Abstract
1. Ionic dependence and kinetic properties of the peptide-evoked potentials across everted toad intestine were investigated with eighteen dipeptides and four tripeptides. All peptides evoked saturable increases in the mucosal negativity regardless of the presence of Na+. 2. The peptide-evoked potentials recorded in the absence of Na+ were sensitive to external pH (pHo); lowering pHo from 7.4 to 6.5 and 5.5 caused stepwise increases in their amplitude. 3. Loading epithelial cells with 9-aminoacridine or acetate caused a significant increase or decrease in amplitude of the Gly-Gly-evoked potential, suggesting intracellular alkalinization or acidification also has a great influence on the peptide-evoked potential. 4. Kinetically, Na+-independent peptide-evoked potentials conformed to simple Michaelis-Menten kinetics, and lowering pHo caused a decrease of the half-saturation concentration (Kt) for Gly-Gly without changing the maximum potential difference increase. Similar affinity-type kinetic effect was also seen for Gly-Gly influx. 5. Simultaneous measurements of Gly-Gly-induced increase in short-circuit current and Gly-Gly influx revealed that the coupling ratio of H+ and Gly-Gly flows was 1.78 +/- 0.12, suggesting the stoichiometry of the H+-peptide co-transport being 2:1. 6. Kinetic analyses of the peptide-evoked potentials indicated that all glycyl-dipeptides tested (Gly-Gly, Gly-Pro, Gly-Sar, Gly-Leu, Gly-Phe) and other dipeptides (Ala-Ala, Ala-Phe, Phe-Ala) shared a common carrier. Gly-Gly-Gly and Ala-Ala-Ala were also found to share the same carrier, while Phe-Phe, Leu-Leu and Phe-Leu appeared to be transported by a different carrier. 7. Kt values for di- and tripeptides, which apparently shared a common carrier, fell in a narrow range (0.5-2.2 mM). There was no clear correlation between 1/Kt value and molecular weight.
Full text
PDF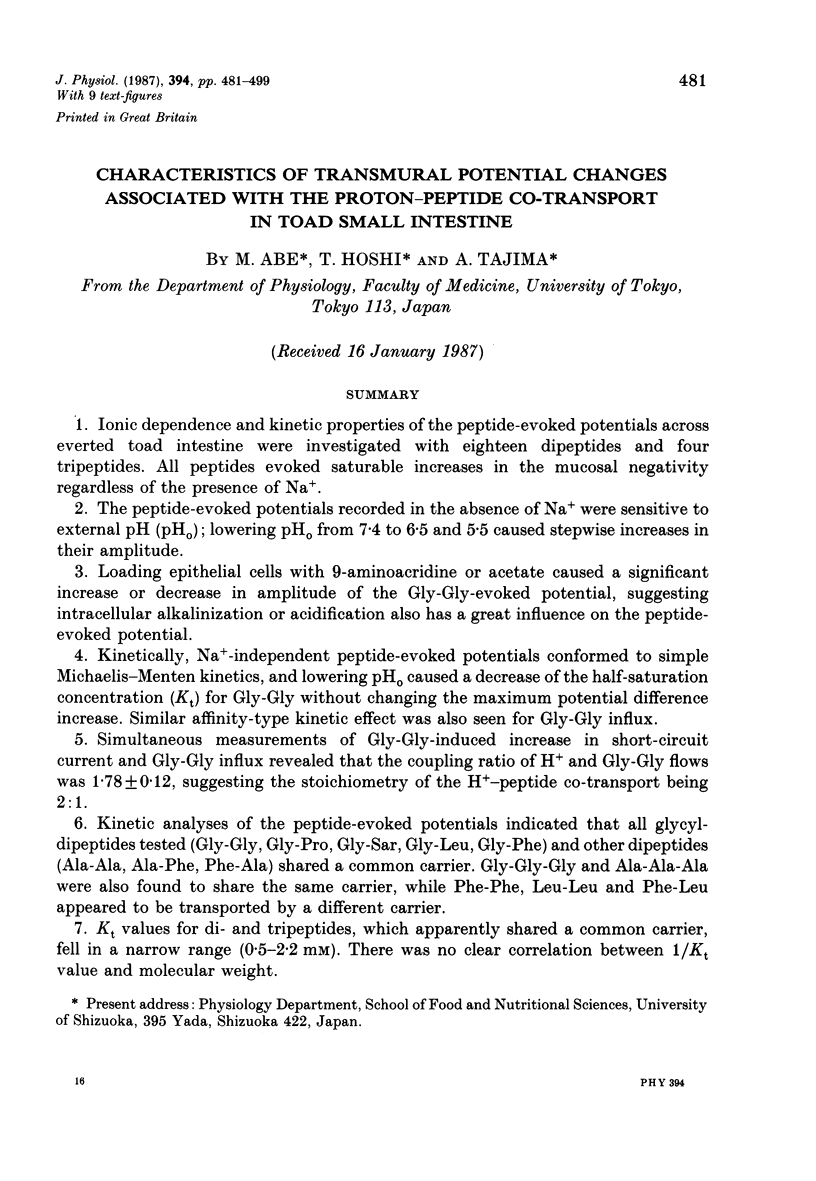
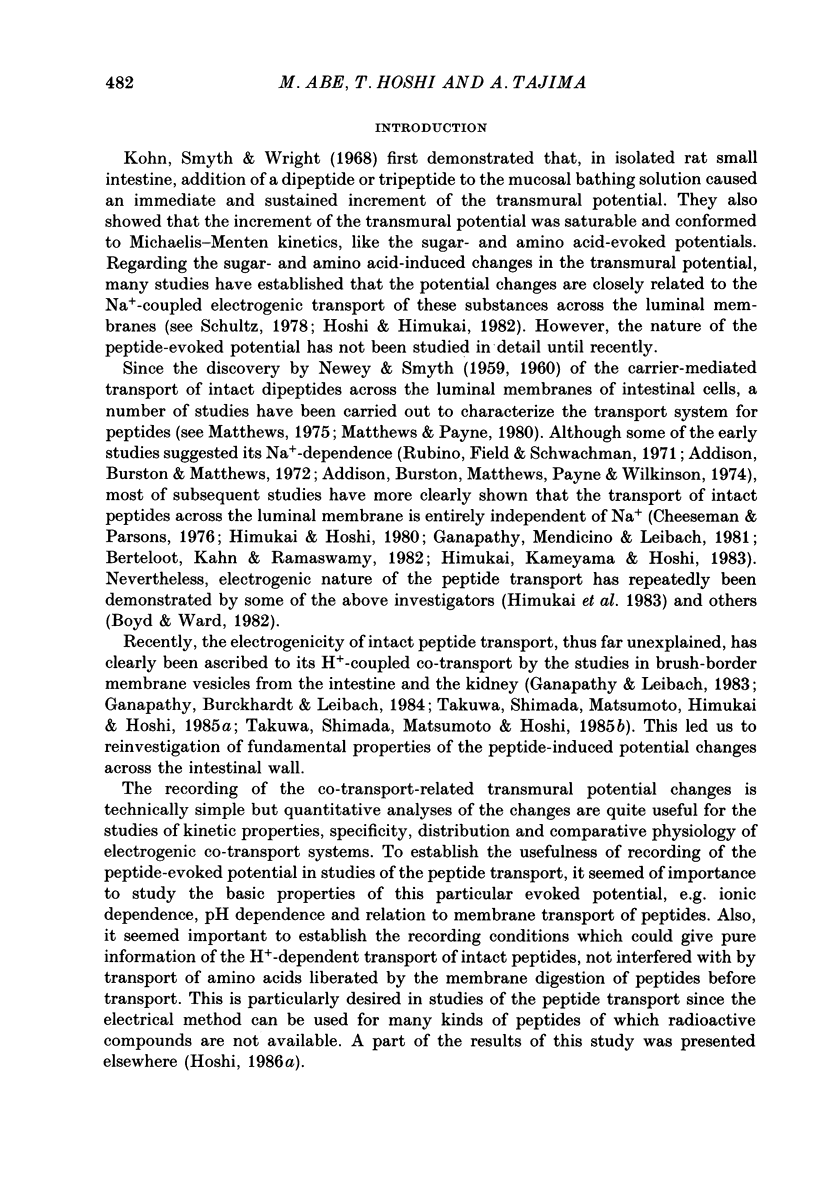
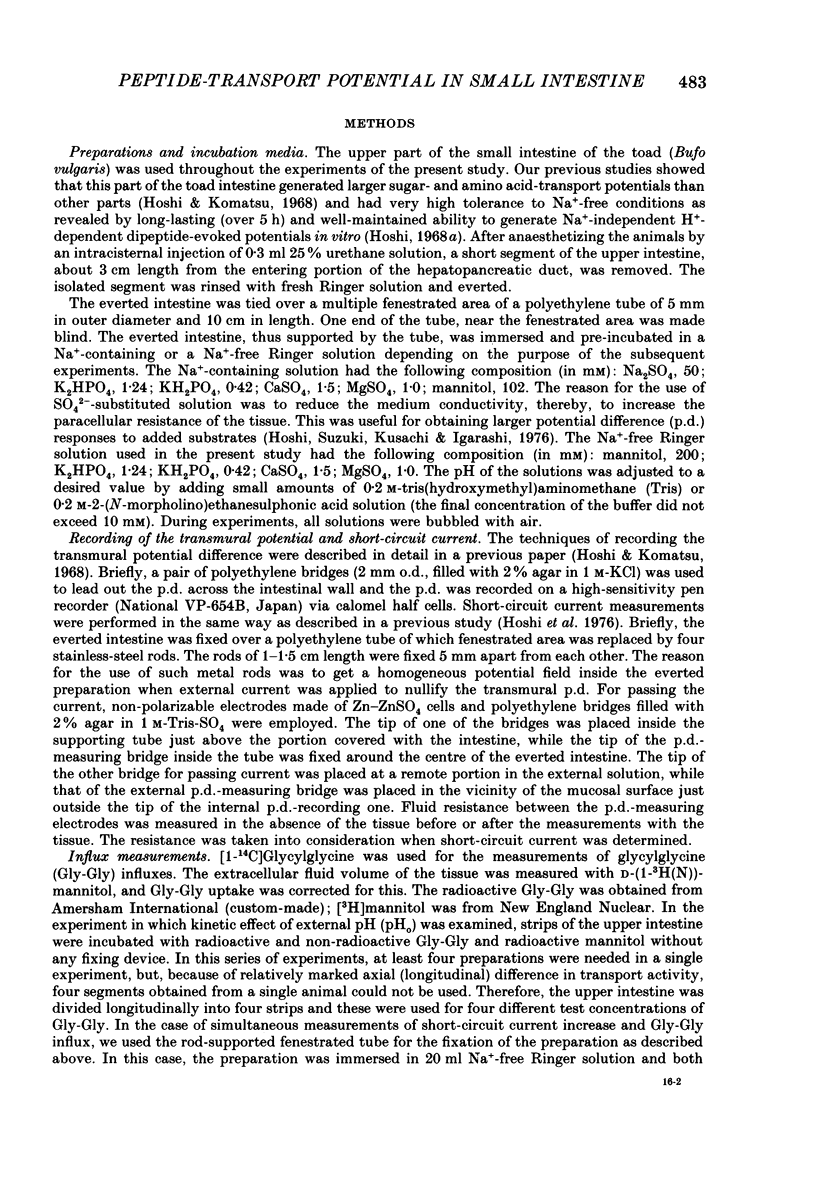
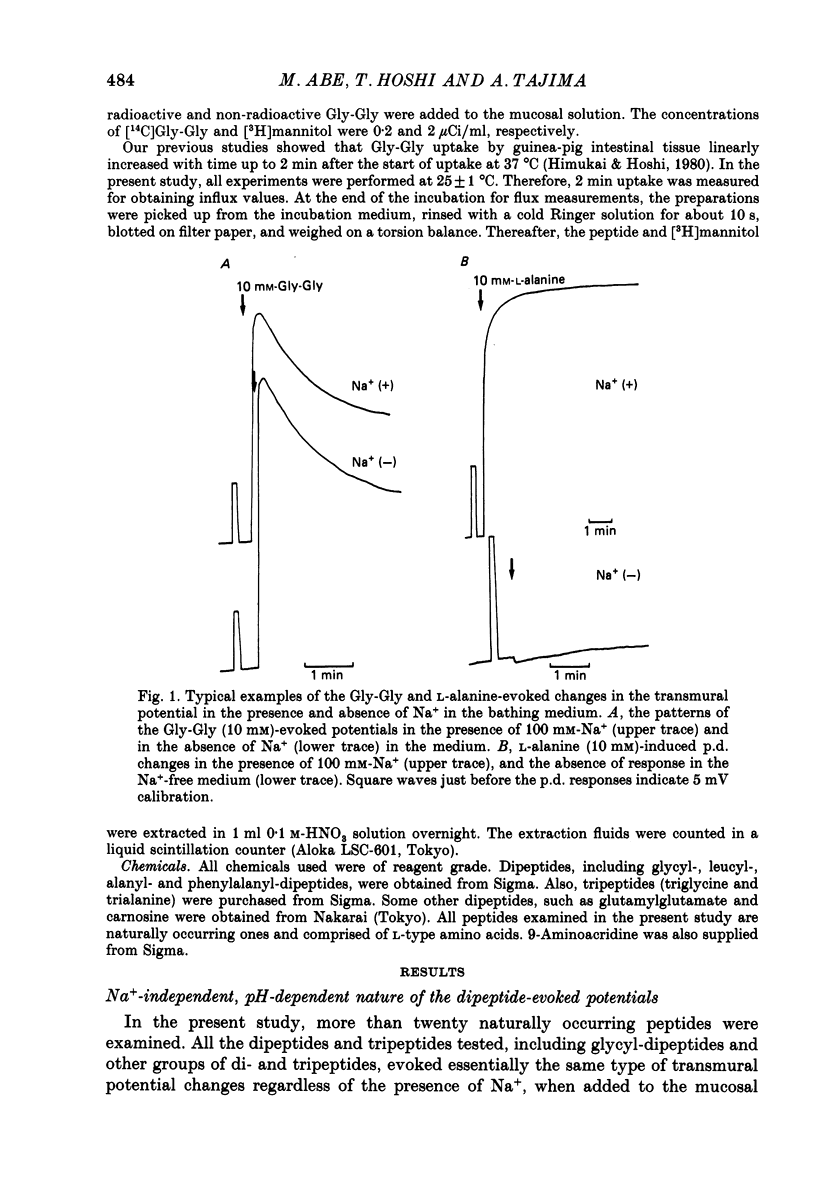
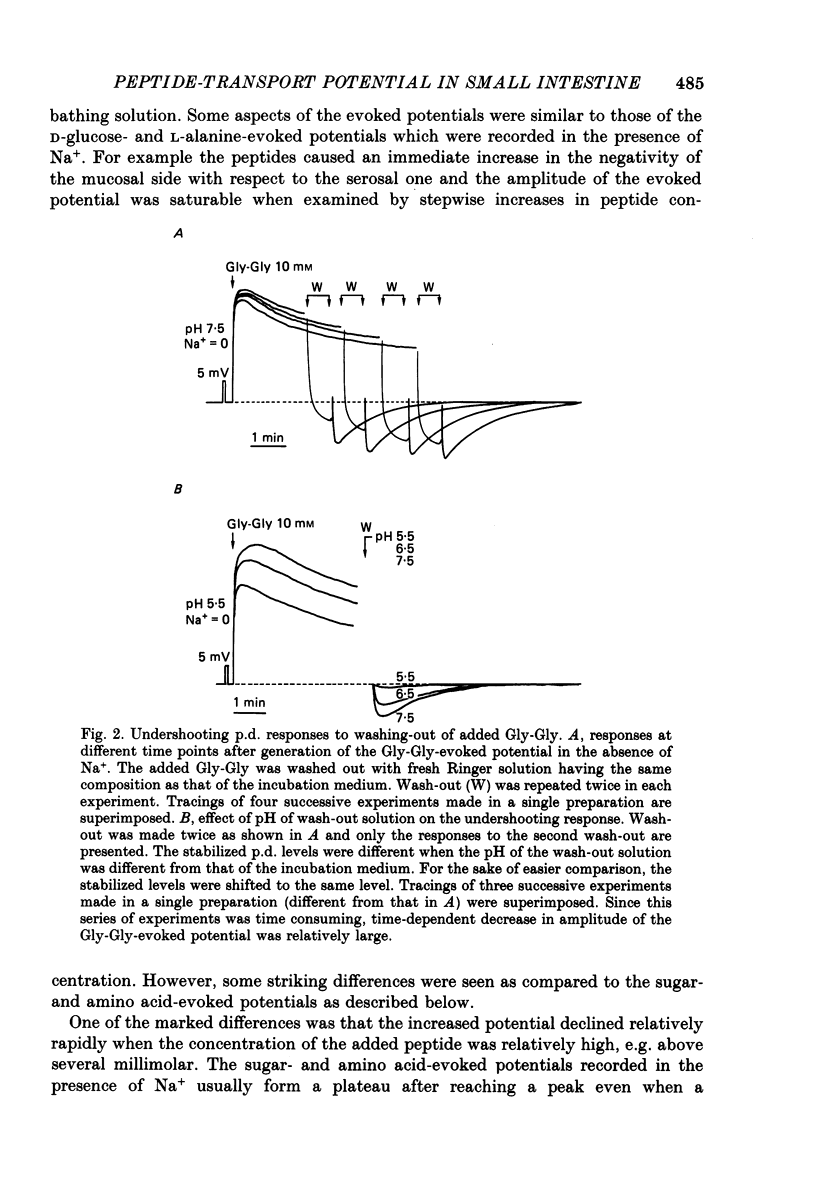
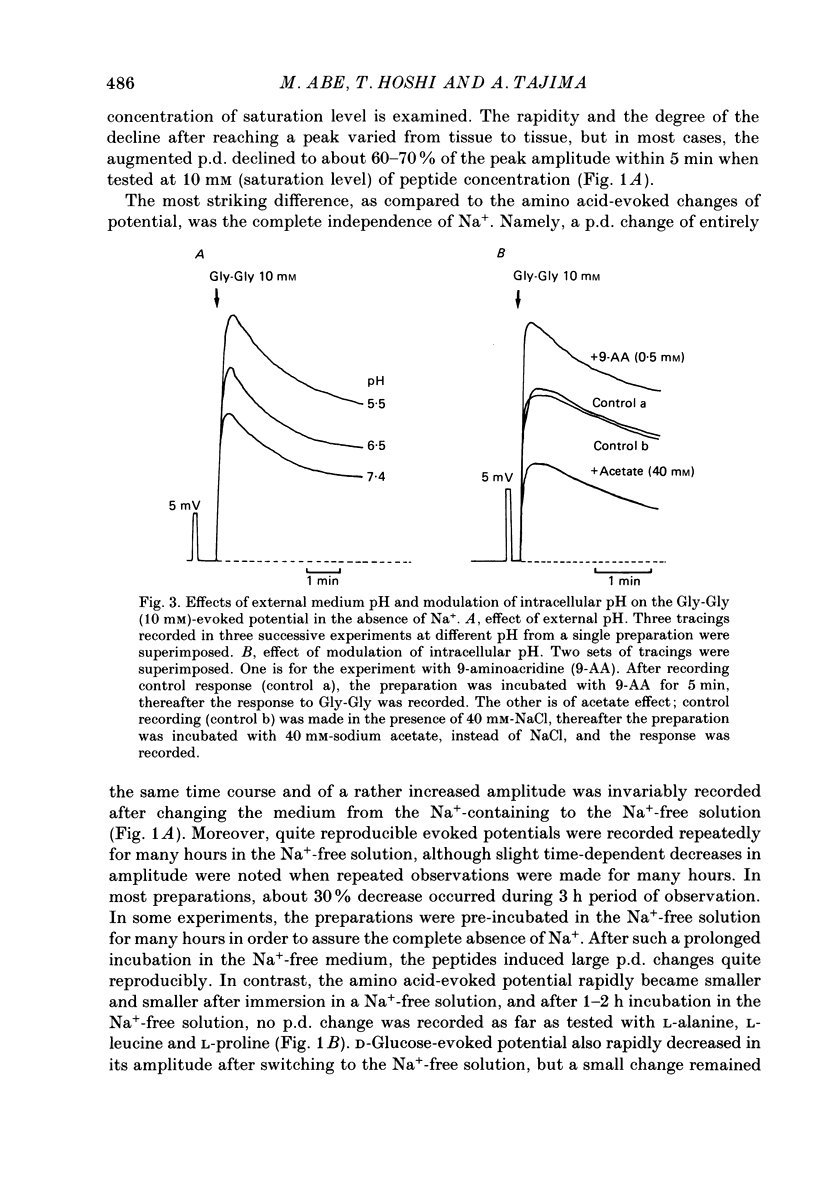
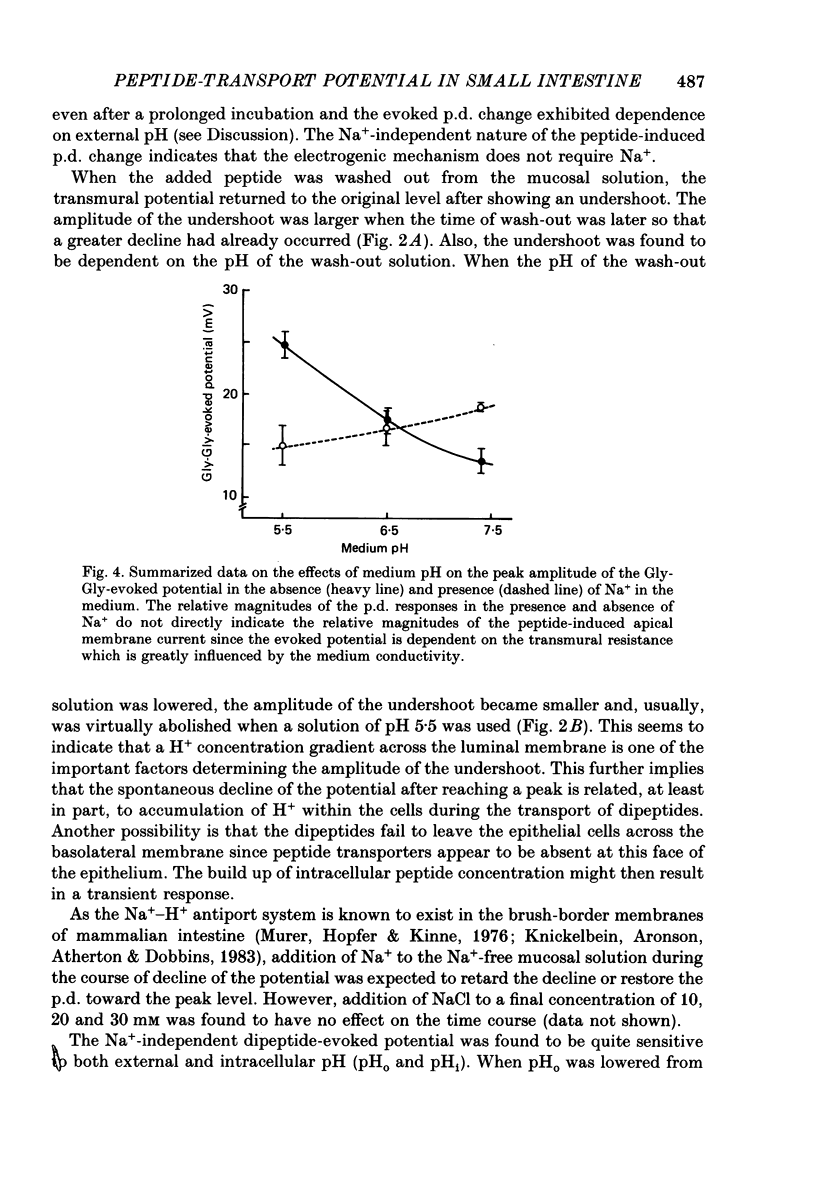
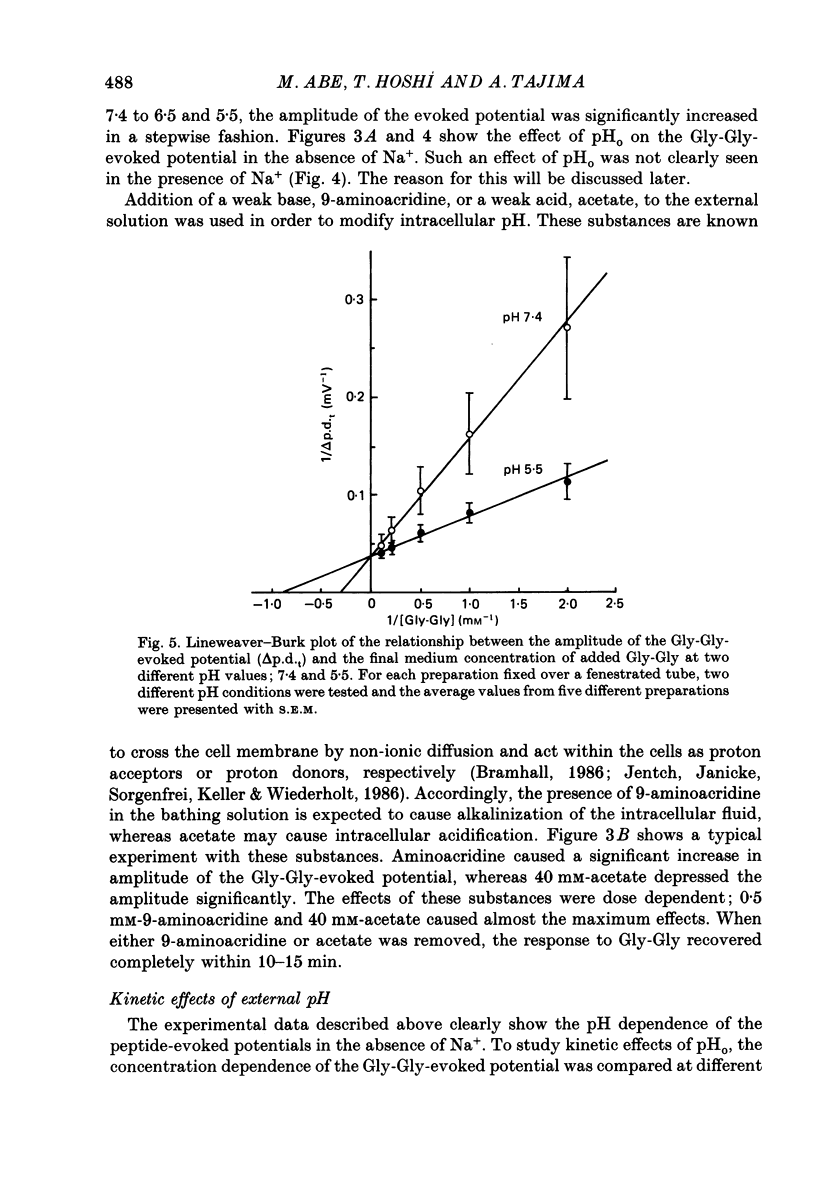
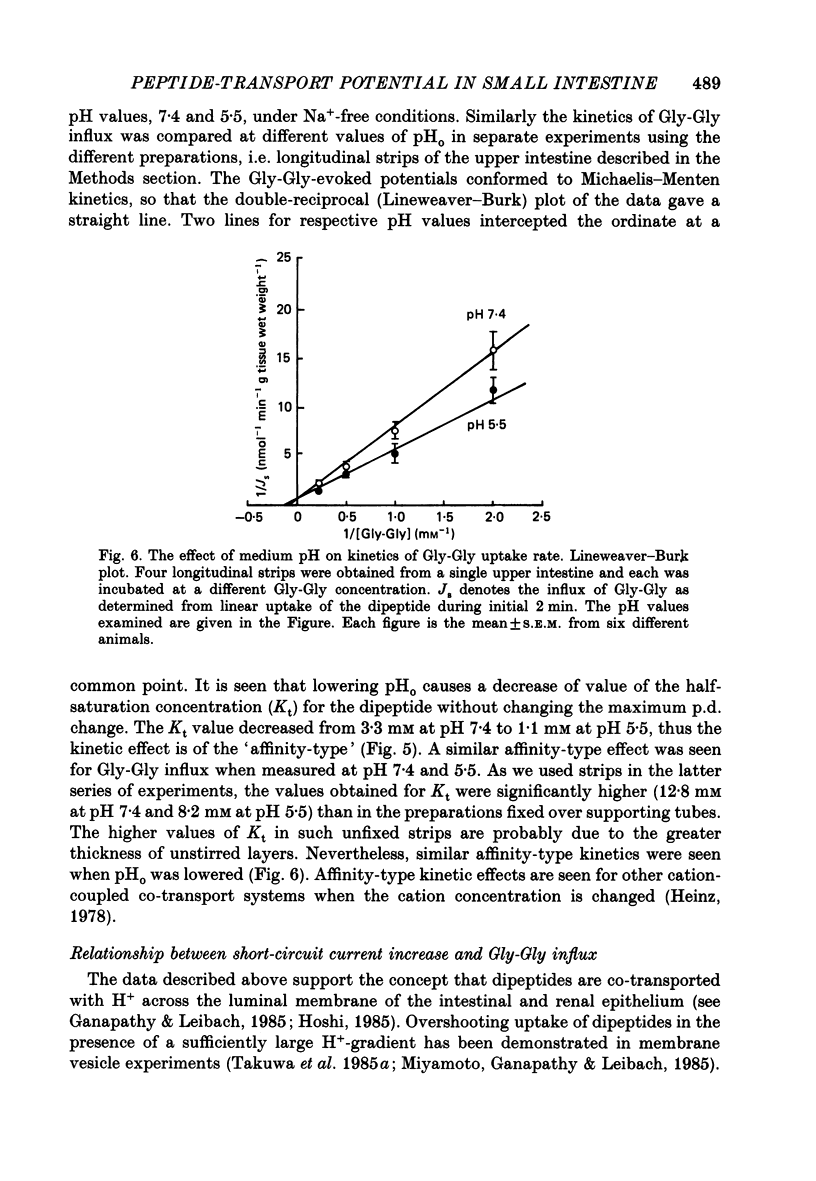
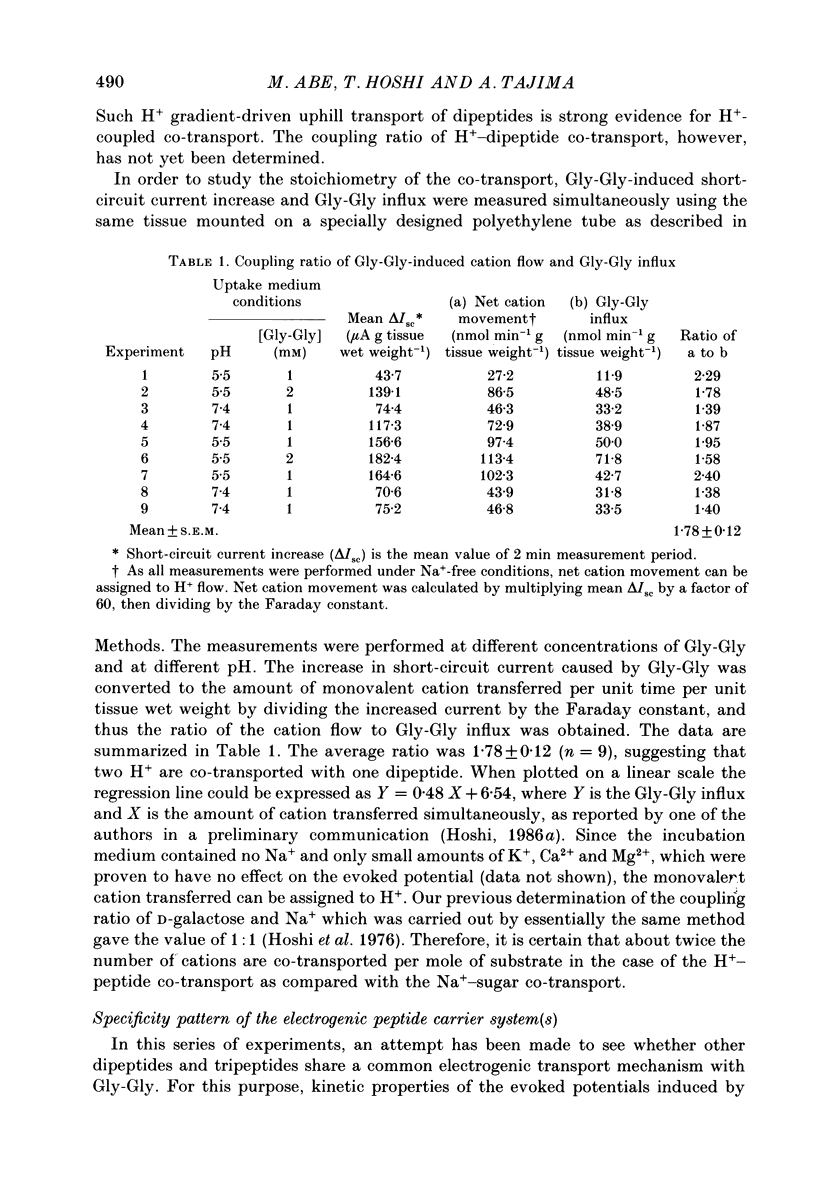
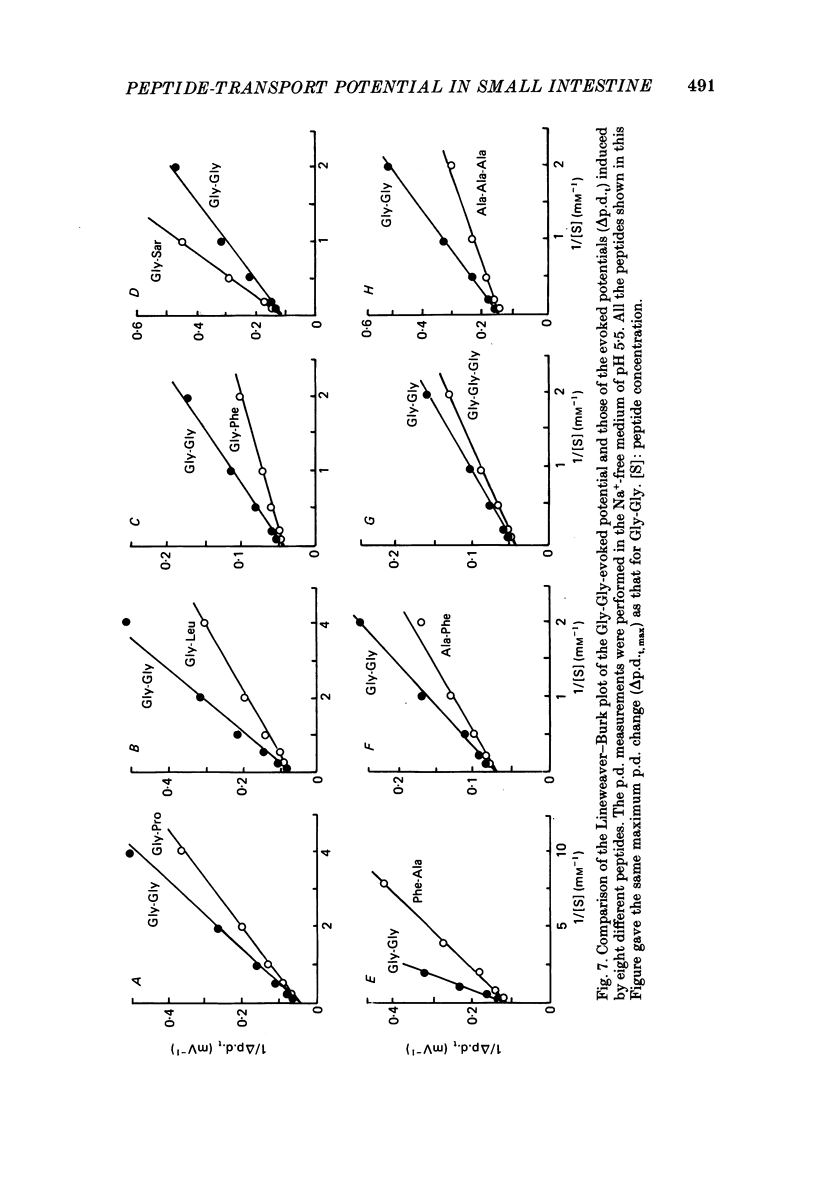
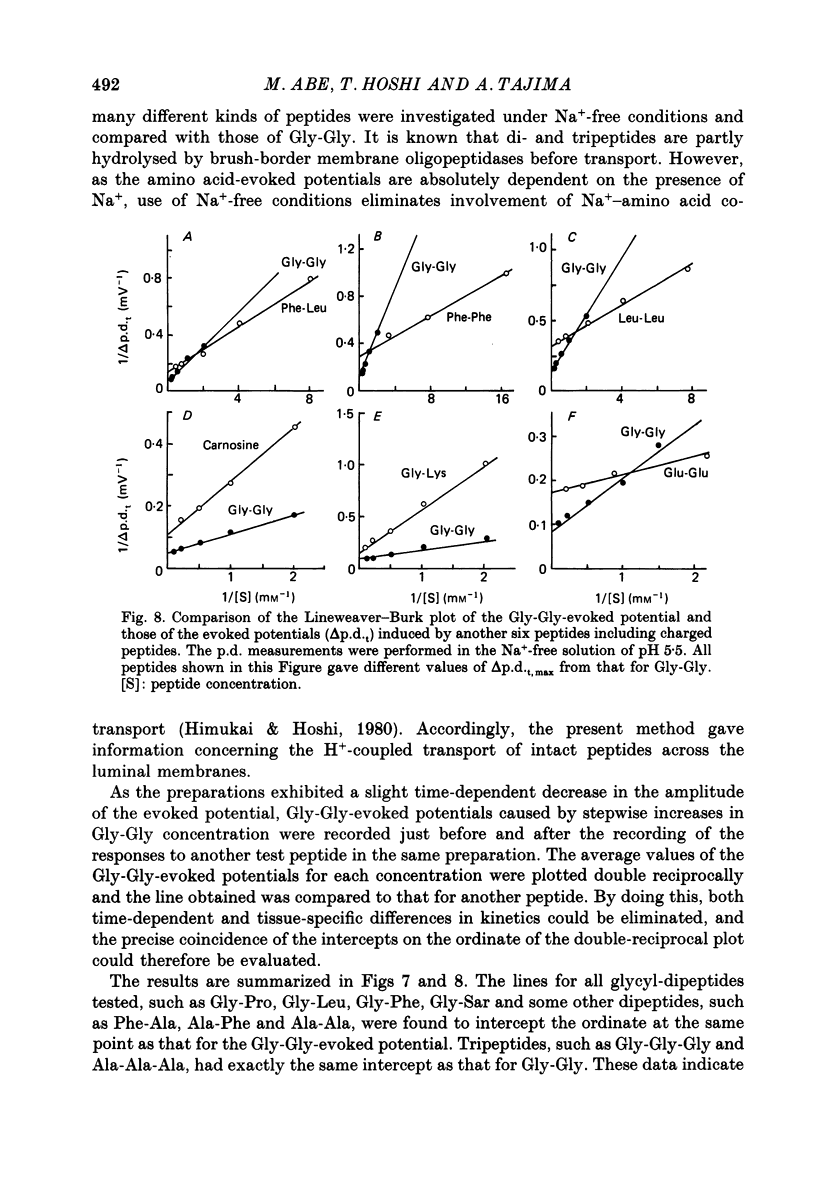
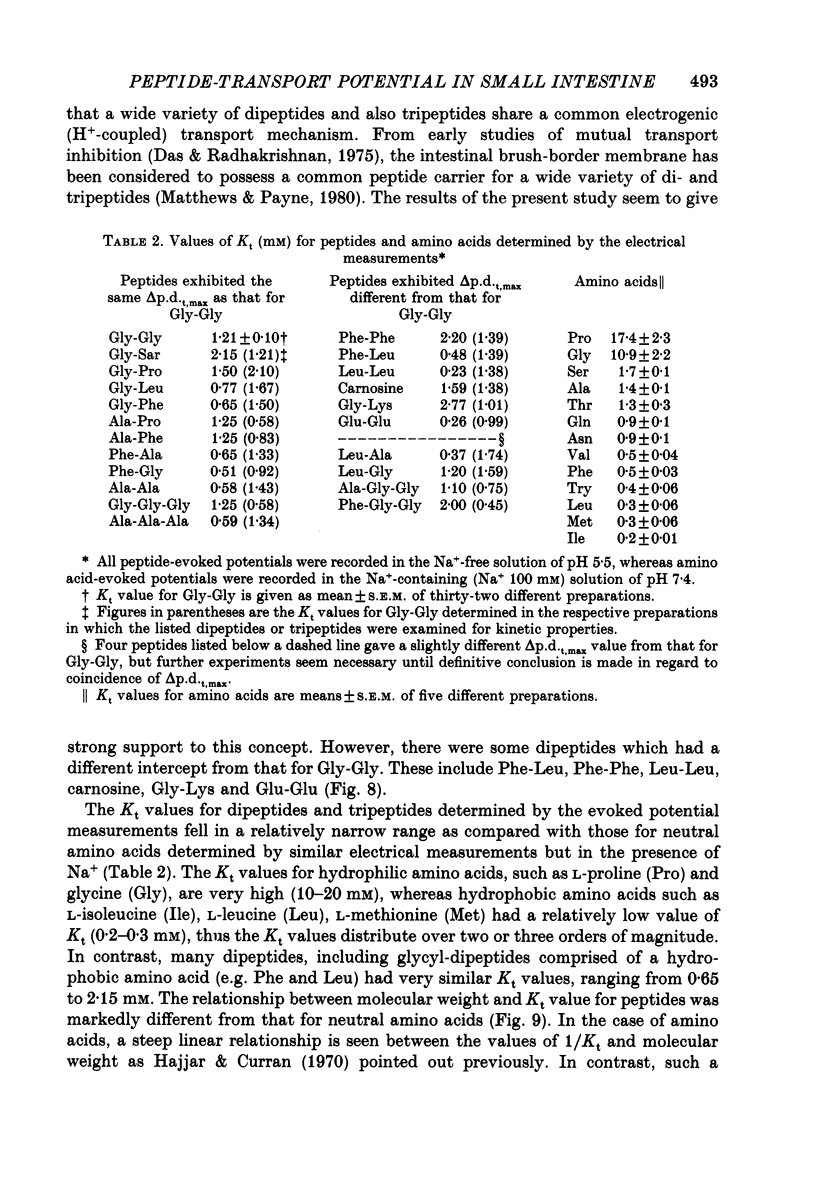
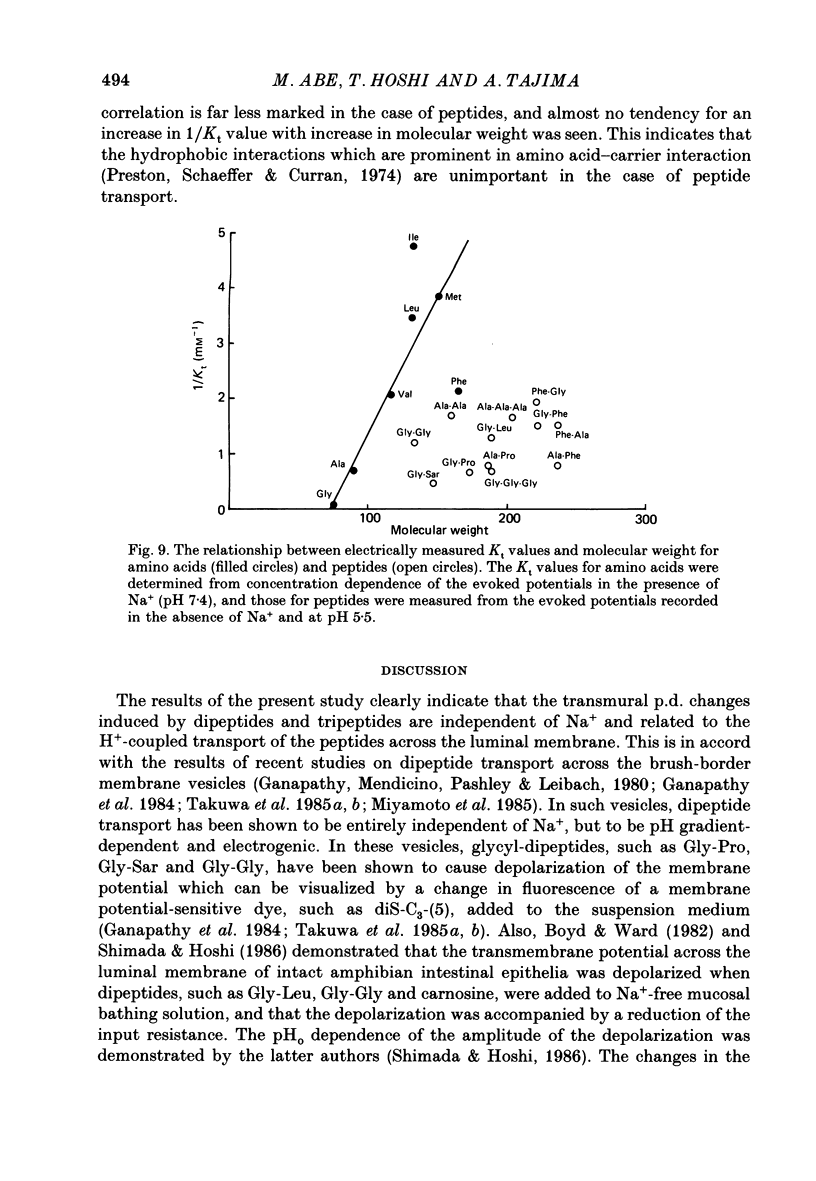
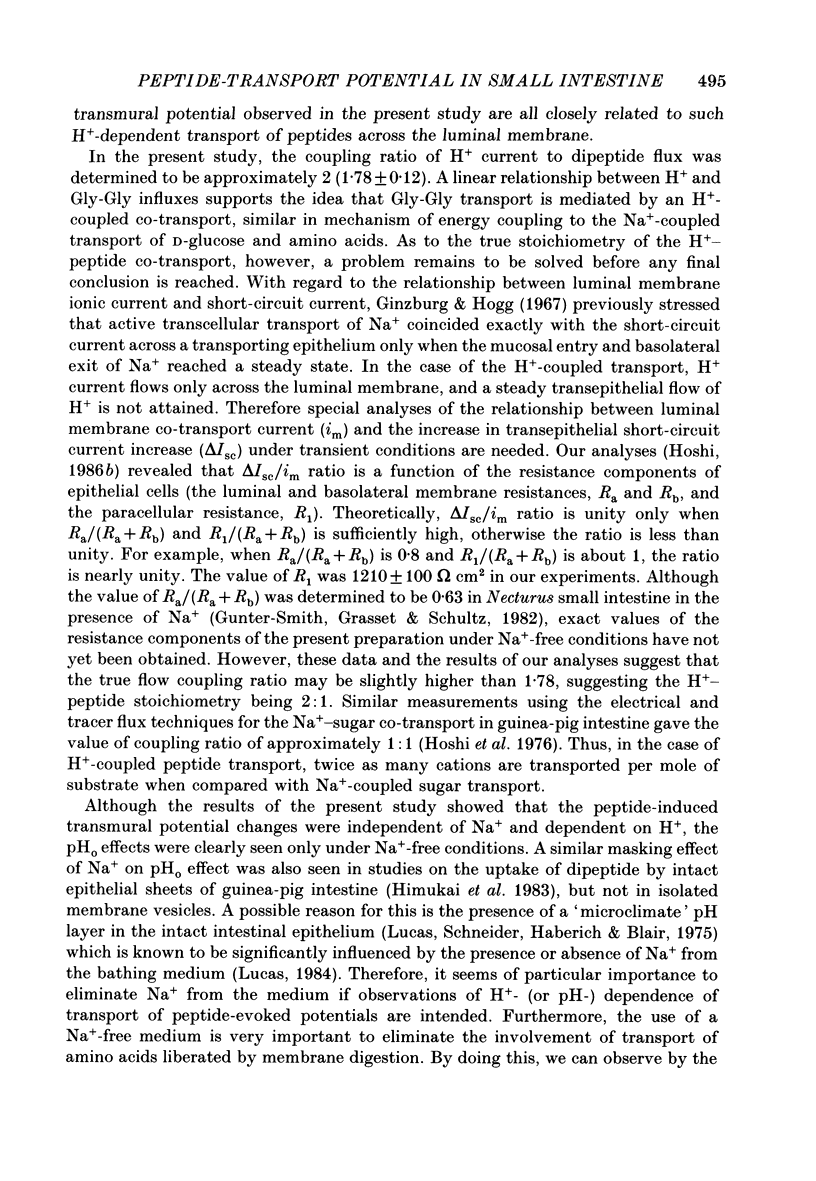
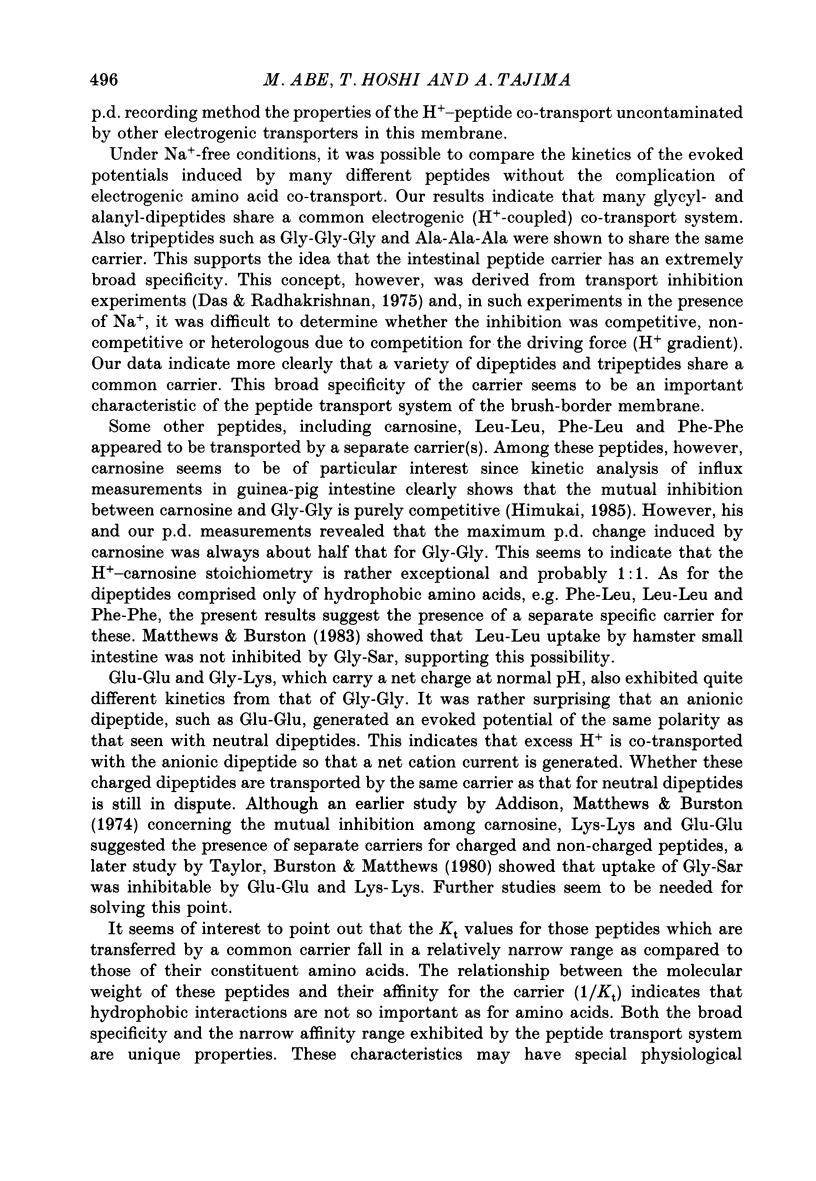
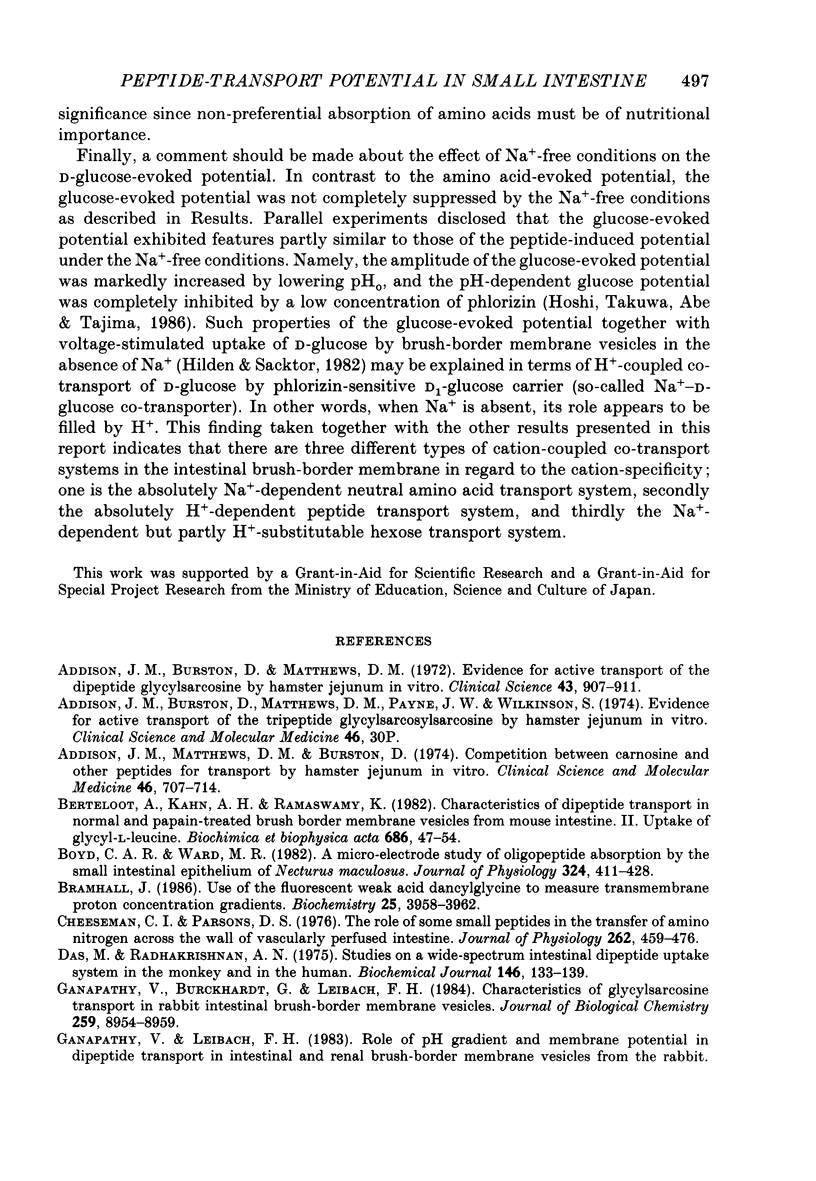
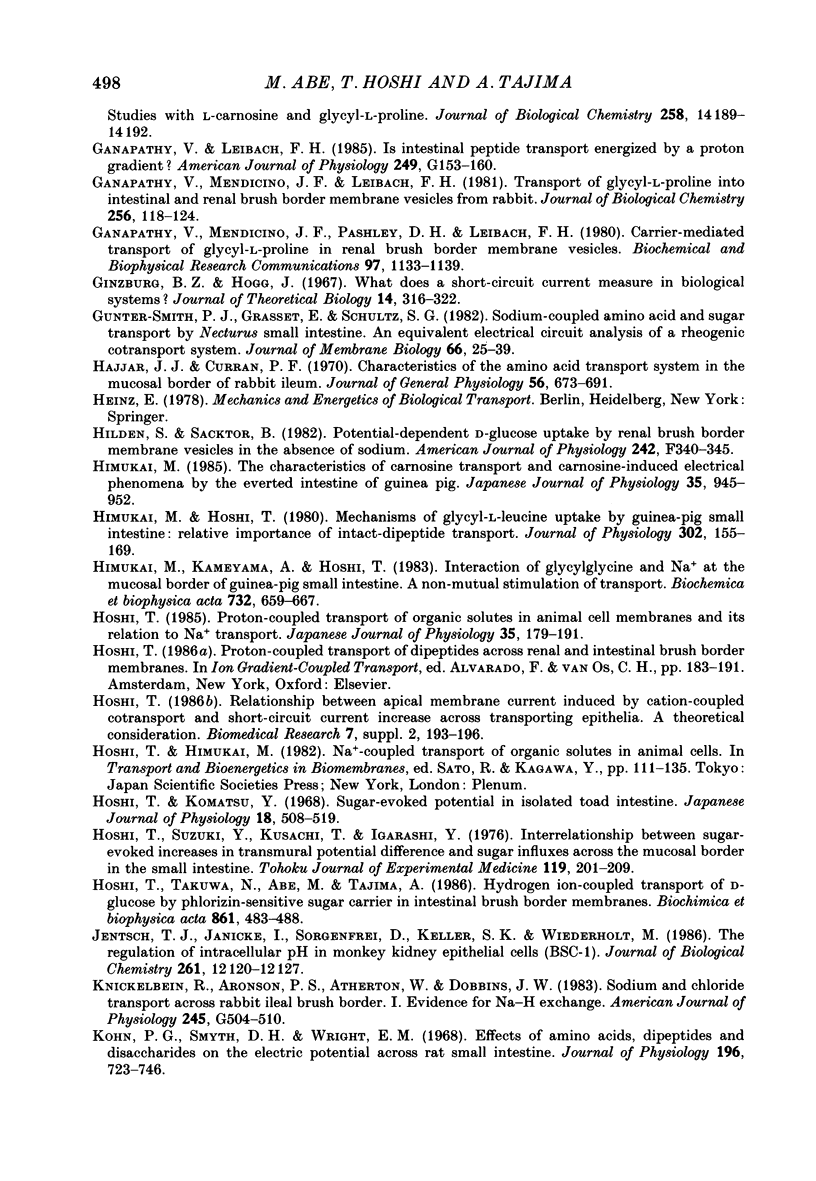
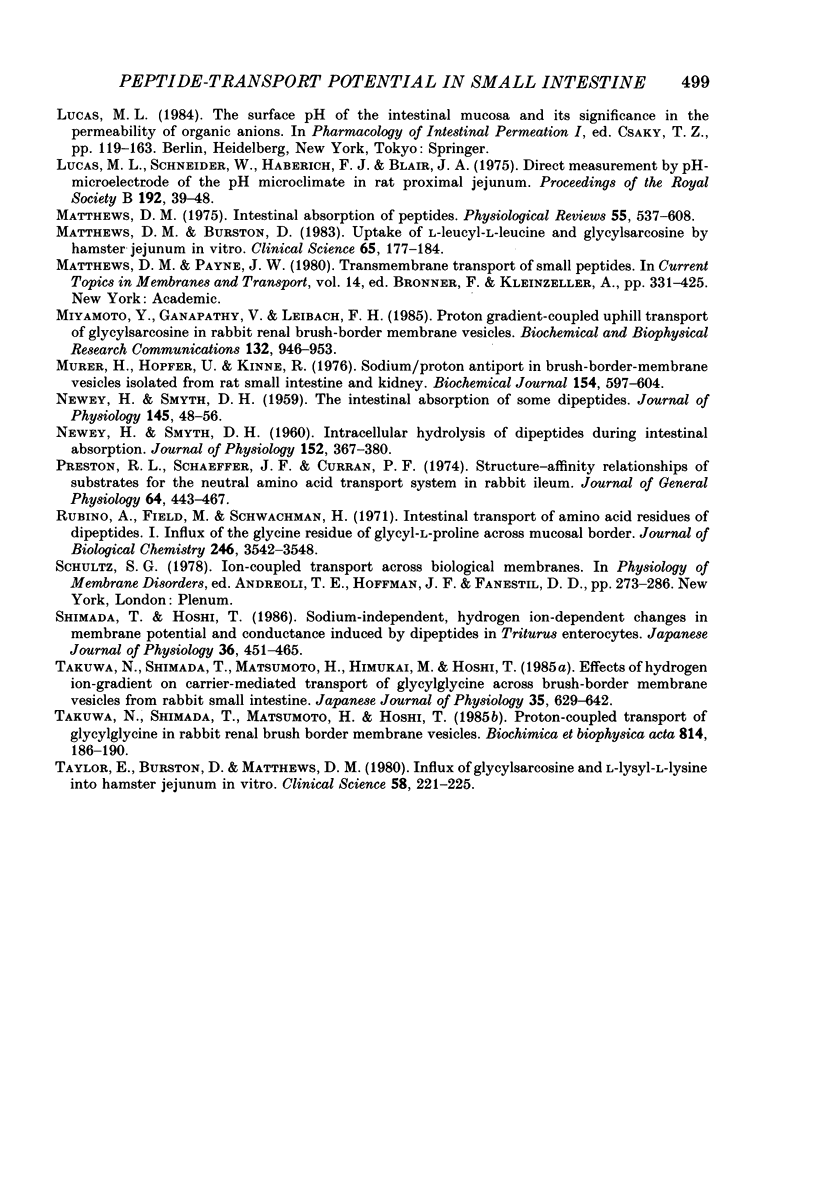
Selected References
These references are in PubMed. This may not be the complete list of references from this article.
- Addison J. M., Burston D., Matthews D. M. Evidence for active transport of the dipeptide glycylsarcosine by hamster jejunum in vitro. Clin Sci. 1972 Dec;43(6):907–911. doi: 10.1042/cs0430907. [DOI] [PubMed] [Google Scholar]
- Addison J. M., Burston D., Matthews D. M., Payne J. W., Wilkinson S. Proceedings: Evidence for active transport of the tripeptide glycylsarcosylsarcosine by hamster jejunum in vitro. Clin Sci Mol Med. 1974 Jun;46(6):30P–30P. [PubMed] [Google Scholar]
- Addison J. M., Matthews D. M., Burston D. Competition between carnosine and other peptides for transport by hamster jejunum in vitro. Clin Sci Mol Med. 1974 Jun;46(6):707–714. doi: 10.1042/cs0460707. [DOI] [PubMed] [Google Scholar]
- Berteloot A., Khan A. H., Ramaswamy K. Characteristics of dipeptide transport in normal and papain-treated brush border membrane vesicles from mouse intestine. II. Uptake of glycyl-L-leucine. Biochim Biophys Acta. 1982 Mar 23;686(1):47–54. doi: 10.1016/0005-2736(82)90150-x. [DOI] [PubMed] [Google Scholar]
- Boyd C. A., Ward M. R. A micro-electrode study of oligopeptide absorption by the small intestinal epithelium of Necturus maculosus. J Physiol. 1982 Mar;324:411–428. doi: 10.1113/jphysiol.1982.sp014121. [DOI] [PMC free article] [PubMed] [Google Scholar]
- Bramhall J. Use of the fluorescent weak acid dansylglycine to measure transmembrane proton concentration gradients. Biochemistry. 1986 Jul 1;25(13):3958–3962. doi: 10.1021/bi00361a033. [DOI] [PubMed] [Google Scholar]
- Cheeseman C. I., Parsons D. S. The role of some small peptides in the transfer of amino nitrogen across the wall of vascularly perfused intestine. J Physiol. 1976 Nov;262(2):459–476. doi: 10.1113/jphysiol.1976.sp011605. [DOI] [PMC free article] [PubMed] [Google Scholar]
- Das M., Radhakrishnan A. N. Studies on a wide-spectrum intestinal dipeptide uptake system in the monkey and in the human. Biochem J. 1975 Jan;146(1):133–139. doi: 10.1042/bj1460133. [DOI] [PMC free article] [PubMed] [Google Scholar]
- Ganapathy, Leibach F. H. Is intestinal peptide transport energized by a proton gradient? Am J Physiol. 1985 Aug;249(2 Pt 1):G153–G160. doi: 10.1152/ajpgi.1985.249.2.G153. [DOI] [PubMed] [Google Scholar]
- Ganapathy V., Burckhardt G., Leibach F. H. Characteristics of glycylsarcosine transport in rabbit intestinal brush-border membrane vesicles. J Biol Chem. 1984 Jul 25;259(14):8954–8959. [PubMed] [Google Scholar]
- Ganapathy V., Mendicino J. F., Leibach F. H. Transport of glycyl-L-proline into intestinal and renal brush border vesicles from rabbit. J Biol Chem. 1981 Jan 10;256(1):118–124. [PubMed] [Google Scholar]
- Ganapathy V., Mendicino J., Pashley D. H., Leibach F. H. Carrier-mediated transport of glycyl-L-proline in renal brush border vesicles. Biochem Biophys Res Commun. 1980 Dec 16;97(3):1133–1139. doi: 10.1016/0006-291x(80)91493-x. [DOI] [PubMed] [Google Scholar]
- Gunter-Smith P. J., Grasset E., Schultz S. G. Sodium-coupled amino acid and sugar transport by Necturus small intestine. An equivalent electrical circuit analysis of a rheogenic co-transport system. J Membr Biol. 1982;66(1):25–39. doi: 10.1007/BF01868479. [DOI] [PubMed] [Google Scholar]
- Hajjar J. J., Curran P. F. Characteristics of the amino acid transport system in the mucosal border of rabbit ileum. J Gen Physiol. 1970 Dec;56(6):673–691. doi: 10.1085/jgp.56.6.673. [DOI] [PMC free article] [PubMed] [Google Scholar]
- Hilden S., Sacktor B. Potential-dependent D-glucose uptake by renal brush border membrane vesicles in the absence of sodium. Am J Physiol. 1982 Apr;242(4):F340–F345. doi: 10.1152/ajprenal.1982.242.4.F340. [DOI] [PubMed] [Google Scholar]
- Himukai M., Hoshi T. Mechanisms of glycyl-L-leucine uptake by guinea-pig small intestine: relative importance of intact-peptide transport. J Physiol. 1980 May;302:155–169. doi: 10.1113/jphysiol.1980.sp013235. [DOI] [PMC free article] [PubMed] [Google Scholar]
- Himukai M., Kameyama A., Hoshi T. Interaction of glycylglycine and Na+ at the mucosal border of guinea-pig small intestine. A non-mutual stimulation of transport. Biochim Biophys Acta. 1983 Aug 10;732(3):659–667. doi: 10.1016/0005-2736(83)90244-4. [DOI] [PubMed] [Google Scholar]
- Himukai M. The characteristics of carnosine transport and carnosine-induced electrical phenomena by the everted intestine of guinea pig. Jpn J Physiol. 1985;35(6):945–952. doi: 10.2170/jjphysiol.35.945. [DOI] [PubMed] [Google Scholar]
- Hoshi T., Komatsu Y. Sugar-evoked potential in isolated toad intestine. Jpn J Physiol. 1968 Aug 15;18(4):508–519. doi: 10.2170/jjphysiol.18.508. [DOI] [PubMed] [Google Scholar]
- Hoshi T. Proton-coupled transport of organic solutes in animal cell membranes and its relation to Na+ transport. Jpn J Physiol. 1985;35(2):179–191. doi: 10.2170/jjphysiol.35.179. [DOI] [PubMed] [Google Scholar]
- Hoshi T., Suzuki Y., Kusachi T., Igarashi Y. Interrelationship between sugar-evoked increases in transmural potential difference and sugar influxes across the mucosal border in the small intestine. Tohoku J Exp Med. 1976 Jul;119(3):201–209. doi: 10.1620/tjem.119.201. [DOI] [PubMed] [Google Scholar]
- Hoshi T., Takuwa N., Abe M., Tajima A. Hydrogen ion-coupled transport of D-glucose by phlorizin-sensitive sugar carrier in intestinal brush-border membranes. Biochim Biophys Acta. 1986 Oct 23;861(3):483–488. doi: 10.1016/0005-2736(86)90458-x. [DOI] [PubMed] [Google Scholar]
- Jentsch T. J., Janicke I., Sorgenfrei D., Keller S. K., Wiederholt M. The regulation of intracellular pH in monkey kidney epithelial cells (BSC-1). Roles of Na+/H+ antiport, Na+-HCO3(-)-(NaCO3-) symport, and Cl-/HCO3- exchange. J Biol Chem. 1986 Sep 15;261(26):12120–12127. [PubMed] [Google Scholar]
- Knickelbein R., Aronson P. S., Atherton W., Dobbins J. W. Sodium and chloride transport across rabbit ileal brush border. I. Evidence for Na-H exchange. Am J Physiol. 1983 Oct;245(4):G504–G510. doi: 10.1152/ajpgi.1983.245.4.G504. [DOI] [PubMed] [Google Scholar]
- Kohn P. G., Smyth D. H., Wright E. M. Effects of amino acids, dipeptides and disaccharides on the electric potential across rat small intestine. J Physiol. 1968 Jun;196(3):723–746. doi: 10.1113/jphysiol.1968.sp008533. [DOI] [PMC free article] [PubMed] [Google Scholar]
- Lucas M. L., Schneider W., Haberich F. J., Blair J. A. Direct measurement by pH-microelectrode of the pH microclimate in rat proximal jejunum. Proc R Soc Lond B Biol Sci. 1975 Dec 31;192(1106):39–48. doi: 10.1098/rspb.1975.0150. [DOI] [PubMed] [Google Scholar]
- Matthews D. M., Burston D. Uptake of L-leucyl-L-leucine and glycylsarcosine by hamster jejunum in vitro. Clin Sci (Lond) 1983 Aug;65(2):177–184. doi: 10.1042/cs0650177. [DOI] [PubMed] [Google Scholar]
- Matthews D. M. Intestinal absorption of peptides. Physiol Rev. 1975 Oct;55(4):537–608. doi: 10.1152/physrev.1975.55.4.537. [DOI] [PubMed] [Google Scholar]
- Miyamoto Y., Ganapathy V., Leibach F. H. Proton gradient-coupled uphill transport of glycylsarcosine in rabbit renal brush-border membrane vesicles. Biochem Biophys Res Commun. 1985 Nov 15;132(3):946–953. doi: 10.1016/0006-291x(85)91899-6. [DOI] [PubMed] [Google Scholar]
- Murer H., Hopfer U., Kinne R. Sodium/proton antiport in brush-border-membrane vesicles isolated from rat small intestine and kidney. Biochem J. 1976 Mar 15;154(3):597–604. [PMC free article] [PubMed] [Google Scholar]
- NEWEY H., SMYTH D. H. Intracellular hydrolysis of dipeptides during intestinal absorption. J Physiol. 1960 Jul;152:367–380. doi: 10.1113/jphysiol.1960.sp006493. [DOI] [PMC free article] [PubMed] [Google Scholar]
- NEWEY H., SMYTH D. H. The intestinal absorption of some dipeptides. J Physiol. 1959 Jan 28;145(1):48–56. doi: 10.1113/jphysiol.1959.sp006125. [DOI] [PMC free article] [PubMed] [Google Scholar]
- Preston R. L., Schaeffer J. F., Curran P. F. Structure-affinity relationships of substrates for the neutral amino acid transport system in rabbit ileum. J Gen Physiol. 1974 Oct;64(4):443–467. doi: 10.1085/jgp.64.4.443. [DOI] [PMC free article] [PubMed] [Google Scholar]
- Rubino A., Field M., Shwachman H. Intestinal transport of amino acid residues of dipeptides. I. Influx of the glycine residue of glycyl-L-proline across mucosal border. J Biol Chem. 1971 Jun 10;246(11):3542–3548. [PubMed] [Google Scholar]
- Shimada T., Hoshi T. Sodium-independent, hydrogen ion-dependent changes in membrane potential and conductance induced by dipeptides in Triturus enterocytes. Jpn J Physiol. 1986;36(3):451–465. doi: 10.2170/jjphysiol.36.451. [DOI] [PubMed] [Google Scholar]
- Takuwa N., Shimada T., Matsumoto H., Himukai M., Hoshi T. Effect of hydrogen ion-gradient on carrier-mediated transport of glycylglycine across brush border membrane vesicles from rabbit small intestine. Jpn J Physiol. 1985;35(4):629–642. doi: 10.2170/jjphysiol.35.629. [DOI] [PubMed] [Google Scholar]
- Takuwa N., Shimada T., Matsumoto H., Hoshi T. Proton-coupled transport of glycylglycine in rabbit renal brush-border membrane vesicles. Biochim Biophys Acta. 1985 Mar 28;814(1):186–190. doi: 10.1016/0005-2736(85)90435-3. [DOI] [PubMed] [Google Scholar]
- Taylor E., Burston D., Matthews D. M. Influx of glycylsarcosine and L-lysyl-L-lysine into hamster jejunum in vitro. Clin Sci (Lond) 1980 Mar;58(3):221–225. doi: 10.1042/cs0580221. [DOI] [PubMed] [Google Scholar]