Abstract
1. The slowly decaying, calcium-dependent after-hyperpolarization (a.h.p.) that follows action potentials in bullfrog ganglion B cells has previously been shown to be generated by a potassium current called IAHP. We have recorded IAHP using a switched, single-electrode hybrid clamp where current-clamp mode was changed to voltage-clamp mode immediately after repolarization of a spike or the last spike of a train. 2. Reduction of extracellular calcium reduced the decay time of IAHP following a single spike. At all levels of extracellular calcium tested (0.5-4 mM), the decay time of IAHP was longer following a train of action potentials than following a single action potential. Thus, the time course of IAHP evoked by action potentials is a function of the calcium load induced by the action potentials. Conversely, agents that reduce the amount of IAHP activated without affecting its rate of decay, probably do not affect calcium influx. 3. Muscarine (2 or 10 microM) inhibits IAHP following an action potential by at most 30% and has no effect on decay rate of IAHP. These results suggest that muscarine has little or no effect on either calcium influx or sequestration. Decay of the a.h.p. is accelerated by muscarine but this effect is due to an increased leak conductance. 4. Charybdotoxin (CTX) between 4 and 20 nM, prolongs action potential duration in a manner consistent with blockade of the voltage- and calcium-dependent potassium current (Ic) involved in spike repolarization in these cells. This action is consistent with its reported action on analogous channels in other systems. However, CTX also reduces IAHP. Thus, in bullfrog ganglion neurones, two distinct calcium-dependent potassium currents exhibit a comparable sensitivity to CTX. This cannot be due to a decreased influx of calcium because the decay rate of IAHP following an action potential is unchanged. The action of CTX was observed with both crude and purified preparations of CTX. 5. Apamin (25 nM) and (+)-tubocurarine (concentration giving 50% of maximal inhibition = 20 microM) block IAHP without affecting action potential duration. The action of (+)-tubocurarine is more readily reversible than apamin. Approximately 20% of IAHP is resistant to blockade by either apamin or (+)-tubocurarine. 6. Muscarine was used to block the M-current (IM) selectively and (+)-tubocurarine was used to inhibit IAHP selectively. Both currents were shown to contribute to spike frequency adaptation. Inhibition of both IM and IAHP has a synergistic action to increase repetitive firing.
Full text
PDF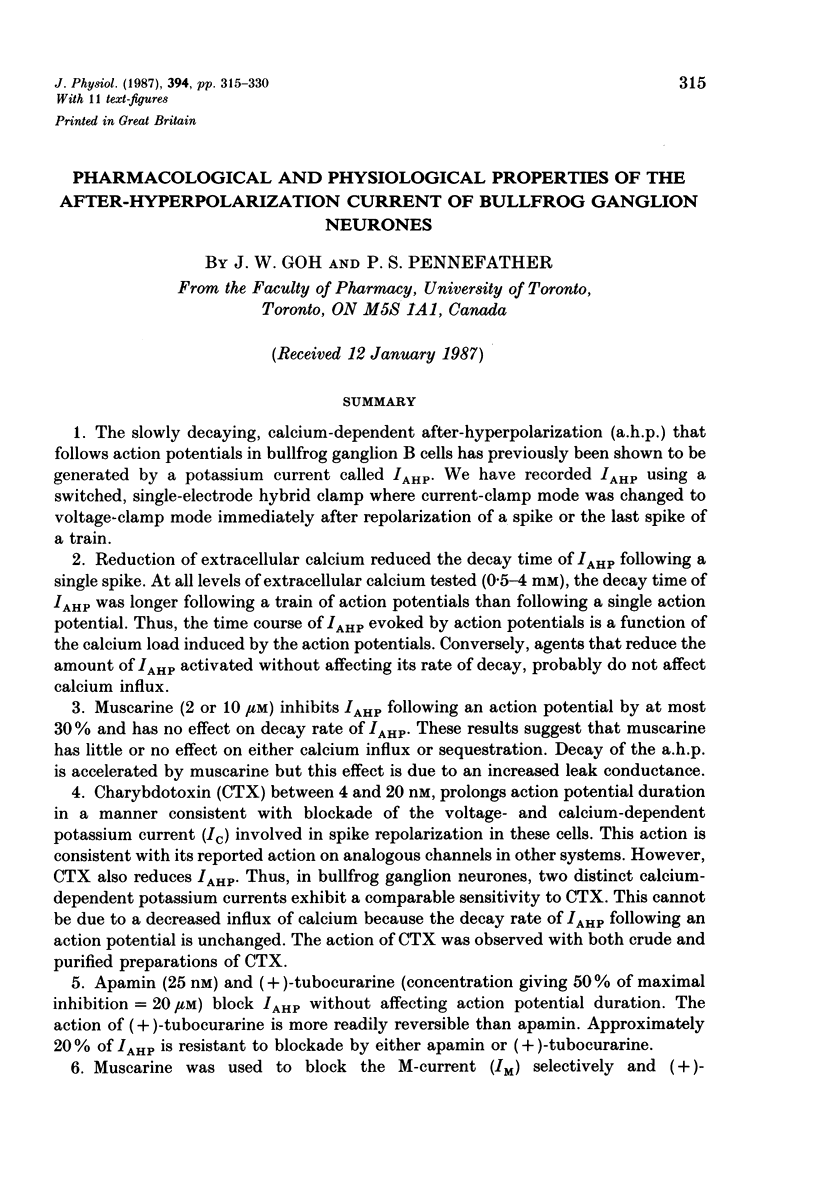
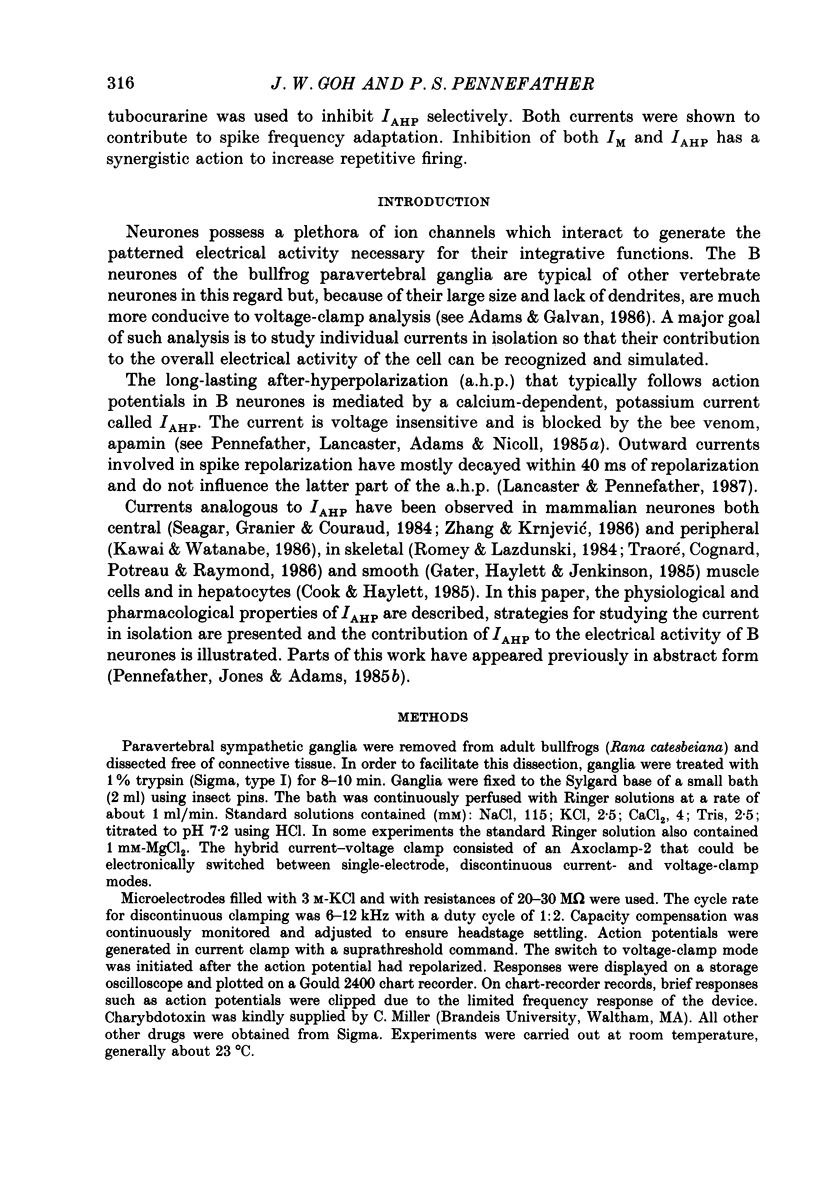
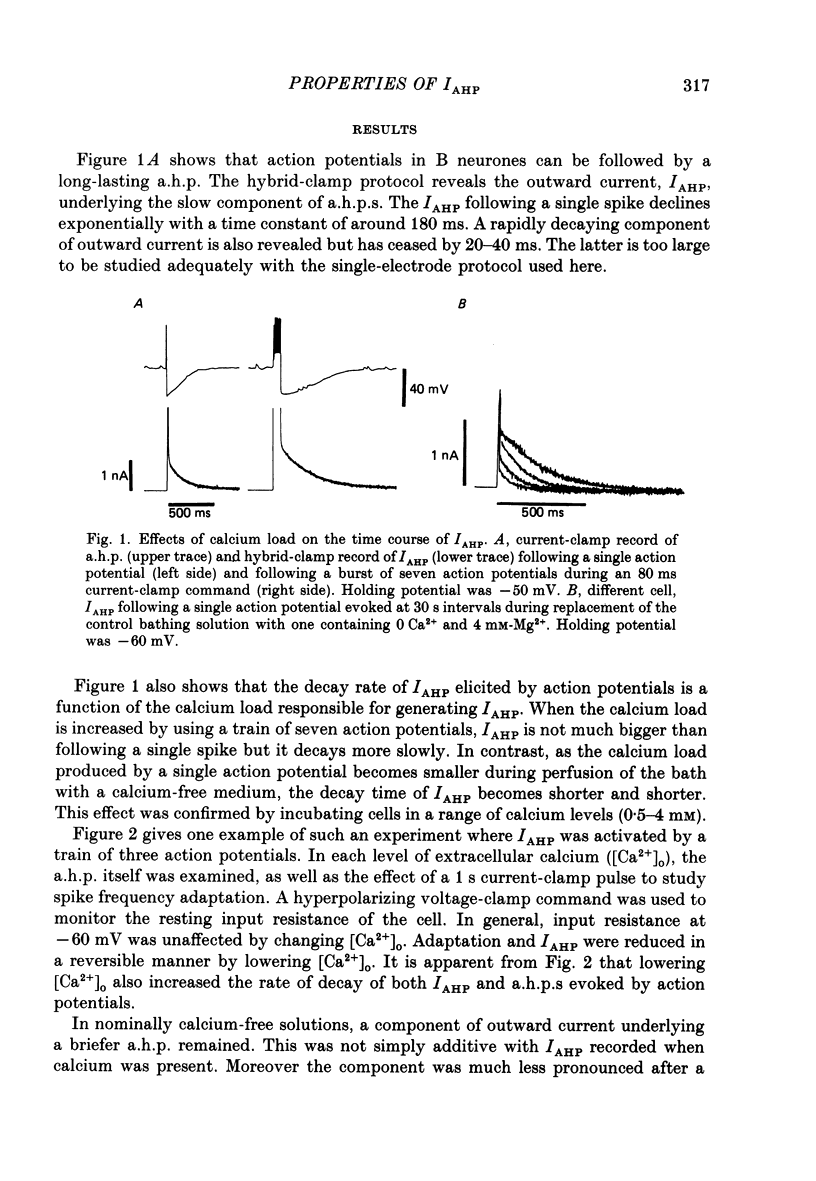
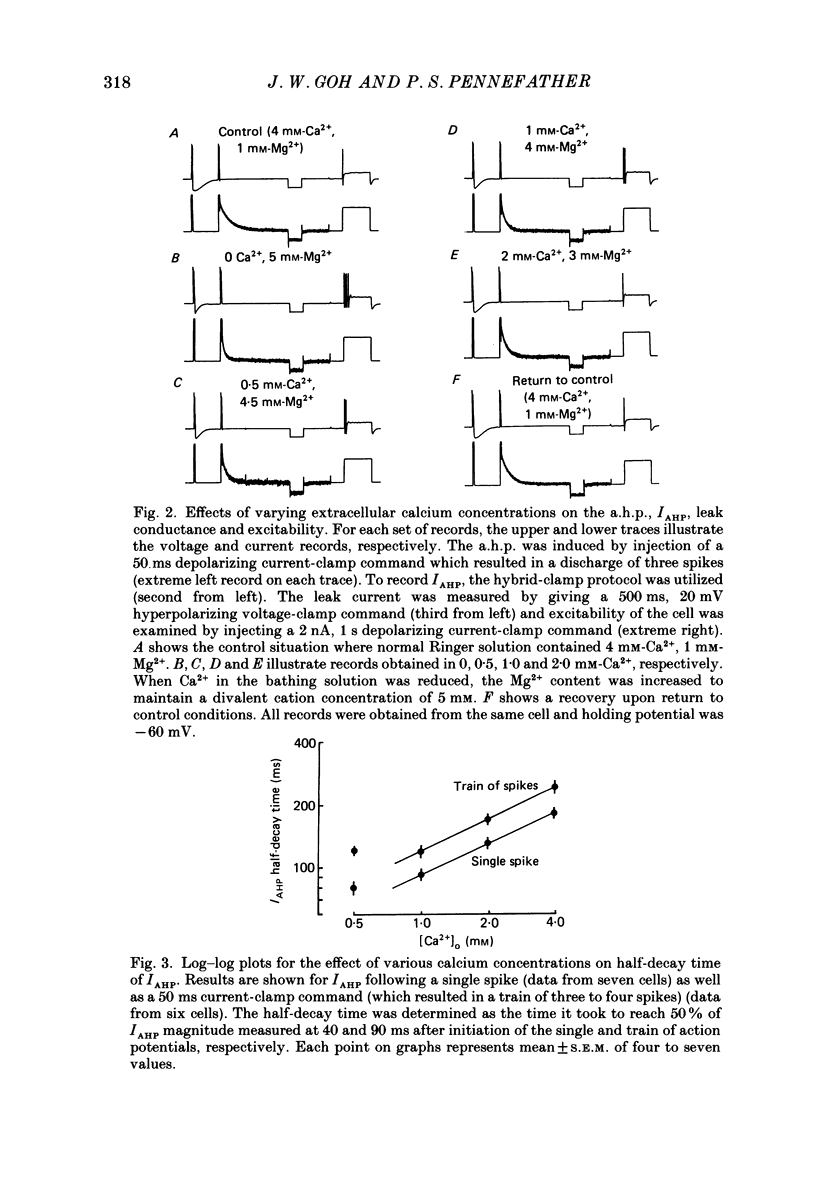
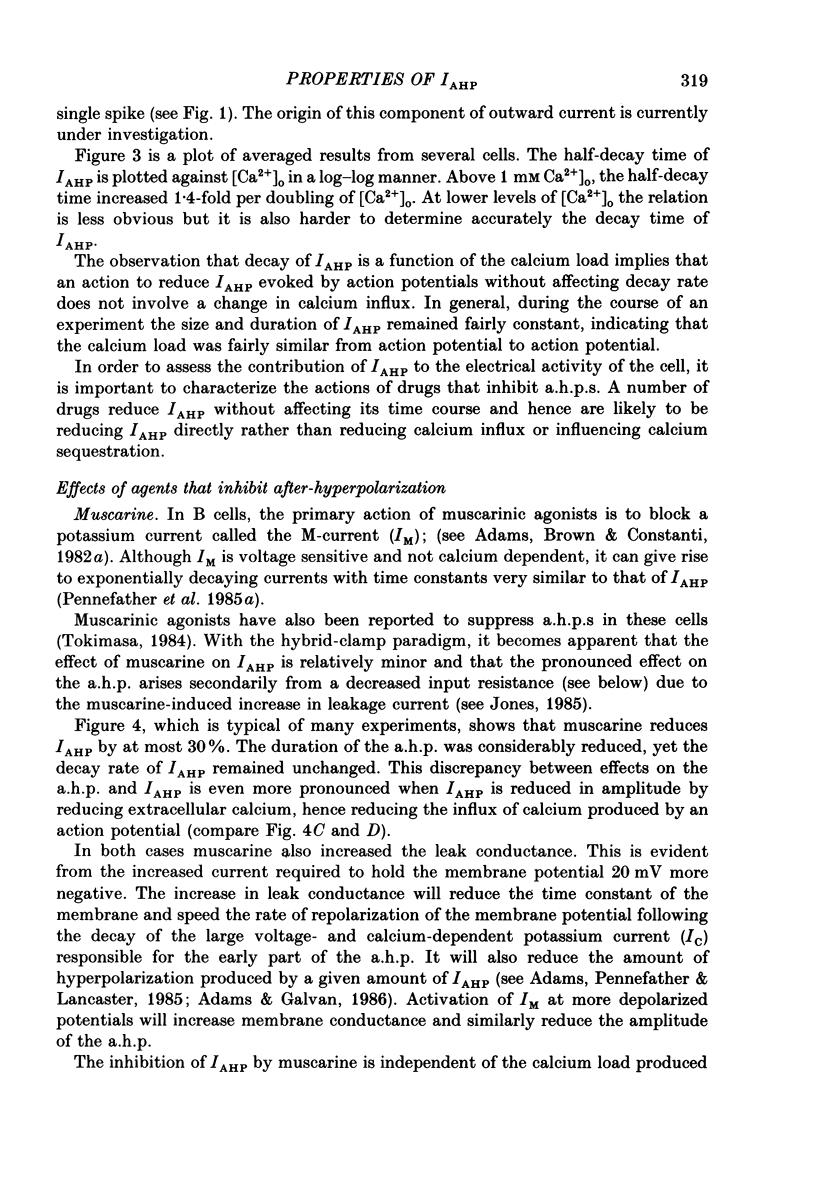
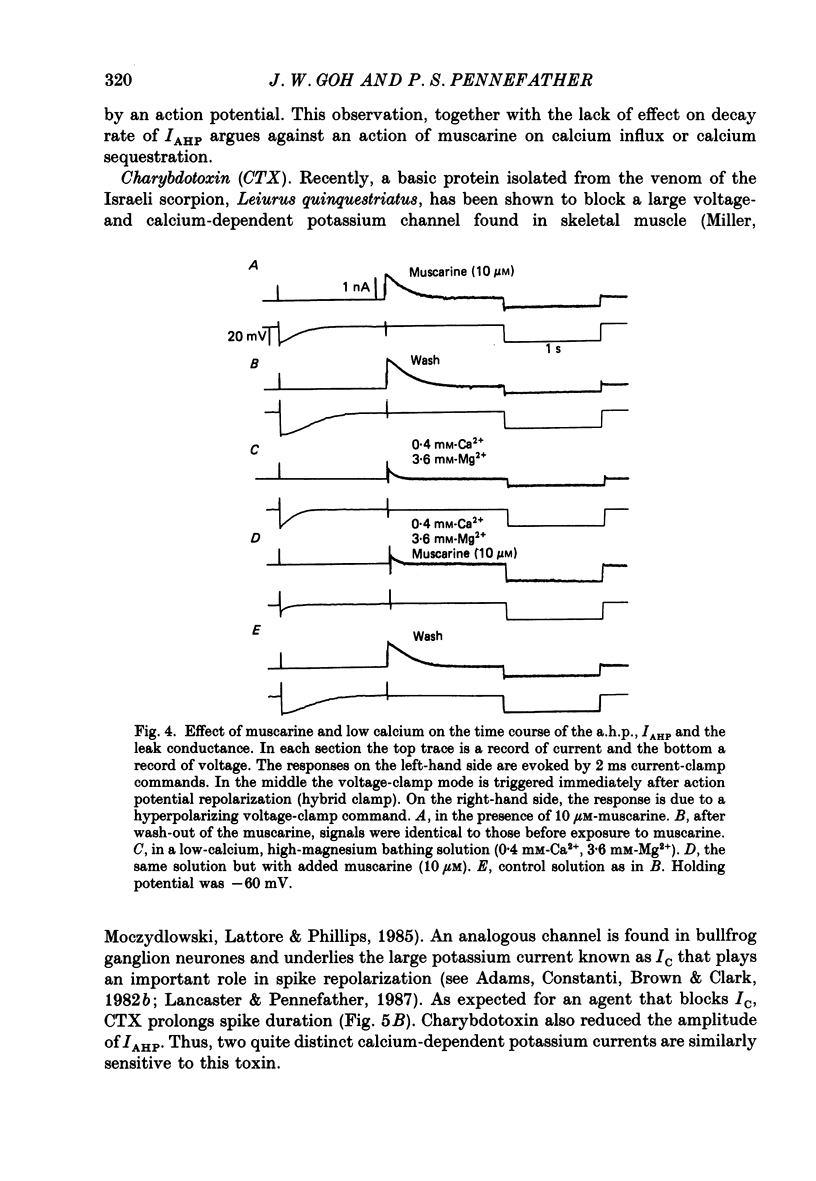
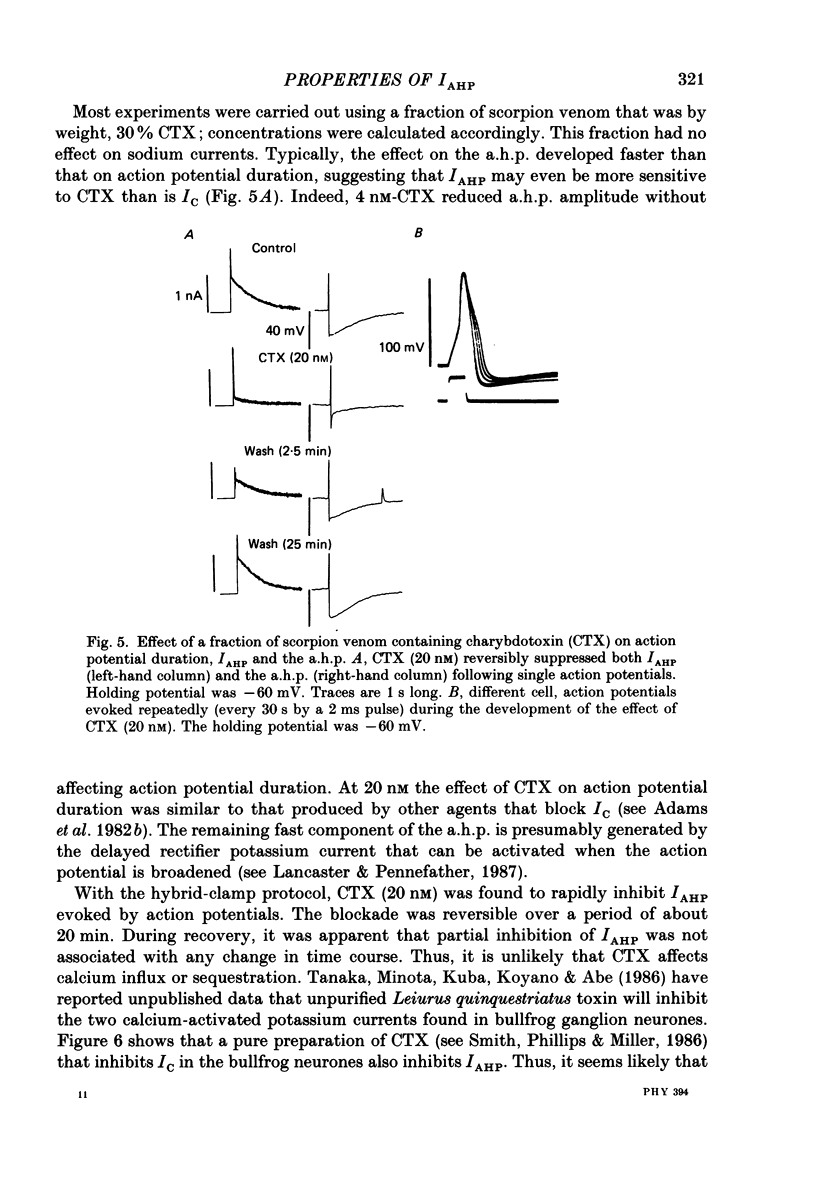
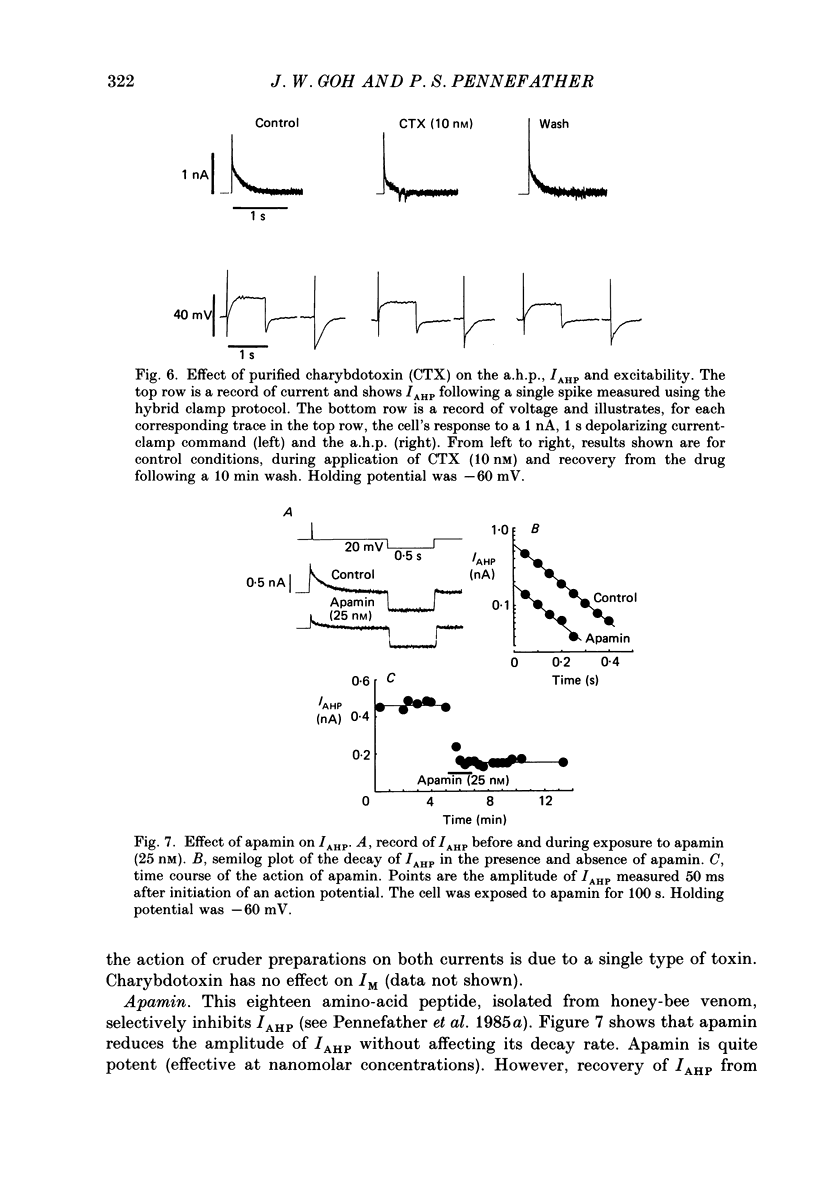
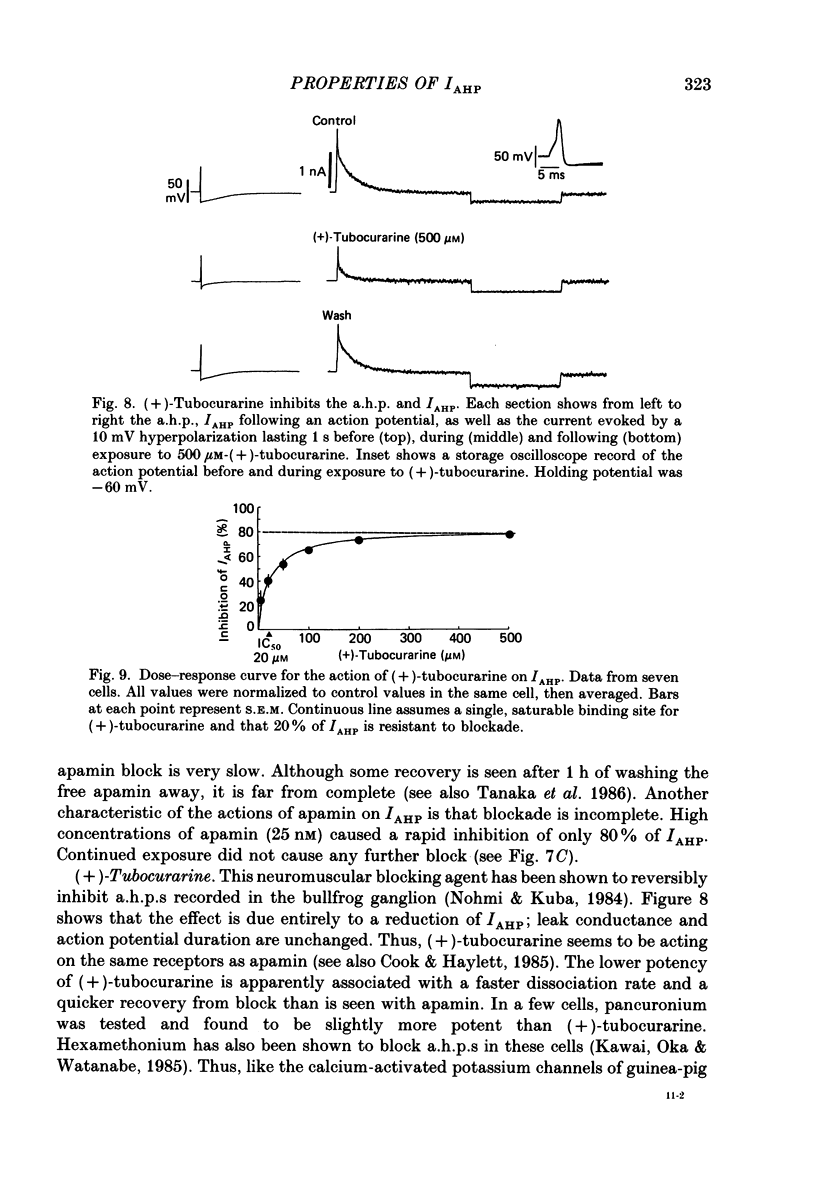
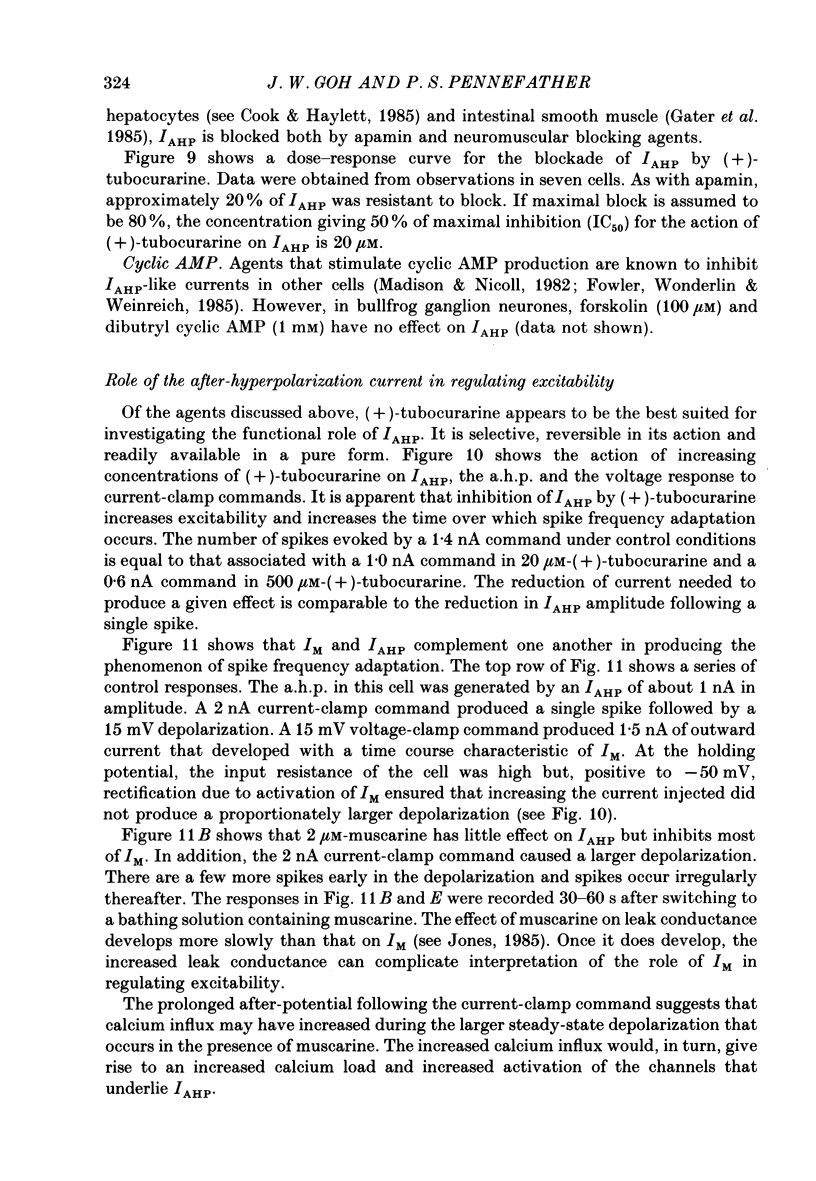
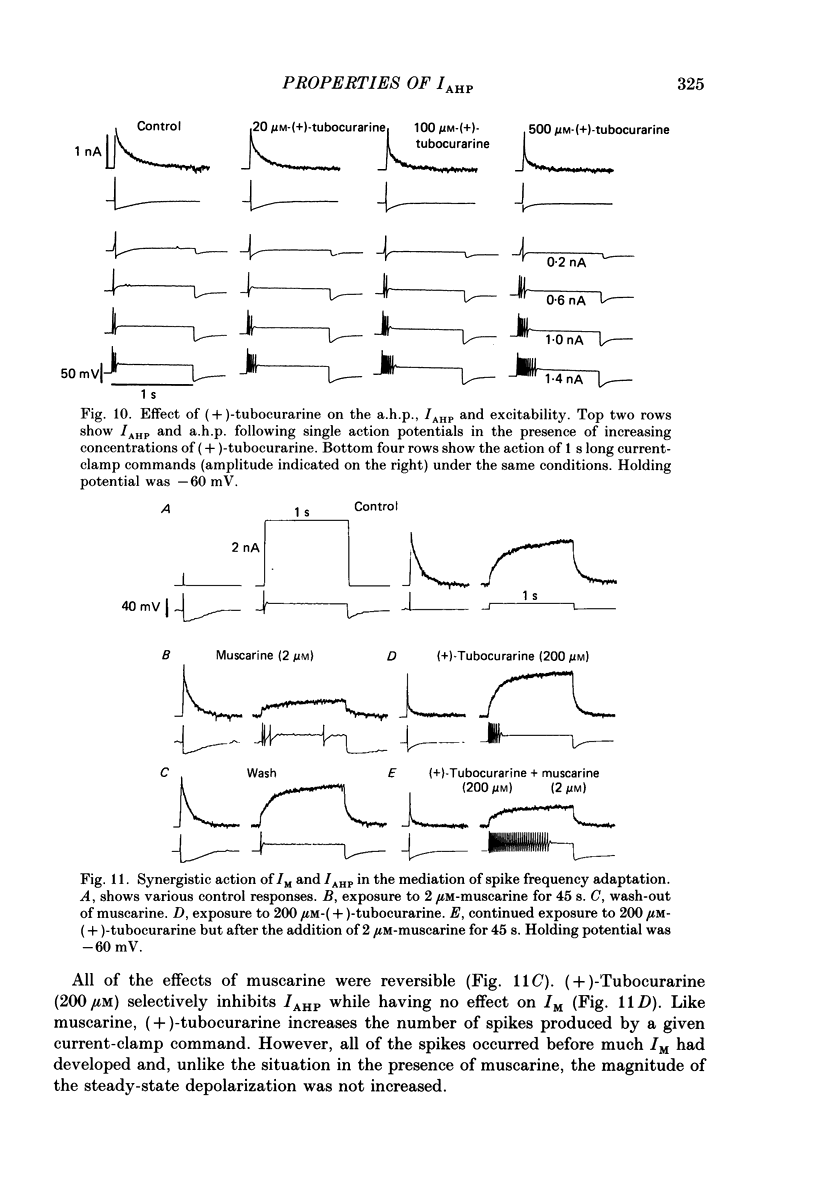
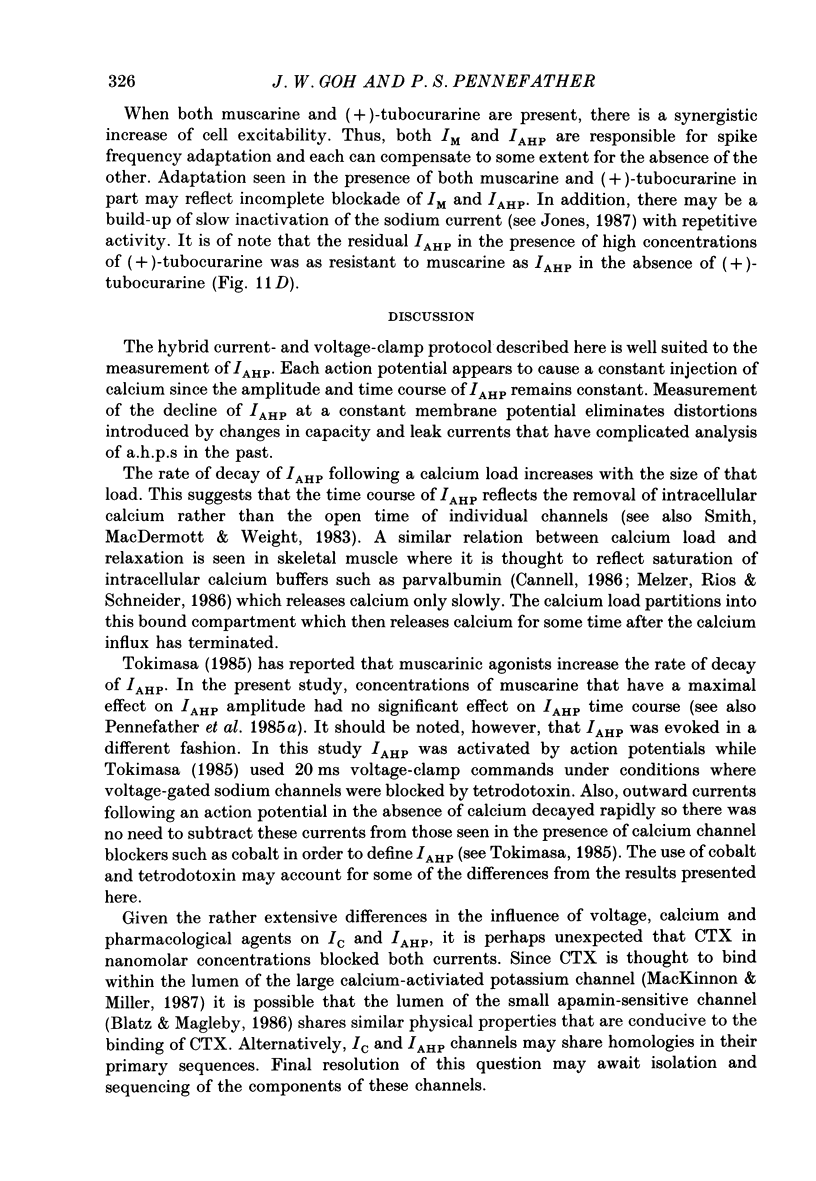
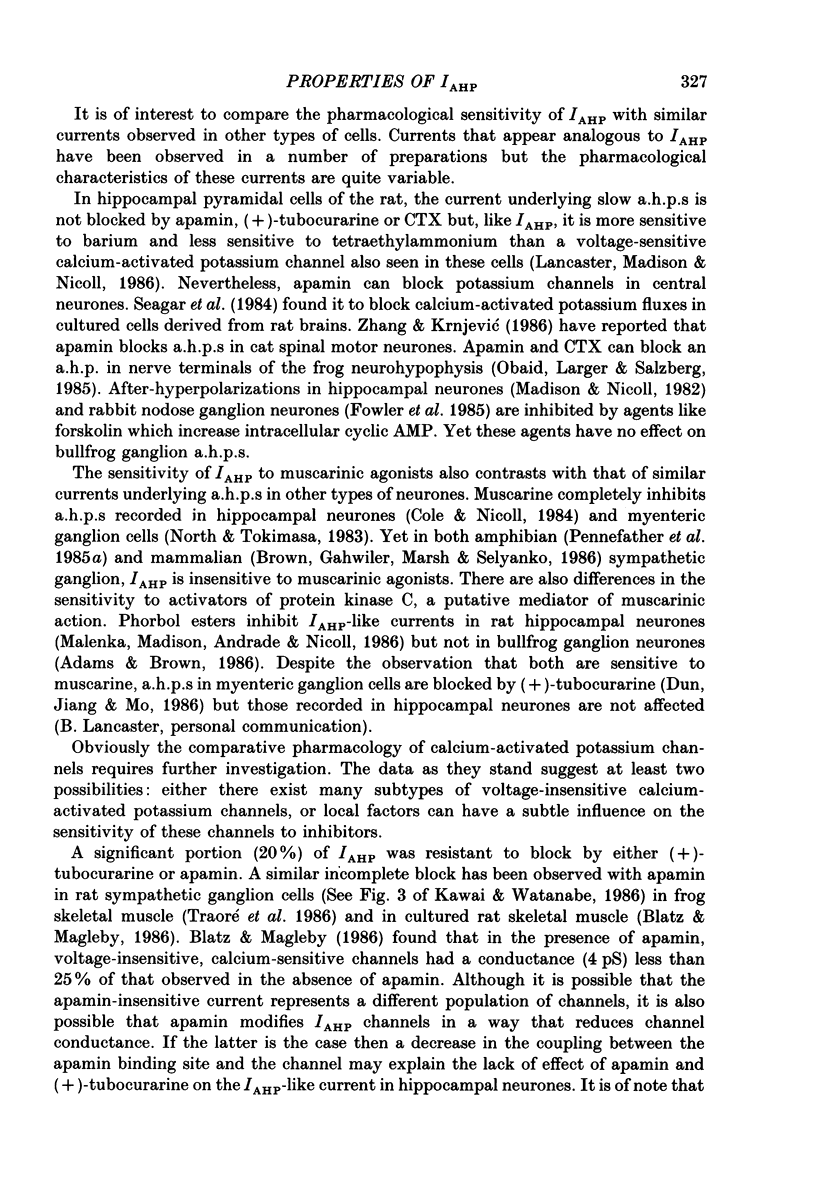
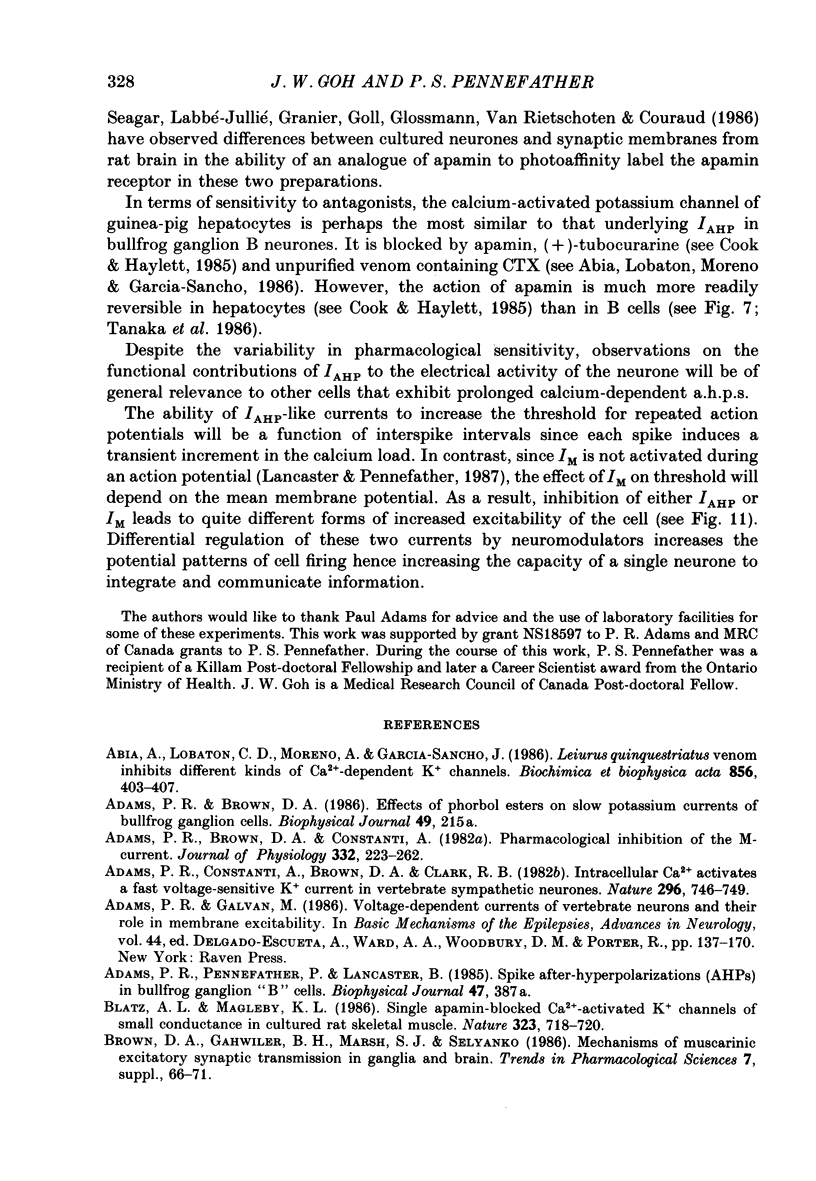
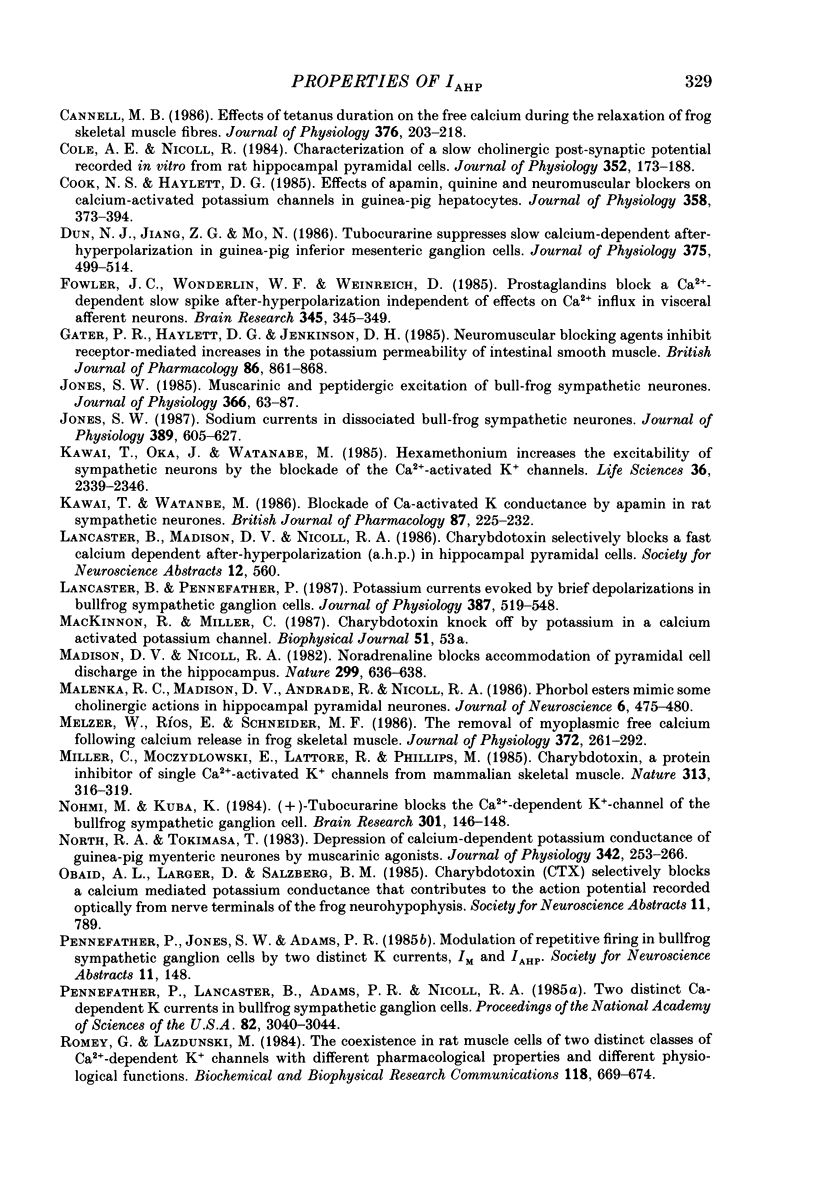
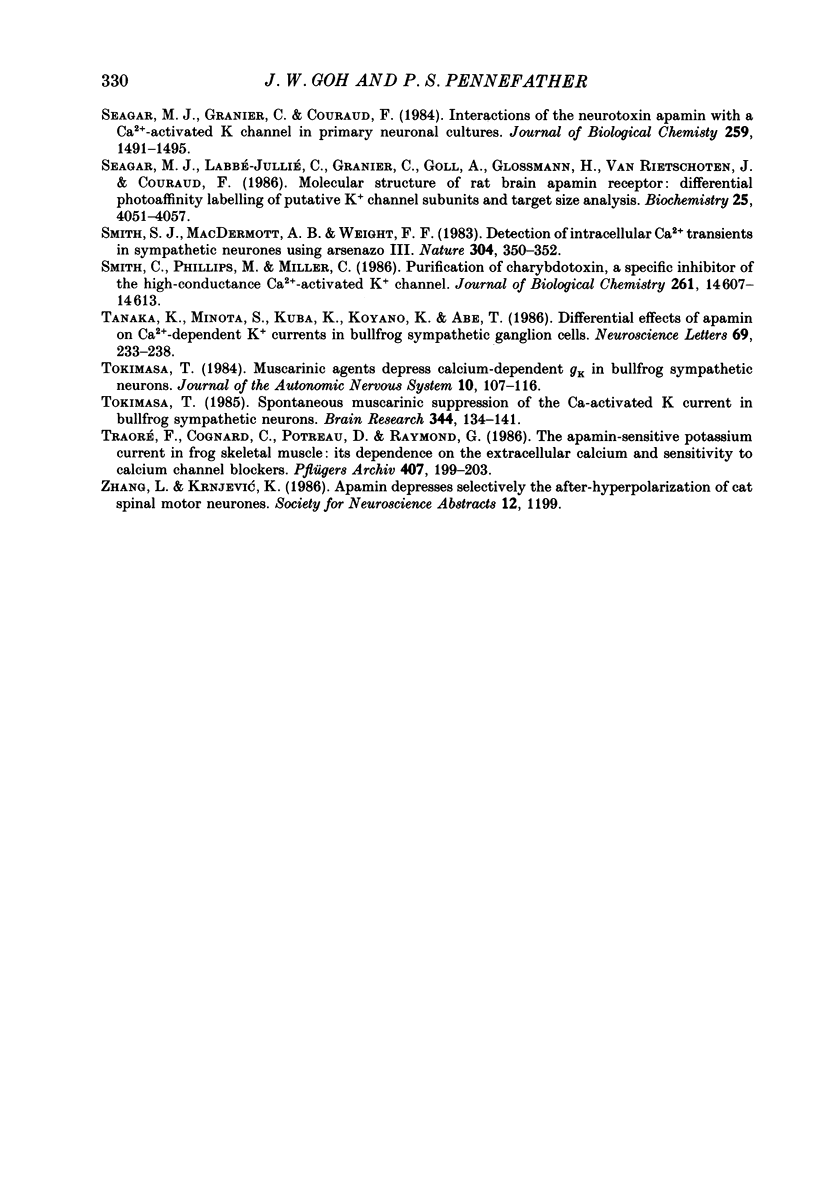
Selected References
These references are in PubMed. This may not be the complete list of references from this article.
- Abia A., Lobatón C. D., Moreno A., García-Sancho J. Leiurus quinquestriatus venom inhibits different kinds of Ca2+-dependent K+ channels. Biochim Biophys Acta. 1986 Apr 14;856(2):403–407. doi: 10.1016/0005-2736(86)90054-4. [DOI] [PubMed] [Google Scholar]
- Adams P. R., Brown D. A., Constanti A. Pharmacological inhibition of the M-current. J Physiol. 1982 Nov;332:223–262. doi: 10.1113/jphysiol.1982.sp014411. [DOI] [PMC free article] [PubMed] [Google Scholar]
- Adams P. R., Constanti A., Brown D. A., Clark R. B. Intracellular Ca2+ activates a fast voltage-sensitive K+ current in vertebrate sympathetic neurones. Nature. 1982 Apr 22;296(5859):746–749. doi: 10.1038/296746a0. [DOI] [PubMed] [Google Scholar]
- Adams P. R., Galvan M. Voltage-dependent currents of vertebrate neurons and their role in membrane excitability. Adv Neurol. 1986;44:137–170. [PubMed] [Google Scholar]
- Blatz A. L., Magleby K. L. Single apamin-blocked Ca-activated K+ channels of small conductance in cultured rat skeletal muscle. Nature. 1986 Oct 23;323(6090):718–720. doi: 10.1038/323718a0. [DOI] [PubMed] [Google Scholar]
- Cannell M. B. Effect of tetanus duration on the free calcium during the relaxation of frog skeletal muscle fibres. J Physiol. 1986 Jul;376:203–218. doi: 10.1113/jphysiol.1986.sp016149. [DOI] [PMC free article] [PubMed] [Google Scholar]
- Cole A. E., Nicoll R. A. Characterization of a slow cholinergic post-synaptic potential recorded in vitro from rat hippocampal pyramidal cells. J Physiol. 1984 Jul;352:173–188. doi: 10.1113/jphysiol.1984.sp015285. [DOI] [PMC free article] [PubMed] [Google Scholar]
- Cook N. S., Haylett D. G. Effects of apamin, quinine and neuromuscular blockers on calcium-activated potassium channels in guinea-pig hepatocytes. J Physiol. 1985 Jan;358:373–394. doi: 10.1113/jphysiol.1985.sp015556. [DOI] [PMC free article] [PubMed] [Google Scholar]
- Dun N. J., Jiang Z. G., Mo N. Tubocurarine suppresses slow calcium-dependent after-hyperpolarization in guinea-pig inferior mesenteric ganglion cells. J Physiol. 1986 Jun;375:499–514. doi: 10.1113/jphysiol.1986.sp016130. [DOI] [PMC free article] [PubMed] [Google Scholar]
- Fowler J. C., Wonderlin W. F., Weinreich D. Prostaglandins block a Ca2+-dependent slow spike afterhyperpolarization independent of effects on Ca2+ influx in visceral afferent neurons. Brain Res. 1985 Oct 21;345(2):345–349. doi: 10.1016/0006-8993(85)91014-5. [DOI] [PubMed] [Google Scholar]
- Gater P. R., Haylett D. G., Jenkinson D. H. Neuromuscular blocking agents inhibit receptor-mediated increases in the potassium permeability of intestinal smooth muscle. Br J Pharmacol. 1985 Dec;86(4):861–868. doi: 10.1111/j.1476-5381.1985.tb11108.x. [DOI] [PMC free article] [PubMed] [Google Scholar]
- Jones S. W. Muscarinic and peptidergic excitation of bull-frog sympathetic neurones. J Physiol. 1985 Sep;366:63–87. doi: 10.1113/jphysiol.1985.sp015785. [DOI] [PMC free article] [PubMed] [Google Scholar]
- Jones S. W. Sodium currents in dissociated bull-frog sympathetic neurones. J Physiol. 1987 Aug;389:605–627. doi: 10.1113/jphysiol.1987.sp016674. [DOI] [PMC free article] [PubMed] [Google Scholar]
- Kawai T., Oka J., Watanabe M. Hexamethonium increases the excitability of sympathetic neurons by the blockade of the Ca2+-activated K+ channels. Life Sci. 1985 Jun 17;36(24):2339–2346. doi: 10.1016/0024-3205(85)90324-8. [DOI] [PubMed] [Google Scholar]
- Kawai T., Watanabe M. Blockade of Ca-activated K conductance by apamin in rat sympathetic neurones. Br J Pharmacol. 1986 Jan;87(1):225–232. doi: 10.1111/j.1476-5381.1986.tb10175.x. [DOI] [PMC free article] [PubMed] [Google Scholar]
- Lancaster B., Pennefather P. Potassium currents evoked by brief depolarizations in bull-frog sympathetic ganglion cells. J Physiol. 1987 Jun;387:519–548. doi: 10.1113/jphysiol.1987.sp016587. [DOI] [PMC free article] [PubMed] [Google Scholar]
- Madison D. V., Nicoll R. A. Noradrenaline blocks accommodation of pyramidal cell discharge in the hippocampus. Nature. 1982 Oct 14;299(5884):636–638. doi: 10.1038/299636a0. [DOI] [PubMed] [Google Scholar]
- Malenka R. C., Madison D. V., Andrade R., Nicoll R. A. Phorbol esters mimic some cholinergic actions in hippocampal pyramidal neurons. J Neurosci. 1986 Feb;6(2):475–480. doi: 10.1523/JNEUROSCI.06-02-00475.1986. [DOI] [PMC free article] [PubMed] [Google Scholar]
- Melzer W., Ríos E., Schneider M. F. The removal of myoplasmic free calcium following calcium release in frog skeletal muscle. J Physiol. 1986 Mar;372:261–292. doi: 10.1113/jphysiol.1986.sp016008. [DOI] [PMC free article] [PubMed] [Google Scholar]
- Miller C., Moczydlowski E., Latorre R., Phillips M. Charybdotoxin, a protein inhibitor of single Ca2+-activated K+ channels from mammalian skeletal muscle. Nature. 1985 Jan 24;313(6000):316–318. doi: 10.1038/313316a0. [DOI] [PubMed] [Google Scholar]
- Nohmi M., Kuba K. (+)-Tubocurarine blocks the Ca2+-dependent K+-channel of the bullfrog sympathetic ganglion cell. Brain Res. 1984 May 28;301(1):146–148. doi: 10.1016/0006-8993(84)90412-8. [DOI] [PubMed] [Google Scholar]
- North R. A., Tokimasa T. Depression of calcium-dependent potassium conductance of guinea-pig myenteric neurones by muscarinic agonists. J Physiol. 1983 Sep;342:253–266. doi: 10.1113/jphysiol.1983.sp014849. [DOI] [PMC free article] [PubMed] [Google Scholar]
- Pennefather P., Lancaster B., Adams P. R., Nicoll R. A. Two distinct Ca-dependent K currents in bullfrog sympathetic ganglion cells. Proc Natl Acad Sci U S A. 1985 May;82(9):3040–3044. doi: 10.1073/pnas.82.9.3040. [DOI] [PMC free article] [PubMed] [Google Scholar]
- Romey G., Lazdunski M. The coexistence in rat muscle cells of two distinct classes of Ca2+-dependent K+ channels with different pharmacological properties and different physiological functions. Biochem Biophys Res Commun. 1984 Jan 30;118(2):669–674. doi: 10.1016/0006-291x(84)91355-x. [DOI] [PubMed] [Google Scholar]
- Seagar M. J., Granier C., Couraud F. Interactions of the neurotoxin apamin with a Ca2+-activated K+ channel in primary neuronal cultures. J Biol Chem. 1984 Feb 10;259(3):1491–1495. [PubMed] [Google Scholar]
- Seagar M. J., Labbé-Jullié C., Granier C., Goll A., Glossmann H., Van Rietschoten J., Couraud F. Molecular structure of rat brain apamin receptor: differential photoaffinity labeling of putative K+ channel subunits and target size analysis. Biochemistry. 1986 Jul 15;25(14):4051–4057. doi: 10.1021/bi00362a010. [DOI] [PubMed] [Google Scholar]
- Smith C., Phillips M., Miller C. Purification of charybdotoxin, a specific inhibitor of the high-conductance Ca2+-activated K+ channel. J Biol Chem. 1986 Nov 5;261(31):14607–14613. [PubMed] [Google Scholar]
- Smith S. J., MacDermott A. B., Weight F. F. Detection of intracellular Ca2+ transients in sympathetic neurones using arsenazo III. 1983 Jul 28-Aug 3Nature. 304(5924):350–352. doi: 10.1038/304350a0. [DOI] [PubMed] [Google Scholar]
- Tanaka K., Minota S., Kuba K., Koyano K., Abe T. Differential effects of apamin on Ca2+-dependent K+ currents in bullfrog sympathetic ganglion cells. Neurosci Lett. 1986 Sep 12;69(3):233–238. doi: 10.1016/0304-3940(86)90485-4. [DOI] [PubMed] [Google Scholar]
- Tokimasa T. Muscarinic agonists depress calcium-dependent gK in bullfrog sympathetic neurons. J Auton Nerv Syst. 1984 Apr;10(2):107–116. doi: 10.1016/0165-1838(84)90049-3. [DOI] [PubMed] [Google Scholar]
- Tokimasa T. Spontaneous muscarinic suppression of the Ca-activated K-current in bullfrog sympathetic neurons. Brain Res. 1985 Sep 30;344(1):134–141. doi: 10.1016/0006-8993(85)91197-7. [DOI] [PubMed] [Google Scholar]
- Traoré F., Cognard C., Potreau D., Raymond G. The apamin-sensitive potassium current in frog skeletal muscle: its dependence on the extracellular calcium and sensitivity to calcium channel blockers. Pflugers Arch. 1986 Aug;407(2):199–203. doi: 10.1007/BF00580676. [DOI] [PubMed] [Google Scholar]