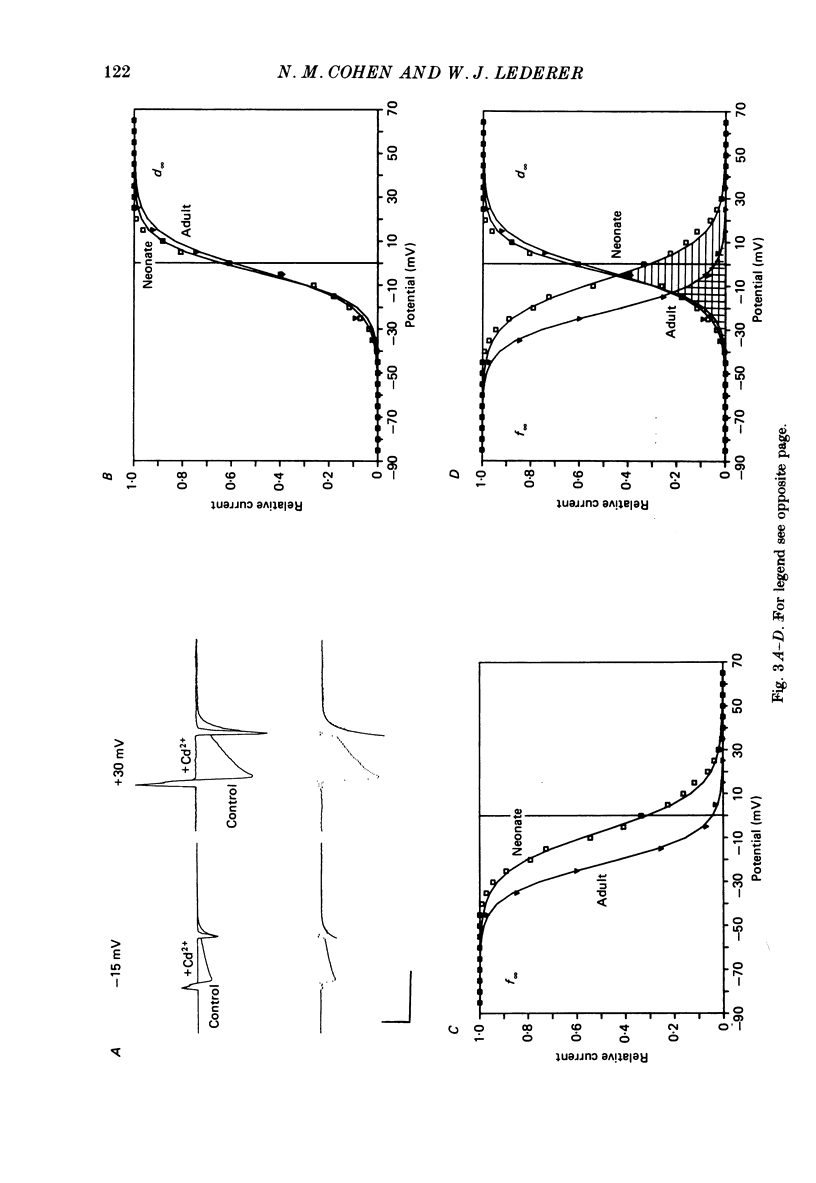
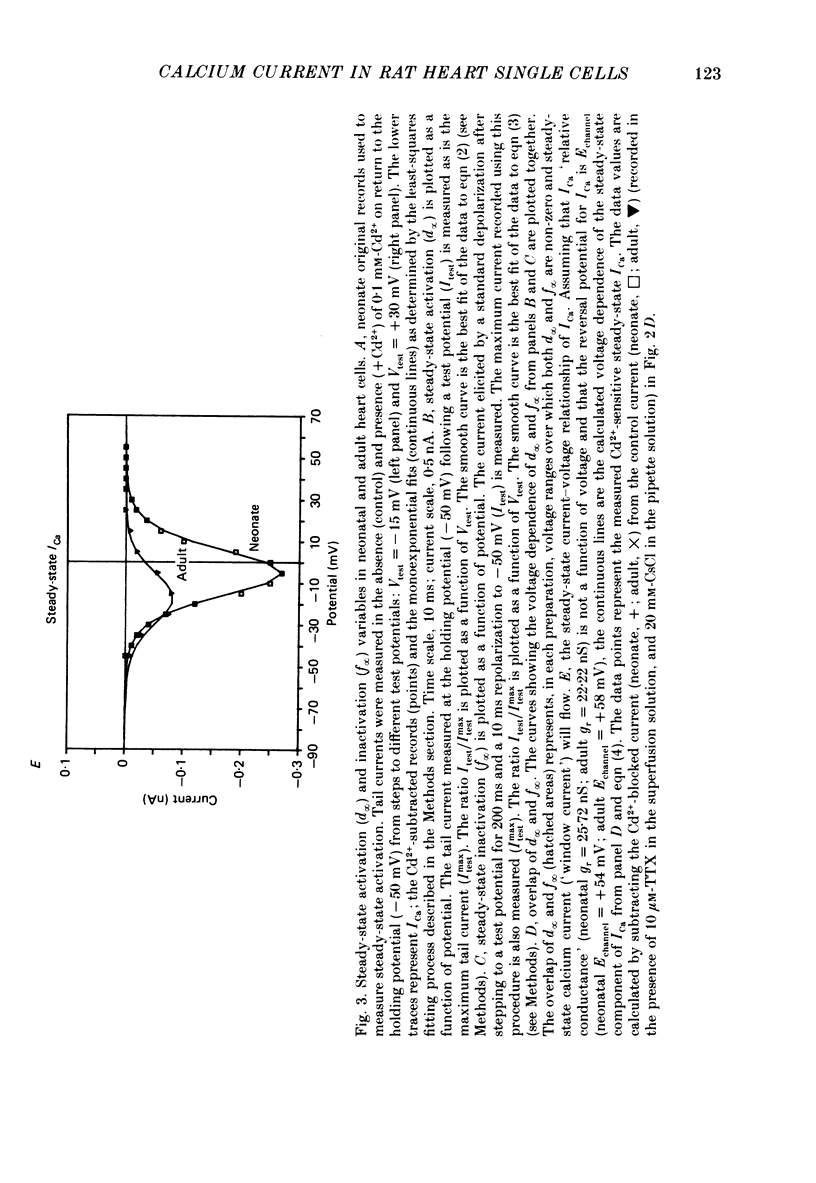
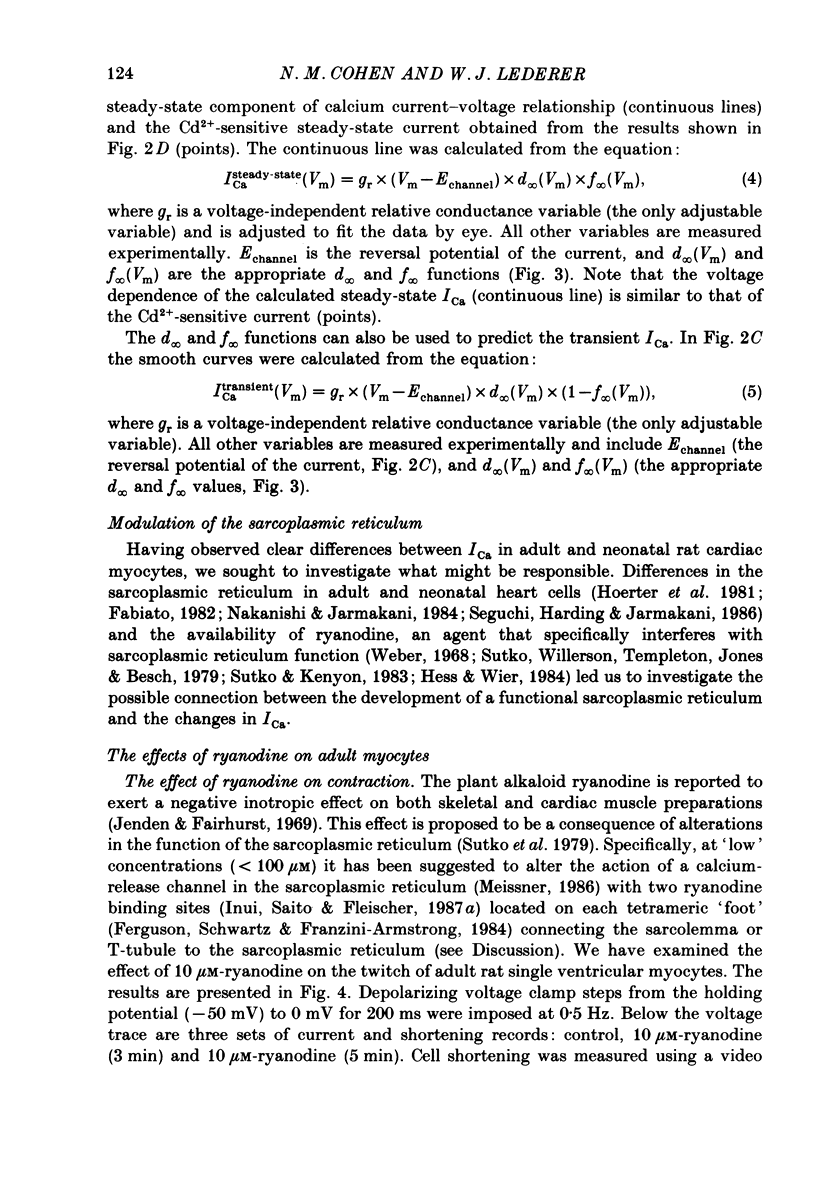
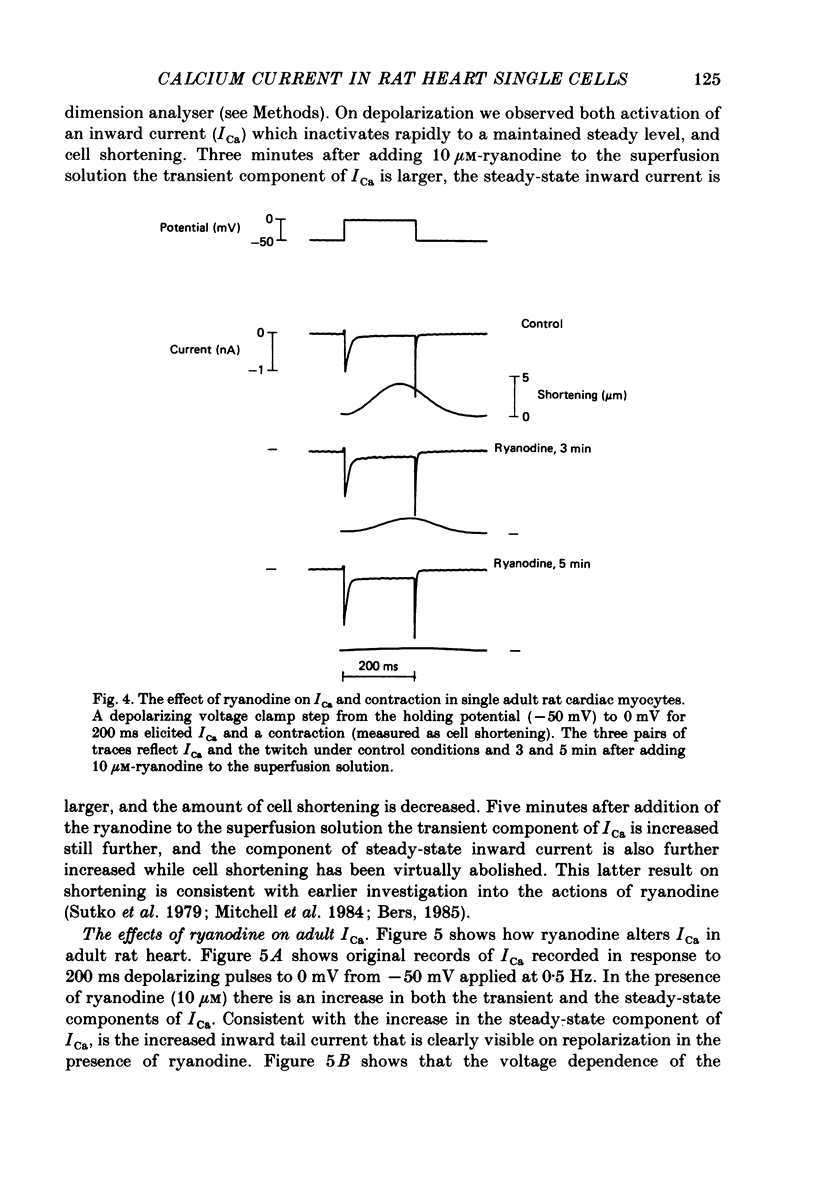
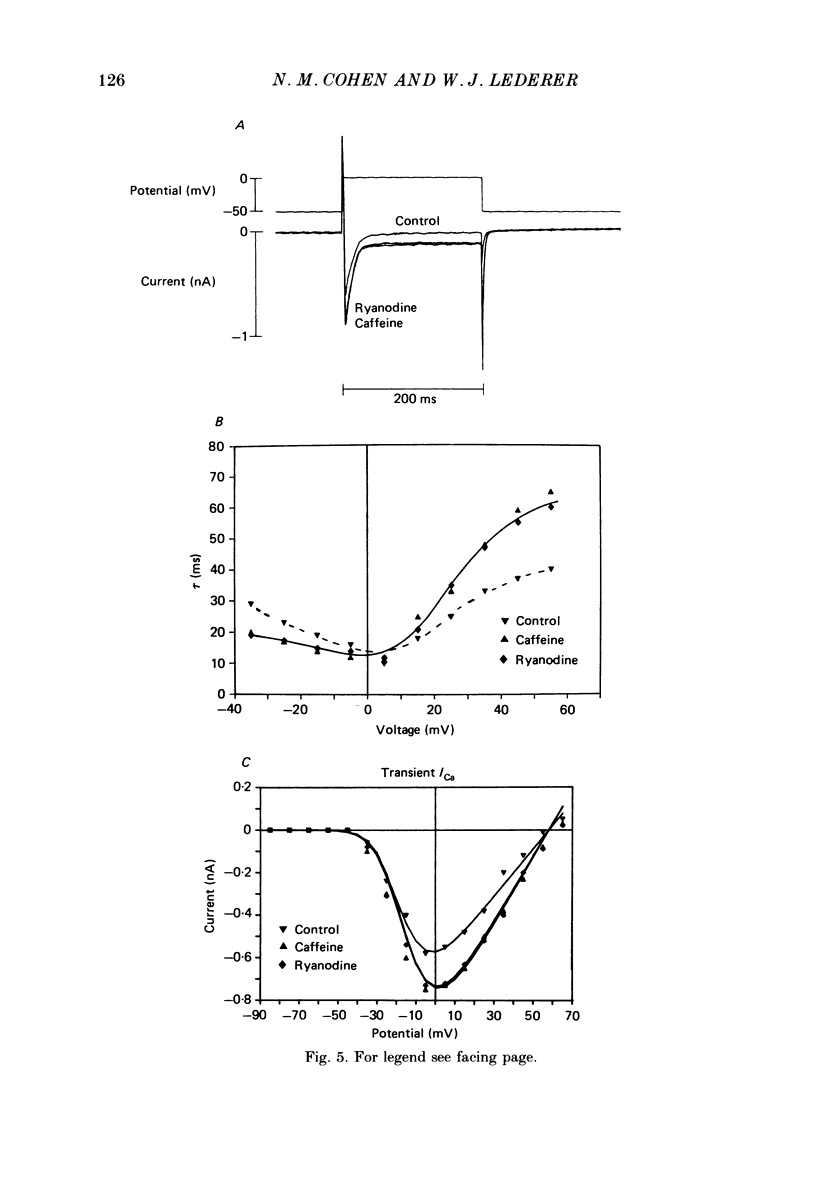
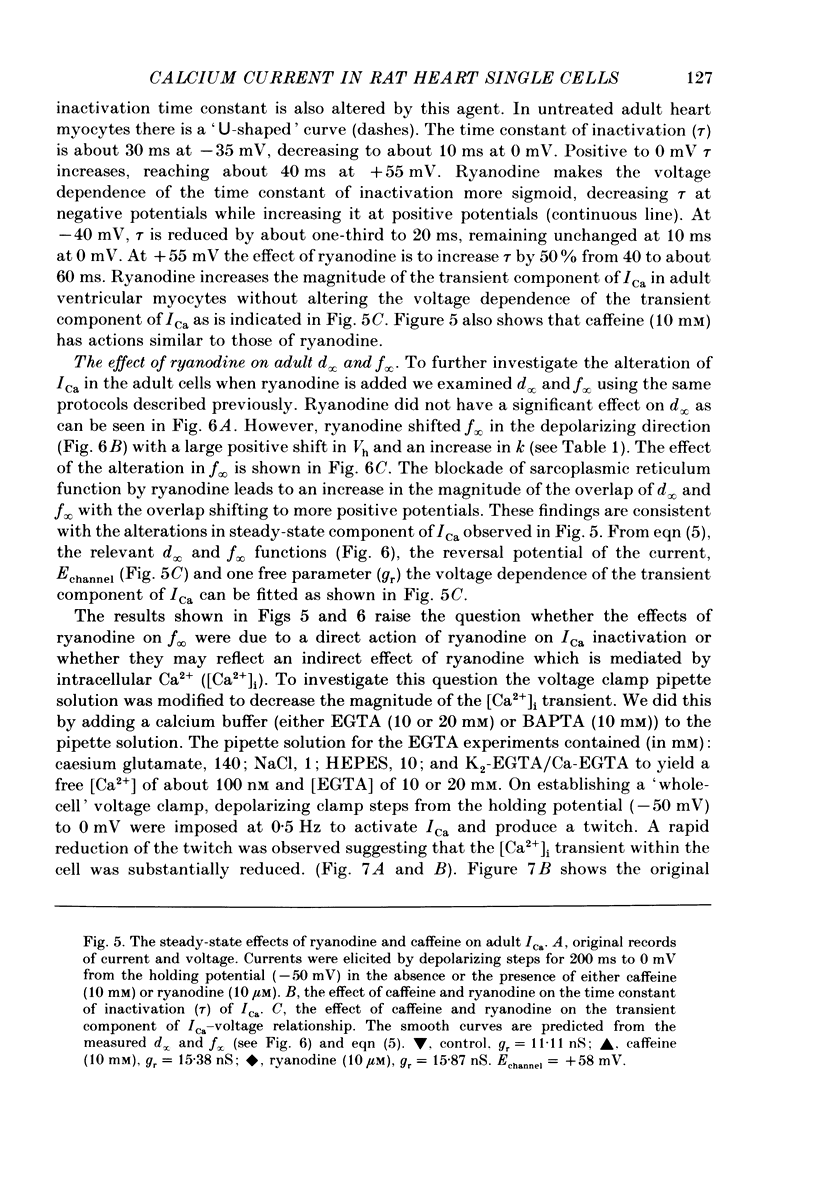
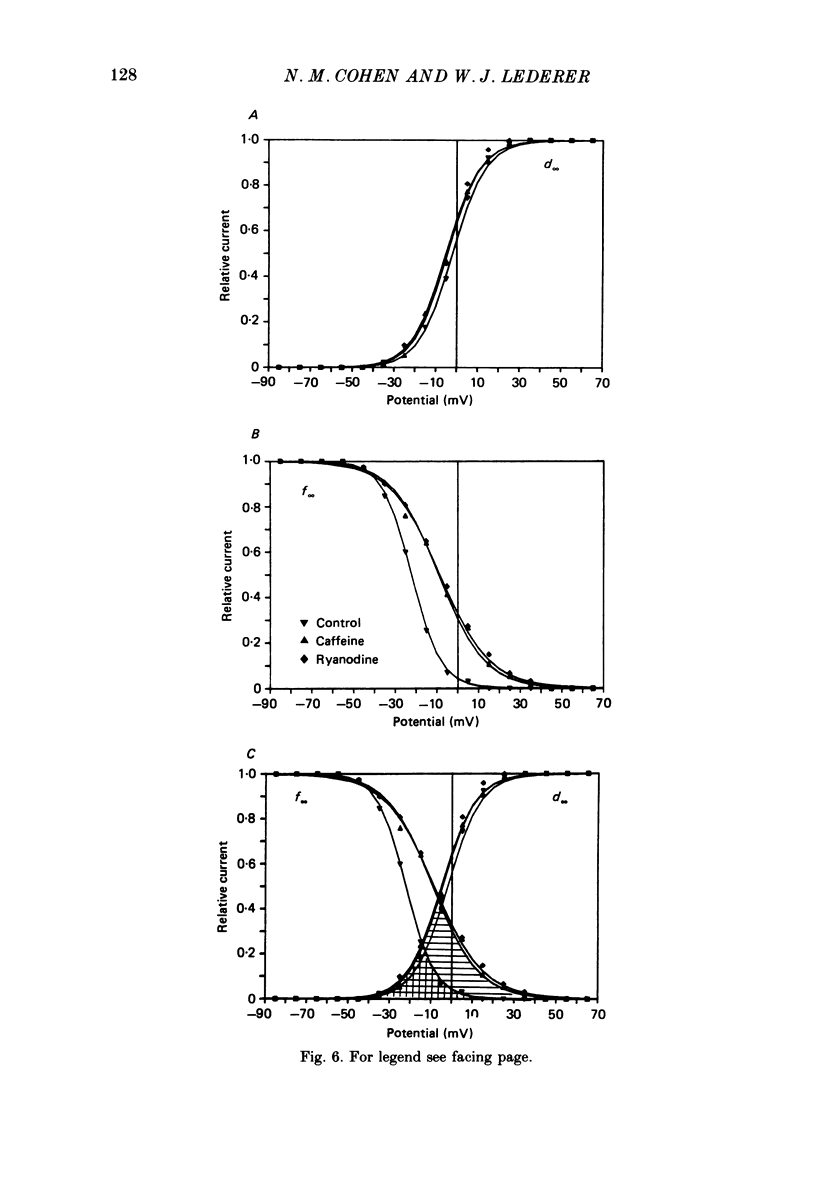
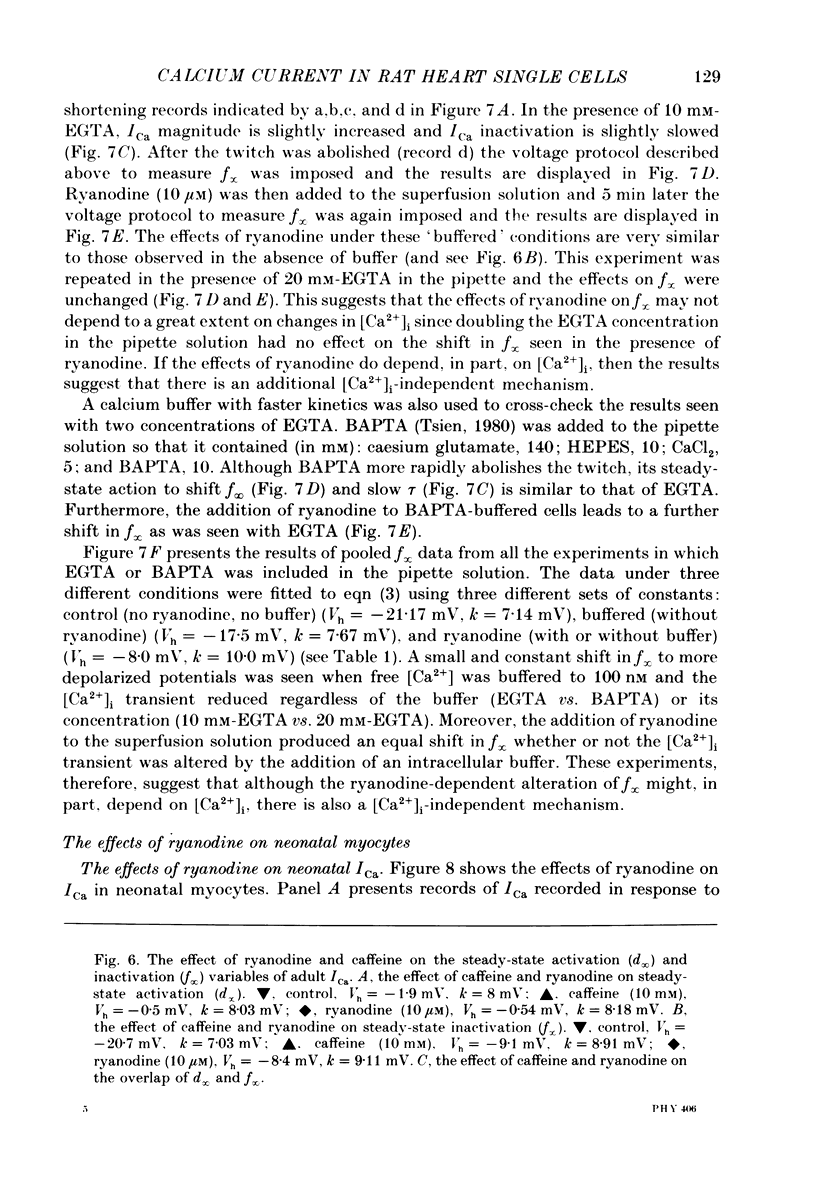
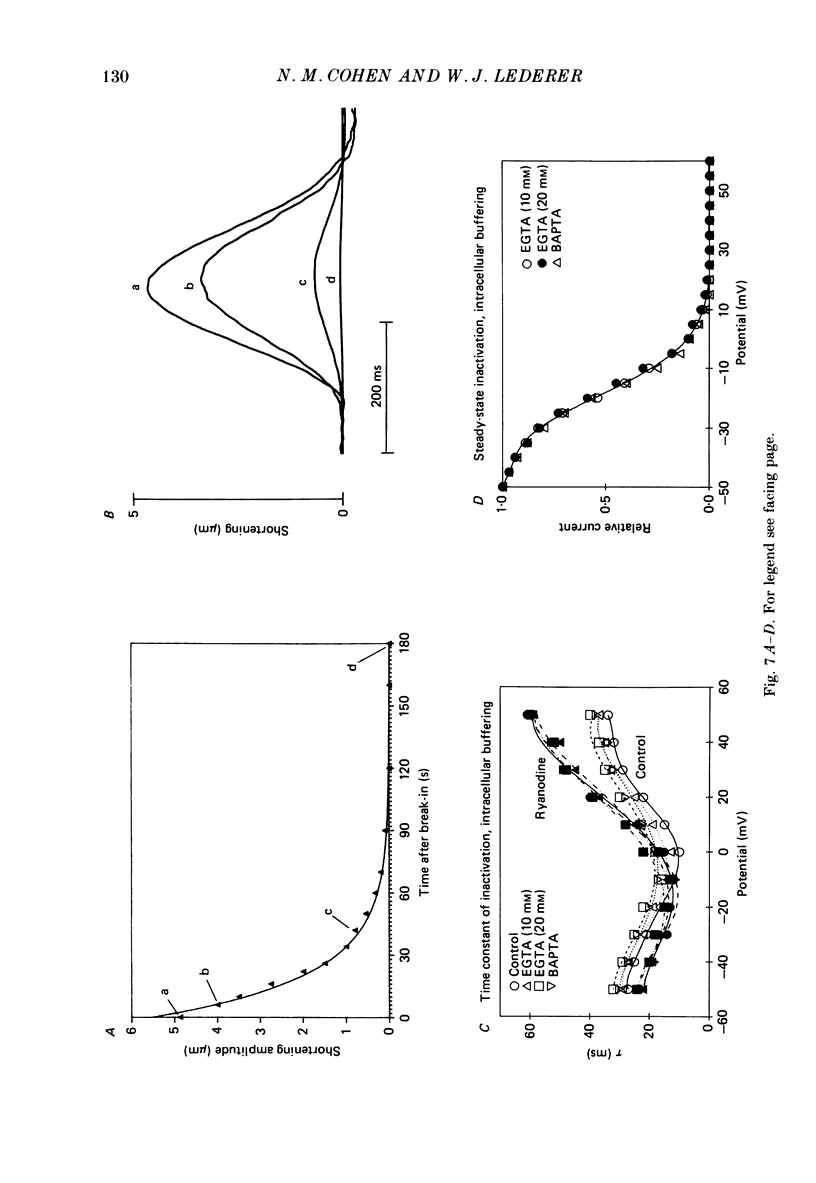
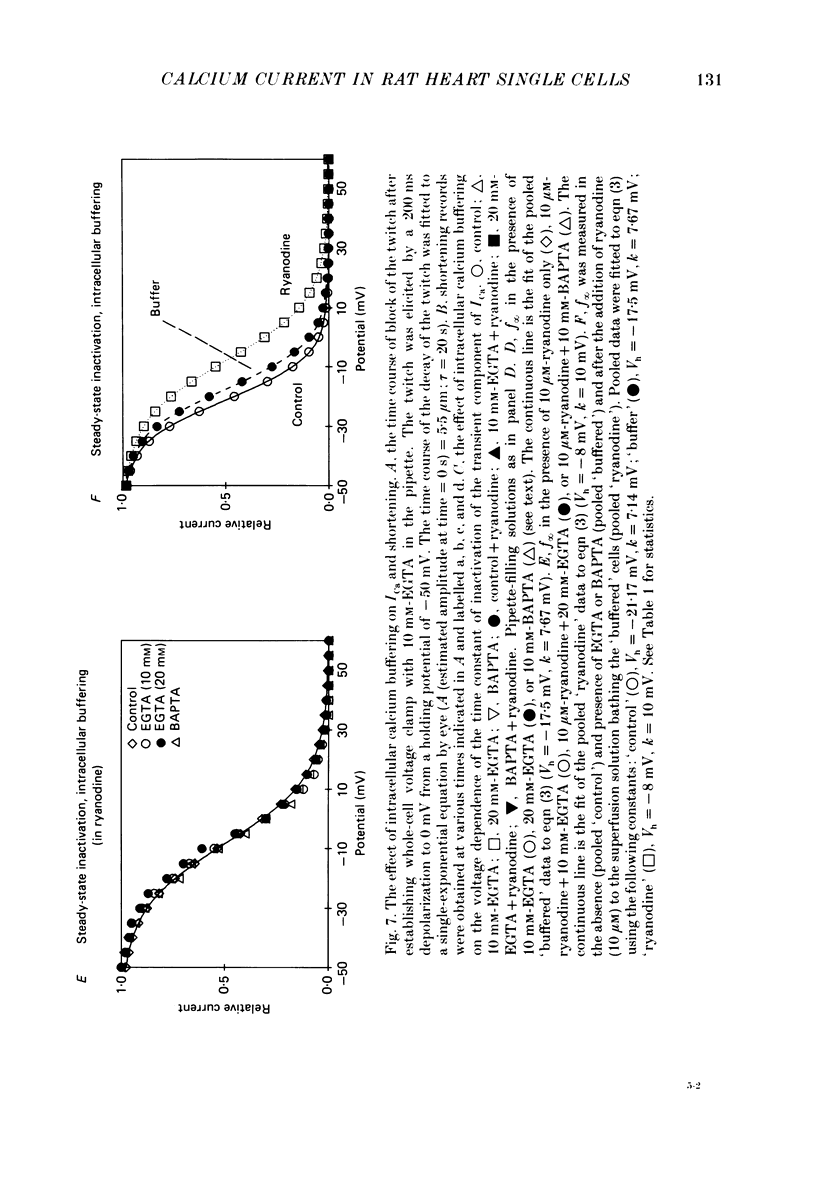
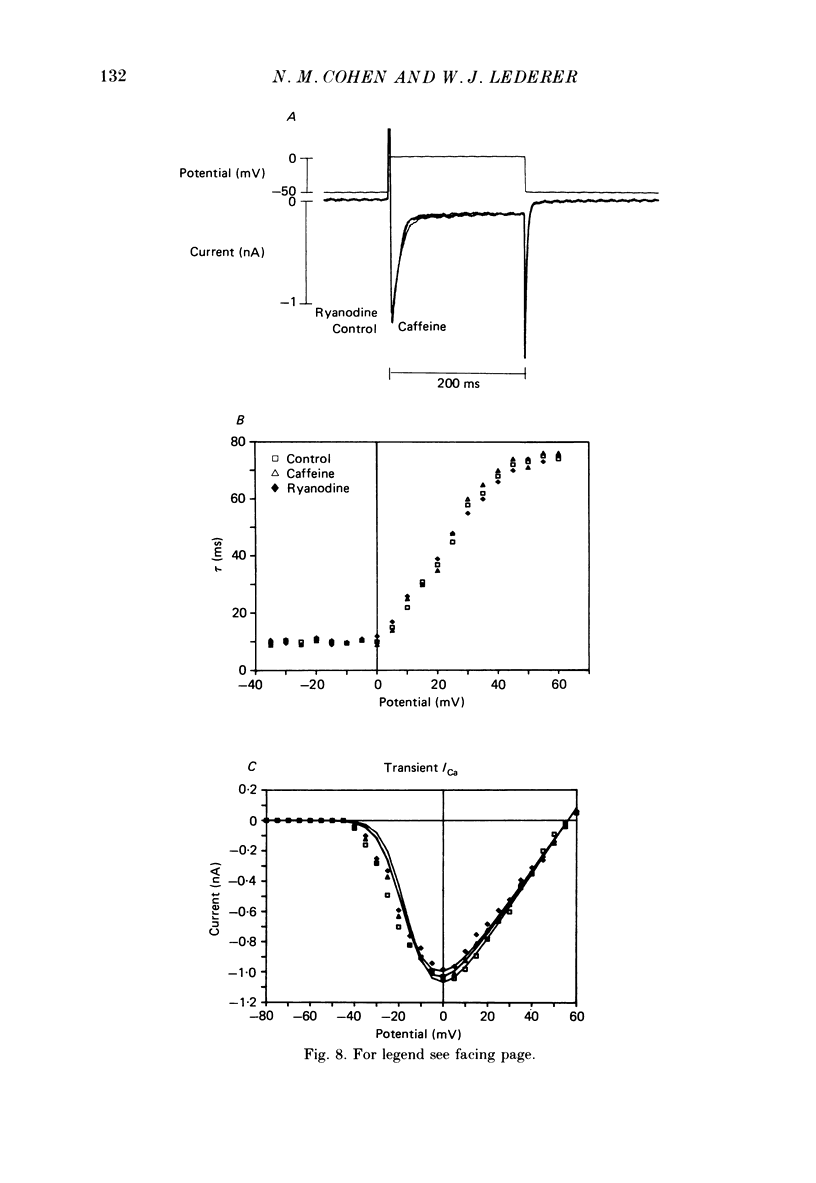
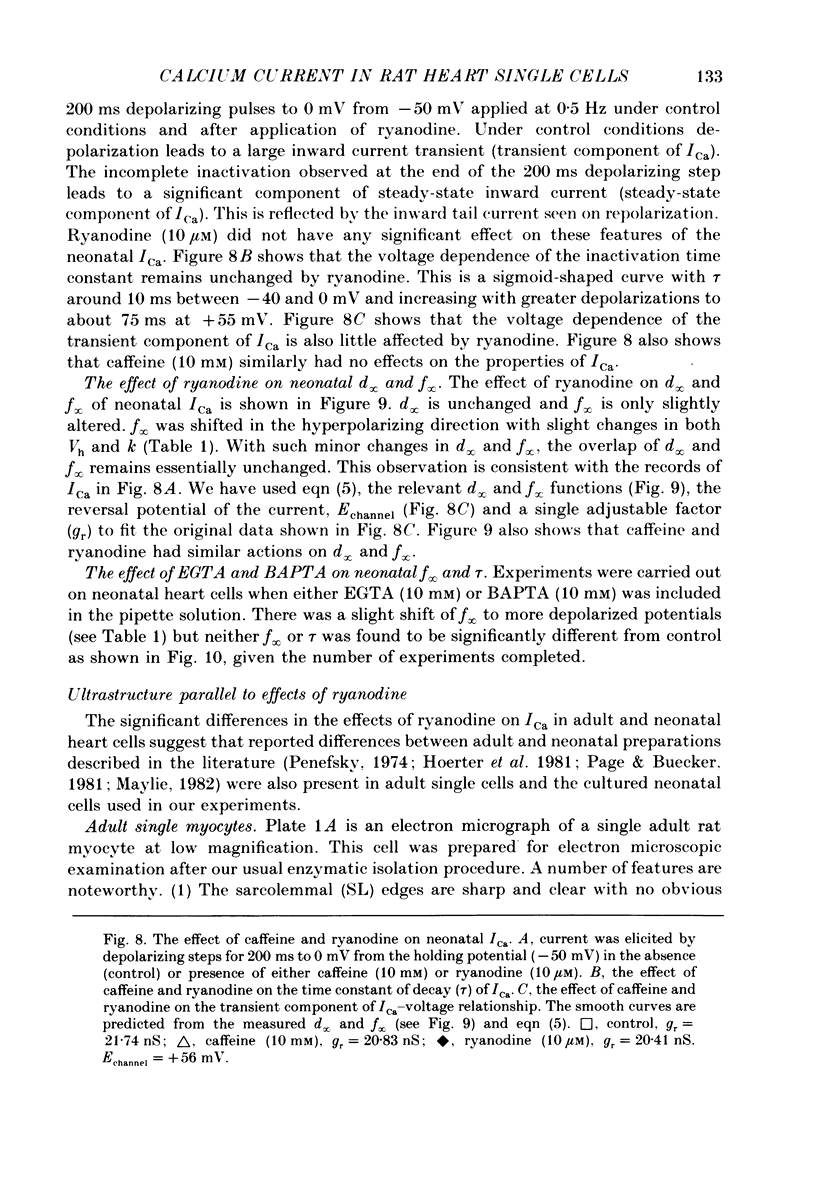
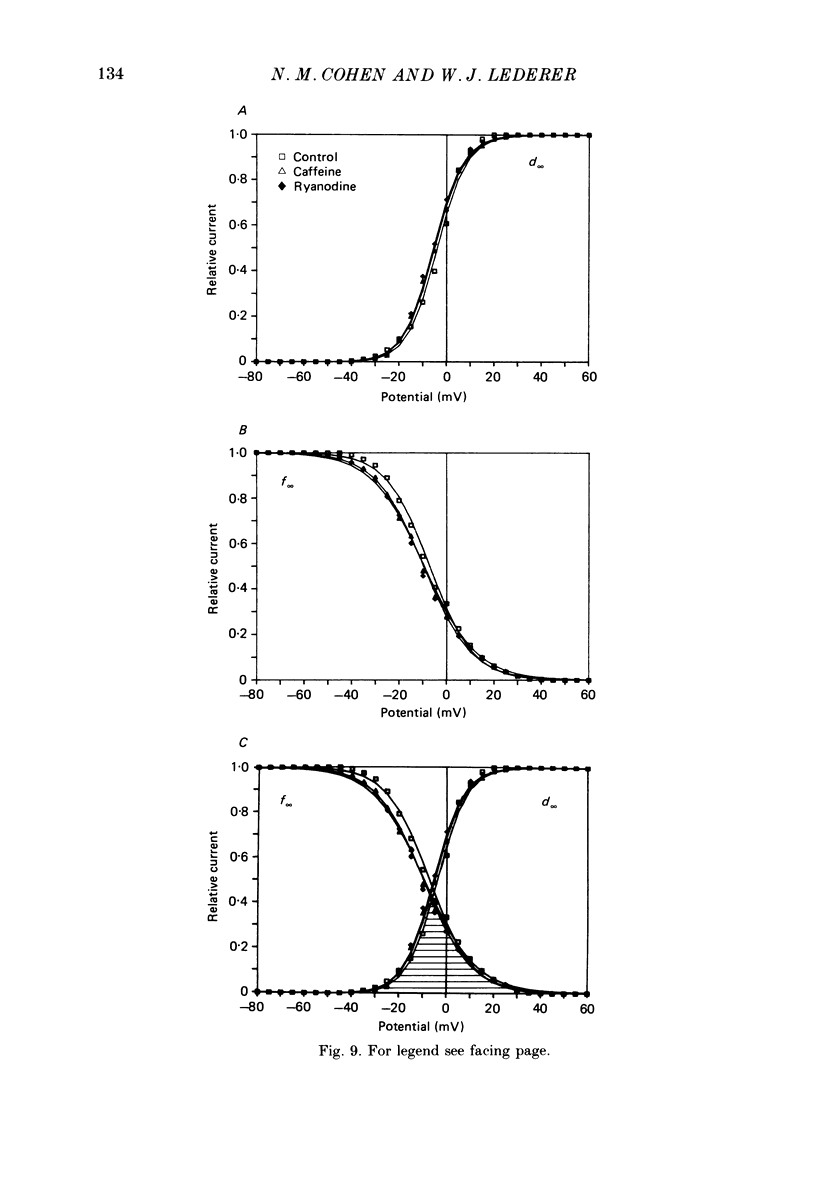
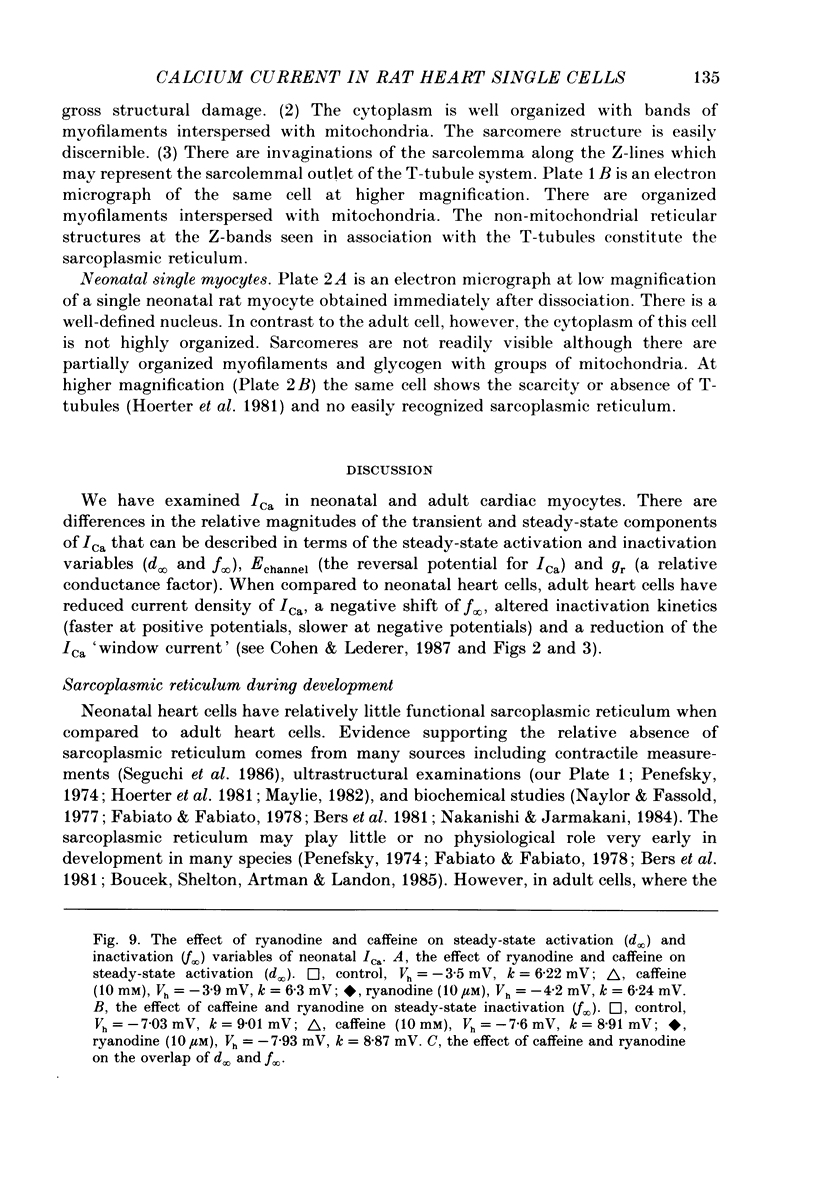
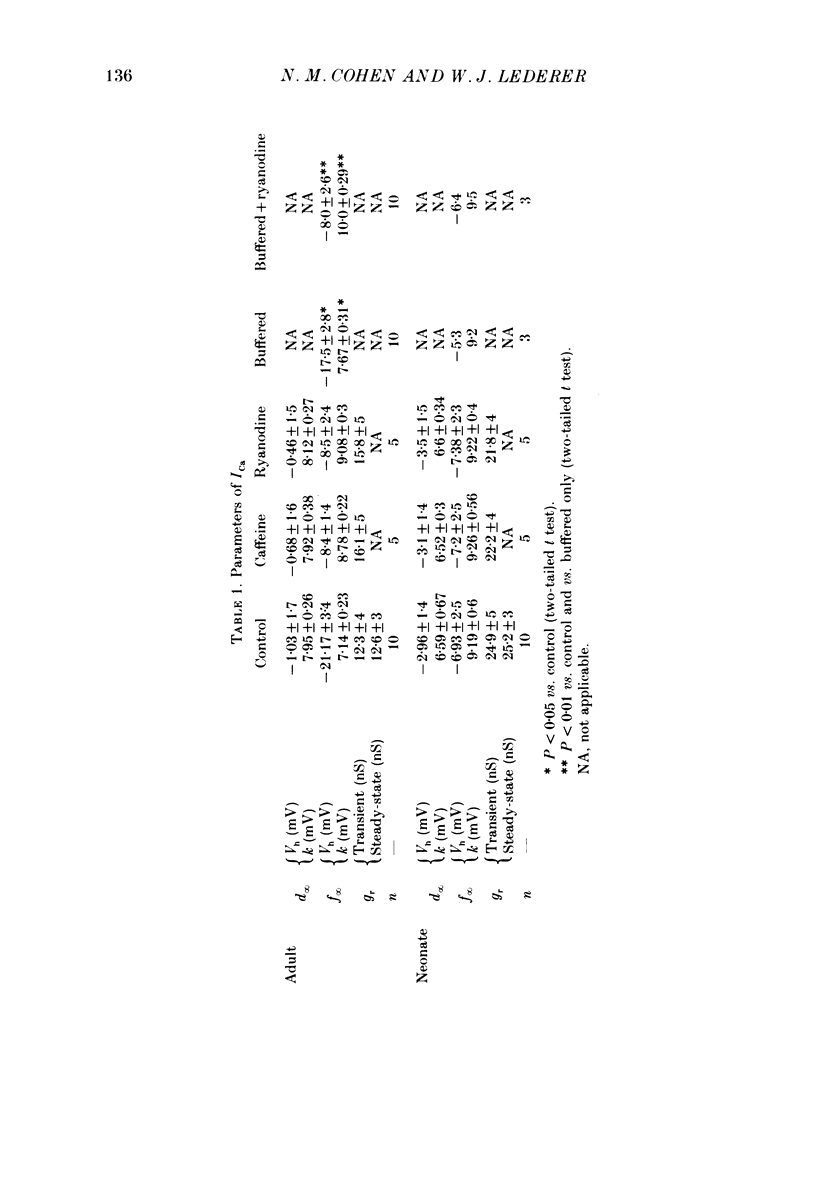
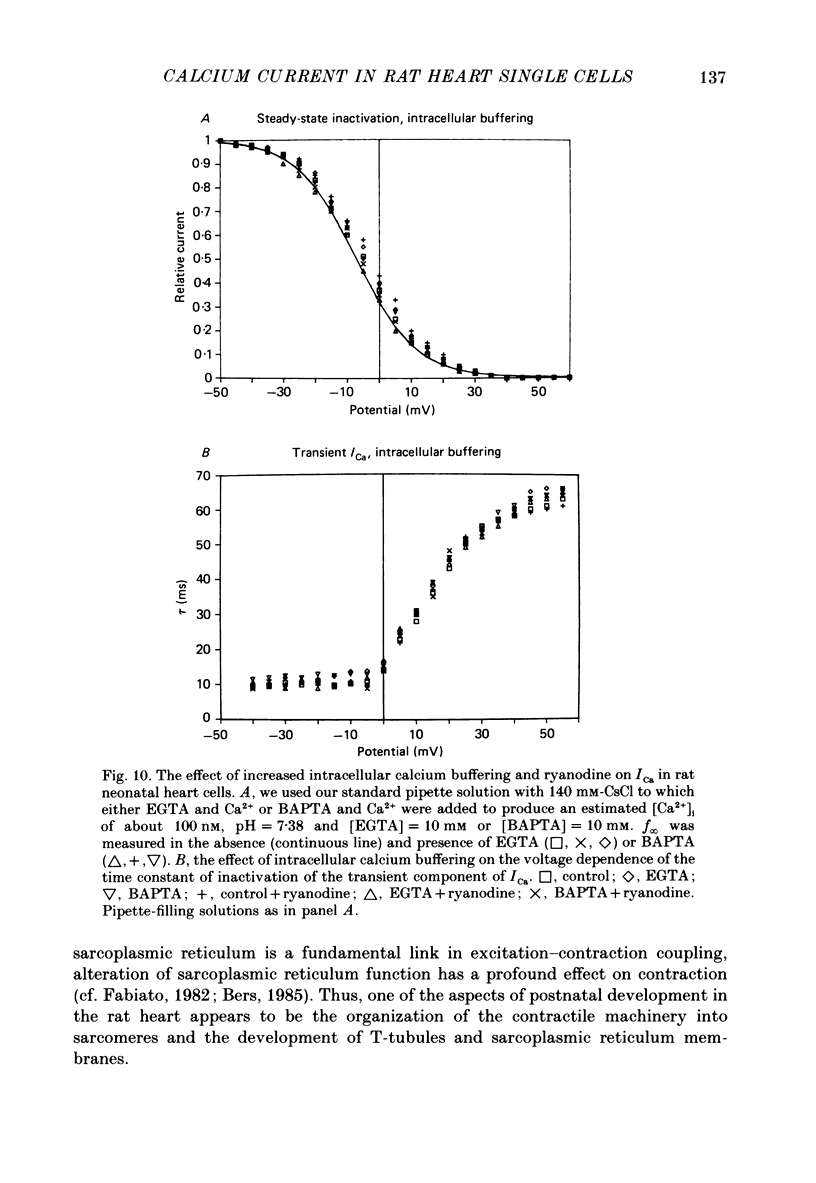
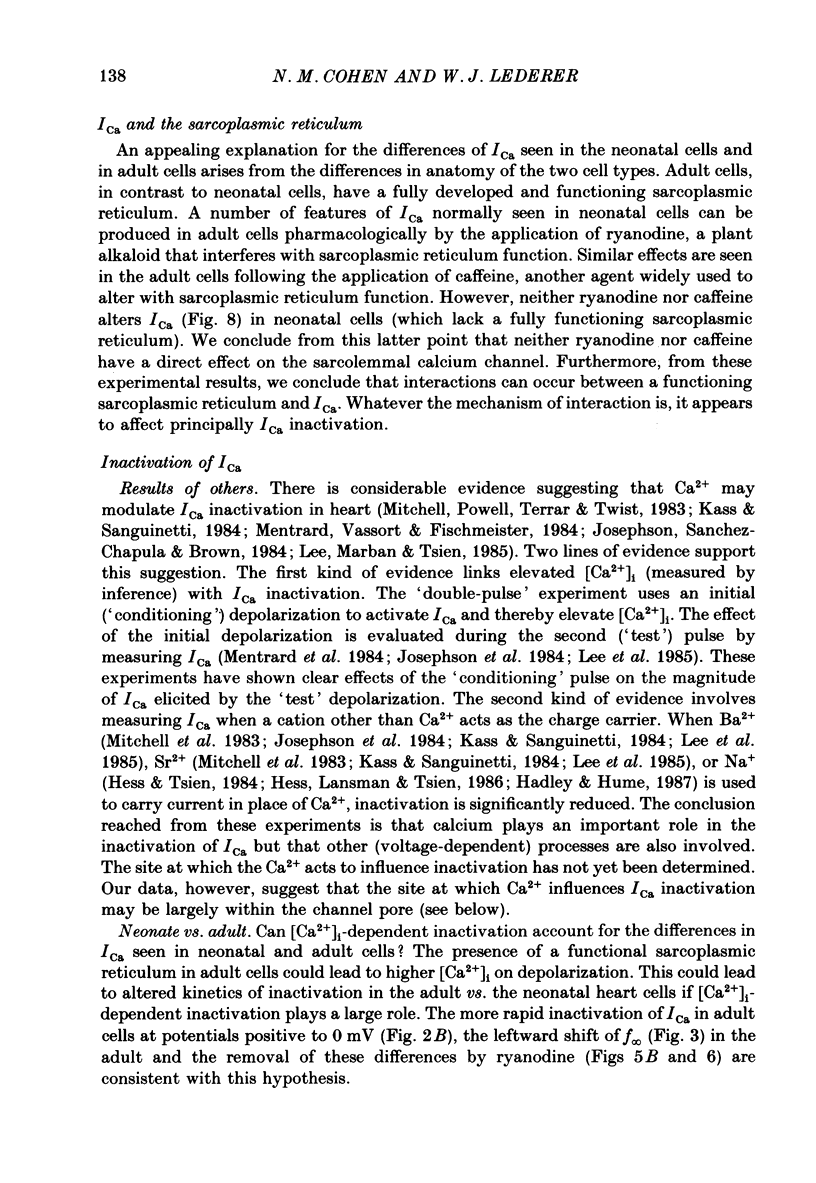
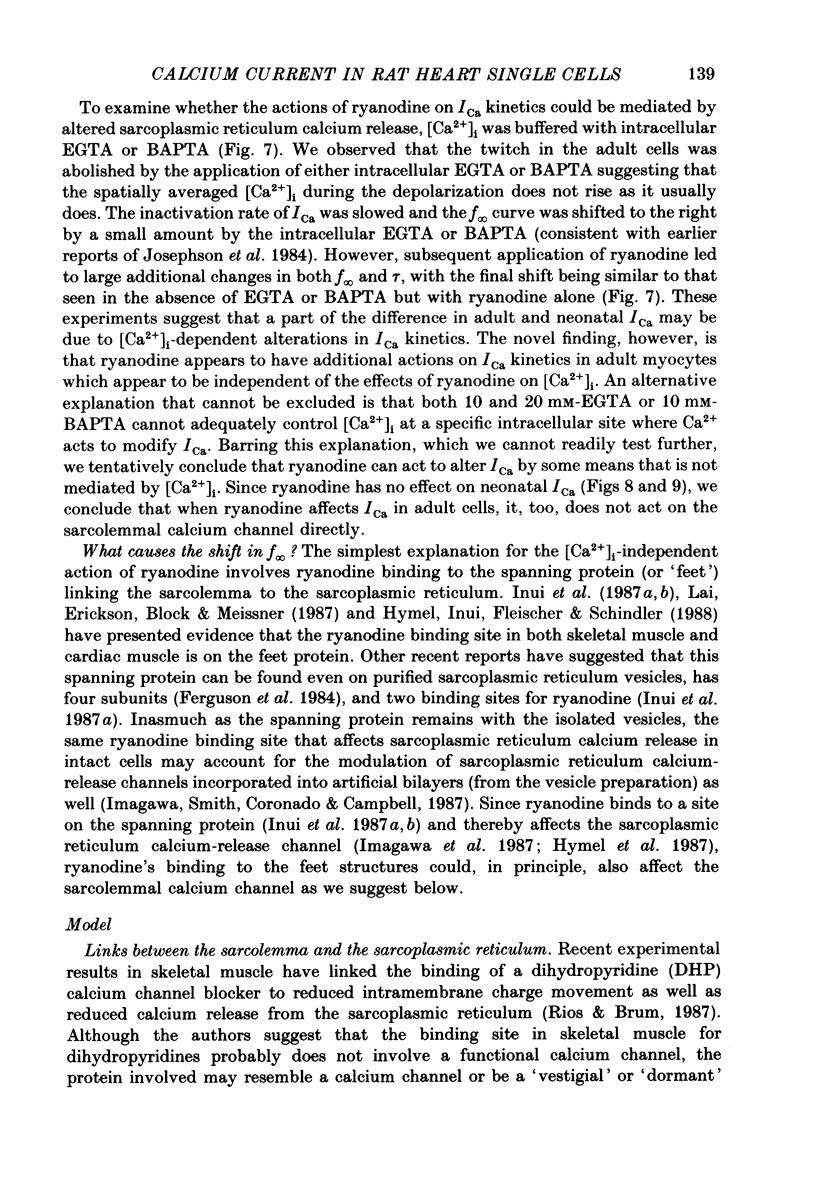
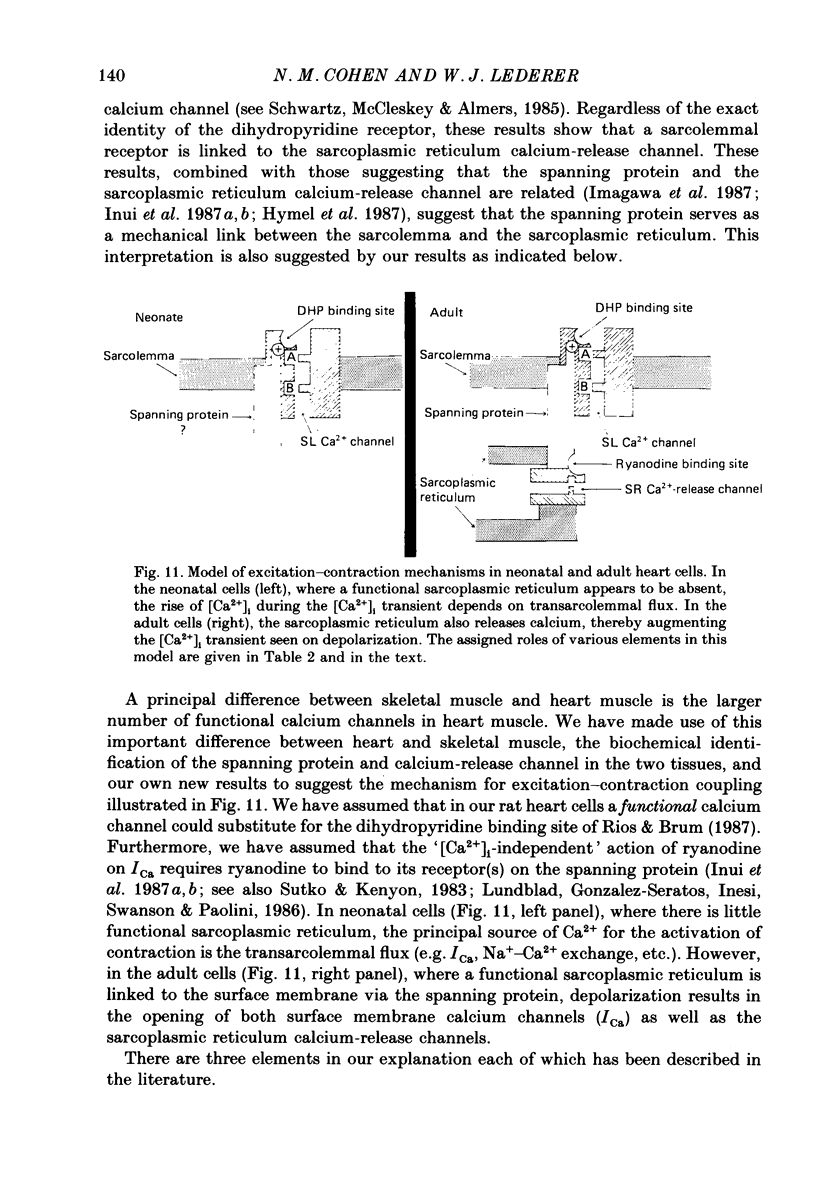
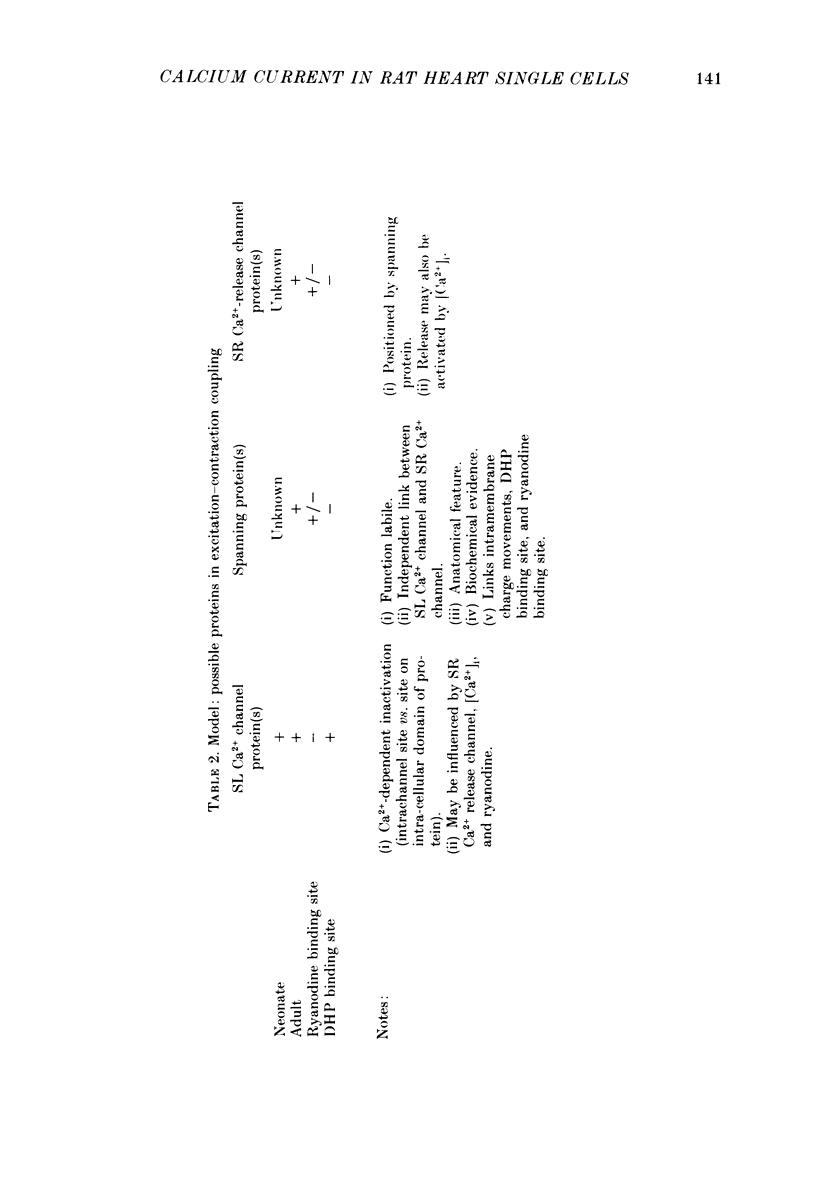
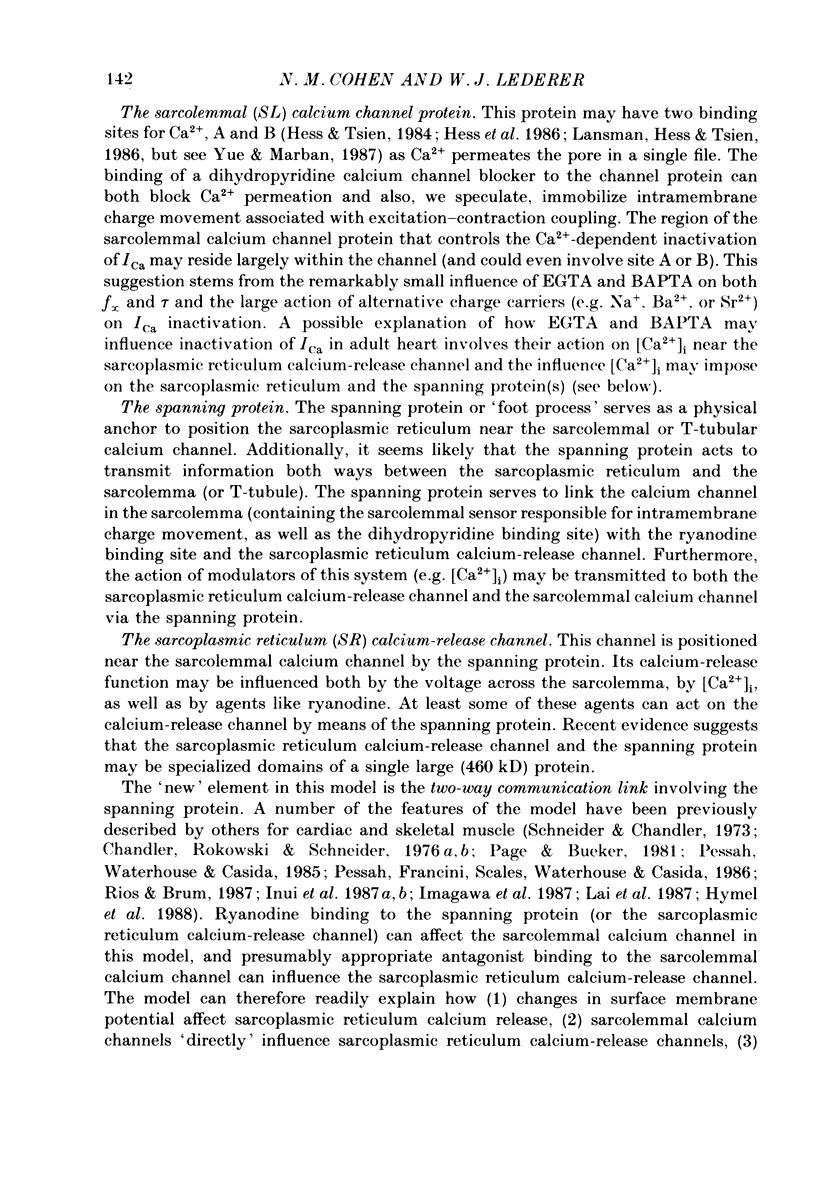
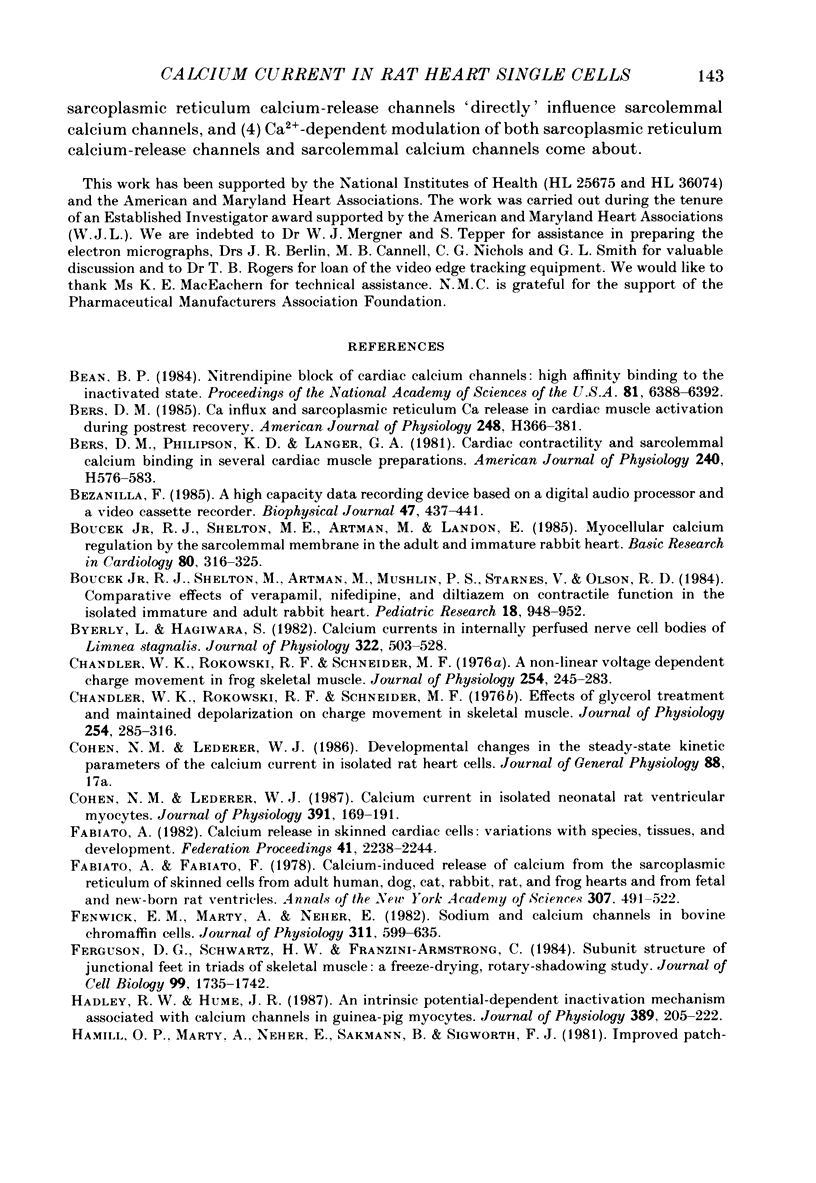
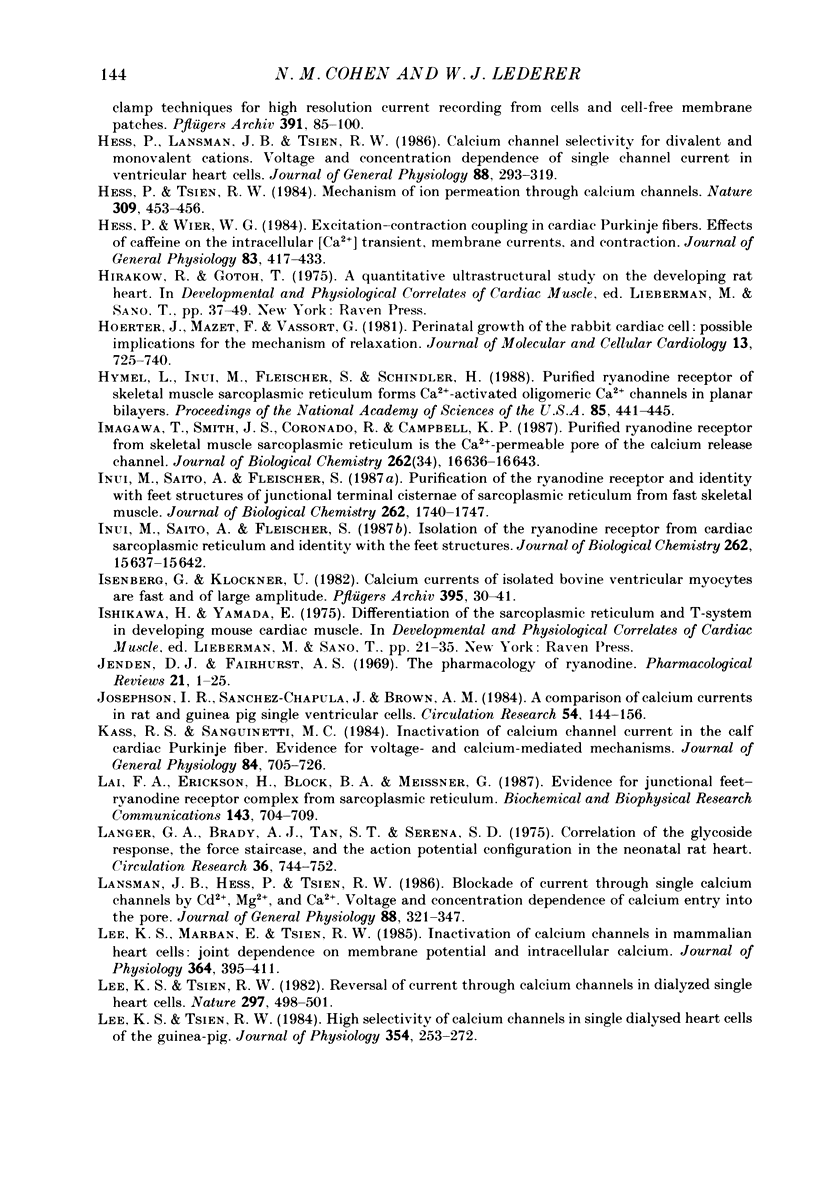
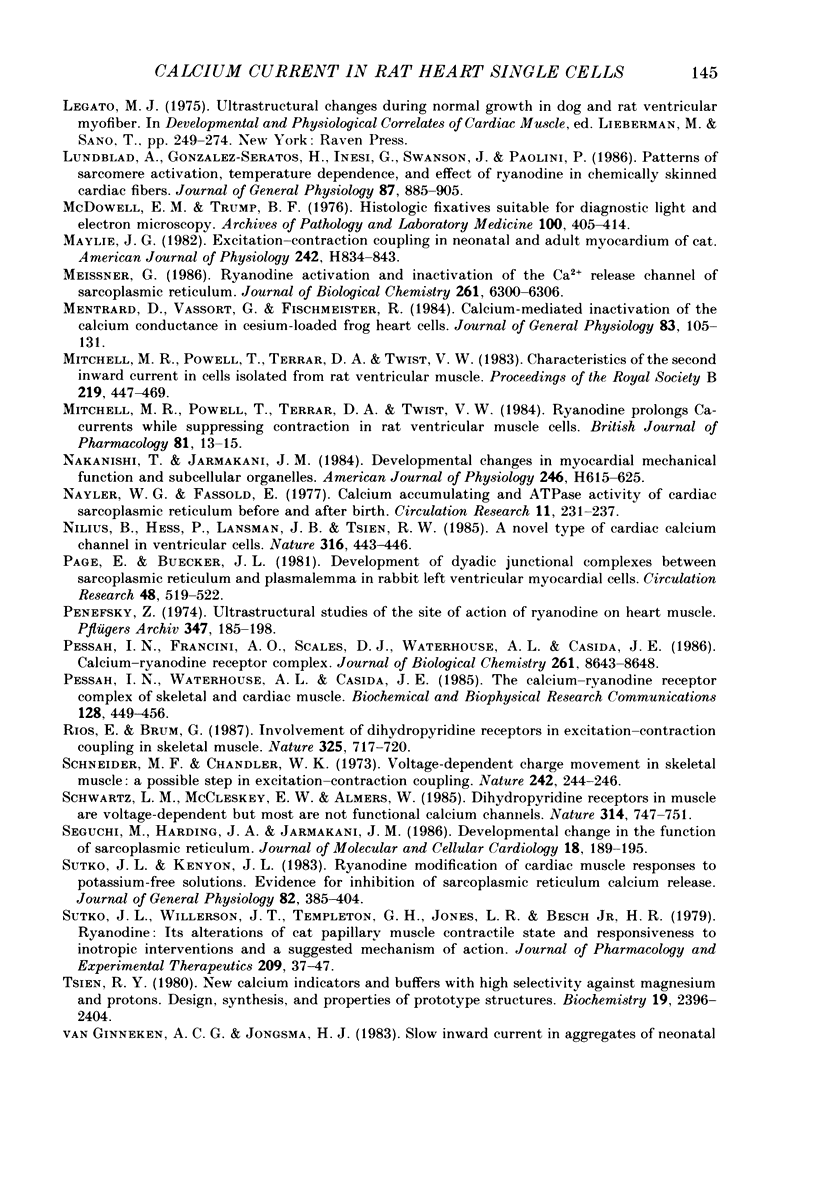
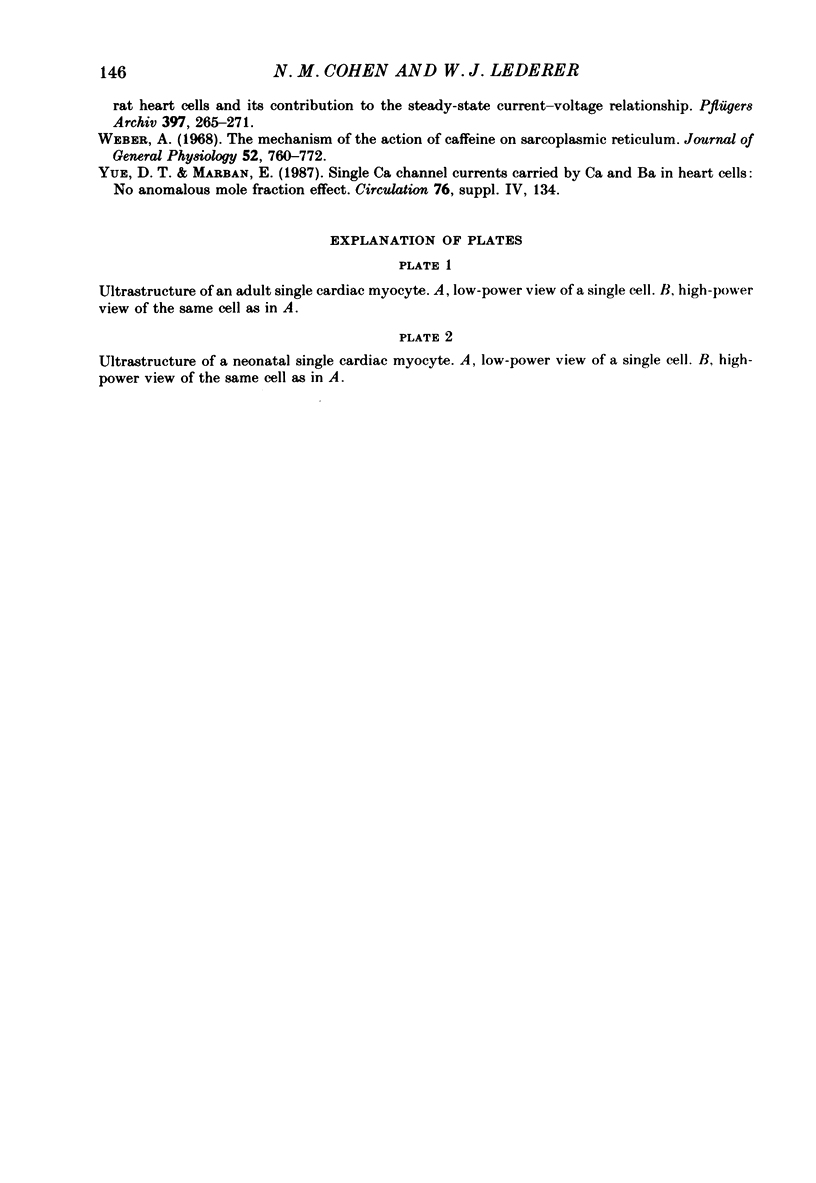
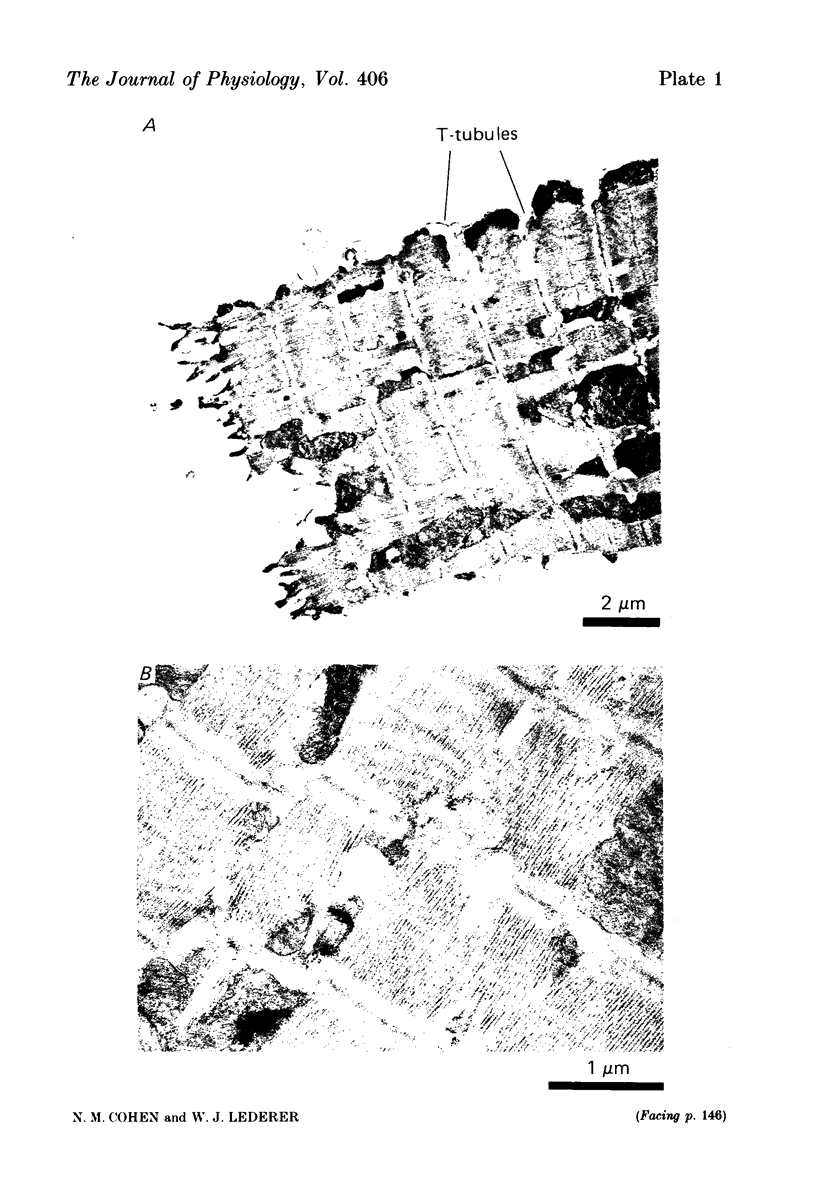
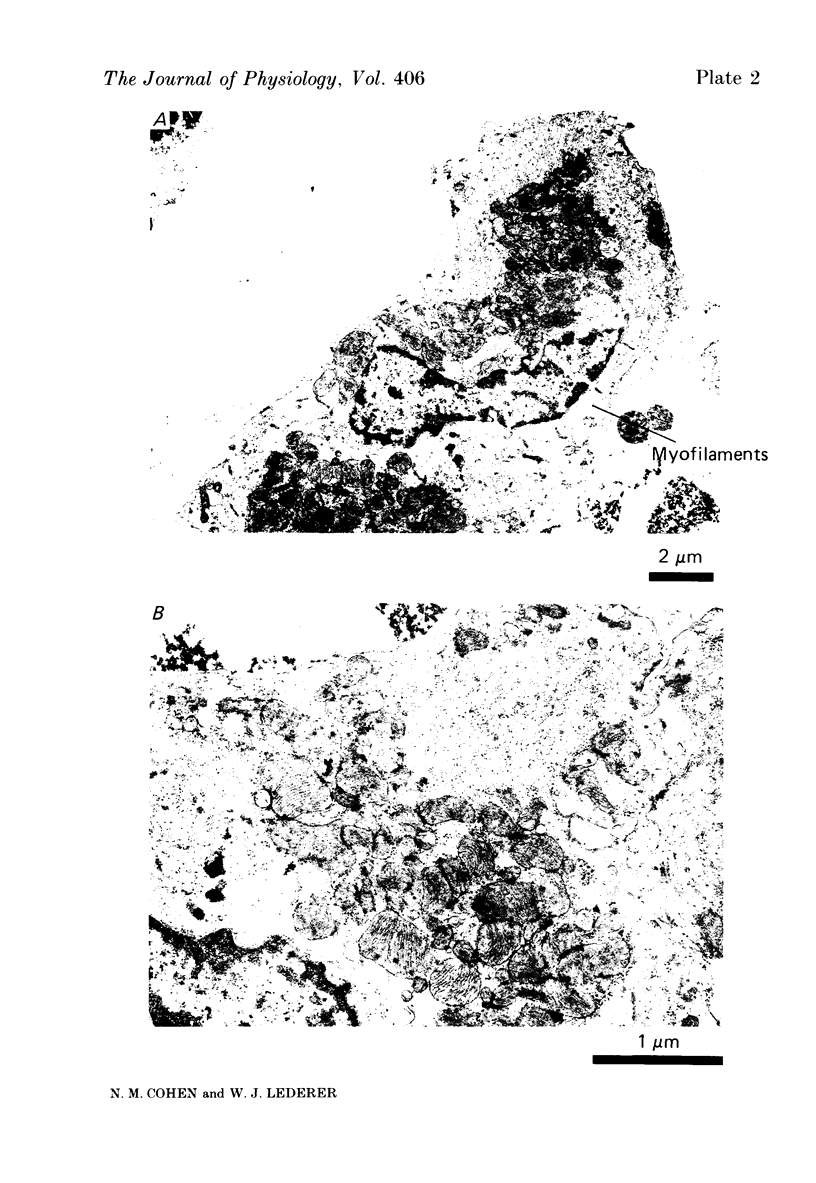
Images in this article
Selected References
These references are in PubMed. This may not be the complete list of references from this article.
- Bean B. P. Nitrendipine block of cardiac calcium channels: high-affinity binding to the inactivated state. Proc Natl Acad Sci U S A. 1984 Oct;81(20):6388–6392. doi: 10.1073/pnas.81.20.6388. [DOI] [PMC free article] [PubMed] [Google Scholar]
- Bers D. M. Ca influx and sarcoplasmic reticulum Ca release in cardiac muscle activation during postrest recovery. Am J Physiol. 1985 Mar;248(3 Pt 2):H366–H381. doi: 10.1152/ajpheart.1985.248.3.H366. [DOI] [PubMed] [Google Scholar]
- Bers D. M., Philipson K. D., Langer G. A. Cardiac contractility and sarcolemmal calcium binding in several cardiac muscle preparations. Am J Physiol. 1981 Apr;240(4):H576–H583. doi: 10.1152/ajpheart.1981.240.4.H576. [DOI] [PubMed] [Google Scholar]
- Bezanilla F. A high capacity data recording device based on a digital audio processor and a video cassette recorder. Biophys J. 1985 Mar;47(3):437–441. doi: 10.1016/S0006-3495(85)83935-7. [DOI] [PMC free article] [PubMed] [Google Scholar]
- Boucek R. J., Jr, Shelton M. E., Artman M., Landon E. Myocellular calcium regulation by the sarcolemmal membrane in the adult and immature rabbit heart. Basic Res Cardiol. 1985 May-Jun;80(3):316–325. doi: 10.1007/BF01907907. [DOI] [PubMed] [Google Scholar]
- Boucek R. J., Jr, Shelton M., Artman M., Mushlin P. S., Starnes V. A., Olson R. D. Comparative effects of verapamil, nifedipine, and diltiazem on contractile function in the isolated immature and adult rabbit heart. Pediatr Res. 1984 Oct;18(10):948–952. doi: 10.1203/00006450-198410000-00008. [DOI] [PubMed] [Google Scholar]
- Byerly L., Hagiwara S. Calcium currents in internally perfused nerve cell bodies of Limnea stagnalis. J Physiol. 1982 Jan;322:503–528. doi: 10.1113/jphysiol.1982.sp014052. [DOI] [PMC free article] [PubMed] [Google Scholar]
- Chandler W. K., Rakowski R. F., Schneider M. F. A non-linear voltage dependent charge movement in frog skeletal muscle. J Physiol. 1976 Jan;254(2):245–283. doi: 10.1113/jphysiol.1976.sp011232. [DOI] [PMC free article] [PubMed] [Google Scholar]
- Chandler W. K., Rakowski R. F., Schneider M. F. Effects of glycerol treatment and maintained depolarization on charge movement in skeletal muscle. J Physiol. 1976 Jan;254(2):285–316. doi: 10.1113/jphysiol.1976.sp011233. [DOI] [PMC free article] [PubMed] [Google Scholar]
- Cohen N. M., Lederer W. J. Calcium current in isolated neonatal rat ventricular myocytes. J Physiol. 1987 Oct;391:169–191. doi: 10.1113/jphysiol.1987.sp016732. [DOI] [PMC free article] [PubMed] [Google Scholar]
- Fabiato A. Calcium release in skinned cardiac cells: variations with species, tissues, and development. Fed Proc. 1982 May;41(7):2238–2244. [PubMed] [Google Scholar]
- Fabiato A., Fabiato F. Calcium-induced release of calcium from the sarcoplasmic reticulum of skinned cells from adult human, dog, cat, rabbit, rat, and frog hearts and from fetal and new-born rat ventricles. Ann N Y Acad Sci. 1978 Apr 28;307:491–522. doi: 10.1111/j.1749-6632.1978.tb41979.x. [DOI] [PubMed] [Google Scholar]
- Fenwick E. M., Marty A., Neher E. Sodium and calcium channels in bovine chromaffin cells. J Physiol. 1982 Oct;331:599–635. doi: 10.1113/jphysiol.1982.sp014394. [DOI] [PMC free article] [PubMed] [Google Scholar]
- Ferguson D. G., Schwartz H. W., Franzini-Armstrong C. Subunit structure of junctional feet in triads of skeletal muscle: a freeze-drying, rotary-shadowing study. J Cell Biol. 1984 Nov;99(5):1735–1742. doi: 10.1083/jcb.99.5.1735. [DOI] [PMC free article] [PubMed] [Google Scholar]
- Hadley R. W., Hume J. R. An intrinsic potential-dependent inactivation mechanism associated with calcium channels in guinea-pig myocytes. J Physiol. 1987 Aug;389:205–222. doi: 10.1113/jphysiol.1987.sp016654. [DOI] [PMC free article] [PubMed] [Google Scholar]
- Hamill O. P., Marty A., Neher E., Sakmann B., Sigworth F. J. Improved patch-clamp techniques for high-resolution current recording from cells and cell-free membrane patches. Pflugers Arch. 1981 Aug;391(2):85–100. doi: 10.1007/BF00656997. [DOI] [PubMed] [Google Scholar]
- Hess P., Lansman J. B., Tsien R. W. Calcium channel selectivity for divalent and monovalent cations. Voltage and concentration dependence of single channel current in ventricular heart cells. J Gen Physiol. 1986 Sep;88(3):293–319. doi: 10.1085/jgp.88.3.293. [DOI] [PMC free article] [PubMed] [Google Scholar]
- Hess P., Tsien R. W. Mechanism of ion permeation through calcium channels. 1984 May 31-Jun 6Nature. 309(5967):453–456. doi: 10.1038/309453a0. [DOI] [PubMed] [Google Scholar]
- Hess P., Wier W. G. Excitation-contraction coupling in cardiac Purkinje fibers. Effects of caffeine on the intracellular [Ca2+] transient, membrane currents, and contraction. J Gen Physiol. 1984 Mar;83(3):417–433. doi: 10.1085/jgp.83.3.417. [DOI] [PMC free article] [PubMed] [Google Scholar]
- Hoerter J., Mazet F., Vassort G. Perinatal growth of the rabbit cardiac cell: possible implications for the mechanism of relaxation. J Mol Cell Cardiol. 1981 Aug;13(8):725–740. doi: 10.1016/0022-2828(81)90255-8. [DOI] [PubMed] [Google Scholar]
- Hymel L., Inui M., Fleischer S., Schindler H. Purified ryanodine receptor of skeletal muscle sarcoplasmic reticulum forms Ca2+-activated oligomeric Ca2+ channels in planar bilayers. Proc Natl Acad Sci U S A. 1988 Jan;85(2):441–445. doi: 10.1073/pnas.85.2.441. [DOI] [PMC free article] [PubMed] [Google Scholar]
- Imagawa T., Smith J. S., Coronado R., Campbell K. P. Purified ryanodine receptor from skeletal muscle sarcoplasmic reticulum is the Ca2+-permeable pore of the calcium release channel. J Biol Chem. 1987 Dec 5;262(34):16636–16643. [PubMed] [Google Scholar]
- Inui M., Saito A., Fleischer S. Isolation of the ryanodine receptor from cardiac sarcoplasmic reticulum and identity with the feet structures. J Biol Chem. 1987 Nov 15;262(32):15637–15642. [PubMed] [Google Scholar]
- Inui M., Saito A., Fleischer S. Purification of the ryanodine receptor and identity with feet structures of junctional terminal cisternae of sarcoplasmic reticulum from fast skeletal muscle. J Biol Chem. 1987 Feb 5;262(4):1740–1747. [PubMed] [Google Scholar]
- Isenberg G., Klöckner U. Calcium currents of isolated bovine ventricular myocytes are fast and of large amplitude. Pflugers Arch. 1982 Oct;395(1):30–41. doi: 10.1007/BF00584965. [DOI] [PubMed] [Google Scholar]
- Jenden D. J., Fairhurst A. S. The pharmacology of ryanodine. Pharmacol Rev. 1969 Mar;21(1):1–25. [PubMed] [Google Scholar]
- Josephson I. R., Sanchez-Chapula J., Brown A. M. A comparison of calcium currents in rat and guinea pig single ventricular cells. Circ Res. 1984 Feb;54(2):144–156. doi: 10.1161/01.res.54.2.144. [DOI] [PubMed] [Google Scholar]
- Kass R. S., Sanguinetti M. C. Inactivation of calcium channel current in the calf cardiac Purkinje fiber. Evidence for voltage- and calcium-mediated mechanisms. J Gen Physiol. 1984 Nov;84(5):705–726. doi: 10.1085/jgp.84.5.705. [DOI] [PMC free article] [PubMed] [Google Scholar]
- Lai F. A., Erickson H., Block B. A., Meissner G. Evidence for a junctional feet-ryanodine receptor complex from sarcoplasmic reticulum. Biochem Biophys Res Commun. 1987 Mar 13;143(2):704–709. doi: 10.1016/0006-291x(87)91411-2. [DOI] [PubMed] [Google Scholar]
- Langer G. A., Brady A. J., Tan S. T., Serena D. Correlation of the glycoside response, the force staircase, and the action potential configuration in the neonatal rat heart. Circ Res. 1975 Jun;36(6):744–752. doi: 10.1161/01.res.36.6.744. [DOI] [PubMed] [Google Scholar]
- Lansman J. B., Hess P., Tsien R. W. Blockade of current through single calcium channels by Cd2+, Mg2+, and Ca2+. Voltage and concentration dependence of calcium entry into the pore. J Gen Physiol. 1986 Sep;88(3):321–347. doi: 10.1085/jgp.88.3.321. [DOI] [PMC free article] [PubMed] [Google Scholar]
- Lee K. S., Marban E., Tsien R. W. Inactivation of calcium channels in mammalian heart cells: joint dependence on membrane potential and intracellular calcium. J Physiol. 1985 Jul;364:395–411. doi: 10.1113/jphysiol.1985.sp015752. [DOI] [PMC free article] [PubMed] [Google Scholar]
- Lee K. S., Tsien R. W. High selectivity of calcium channels in single dialysed heart cells of the guinea-pig. J Physiol. 1984 Sep;354:253–272. doi: 10.1113/jphysiol.1984.sp015374. [DOI] [PMC free article] [PubMed] [Google Scholar]
- Lee K. S., Tsien R. W. Reversal of current through calcium channels in dialysed single heart cells. Nature. 1982 Jun 10;297(5866):498–501. doi: 10.1038/297498a0. [DOI] [PubMed] [Google Scholar]
- Lundblad A., Gonzalez-Serratos H., Inesi G., Swanson J., Paolini P. Patterns of sarcomere activation, temperature dependence, and effect of ryanodine in chemically skinned cardiac fibers. J Gen Physiol. 1986 Jun;87(6):885–905. doi: 10.1085/jgp.87.6.885. [DOI] [PMC free article] [PubMed] [Google Scholar]
- Maylie J. G. Excitation-contraction coupling in neonatal and adult myocardium of cat. Am J Physiol. 1982 May;242(5):H834–H843. doi: 10.1152/ajpheart.1982.242.5.H834. [DOI] [PubMed] [Google Scholar]
- McDowell E. M., Trump B. F. Histologic fixatives suitable for diagnostic light and electron microscopy. Arch Pathol Lab Med. 1976 Aug;100(8):405–414. [PubMed] [Google Scholar]
- Meissner G. Ryanodine activation and inhibition of the Ca2+ release channel of sarcoplasmic reticulum. J Biol Chem. 1986 May 15;261(14):6300–6306. [PubMed] [Google Scholar]
- Mentrard D., Vassort G., Fischmeister R. Calcium-mediated inactivation of the calcium conductance in cesium-loaded frog heart cells. J Gen Physiol. 1984 Jan;83(1):105–131. doi: 10.1085/jgp.83.1.105. [DOI] [PMC free article] [PubMed] [Google Scholar]
- Mitchell M. R., Powell T., Terrar D. A., Twist V. W. Characteristics of the second inward current in cells isolated from rat ventricular muscle. Proc R Soc Lond B Biol Sci. 1983 Oct 22;219(1217):447–469. doi: 10.1098/rspb.1983.0084. [DOI] [PubMed] [Google Scholar]
- Mitchell M. R., Powell T., Terrar D. A., Twist V. W. Ryanodine prolongs Ca-currents while suppressing contraction in rat ventricular muscle cells. Br J Pharmacol. 1984 Jan;81(1):13–15. doi: 10.1111/j.1476-5381.1984.tb10735.x. [DOI] [PMC free article] [PubMed] [Google Scholar]
- Nakanishi T., Jarmakani J. M. Developmental changes in myocardial mechanical function and subcellular organelles. Am J Physiol. 1984 Apr;246(4 Pt 2):H615–H625. doi: 10.1152/ajpheart.1984.246.4.H615. [DOI] [PubMed] [Google Scholar]
- Nayler W. G., Fassold E. Calcium accumulating and ATPase activity of cardiac sarcoplasmic reticulum before and after birth. Cardiovasc Res. 1977 May;11(3):231–237. doi: 10.1093/cvr/11.3.231. [DOI] [PubMed] [Google Scholar]
- Nilius B., Hess P., Lansman J. B., Tsien R. W. A novel type of cardiac calcium channel in ventricular cells. Nature. 1985 Aug 1;316(6027):443–446. doi: 10.1038/316443a0. [DOI] [PubMed] [Google Scholar]
- Page E., Buecker J. L. Development of dyadic junctional complexes between sarcoplasmic reticulum and plasmalemma in rabbit left ventricular myocardial cells. Morphometric analysis. Circ Res. 1981 Apr;48(4):519–522. doi: 10.1161/01.res.48.4.519. [DOI] [PubMed] [Google Scholar]
- Penefsky Z. J. Ultrastructural studies of the site of action of ryanodine on heart muscle. Pflugers Arch. 1974 Mar 11;347(3):185–198. doi: 10.1007/BF00592596. [DOI] [PubMed] [Google Scholar]
- Pessah I. N., Francini A. O., Scales D. J., Waterhouse A. L., Casida J. E. Calcium-ryanodine receptor complex. Solubilization and partial characterization from skeletal muscle junctional sarcoplasmic reticulum vesicles. J Biol Chem. 1986 Jul 5;261(19):8643–8648. [PubMed] [Google Scholar]
- Pessah I. N., Waterhouse A. L., Casida J. E. The calcium-ryanodine receptor complex of skeletal and cardiac muscle. Biochem Biophys Res Commun. 1985 Apr 16;128(1):449–456. doi: 10.1016/0006-291x(85)91699-7. [DOI] [PubMed] [Google Scholar]
- Rios E., Brum G. Involvement of dihydropyridine receptors in excitation-contraction coupling in skeletal muscle. Nature. 1987 Feb 19;325(6106):717–720. doi: 10.1038/325717a0. [DOI] [PubMed] [Google Scholar]
- Schneider M. F., Chandler W. K. Voltage dependent charge movement of skeletal muscle: a possible step in excitation-contraction coupling. Nature. 1973 Mar 23;242(5395):244–246. doi: 10.1038/242244a0. [DOI] [PubMed] [Google Scholar]
- Schwartz L. M., McCleskey E. W., Almers W. Dihydropyridine receptors in muscle are voltage-dependent but most are not functional calcium channels. 1985 Apr 25-May 1Nature. 314(6013):747–751. doi: 10.1038/314747a0. [DOI] [PubMed] [Google Scholar]
- Seguchi M., Harding J. A., Jarmakani J. M. Developmental change in the function of sarcoplasmic reticulum. J Mol Cell Cardiol. 1986 Feb;18(2):189–195. doi: 10.1016/s0022-2828(86)80471-0. [DOI] [PubMed] [Google Scholar]
- Sutko J. L., Kenyon J. L. Ryanodine modification of cardiac muscle responses to potassium-free solutions. Evidence for inhibition of sarcoplasmic reticulum calcium release. J Gen Physiol. 1983 Sep;82(3):385–404. doi: 10.1085/jgp.82.3.385. [DOI] [PMC free article] [PubMed] [Google Scholar]
- Sutko J. L., Willerson J. T., Templeton G. H., Jones L. R., Besch H. R., Jr Ryanodine: its alterations of cat papillary muscle contractile state and responsiveness to inotropic interventions and a suggested mechanism of action. J Pharmacol Exp Ther. 1979 Apr;209(1):37–47. [PubMed] [Google Scholar]
- Tsien R. Y. New calcium indicators and buffers with high selectivity against magnesium and protons: design, synthesis, and properties of prototype structures. Biochemistry. 1980 May 27;19(11):2396–2404. doi: 10.1021/bi00552a018. [DOI] [PubMed] [Google Scholar]
- Weber A. The mechanism of the action of caffeine on sarcoplasmic reticulum. J Gen Physiol. 1968 Nov;52(5):760–772. doi: 10.1085/jgp.52.5.760. [DOI] [PMC free article] [PubMed] [Google Scholar]
- van Ginneken A. C., Jongsma H. J. Slow inward current in aggregates of neonatal rat heart cells and its contribution to the steady state current-voltage relationship. Pflugers Arch. 1983 Jun 1;397(4):265–271. doi: 10.1007/BF00580259. [DOI] [PubMed] [Google Scholar]