Abstract
1. The whole-cell voltage clamp technique was used to record Ba2+ currents in voltage-sensitive Ca2+ channels in mouse flexor digitorum brevis muscles developing in situ from day 1 to 30 after birth. Effects of denervation and tissue culture on the Ca2+ channel currents were also studied. 2. The muscle fibres in newborn mice showed two distinct types of Ca2+ channel currents, a low-threshold transient current and a high-threshold sustained current. 3. The specific amplitude of the transient current was 2.7 +/- 1.7 (S.D.) A/F in response to -30 mV test pulses in medium containing 30 mM-Ba2+ on day 1 after birth. The transient current decreased progressively in the post-natal days and became undetectable by day 17. In contrast, the specific amplitude of the sustained current in response to +20 mV test pulses increased 4-fold from 6.9 A/F on day 1 to 27.7 A/F on day 30. 4. The disappearance of the transient current could not be accounted for by either shifts in voltage dependence of activation and inactivation or changes in activation and inactivation times of the two types of current during development. 5. Denervating muscle fibres on day 8 after birth did not prevent the disappearance of the transient current. Denervating them on day 17 did not allow reappearance of the transient current. However, the increase of the sustained current was suppressed by the denervation either on day 8 or day 17. 6. In muscle fibres isolated on day 8 after birth and cultured thereafter, the transient current did not disappear until day 19 in culture (27 days after birth), while the sustained current was maintained at the level on day 8. 7. In muscle fibres isolated on day 17, when the transient current had become undetectable, and cultured thereafter, the transient current did not reappear until day 15 in culture (32 days after birth), while the sustained current was maintained at a level similar to that on day 17. 8. We conclude that innervation has little influence on the developmental disappearance of the transient Ca2+ channel current in mouse muscle fibres, and suggest that some influencing factors from surroundings other than the nerve may be required for the disappearance of the functional transient channels.
Full text
PDF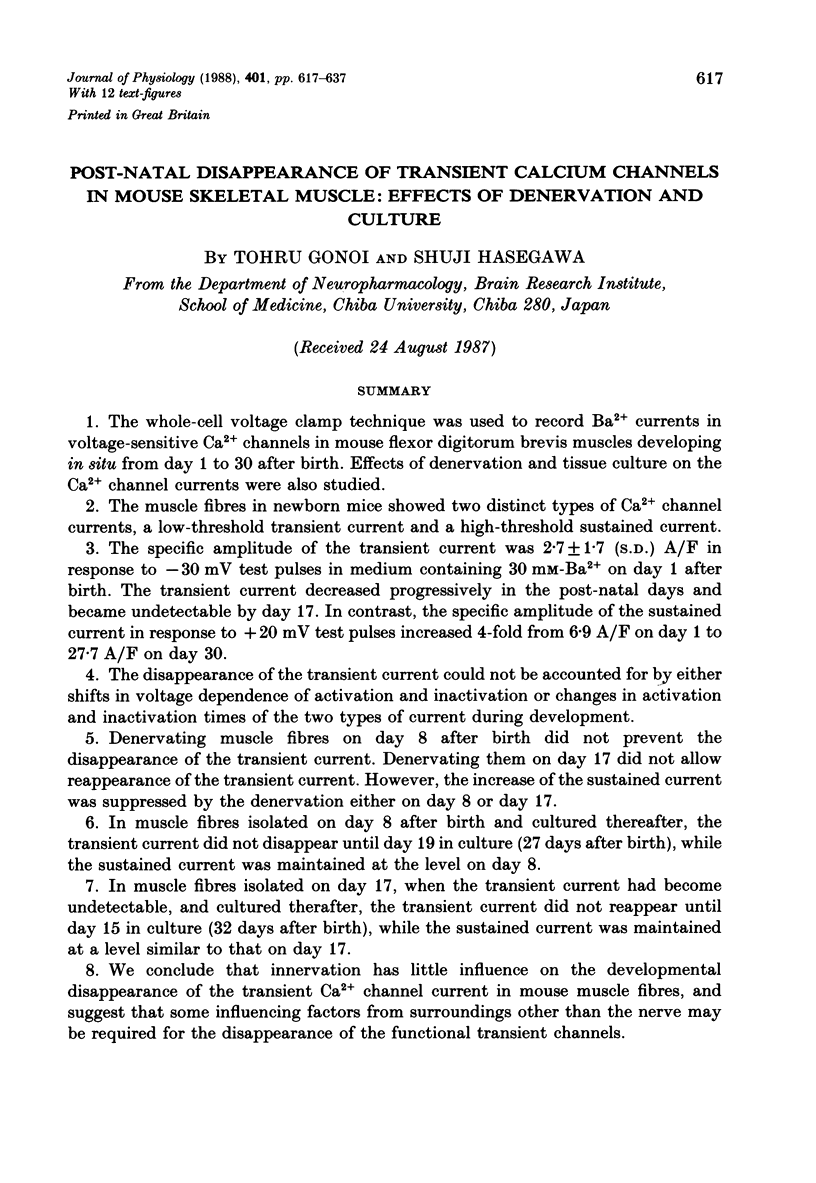
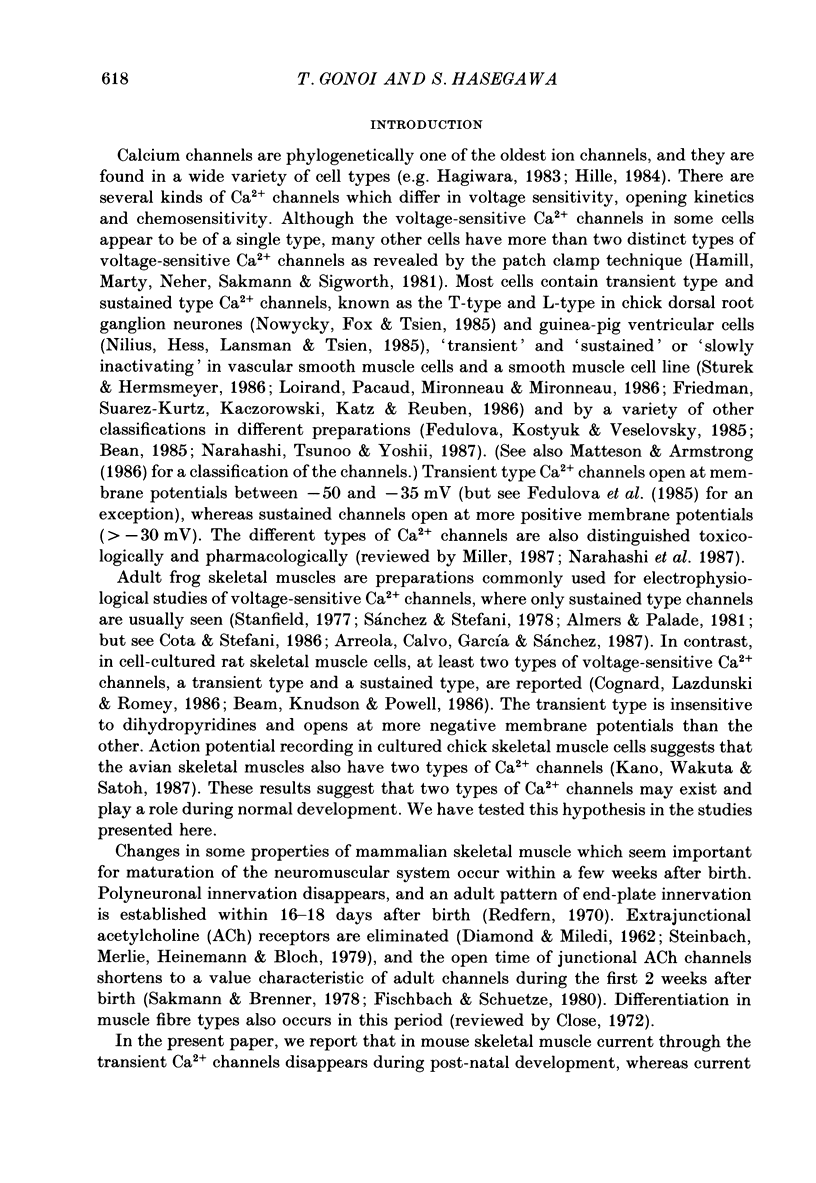
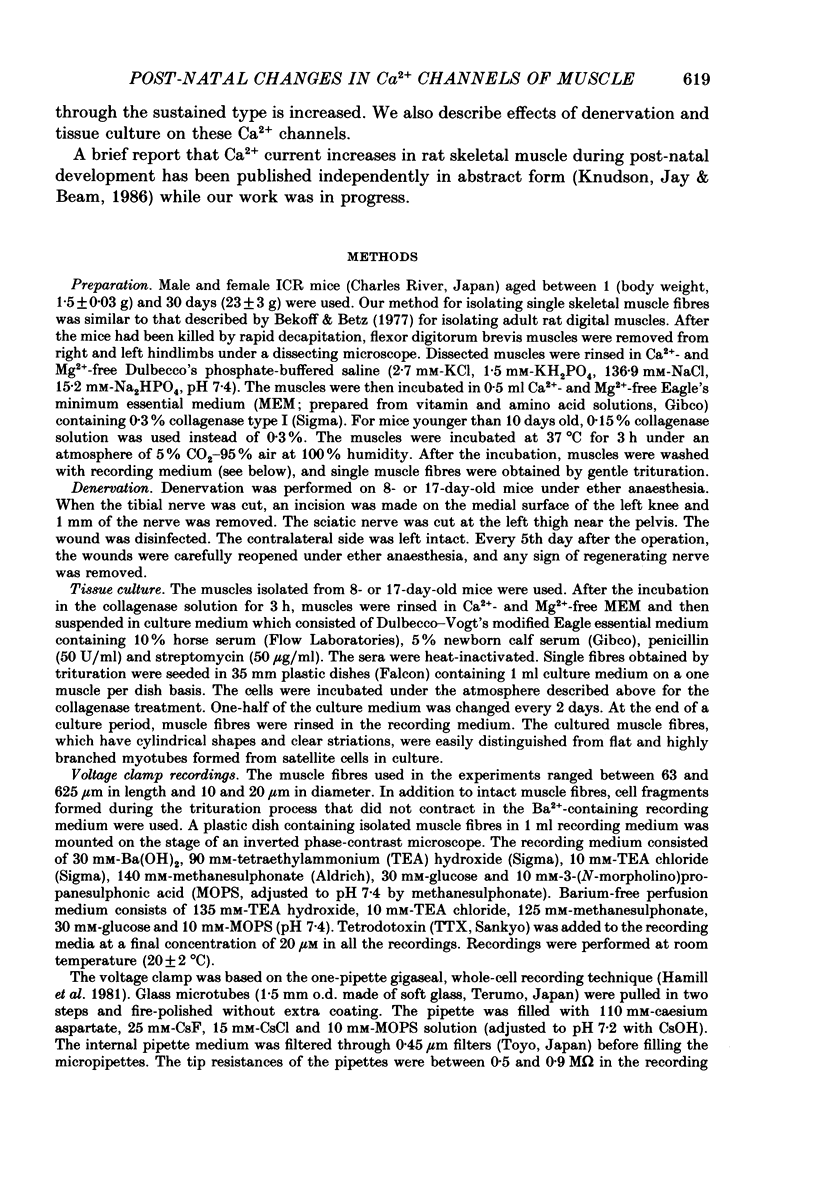
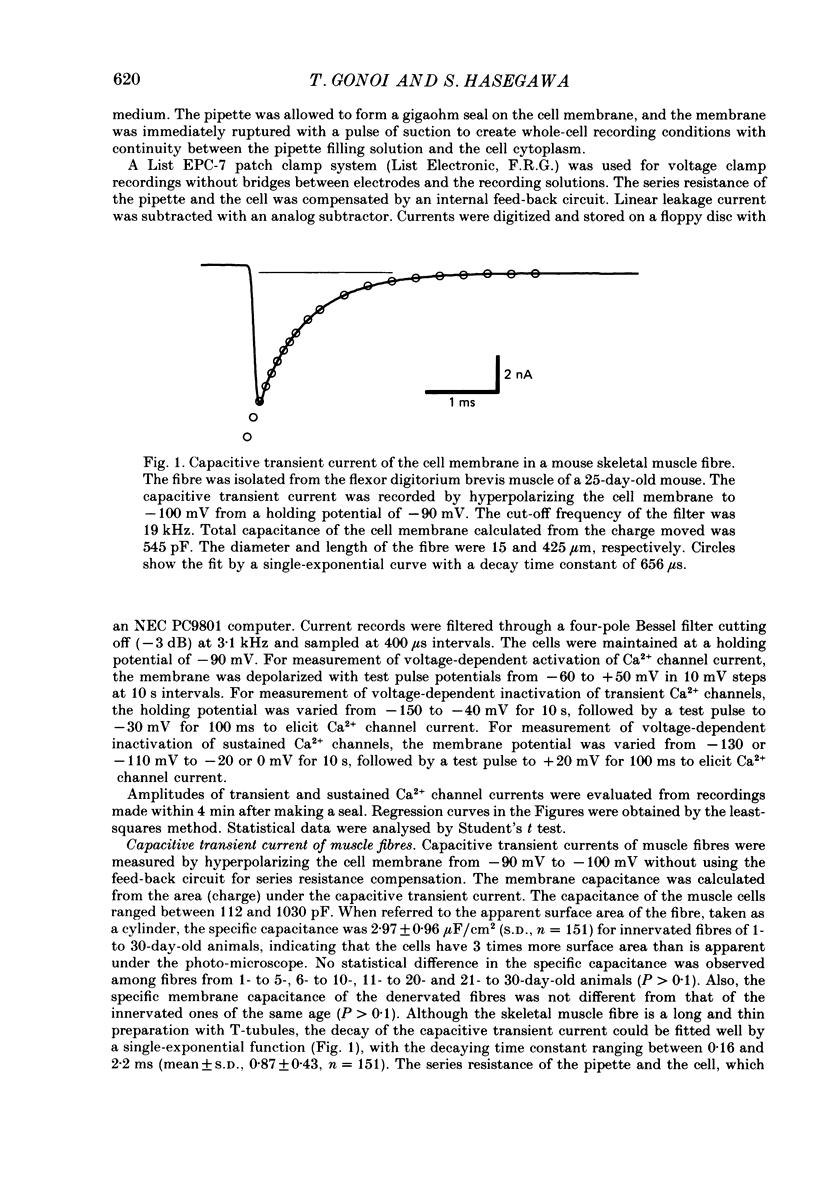
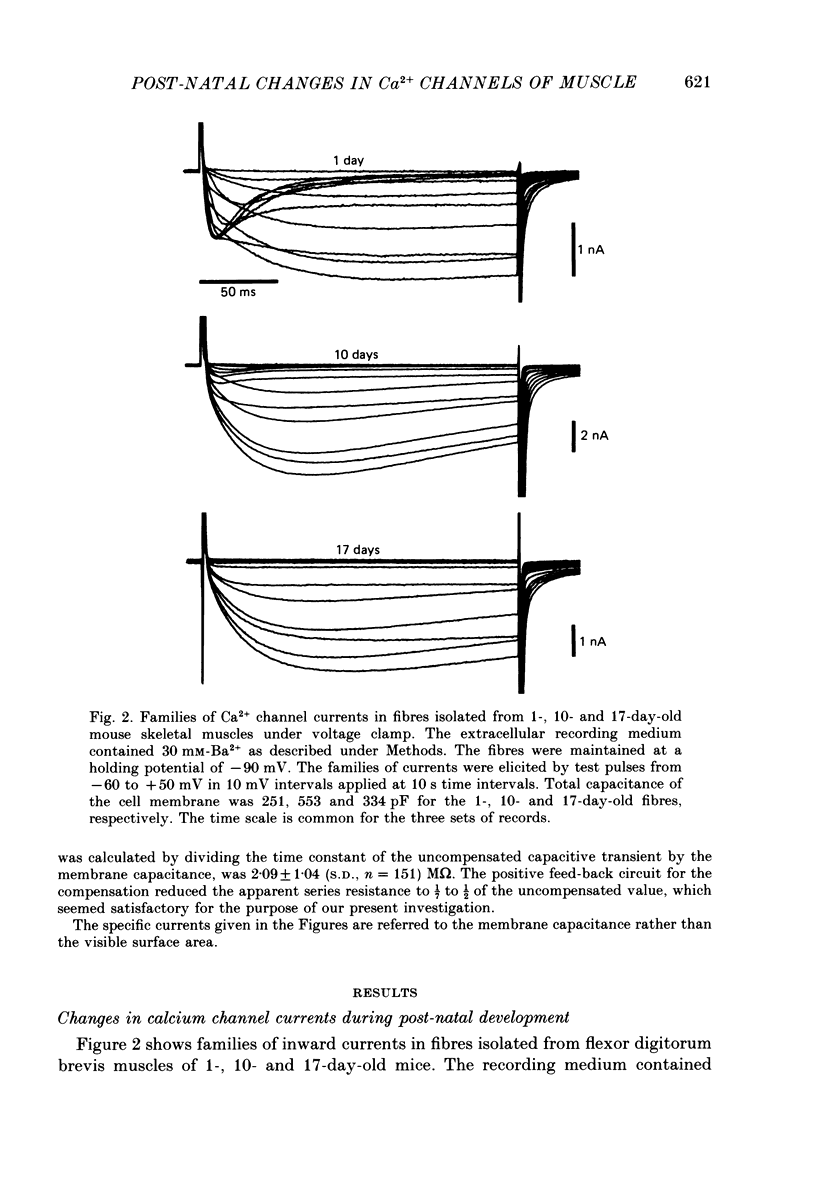
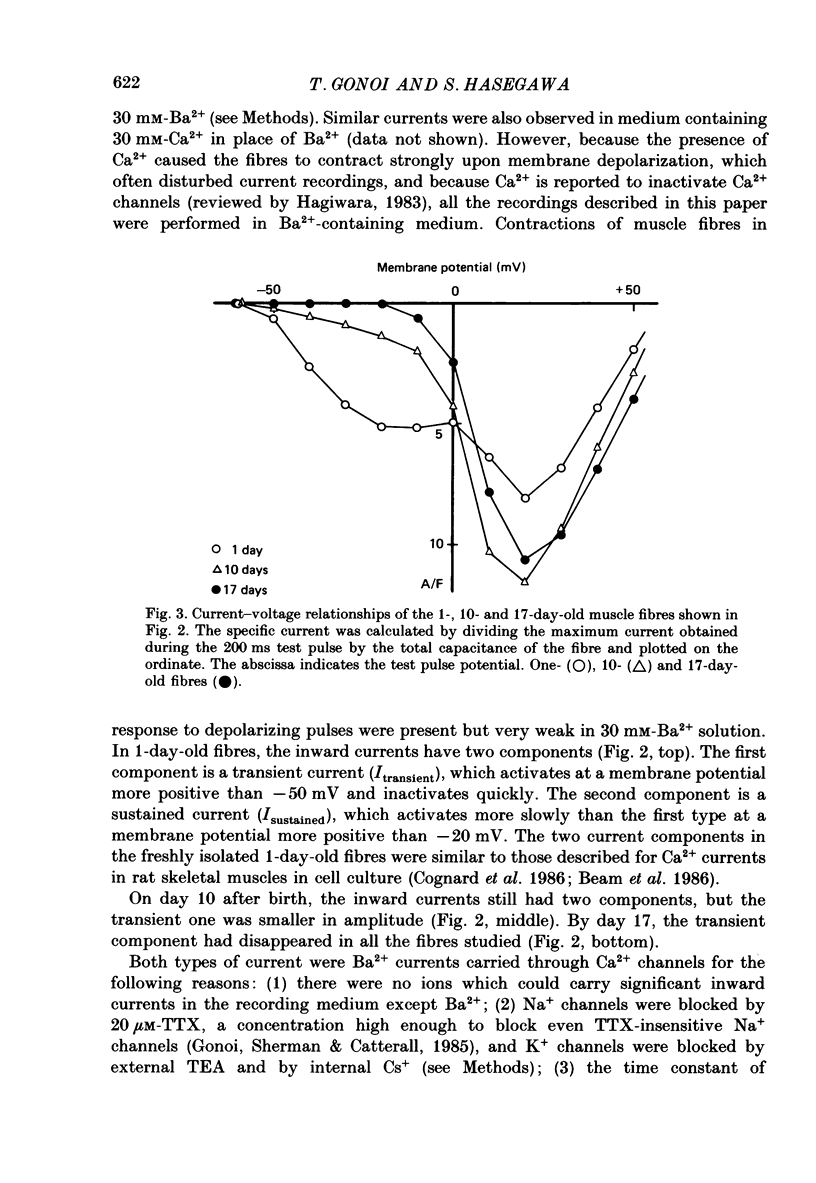
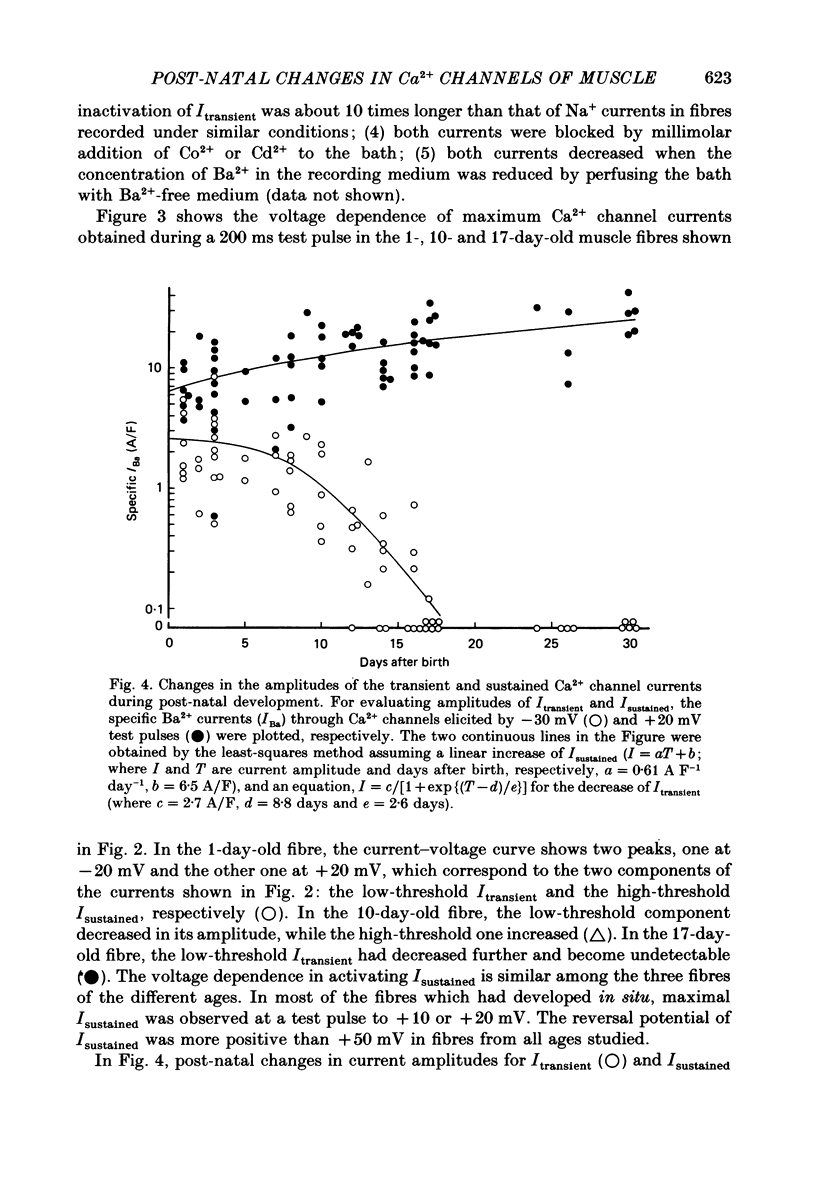
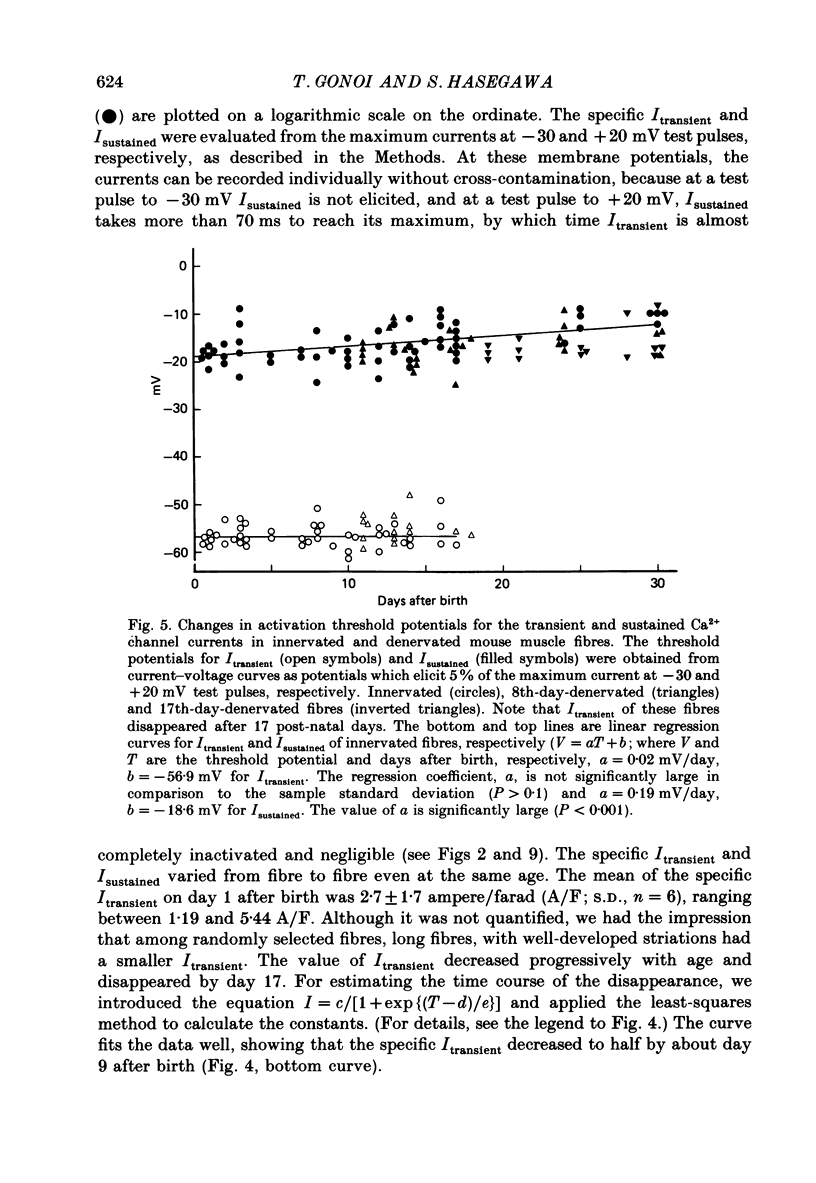
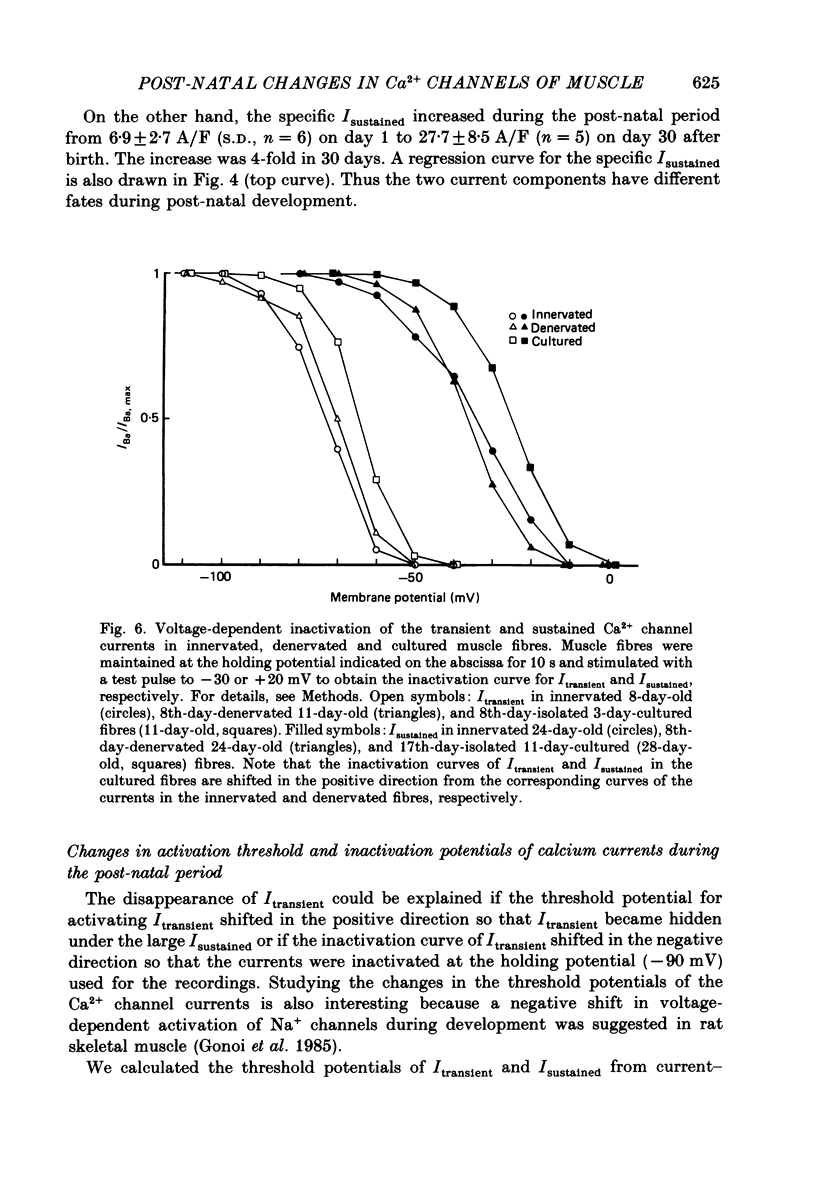
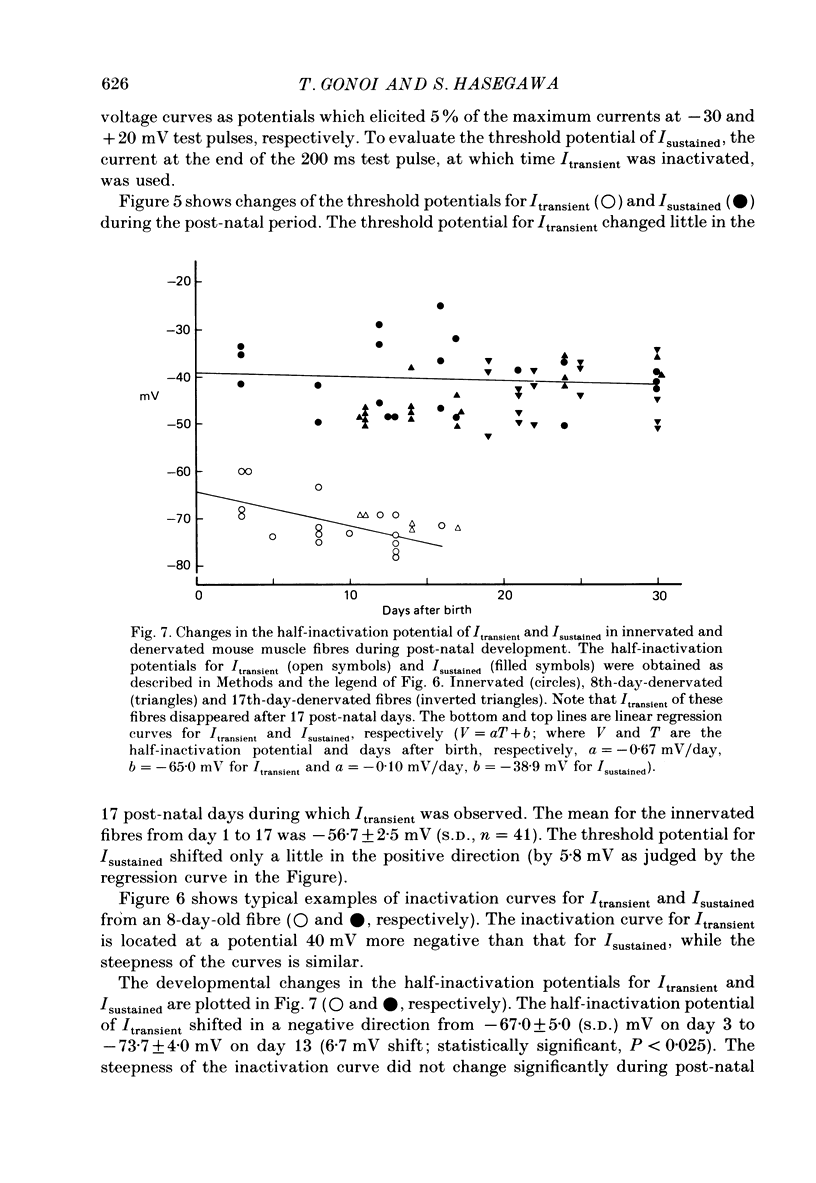
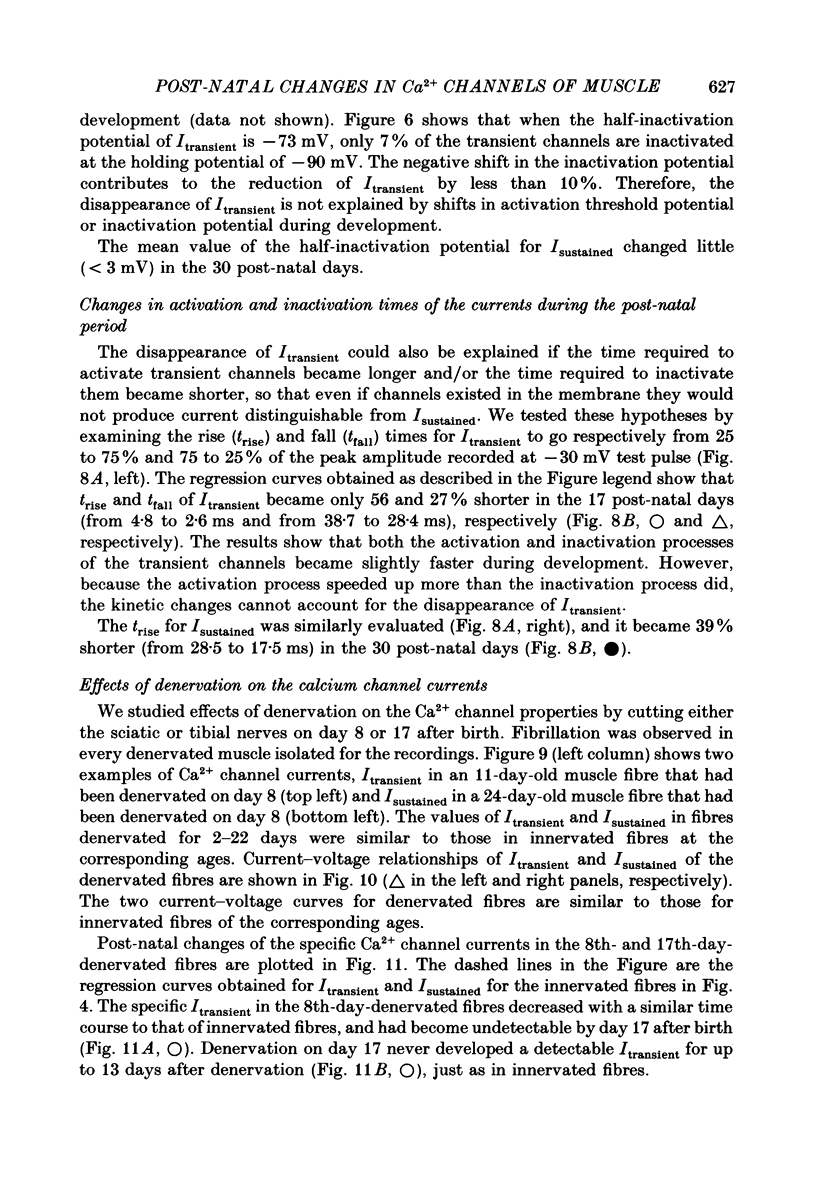
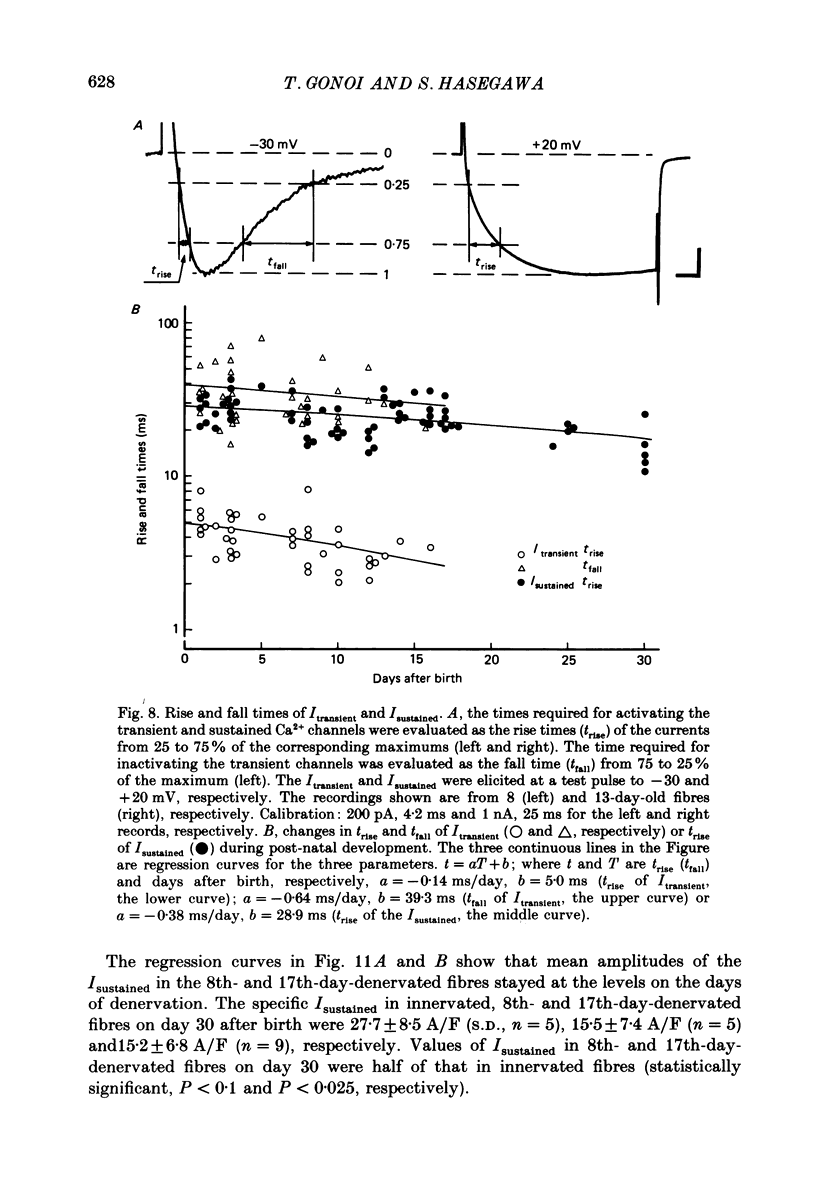
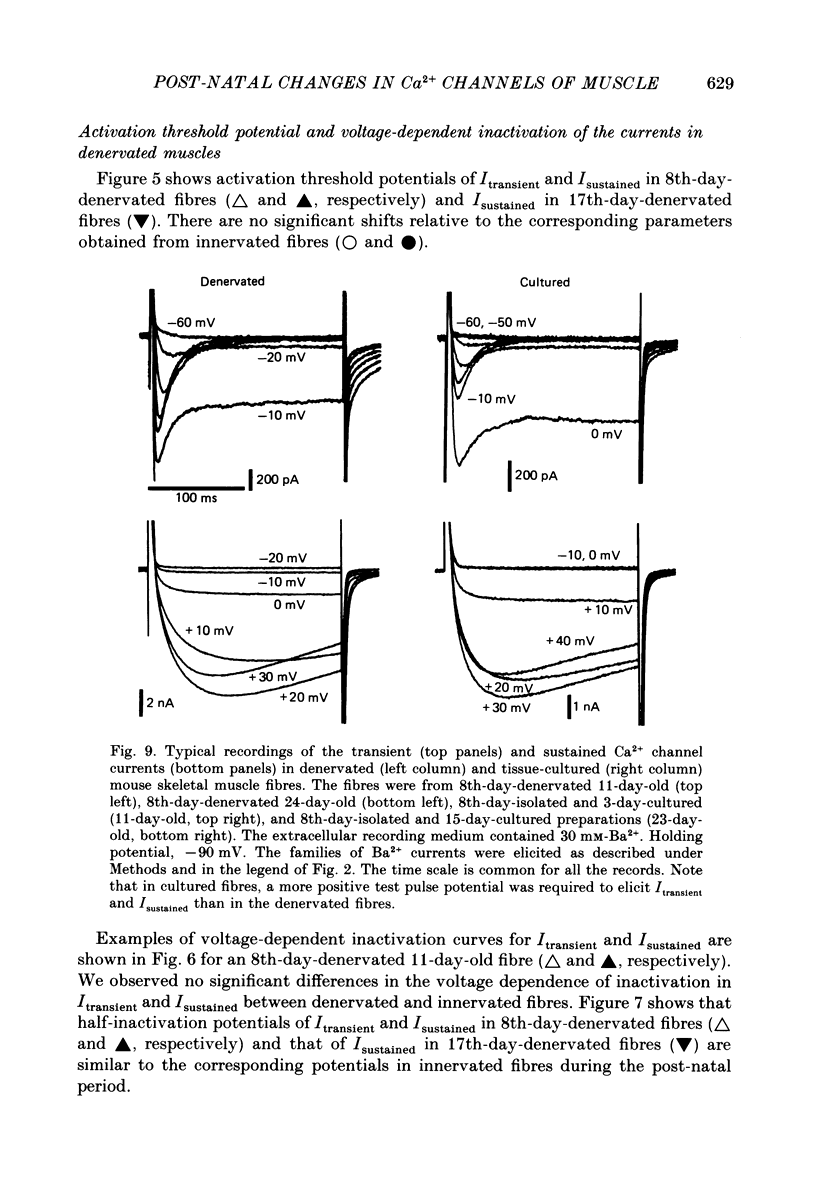
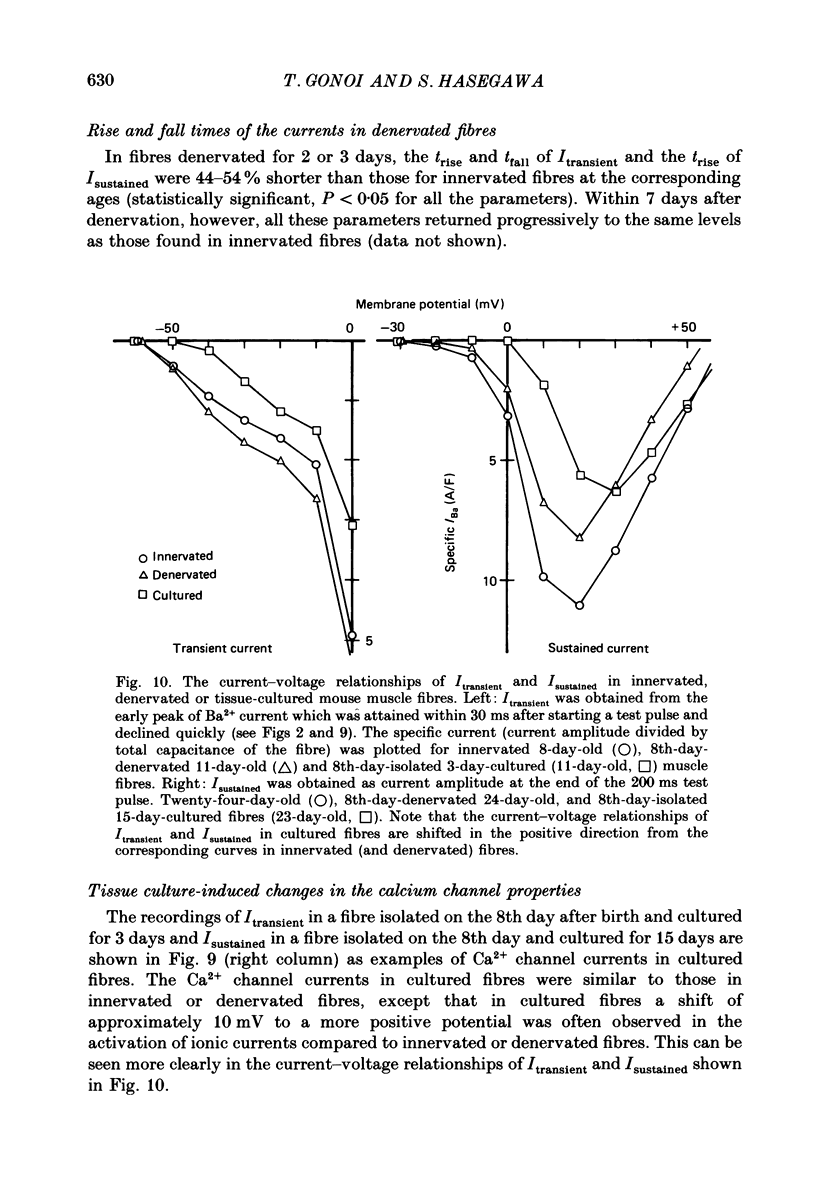
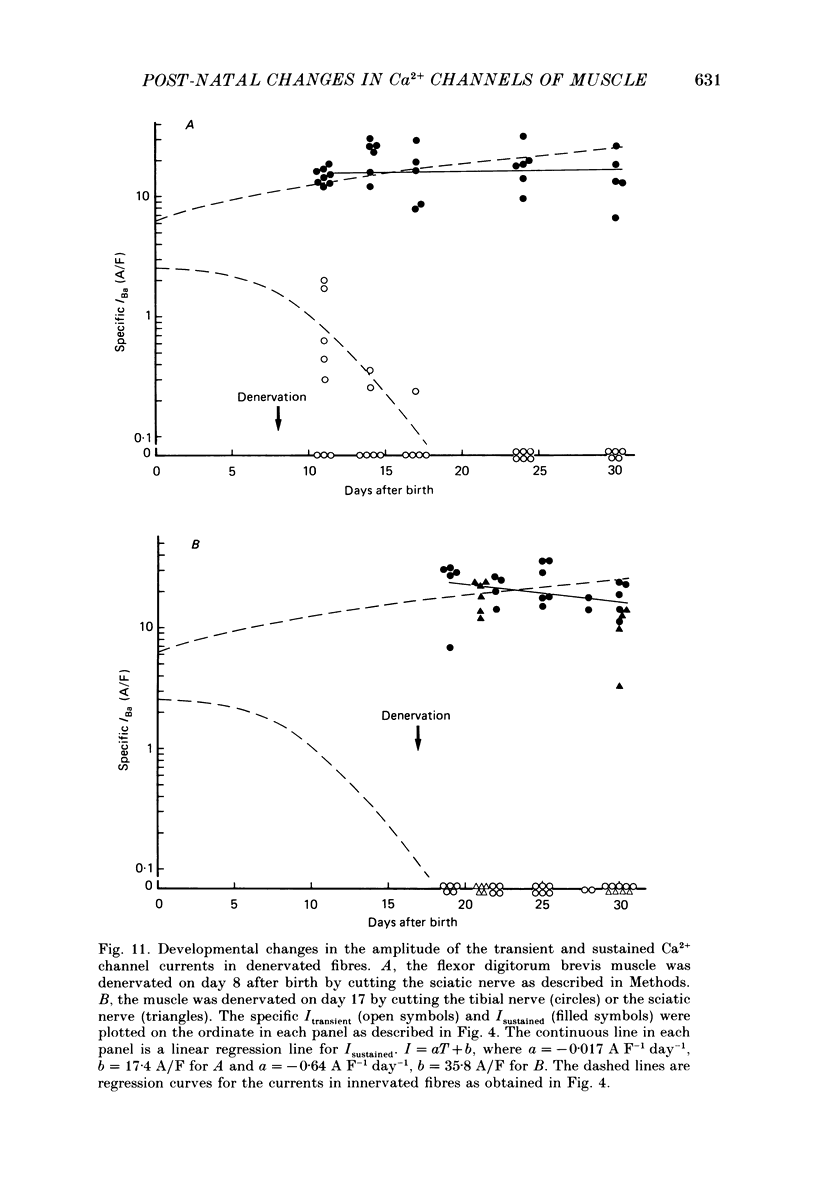
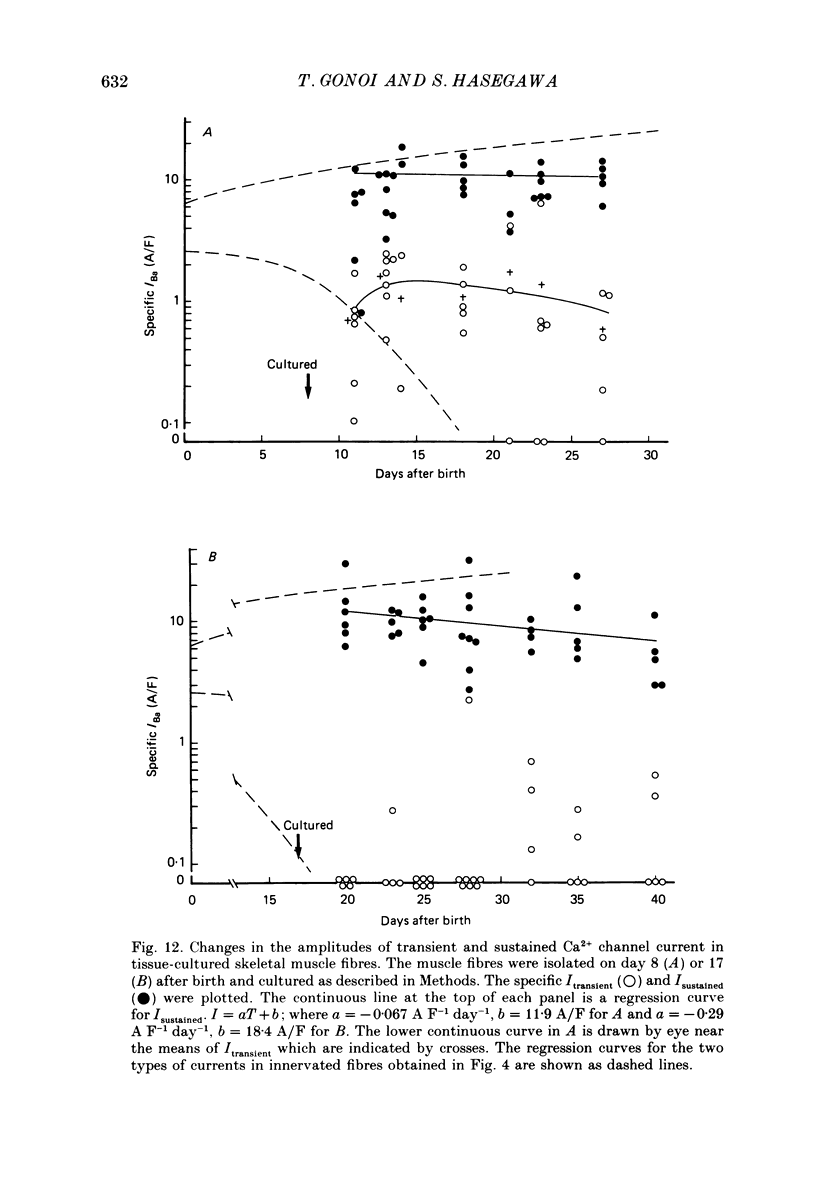
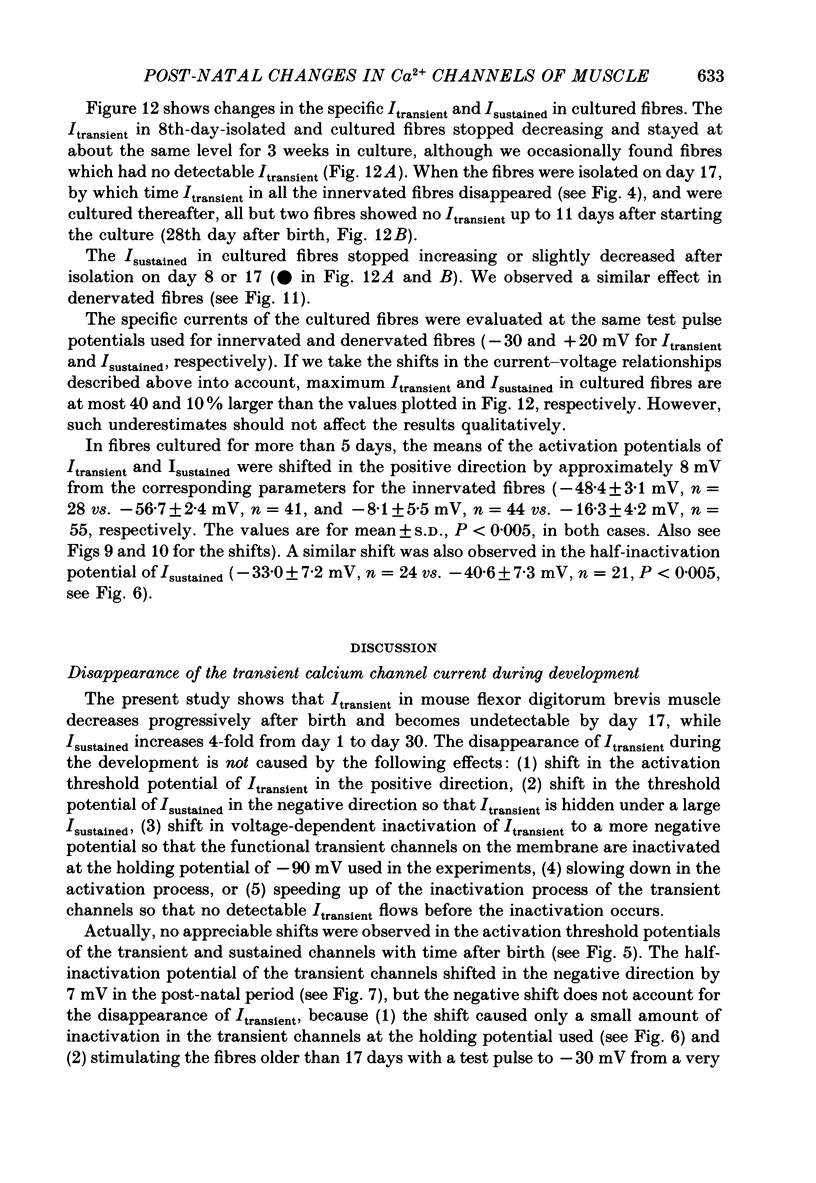
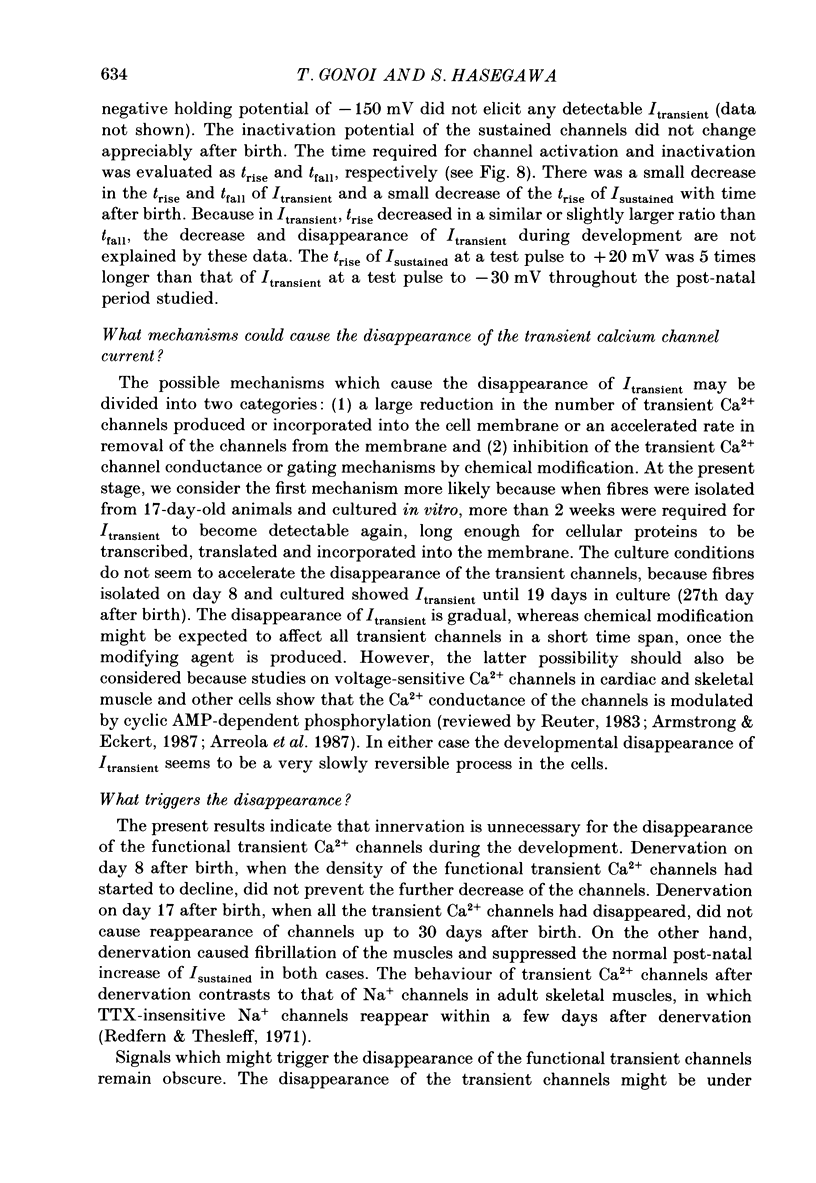
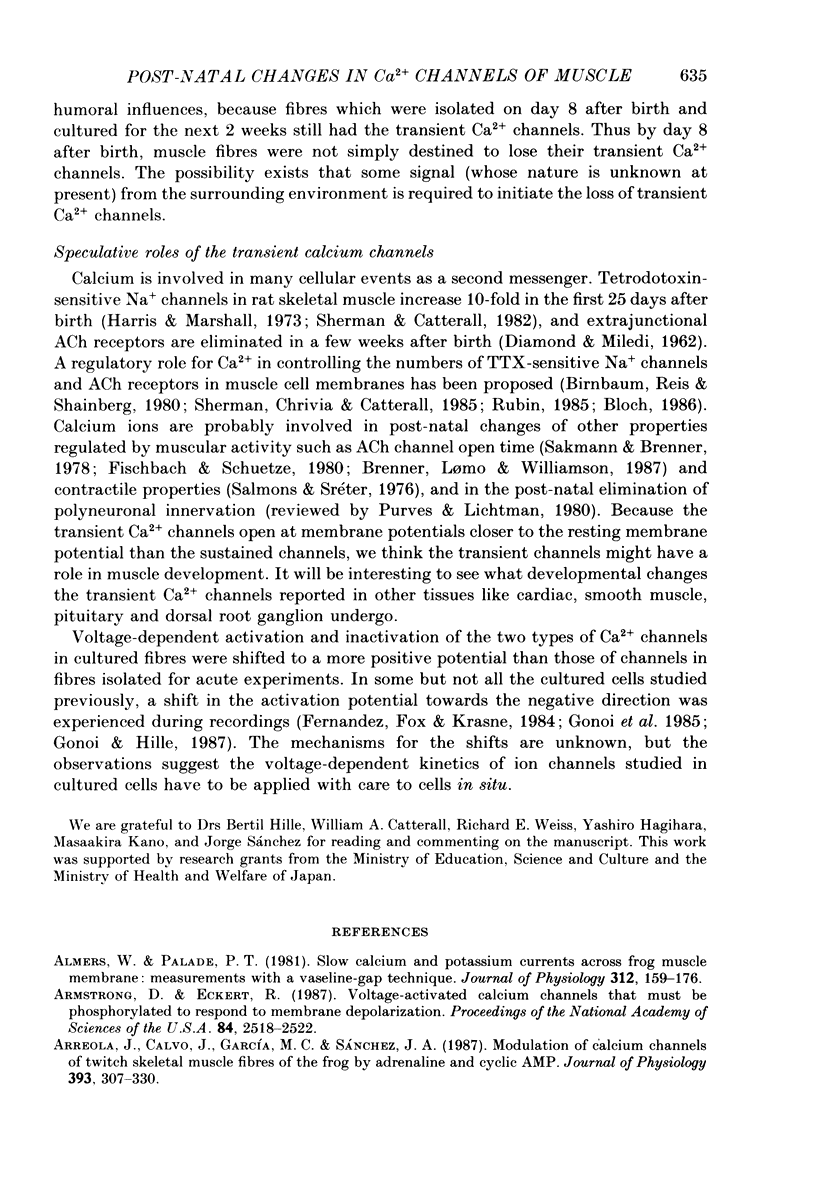
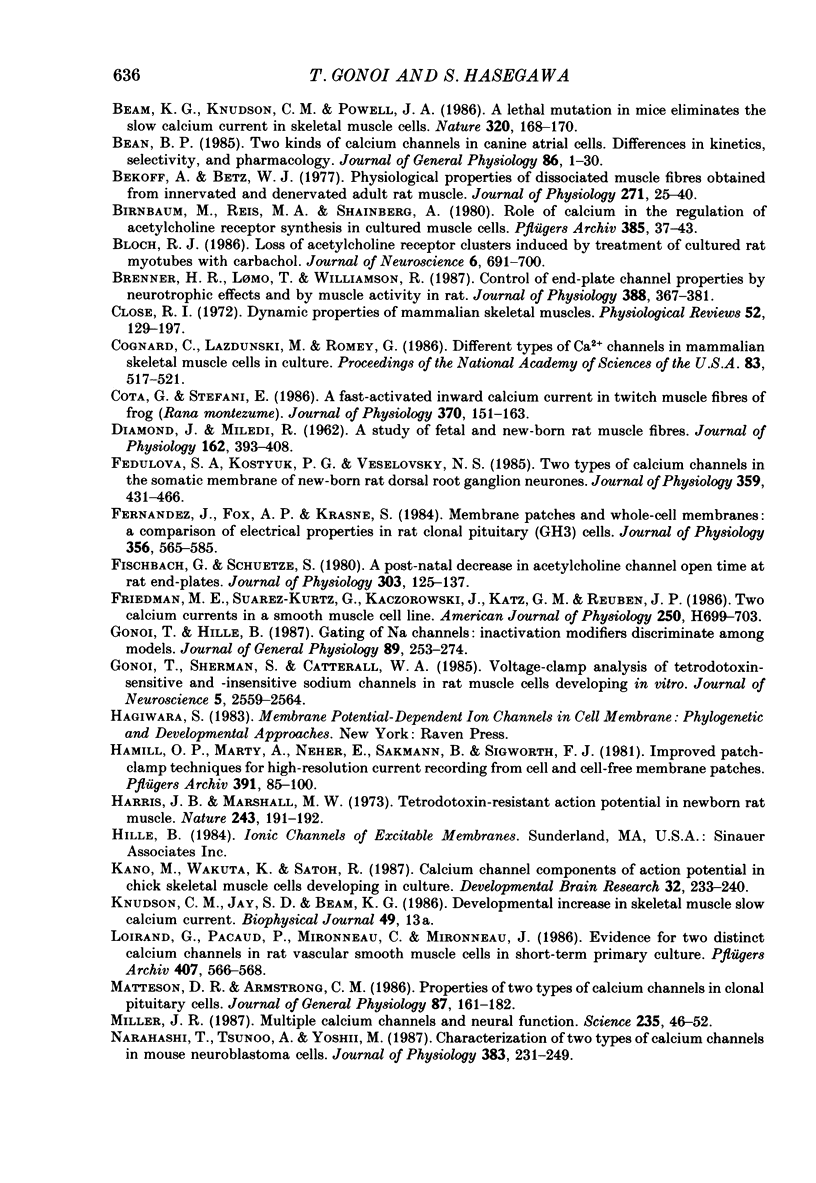
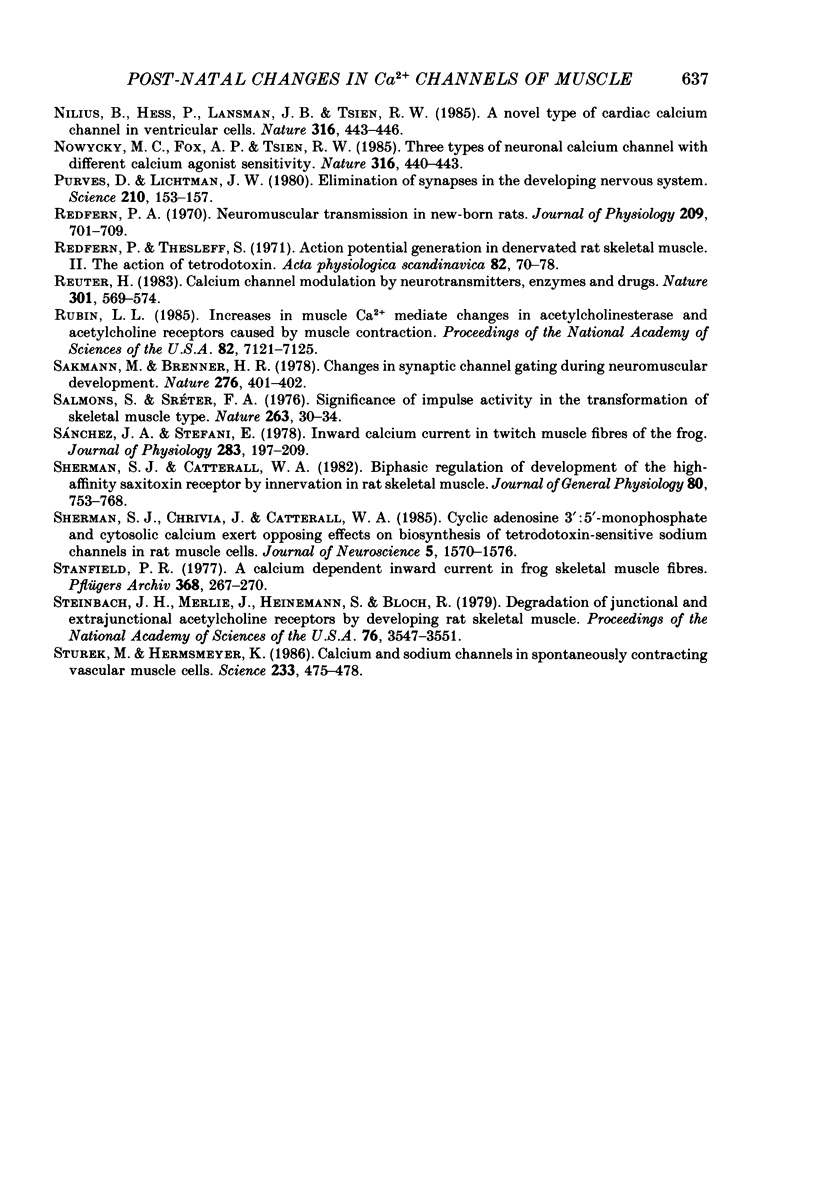
Selected References
These references are in PubMed. This may not be the complete list of references from this article.
- Almers W., Palade P. T. Slow calcium and potassium currents across frog muscle membrane: measurements with a vaseline-gap technique. J Physiol. 1981 Mar;312:159–176. doi: 10.1113/jphysiol.1981.sp013622. [DOI] [PMC free article] [PubMed] [Google Scholar]
- Armstrong D., Eckert R. Voltage-activated calcium channels that must be phosphorylated to respond to membrane depolarization. Proc Natl Acad Sci U S A. 1987 Apr;84(8):2518–2522. doi: 10.1073/pnas.84.8.2518. [DOI] [PMC free article] [PubMed] [Google Scholar]
- Arreola J., Calvo J., García M. C., Sánchez J. A. Modulation of calcium channels of twitch skeletal muscle fibres of the frog by adrenaline and cyclic adenosine monophosphate. J Physiol. 1987 Dec;393:307–330. doi: 10.1113/jphysiol.1987.sp016825. [DOI] [PMC free article] [PubMed] [Google Scholar]
- Beam K. G., Knudson C. M., Powell J. A. A lethal mutation in mice eliminates the slow calcium current in skeletal muscle cells. Nature. 1986 Mar 13;320(6058):168–170. doi: 10.1038/320168a0. [DOI] [PubMed] [Google Scholar]
- Bean B. P. Two kinds of calcium channels in canine atrial cells. Differences in kinetics, selectivity, and pharmacology. J Gen Physiol. 1985 Jul;86(1):1–30. doi: 10.1085/jgp.86.1.1. [DOI] [PMC free article] [PubMed] [Google Scholar]
- Bekoff A., Betz W. J. Physiological properties of dissociated muscle fibres obtained from innervated and denervated adult rat muscle. J Physiol. 1977 Sep;271(1):25–40. doi: 10.1113/jphysiol.1977.sp011988. [DOI] [PMC free article] [PubMed] [Google Scholar]
- Birnbaum M., Reis M. A., Shainberg A. Role of calcium in the regulation of acetylcholine receptor synthese in cultured muscle cells*. Pflugers Arch. 1980 May;385(1):37–43. doi: 10.1007/BF00583913. [DOI] [PubMed] [Google Scholar]
- Bloch R. J. Loss of acetylcholine receptor clusters induced by treatment of cultured rat myotubes with carbachol. J Neurosci. 1986 Mar;6(3):691–700. doi: 10.1523/JNEUROSCI.06-03-00691.1986. [DOI] [PMC free article] [PubMed] [Google Scholar]
- Brenner H. R., Lømo T., Williamson R. Control of end-plate channel properties by neurotrophic effects and by muscle activity in rat. J Physiol. 1987 Jul;388:367–381. doi: 10.1113/jphysiol.1987.sp016619. [DOI] [PMC free article] [PubMed] [Google Scholar]
- Close R. I. Dynamic properties of mammalian skeletal muscles. Physiol Rev. 1972 Jan;52(1):129–197. doi: 10.1152/physrev.1972.52.1.129. [DOI] [PubMed] [Google Scholar]
- Cognard C., Lazdunski M., Romey G. Different types of Ca2+ channels in mammalian skeletal muscle cells in culture. Proc Natl Acad Sci U S A. 1986 Jan;83(2):517–521. doi: 10.1073/pnas.83.2.517. [DOI] [PMC free article] [PubMed] [Google Scholar]
- Cota G., Stefani E. A fast-activated inward calcium current in twitch muscle fibres of the frog (Rana montezume). J Physiol. 1986 Jan;370:151–163. doi: 10.1113/jphysiol.1986.sp015927. [DOI] [PMC free article] [PubMed] [Google Scholar]
- DIAMOND J., MILEDI R. A study of foetal and new-born rat muscle fibres. J Physiol. 1962 Aug;162:393–408. doi: 10.1113/jphysiol.1962.sp006941. [DOI] [PMC free article] [PubMed] [Google Scholar]
- Fedulova S. A., Kostyuk P. G., Veselovsky N. S. Two types of calcium channels in the somatic membrane of new-born rat dorsal root ganglion neurones. J Physiol. 1985 Feb;359:431–446. doi: 10.1113/jphysiol.1985.sp015594. [DOI] [PMC free article] [PubMed] [Google Scholar]
- Fernandez J. M., Fox A. P., Krasne S. Membrane patches and whole-cell membranes: a comparison of electrical properties in rat clonal pituitary (GH3) cells. J Physiol. 1984 Nov;356:565–585. doi: 10.1113/jphysiol.1984.sp015483. [DOI] [PMC free article] [PubMed] [Google Scholar]
- Fischbach G. D., Schuetze S. M. A post-natal decrease in acetylcholine channel open time at rat end-plates. J Physiol. 1980 Jun;303:125–137. doi: 10.1113/jphysiol.1980.sp013275. [DOI] [PMC free article] [PubMed] [Google Scholar]
- Friedman M. E., Suarez-Kurtz G., Kaczorowski G. J., Katz G. M., Reuben J. P. Two calcium currents in a smooth muscle cell line. Am J Physiol. 1986 Apr;250(4 Pt 2):H699–H703. doi: 10.1152/ajpheart.1986.250.4.H699. [DOI] [PubMed] [Google Scholar]
- Gonoi T., Hille B. Gating of Na channels. Inactivation modifiers discriminate among models. J Gen Physiol. 1987 Feb;89(2):253–274. doi: 10.1085/jgp.89.2.253. [DOI] [PMC free article] [PubMed] [Google Scholar]
- Gonoi T., Sherman S. J., Catterall W. A. Voltage clamp analysis of tetrodotoxin-sensitive and -insensitive sodium channels in rat muscle cells developing in vitro. J Neurosci. 1985 Sep;5(9):2559–2564. doi: 10.1523/JNEUROSCI.05-09-02559.1985. [DOI] [PMC free article] [PubMed] [Google Scholar]
- Hamill O. P., Marty A., Neher E., Sakmann B., Sigworth F. J. Improved patch-clamp techniques for high-resolution current recording from cells and cell-free membrane patches. Pflugers Arch. 1981 Aug;391(2):85–100. doi: 10.1007/BF00656997. [DOI] [PubMed] [Google Scholar]
- Harris J. B., Marshall M. W. Tetrodotoxin-resistant action potentials in newborn rat muscle. Nat New Biol. 1973 Jun 6;243(127):191–192. doi: 10.1038/newbio243191a0. [DOI] [PubMed] [Google Scholar]
- Kano M., Wakuta K., Satoh R. Calcium channel components of action potential in chick skeletal muscle cells developing in culture. Brain Res. 1987 Apr;429(2):233–240. doi: 10.1016/0165-3806(87)90103-9. [DOI] [PubMed] [Google Scholar]
- Loirand G., Pacaud P., Mironneau C., Mironneau J. Evidence for two distinct calcium channels in rat vascular smooth muscle cells in short-term primary culture. Pflugers Arch. 1986 Nov;407(5):566–568. doi: 10.1007/BF00657519. [DOI] [PubMed] [Google Scholar]
- Matteson D. R., Armstrong C. M. Properties of two types of calcium channels in clonal pituitary cells. J Gen Physiol. 1986 Jan;87(1):161–182. doi: 10.1085/jgp.87.1.161. [DOI] [PMC free article] [PubMed] [Google Scholar]
- Miller R. J. Multiple calcium channels and neuronal function. Science. 1987 Jan 2;235(4784):46–52. doi: 10.1126/science.2432656. [DOI] [PubMed] [Google Scholar]
- Narahashi T., Tsunoo A., Yoshii M. Characterization of two types of calcium channels in mouse neuroblastoma cells. J Physiol. 1987 Feb;383:231–249. doi: 10.1113/jphysiol.1987.sp016406. [DOI] [PMC free article] [PubMed] [Google Scholar]
- Nilius B., Hess P., Lansman J. B., Tsien R. W. A novel type of cardiac calcium channel in ventricular cells. Nature. 1985 Aug 1;316(6027):443–446. doi: 10.1038/316443a0. [DOI] [PubMed] [Google Scholar]
- Nowycky M. C., Fox A. P., Tsien R. W. Three types of neuronal calcium channel with different calcium agonist sensitivity. Nature. 1985 Aug 1;316(6027):440–443. doi: 10.1038/316440a0. [DOI] [PubMed] [Google Scholar]
- Purves D., Lichtman J. W. Elimination of synapses in the developing nervous system. Science. 1980 Oct 10;210(4466):153–157. doi: 10.1126/science.7414326. [DOI] [PubMed] [Google Scholar]
- Redfern P. A. Neuromuscular transmission in new-born rats. J Physiol. 1970 Aug;209(3):701–709. doi: 10.1113/jphysiol.1970.sp009187. [DOI] [PMC free article] [PubMed] [Google Scholar]
- Redfern P., Thesleff S. Action potential generation in denervated rat skeletal muscle. II. The action of tetrodotoxin. Acta Physiol Scand. 1971 May;82(1):70–78. doi: 10.1111/j.1748-1716.1971.tb04943.x. [DOI] [PubMed] [Google Scholar]
- Reuter H. Calcium channel modulation by neurotransmitters, enzymes and drugs. Nature. 1983 Feb 17;301(5901):569–574. doi: 10.1038/301569a0. [DOI] [PubMed] [Google Scholar]
- Rubin L. L. Increases in muscle Ca2+ mediate changes in acetylcholinesterase and acetylcholine receptors caused by muscle contraction. Proc Natl Acad Sci U S A. 1985 Oct;82(20):7121–7125. doi: 10.1073/pnas.82.20.7121. [DOI] [PMC free article] [PubMed] [Google Scholar]
- Sakmann B., Brenner H. R. Change in synaptic channel gating during neuromuscular development. Nature. 1978 Nov 23;276(5686):401–402. doi: 10.1038/276401a0. [DOI] [PubMed] [Google Scholar]
- Salmons S., Sréter F. A. Significance of impulse activity in the transformation of skeletal muscle type. Nature. 1976 Sep 2;263(5572):30–34. doi: 10.1038/263030a0. [DOI] [PubMed] [Google Scholar]
- Sanchez J. A., Stefani E. Inward calcium current in twitch muscle fibres of the frog. J Physiol. 1978 Oct;283:197–209. doi: 10.1113/jphysiol.1978.sp012496. [DOI] [PMC free article] [PubMed] [Google Scholar]
- Sherman S. J., Catterall W. A. Biphasic regulation of development of the high-affinity saxitoxin receptor by innervation in rat skeletal muscle. J Gen Physiol. 1982 Nov;80(5):753–768. doi: 10.1085/jgp.80.5.753. [DOI] [PMC free article] [PubMed] [Google Scholar]
- Sherman S. J., Chrivia J., Catterall W. A. Cyclic adenosine 3':5'-monophosphate and cytosolic calcium exert opposing effects on biosynthesis of tetrodotoxin-sensitive sodium channels in rat muscle cells. J Neurosci. 1985 Jun;5(6):1570–1576. doi: 10.1523/JNEUROSCI.05-06-01570.1985. [DOI] [PMC free article] [PubMed] [Google Scholar]
- Stanfield P. R. A calcium dependent inward current in frog skeletal muscle fibres. Pflugers Arch. 1977 Apr 25;368(3):267–270. doi: 10.1007/BF00585206. [DOI] [PubMed] [Google Scholar]
- Steinbach J. H., Merlie J., Heinemann S., Bloch R. Degradation of junctional and extrajunctional acetylcholine receptors by developing rat skeletal muscle. Proc Natl Acad Sci U S A. 1979 Jul;76(7):3547–3551. doi: 10.1073/pnas.76.7.3547. [DOI] [PMC free article] [PubMed] [Google Scholar]
- Sturek M., Hermsmeyer K. Calcium and sodium channels in spontaneously contracting vascular muscle cells. Science. 1986 Jul 25;233(4762):475–478. doi: 10.1126/science.2425434. [DOI] [PubMed] [Google Scholar]