Abstract
1. Pyramidal neurones in the precentral motor area of the monkey were studied using intracellular techniques. Pyramidal tract neurones (PTNs) were identified by antidromic activation from the cerebral peduncles or medullary pyramids. Orthodromic responses were recorded in PTNs and in other pyramidal neurones when antidromic volleys were set up by stimulation of the peduncles or pyramids. The neurones were then labelled by intracellular ionophoresis of horseradish peroxidase and their morphology examined. All neurones studied were identified as pyramidal cells according to their morphology. 2. Six pyramidal neurones located in lamina V were well stained; they included two fast PTNs and two slow PTNs. The morphology of all pyramidal neurones in this lamina (fast PTNs, slow PTNs and those pyramidal cells that were not antidromically characterized) was essentially similar. A single apical dendrite branched as it ascended and its terminals arborized subpially. Numerous lateral and oblique dendrites branched from the apical dendrites in lamina V and near its border with lamina III: short basal dendrites arborized in the vicinity of the soma in lamina V. Long basal dendrites had a wider field of arborization in lamina V and sometimes extended into lamina VI. 3. Three to five collaterals arose from the axon of lamina V cells in the cortex and arborized in laminae V and VI. Short collateral branches arborized in the vicinity of the soma in the region of the basal and lateral dendrites. Long collateral branches could be traced over long distances (often more than 1 mm). One pyramidal neurone in this lamina (a fast PTN) lacked short collateral branches from the axon. 4. Four pyramidal neurones in lamina III were stained well. The dendritic morphology of all these neurones was similar. Apical dendrites branched as they ascended and terminated subpially. Lateral and basal dendrites formed a column of dendritic branches around the soma. No long basal dendrites were seen. 5. The number and arborization of intracortical collaterals from the axon of lamina III cells varied widely; from three to twelve collaterals arose from the axon. The biggest arbor of collateral branches involved all the cortical laminae and was about 3 mm wide mediolaterally, while the smallest arbor was restricted mainly to lamina III in the vicinity of the soma. One neurone in this lamina also lacked short collateral branches from the axon. 6. Antidromic volleys from the pyramidal tract evoked excitatory responses in fast PTNs, predominantly inhibitory responses in slow PTNs and either excitatory or inhibitory responses in other pyramidal neurones in lamina V.(ABSTRACT TRUNCATED AT 400 WORDS)
Full text
PDF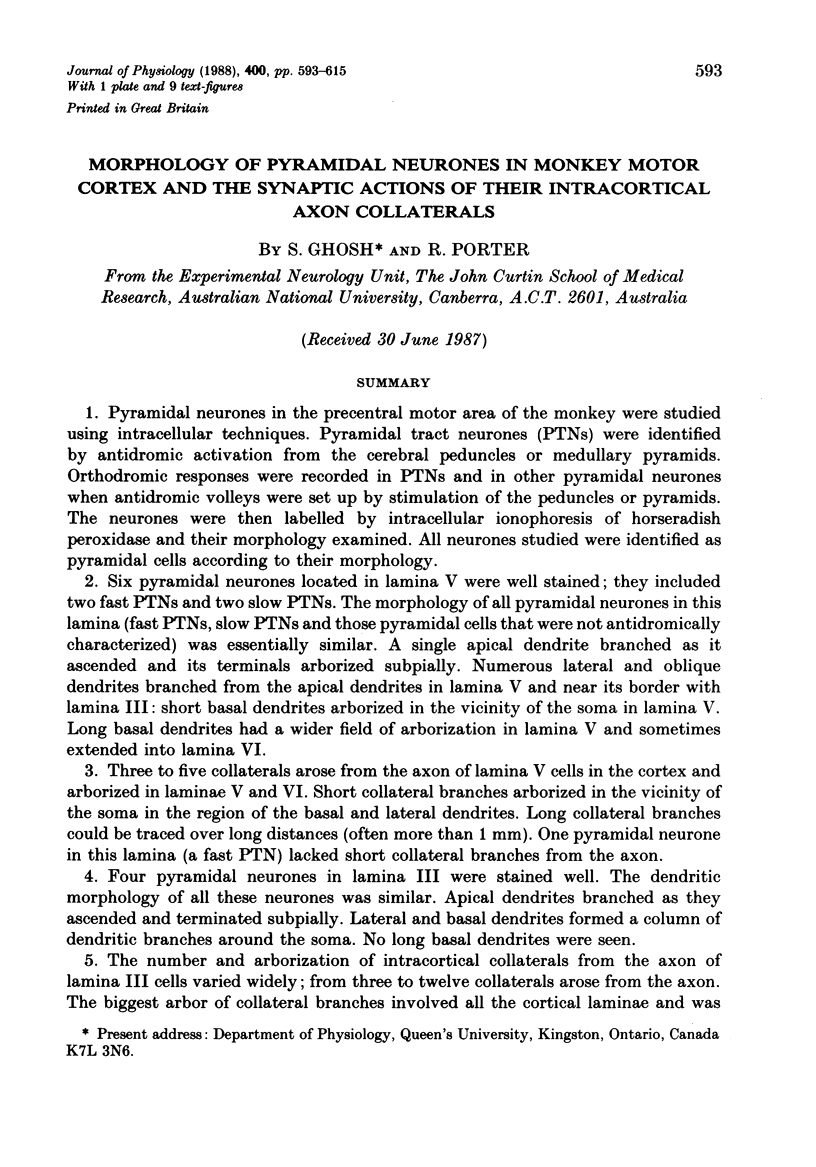
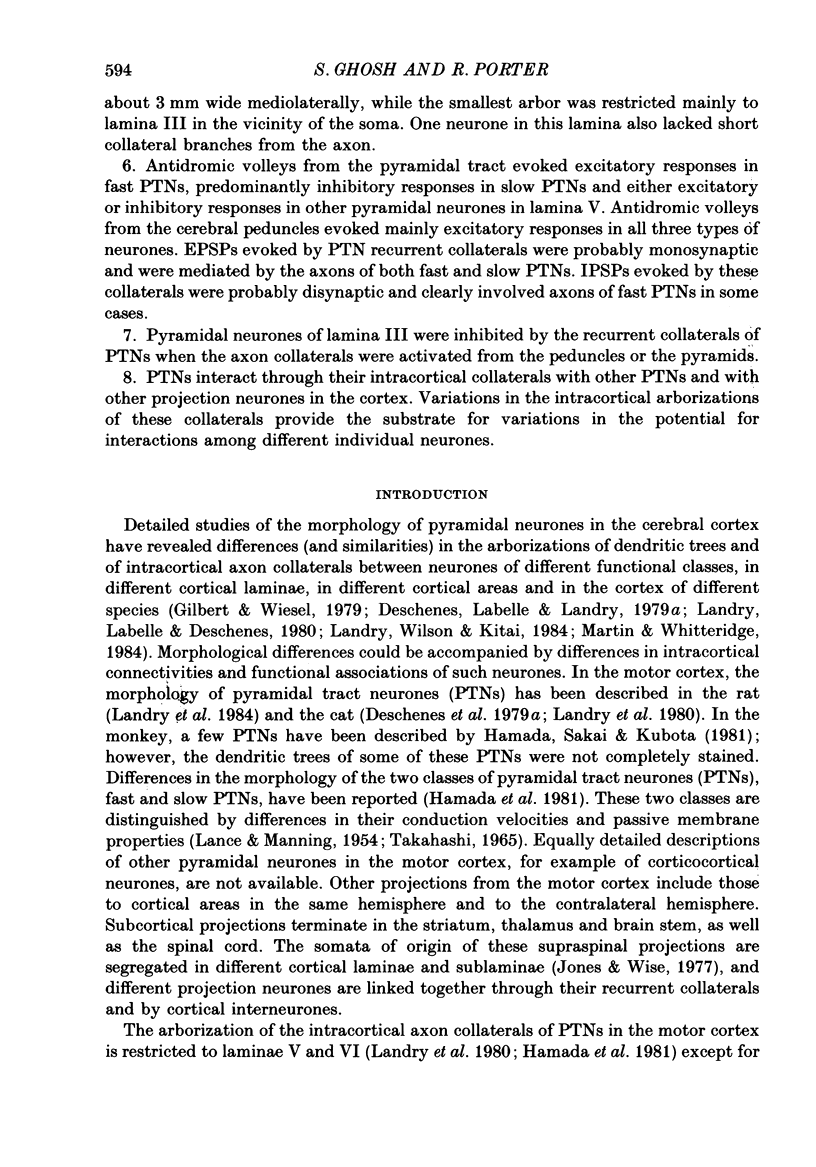
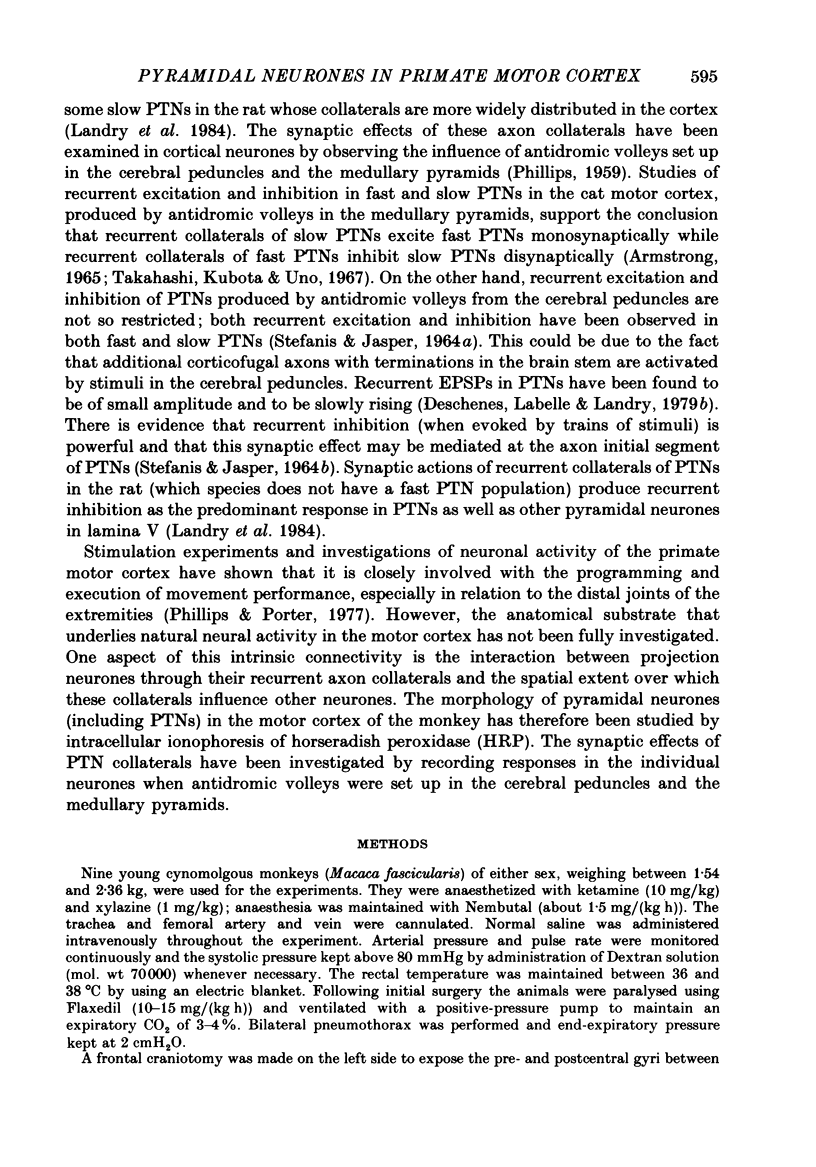
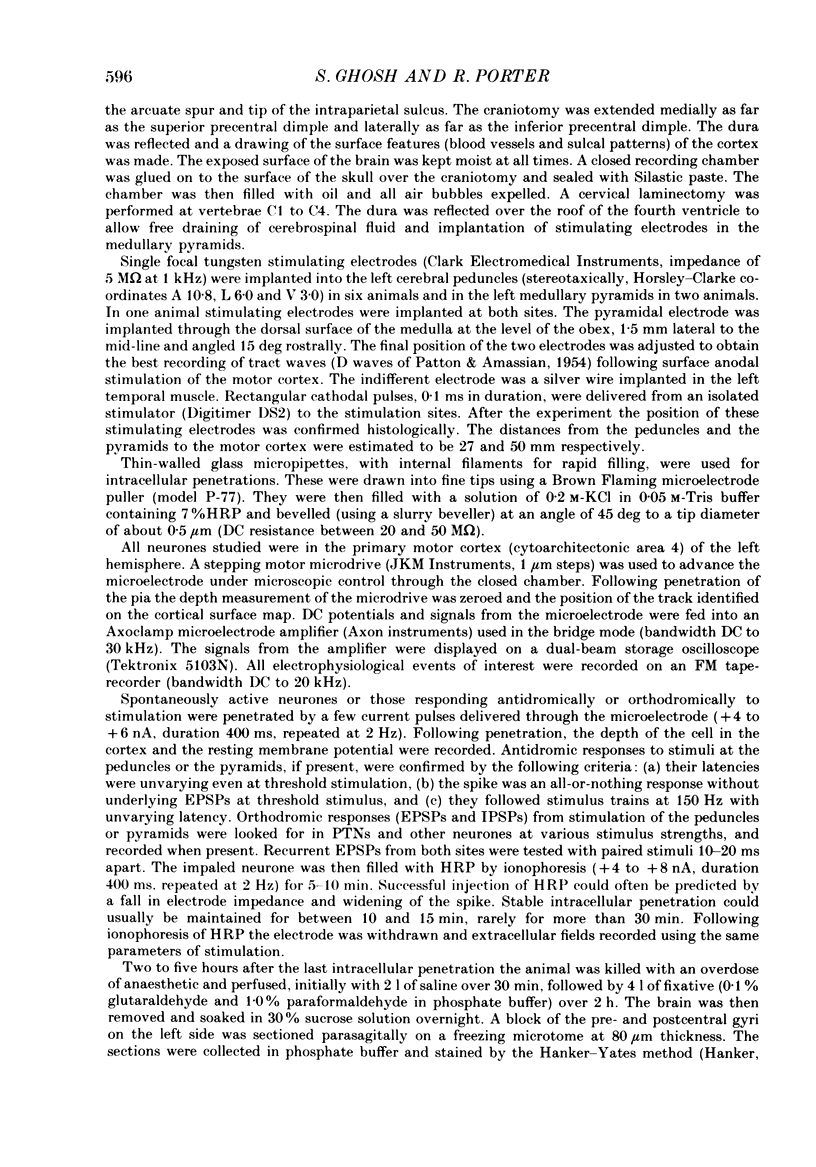
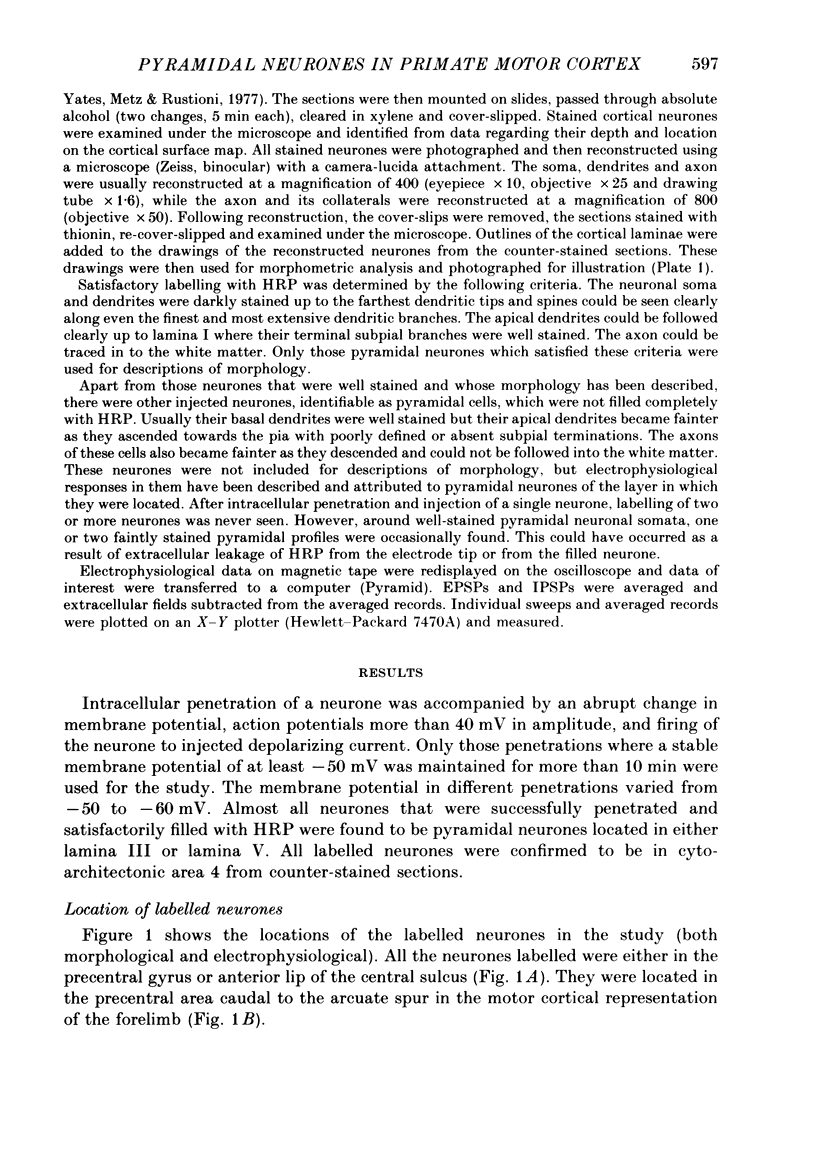
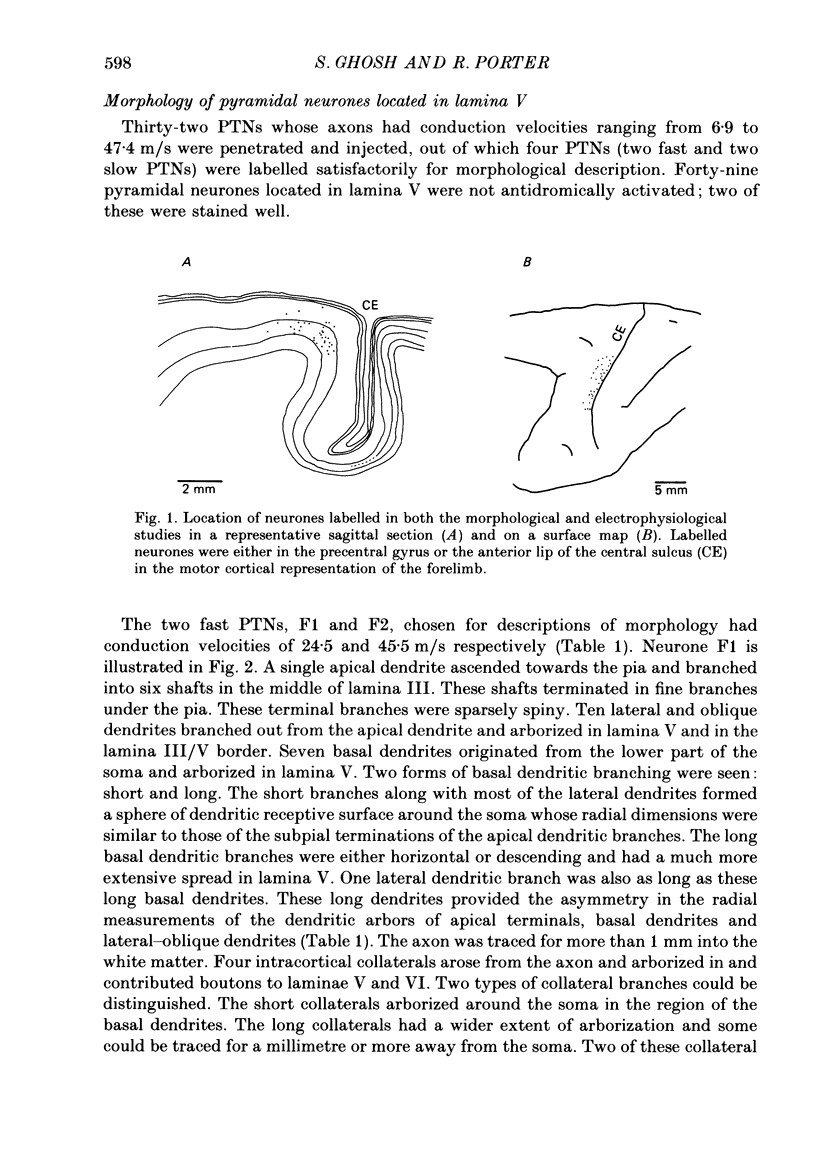
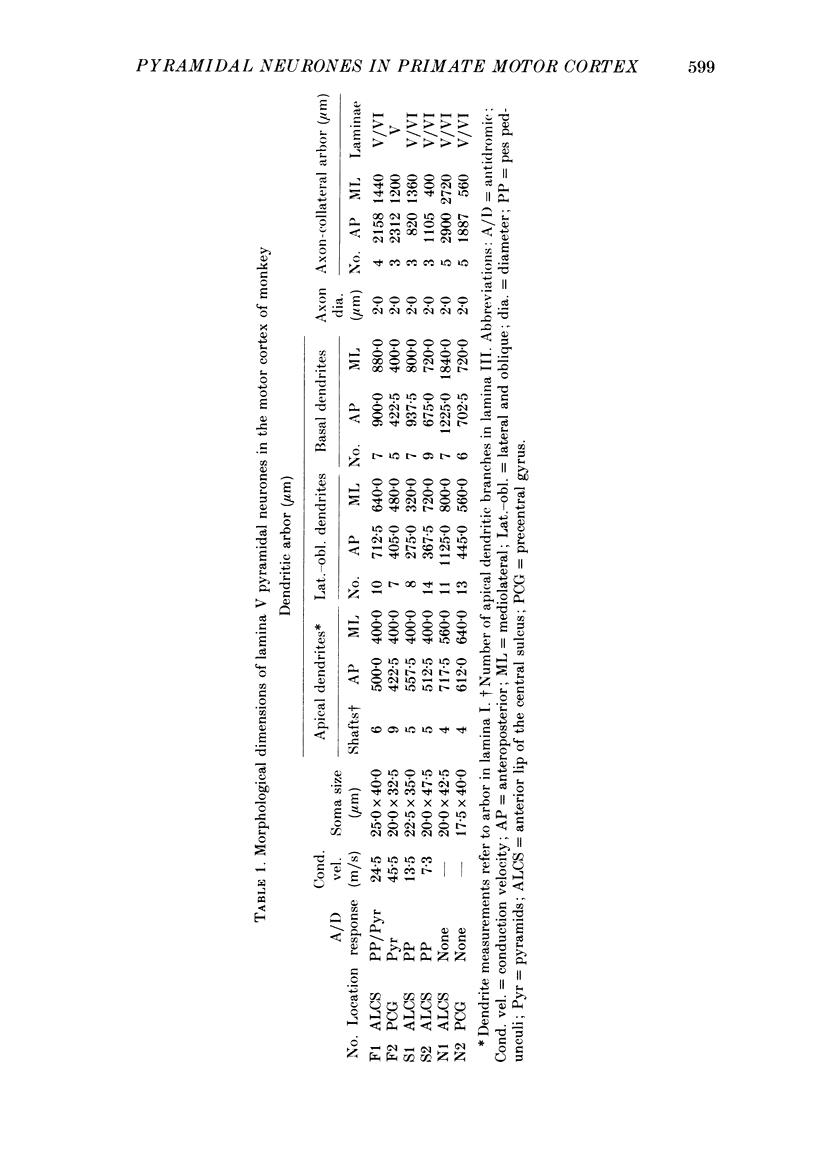
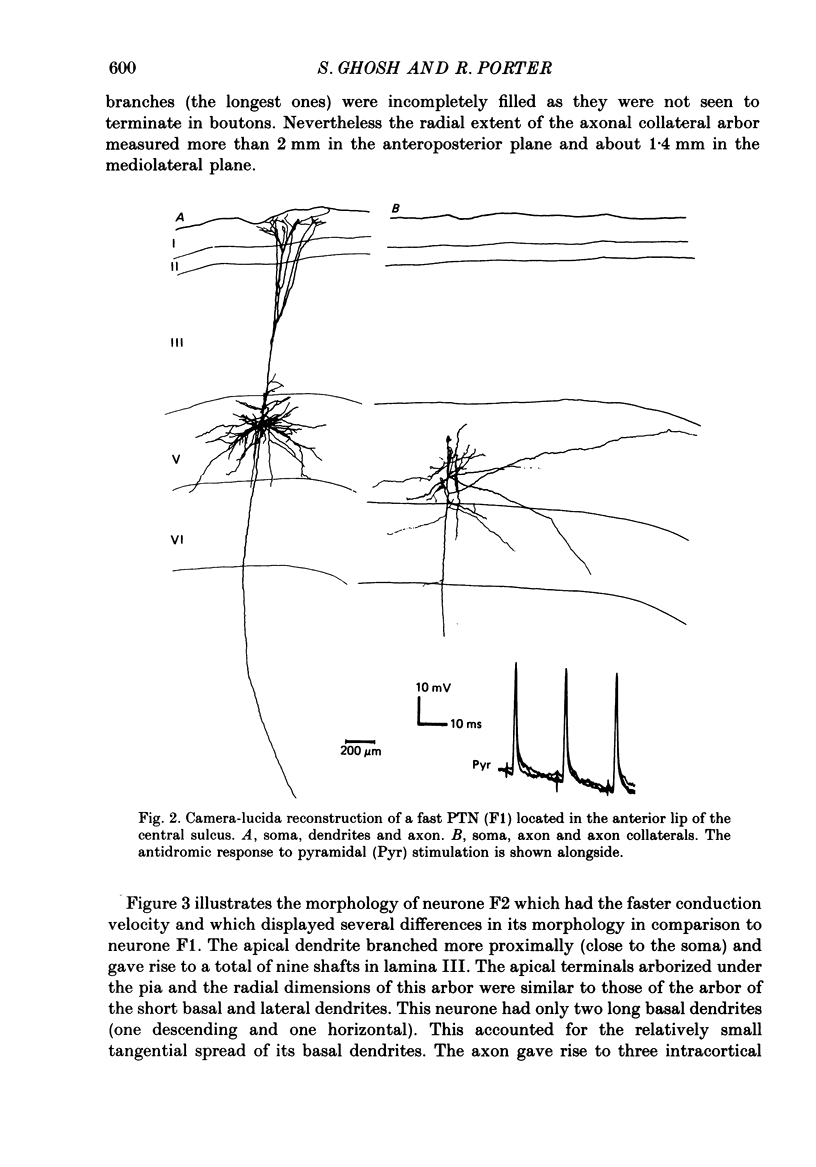
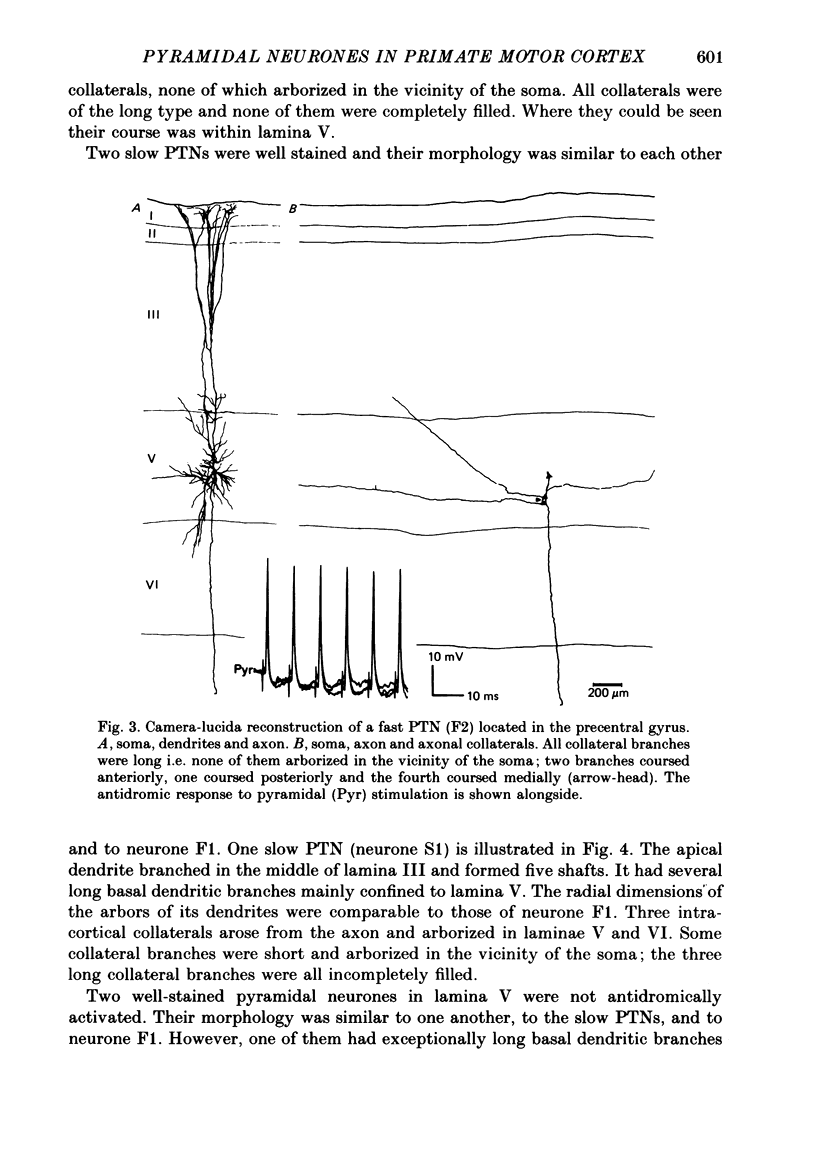
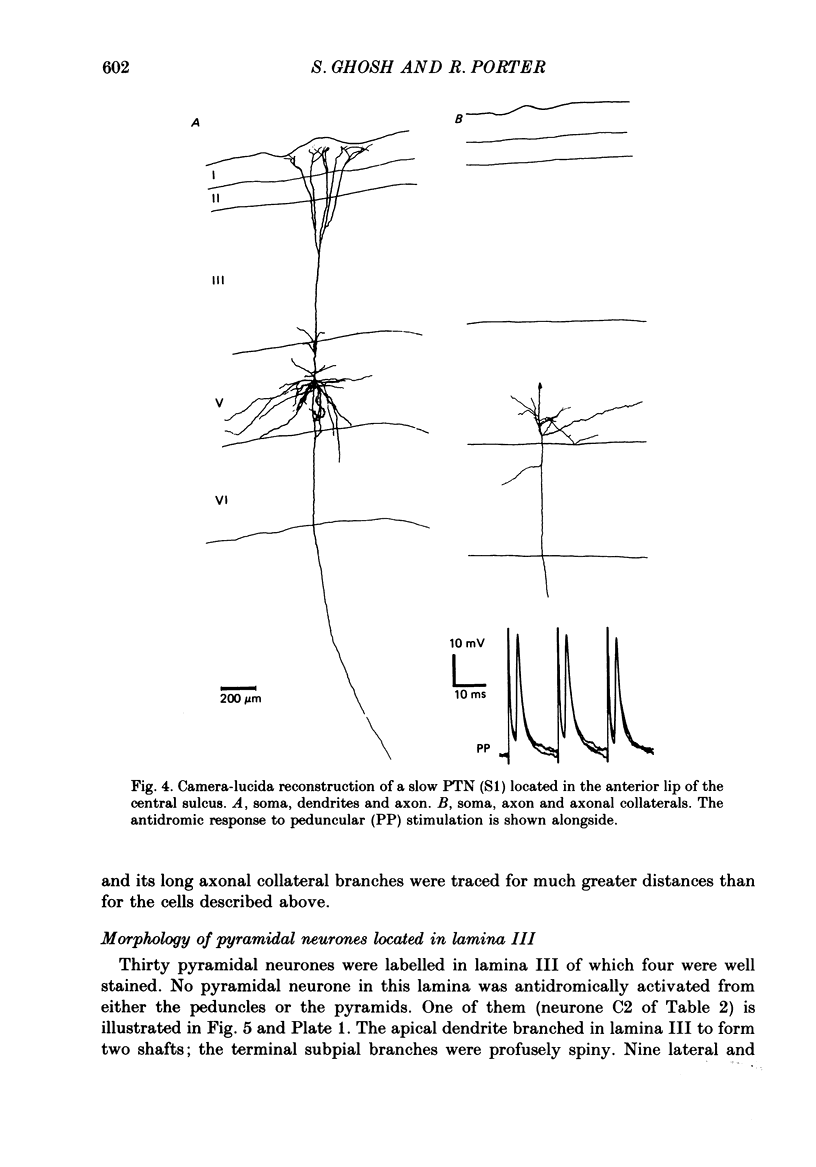
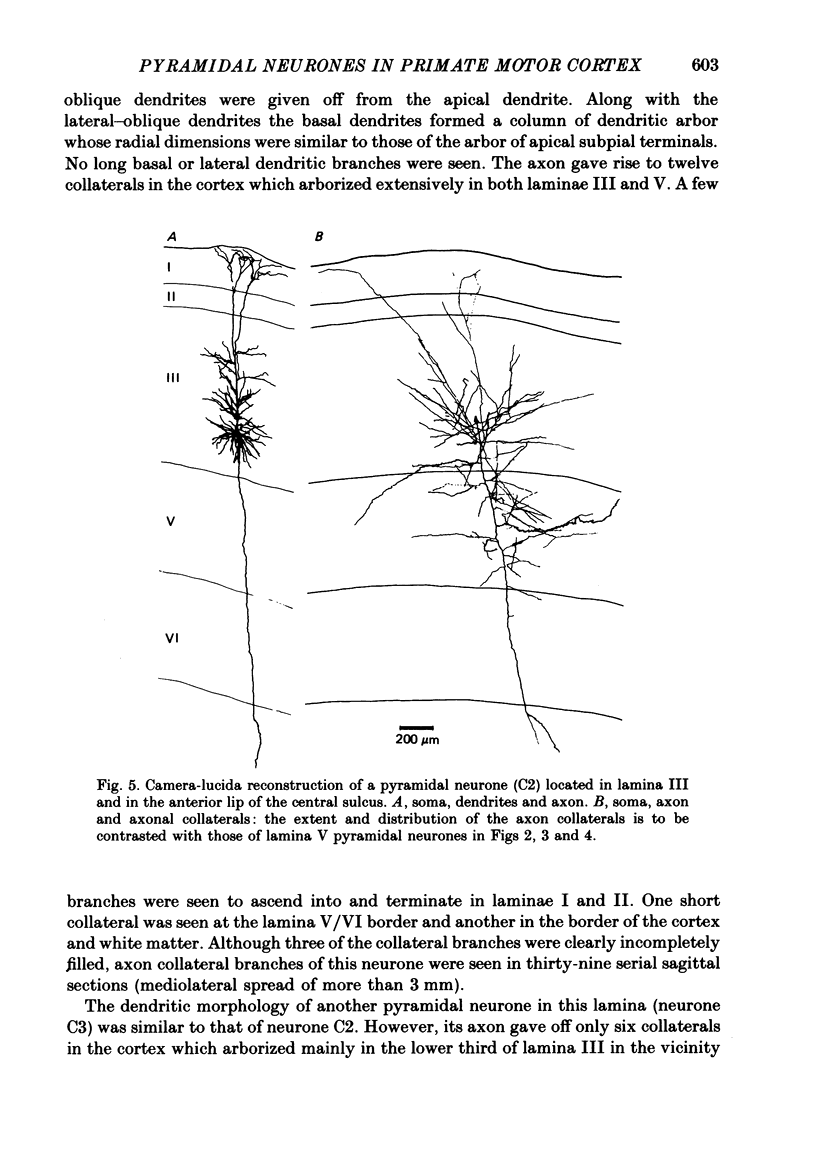
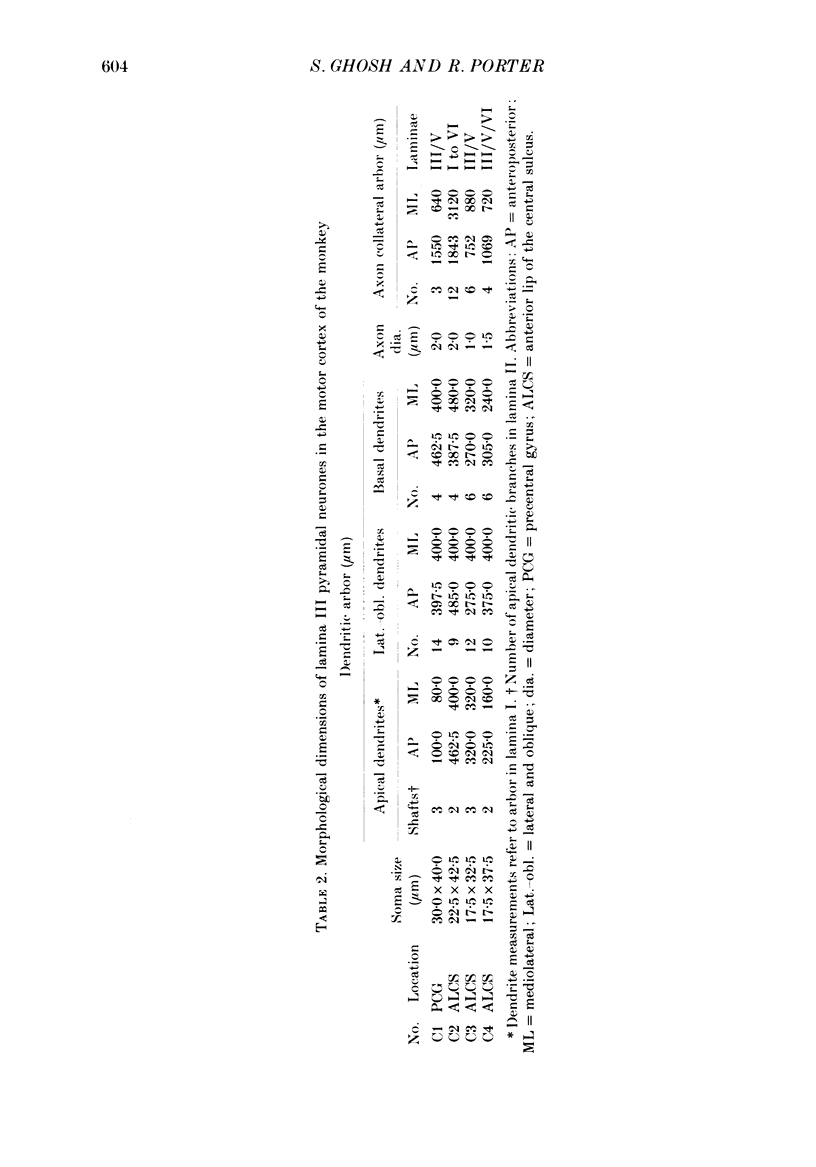
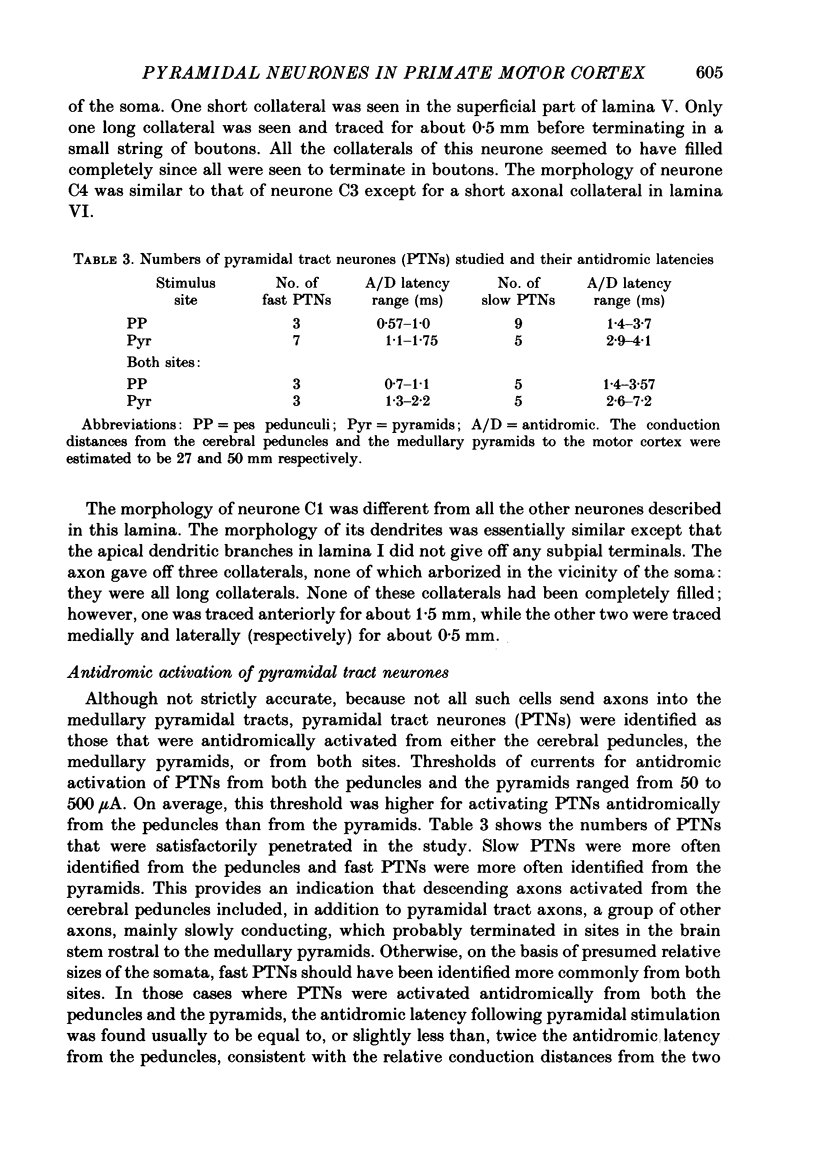
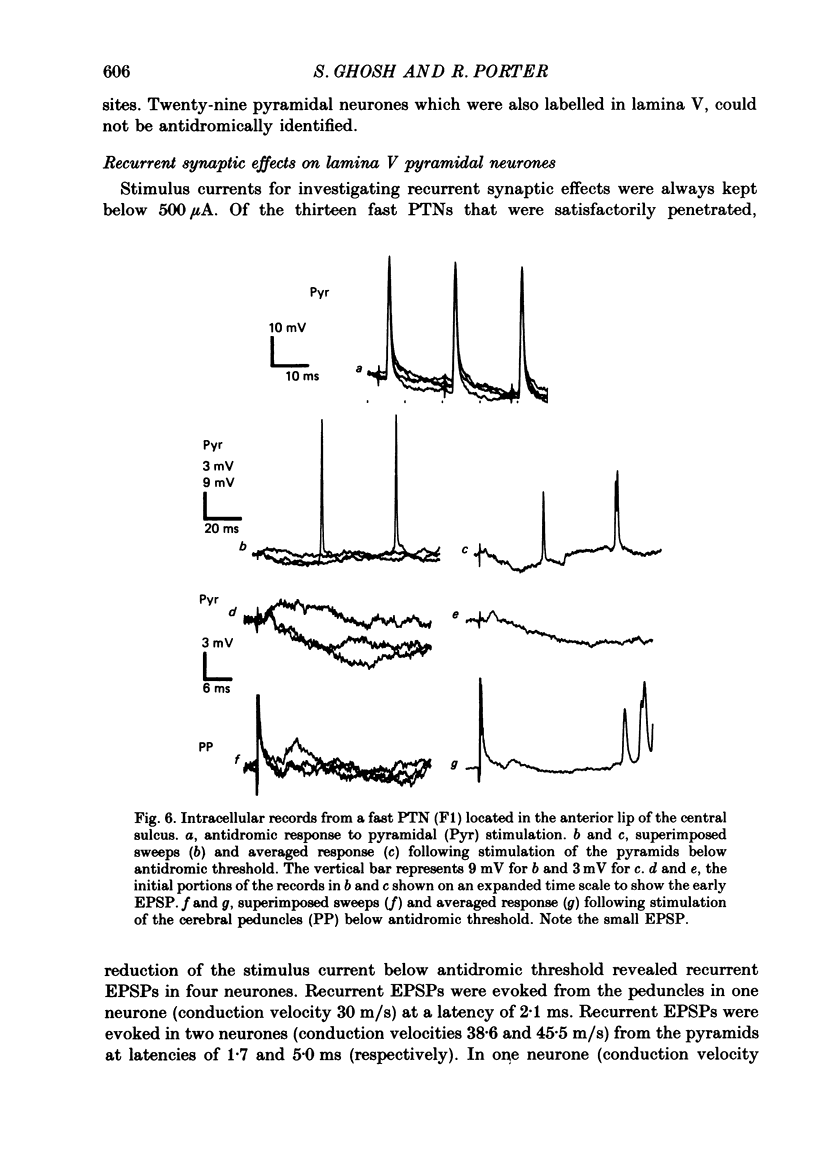
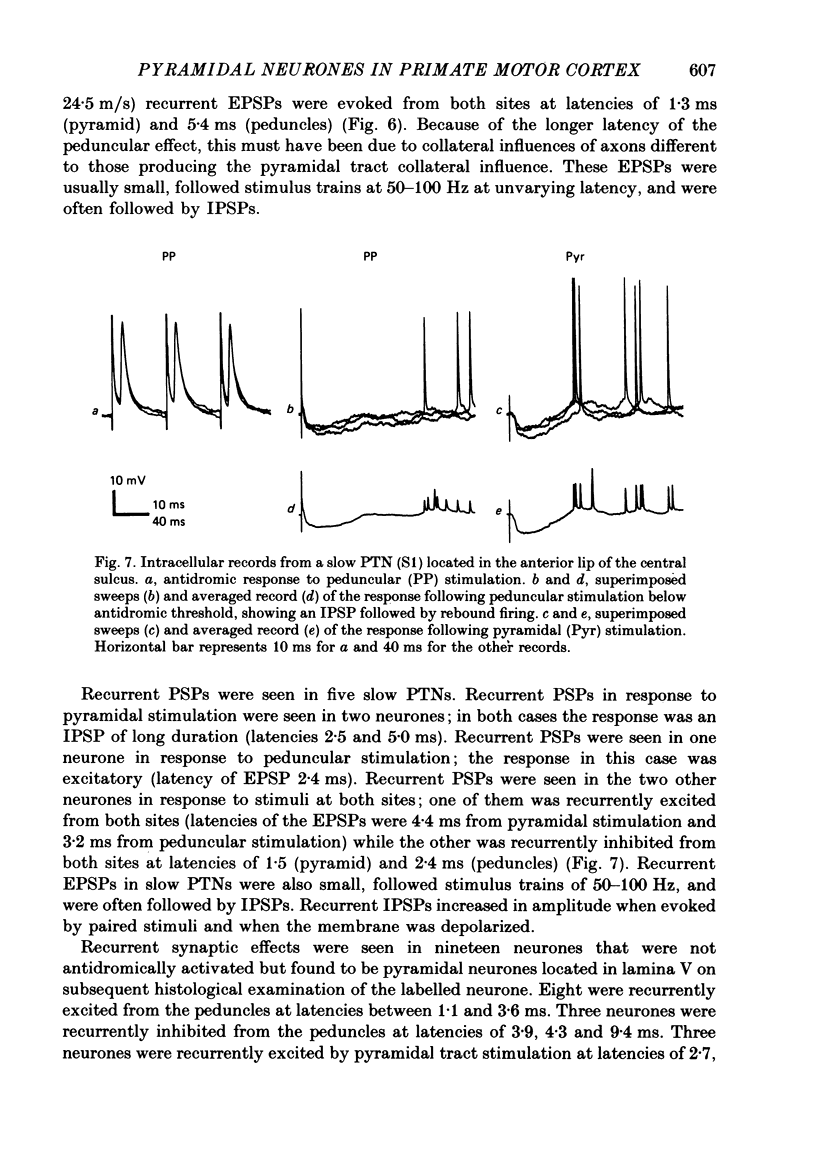
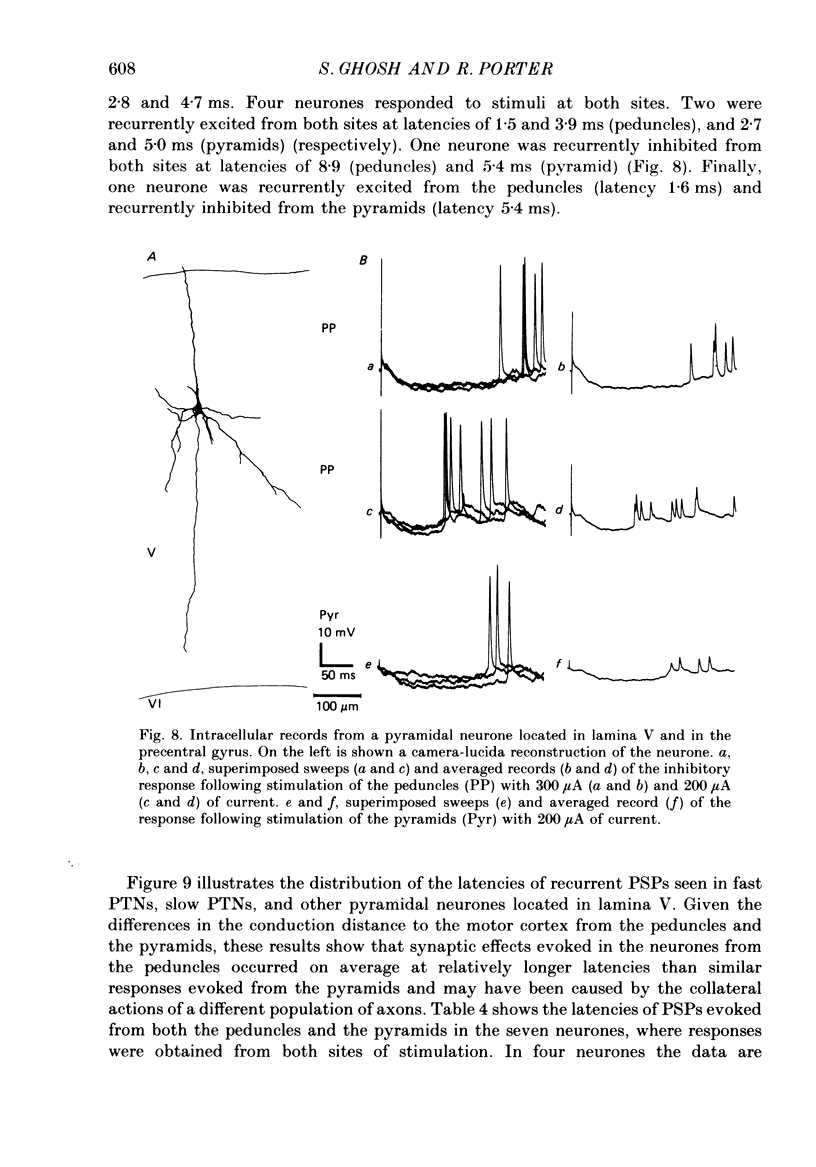
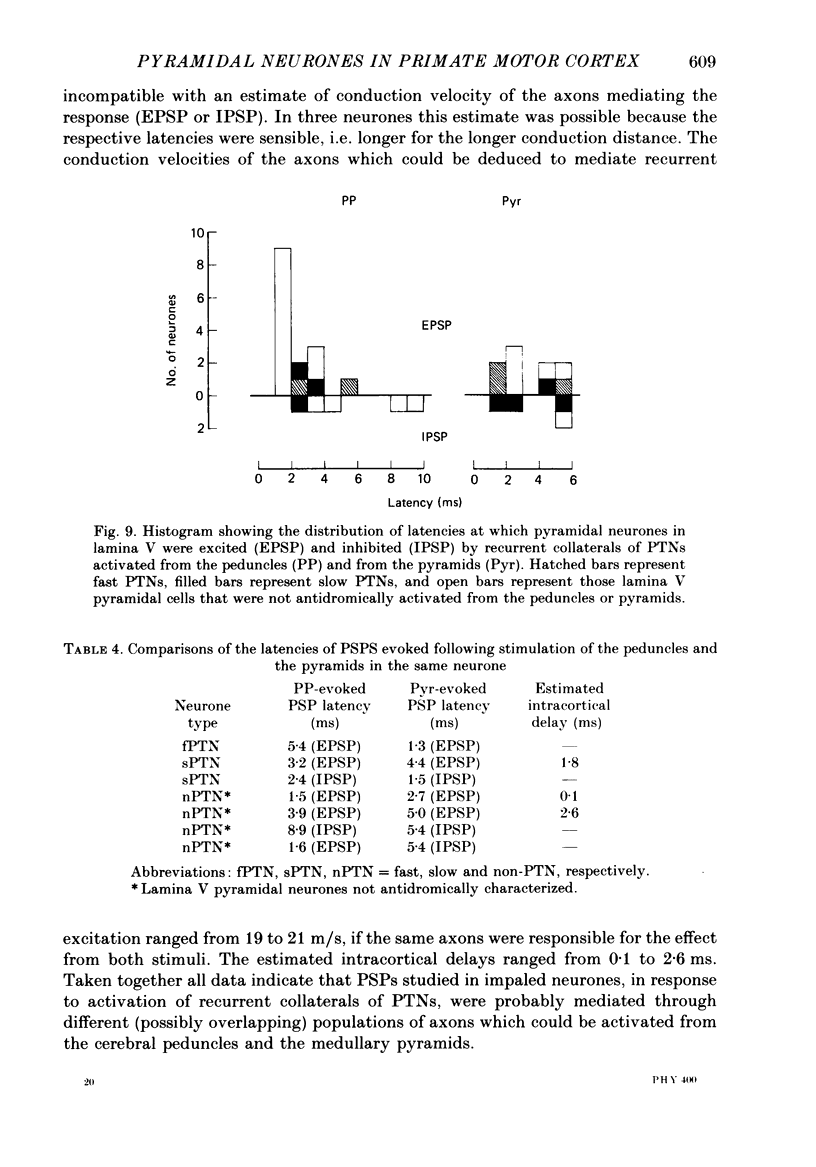
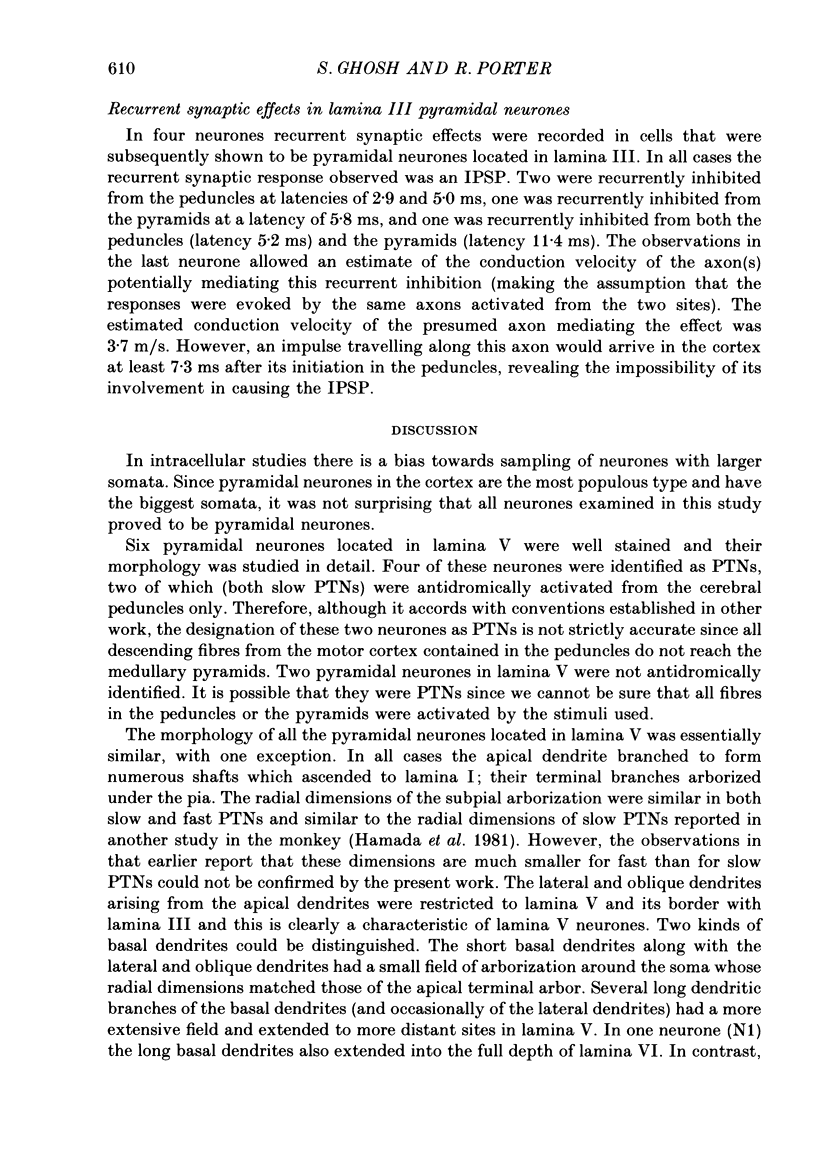
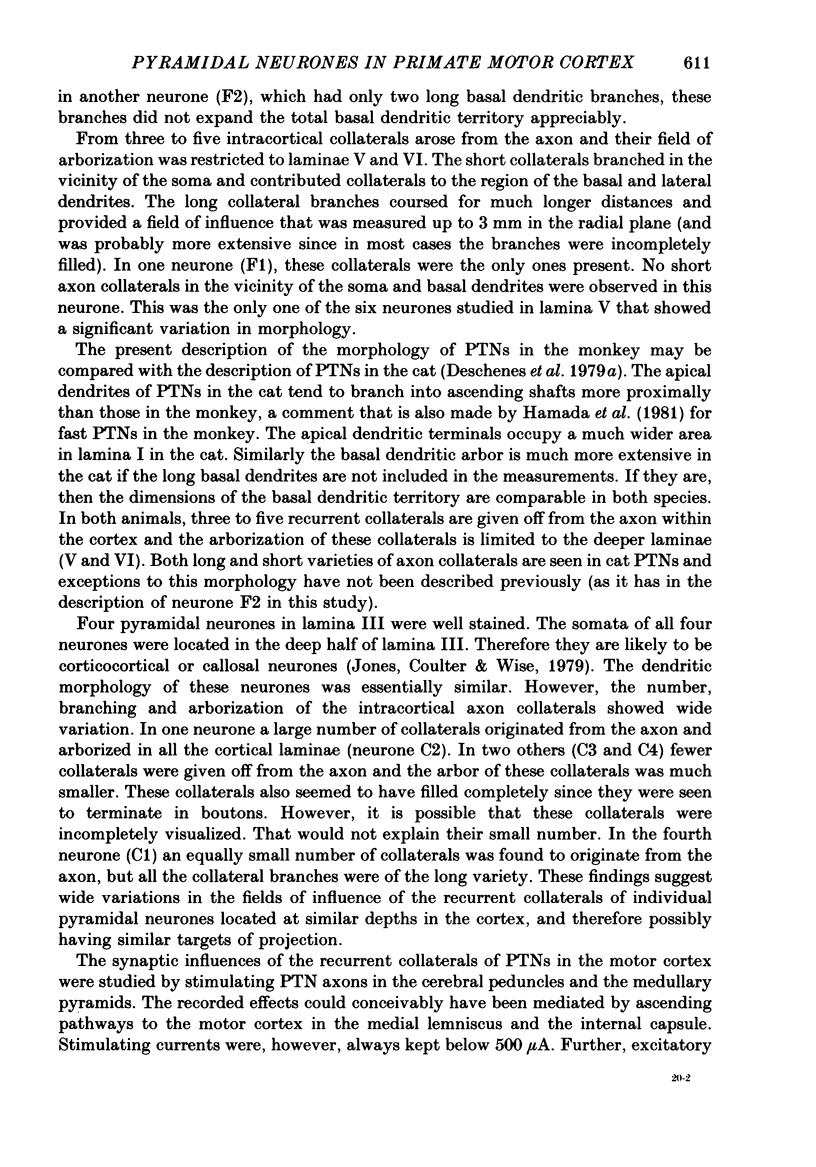
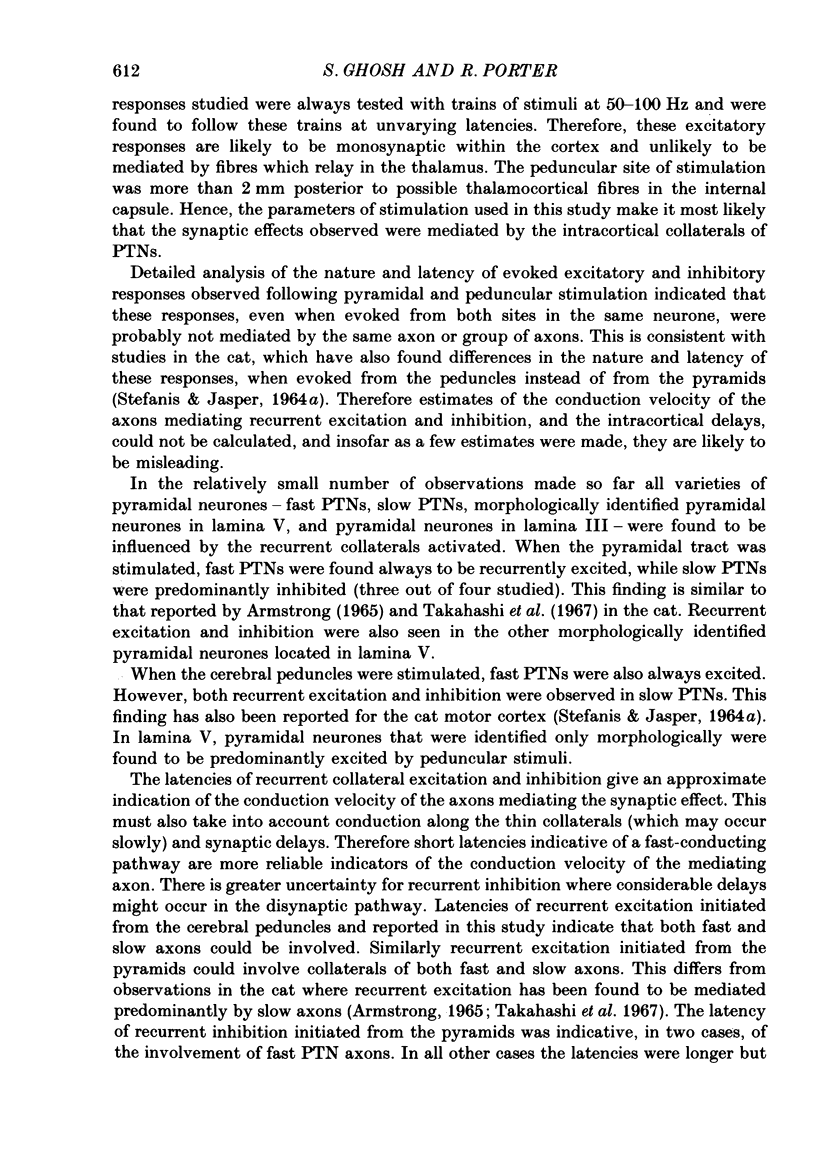
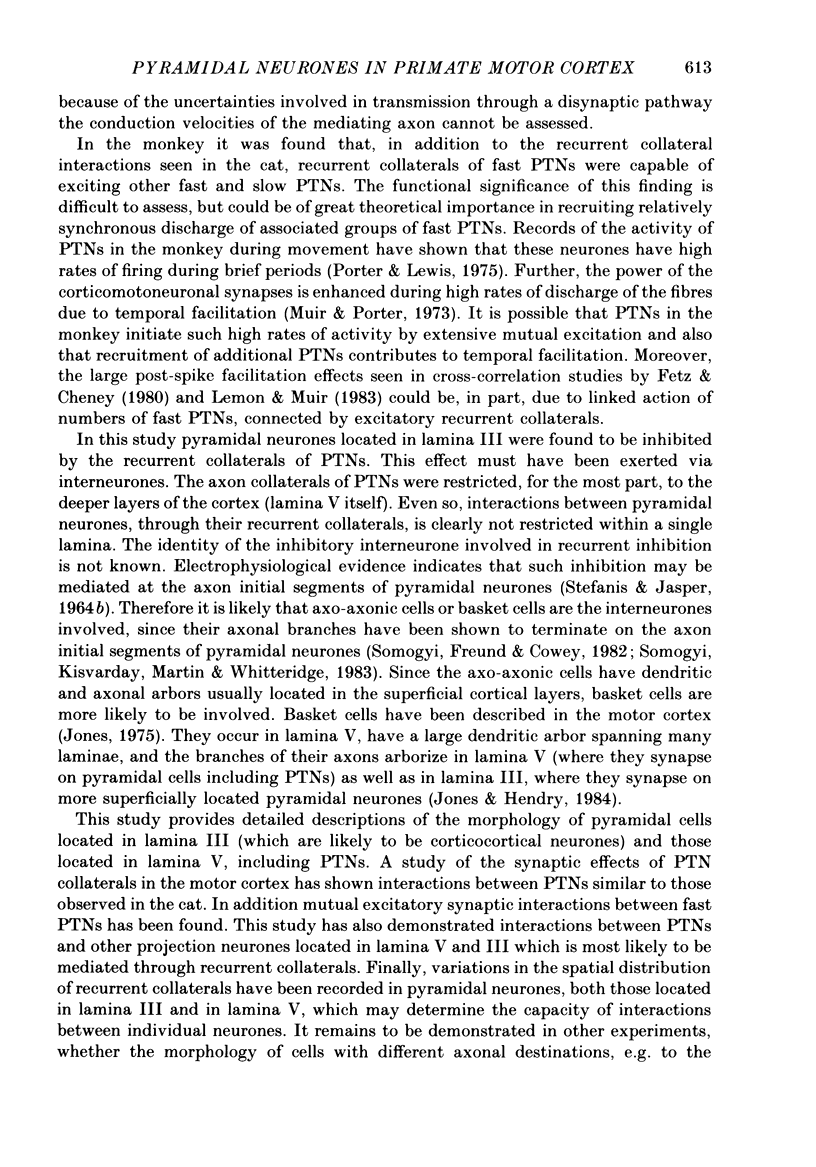
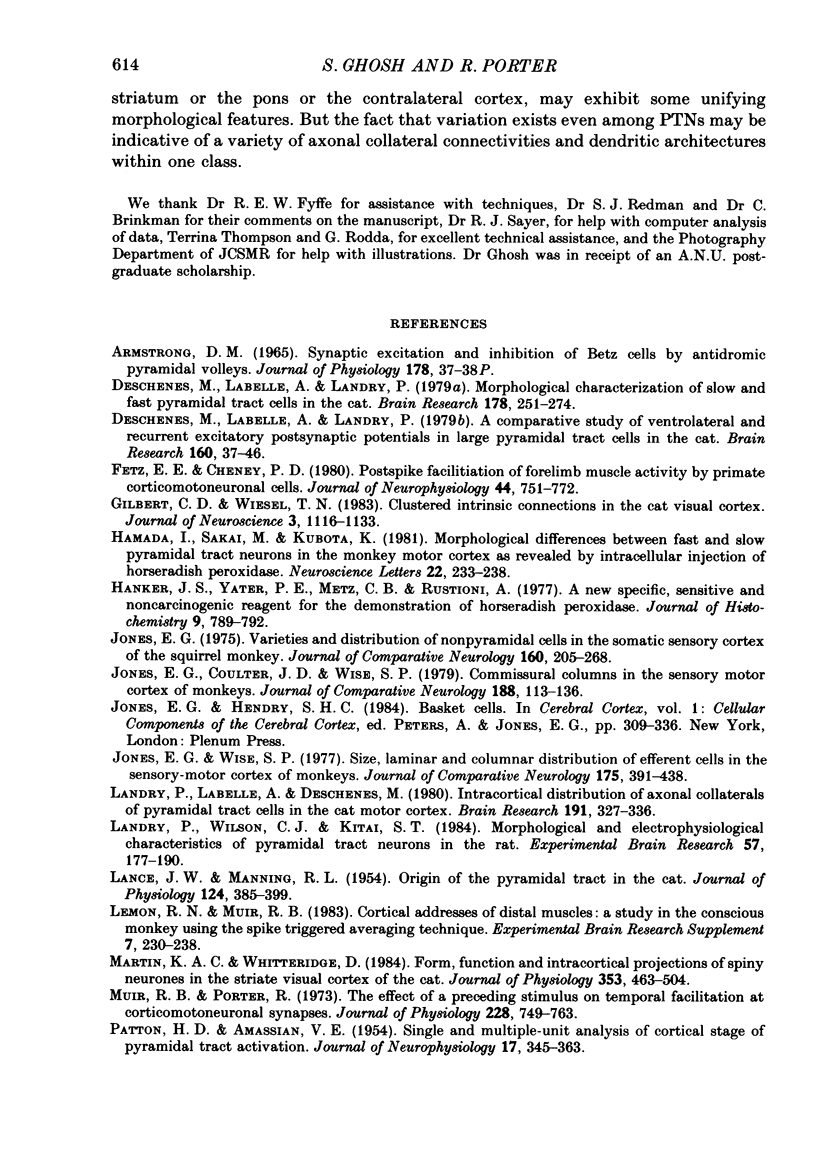
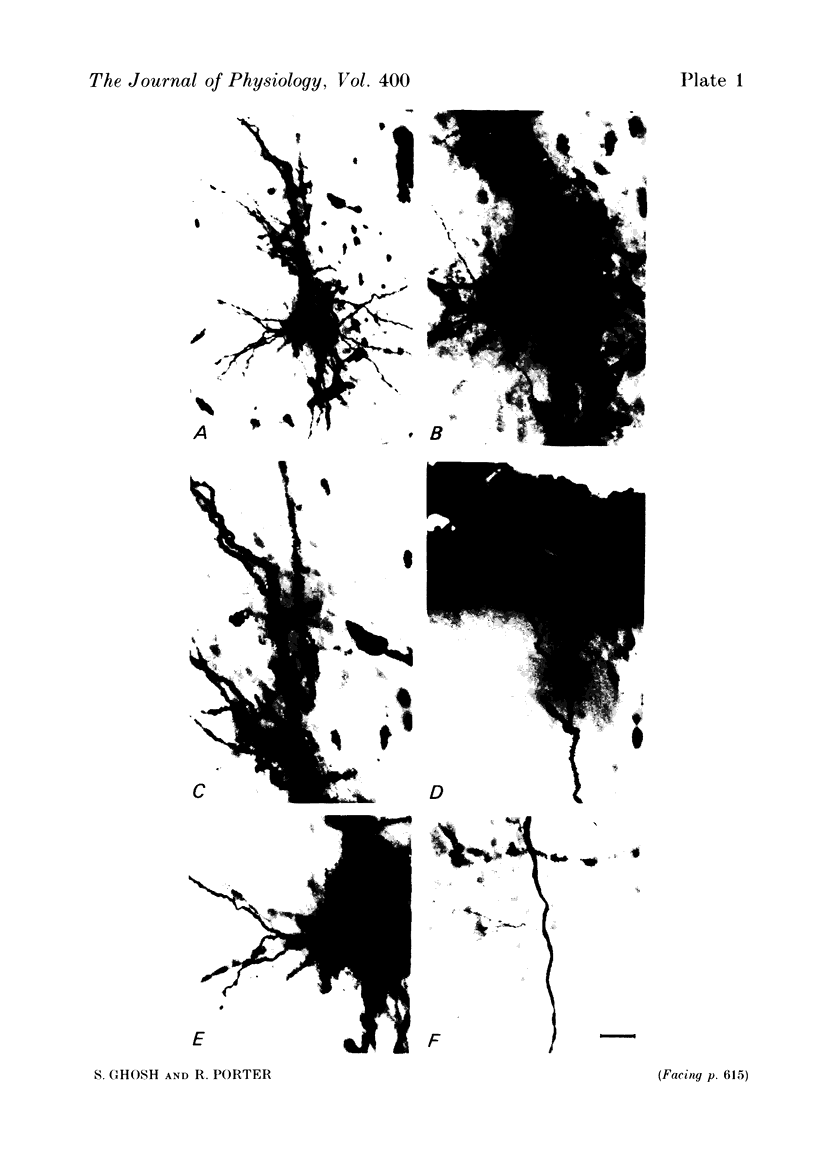
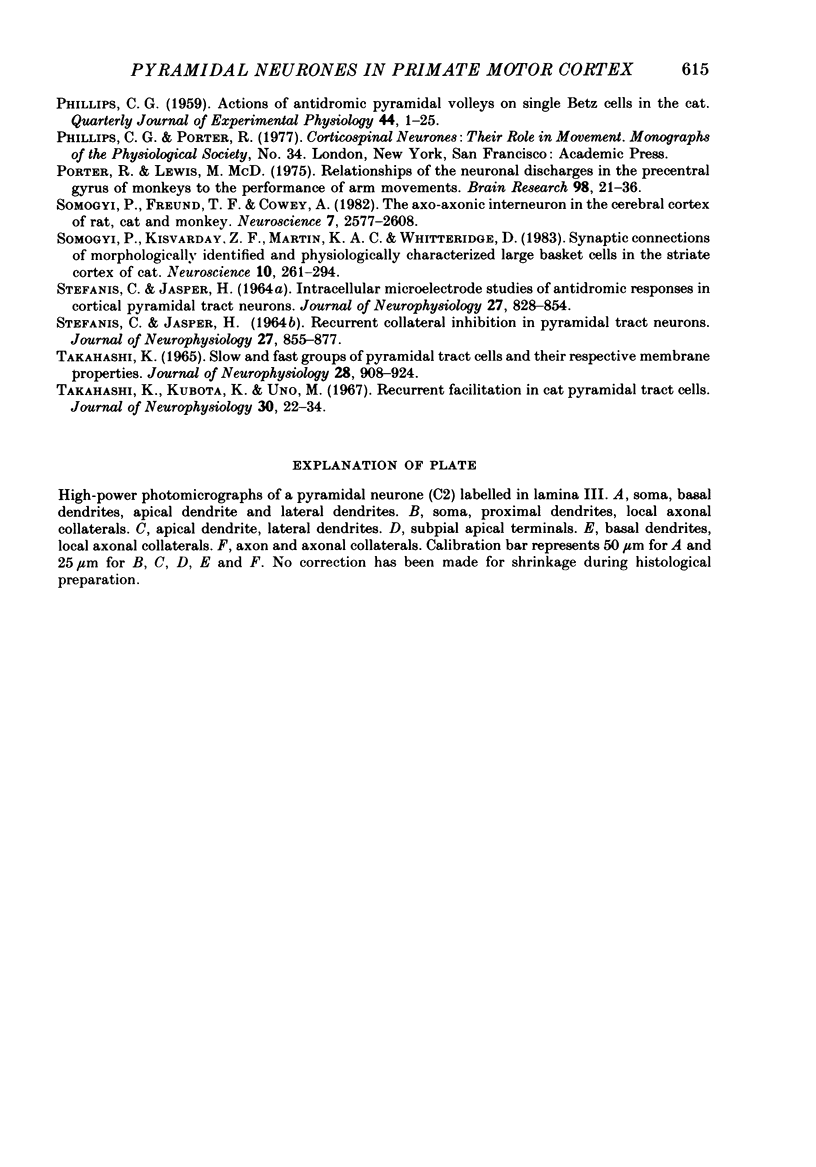
Images in this article
Selected References
These references are in PubMed. This may not be the complete list of references from this article.
- Deschênes M., Labelle A., Landry P. A comparative study of ventrolateral and recurrent excitatory postsynaptic potentials in large pyramidal tract cells in the cat. Brain Res. 1979 Jan 5;160(1):37–46. doi: 10.1016/0006-8993(79)90598-5. [DOI] [PubMed] [Google Scholar]
- Deschênes M., Labelle A., Landry P. Morphological characterization of slow and fast pyramidal tract cells in the cat. Brain Res. 1979 Dec 14;178(2-3):251–274. doi: 10.1016/0006-8993(79)90693-0. [DOI] [PubMed] [Google Scholar]
- Fetz E. E., Cheney P. D. Postspike facilitation of forelimb muscle activity by primate corticomotoneuronal cells. J Neurophysiol. 1980 Oct;44(4):751–772. doi: 10.1152/jn.1980.44.4.751. [DOI] [PubMed] [Google Scholar]
- Gilbert C. D., Wiesel T. N. Clustered intrinsic connections in cat visual cortex. J Neurosci. 1983 May;3(5):1116–1133. doi: 10.1523/JNEUROSCI.03-05-01116.1983. [DOI] [PMC free article] [PubMed] [Google Scholar]
- Hanker J. S., Yates P. E., Metz C. B., Rustioni A. A new specific, sensitive and non-carcinogenic reagent for the demonstration of horseradish peroxidase. Histochem J. 1977 Nov;9(6):789–792. doi: 10.1007/BF01003075. [DOI] [PubMed] [Google Scholar]
- Jones E. G., Coulter J. D., Wise S. P. Commissural columns in the sensory-motor cortex of monkeys. J Comp Neurol. 1979 Nov 1;188(1):113–135. doi: 10.1002/cne.901880110. [DOI] [PubMed] [Google Scholar]
- Jones E. G. Varieties and distribution of non-pyramidal cells in the somatic sensory cortex of the squirrel monkey. J Comp Neurol. 1975 Mar 15;160(2):205–267. doi: 10.1002/cne.901600204. [DOI] [PubMed] [Google Scholar]
- Jones E. G., Wise S. P. Size, laminar and columnar distribution of efferent cells in the sensory-motor cortex of monkeys. J Comp Neurol. 1977 Oct 15;175(4):391–438. doi: 10.1002/cne.901750403. [DOI] [PubMed] [Google Scholar]
- LANCE J. W., MANNING R. L. Origin of the pyramidal tract in the cat. J Physiol. 1954 May 28;124(2):385–399. doi: 10.1113/jphysiol.1954.sp005115. [DOI] [PMC free article] [PubMed] [Google Scholar]
- Landry P., Labelle A., Deschênes M. Intracortical distribution of axonal collaterals of pyramidal tract cells in the cat motor cortex. Brain Res. 1980 Jun 9;191(2):327–336. doi: 10.1016/0006-8993(80)91284-6. [DOI] [PubMed] [Google Scholar]
- Landry P., Wilson C. J., Kitai S. T. Morphological and electrophysiological characteristics of pyramidal tract neurons in the rat. Exp Brain Res. 1984;57(1):177–190. doi: 10.1007/BF00231144. [DOI] [PubMed] [Google Scholar]
- Martin K. A., Whitteridge D. Form, function and intracortical projections of spiny neurones in the striate visual cortex of the cat. J Physiol. 1984 Aug;353:463–504. doi: 10.1113/jphysiol.1984.sp015347. [DOI] [PMC free article] [PubMed] [Google Scholar]
- Muir R. B., Porter R. The effect of a preceding stimulus on temporal facilitation at corticomotoneuronal synapses. J Physiol. 1973 Feb;228(3):749–763. doi: 10.1113/jphysiol.1973.sp010110. [DOI] [PMC free article] [PubMed] [Google Scholar]
- PATTON H. D., AMASSIAN V. E. Single and multiple-unit analysis of cortical stage of pyramidal tract activation. J Neurophysiol. 1954 Jul;17(4):345–363. doi: 10.1152/jn.1954.17.4.345. [DOI] [PubMed] [Google Scholar]
- PHILLIPS C. G. Actions of antidromic pyramidal volleys on single Betz cells in the cat. Q J Exp Physiol Cogn Med Sci. 1959 Jan;44(1):1–25. doi: 10.1113/expphysiol.1959.sp001364. [DOI] [PubMed] [Google Scholar]
- Porter R., Lewis M. M. Relationship of neuronal discharges in the precentral gyrus of monkeys to the performance of arm movements. Brain Res. 1975 Nov 7;98(1):21–36. doi: 10.1016/0006-8993(75)90507-7. [DOI] [PubMed] [Google Scholar]
- STEFANIS C., JASPER H. INTRACELLULAR MICROELECTRODE STUDIES OF ANTIDROMIC RESPONSES IN CORTICAL PYRAMIDAL TRACT NEURONS. J Neurophysiol. 1964 Sep;27:828–854. doi: 10.1152/jn.1964.27.5.828. [DOI] [PubMed] [Google Scholar]
- STEFANIS C., JASPER H. RECURRENT COLLATERAL INHIBITION IN PYRAMIDAL TRACT NEURONS. J Neurophysiol. 1964 Sep;27:855–877. doi: 10.1152/jn.1964.27.5.855. [DOI] [PubMed] [Google Scholar]
- Somogyi P., Freund T. F., Cowey A. The axo-axonic interneuron in the cerebral cortex of the rat, cat and monkey. Neuroscience. 1982;7(11):2577–2607. doi: 10.1016/0306-4522(82)90086-0. [DOI] [PubMed] [Google Scholar]
- Somogyi P., Kisvárday Z. F., Martin K. A., Whitteridge D. Synaptic connections of morphologically identified and physiologically characterized large basket cells in the striate cortex of cat. Neuroscience. 1983 Oct;10(2):261–294. doi: 10.1016/0306-4522(83)90133-1. [DOI] [PubMed] [Google Scholar]
- Takahashi K. Slow and fast groups of pyramidal tract cells and their respective membrane properties. J Neurophysiol. 1965 Sep;28(5):908–924. doi: 10.1152/jn.1965.28.5.908. [DOI] [PubMed] [Google Scholar]