Abstract
1. Transmembrane potential (TMP) responses of Purkinje cells (PCs) in isolated turtle cerebellum to externally applied quasi-steady-state electric fields aligned with the dendritic axis were continuously measured using simultaneous intracellular and extracellular recording. TMP was obtained by subtraction of extracellular voltage fields from intracellular potential recorded at the same depth in the cerebellum. 2. The applied field changed the TMP with the polarity and amplitude dependent on the location on the PC membrane. This response at a given location increased linearly with external field up to a threshold level, beyond which active responses appeared. 3. The basic effect on TMP consisted of depolarization in the half of the dendrite towards which the fields were directed, and hyperpolarization in the other half. A pooled TMP depth-profile shows a steady increase in polarization from the middle of the molecular layer towards each end. This profile correlates with that predicted from previously proposed cable models, giving them empirical support for the first time. 4. Active responses were triggered by the field-induced depolarization. Tetrodotoxin (TTX)-sensitive action potentials arose with the primary depolarization in the somatic region. Notched, Ca2+-dependent action potentials arose with primary depolarization in the distal and mid-dendritic regions. 5. A TTX-sensitive voltage plateau was triggered by TMP-depolarization in the proximal region. It in turn activated Na+-spike trains. The frequency of spiking was proportional to the external field. At around 160 spikes/s, the Na+ spikes inactivated, and the TMP level rose to a more depolarized plateau. This latter plateau was also TTX-sensitive. 6. During depolarization of the distal dendritic region, sometimes a Ca2+-dependent plateau was observed. It appears to be associated with a small conductance increase. 7. Field-induced hyperpolarization suppressed local spiking and voltage plateaux, but remote Ca2+ spikes with reduced amplitude appeared in recordings from the proximal region. Similarly, in the distal region, low-amplitude, remote Na+ spikes and a Na+ plateau were observed superimposed on the hyperpolarizing baseline. The Na+ plateau apparently did not contribute to shunting of membrane currents in the distal dendrite. 8. The phase characteristics of the action potentials correlate with the modulation pattern noted in our extracellular study (Chan & Nicholson, 1986). Thus, the extracellular units ("giant spikes") were probably Na+ spikes activated in the soma and spread distally. Occasionally Ca2+ spikes, with a higher threshold, might also be activated to give dual-phase response.(ABSTRACT TRUNCATED AT 400 WORDS)
Full text
PDF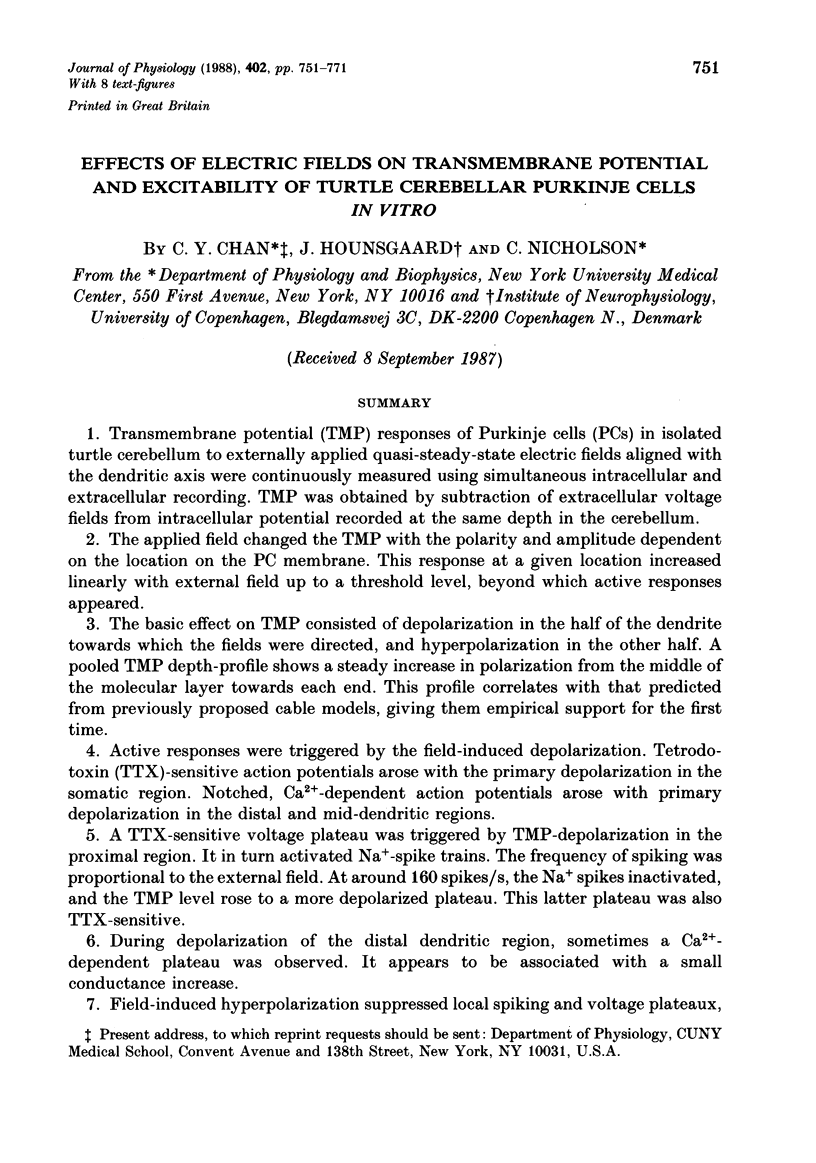
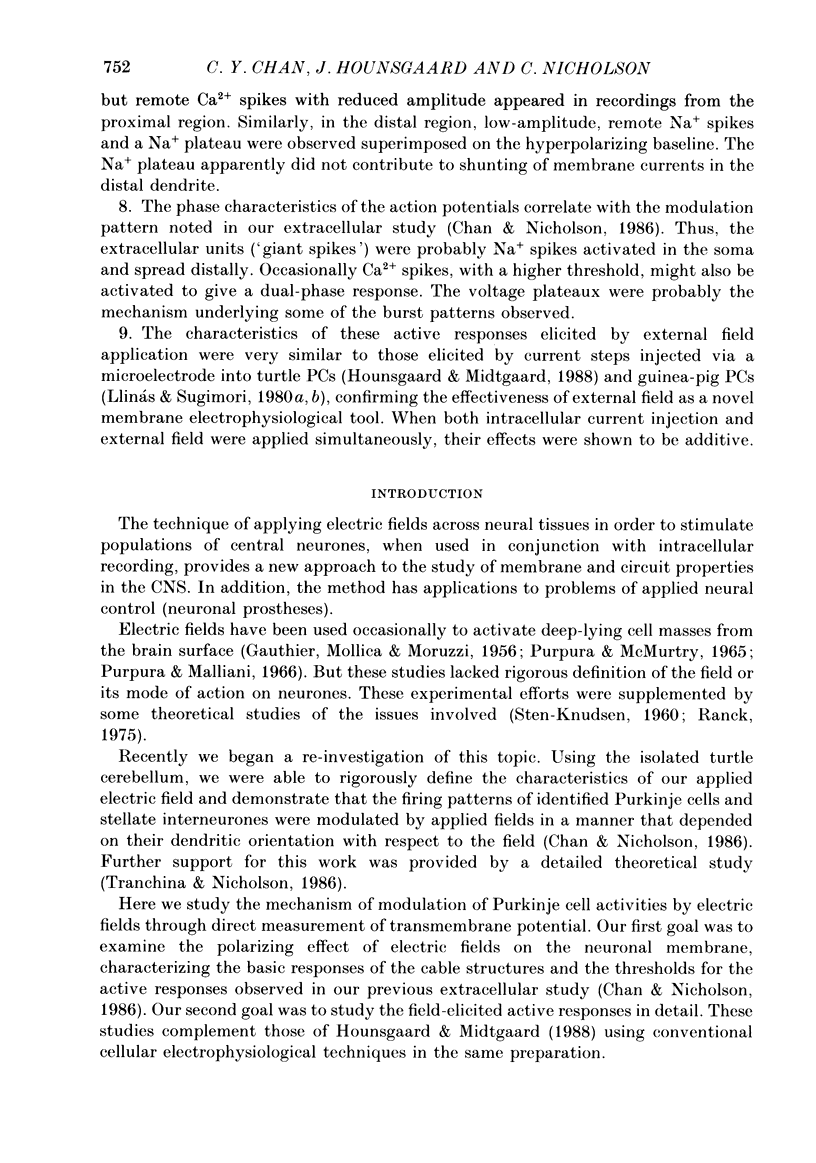
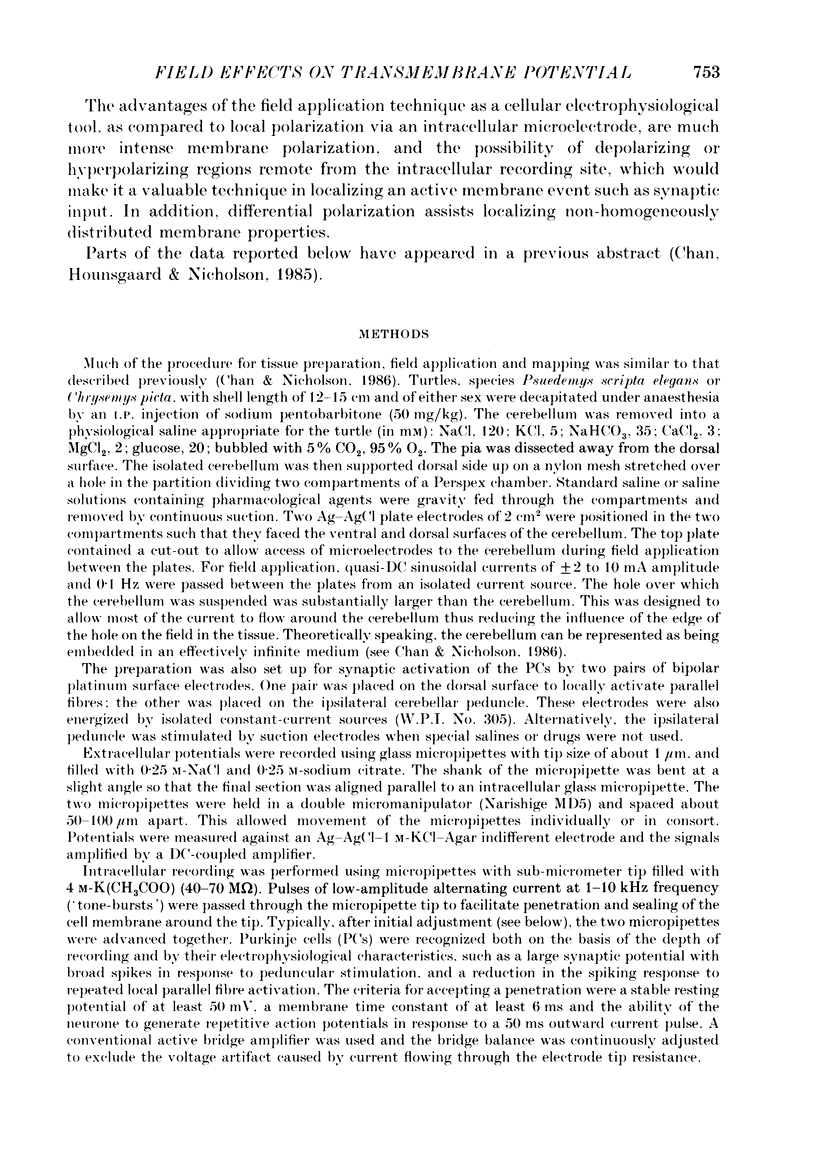
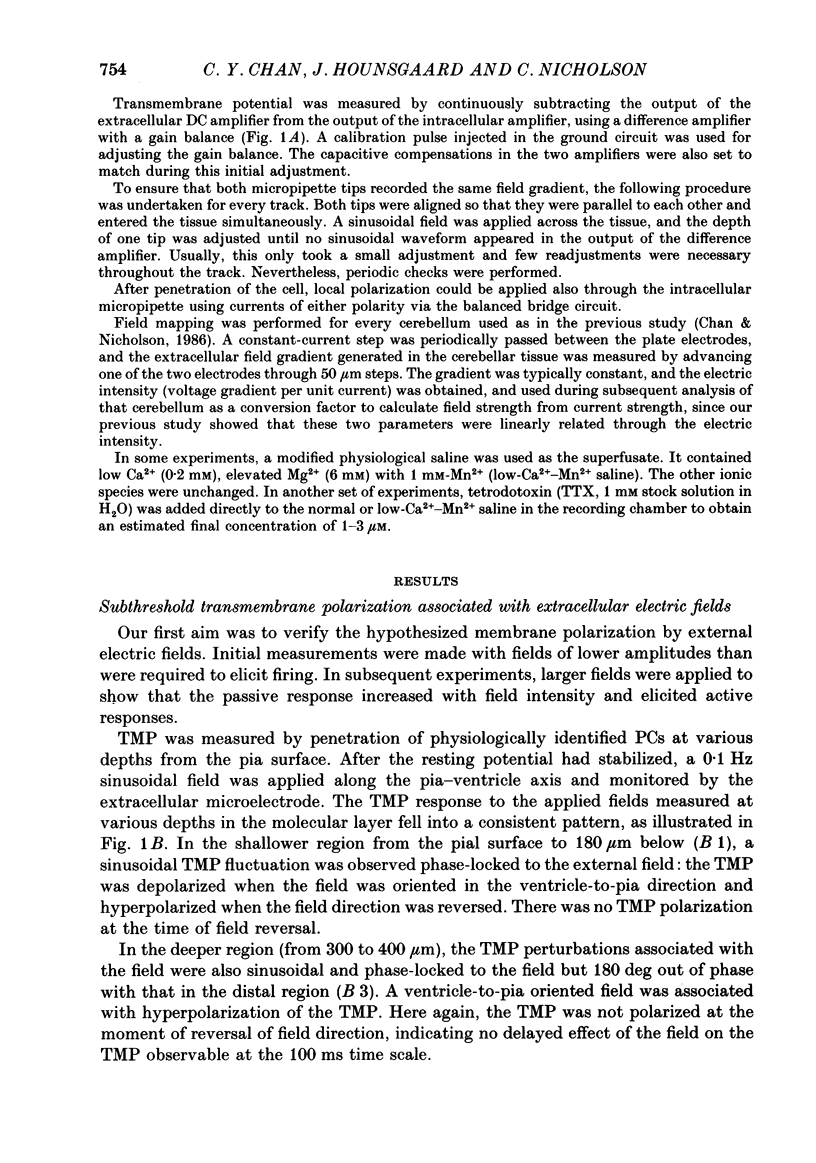
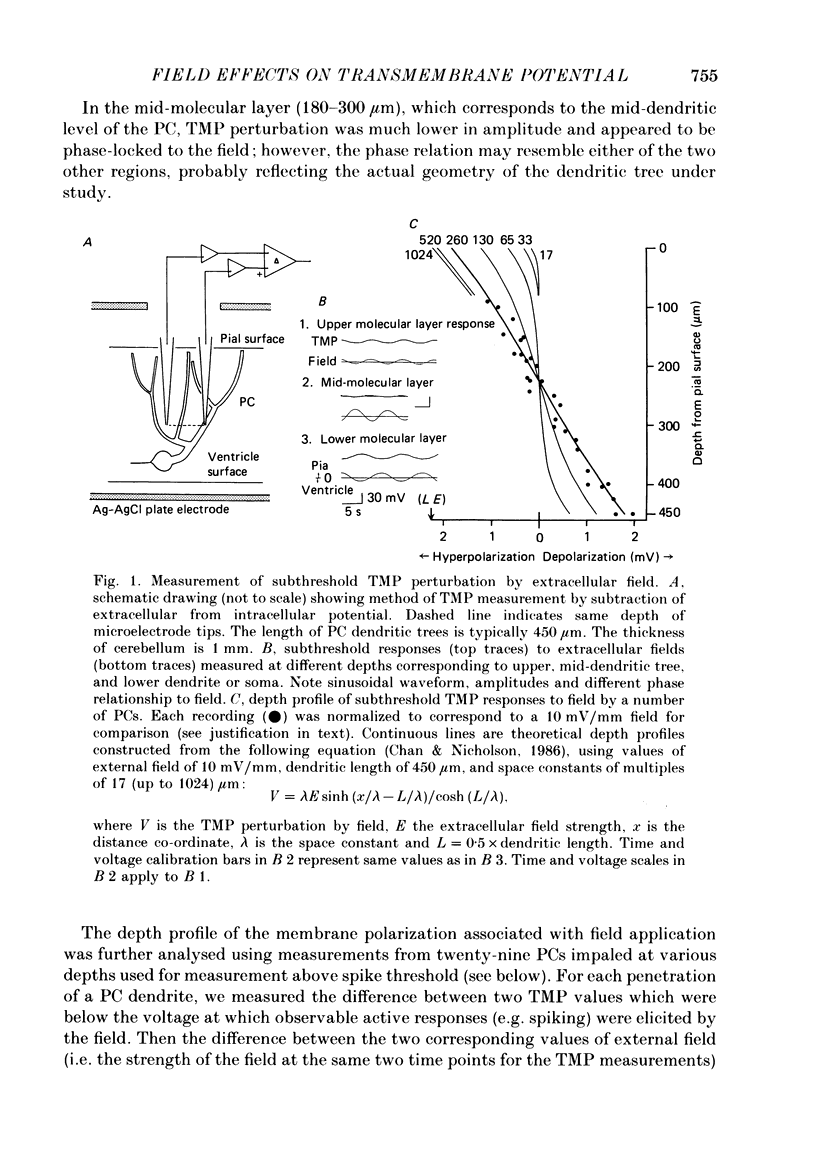
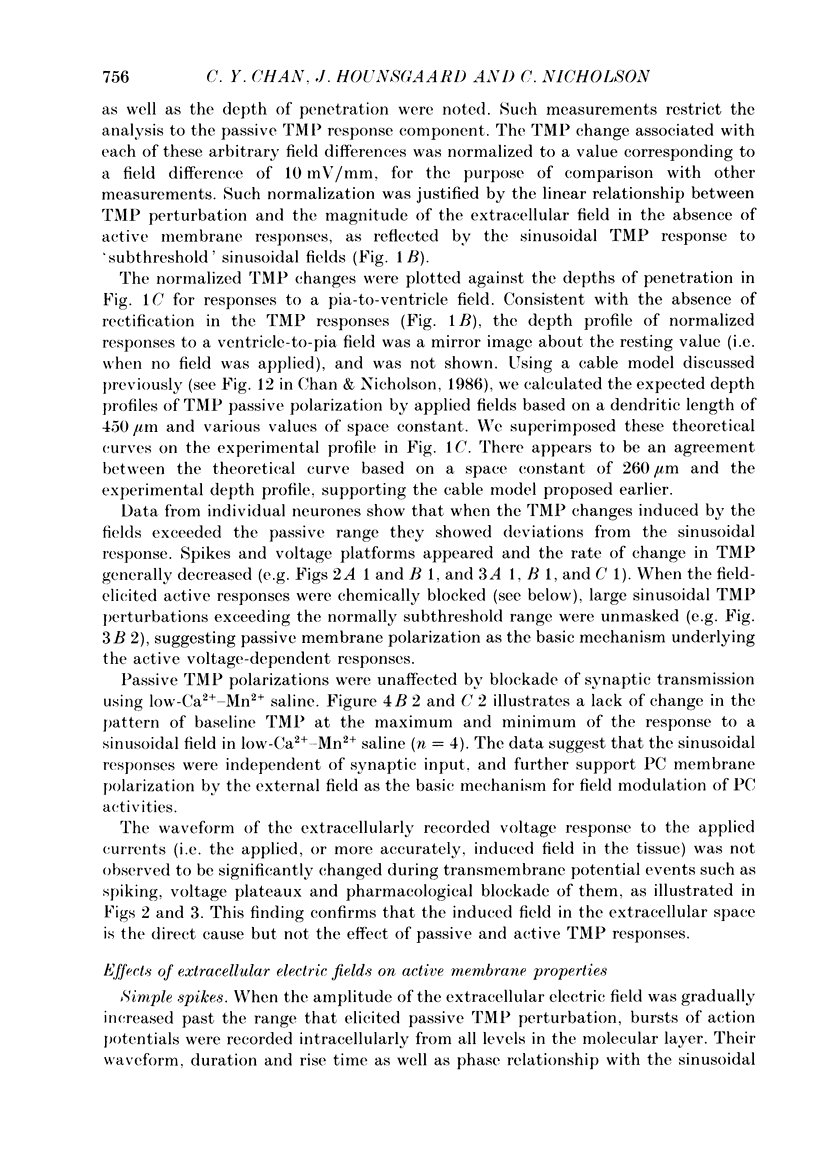
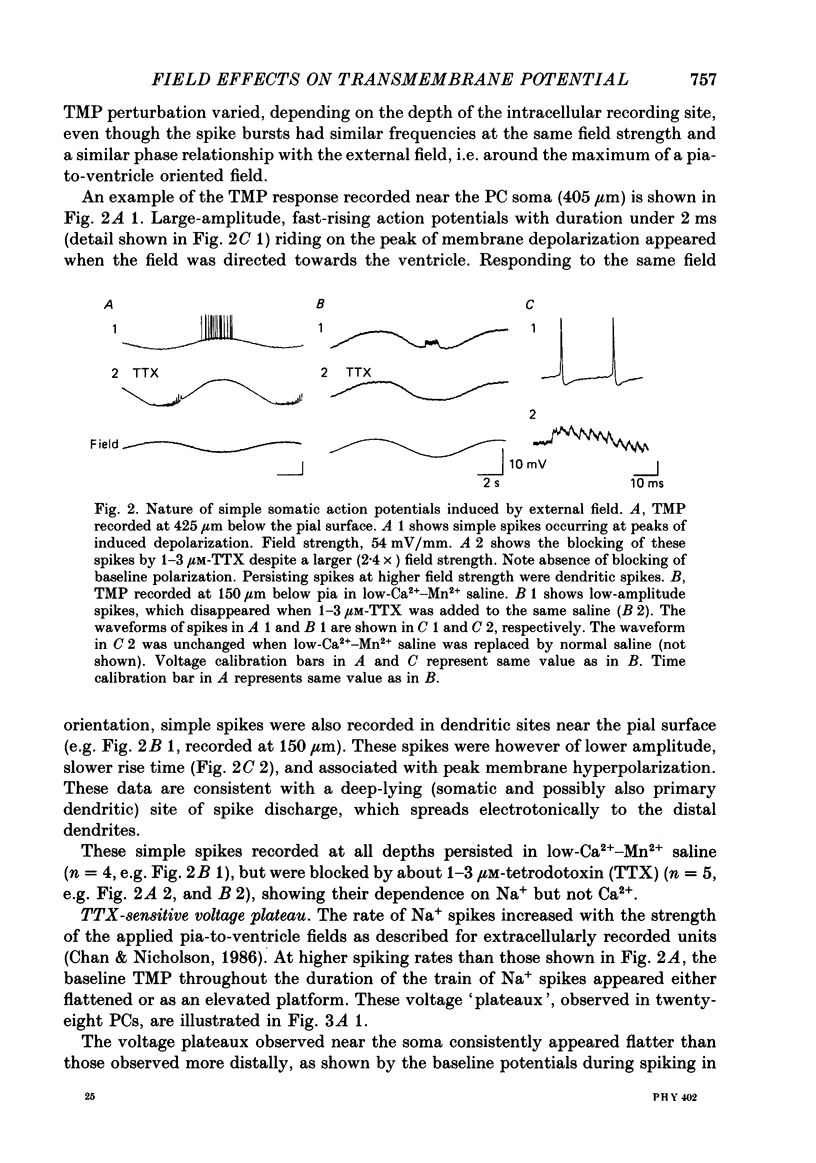
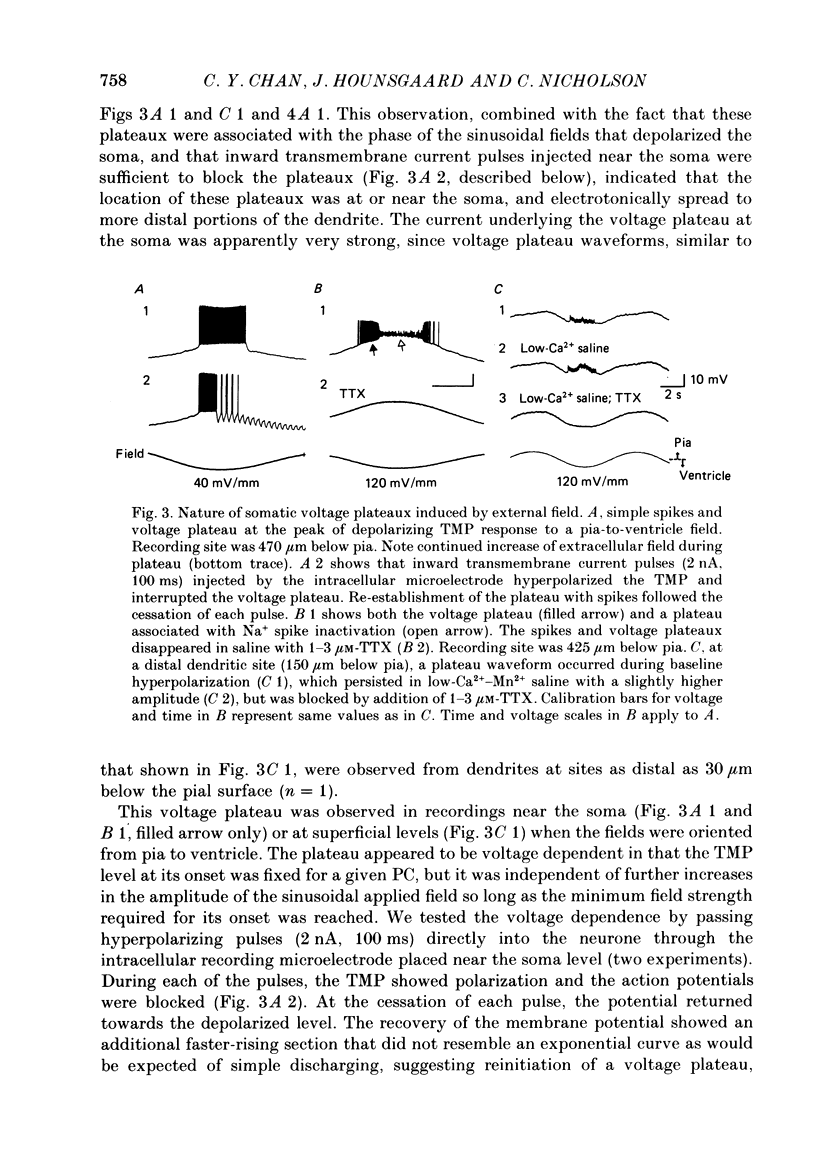
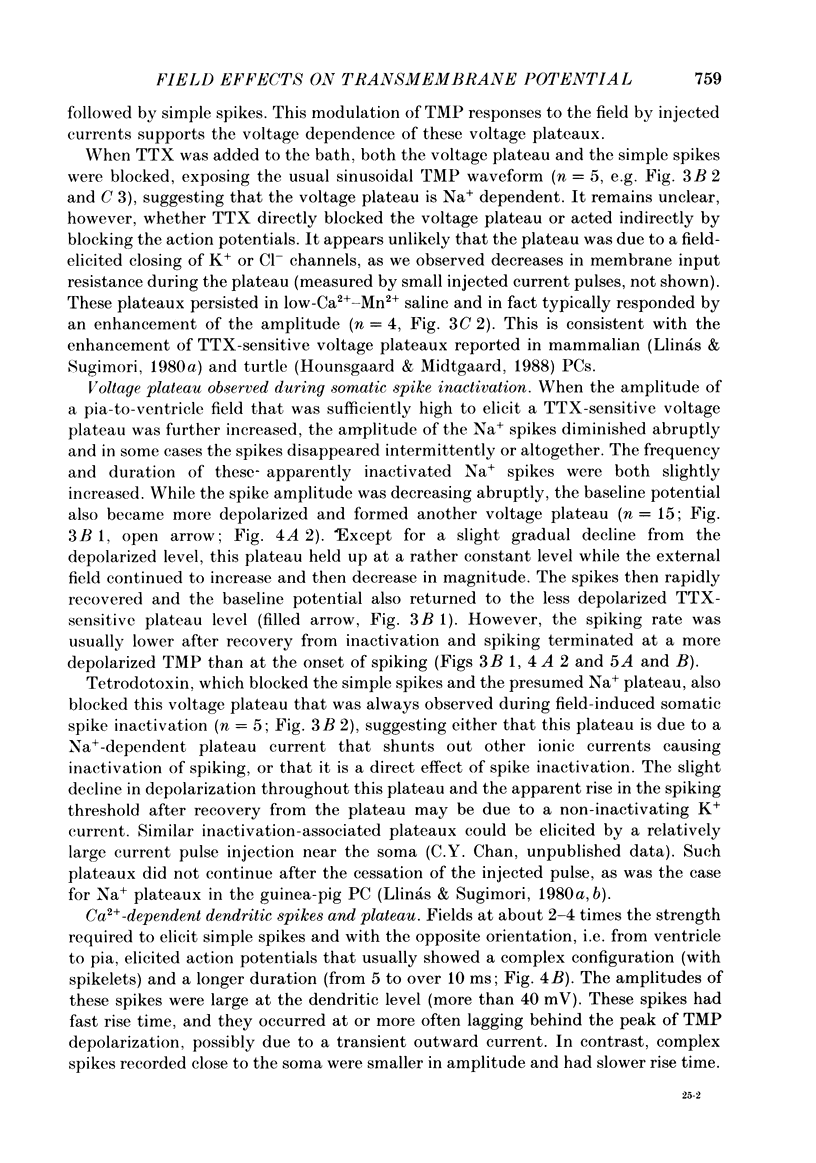
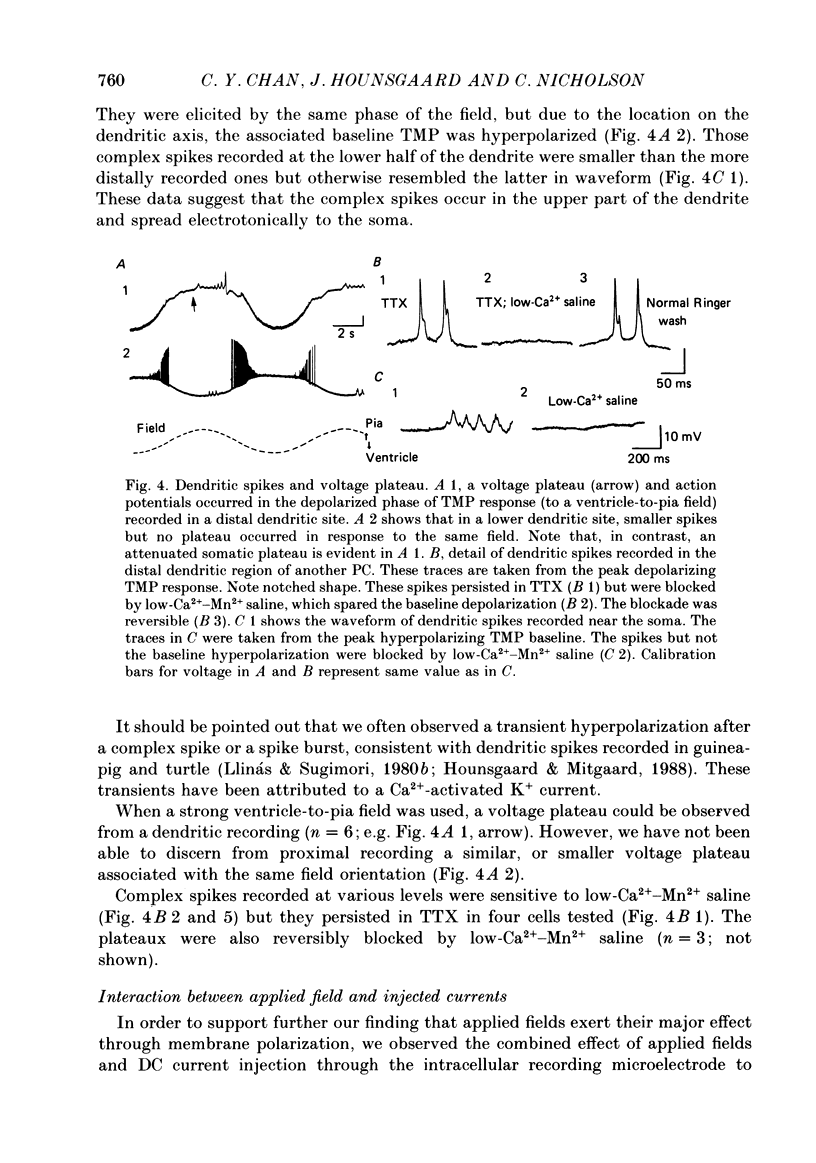
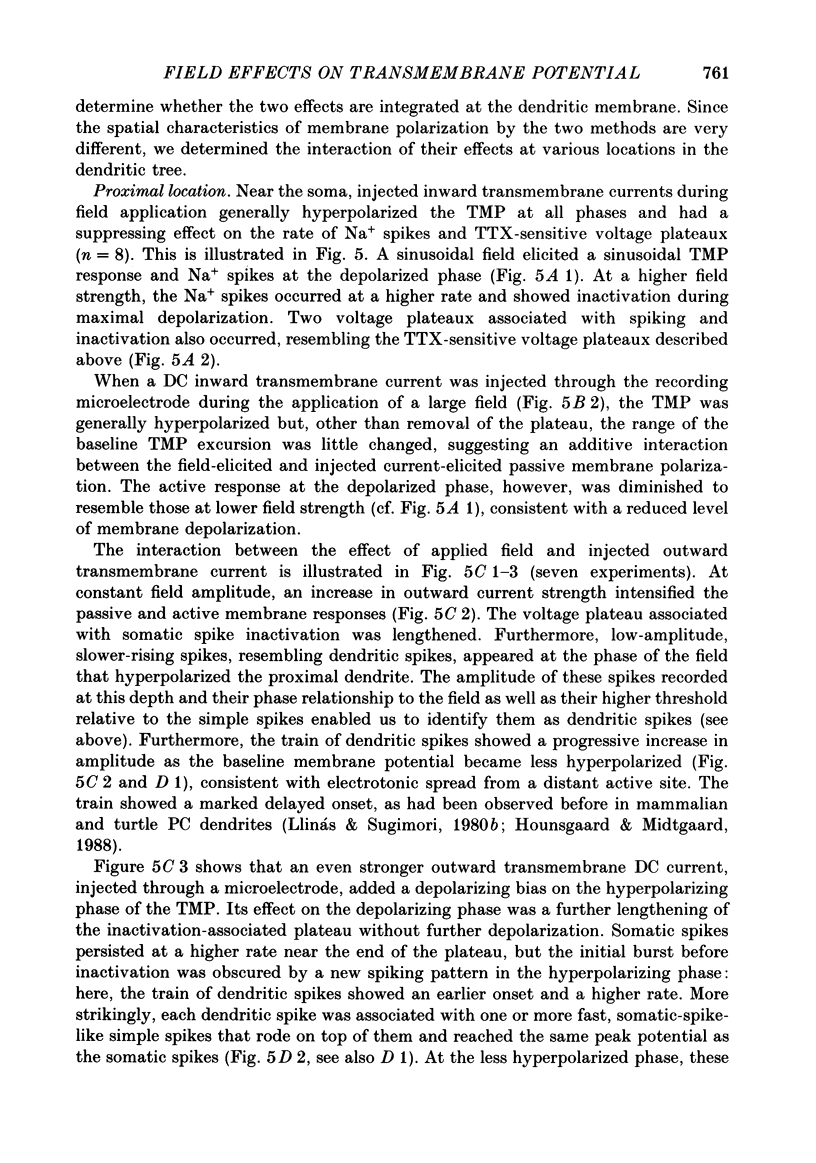
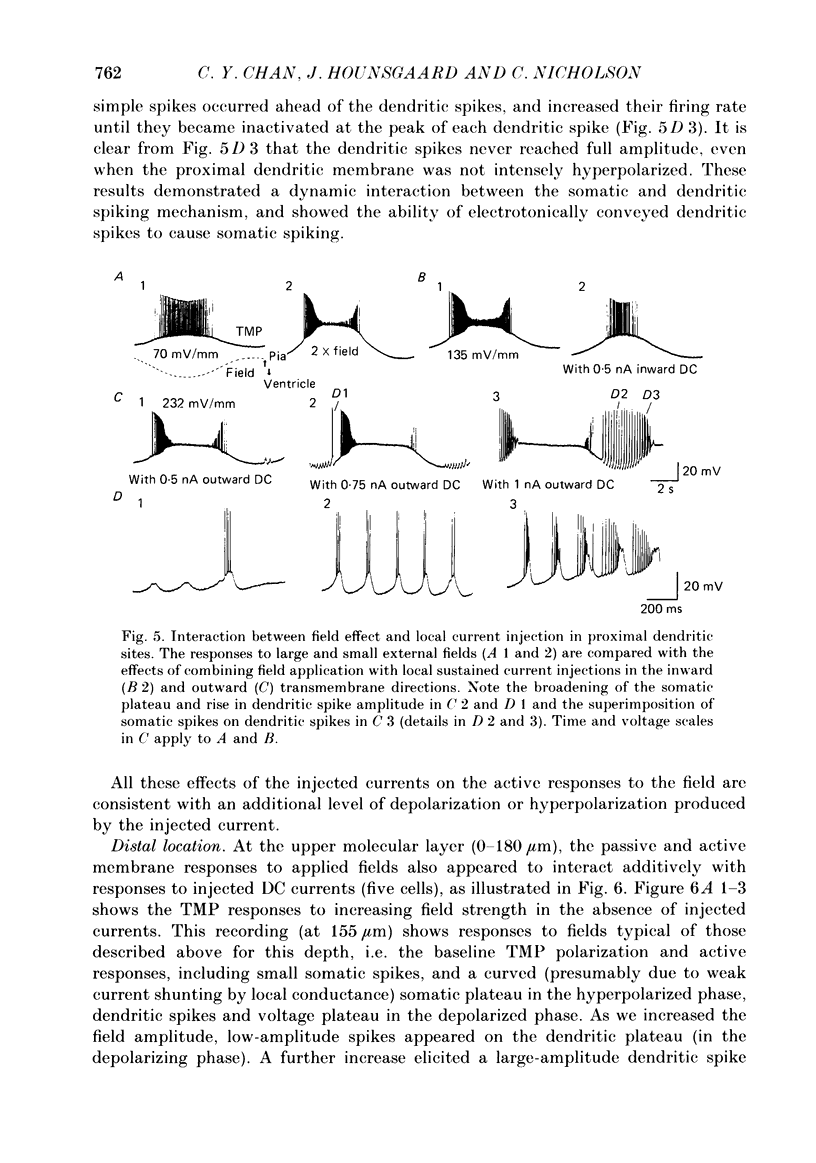
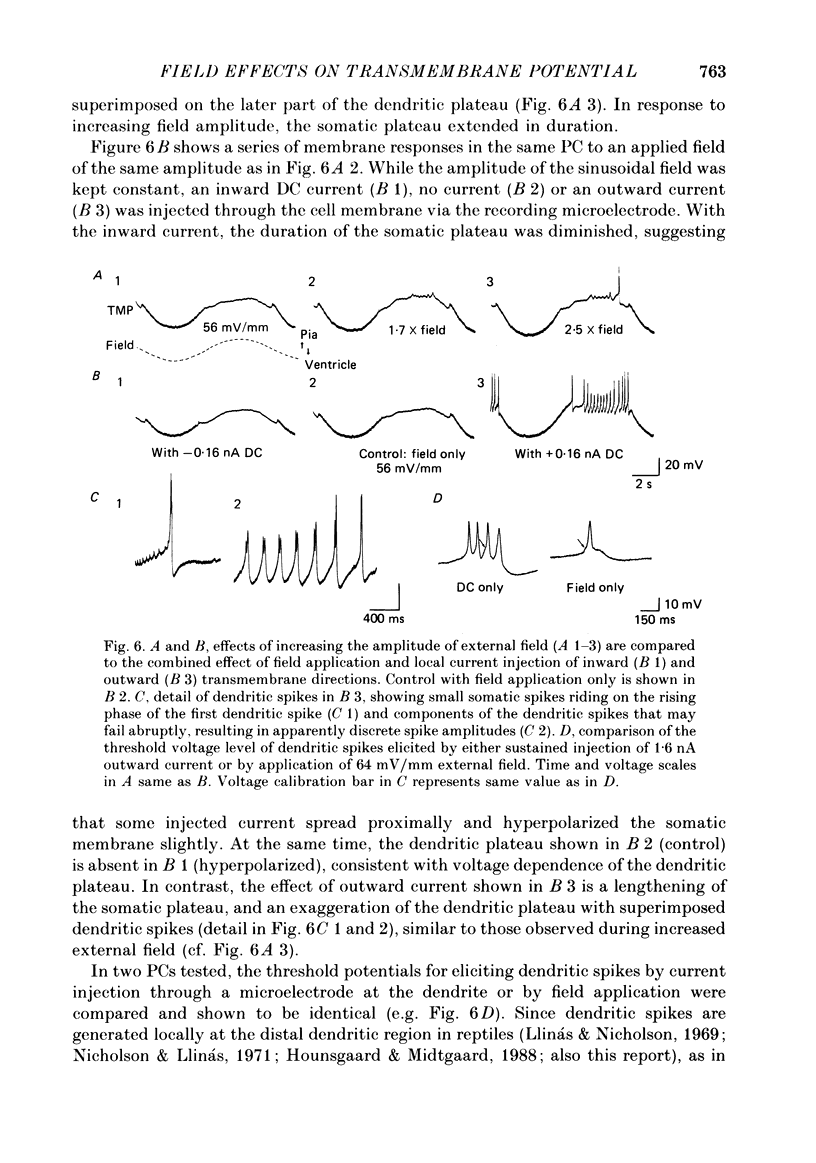
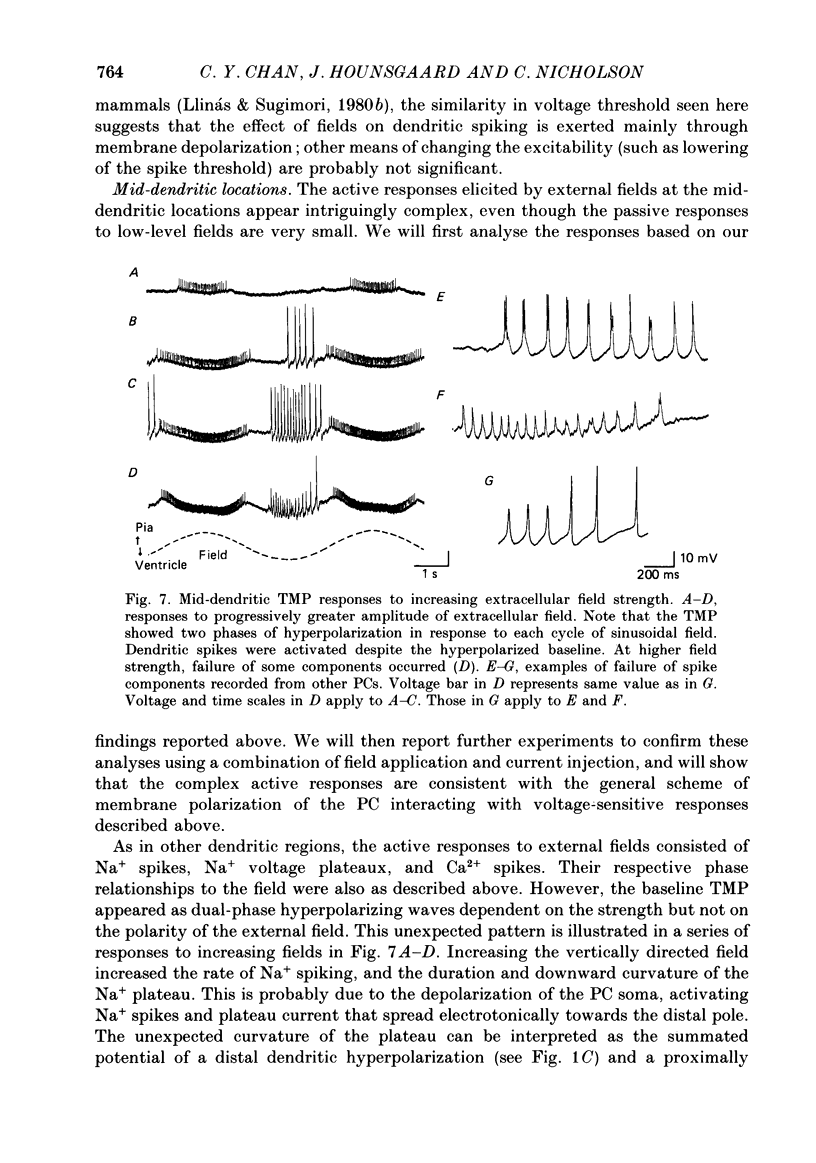
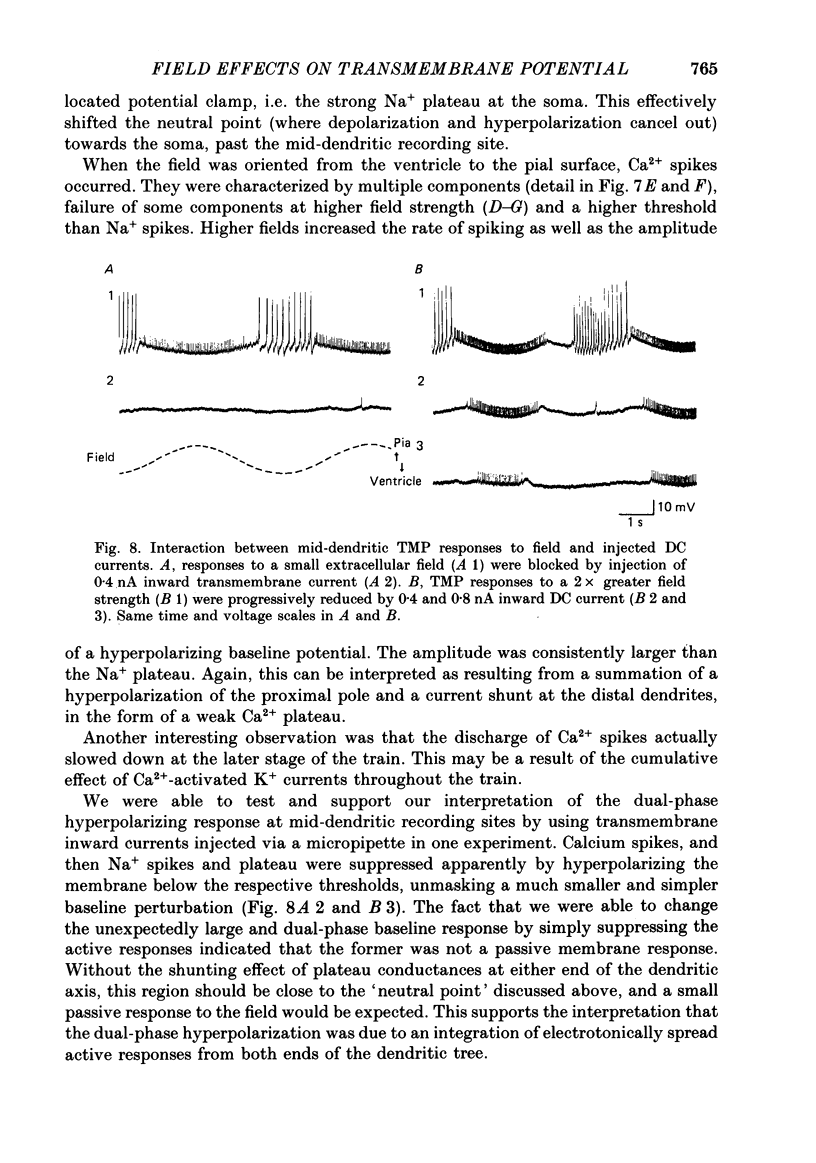
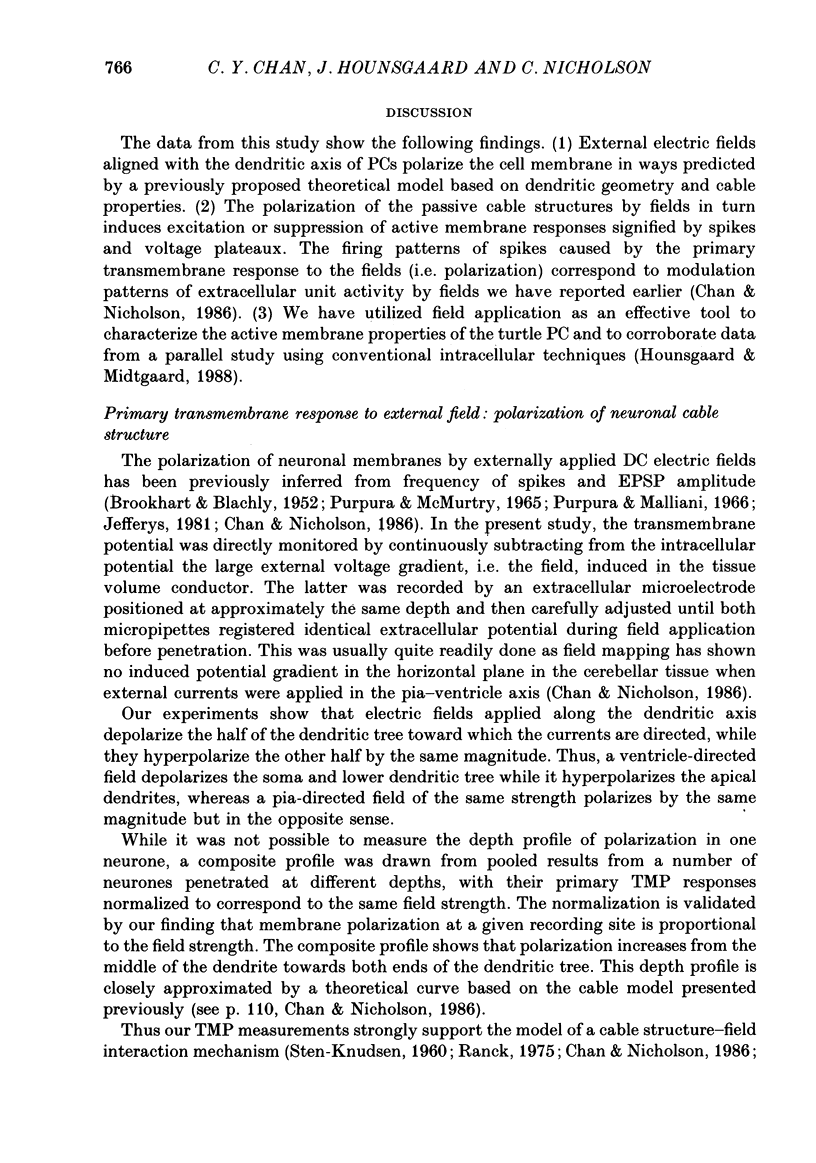
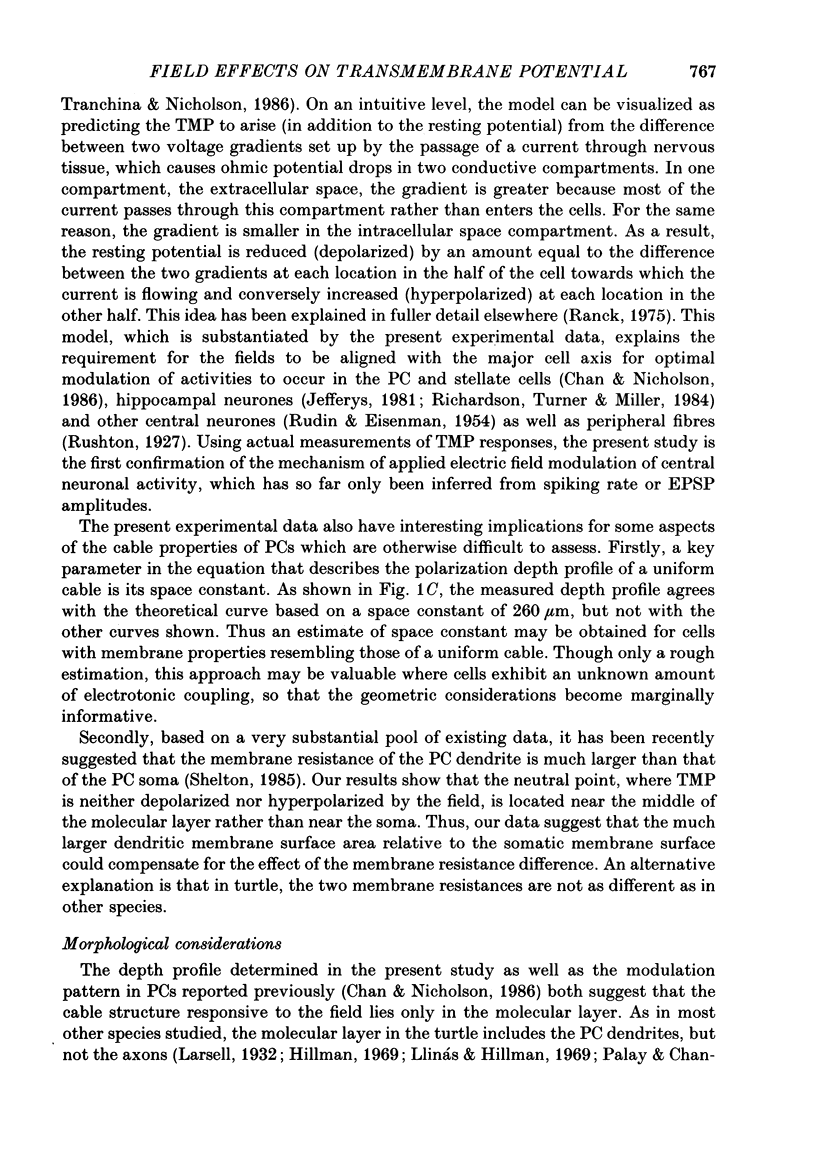
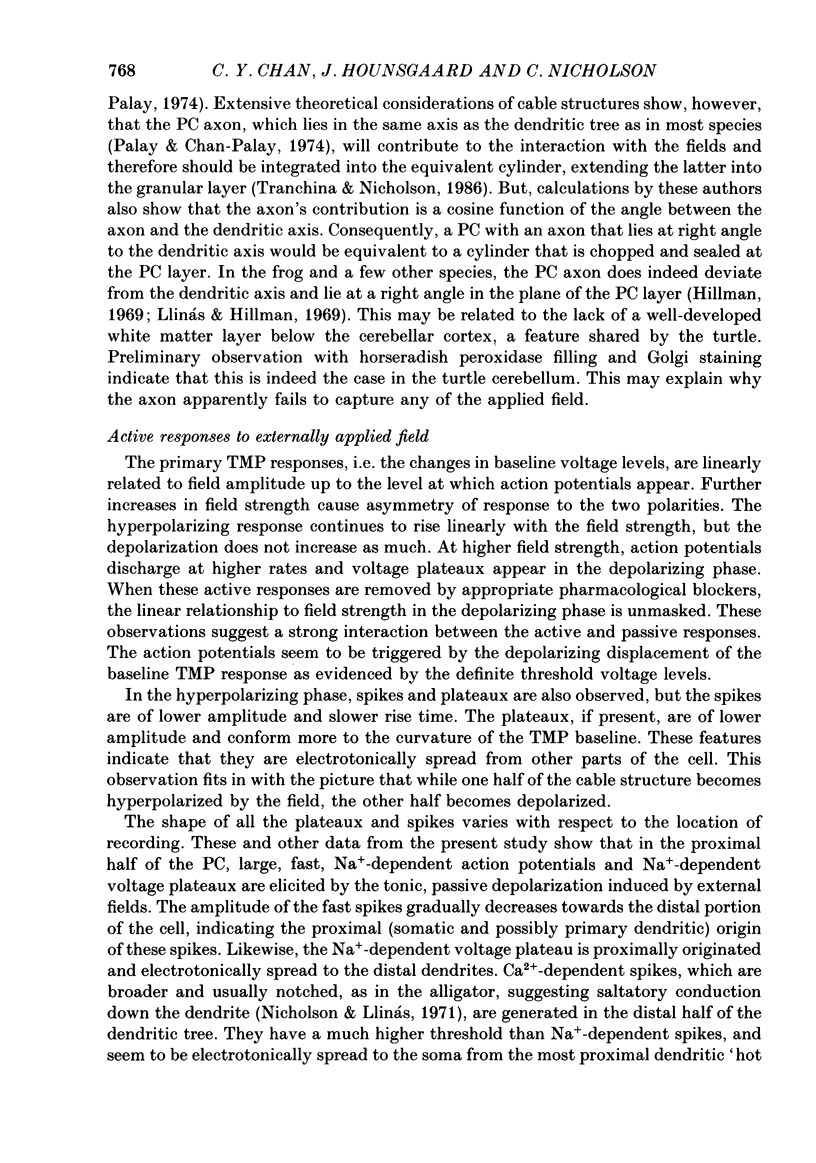
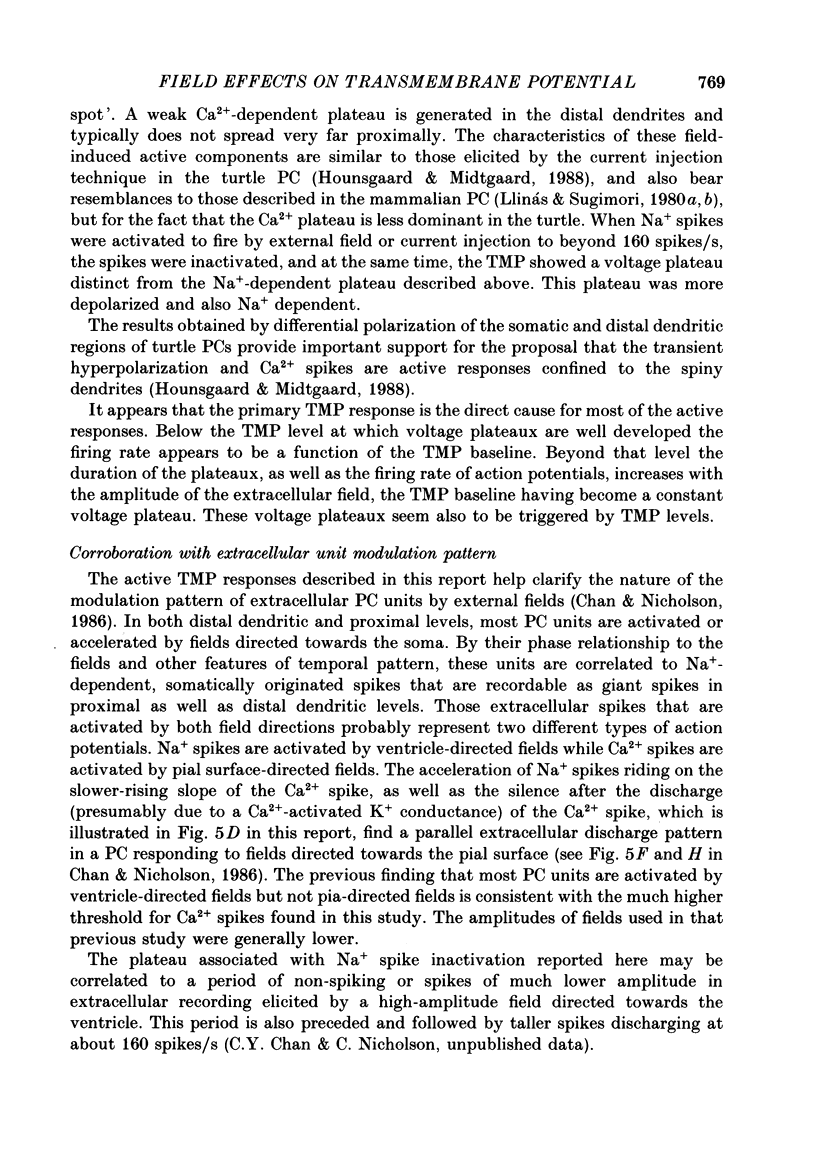
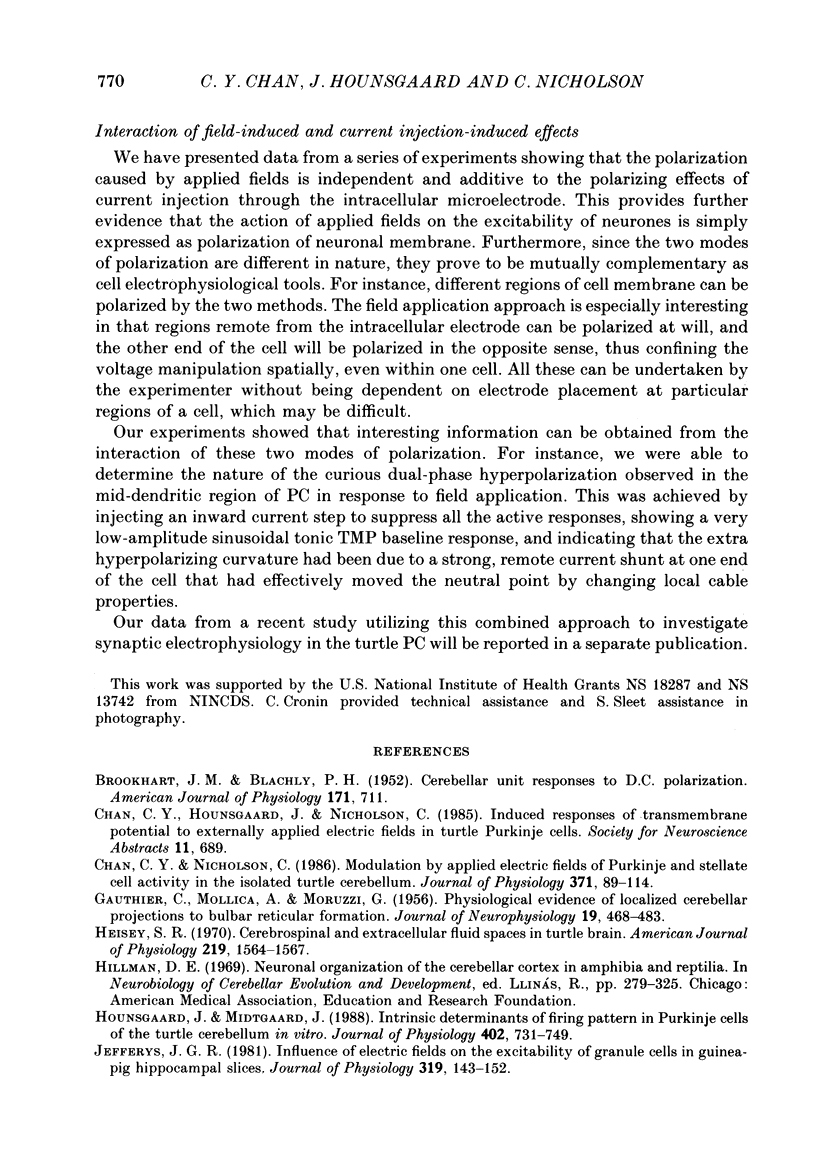
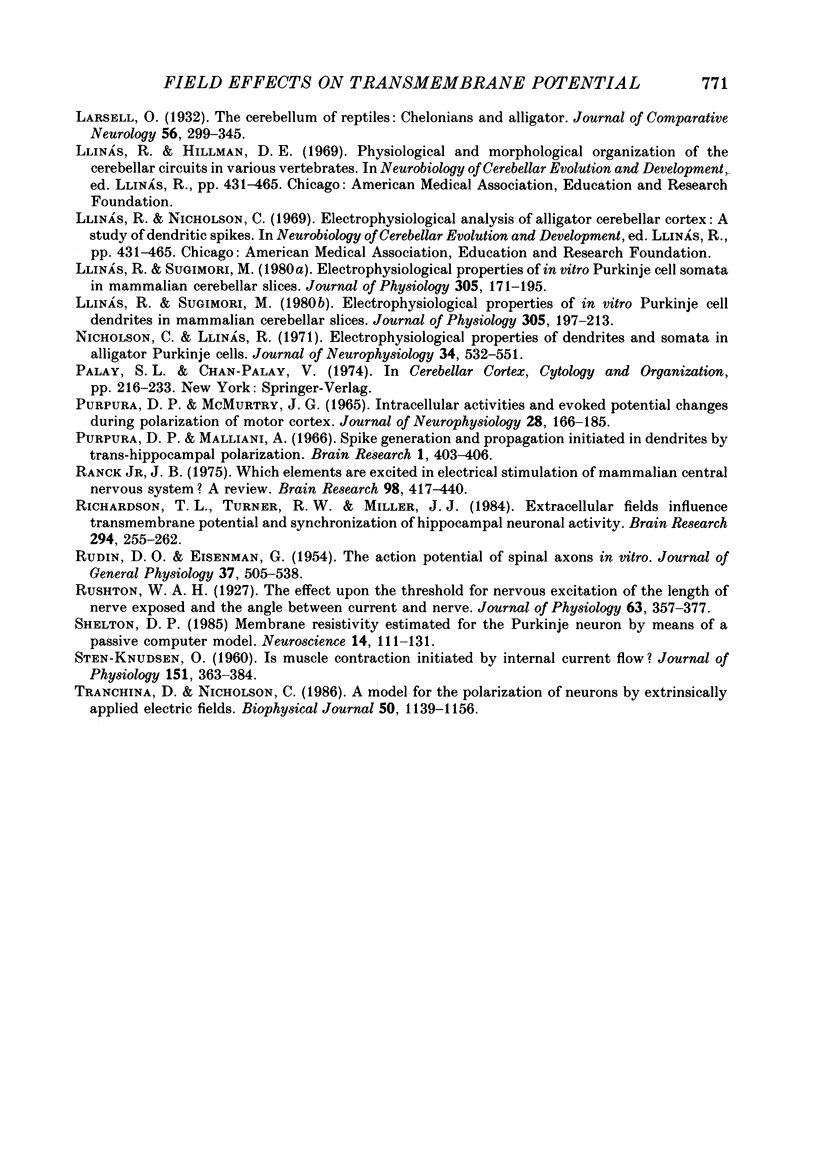
Selected References
These references are in PubMed. This may not be the complete list of references from this article.
- Chan C. Y., Nicholson C. Modulation by applied electric fields of Purkinje and stellate cell activity in the isolated turtle cerebellum. J Physiol. 1986 Feb;371:89–114. doi: 10.1113/jphysiol.1986.sp015963. [DOI] [PMC free article] [PubMed] [Google Scholar]
- GAUTHIER C., MOLLICA A., MORUZZI G. Physiological evidence of localized cerebellar projections to bulbar reticular formation. J Neurophysiol. 1956 Sep;19(5):468–483. doi: 10.1152/jn.1956.19.5.468. [DOI] [PubMed] [Google Scholar]
- Heisey S. R. Cerebrospinal and extracellular fluid spaces in turtle brain. Am J Physiol. 1970 Dec;219(6):1564–1567. doi: 10.1152/ajplegacy.1970.219.6.1564. [DOI] [PubMed] [Google Scholar]
- Hounsgaard J., Midtgaard J. Intrinsic determinants of firing pattern in Purkinje cells of the turtle cerebellum in vitro. J Physiol. 1988 Aug;402:731–749. doi: 10.1113/jphysiol.1988.sp017231. [DOI] [PMC free article] [PubMed] [Google Scholar]
- Jefferys J. G. Influence of electric fields on the excitability of granule cells in guinea-pig hippocampal slices. J Physiol. 1981;319:143–152. doi: 10.1113/jphysiol.1981.sp013897. [DOI] [PMC free article] [PubMed] [Google Scholar]
- Llinas R., Nicholson C. Electrophysiological properties of dendrites and somata in alligator Purkinje cells. J Neurophysiol. 1971 Jul;34(4):532–551. doi: 10.1152/jn.1971.34.4.532. [DOI] [PubMed] [Google Scholar]
- Llinás R., Sugimori M. Electrophysiological properties of in vitro Purkinje cell dendrites in mammalian cerebellar slices. J Physiol. 1980 Aug;305:197–213. doi: 10.1113/jphysiol.1980.sp013358. [DOI] [PMC free article] [PubMed] [Google Scholar]
- Llinás R., Sugimori M. Electrophysiological properties of in vitro Purkinje cell somata in mammalian cerebellar slices. J Physiol. 1980 Aug;305:171–195. doi: 10.1113/jphysiol.1980.sp013357. [DOI] [PMC free article] [PubMed] [Google Scholar]
- PURPURA D. P., MCMURTRY J. G. INTRACELLULAR ACTIVITIES AND EVOKED POTENTIAL CHANGES DURING POLARIZATION OF MOTOR CORTEX. J Neurophysiol. 1965 Jan;28:166–185. doi: 10.1152/jn.1965.28.1.166. [DOI] [PubMed] [Google Scholar]
- Purpura D. P., Malliani A. Spike generation and propagation initiated in dendrites by transhippocampal polarization. Brain Res. 1966 May-Jun;1(4):403–406. doi: 10.1016/0006-8993(66)90132-6. [DOI] [PubMed] [Google Scholar]
- RUDIN D. O., EISENMAN G. The action potential of spinal axons in vitro. J Gen Physiol. 1954 Mar;37(4):505–538. doi: 10.1085/jgp.37.4.505. [DOI] [PMC free article] [PubMed] [Google Scholar]
- Ranck J. B., Jr Which elements are excited in electrical stimulation of mammalian central nervous system: a review. Brain Res. 1975 Nov 21;98(3):417–440. doi: 10.1016/0006-8993(75)90364-9. [DOI] [PubMed] [Google Scholar]
- Richardson T. L., Turner R. W., Miller J. J. Extracellular fields influence transmembrane potentials and synchronization of hippocampal neuronal activity. Brain Res. 1984 Mar 5;294(2):255–262. doi: 10.1016/0006-8993(84)91037-0. [DOI] [PubMed] [Google Scholar]
- Rushton W. A. The effect upon the threshold for nervous excitation of the length of nerve exposed, and the angle between current and nerve. J Physiol. 1927 Sep 9;63(4):357–377. doi: 10.1113/jphysiol.1927.sp002409. [DOI] [PMC free article] [PubMed] [Google Scholar]
- STEN-KNUDSEN O. Is muscle contraction initiated by internal current flow? J Physiol. 1960 May;151:363–384. doi: 10.1113/jphysiol.1960.sp006444. [DOI] [PMC free article] [PubMed] [Google Scholar]
- Shelton D. P. Membrane resistivity estimated for the Purkinje neuron by means of a passive computer model. Neuroscience. 1985 Jan;14(1):111–131. doi: 10.1016/0306-4522(85)90168-x. [DOI] [PubMed] [Google Scholar]
- Tranchina D., Nicholson C. A model for the polarization of neurons by extrinsically applied electric fields. Biophys J. 1986 Dec;50(6):1139–1156. doi: 10.1016/S0006-3495(86)83558-5. [DOI] [PMC free article] [PubMed] [Google Scholar]