Abstract
1. Ferret ventricular muscles were skinned by prolonged application of Triton X-100. Aequorin was allowed to diffuse into the myoplasmic space and the resulting light emission was used to monitor the myoplasmic [Ca2+]. The muscle was then activated with a lightly buffered Ca2+ solution and the changes in myoplasmic [Ca2+] and tension in response to length changes were investigated. 2. A sudden reduction in muscle length led to a rapid increase in myoplasmic [Ca2+] to a new level which was maintained as long as muscle length was reduced and which was reversed when the muscle was stretched back to the control length. The rate of increase of [Ca2+] when the muscle length was reduced was greater than the rate of decrease in [Ca2+] when the muscle was stretched. 3. Increasing the concentration of EGTA in the activating solution, so as to increase its Ca2+-buffering capacity, eliminated the changes in myoplasmic [Ca2+] in response to a length change but had little effect on developed tension. 4. On stretching the muscle there was a slow component of recovery of tension with a time course broadly similar to the rate of decrease of myoplasmic [Ca2+]. The time course of tension redevelopment and of the accompanying reduction in myoplasmic [Ca2+] both decreased to a similar extent when the [Ca2+] used to activate the muscle was increased. 5. Step reductions of length of increasing amplitude caused increases in myoplasmic [Ca2+] which were larger in proportion to the size of the step. 6. Step reductions of length of equal size but from different starting lengths caused changes in myoplasmic [Ca2+] the amplitude of which correlated with the change in tension rather than the change in length. 7. The increase in myoplasmic [Ca2+] when muscle length is reduced suggests that Ca2+ is released from a site in the muscle, probably troponin C. The time course and magnitude of the changes in myoplasmic [Ca2+] correlate more closely with the changes in developed tension than muscle length.
Full text
PDF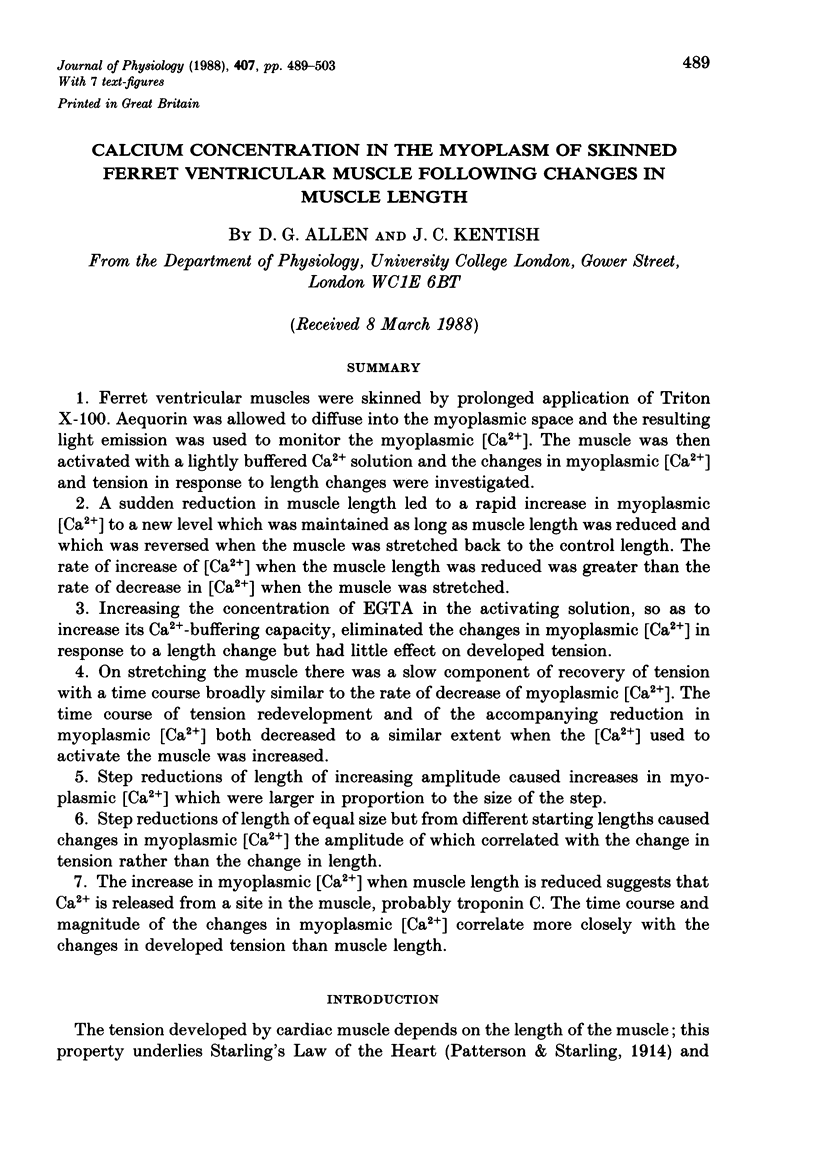
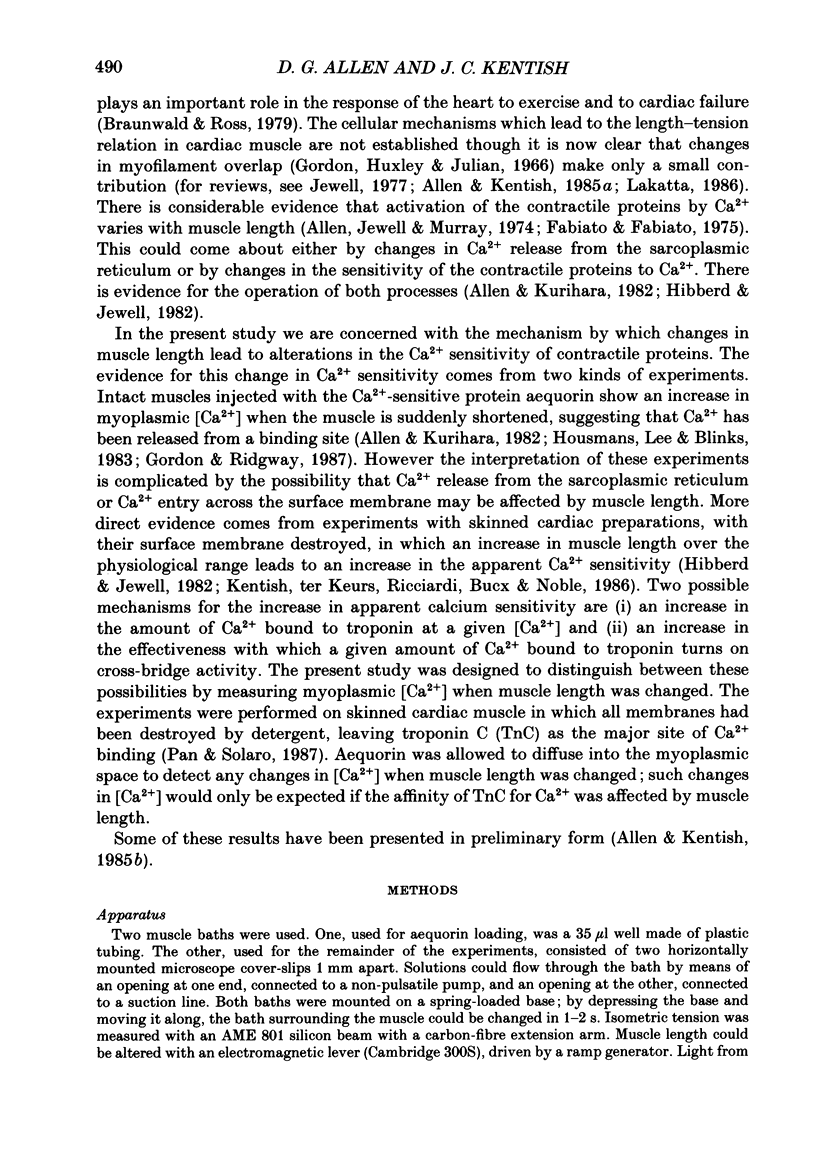
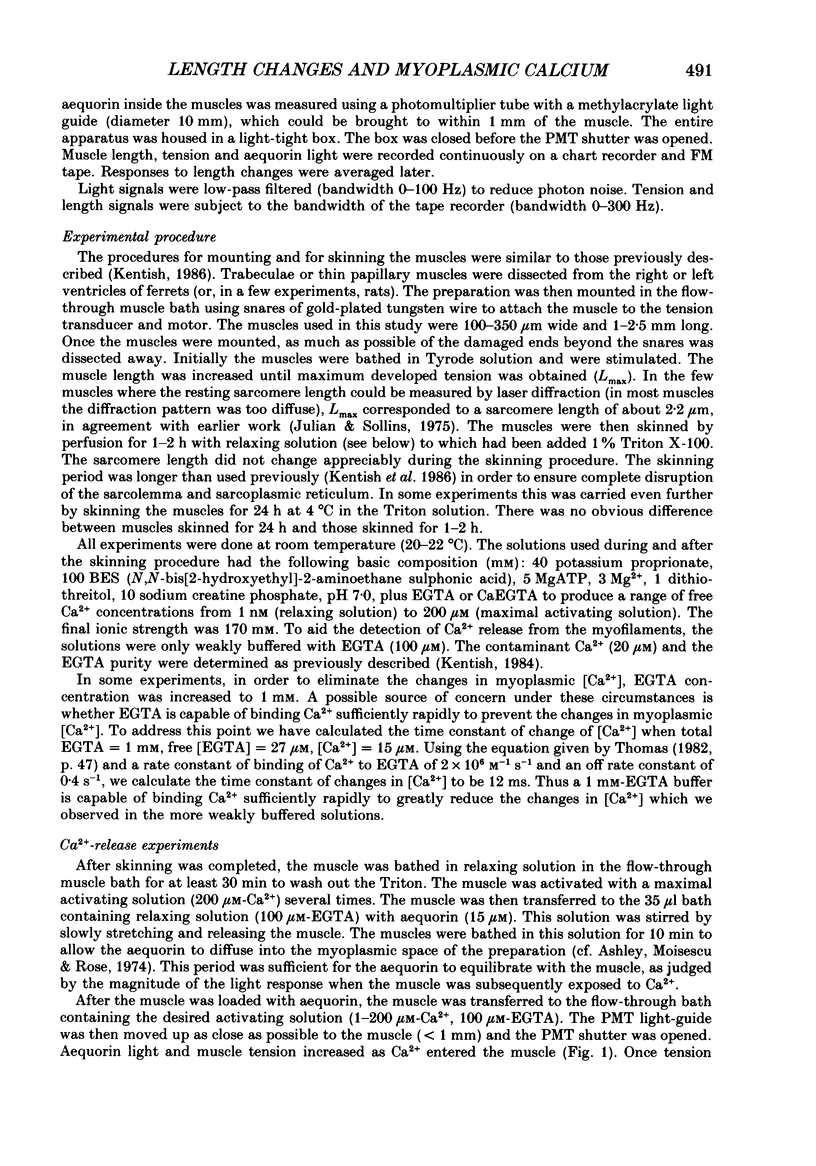
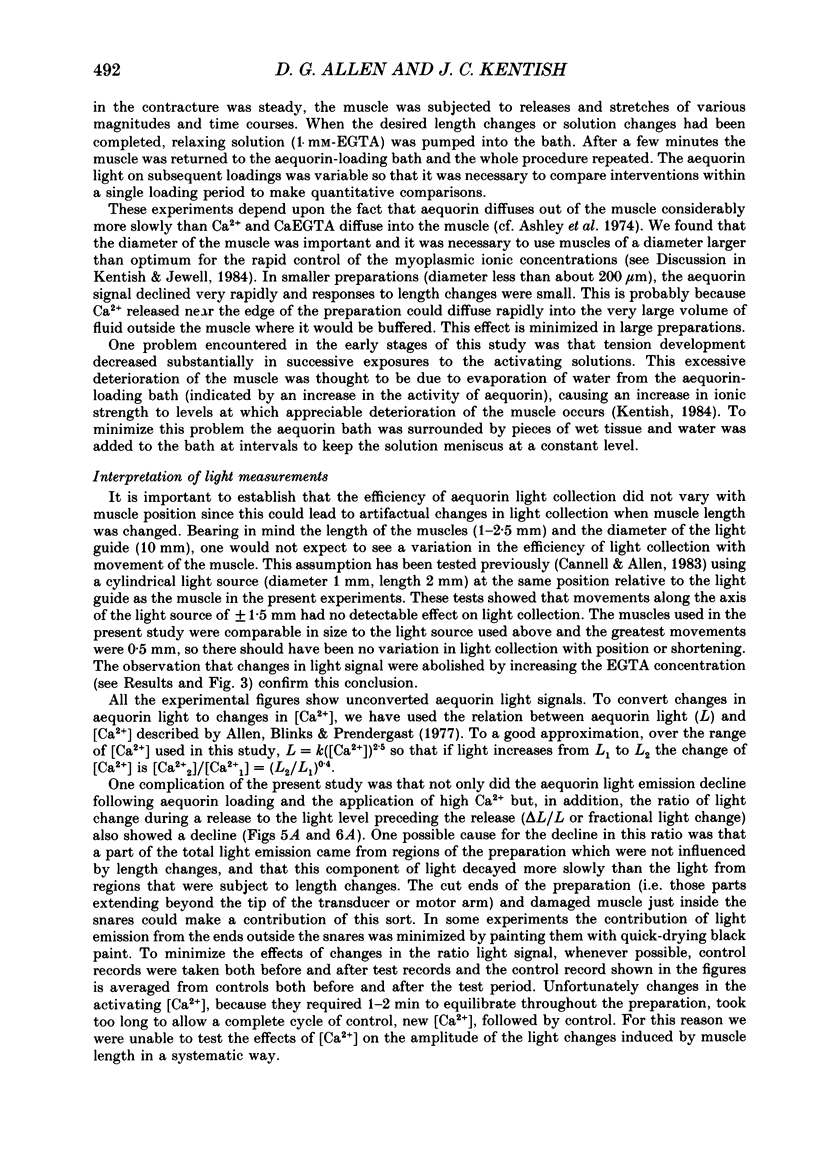
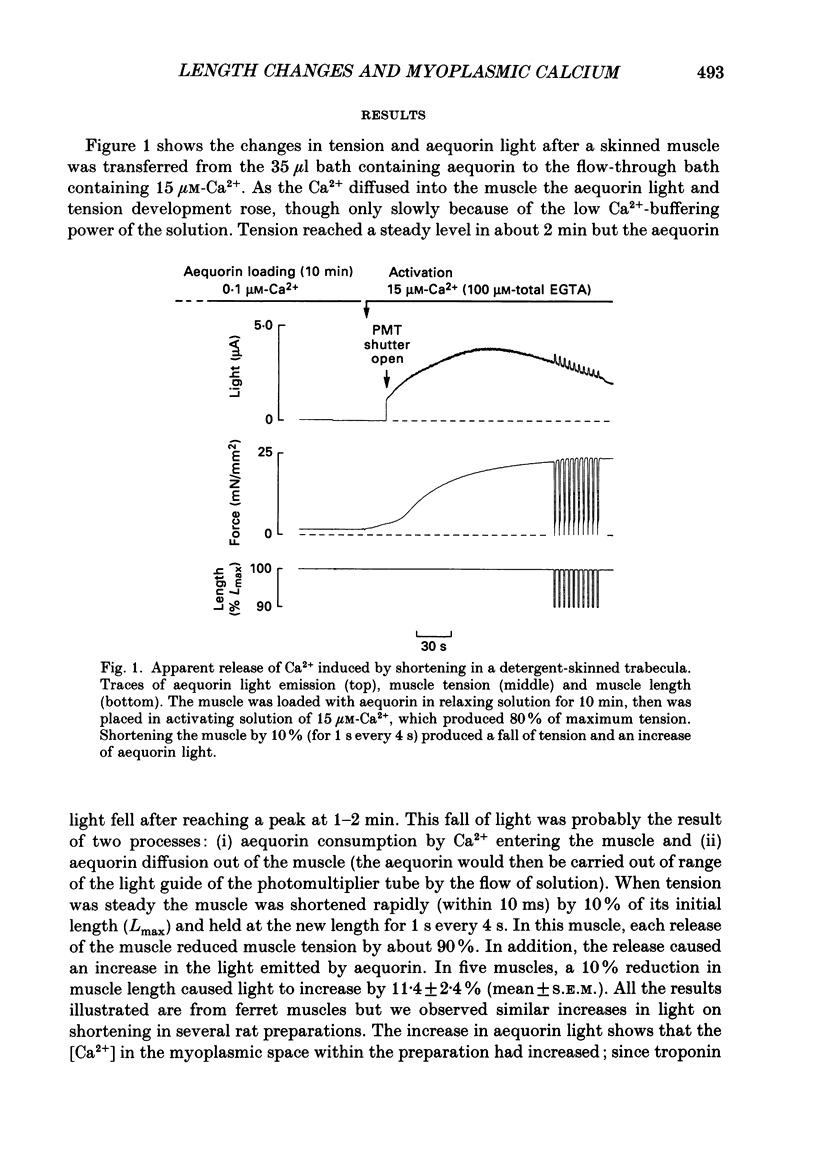
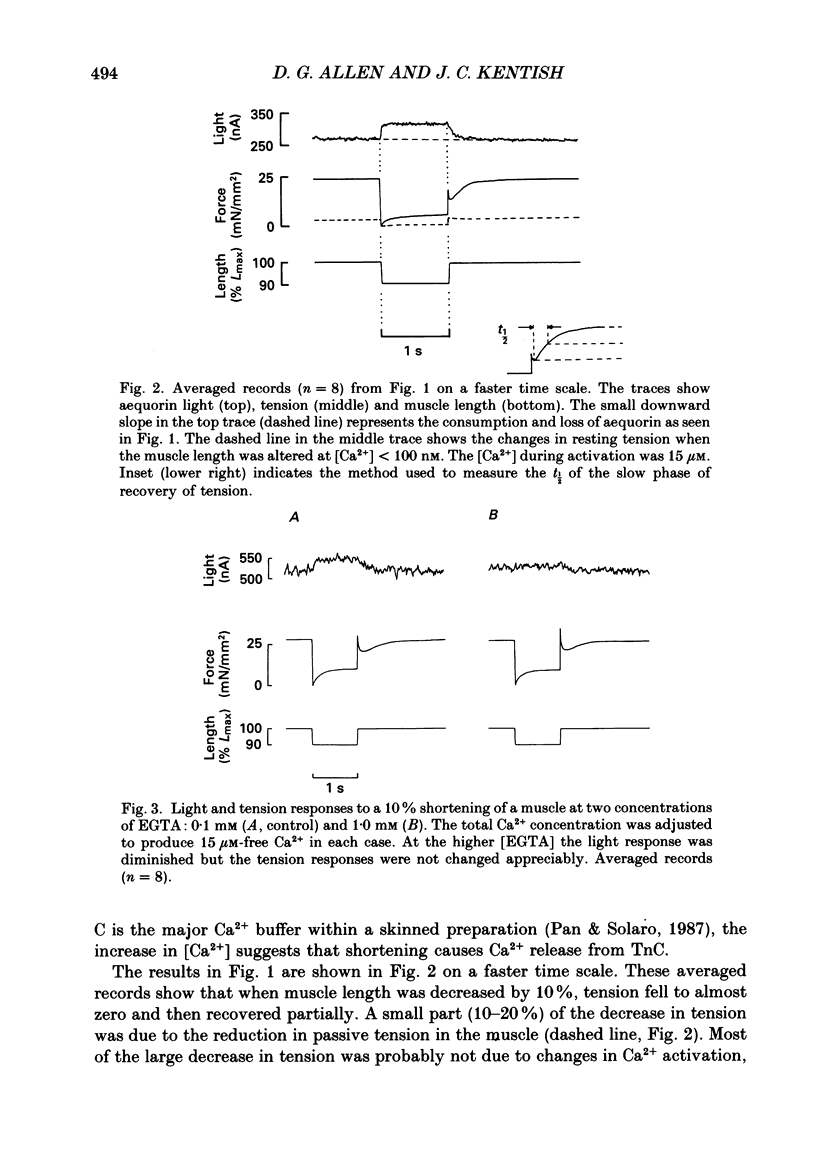
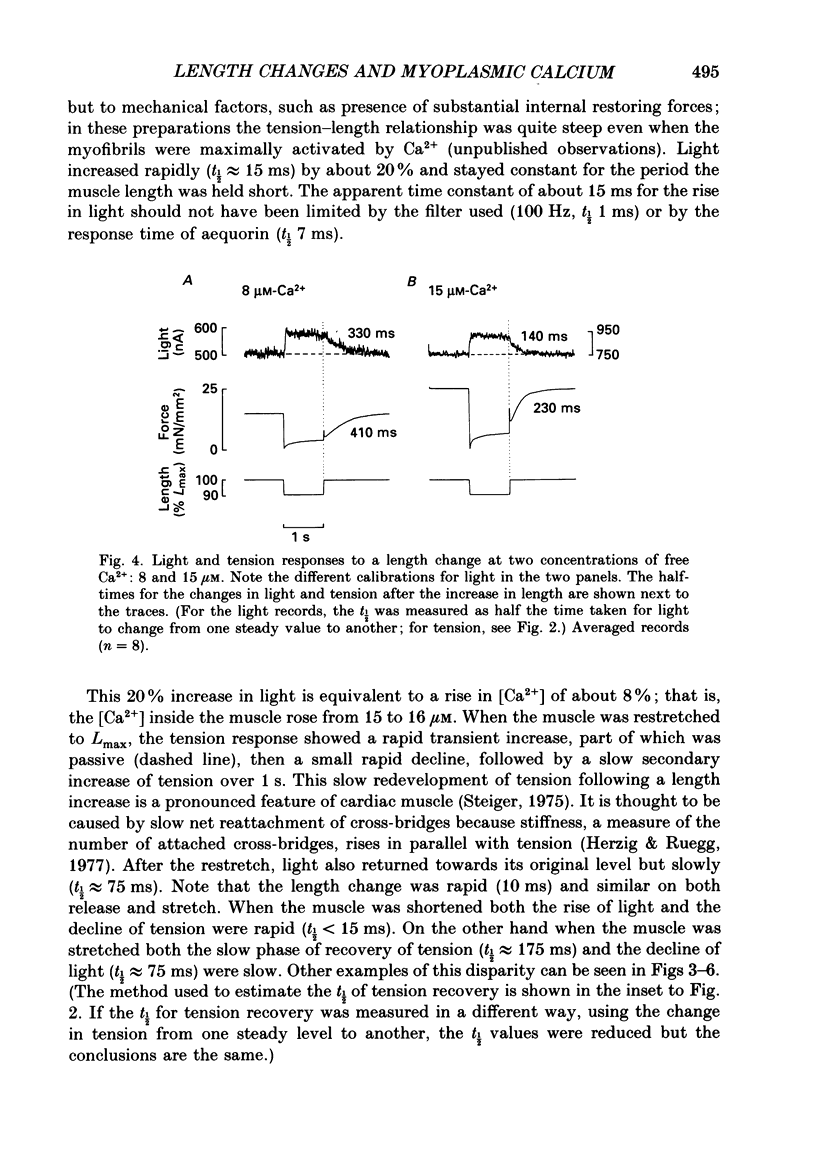
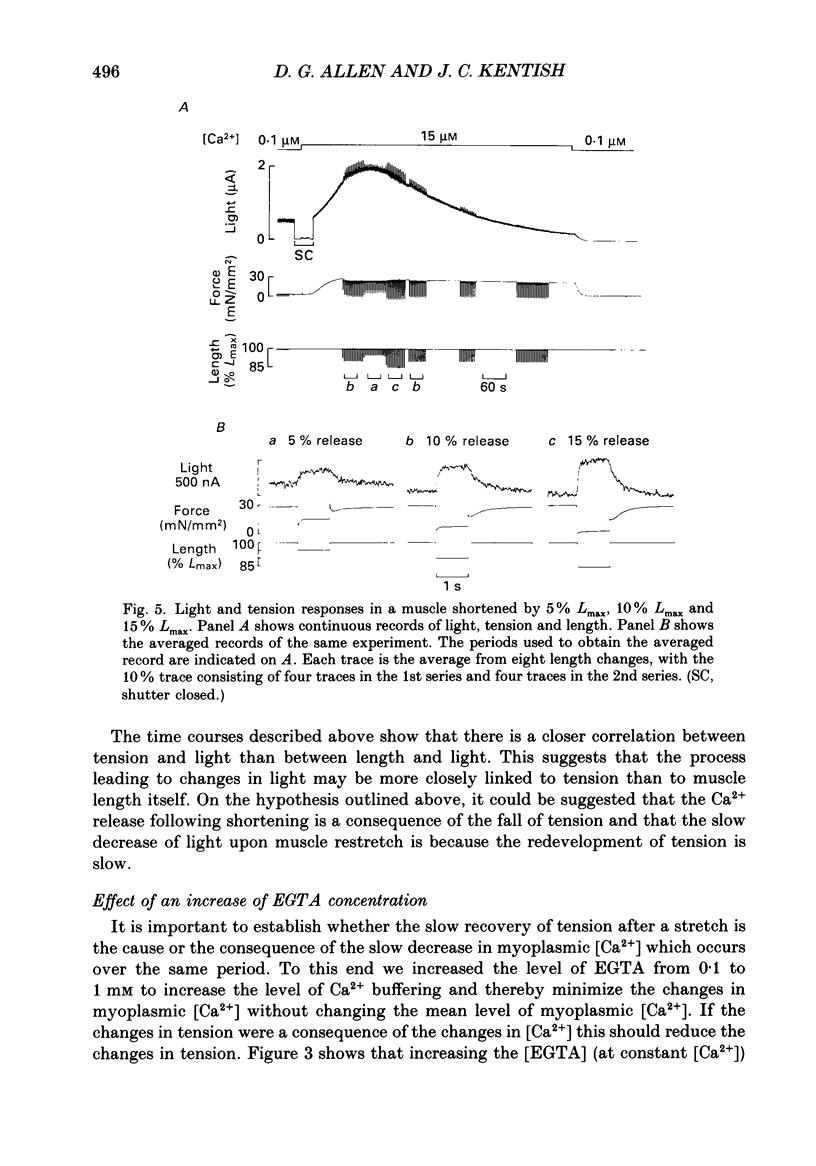
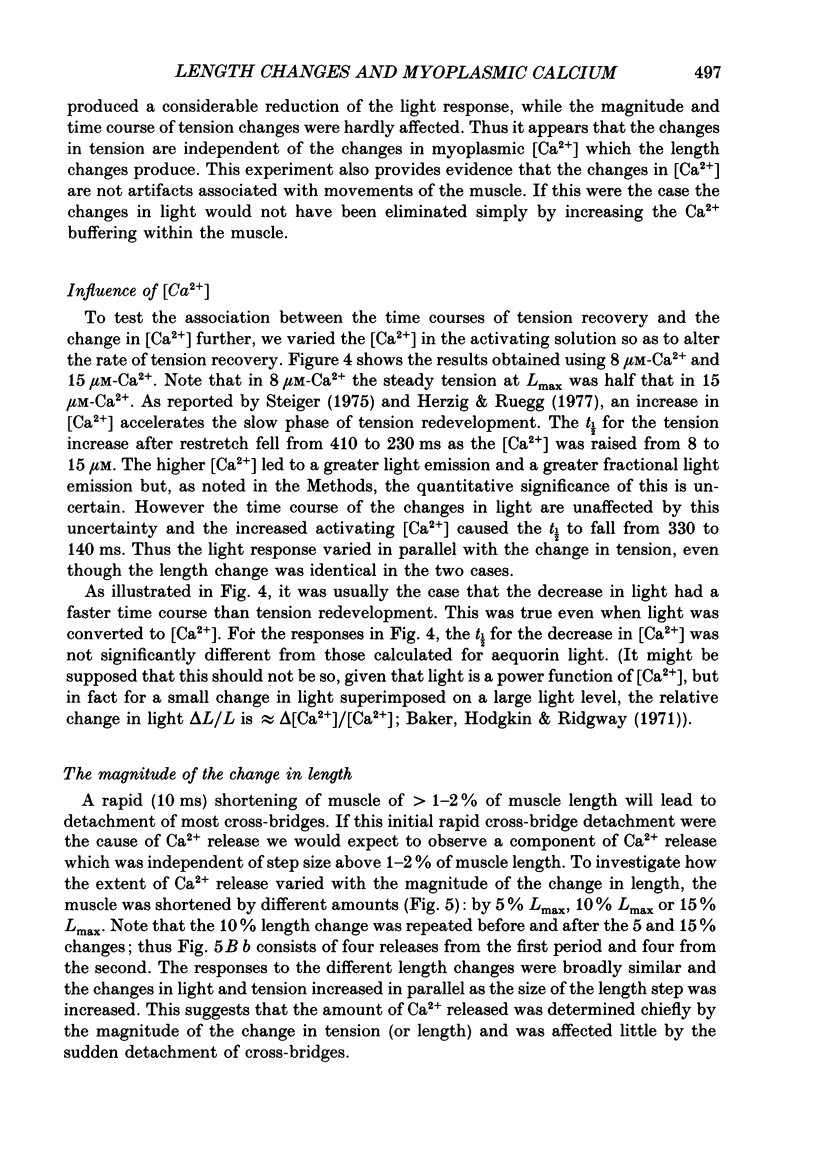
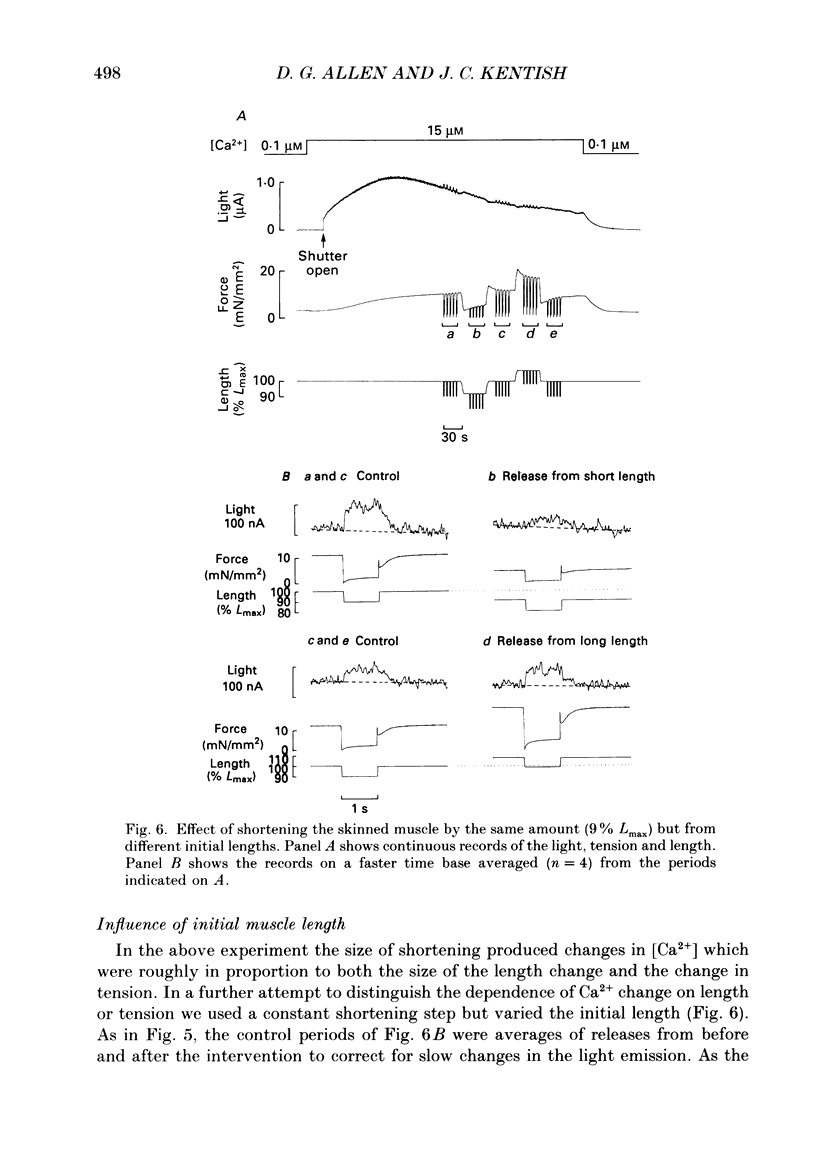
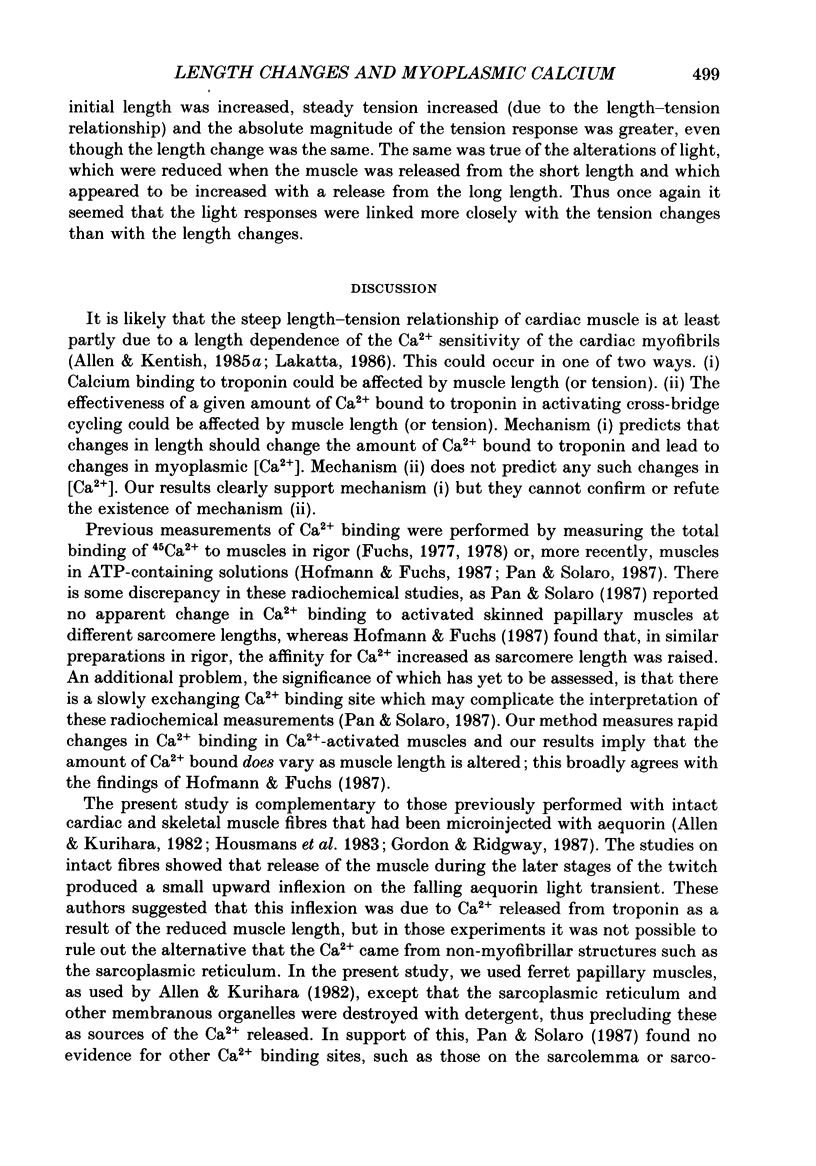
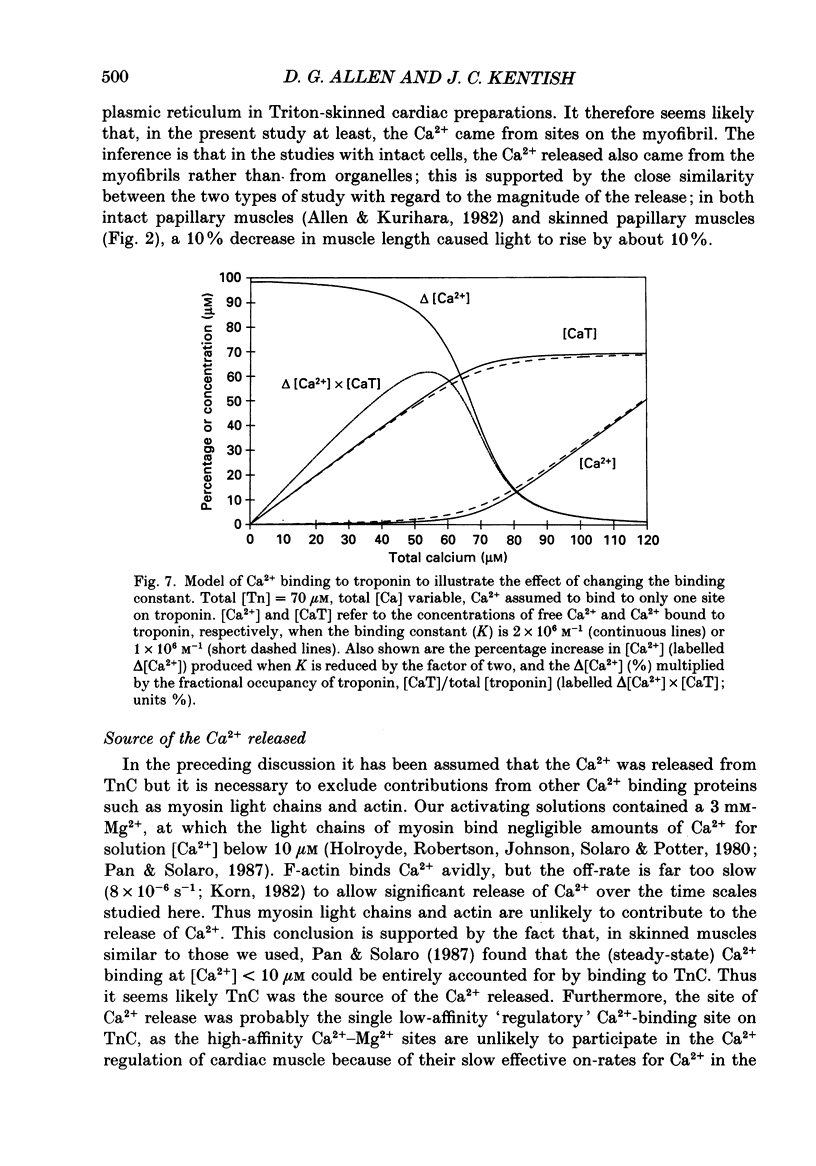
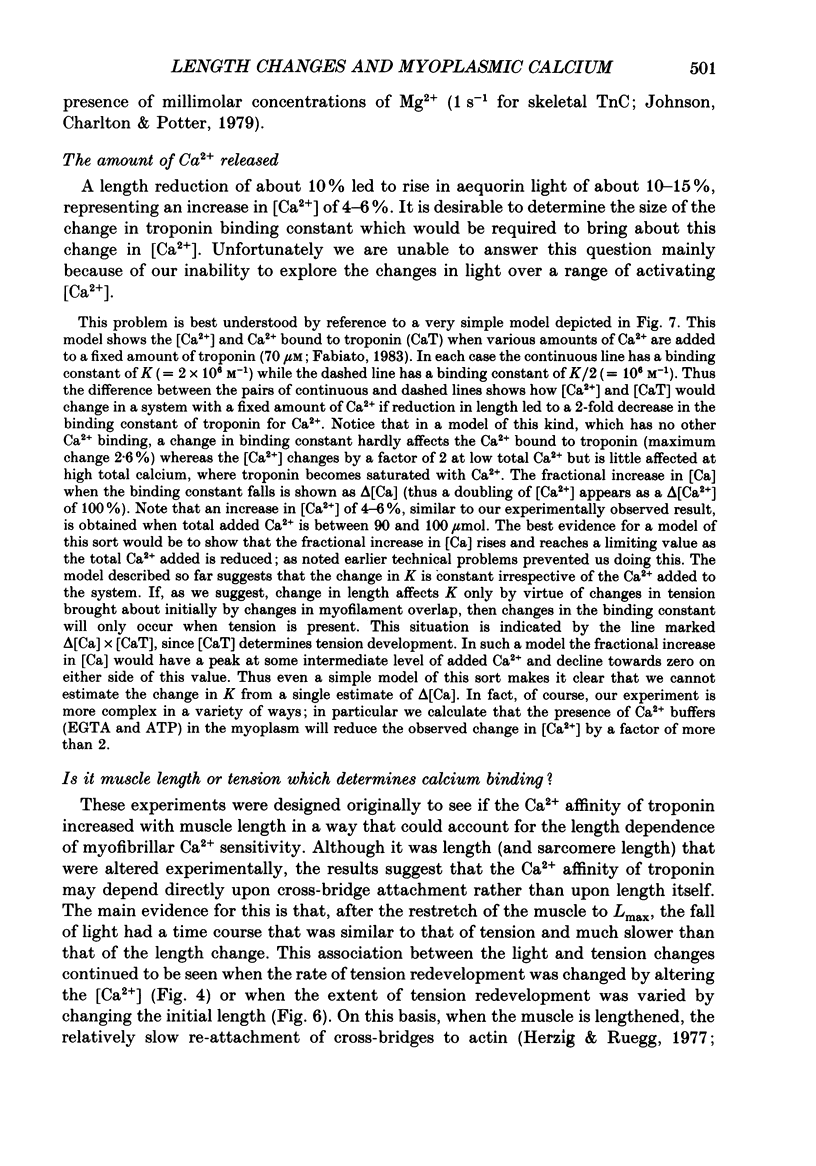
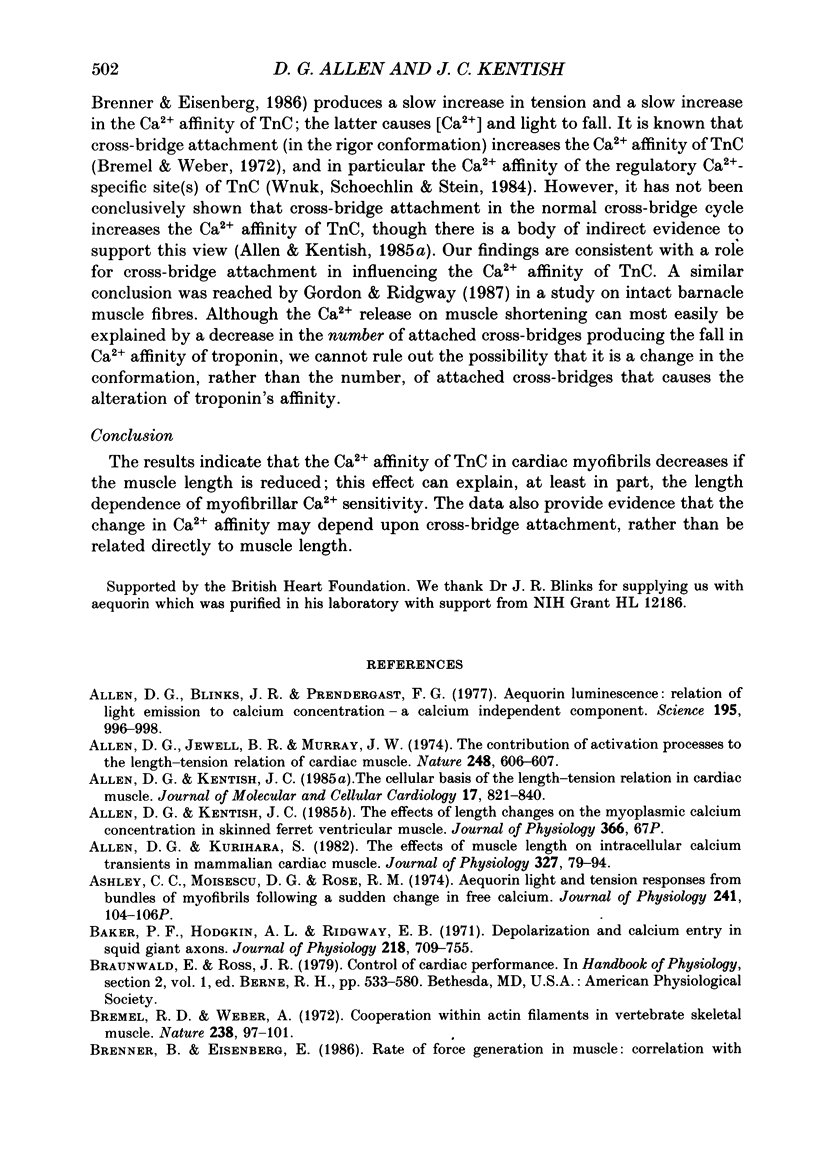
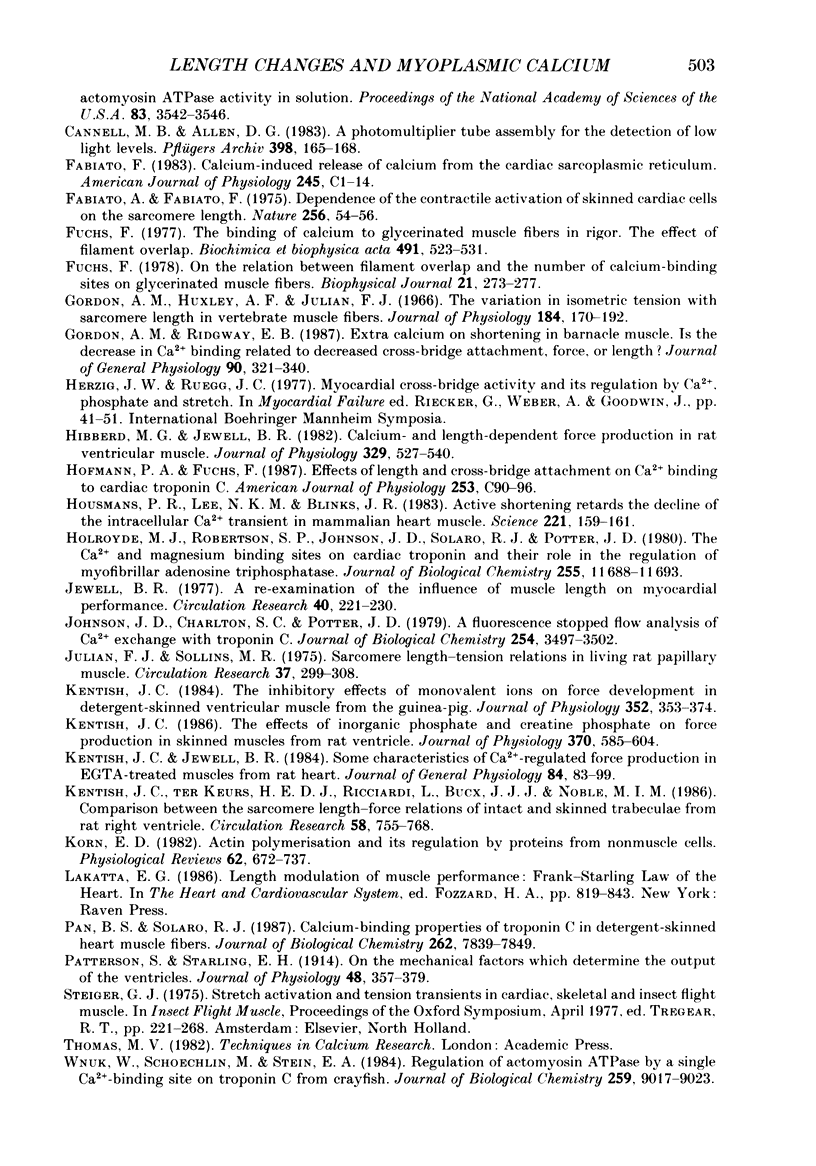
Selected References
These references are in PubMed. This may not be the complete list of references from this article.
- Allen D. G., Blinks J. R., Prendergast F. G. Aequorin luminescence: relation of light emission to calcium concentration--a calcium-independent component. Science. 1977 Mar 11;195(4282):996–998. doi: 10.1126/science.841325. [DOI] [PubMed] [Google Scholar]
- Allen D. G., Jewell B. R., Murray J. W. The contribution of activation processes to the length-tension relation of cardiac muscle. Nature. 1974 Apr 12;248(449):606–607. doi: 10.1038/248606a0. [DOI] [PubMed] [Google Scholar]
- Allen D. G., Kentish J. C. The cellular basis of the length-tension relation in cardiac muscle. J Mol Cell Cardiol. 1985 Sep;17(9):821–840. doi: 10.1016/s0022-2828(85)80097-3. [DOI] [PubMed] [Google Scholar]
- Allen D. G., Kurihara S. The effects of muscle length on intracellular calcium transients in mammalian cardiac muscle. J Physiol. 1982 Jun;327:79–94. doi: 10.1113/jphysiol.1982.sp014221. [DOI] [PMC free article] [PubMed] [Google Scholar]
- Ashley C. C., Moisescu D. G., Rose R. M. Proceedings: Aequorin-light and tension responses from bundles of myofibrils following a sudden change in free calcium. J Physiol. 1974 Sep;241(2):104P–106P. [PubMed] [Google Scholar]
- Baker P. F., Hodgkin A. L., Ridgway E. B. Depolarization and calcium entry in squid giant axons. J Physiol. 1971 Nov;218(3):709–755. doi: 10.1113/jphysiol.1971.sp009641. [DOI] [PMC free article] [PubMed] [Google Scholar]
- Bremel R. D., Weber A. Cooperation within actin filament in vertebrate skeletal muscle. Nat New Biol. 1972 Jul 26;238(82):97–101. doi: 10.1038/newbio238097a0. [DOI] [PubMed] [Google Scholar]
- Brenner B., Eisenberg E. Rate of force generation in muscle: correlation with actomyosin ATPase activity in solution. Proc Natl Acad Sci U S A. 1986 May;83(10):3542–3546. doi: 10.1073/pnas.83.10.3542. [DOI] [PMC free article] [PubMed] [Google Scholar]
- Cannell M. B., Allen D. G. A photomultiplier tube assembly for the detection of low light levels. Pflugers Arch. 1983 Jul;398(2):165–168. doi: 10.1007/BF00581066. [DOI] [PubMed] [Google Scholar]
- Fabiato A., Fabiato F. Dependence of the contractile activation of skinned cardiac cells on the sarcomere length. Nature. 1975 Jul 3;256(5512):54–56. doi: 10.1038/256054a0. [DOI] [PubMed] [Google Scholar]
- Fuchs F. On the relation between filament overlap and the number of calcium-binding sites on glycerinated muscle fibers. Biophys J. 1978 Mar;21(3):273–277. doi: 10.1016/S0006-3495(78)85524-6. [DOI] [PMC free article] [PubMed] [Google Scholar]
- Fuchs F. The binding of calcium to glycerinated muscle fibers in rigor. The effect of filament overlap. Biochim Biophys Acta. 1977 Apr 25;491(2):523–531. doi: 10.1016/0005-2795(77)90297-5. [DOI] [PubMed] [Google Scholar]
- Gordon A. M., Huxley A. F., Julian F. J. The variation in isometric tension with sarcomere length in vertebrate muscle fibres. J Physiol. 1966 May;184(1):170–192. doi: 10.1113/jphysiol.1966.sp007909. [DOI] [PMC free article] [PubMed] [Google Scholar]
- Gordon A. M., Ridgway E. B. Extra calcium on shortening in barnacle muscle. Is the decrease in calcium binding related to decreased cross-bridge attachment, force, or length? J Gen Physiol. 1987 Sep;90(3):321–340. doi: 10.1085/jgp.90.3.321. [DOI] [PMC free article] [PubMed] [Google Scholar]
- Hibberd M. G., Jewell B. R. Calcium- and length-dependent force production in rat ventricular muscle. J Physiol. 1982 Aug;329:527–540. doi: 10.1113/jphysiol.1982.sp014317. [DOI] [PMC free article] [PubMed] [Google Scholar]
- Hofmann P. A., Fuchs F. Effect of length and cross-bridge attachment on Ca2+ binding to cardiac troponin C. Am J Physiol. 1987 Jul;253(1 Pt 1):C90–C96. doi: 10.1152/ajpcell.1987.253.1.C90. [DOI] [PubMed] [Google Scholar]
- Housmans P. R., Lee N. K., Blinks J. R. Active shortening retards the decline of the intracellular calcium transient in mammalian heart muscle. Science. 1983 Jul 8;221(4606):159–161. doi: 10.1126/science.6857274. [DOI] [PubMed] [Google Scholar]
- Jewell B. R. A reexamination of the influence of muscle length on myocardial performance. Circ Res. 1977 Mar;40(3):221–230. doi: 10.1161/01.res.40.3.221. [DOI] [PubMed] [Google Scholar]
- Johnson J. D., Charlton S. C., Potter J. D. A fluorescence stopped flow analysis of Ca2+ exchange with troponin C. J Biol Chem. 1979 May 10;254(9):3497–3502. [PubMed] [Google Scholar]
- Julian F. J., Sollins M. R. Sarcomere length-tension relations in living rat papillary muscle. Circ Res. 1975 Sep;37(3):299–308. doi: 10.1161/01.res.37.3.299. [DOI] [PubMed] [Google Scholar]
- Kentish J. C., Jewell B. R. Some characteristics of Ca2+- regulated force production in EGTA-treated muscles from rat heart. J Gen Physiol. 1984 Jul;84(1):83–99. doi: 10.1085/jgp.84.1.83. [DOI] [PMC free article] [PubMed] [Google Scholar]
- Kentish J. C. The effects of inorganic phosphate and creatine phosphate on force production in skinned muscles from rat ventricle. J Physiol. 1986 Jan;370:585–604. doi: 10.1113/jphysiol.1986.sp015952. [DOI] [PMC free article] [PubMed] [Google Scholar]
- Kentish J. C. The inhibitory effects of monovalent ions on force development in detergent-skinned ventricular muscle from guinea-pig. J Physiol. 1984 Jul;352:353–374. doi: 10.1113/jphysiol.1984.sp015296. [DOI] [PMC free article] [PubMed] [Google Scholar]
- Kentish J. C., ter Keurs H. E., Ricciardi L., Bucx J. J., Noble M. I. Comparison between the sarcomere length-force relations of intact and skinned trabeculae from rat right ventricle. Influence of calcium concentrations on these relations. Circ Res. 1986 Jun;58(6):755–768. doi: 10.1161/01.res.58.6.755. [DOI] [PubMed] [Google Scholar]
- Korn E. D. Actin polymerization and its regulation by proteins from nonmuscle cells. Physiol Rev. 1982 Apr;62(2):672–737. doi: 10.1152/physrev.1982.62.2.672. [DOI] [PubMed] [Google Scholar]
- Pan B. S., Solaro R. J. Calcium-binding properties of troponin C in detergent-skinned heart muscle fibers. J Biol Chem. 1987 Jun 5;262(16):7839–7849. [PubMed] [Google Scholar]
- Patterson S. W., Starling E. H. On the mechanical factors which determine the output of the ventricles. J Physiol. 1914 Sep 8;48(5):357–379. doi: 10.1113/jphysiol.1914.sp001669. [DOI] [PMC free article] [PubMed] [Google Scholar]
- Wnuk W., Schoechlin M., Stein E. A. Regulation of actomyosin ATPase by a single calcium-binding site on troponin C from crayfish. J Biol Chem. 1984 Jul 25;259(14):9017–9023. [PubMed] [Google Scholar]