Abstract
1. Calcium permeabilization of inosine-fed human red cells using the divalent cation ionophore A23187 induces pump-leak steady states in which the mean total calcium content of the cells may be held below electrochemical equilibrium for hours. A new method developed to detect and separate cells with different calcium contents revealed a striking heterogeneity of calcium contents in subpopulations of cells in pump-leak steady state (García-Sancho & Lew, 1988a). Most of the mean total cell calcium was found within a fraction of cells rendered dense by the separation procedure (H cells), with relatively little within the remaining light cells (L cells). The experiments in this paper were designed to study the nature and origin of the observed heterogeneity. 2. The fraction of steady-state H cells increased, and the mean ATP content of the cells fell, both linearly, as calcium influx was increased. The H/L divide is therefore the result of a continuous variation in cell properties. When calcium influx was above about 30 mmol/(l cells.h), all cells became dense, calcium distribution was at or near equilibrium, and cell ATP was 0.1-0.2 mmol/l cells. 3. Inosine-fed cells, subjected to ionophore-mediated net calcium influx of 13-15 mmol/(l cells.h), attained a steady state with mean calcium contents far below equilibrium. After ionophore removal and reincubation in calcium-free media, the initial calcium efflux was only a fraction of that required to sustain the previous steady state (less than 25% for H cells, and less than 2% for L cells). The ATP content of L cells was normal whereas that of H cells was irreversibly reduced. These results revealed a paradoxical discrepancy between leak influx and calcium pump efflux in H and L cells which were supposed to have been in steady-state pump-leak balance. 4. The changes in cell calcium and ATP were followed in time after calcium permeabilization to characterize the development of steady-state heterogeneity. Calcium influx triggered a sharp peak in the H cell fraction within 15 s of permeabilization. The mean calcium content of H cells increased towards steady-state values as their fraction decreased; most other cells transferred from H to L density fractions (HL cells) within the first 5 min of permeabilization. 5. In substrate-starved cells calcium influx triggered an immediate fall in cell ATP, steeper in H cells than in L cells. The initial calcium and density transients were unattected.(ABSTRACT TRUNCATED AT 400 WORDS)
Full text
PDF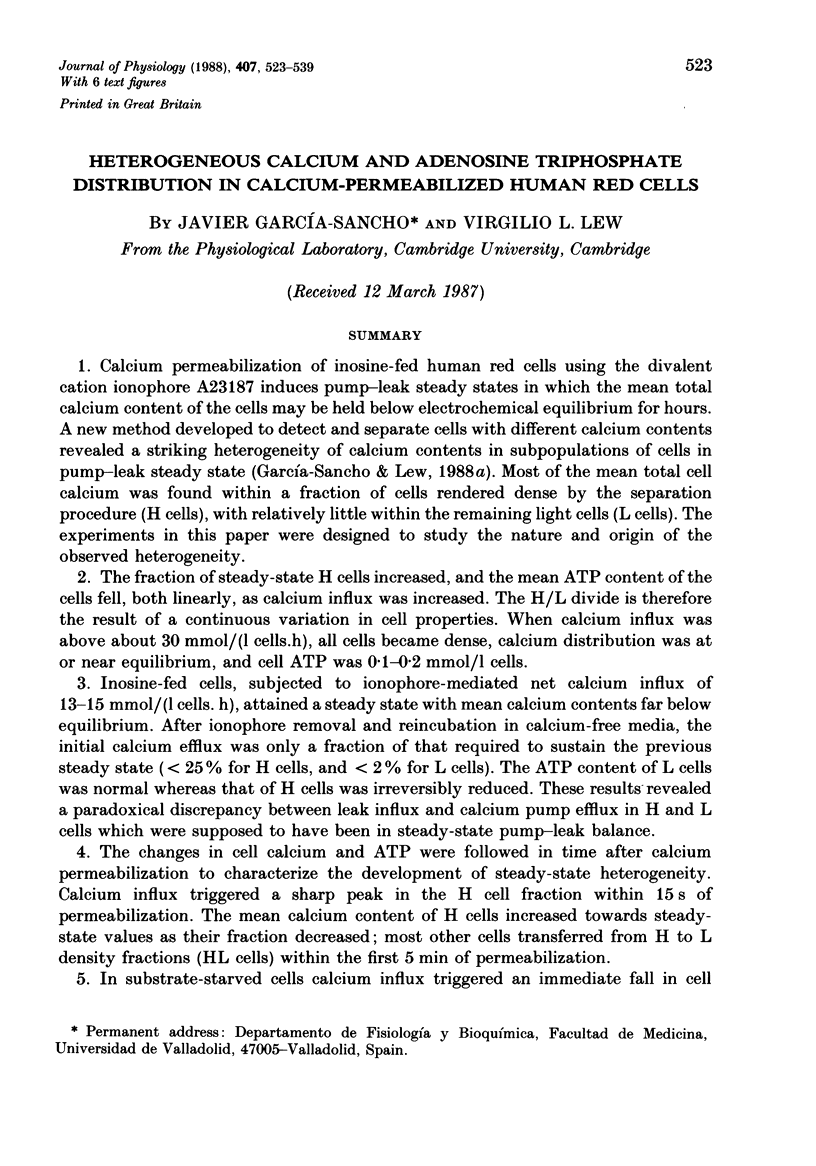
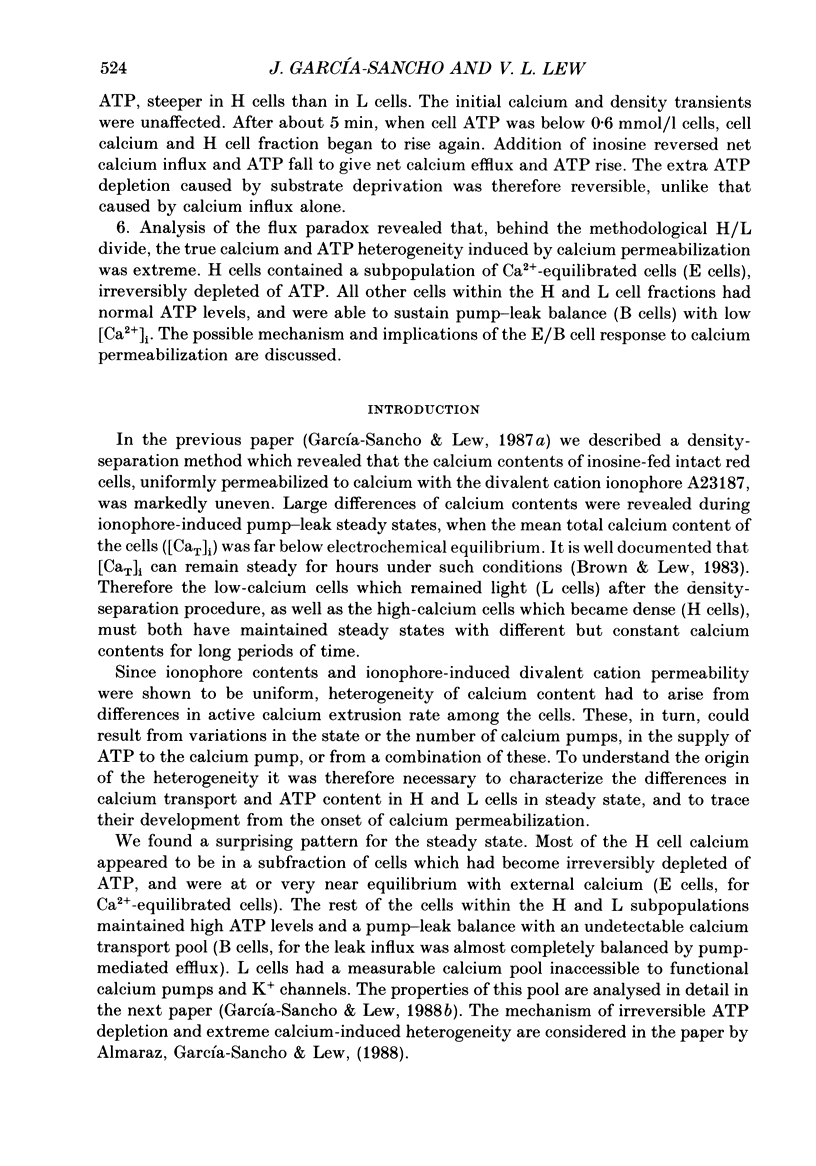
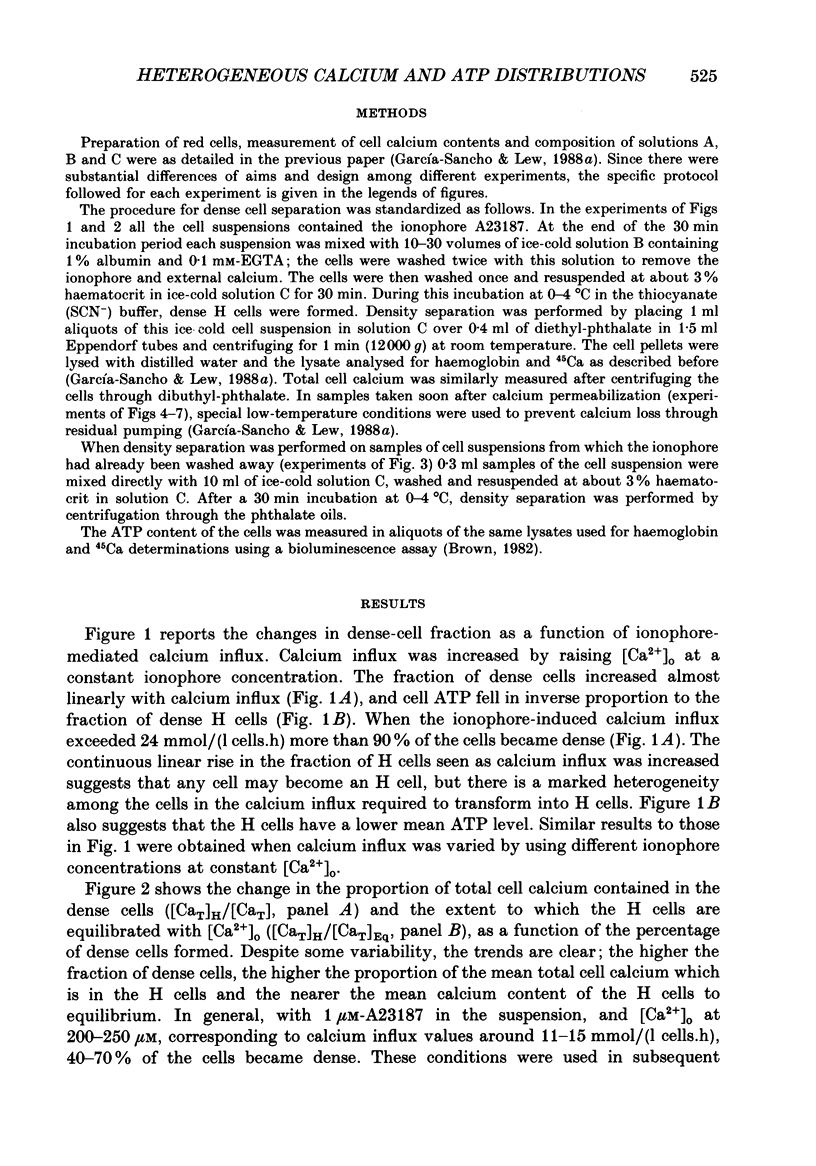
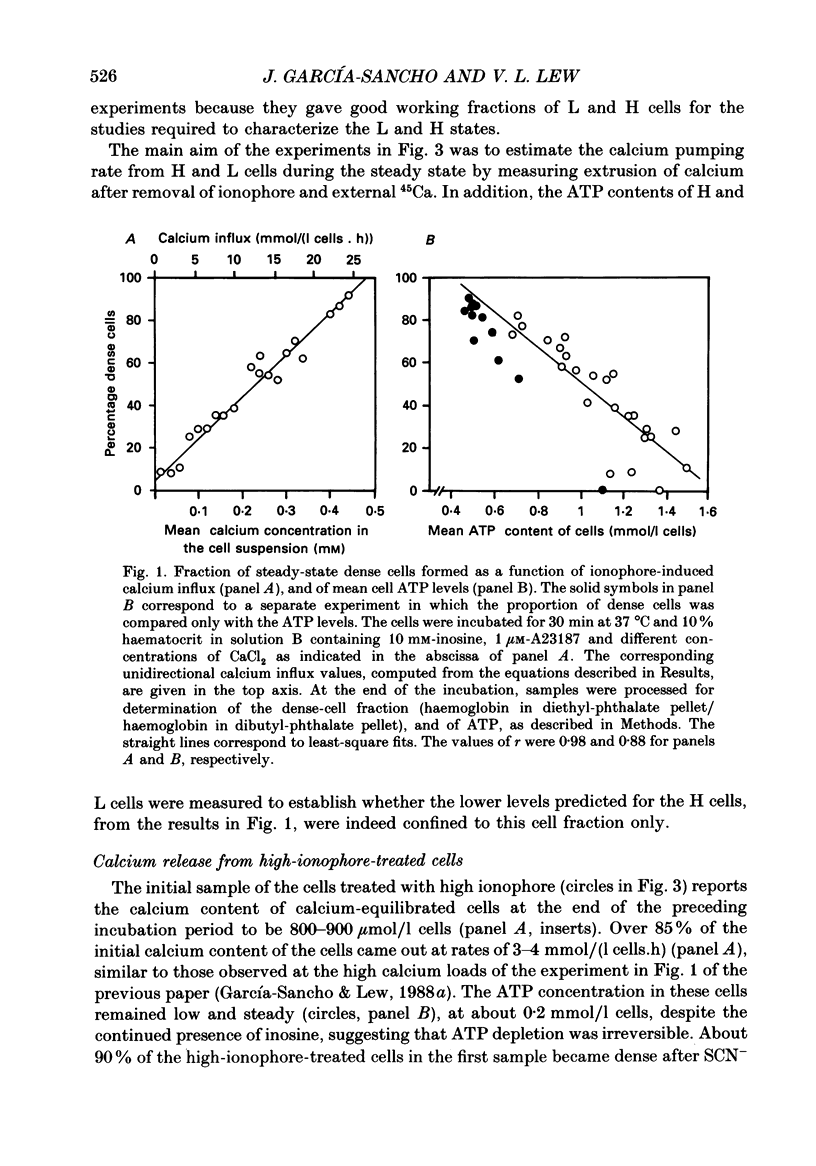
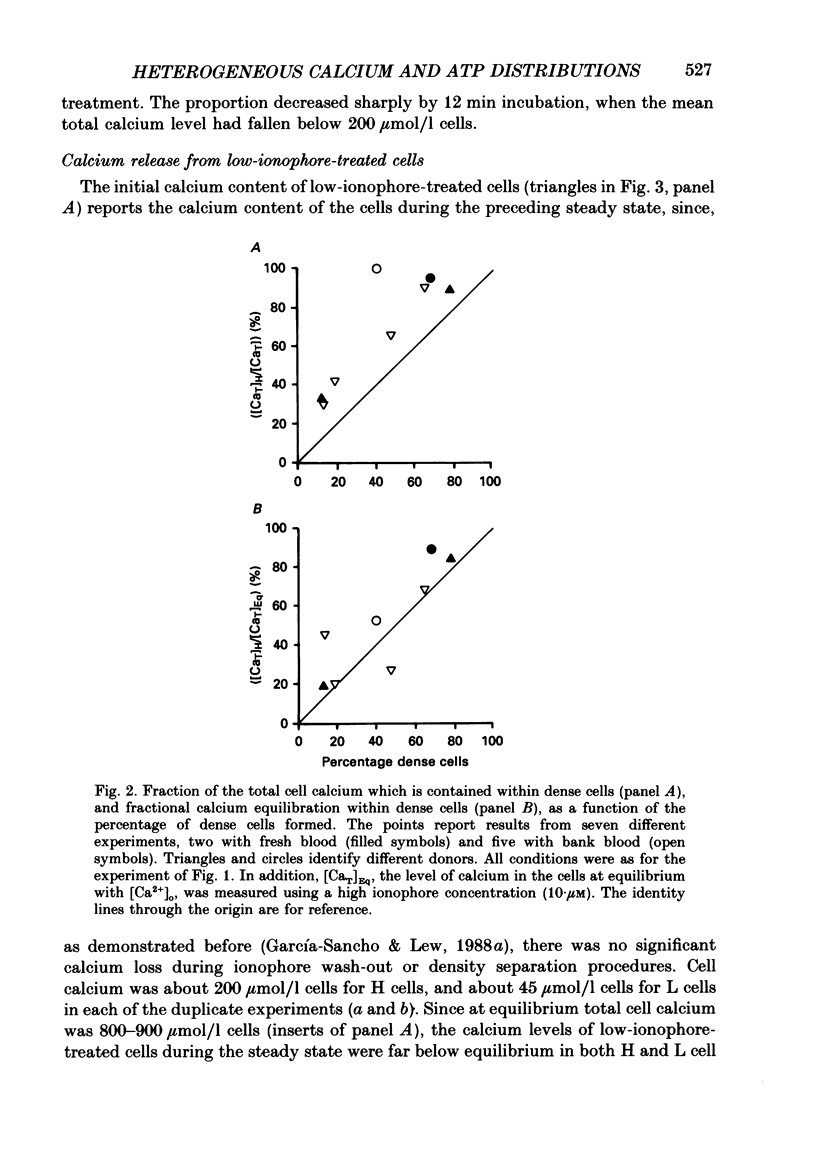
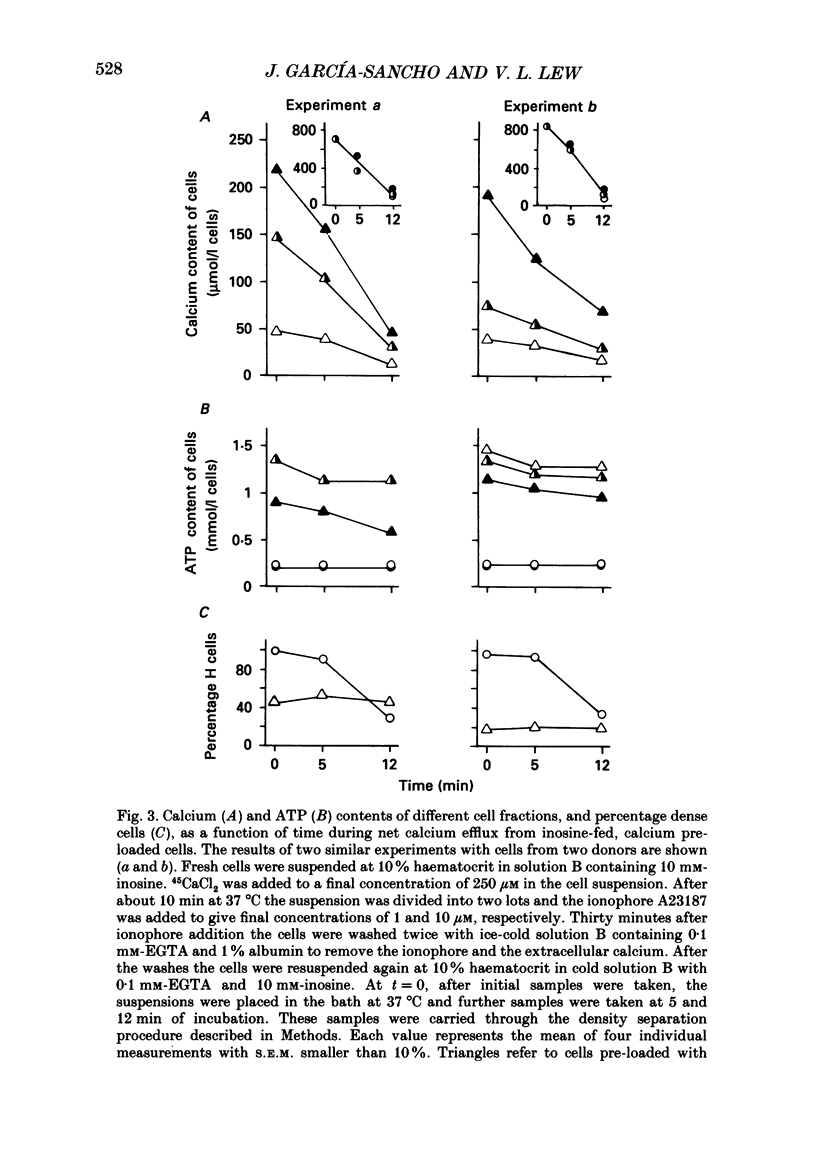
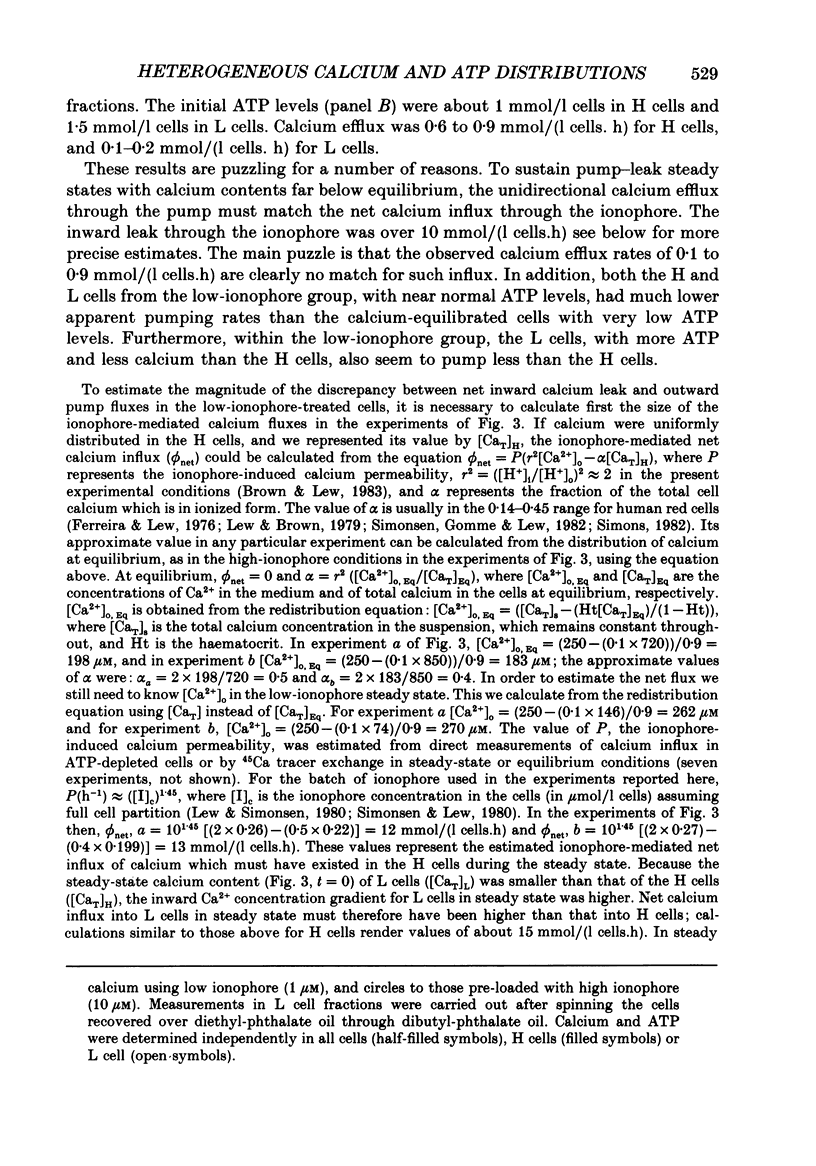
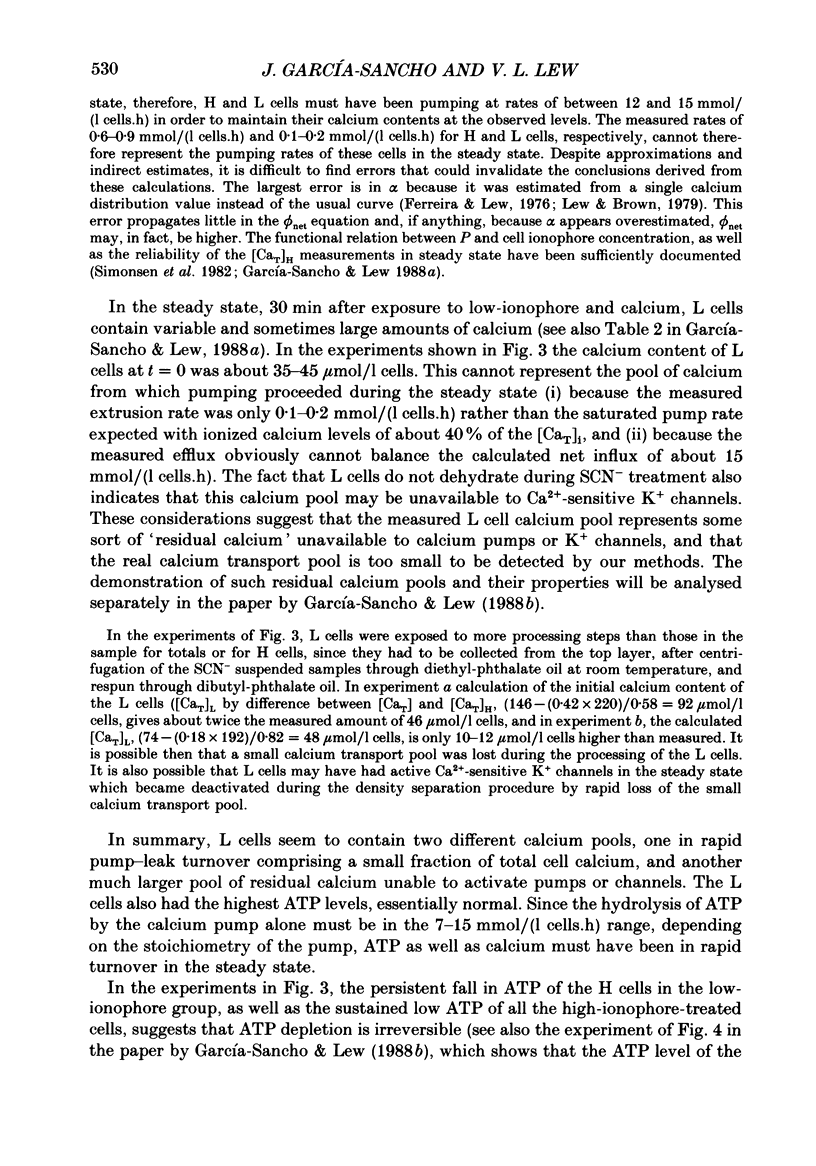
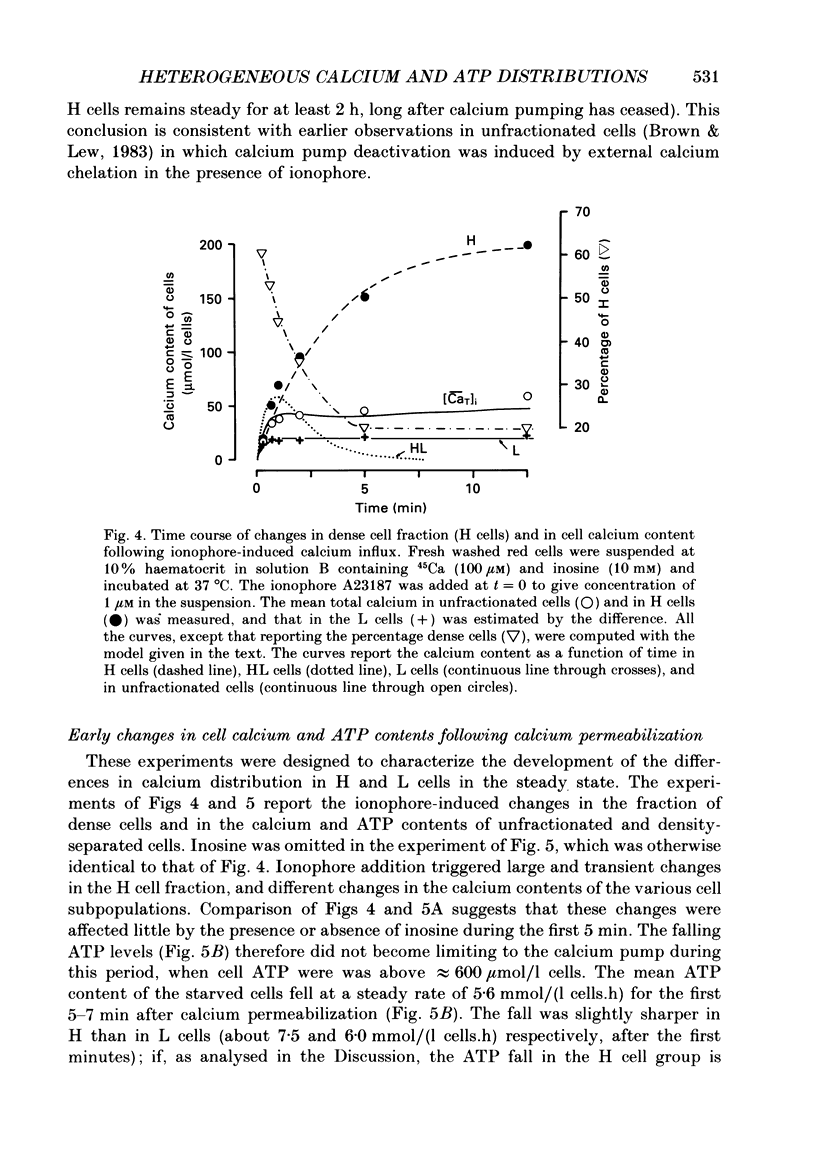
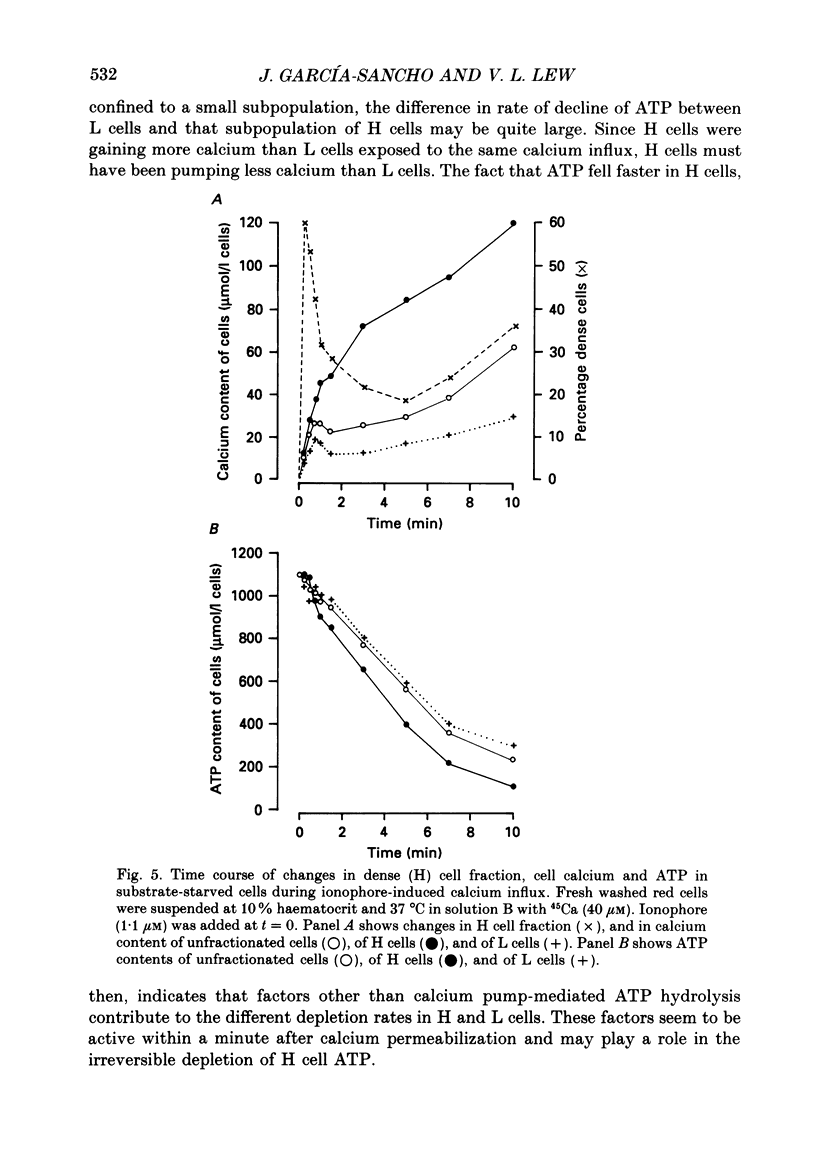
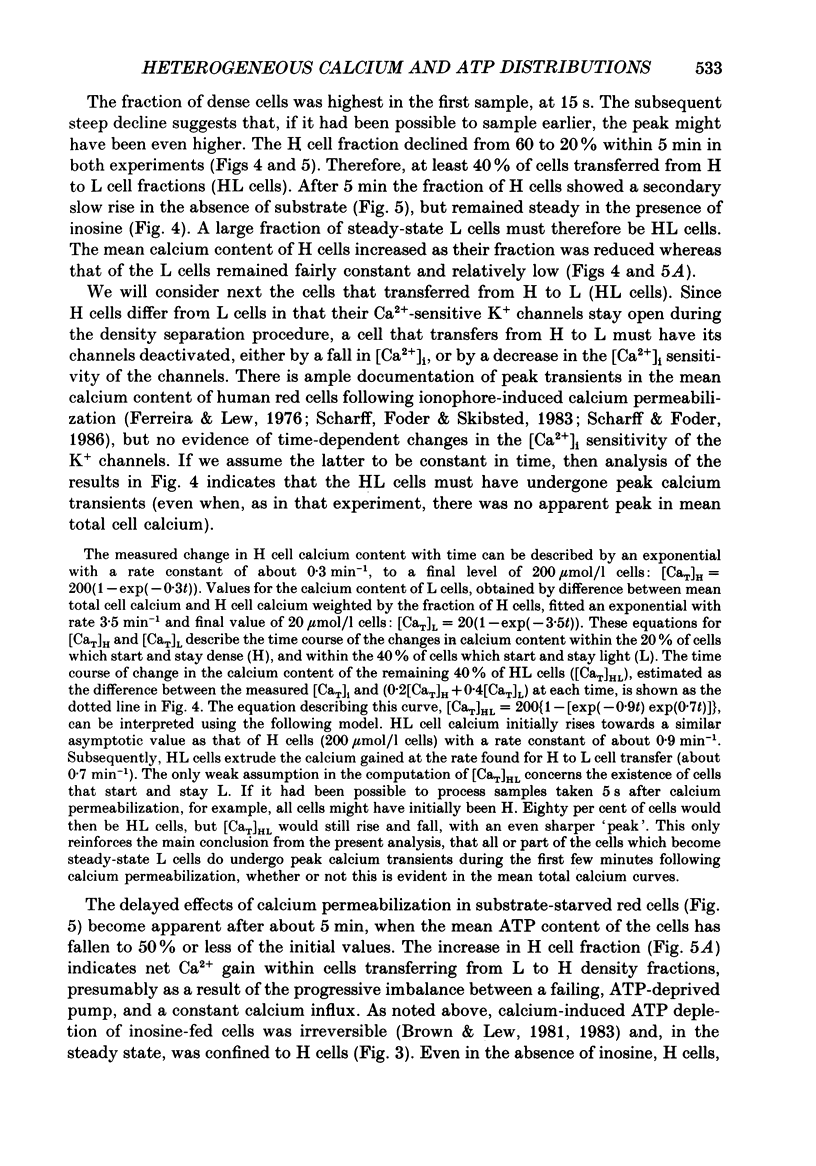
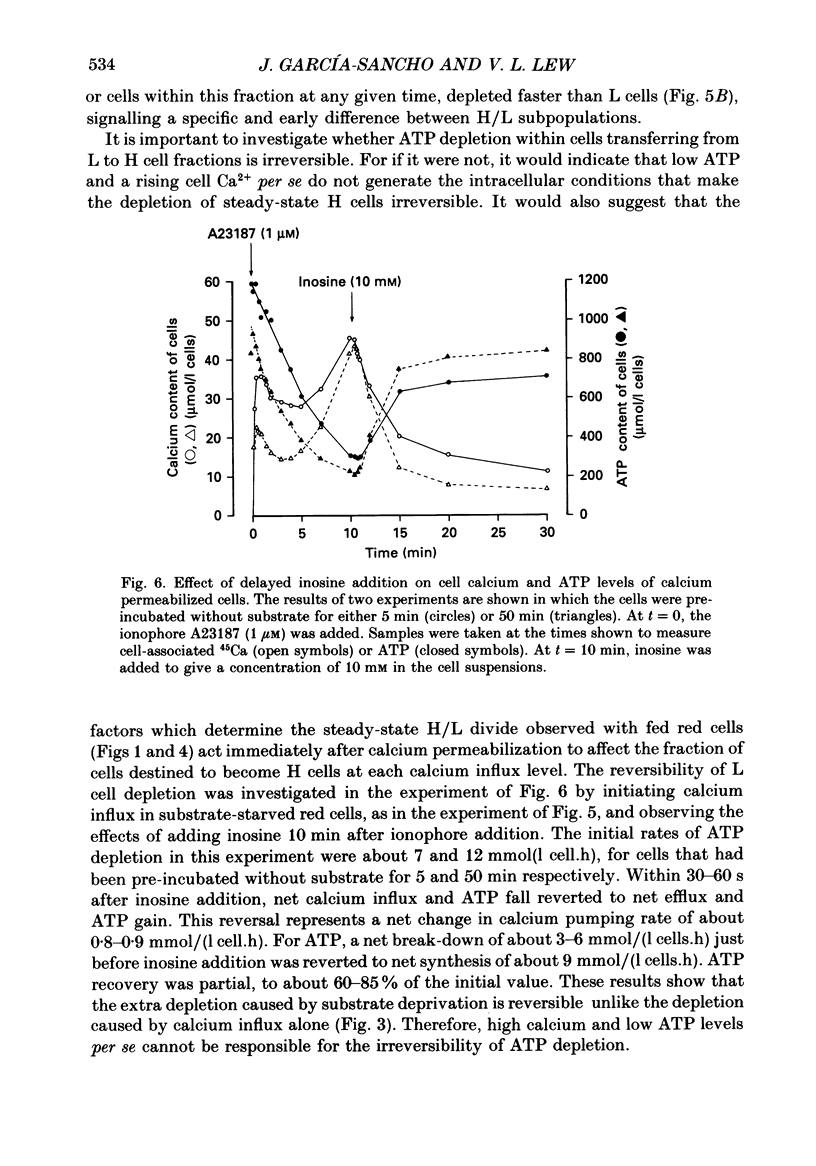
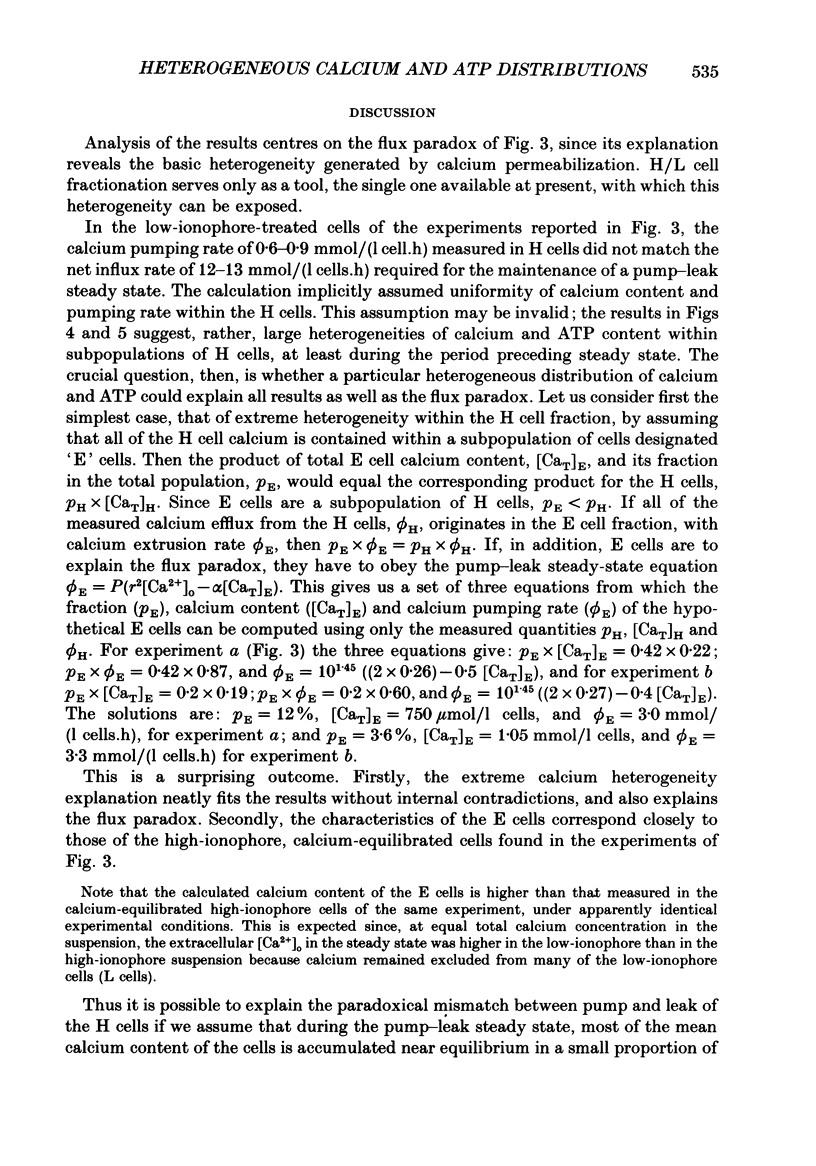
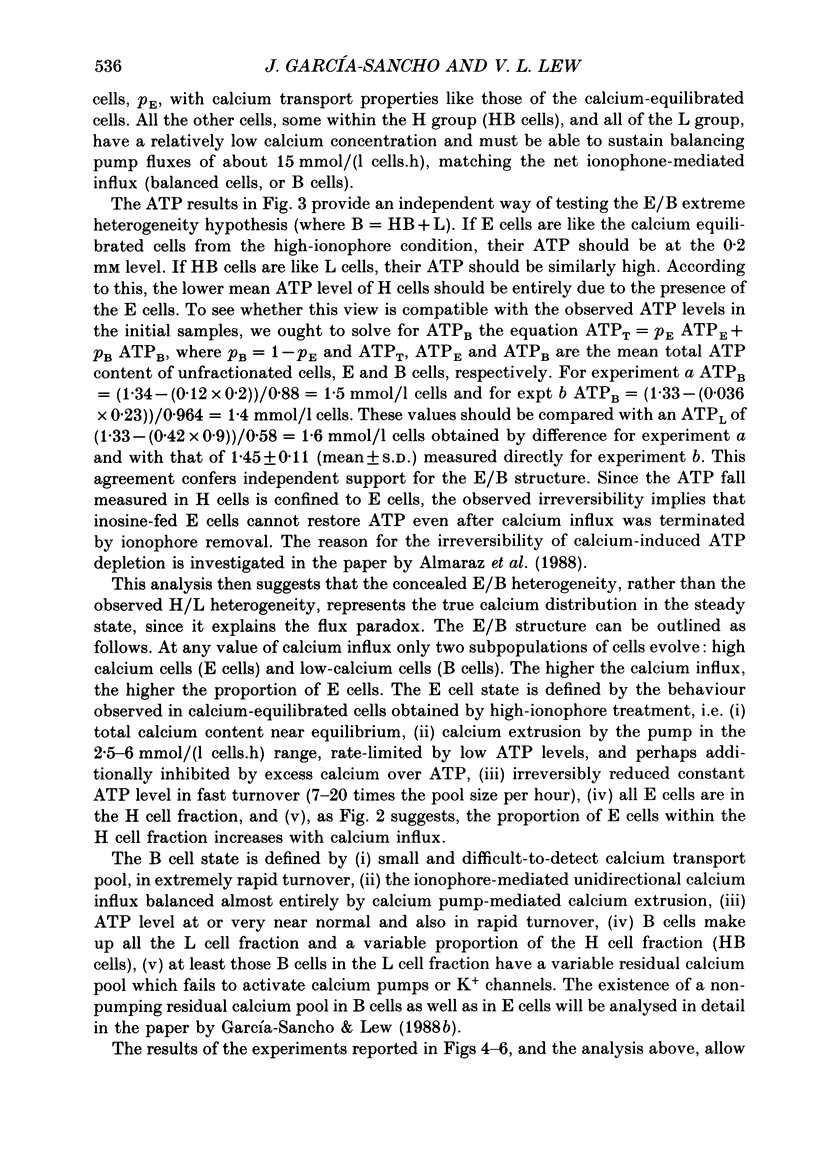
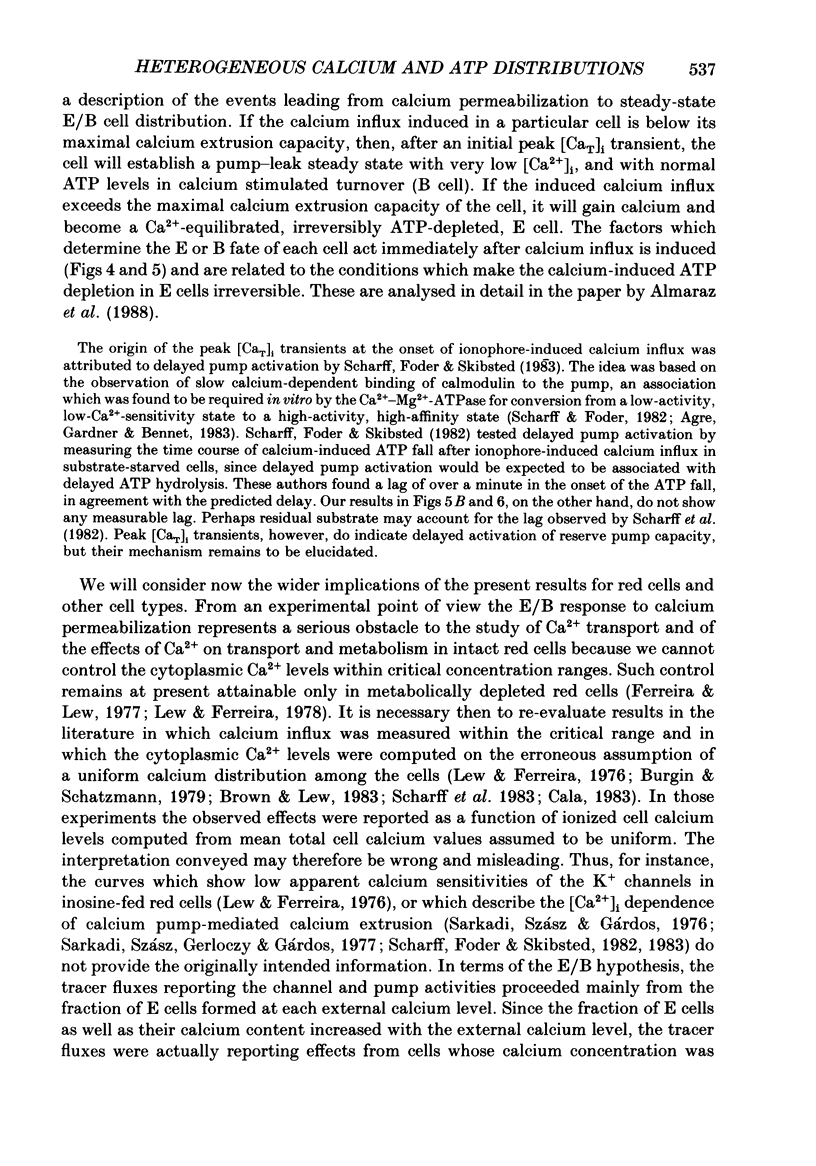
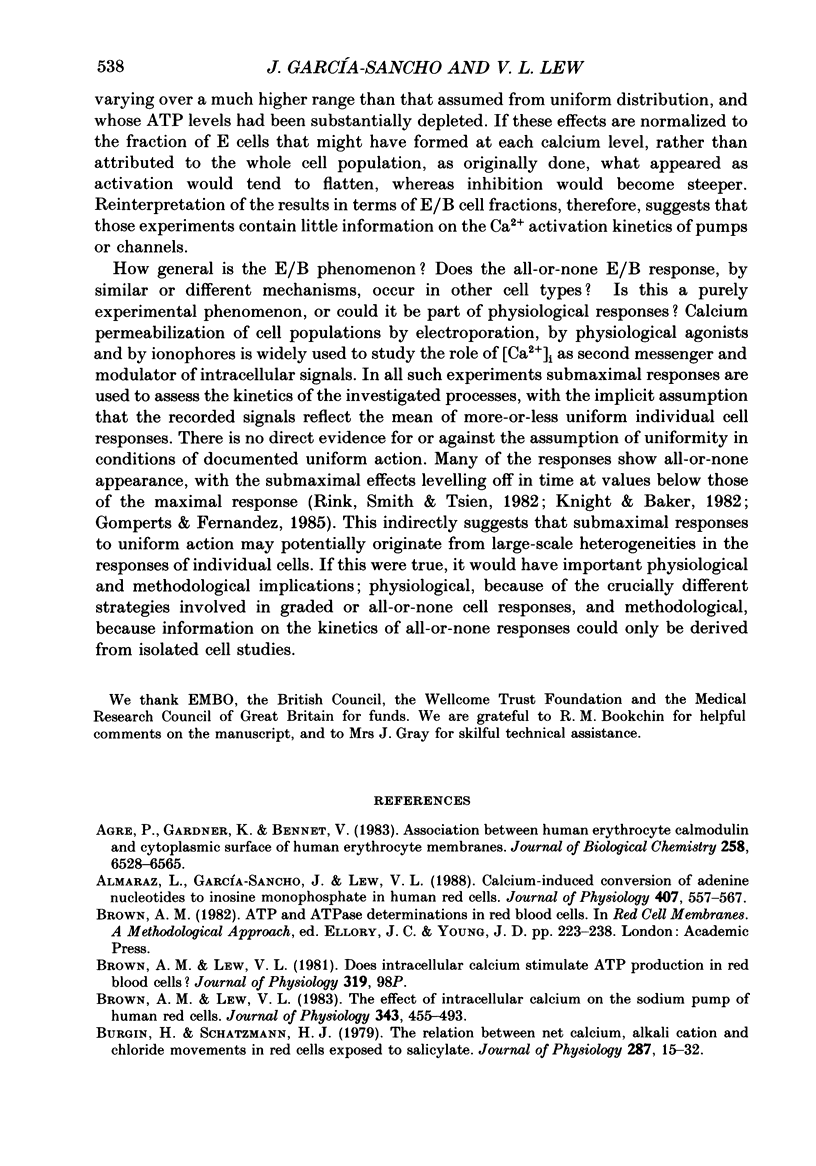
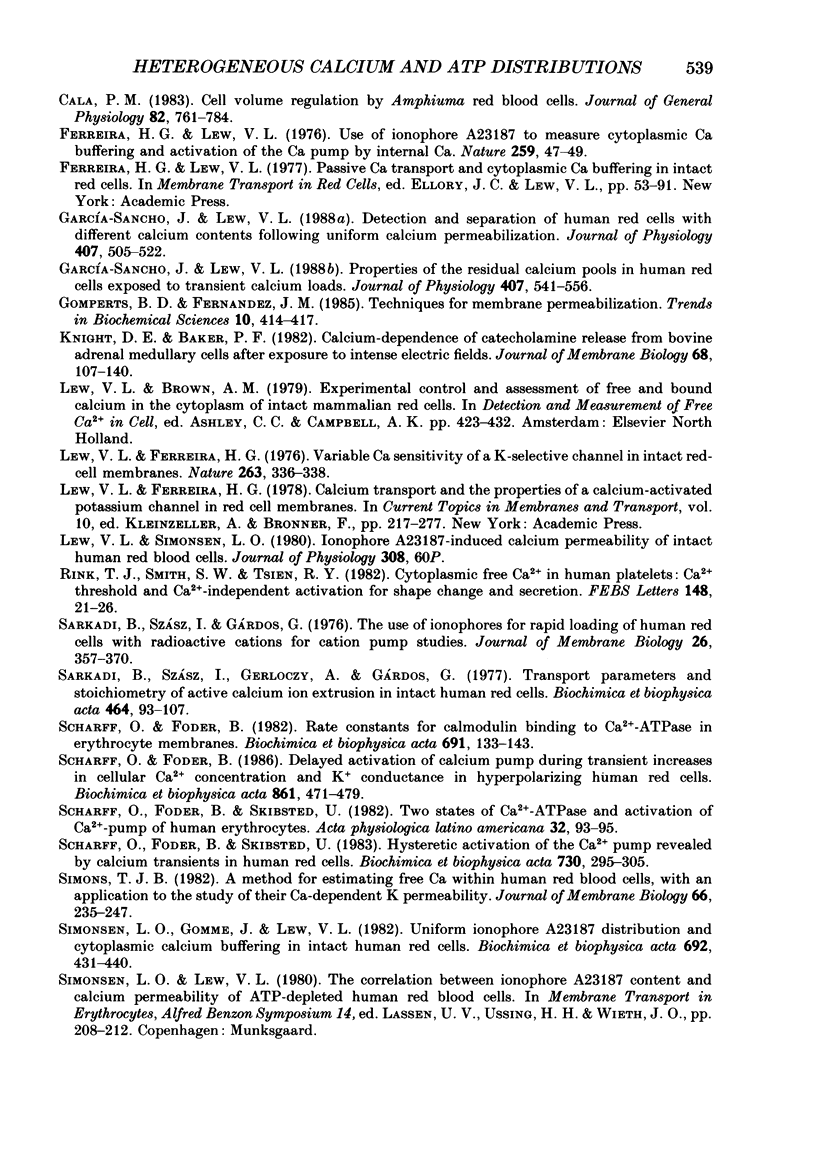
Selected References
These references are in PubMed. This may not be the complete list of references from this article.
- Almaraz L., García-Sancho J., Lew V. L. Calcium-induced conversion of adenine nucleotides to inosine monophosphate in human red cells. J Physiol. 1988 Dec;407:557–567. doi: 10.1113/jphysiol.1988.sp017431. [DOI] [PMC free article] [PubMed] [Google Scholar]
- Brown A. M., Lew V. L. The effect of intracellular calcium on the sodium pump of human red cells. J Physiol. 1983 Oct;343:455–493. doi: 10.1113/jphysiol.1983.sp014904. [DOI] [PMC free article] [PubMed] [Google Scholar]
- Bürgin H., Schatzmann H. J. The relation between net calcium, alkali cation and chloride movements in red cells exposed to salicylate. J Physiol. 1979 Feb;287:15–32. doi: 10.1113/jphysiol.1979.sp012642. [DOI] [PMC free article] [PubMed] [Google Scholar]
- Cala P. M. Cell volume regulation by Amphiuma red blood cells. The role of Ca+2 as a modulator of alkali metal/H+ exchange. J Gen Physiol. 1983 Dec;82(6):761–784. doi: 10.1085/jgp.82.6.761. [DOI] [PMC free article] [PubMed] [Google Scholar]
- Ferreira H. G., Lew V. L. Use of ionophore A23187 to measure cytoplasmic Ca buffering and activation of the Ca pump by internal Ca. Nature. 1976 Jan 1;259(5538):47–49. doi: 10.1038/259047a0. [DOI] [PubMed] [Google Scholar]
- García-Sancho J., Lew V. L. Detection and separation of human red cells with different calcium contents following uniform calcium permeabilization. J Physiol. 1988 Dec;407:505–522. doi: 10.1113/jphysiol.1988.sp017428. [DOI] [PMC free article] [PubMed] [Google Scholar]
- García-Sancho J., Lew V. L. Properties of the residual calcium pools in human red cells exposed to transient calcium loads. J Physiol. 1988 Dec;407:541–556. doi: 10.1113/jphysiol.1988.sp017430. [DOI] [PMC free article] [PubMed] [Google Scholar]
- Knight D. E., Baker P. F. Calcium-dependence of catecholamine release from bovine adrenal medullary cells after exposure to intense electric fields. J Membr Biol. 1982;68(2):107–140. doi: 10.1007/BF01872259. [DOI] [PubMed] [Google Scholar]
- Lew V. L., Ferreira H. G. Variable Ca sensitivity of a K-selective channel in intact red-cell membranes. Nature. 1976 Sep 23;263(5575):336–338. doi: 10.1038/263336a0. [DOI] [PubMed] [Google Scholar]
- Rink T. J., Smith S. W., Tsien R. Y. Cytoplasmic free Ca2+ in human platelets: Ca2+ thresholds and Ca-independent activation for shape-change and secretion. FEBS Lett. 1982 Nov 1;148(1):21–26. doi: 10.1016/0014-5793(82)81234-9. [DOI] [PubMed] [Google Scholar]
- Sarkadi B., Szász I., Gerlóczy A., Gárdos G. Transport parameters and stoichiometry of active calcium ion extrusion in intact human red cells. Biochim Biophys Acta. 1977 Jan 4;464(1):93–107. doi: 10.1016/0005-2736(77)90373-x. [DOI] [PubMed] [Google Scholar]
- Sarkadi B., Szász I., Gárdos G. The use of ionophores of rapid loading of human red cells with radioactive cations for cation-pump studies. J Membr Biol. 1976 May;26(4):357–370. doi: 10.1007/BF01868883. [DOI] [PubMed] [Google Scholar]
- Scharff O., Foder B. Delayed activation of calcium pump during transient increases in cellular Ca2+ concentration and K+ conductance in hyperpolarizing human red cells. Biochim Biophys Acta. 1986 Oct 23;861(3):471–479. doi: 10.1016/0005-2736(86)90456-6. [DOI] [PubMed] [Google Scholar]
- Scharff O., Foder B. Rate constants for calmodulin binding to Ca2+-ATPase in erythrocyte membranes. Biochim Biophys Acta. 1982 Sep 24;691(1):133–143. doi: 10.1016/0005-2736(82)90222-x. [DOI] [PubMed] [Google Scholar]
- Scharff O., Foder B., Skibsted U. Hysteretic activation of the Ca2+ pump revealed by calcium transients in human red cells. Biochim Biophys Acta. 1983 May 5;730(2):295–305. doi: 10.1016/0005-2736(83)90346-2. [DOI] [PubMed] [Google Scholar]
- Simons T. J. A method for estimating free Ca within human red blood cells, with an application to the study of their Ca-dependent K permeability. J Membr Biol. 1982;66(3):235–247. doi: 10.1007/BF01868498. [DOI] [PubMed] [Google Scholar]
- Simonsen L. O., Gomme J., Lew V. L. Uniform ionophore A23187 distribution and cytoplasmic calcium buffering in intact human red cells. Biochim Biophys Acta. 1982 Nov 22;692(3):431–440. doi: 10.1016/0005-2736(82)90394-7. [DOI] [PubMed] [Google Scholar]