Abstract
1. The effects of phosphate and protons on the mechanics and energetics of muscle contraction have been investigated using glycerinated rabbit psoas muscle. 2. Fibres were fully activated by addition of Ca2+ (pCa 4-5) at 10 degrees C. The velocities of contraction were measured in isotonic load clamps, and the velocities of unloaded fibres were measured by applying a series of step changes in fibre length. Fibre ATPase activity was monitored using an enzyme system to couple ADP production to reduced nicotinamide-adenine dinucleotide (NADH) and measuring the depletion of NADH by optical density. 3. At pH 7.0 and 3 mM-phosphate, isometric tension (P0) was 13.2 +/- 0.9 N/cm (mean +/- S.E.M., n = 10 observations), the maximum contraction velocity (Vmax) was 1.63 +/- 0.05 lengths/s (n = 5) and the ATPase activity was 1.27 +/- 0.12 s-1 myosin head-1 (n = 35). Increasing phosphate from 3 to 20 mM at pH 7.0 does not affect Vmax, causes a small decrease in the ATPase activity (15-20%) and decreases P0 by approximately 20%. Changing pH from 7 to 6 at 3 mM-phosphate decreases P0 by 45% and both Vmax and ATPase activity by 25-30%. The effects of changing both pH and phosphate were approximately additive for all parameters measured. The inhibition of these parameters by low pH and high concentration of phosphate was reversible. 4. The force-velocity relation was fitted by the Hill equation using a non-linear least-squares method. The value of the parameter which describes the curvature, a/P0, was 0.20. The curvature of the force-velocity relation was not changed by addition of phosphate or by changes in pH. 5. These data provide information on both the kinetics of the actomyosin interaction and on the process of muscle fatigue. The data are consistent with models of cross-bridge kinetics in which phosphate is released within the powerstroke in a step involving a rapid equilibrium between states. The inhibition by protons is more complex, and may involve less specific effects on protein structure. 6. During moderate fatigue of living skeletal muscle, MgATP concentration is known to remain approximately constant at 4 mM, phosphate to increase from 3 to 20 mM, and protons from 0.1 to 1 microM. The data suggest that much of the inhibition of P0 observed during moderate fatigue can be explained by the increased levels of phosphate and protons, and that much of the inhibition of fibre Vmax and ATPase activity can be explained by the increase in protons.
Full text
PDF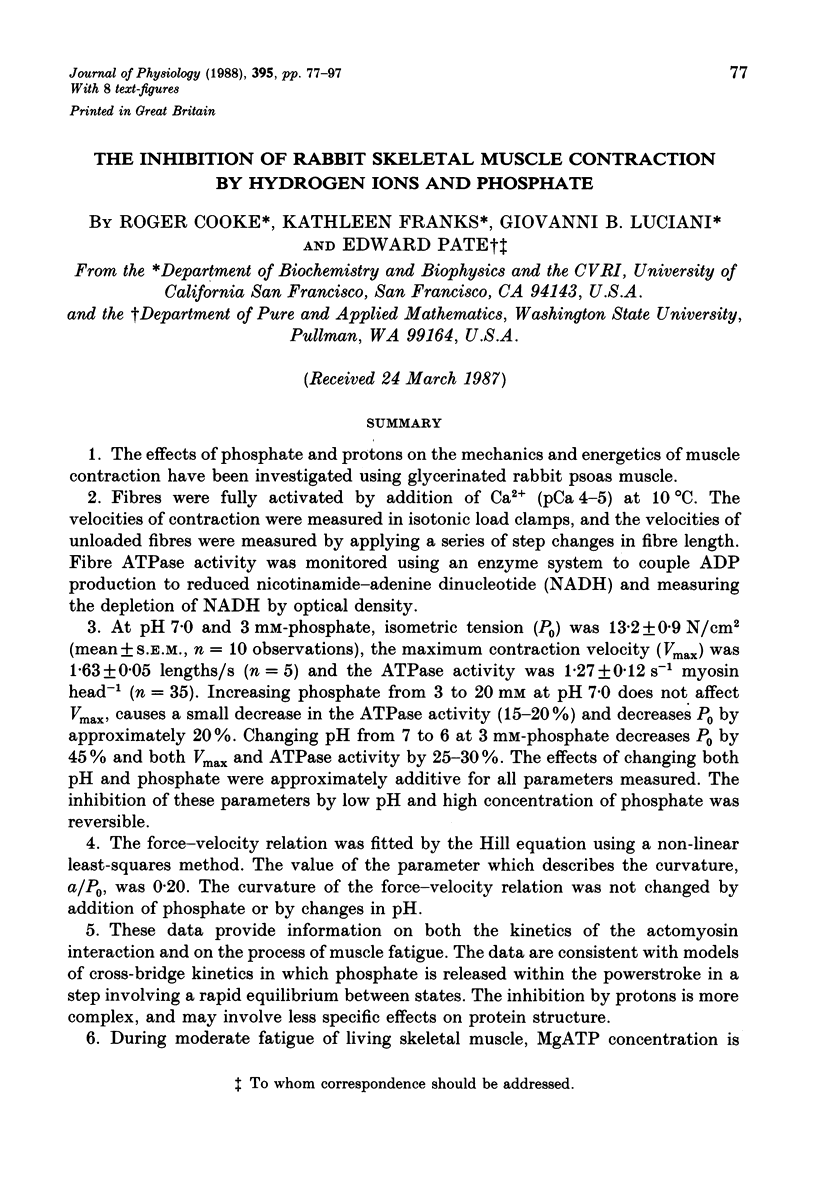
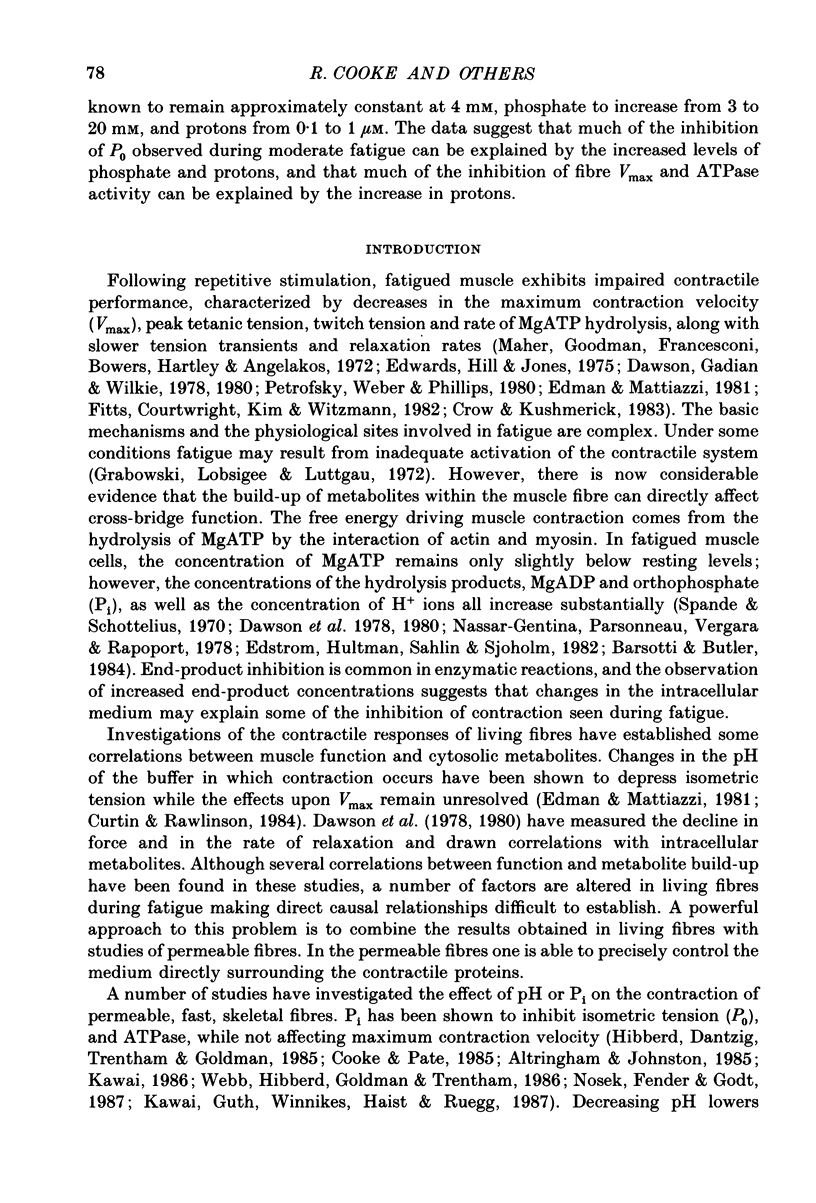
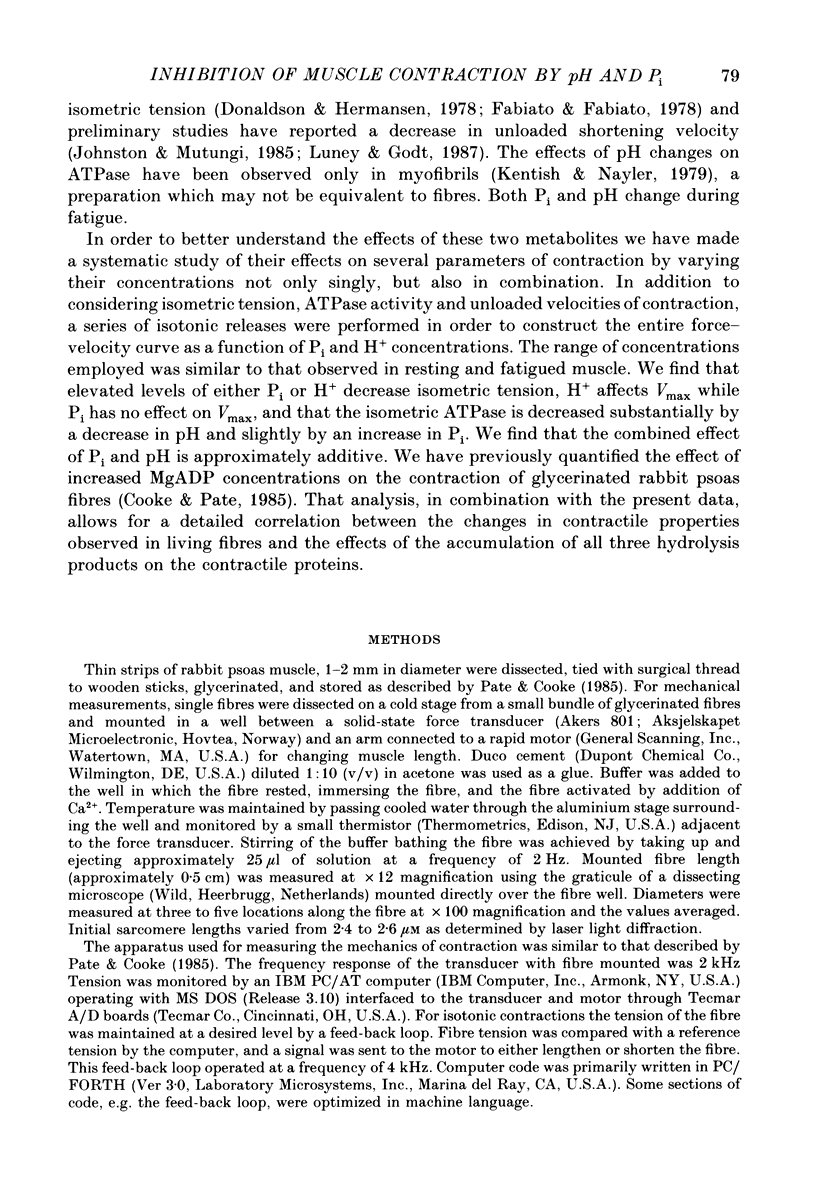
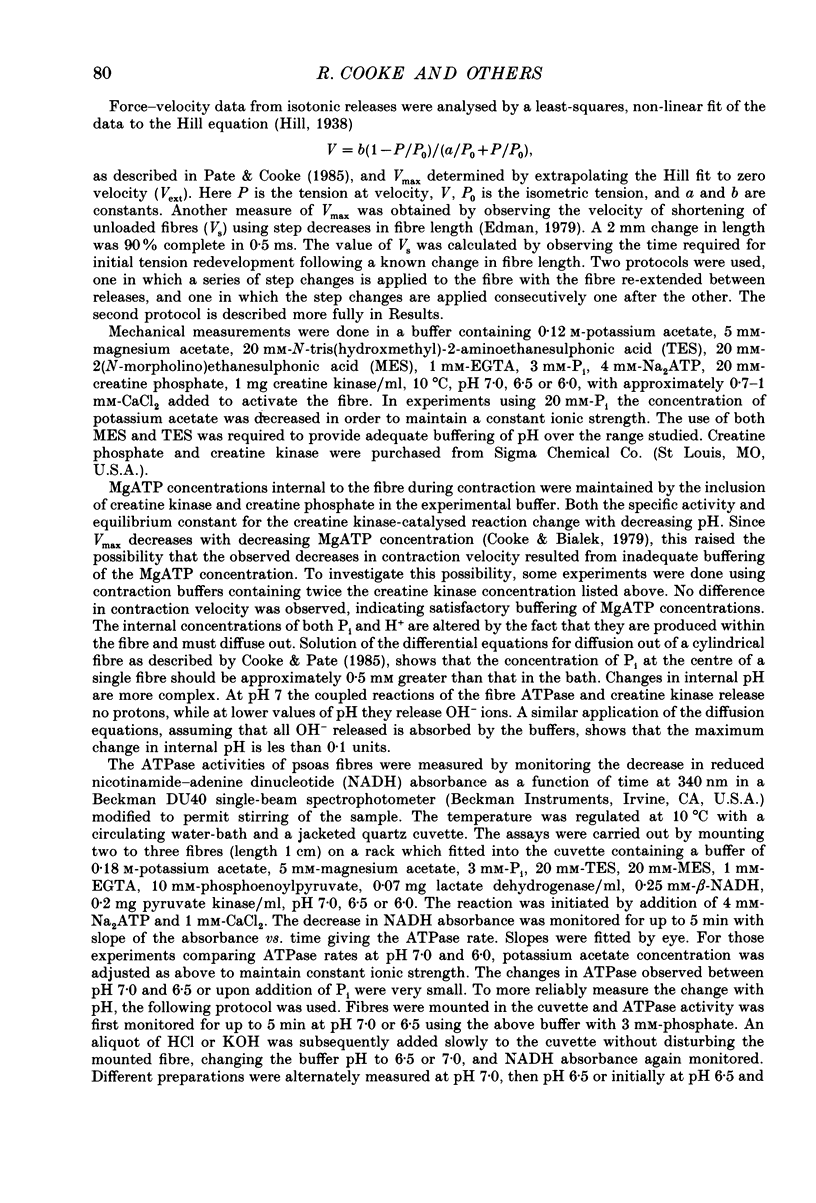
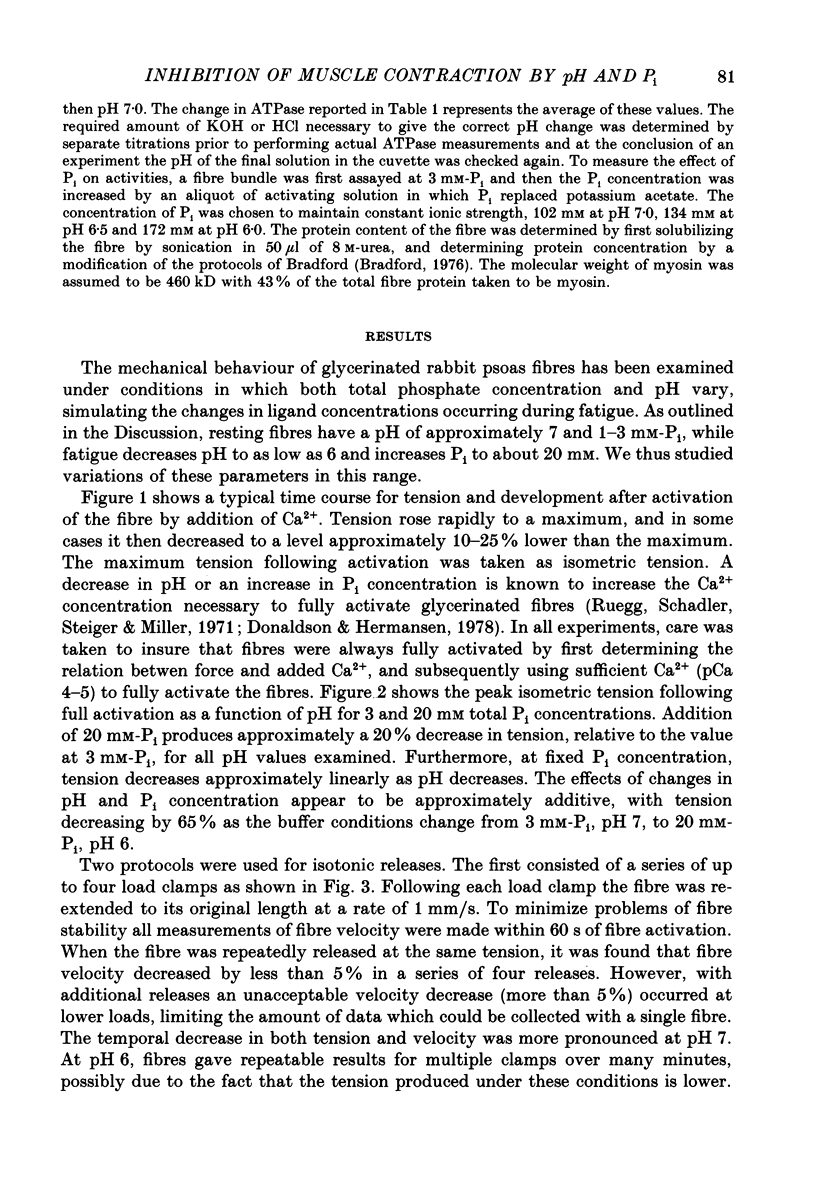
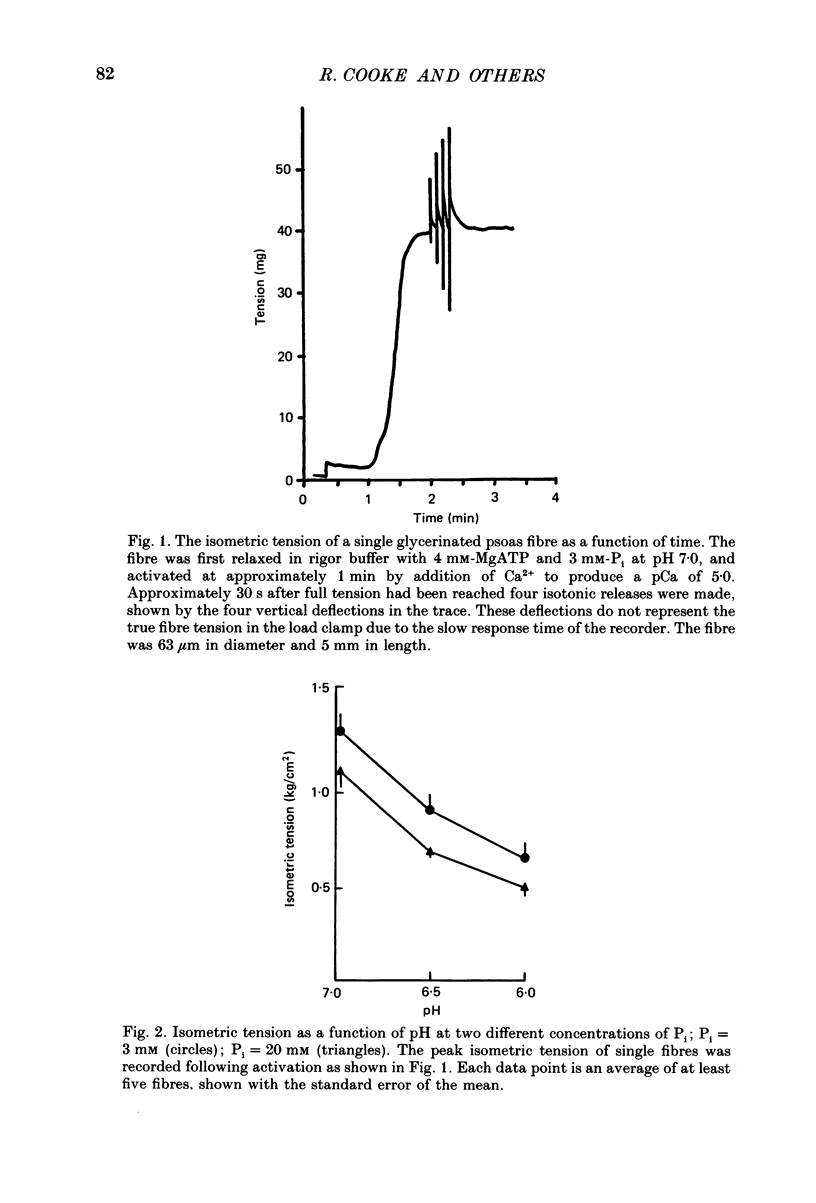
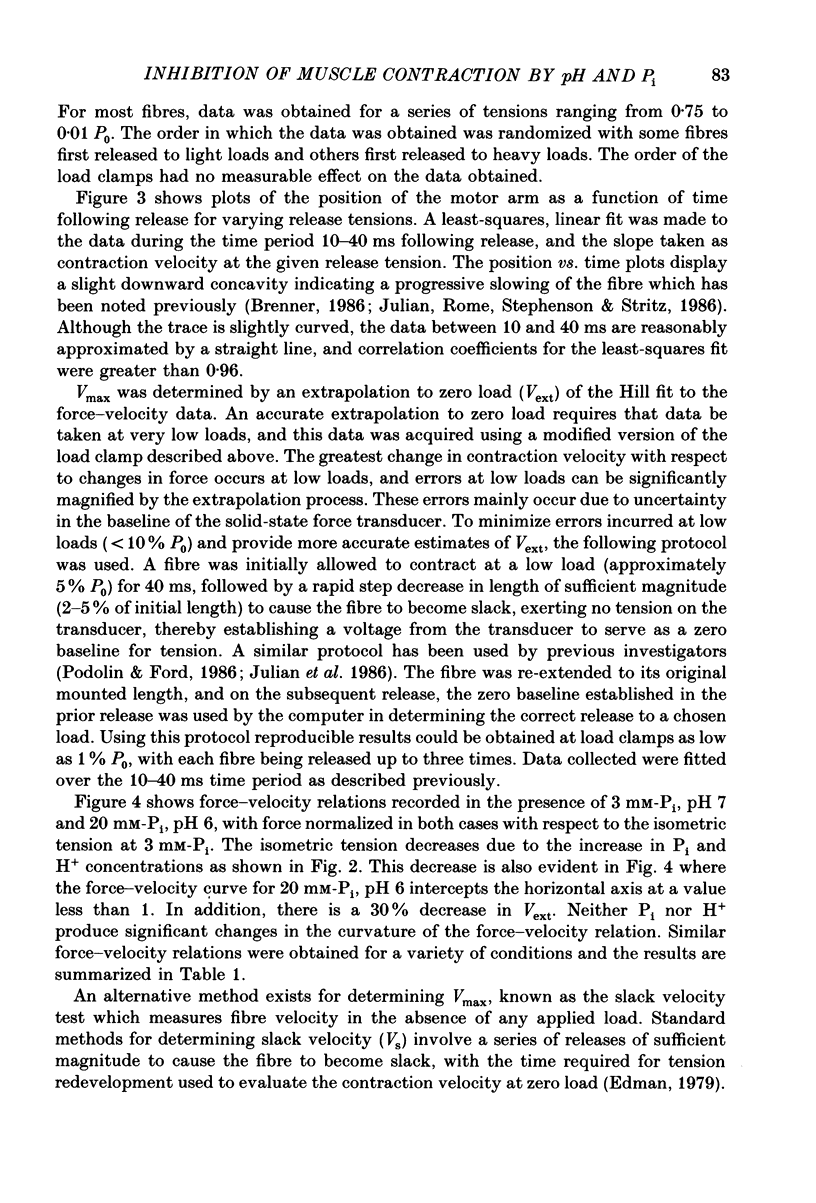
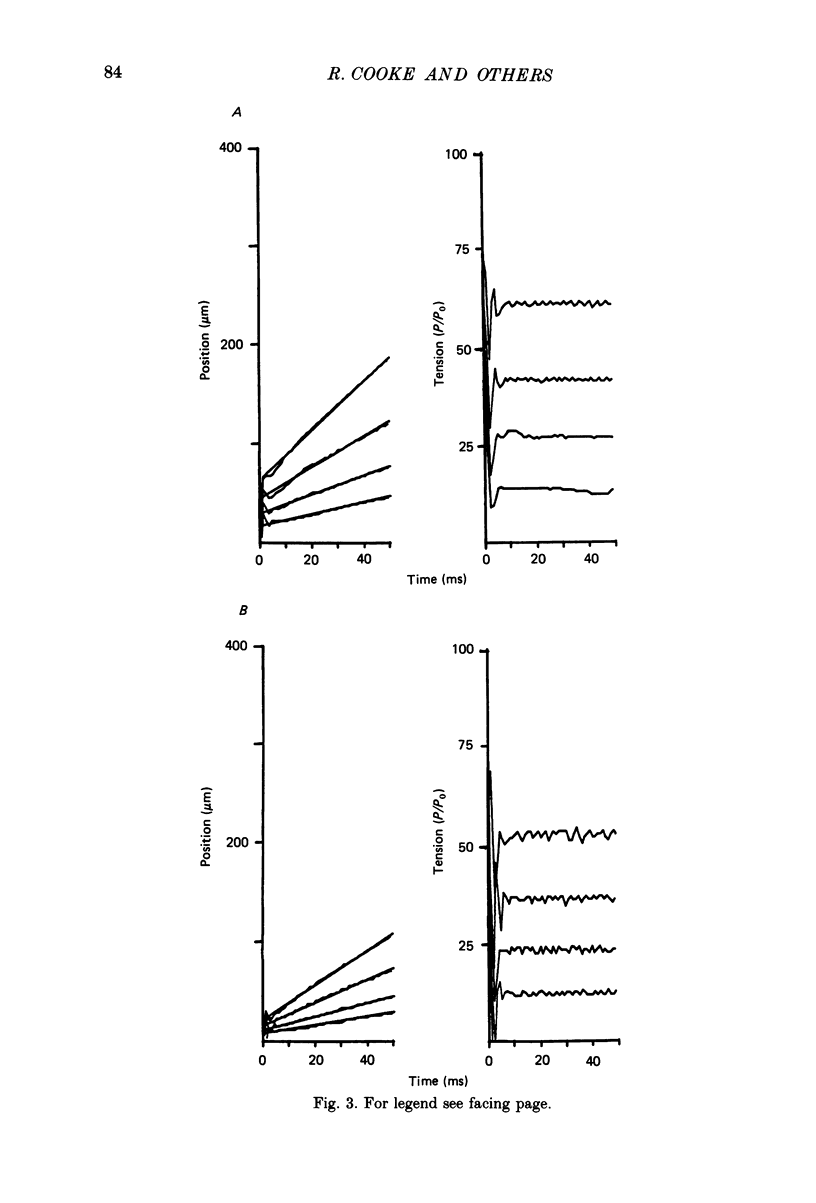
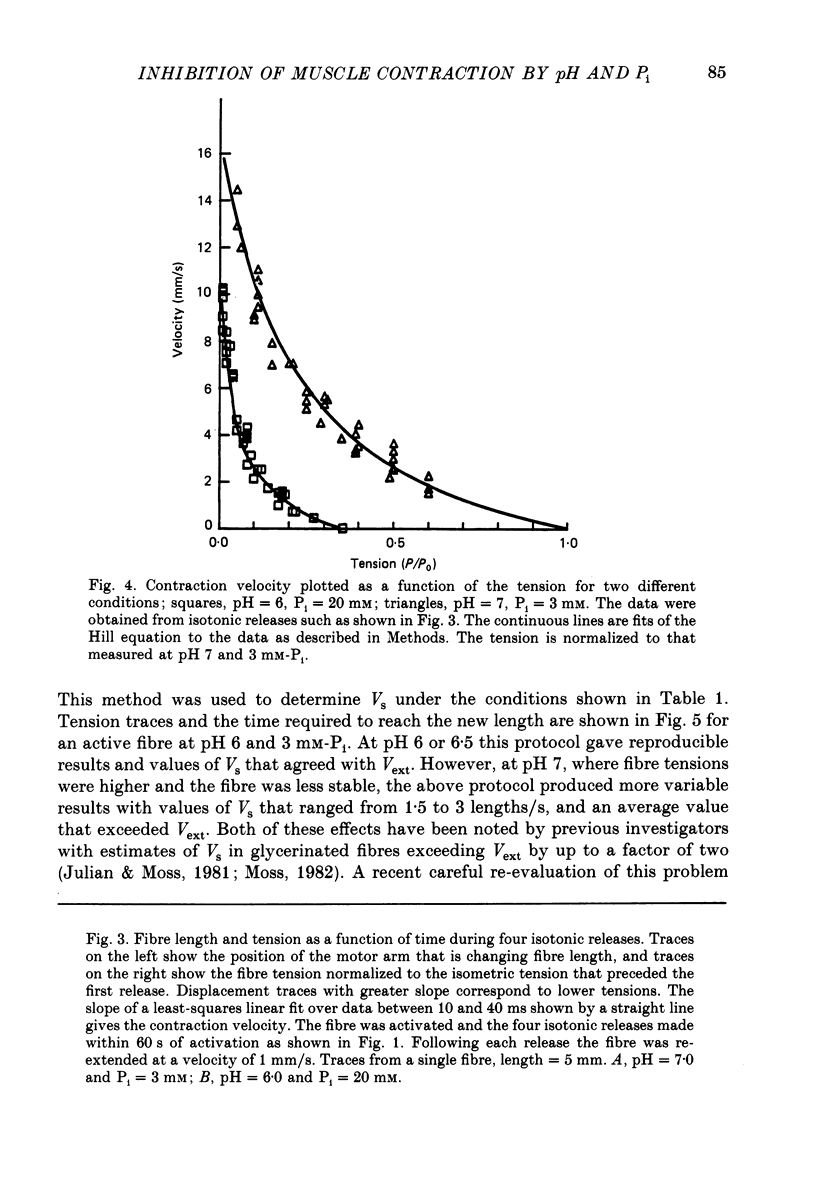
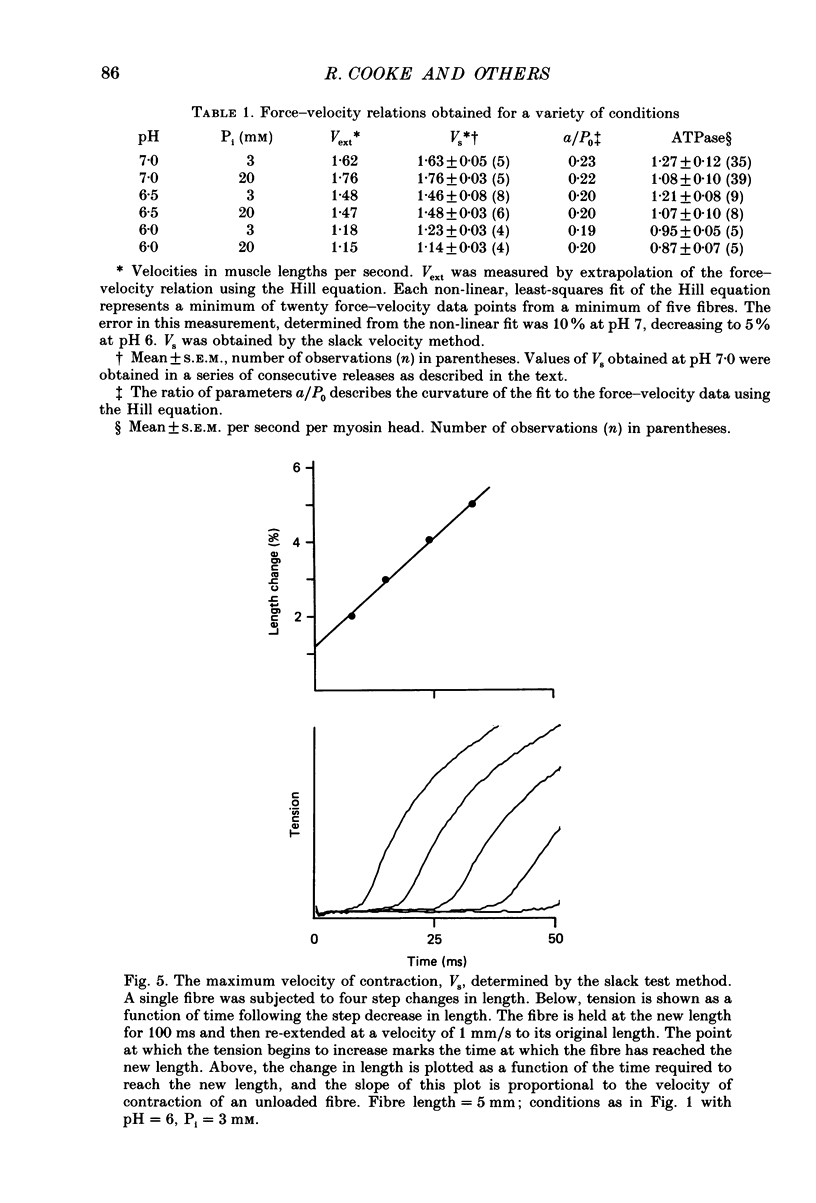
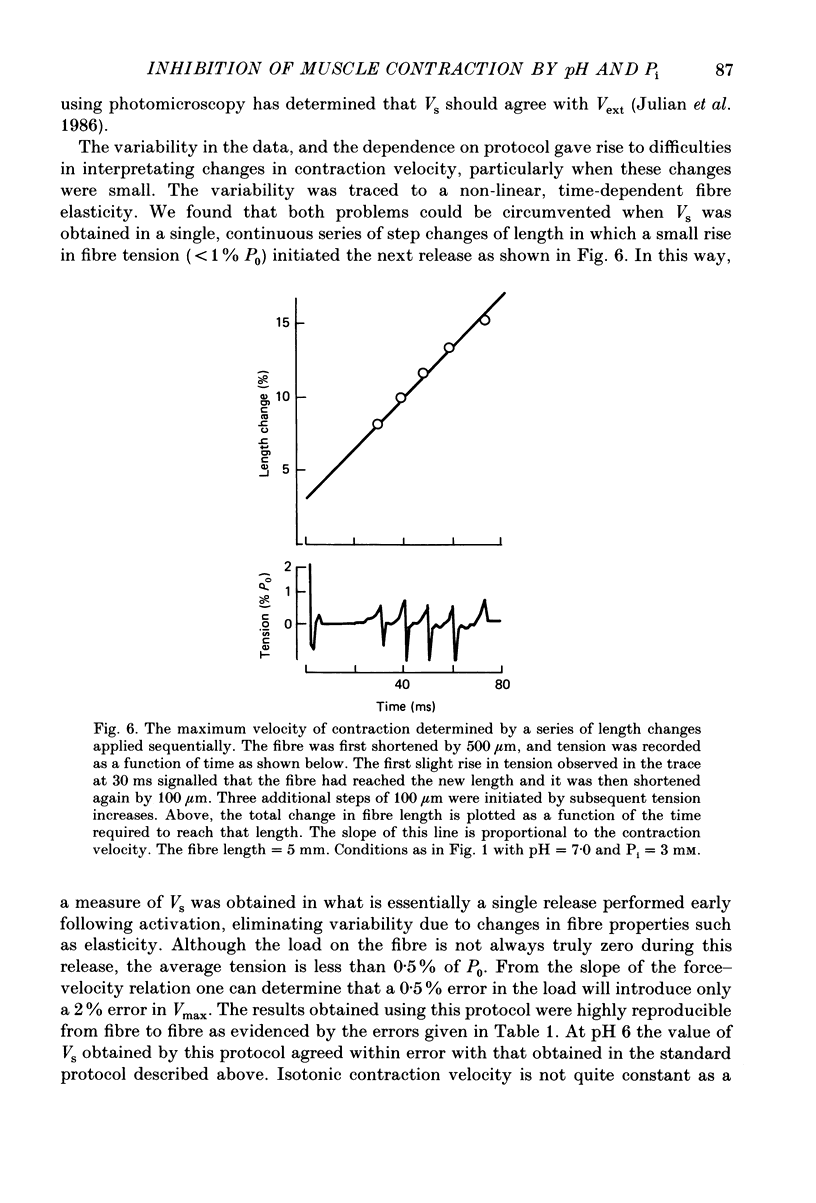
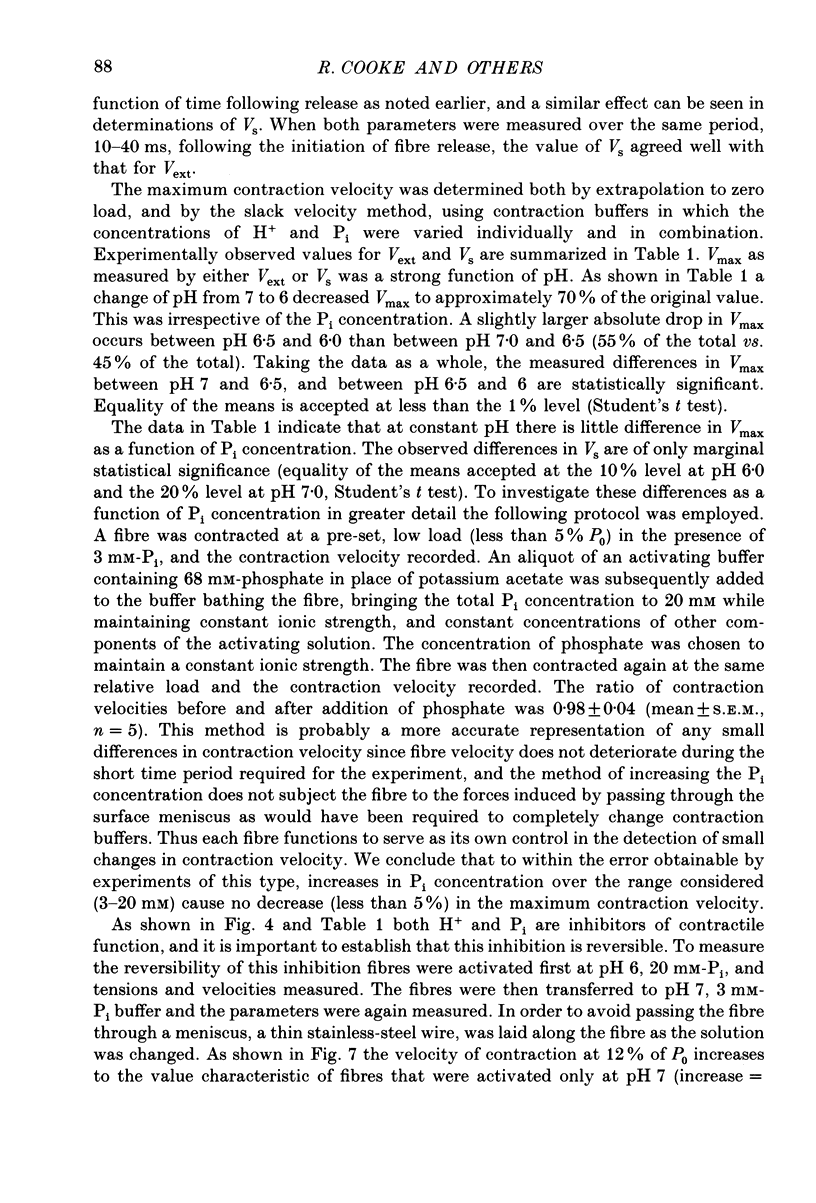
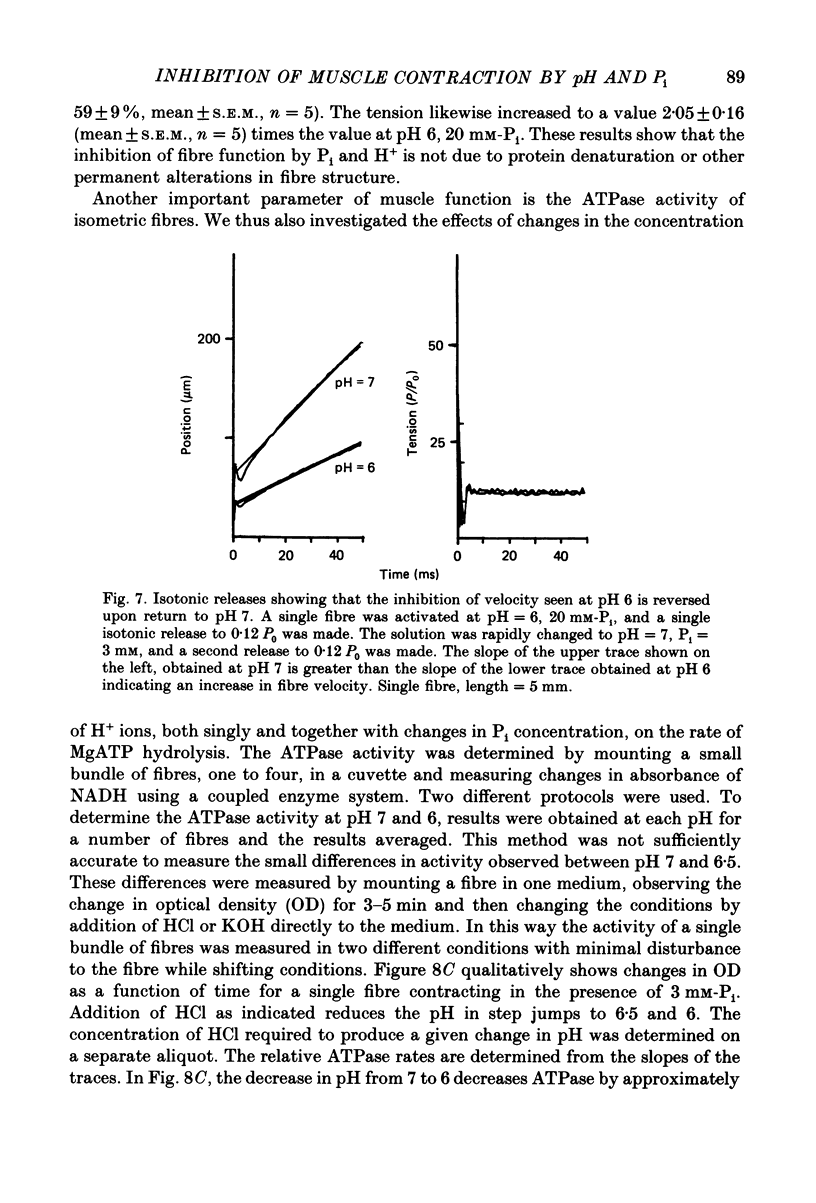
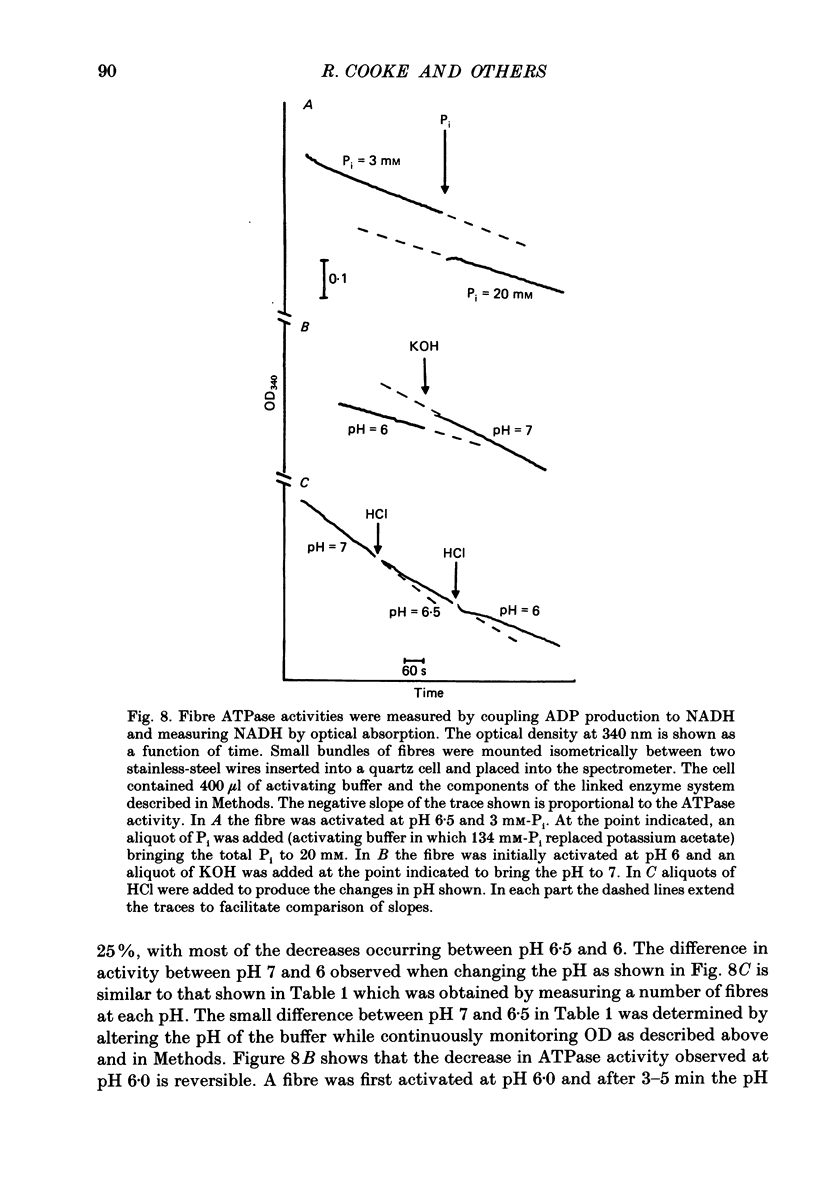
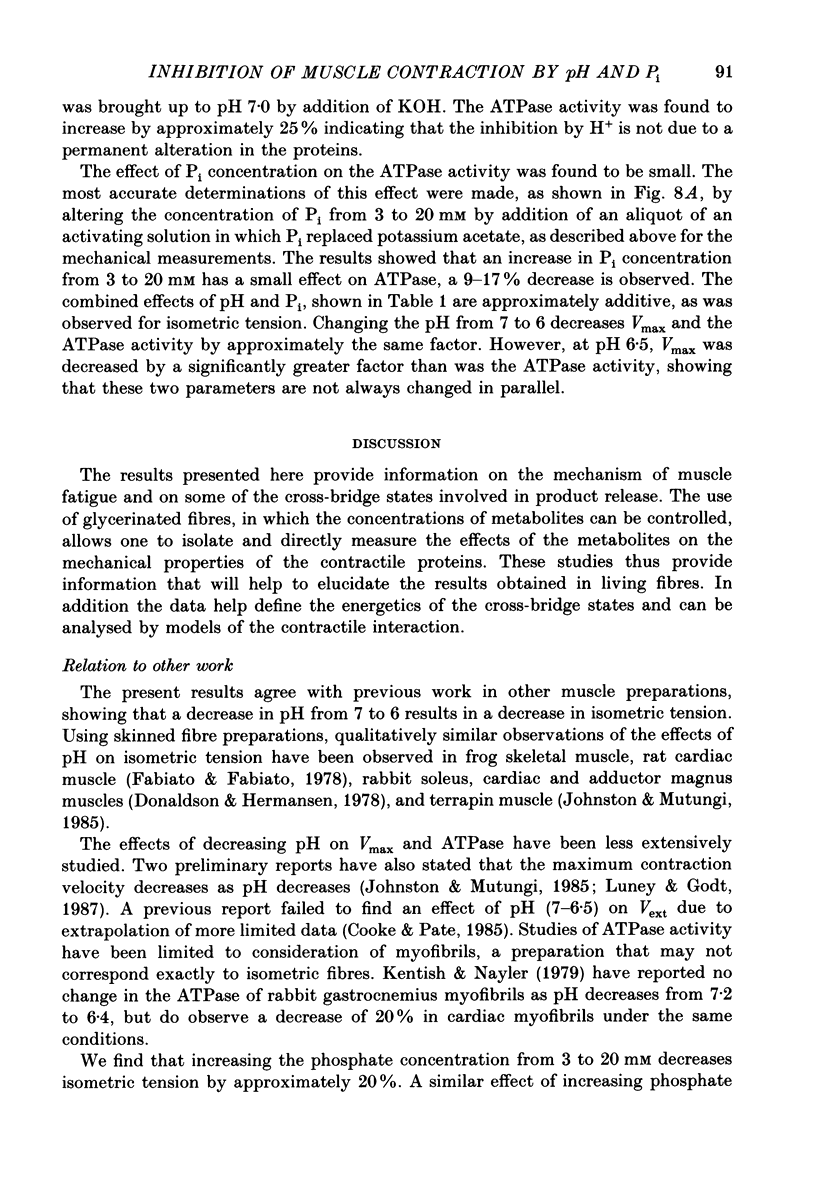
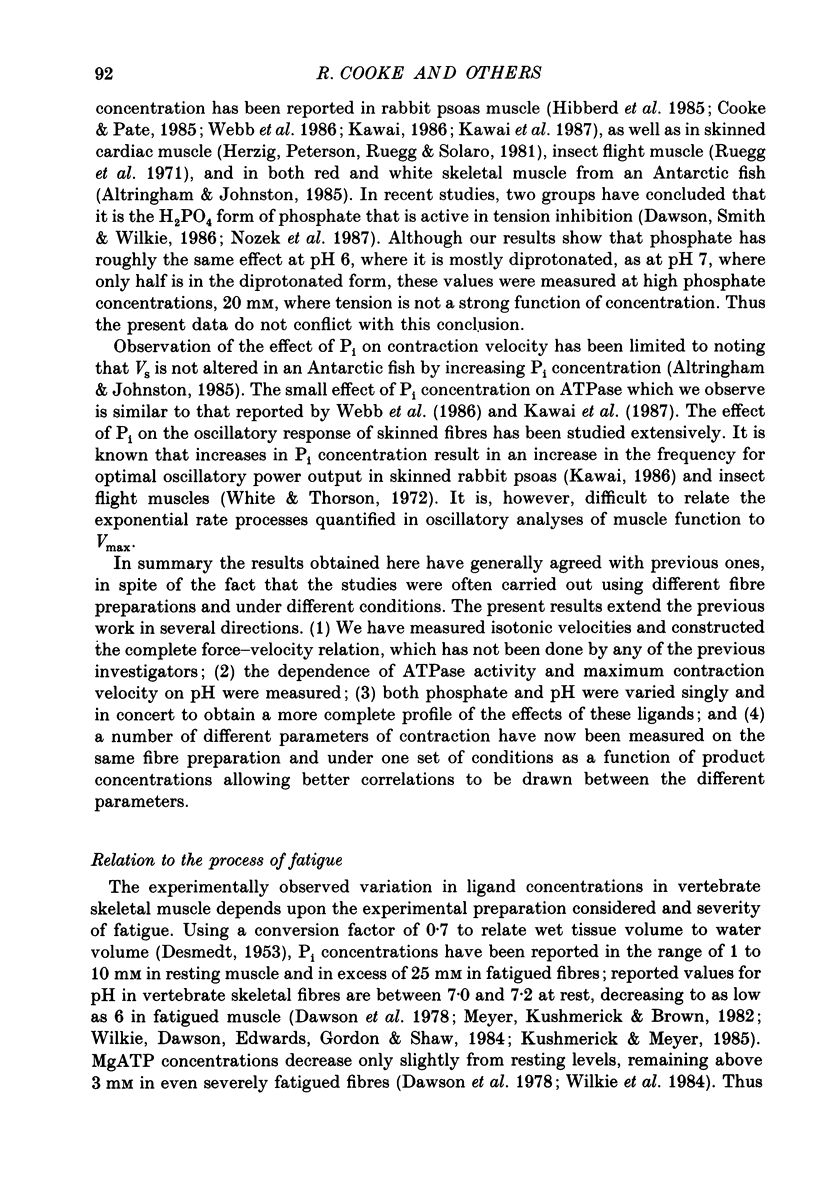
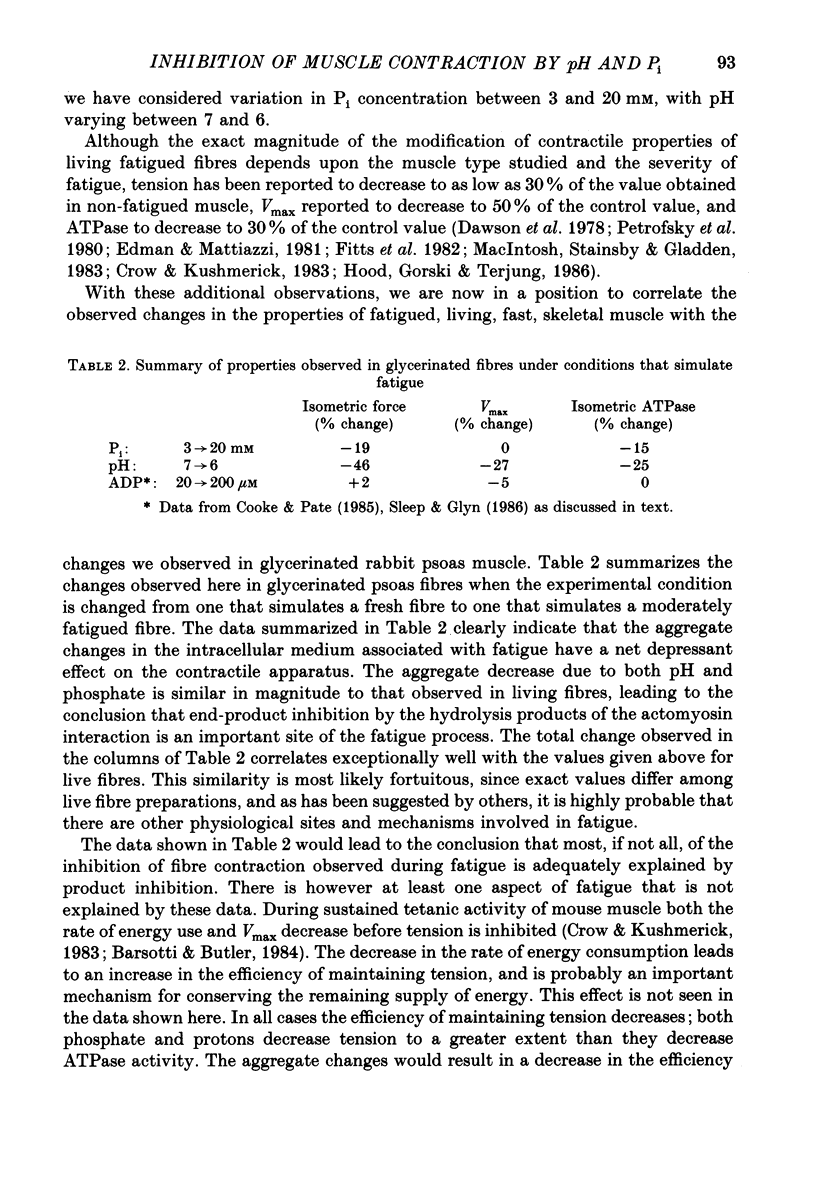
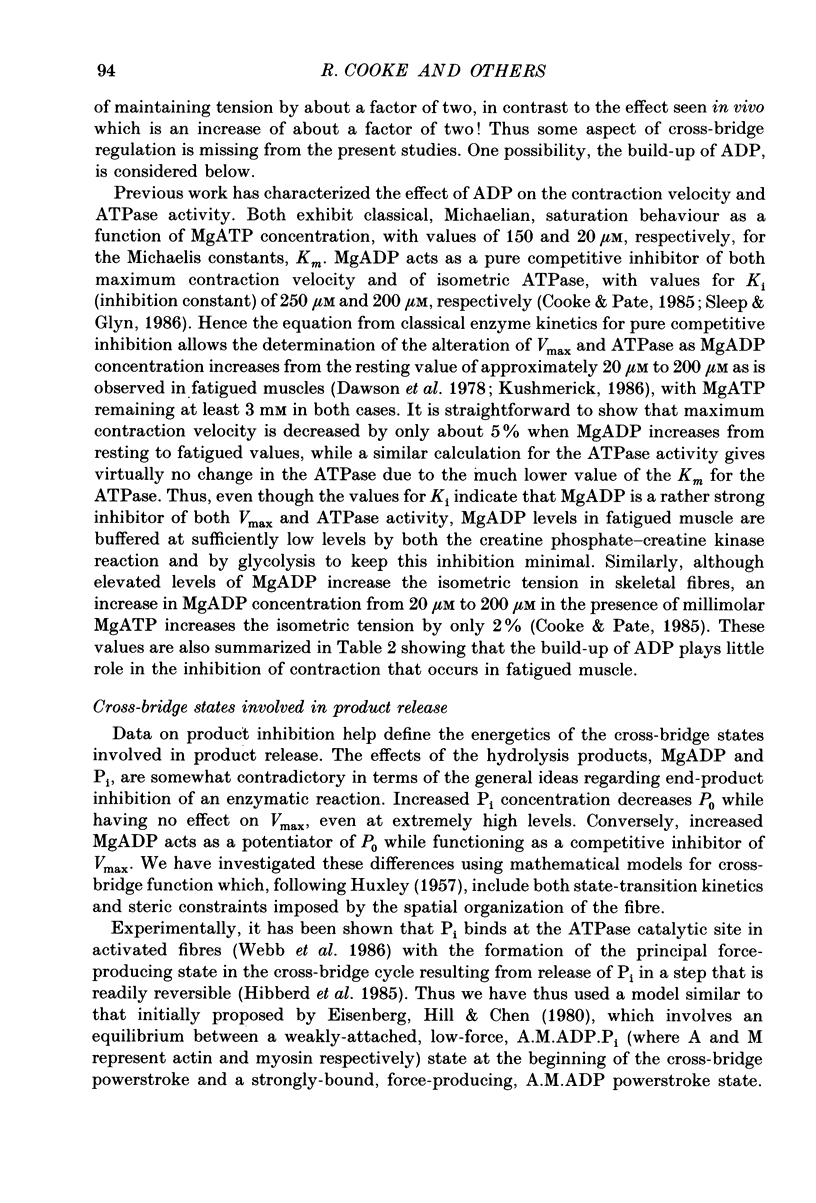
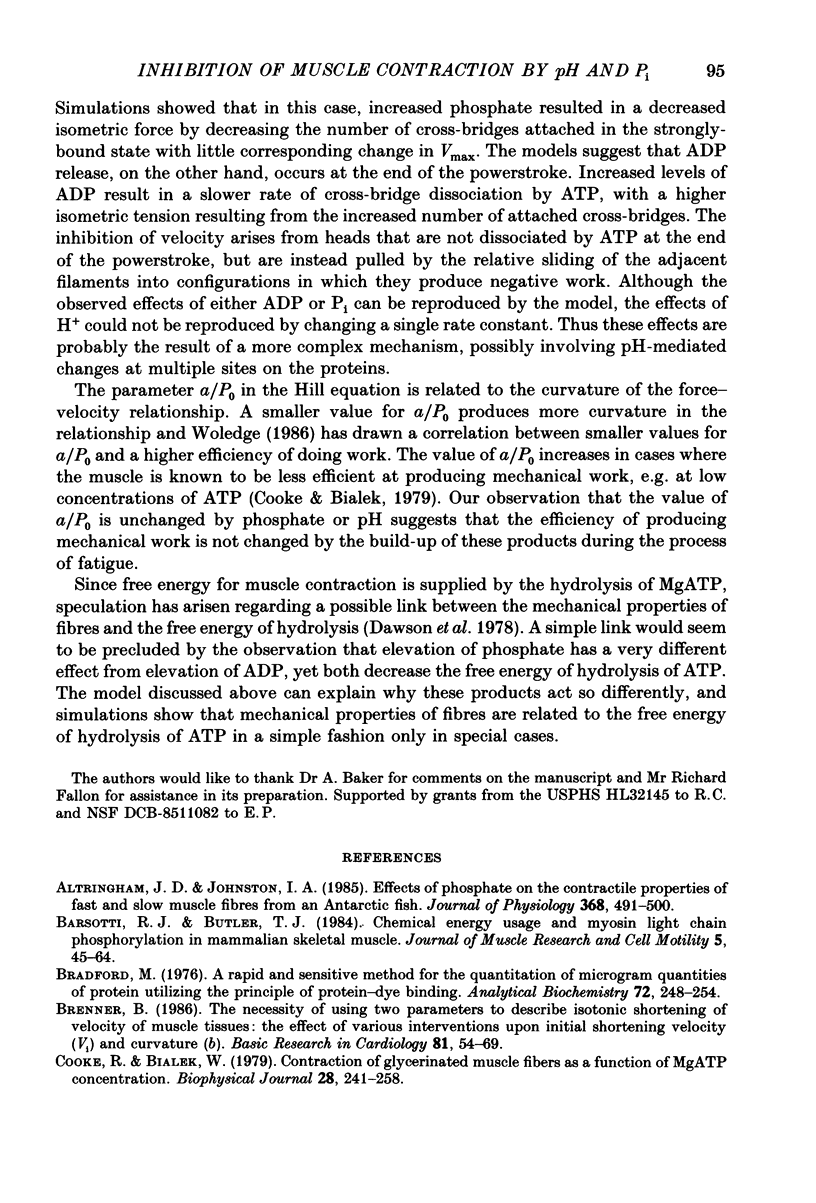
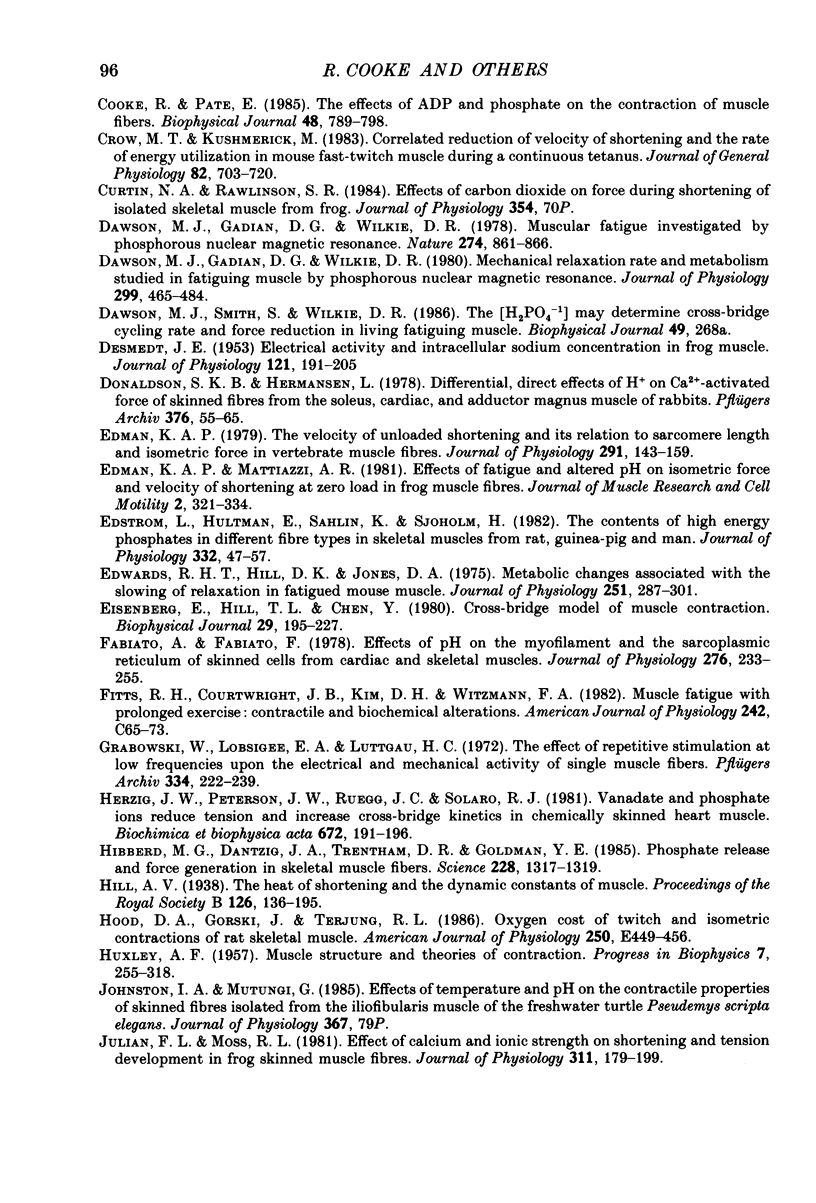
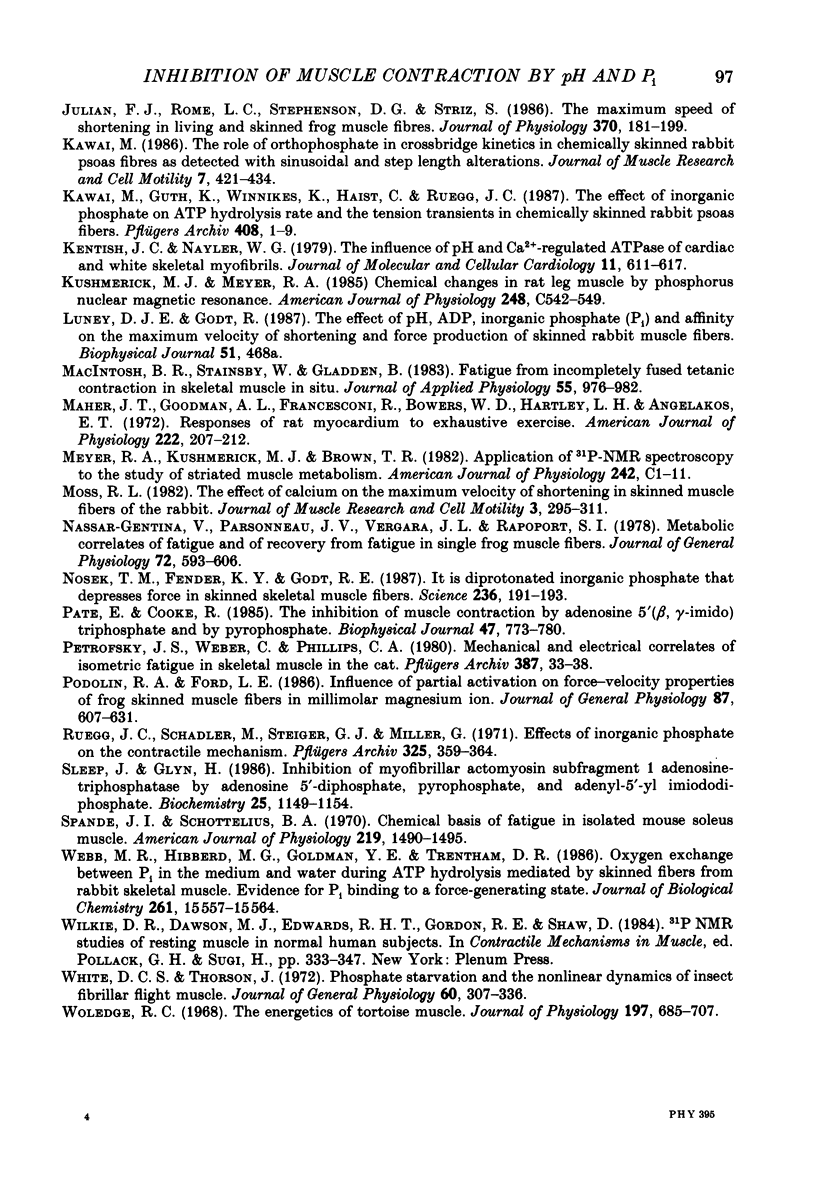
Selected References
These references are in PubMed. This may not be the complete list of references from this article.
- Altringham J. D., Johnston I. A. Effects of phosphate on the contractile properties of fast and slow muscle fibres from an Antarctic fish. J Physiol. 1985 Nov;368:491–500. doi: 10.1113/jphysiol.1985.sp015871. [DOI] [PMC free article] [PubMed] [Google Scholar]
- Barsotti R. J., Butler T. M. Chemical energy usage and myosin light chain phosphorylation in mammalian skeletal muscle. J Muscle Res Cell Motil. 1984 Feb;5(1):45–64. doi: 10.1007/BF00713151. [DOI] [PubMed] [Google Scholar]
- Bradford M. M. A rapid and sensitive method for the quantitation of microgram quantities of protein utilizing the principle of protein-dye binding. Anal Biochem. 1976 May 7;72:248–254. doi: 10.1006/abio.1976.9999. [DOI] [PubMed] [Google Scholar]
- Brenner B. The necessity of using two parameters to describe isotonic shortening velocity of muscle tissues: the effect of various interventions upon initial shortening velocity (vi) and curvature (b). Basic Res Cardiol. 1986 Jan-Feb;81(1):54–69. doi: 10.1007/BF01907427. [DOI] [PubMed] [Google Scholar]
- Cooke R., Bialek W. Contraction of glycerinated muscle fibers as a function of the ATP concentration. Biophys J. 1979 Nov;28(2):241–258. doi: 10.1016/S0006-3495(79)85174-7. [DOI] [PMC free article] [PubMed] [Google Scholar]
- Cooke R., Pate E. The effects of ADP and phosphate on the contraction of muscle fibers. Biophys J. 1985 Nov;48(5):789–798. doi: 10.1016/S0006-3495(85)83837-6. [DOI] [PMC free article] [PubMed] [Google Scholar]
- Crow M. T., Kushmerick M. J. Correlated reduction of velocity of shortening and the rate of energy utilization in mouse fast-twitch muscle during a continuous tetanus. J Gen Physiol. 1983 Nov;82(5):703–720. doi: 10.1085/jgp.82.5.703. [DOI] [PMC free article] [PubMed] [Google Scholar]
- DESMEDT J. E. Electrical activity and intracellular sodium concentration in frog muscle. J Physiol. 1953 Jul;121(1):191–205. doi: 10.1113/jphysiol.1953.sp004940. [DOI] [PMC free article] [PubMed] [Google Scholar]
- Dawson M. J., Gadian D. G., Wilkie D. R. Mechanical relaxation rate and metabolism studied in fatiguing muscle by phosphorus nuclear magnetic resonance. J Physiol. 1980 Feb;299:465–484. doi: 10.1113/jphysiol.1980.sp013137. [DOI] [PMC free article] [PubMed] [Google Scholar]
- Dawson M. J., Gadian D. G., Wilkie D. R. Muscular fatigue investigated by phosphorus nuclear magnetic resonance. Nature. 1978 Aug 31;274(5674):861–866. doi: 10.1038/274861a0. [DOI] [PubMed] [Google Scholar]
- Donaldson S. K., Hermansen L., Bolles L. Differential, direct effects of H+ on Ca2+ -activated force of skinned fibers from the soleus, cardiac and adductor magnus muscles of rabbits. Pflugers Arch. 1978 Aug 25;376(1):55–65. doi: 10.1007/BF00585248. [DOI] [PubMed] [Google Scholar]
- Edman K. A., Mattiazzi A. R. Effects of fatigue and altered pH on isometric force and velocity of shortening at zero load in frog muscle fibres. J Muscle Res Cell Motil. 1981 Sep;2(3):321–334. doi: 10.1007/BF00713270. [DOI] [PubMed] [Google Scholar]
- Edman K. A. The velocity of unloaded shortening and its relation to sarcomere length and isometric force in vertebrate muscle fibres. J Physiol. 1979 Jun;291:143–159. doi: 10.1113/jphysiol.1979.sp012804. [DOI] [PMC free article] [PubMed] [Google Scholar]
- Edström L., Hultman E., Sahlin K., Sjöholm H. The contents of high-energy phosphates in different fibre types in skeletal muscles from rat, guinea-pig and man. J Physiol. 1982 Nov;332:47–58. doi: 10.1113/jphysiol.1982.sp014399. [DOI] [PMC free article] [PubMed] [Google Scholar]
- Edwards R. H., Hill D. K., Jones D. A. Metabolic changes associated with the slowing of relaxation in fatigued mouse muscle. J Physiol. 1975 Oct;251(2):287–301. doi: 10.1113/jphysiol.1975.sp011093. [DOI] [PMC free article] [PubMed] [Google Scholar]
- Eisenberg E., Hill T. L., Chen Y. Cross-bridge model of muscle contraction. Quantitative analysis. Biophys J. 1980 Feb;29(2):195–227. doi: 10.1016/S0006-3495(80)85126-5. [DOI] [PMC free article] [PubMed] [Google Scholar]
- Fabiato A., Fabiato F. Effects of pH on the myofilaments and the sarcoplasmic reticulum of skinned cells from cardiace and skeletal muscles. J Physiol. 1978 Mar;276:233–255. doi: 10.1113/jphysiol.1978.sp012231. [DOI] [PMC free article] [PubMed] [Google Scholar]
- Fitts R. H., Courtright J. B., Kim D. H., Witzmann F. A. Muscle fatigue with prolonged exercise: contractile and biochemical alterations. Am J Physiol. 1982 Jan;242(1):C65–C73. doi: 10.1152/ajpcell.1982.242.1.C65. [DOI] [PubMed] [Google Scholar]
- Grabowski W., Lobsiger E. A., Lüttgau H. C. The effect of repetitive stimulation at low frequencies upon the electrical and mechanical activity of single muscle fibres. Pflugers Arch. 1972;334(3):222–239. doi: 10.1007/BF00626225. [DOI] [PubMed] [Google Scholar]
- HUXLEY A. F. Muscle structure and theories of contraction. Prog Biophys Biophys Chem. 1957;7:255–318. [PubMed] [Google Scholar]
- Herzig J. W., Peterson J. W., Rüegg J. C., Solaro R. J. Vanadate and phosphate ions reduce tension and increase cross-bridge kinetics in chemically skinned heart muscle. Biochim Biophys Acta. 1981 Jan 21;672(2):191–196. doi: 10.1016/0304-4165(81)90392-5. [DOI] [PubMed] [Google Scholar]
- Hibberd M. G., Dantzig J. A., Trentham D. R., Goldman Y. E. Phosphate release and force generation in skeletal muscle fibers. Science. 1985 Jun 14;228(4705):1317–1319. doi: 10.1126/science.3159090. [DOI] [PubMed] [Google Scholar]
- Hood D. A., Gorski J., Terjung R. L. Oxygen cost of twitch and tetanic isometric contractions of rat skeletal muscle. Am J Physiol. 1986 Apr;250(4 Pt 1):E449–E456. doi: 10.1152/ajpendo.1986.250.4.E449. [DOI] [PubMed] [Google Scholar]
- Julian F. J., Moss R. L. Effects of calcium and ionic strength on shortening velocity and tension development in frog skinned muscle fibres. J Physiol. 1981 Feb;311:179–199. doi: 10.1113/jphysiol.1981.sp013580. [DOI] [PMC free article] [PubMed] [Google Scholar]
- Julian F. J., Rome L. C., Stephenson D. G., Striz S. The maximum speed of shortening in living and skinned frog muscle fibres. J Physiol. 1986 Jan;370:181–199. doi: 10.1113/jphysiol.1986.sp015929. [DOI] [PMC free article] [PubMed] [Google Scholar]
- Kawai M., Güth K., Winnikes K., Haist C., Rüegg J. C. The effect of inorganic phosphate on the ATP hydrolysis rate and the tension transients in chemically skinned rabbit psoas fibers. Pflugers Arch. 1987 Jan;408(1):1–9. doi: 10.1007/BF00581833. [DOI] [PubMed] [Google Scholar]
- Kawai M. The role of orthophosphate in crossbridge kinetics in chemically skinned rabbit psoas fibres as detected with sinusoidal and step length alterations. J Muscle Res Cell Motil. 1986 Oct;7(5):421–434. doi: 10.1007/BF01753585. [DOI] [PubMed] [Google Scholar]
- Kentish J. C., Nayler W. G. The influence of pH on the Ca2+-regulated ATPase of cardiac and white skeletal myofibrils. J Mol Cell Cardiol. 1979 Jun;11(6):611–617. doi: 10.1016/0022-2828(79)90435-8. [DOI] [PubMed] [Google Scholar]
- Kushmerick M. J., Meyer R. A. Chemical changes in rat leg muscle by phosphorus nuclear magnetic resonance. Am J Physiol. 1985 May;248(5 Pt 1):C542–C549. doi: 10.1152/ajpcell.1985.248.5.C542. [DOI] [PubMed] [Google Scholar]
- MacIntosh B. R., Stainsby W. N., Gladden L. B. Fatigue from incompletely fused tetanic contractions in skeletal muscle in situ. J Appl Physiol Respir Environ Exerc Physiol. 1983 Sep;55(3):976–982. doi: 10.1152/jappl.1983.55.3.976. [DOI] [PubMed] [Google Scholar]
- Maher J. T., Goodman A. L., Francesconi R., Bowers W. D., Hartley L. H., Angelakos E. T. Responses of rat myocardium to exhaustive exercise. Am J Physiol. 1972 Jan;222(1):207–212. doi: 10.1152/ajplegacy.1972.222.1.207. [DOI] [PubMed] [Google Scholar]
- Meyer R. A., Kuchmerick M. J., Brown T. R. Application of 31P-NMR spectroscopy to the study of striated muscle metabolism. Am J Physiol. 1982 Jan;242(1):C1–11. doi: 10.1152/ajpcell.1982.242.1.C1. [DOI] [PubMed] [Google Scholar]
- Moss R. L. The effect of calcium on the maximum velocity of shortening in skinned skeletal muscle fibres of the rabbit. J Muscle Res Cell Motil. 1982 Sep;3(3):295–311. doi: 10.1007/BF00713039. [DOI] [PubMed] [Google Scholar]
- Nassar-Gentina V., Passonneau J. V., Vergara J. L., Rapoport S. I. Metabolic correlates of fatigue and of recovery from fatigue in single frog muscle fibers. J Gen Physiol. 1978 Nov;72(5):593–606. doi: 10.1085/jgp.72.5.593. [DOI] [PMC free article] [PubMed] [Google Scholar]
- Nosek T. M., Fender K. Y., Godt R. E. It is diprotonated inorganic phosphate that depresses force in skinned skeletal muscle fibers. Science. 1987 Apr 10;236(4798):191–193. doi: 10.1126/science.3563496. [DOI] [PubMed] [Google Scholar]
- Pate E., Cooke R. The inhibition of muscle contraction by adenosine 5' (beta, gamma-imido) triphosphate and by pyrophosphate. Biophys J. 1985 Jun;47(6):773–780. doi: 10.1016/S0006-3495(85)83980-1. [DOI] [PMC free article] [PubMed] [Google Scholar]
- Petrofsky J. S., Weber C., Phillips C. A. Mechanical and electrical correlates of isometric muscle fatigue in skeletal muscle in the cat. Pflugers Arch. 1980 Aug;387(1):33–38. doi: 10.1007/BF00580841. [DOI] [PubMed] [Google Scholar]
- Podolin R. A., Ford L. E. Influence of partial activation on force-velocity properties of frog skinned muscle fibers in millimolar magnesium ion. J Gen Physiol. 1986 Apr;87(4):607–631. doi: 10.1085/jgp.87.4.607. [DOI] [PMC free article] [PubMed] [Google Scholar]
- Rüegg J. C., Schädler M., Steiger G. J., Müller G. Effects of inorganic phosphate on the contractile mechanism. Pflugers Arch. 1971;325(4):359–364. doi: 10.1007/BF00592176. [DOI] [PubMed] [Google Scholar]
- Sleep J., Glyn H. Inhibition of myofibrillar and actomyosin subfragment 1 adenosinetriphosphatase by adenosine 5'-diphosphate, pyrophosphate, and adenyl-5'-yl imidodiphosphate. Biochemistry. 1986 Mar 11;25(5):1149–1154. doi: 10.1021/bi00353a030. [DOI] [PubMed] [Google Scholar]
- Spande J. I., Schottelius B. A. Chemical basis of fatigue in isolated mouse soleus muscle. Am J Physiol. 1970 Nov;219(5):1490–1495. doi: 10.1152/ajplegacy.1970.219.5.1490. [DOI] [PubMed] [Google Scholar]
- Webb M. R., Hibberd M. G., Goldman Y. E., Trentham D. R. Oxygen exchange between Pi in the medium and water during ATP hydrolysis mediated by skinned fibers from rabbit skeletal muscle. Evidence for Pi binding to a force-generating state. J Biol Chem. 1986 Nov 25;261(33):15557–15564. [PubMed] [Google Scholar]
- White D. C., Thorson J. Phosphate starvation and the nonlinear dynamics of insect fibrillar flight muscle. J Gen Physiol. 1972 Sep;60(3):307–336. doi: 10.1085/jgp.60.3.307. [DOI] [PMC free article] [PubMed] [Google Scholar]
- Wilkie D. R., Dawson M. J., Edwards R. H., Gordon R. E., Shaw D. 31P NMR studies of resting muscle in normal human subjects. Adv Exp Med Biol. 1984;170:333–347. doi: 10.1007/978-1-4684-4703-3_28. [DOI] [PubMed] [Google Scholar]
- Woledge R. C. The energetics of tortoise muscle. J Physiol. 1968 Aug;197(3):685–707. doi: 10.1113/jphysiol.1968.sp008582. [DOI] [PMC free article] [PubMed] [Google Scholar]