Abstract
1. The influence of repetitive activity upon intracellular pH (pHi), intracellular Na+ activity (aNA(i)) and contraction was examined in isolated sheep cardiac Purkinje fibres. Ion-selective microelectrodes were used to measure intracellular Na+ and H+ ion activity. Twitch tension was elicited by field stimulation or by depolarizing pulses applied using a two-microelectrode voltage clamp. Experiments were performed in HEPES-buffered solution equilibrated either with air or 100% O2. 2. An increase in action potential frequency from a basal rate of 0.1 to 1-4 Hz induced a reversible fall in pHi and a reversible rise in aNa(i). These effects reached a steady state 3-10 min following an increase in stimulation frequency, and showed a linear dependence on frequency with a mean slope of 0.023 pH units Hz-1 and 0.57 mmol l-1 Hz-1, respectively. The rise in total intracellular acid and aNa(i) associated with a single action potential was estimated as 5.3 mu equiv l-1 of acid and 3.5 mu equiv l-1 of Na+. 3. At action potential frequencies greater than 1 Hz, the rate-dependent rise in aNa(i) was usually accompanied by a positive force staircase. 4. The fall in pHi following a rate increase also occurred when fibres were bathed in Tyrode solution equilibrated with 23 mM-HCO3- plus nominally 5% CO2/95% O2. In these cases, however, the fall in pHi was halved in magnitude. 5. In fibres exposed to strophanthidin (0.5 microM), the rate-dependent fall in pHi was doubled in magnitude and its time course was more variable than under drug-free conditions. The rate-dependent rise in aiNa was also usually larger in strophanthidin. 6. In order to examine the influence of the rate-dependent acidosis on developed tension, the acidosis was reversed experimentally by adding 2 mmol l-1 NH4Cl to the bathing solution. This produced a rise in pHi accompanied by a large increase in twitch tension. Such an effect of pHi upon tension was quantitatively similar to that observed in previous work on Purkinje fibres (Vaughan-Jones, Eisner & Lederer, 1987). 7. It is concluded that the rate dependence of pHi will influence both the magnitude and the time course of an inotropic response to a change in heart rate.
Full text
PDF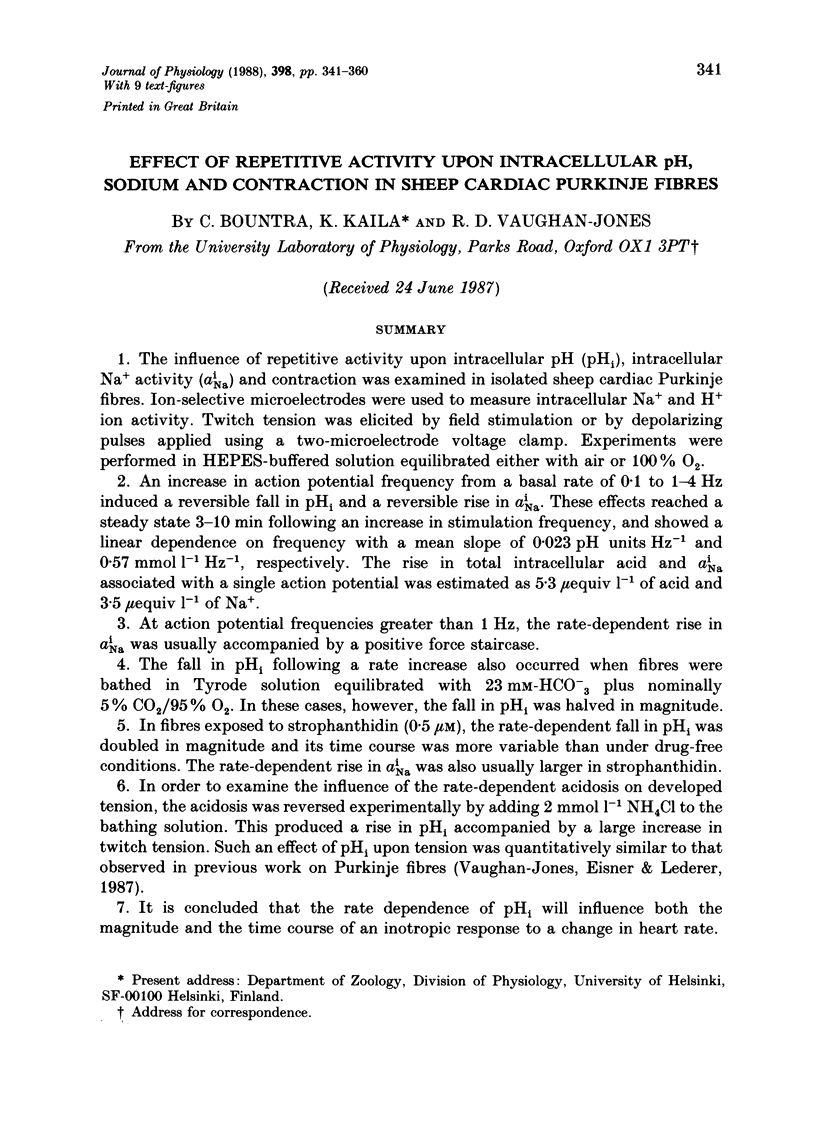
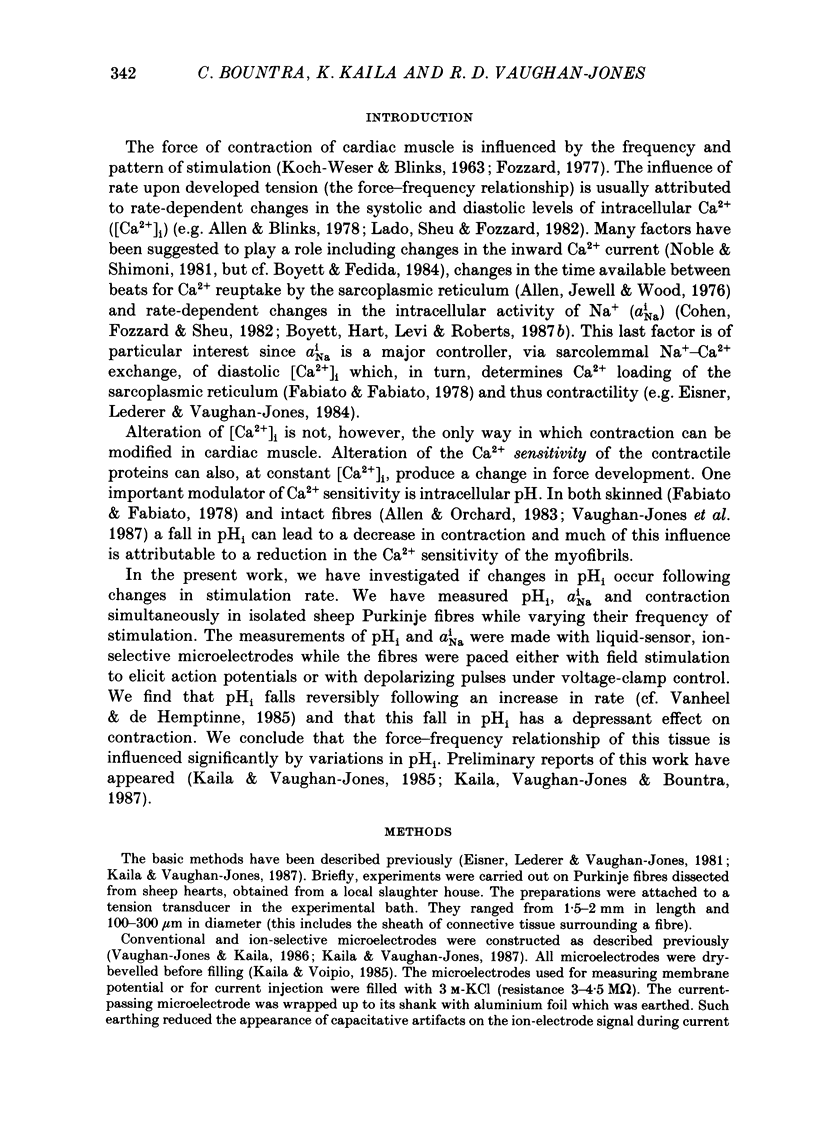
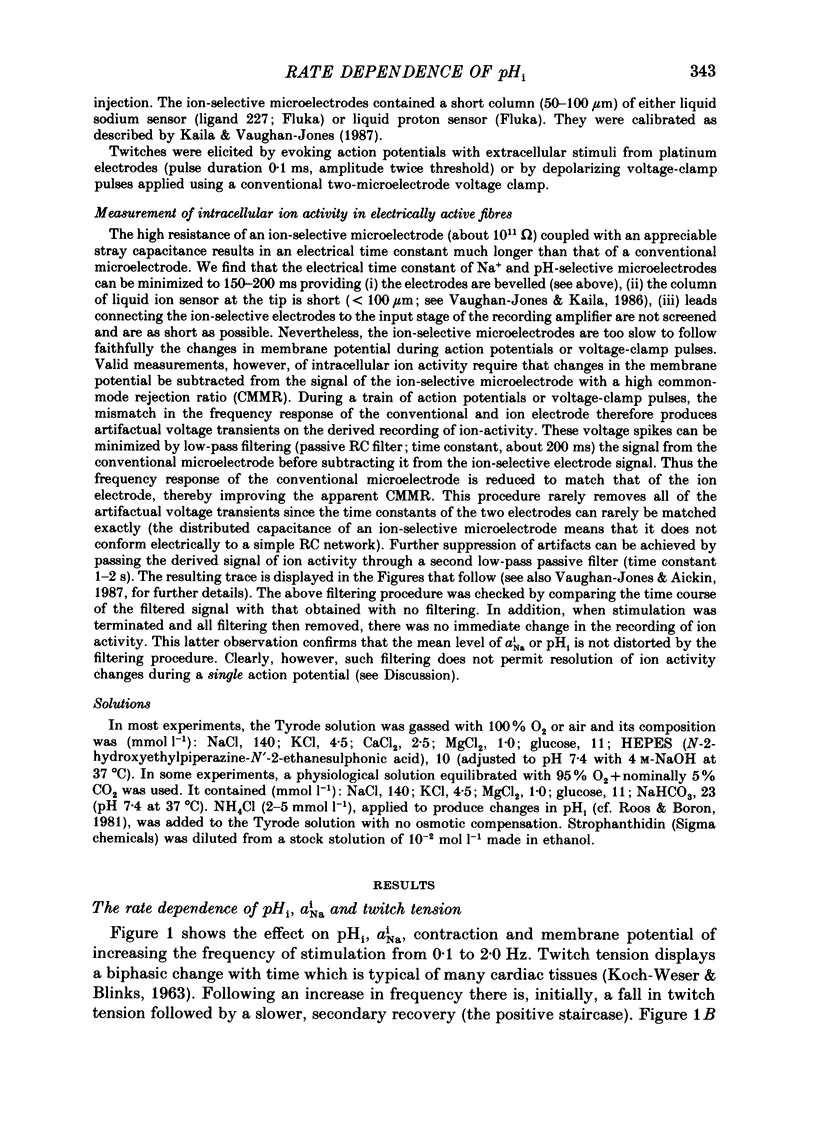
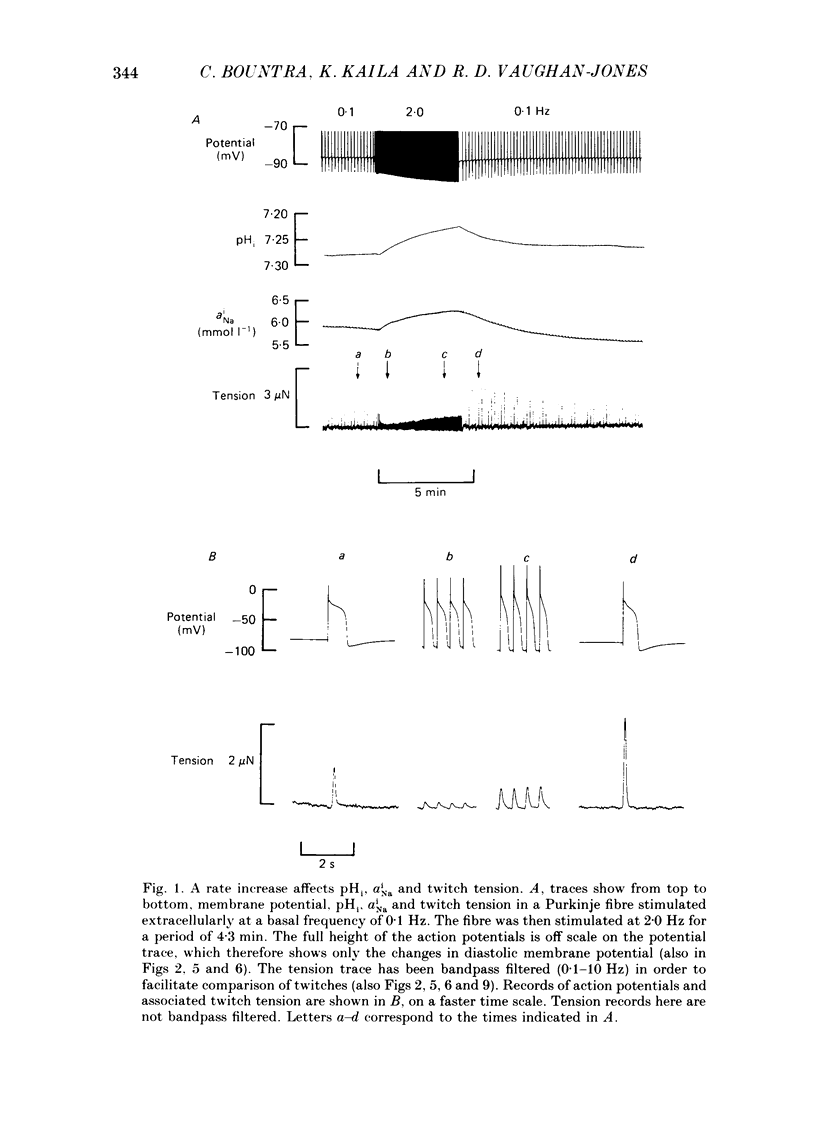
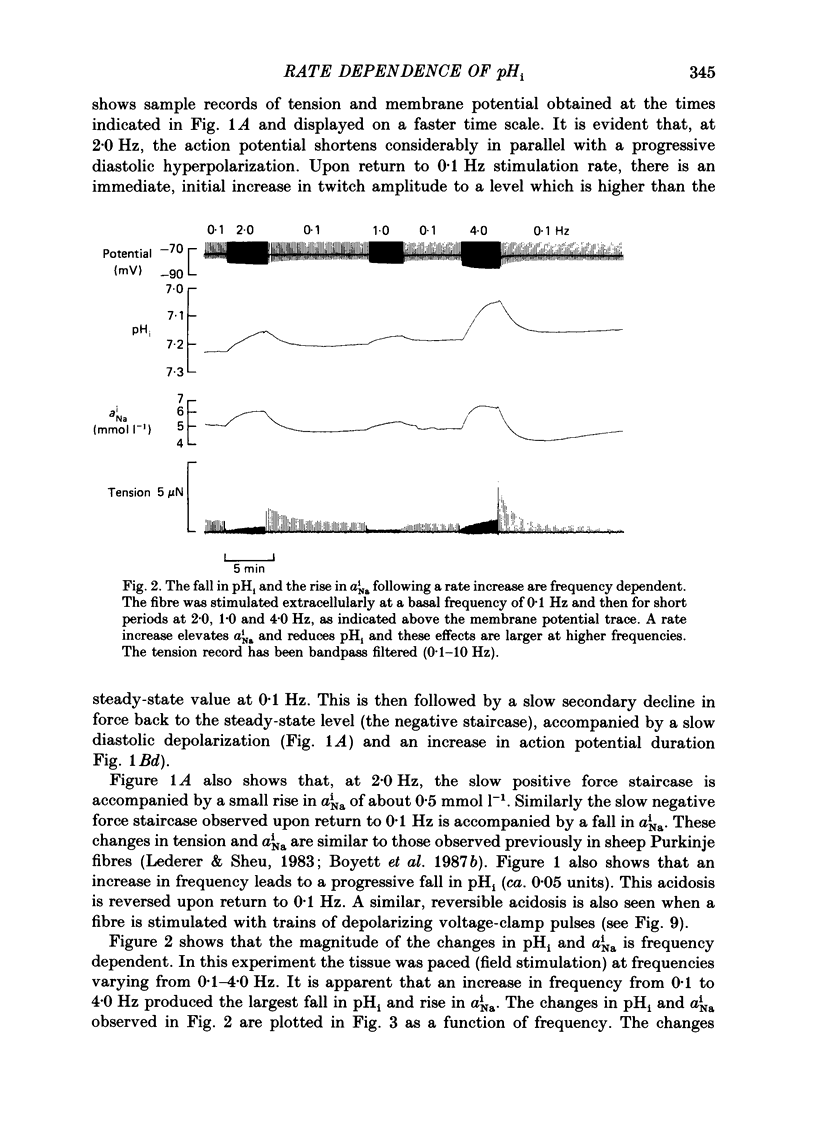
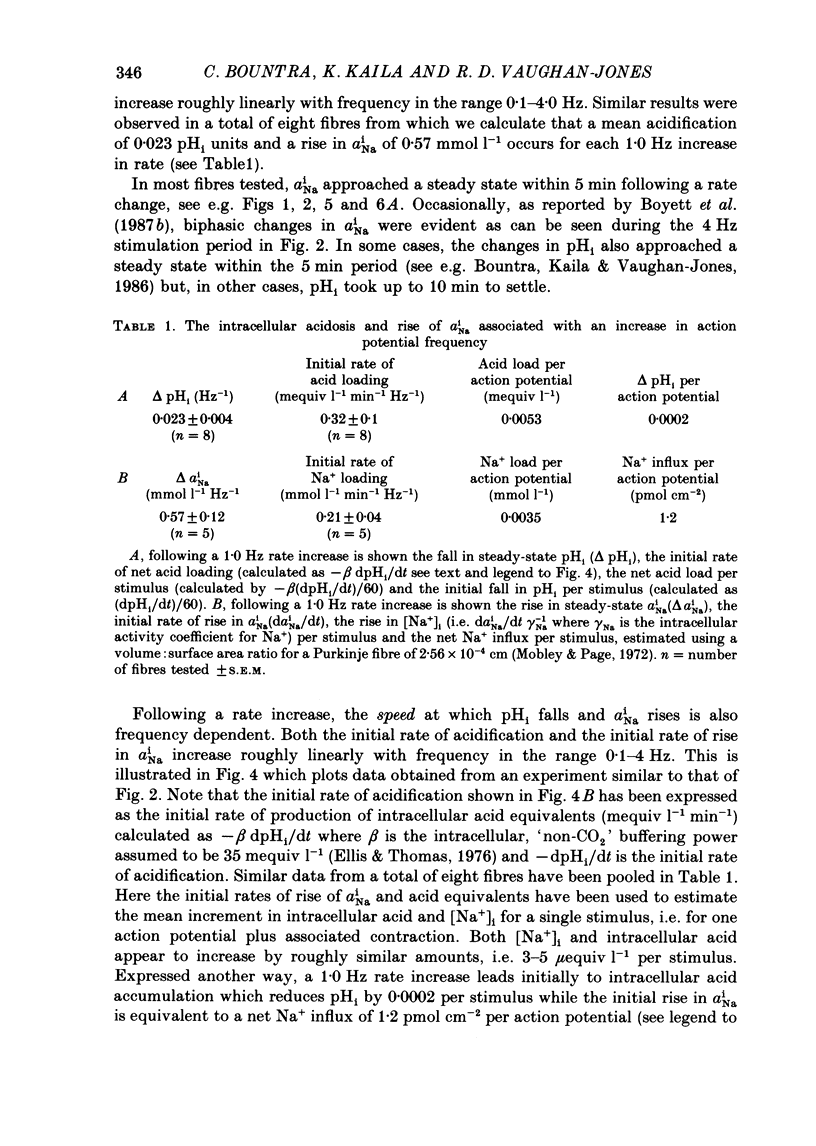
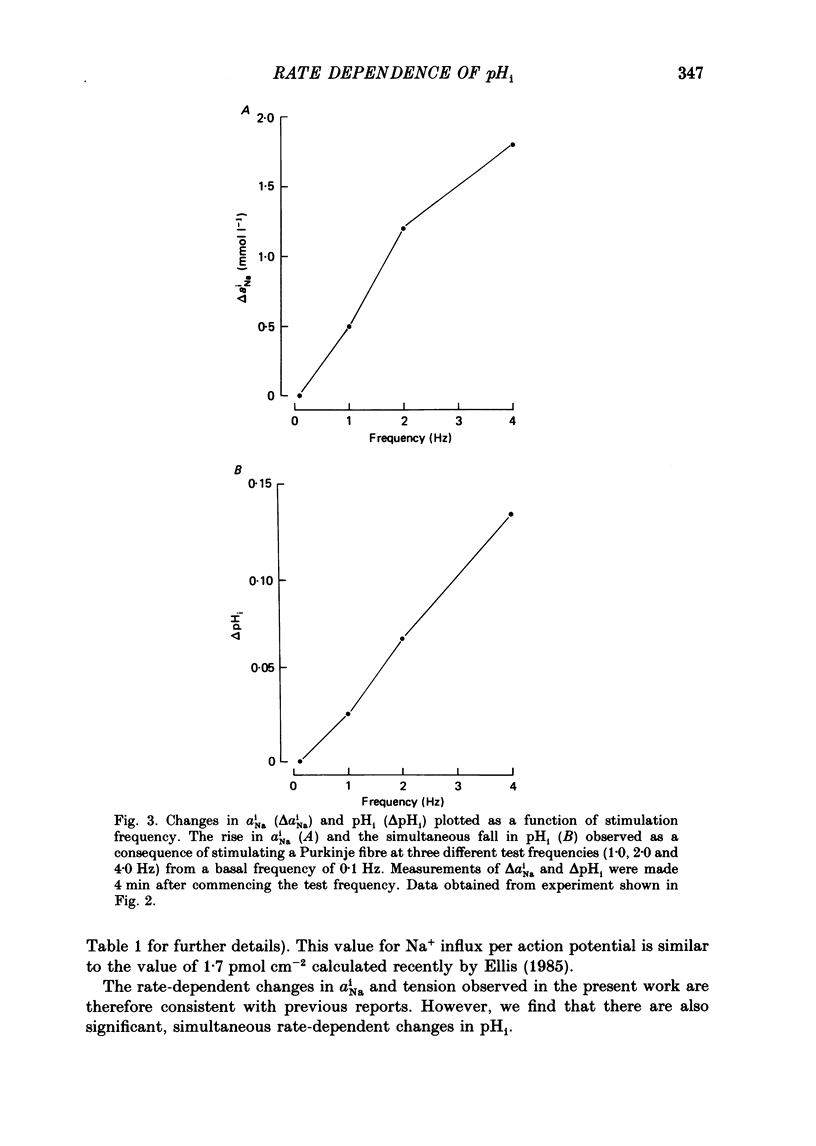
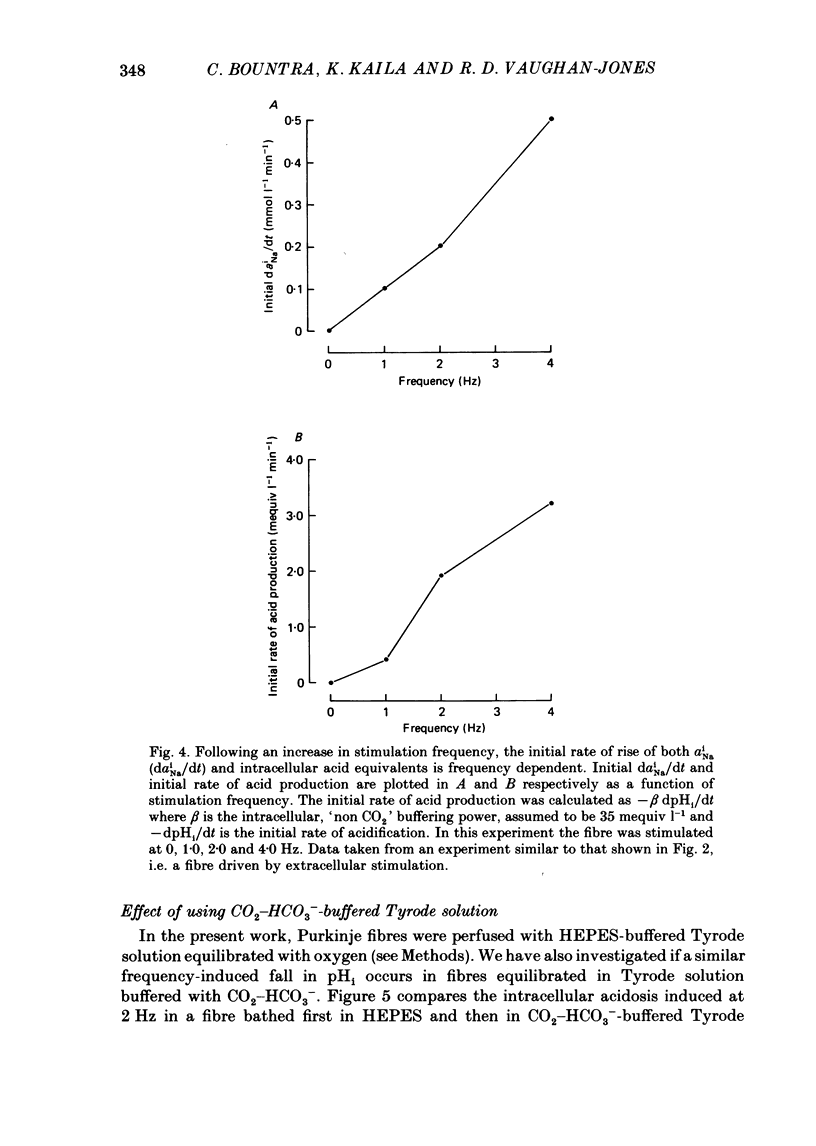
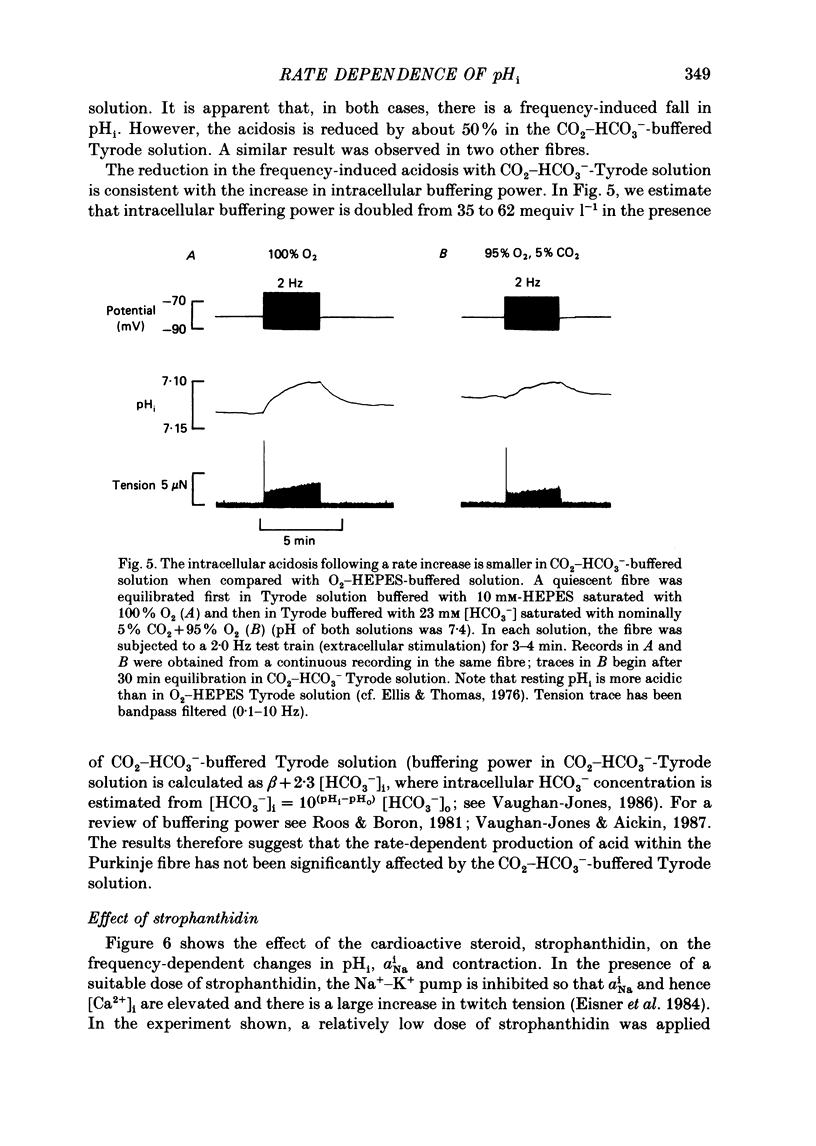
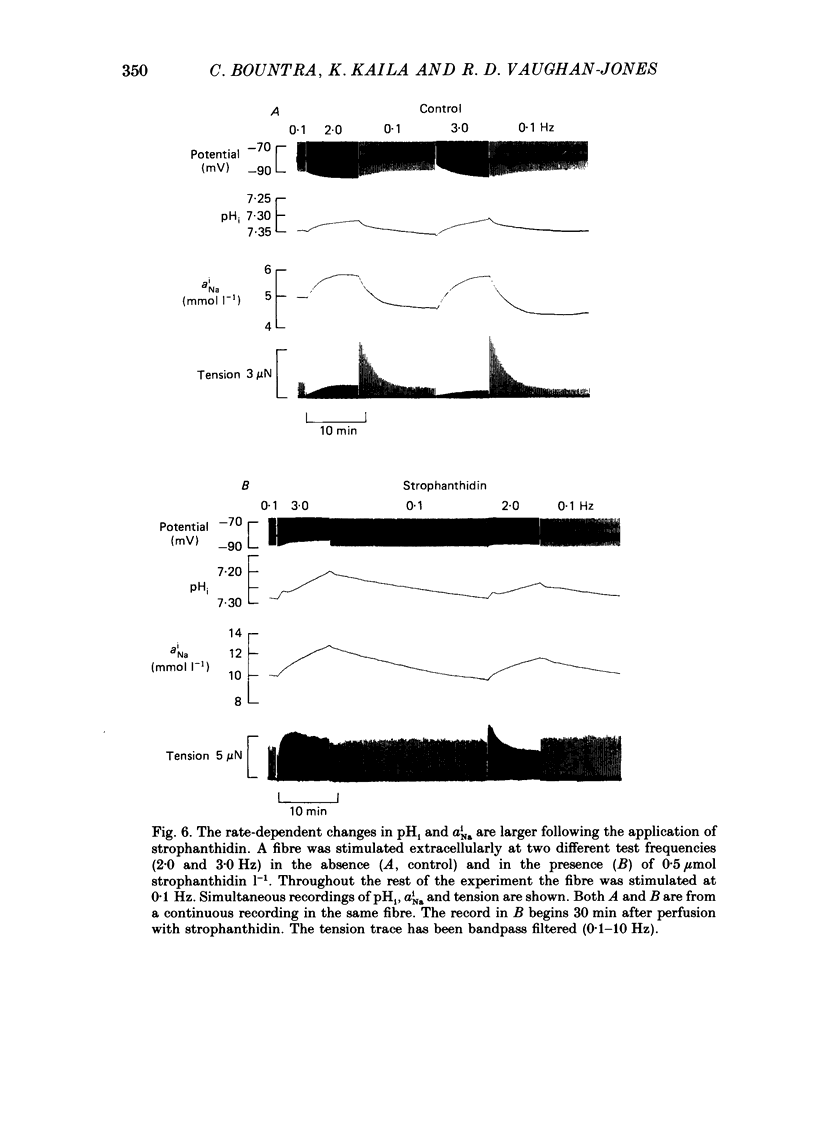
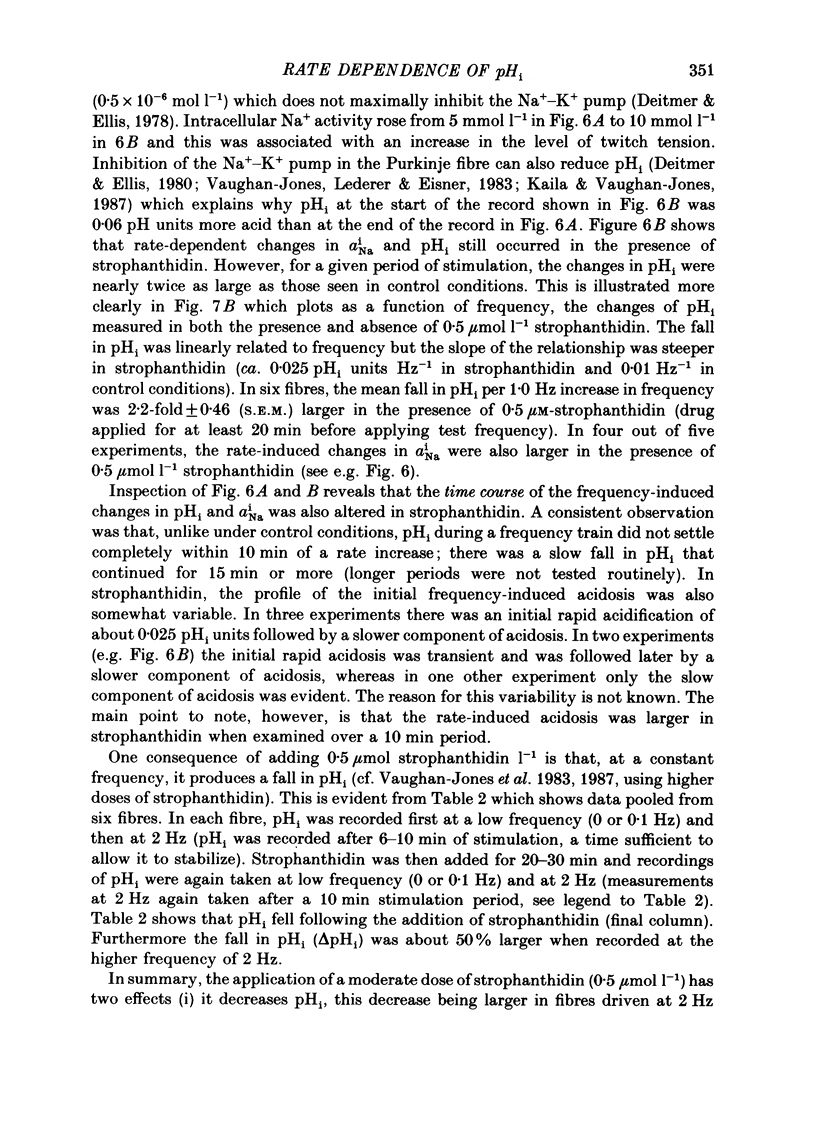
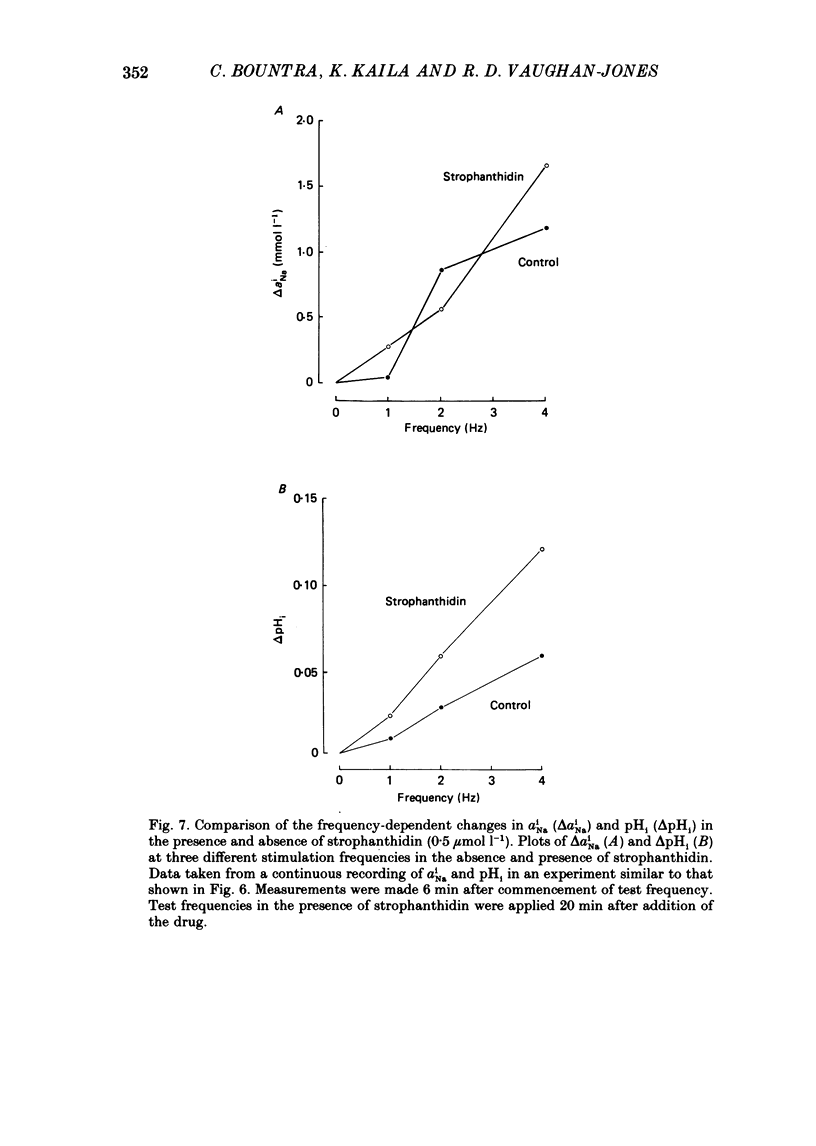
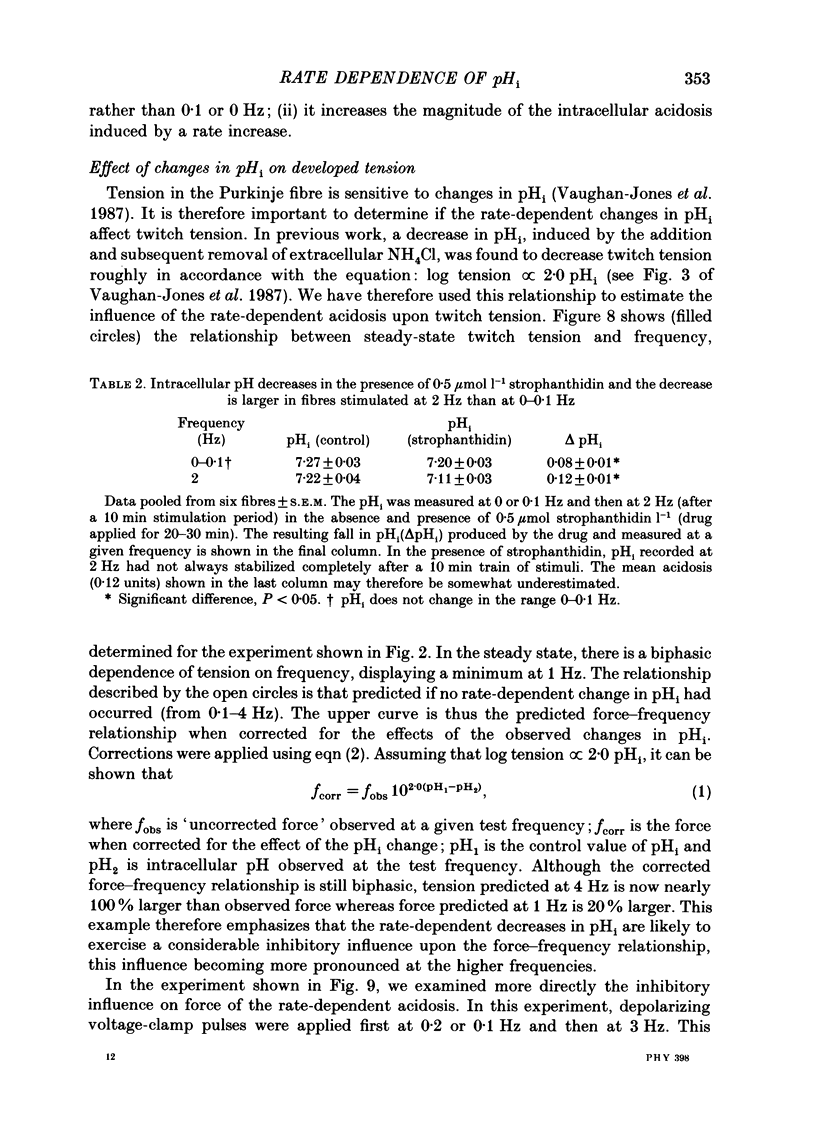
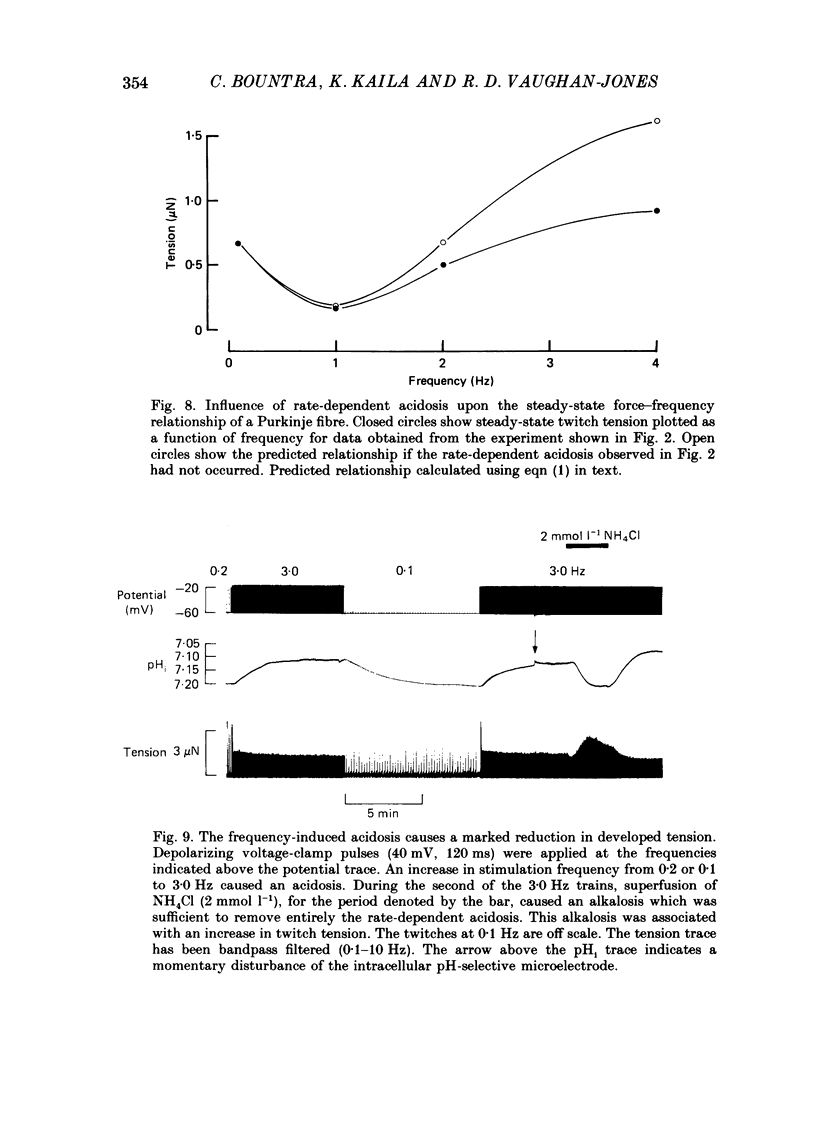
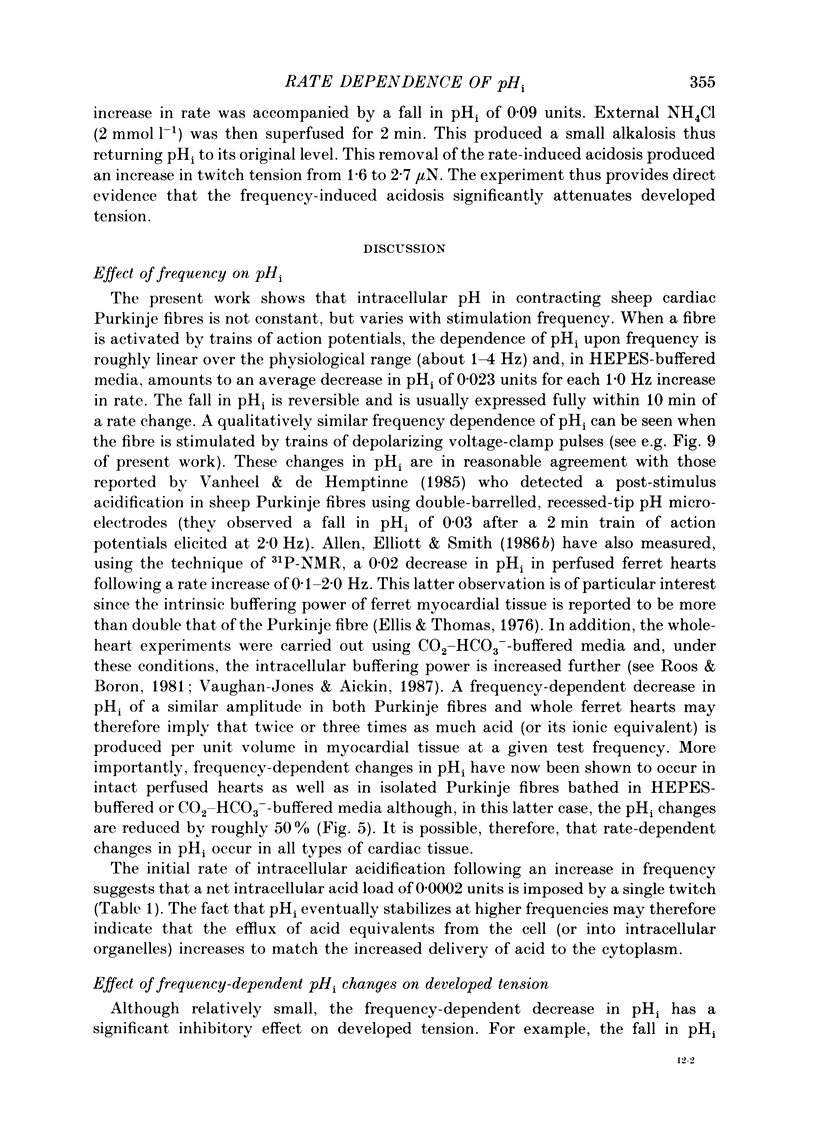
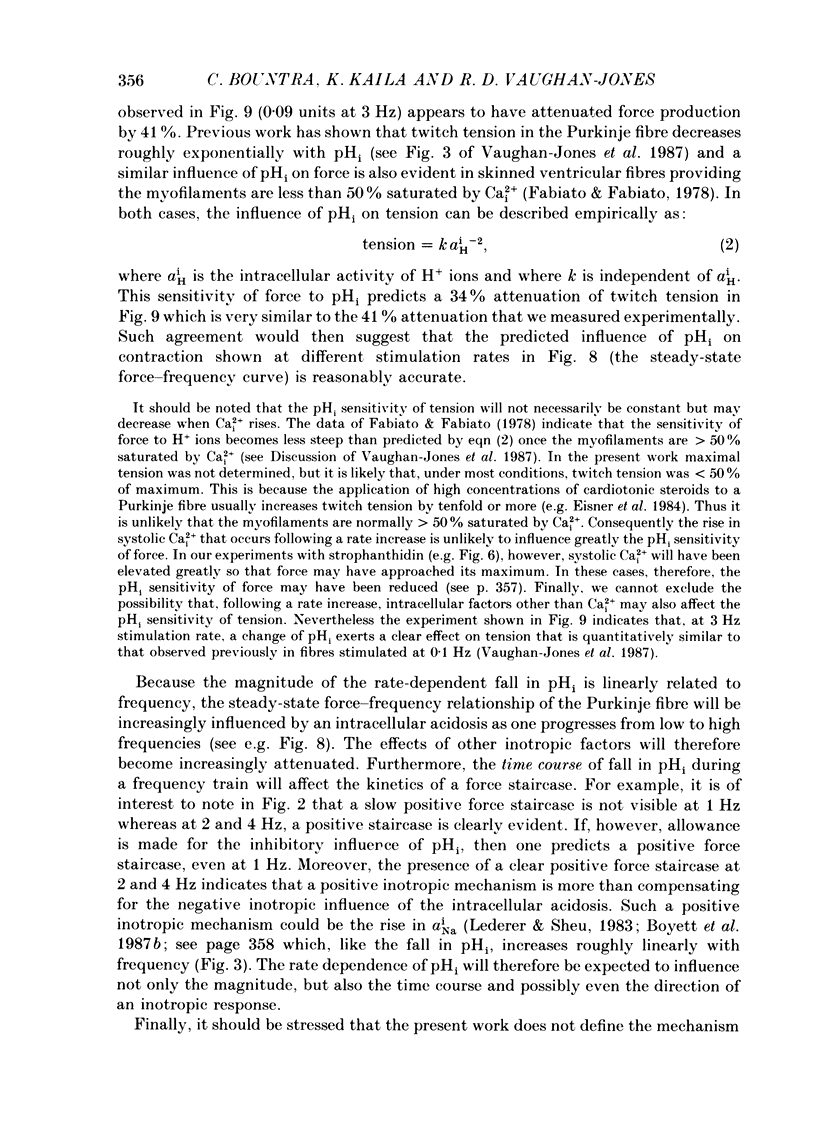
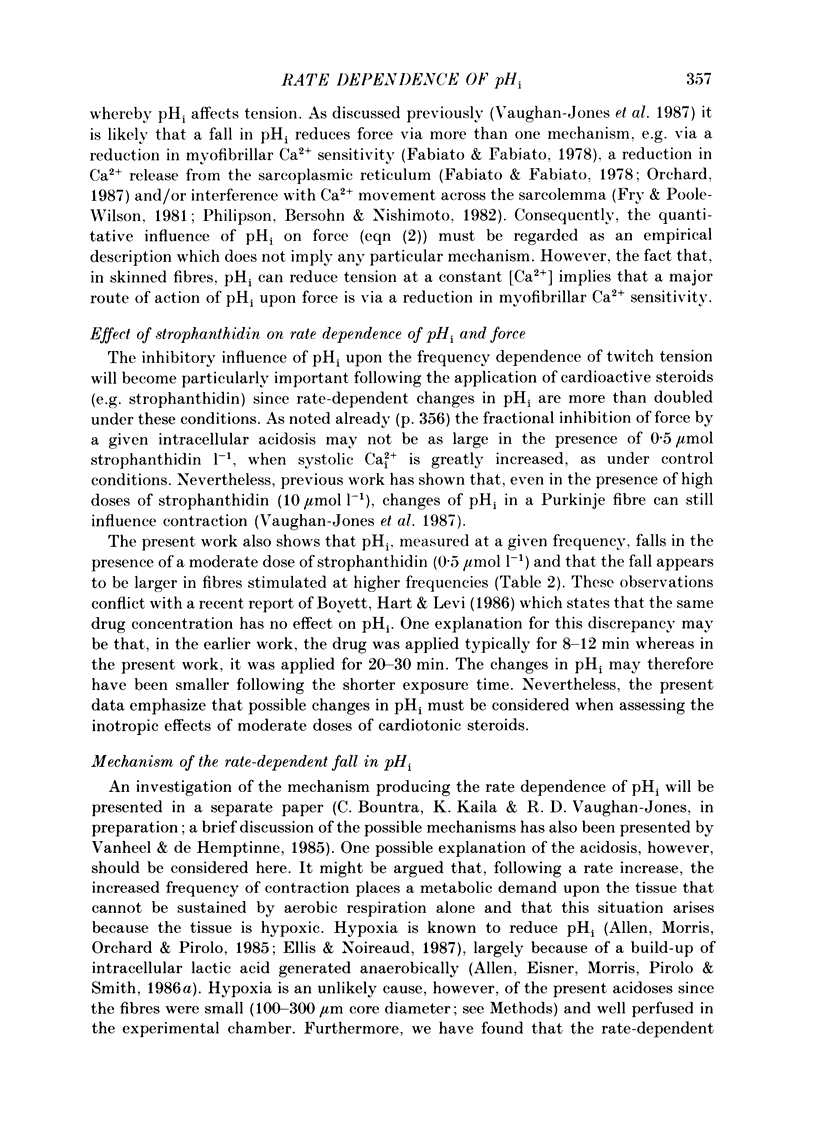
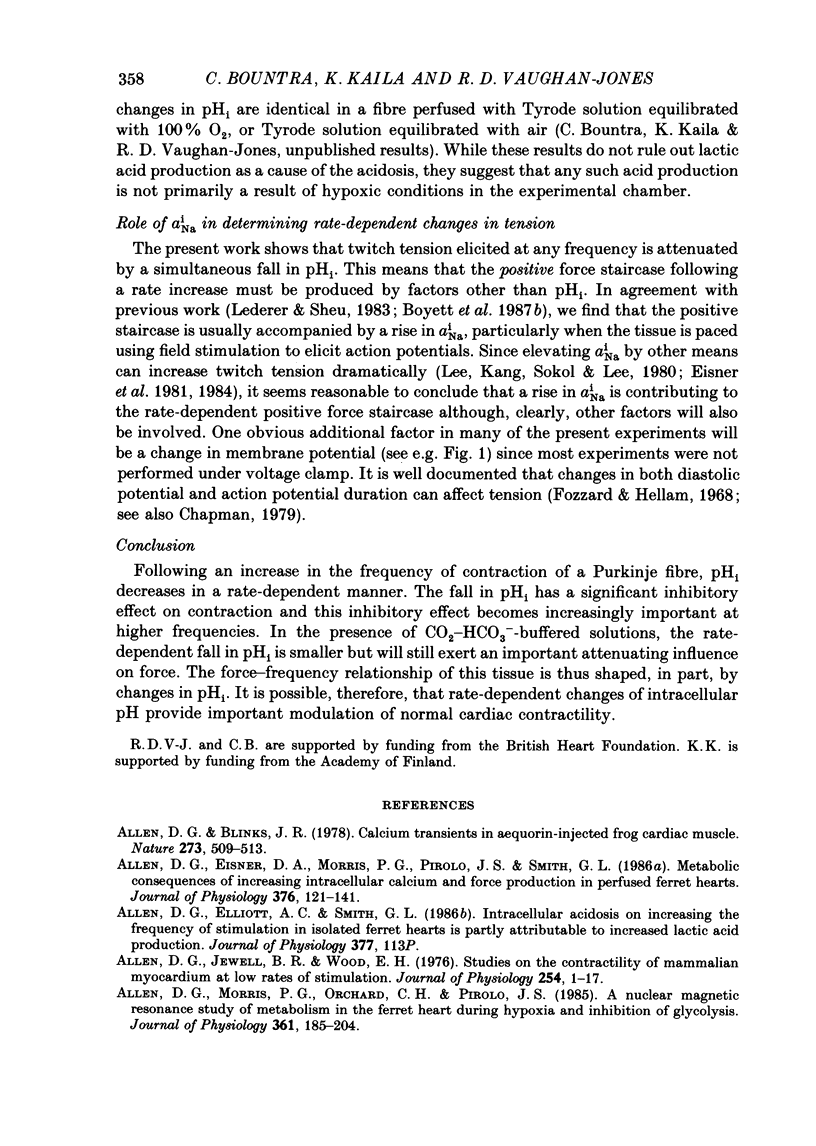
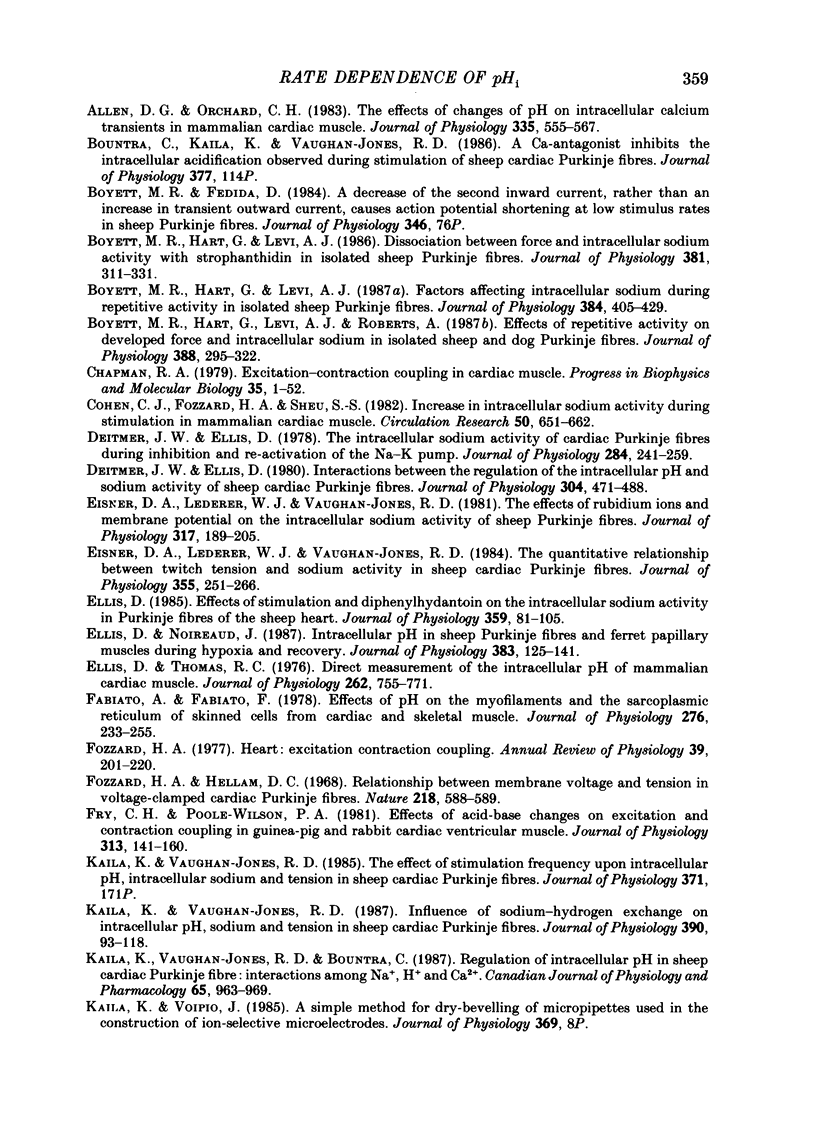
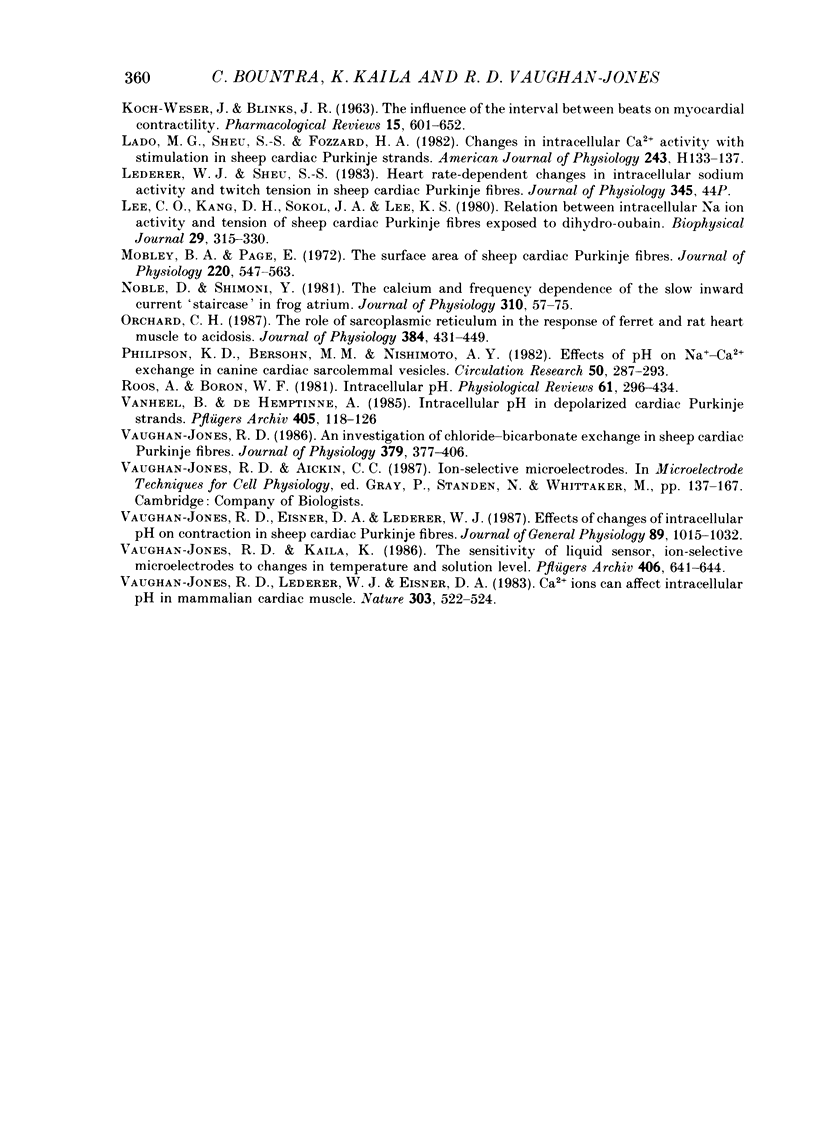
Images in this article
Selected References
These references are in PubMed. This may not be the complete list of references from this article.
- Allen D. G., Blinks J. R. Calcium transients in aequorin-injected frog cardiac muscle. Nature. 1978 Jun 15;273(5663):509–513. doi: 10.1038/273509a0. [DOI] [PubMed] [Google Scholar]
- Allen D. G., Eisner D. A., Morris P. G., Pirolo J. S., Smith G. L. Metabolic consequences of increasing intracellular calcium and force production in perfused ferret hearts. J Physiol. 1986 Jul;376:121–141. doi: 10.1113/jphysiol.1986.sp016145. [DOI] [PMC free article] [PubMed] [Google Scholar]
- Allen D. G., Jewell B. R., Wood E. H. Studies of the contractility of mammalian myocardium at low rates of stimulation. J Physiol. 1976 Jan;254(1):1–17. doi: 10.1113/jphysiol.1976.sp011217. [DOI] [PMC free article] [PubMed] [Google Scholar]
- Allen D. G., Morris P. G., Orchard C. H., Pirolo J. S. A nuclear magnetic resonance study of metabolism in the ferret heart during hypoxia and inhibition of glycolysis. J Physiol. 1985 Apr;361:185–204. doi: 10.1113/jphysiol.1985.sp015640. [DOI] [PMC free article] [PubMed] [Google Scholar]
- Allen D. G., Orchard C. H. The effects of changes of pH on intracellular calcium transients in mammalian cardiac muscle. J Physiol. 1983 Feb;335:555–567. doi: 10.1113/jphysiol.1983.sp014550. [DOI] [PMC free article] [PubMed] [Google Scholar]
- Boyett M. R., Hart G., Levi A. J. Dissociation between force and intracellular sodium activity with strophanthidin in isolated sheep Purkinje fibres. J Physiol. 1986 Dec;381:311–331. doi: 10.1113/jphysiol.1986.sp016329. [DOI] [PMC free article] [PubMed] [Google Scholar]
- Boyett M. R., Hart G., Levi A. J. Factors affecting intracellular sodium during repetitive activity in isolated sheep Purkinje fibres. J Physiol. 1987 Mar;384:405–429. doi: 10.1113/jphysiol.1987.sp016461. [DOI] [PMC free article] [PubMed] [Google Scholar]
- Boyett M. R., Hart G., Levi A. J., Roberts A. Effects of repetitive activity on developed force and intracellular sodium in isolated sheep and dog Purkinje fibres. J Physiol. 1987 Jul;388:295–322. doi: 10.1113/jphysiol.1987.sp016616. [DOI] [PMC free article] [PubMed] [Google Scholar]
- Chapman R. A. Excitation-contraction coupling in cardiac muscle. Prog Biophys Mol Biol. 1979;35(1):1–52. doi: 10.1016/0079-6107(80)90002-4. [DOI] [PubMed] [Google Scholar]
- Cohen C. J., Fozzard H. A., Sheu S. S. Increase in intracellular sodium ion activity during stimulation in mammalian cardiac muscle. Circ Res. 1982 May;50(5):651–662. doi: 10.1161/01.res.50.5.651. [DOI] [PubMed] [Google Scholar]
- Deitmer J. W., Ellis D. Interactions between the regulation of the intracellular pH and sodium activity of sheep cardiac Purkinje fibres. J Physiol. 1980 Jul;304:471–488. doi: 10.1113/jphysiol.1980.sp013337. [DOI] [PMC free article] [PubMed] [Google Scholar]
- Deitmer J. W., Ellis D. The intracellular sodium activity of cardiac Purkinje fibres during inhibition and re-activation of the Na-K pump. J Physiol. 1978 Nov;284:241–259. doi: 10.1113/jphysiol.1978.sp012539. [DOI] [PMC free article] [PubMed] [Google Scholar]
- Eisner D. A., Lederer W. J., Vaughan-Jones R. D. The effects of rubidium ions and membrane potentials on the intracellular sodium activity of sheep Purkinje fibres. J Physiol. 1981 Aug;317:189–205. doi: 10.1113/jphysiol.1981.sp013820. [DOI] [PMC free article] [PubMed] [Google Scholar]
- Eisner D. A., Lederer W. J., Vaughan-Jones R. D. The quantitative relationship between twitch tension and intracellular sodium activity in sheep cardiac Purkinje fibres. J Physiol. 1984 Oct;355:251–266. doi: 10.1113/jphysiol.1984.sp015417. [DOI] [PMC free article] [PubMed] [Google Scholar]
- Ellis D., MacLeod K. T. Sodium-dependent control of intracellular pH in Purkinje fibres of sheep heart. J Physiol. 1985 Feb;359:81–105. doi: 10.1113/jphysiol.1985.sp015576. [DOI] [PMC free article] [PubMed] [Google Scholar]
- Ellis D., Noireaud J. Intracellular pH in sheep Purkinje fibres and ferret papillary muscles during hypoxia and recovery. J Physiol. 1987 Feb;383:125–141. doi: 10.1113/jphysiol.1987.sp016400. [DOI] [PMC free article] [PubMed] [Google Scholar]
- Ellis D., Thomas R. C. Direct measurement of the intracellular pH of mammalian cardiac muscle. J Physiol. 1976 Nov;262(3):755–771. doi: 10.1113/jphysiol.1976.sp011619. [DOI] [PMC free article] [PubMed] [Google Scholar]
- Fabiato A., Fabiato F. Effects of pH on the myofilaments and the sarcoplasmic reticulum of skinned cells from cardiace and skeletal muscles. J Physiol. 1978 Mar;276:233–255. doi: 10.1113/jphysiol.1978.sp012231. [DOI] [PMC free article] [PubMed] [Google Scholar]
- Fozzard H. A. Heart: excitation-contraction coupling. Annu Rev Physiol. 1977;39:201–220. doi: 10.1146/annurev.ph.39.030177.001221. [DOI] [PubMed] [Google Scholar]
- Fozzard H. A., Hellman D. C. Relationship between membrane voltage and tension in voltage-clamped cardiac purkinje fibres. Nature. 1968 May 11;218(5141):588–589. doi: 10.1038/218588a0. [DOI] [PubMed] [Google Scholar]
- Fry C. H., Poole-Wilson P. A. Effects of acid-base changes on excitation--contraction coupling in guinea-pig and rabbit cardiac ventricular muscle. J Physiol. 1981;313:141–160. doi: 10.1113/jphysiol.1981.sp013655. [DOI] [PMC free article] [PubMed] [Google Scholar]
- KOCH-WESER J., BLINKS J. R. THE INFLUENCE OF THE INTERVAL BETWEEN BEATS ON MYOCARDIAL CONTRACTILITY. Pharmacol Rev. 1963 Sep;15:601–652. [PubMed] [Google Scholar]
- Kaila K., Vaughan-Jones R. D., Bountra C. Regulation of intracellular pH in sheep cardiac Purkinje fibre: interactions among Na+, H+, and Ca2+1. Can J Physiol Pharmacol. 1987 May;65(5):963–969. doi: 10.1139/y87-153. [DOI] [PubMed] [Google Scholar]
- Kaila K., Vaughan-Jones R. D. Influence of sodium-hydrogen exchange on intracellular pH, sodium and tension in sheep cardiac Purkinje fibres. J Physiol. 1987 Sep;390:93–118. doi: 10.1113/jphysiol.1987.sp016688. [DOI] [PMC free article] [PubMed] [Google Scholar]
- Lado M. G., Sheu S. S., Fozzard H. A. Changes in intracellular Ca2+ activity with stimulation in sheep cardiac Purkinje strands. Am J Physiol. 1982 Jul;243(1):H133–H137. doi: 10.1152/ajpheart.1982.243.1.H133. [DOI] [PubMed] [Google Scholar]
- Lee C. O., Kang D. H., Sokol J. H., Lee K. S. Relation between intracellular Na ion activity and tension of sheep cardiac Purkinje fibers exposed to dihydro-ouabain. Biophys J. 1980 Feb;29(2):315–330. doi: 10.1016/S0006-3495(80)85135-6. [DOI] [PMC free article] [PubMed] [Google Scholar]
- Mobley B. A., Page E. The surface area of sheep cardiac Purkinje fibres. J Physiol. 1972 Feb;220(3):547–563. doi: 10.1113/jphysiol.1972.sp009722. [DOI] [PMC free article] [PubMed] [Google Scholar]
- Noble S., Shimoni Y. The calcium and frequency dependence of the slow inward current 'staircase' in frog atrium. J Physiol. 1981 Jan;310:57–75. doi: 10.1113/jphysiol.1981.sp013537. [DOI] [PMC free article] [PubMed] [Google Scholar]
- Orchard C. H. The role of the sarcoplasmic reticulum in the response of ferret and rat heart muscle to acidosis. J Physiol. 1987 Mar;384:431–449. doi: 10.1113/jphysiol.1987.sp016462. [DOI] [PMC free article] [PubMed] [Google Scholar]
- Philipson K. D., Bersohn M. M., Nishimoto A. Y. Effects of pH on Na+-Ca2+ exchange in canine cardiac sarcolemmal vesicles. Circ Res. 1982 Feb;50(2):287–293. doi: 10.1161/01.res.50.2.287. [DOI] [PubMed] [Google Scholar]
- Roos A., Boron W. F. Intracellular pH. Physiol Rev. 1981 Apr;61(2):296–434. doi: 10.1152/physrev.1981.61.2.296. [DOI] [PubMed] [Google Scholar]
- Vanheel B., de Hemptinne A. Intracellular pH in depolarized cardiac Purkinje strands. Pflugers Arch. 1985 Sep;405(2):118–126. doi: 10.1007/BF00584532. [DOI] [PubMed] [Google Scholar]
- Vaughan-Jones R. D. An investigation of chloride-bicarbonate exchange in the sheep cardiac Purkinje fibre. J Physiol. 1986 Oct;379:377–406. doi: 10.1113/jphysiol.1986.sp016259. [DOI] [PMC free article] [PubMed] [Google Scholar]
- Vaughan-Jones R. D., Eisner D. A., Lederer W. J. Effects of changes of intracellular pH on contraction in sheep cardiac Purkinje fibers. J Gen Physiol. 1987 Jun;89(6):1015–1032. doi: 10.1085/jgp.89.6.1015. [DOI] [PMC free article] [PubMed] [Google Scholar]
- Vaughan-Jones R. D., Kaila K. The sensitivity of liquid sensor, ion-selective microelectrodes to changes in temperature and solution level. Pflugers Arch. 1986 Jun;406(6):641–644. doi: 10.1007/BF00584033. [DOI] [PubMed] [Google Scholar]
- Vaughan-Jones R. D., Lederer W. J., Eisner D. A. Ca2+ ions can affect intracellular pH in mammalian cardiac muscle. Nature. 1983 Feb 10;301(5900):522–524. doi: 10.1038/301522a0. [DOI] [PubMed] [Google Scholar]