Abstract
1. Further developmental changes in acetylcholine (ACh) receptor channel function were examined in Xenopus muscle cultures using the cell-attached patch-clamp technique. 2. Two types of acetylcholine (ACh) receptor channel events, low- and high-conductance channel events, were distinguished as reported earlier. Apparent open-time histograms for high-conductance channel events were well fitted by a single exponential but those for low-conductance channel events were sometimes fitted better by two exponentials. 3. In low-conductance channel events when the open-time histogram was well fitted by two exponentials, successive open times were correlated: an event with a long open time tended to be followed after a brief interval (less than 1 ms) by another long-duration event. A short-duration event was less frequently followed by an event within a short interval (1 ms) with a long-duration event. 4. Closed-time histograms for the interval between successive low-conductance channel events and between successive high-conductance channel events were both fitted by two exponentials. The fast time constant was 0.36 ms for the high-conductance channel event and 0.31 ms for the low-conductance channel event. There was an indication that a third and faster component was hidden in the first bin (0-200 microseconds) in the closed-time histogram of both types of channel events. 5. Defining a burst as successive openings separated by closures briefer than 1 ms, the number of gaps per burst was different for the two types of channel events. They were 0.16 for high- and 0.37 for low-conductance channel events. In both types of channels, neither the fast component in the closed-time histogram nor the number of gaps per burst changed with time in culture. 6. The apparent open time of both types of channels increased progressively as ACh concentration was increased, suggesting an increasing number of unresolved closures at higher concentrations. At 100 microM-ACh the apparent open time became shorter probably due to channel blockade by ACh molecules. Closed-time histograms were fitted by two exponentials. The time constant of the fast component remained similar to that at low concentration (0.2 microM) up to 20 microM, but the relative number of closures belonging to this component increased with ACh concentration. In contrast, the slow component shortened its time constant as ACh concentration increased and the relative frequency decreased. Again, there was an indication that another faster component existed in the closed-time histogram.(ABSTRACT TRUNCATED AT 400 WORDS)
Full text
PDF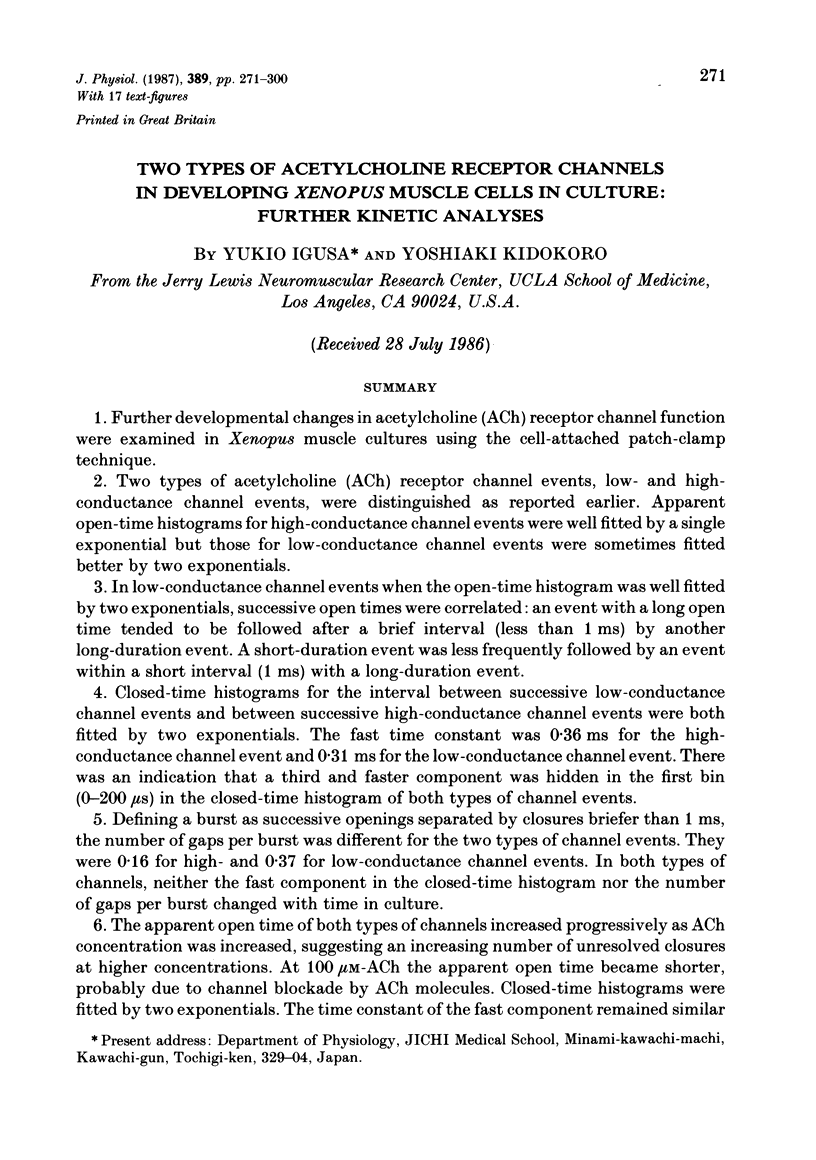
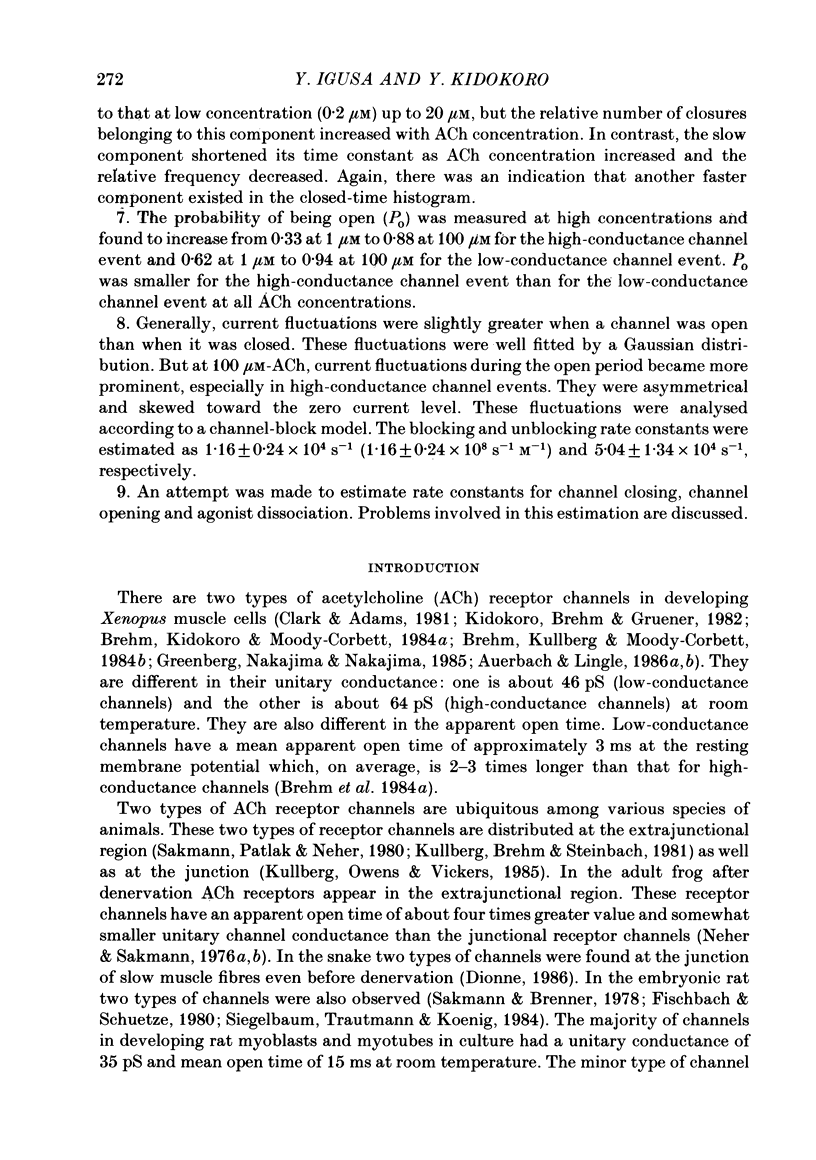
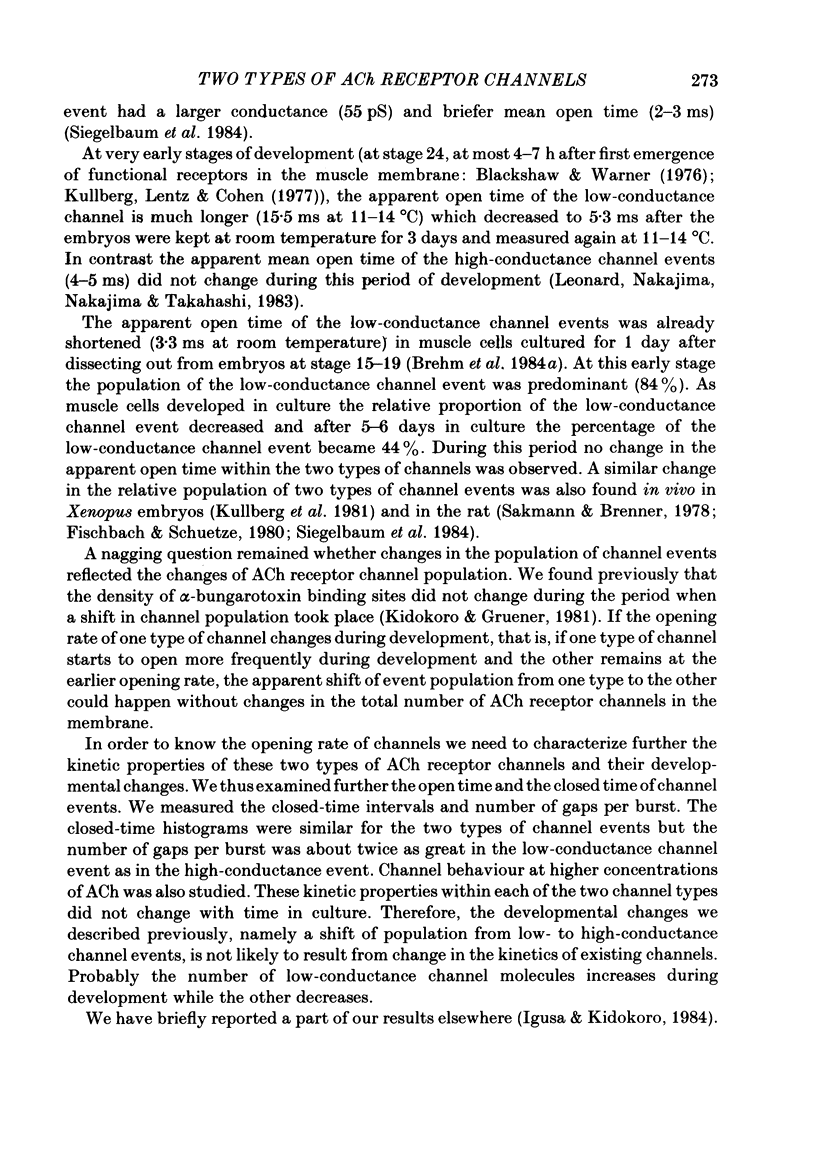
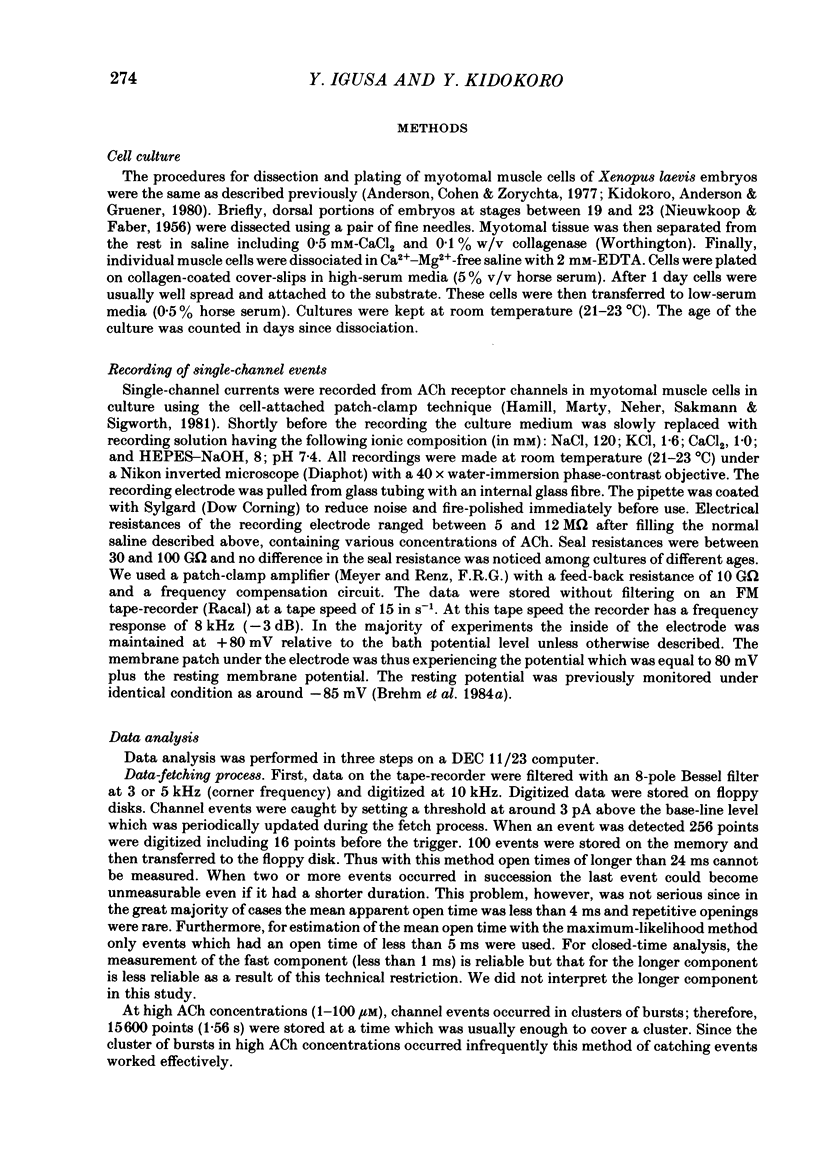
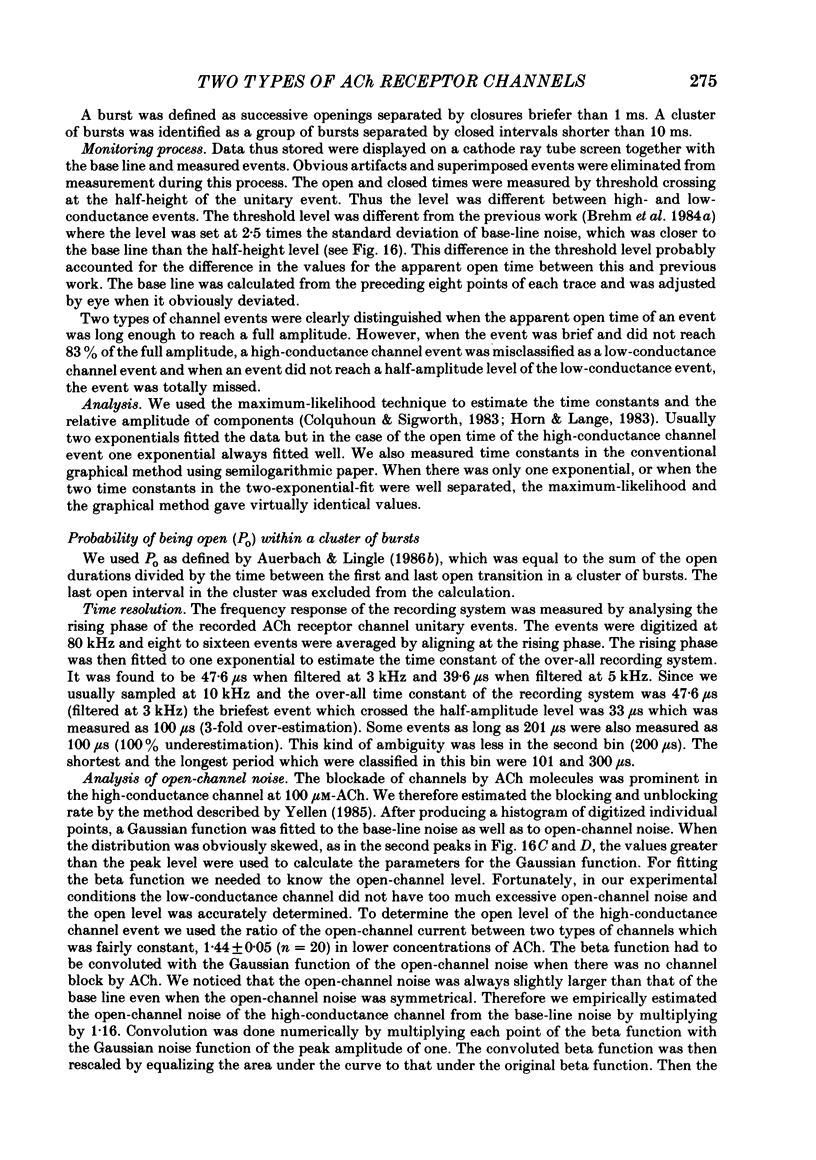
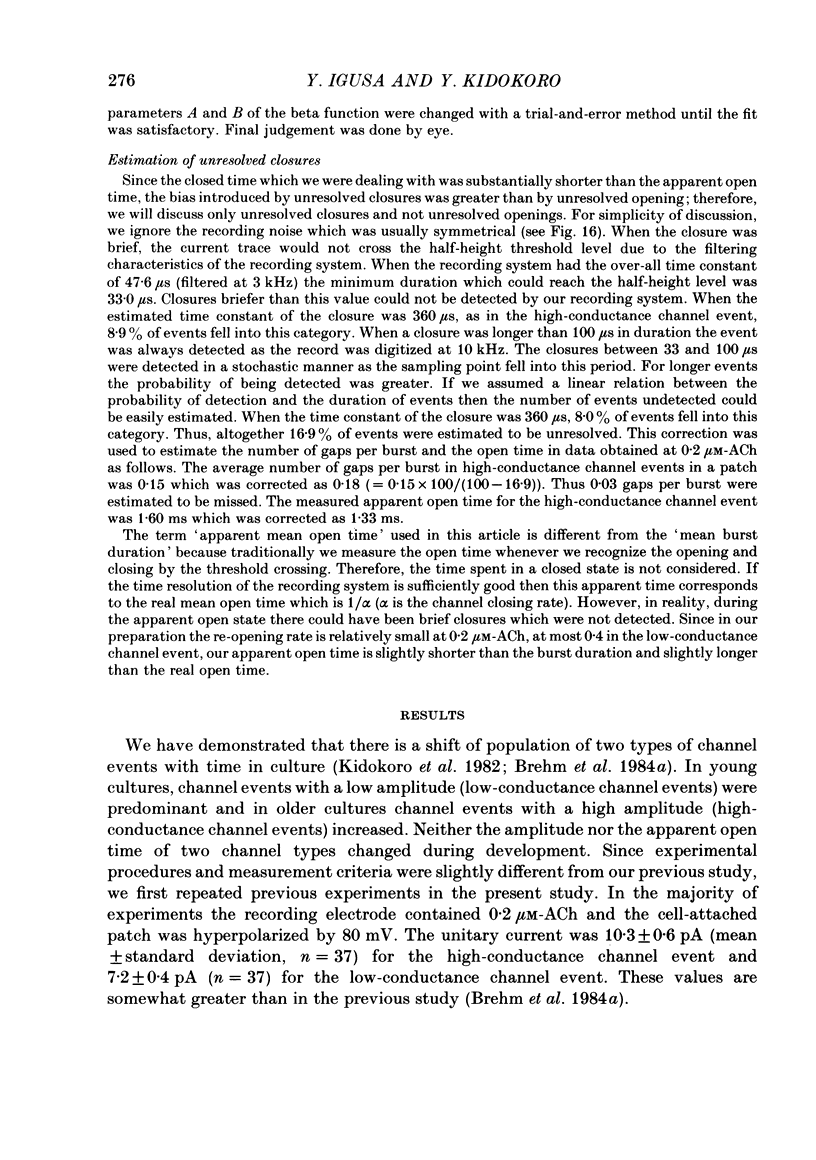
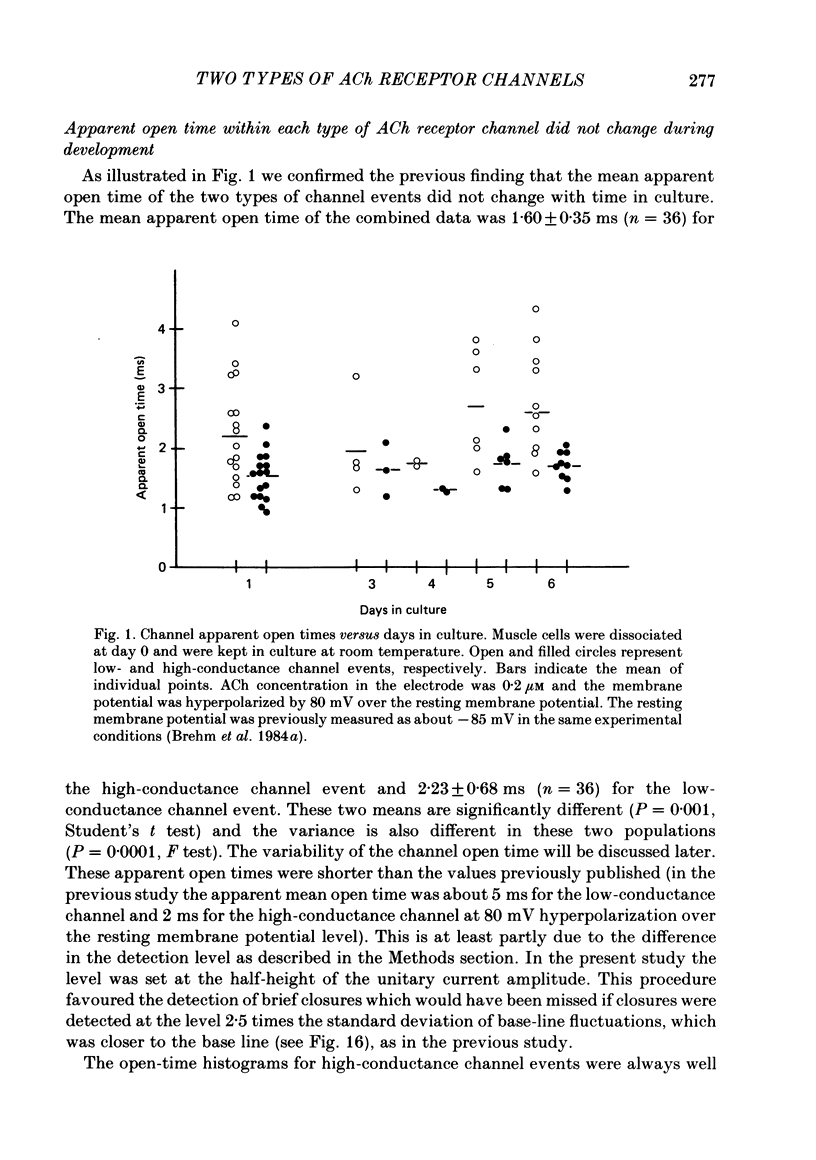
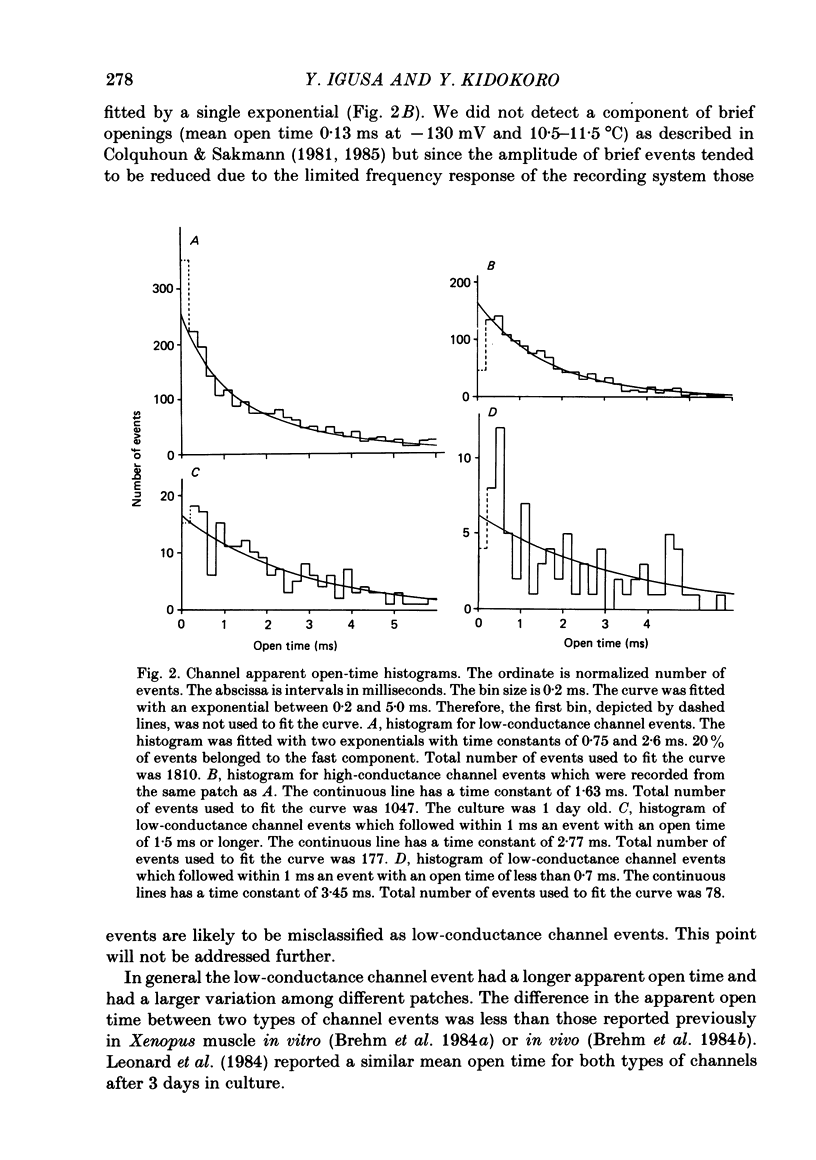
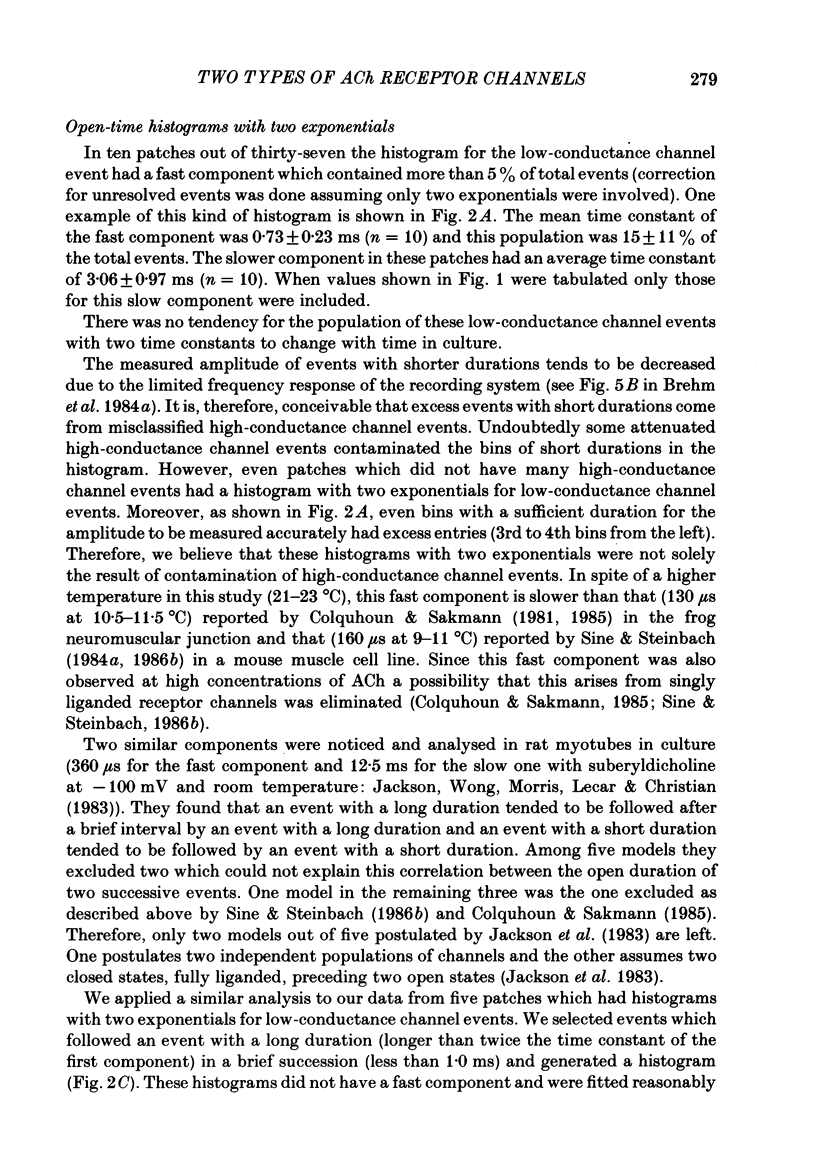
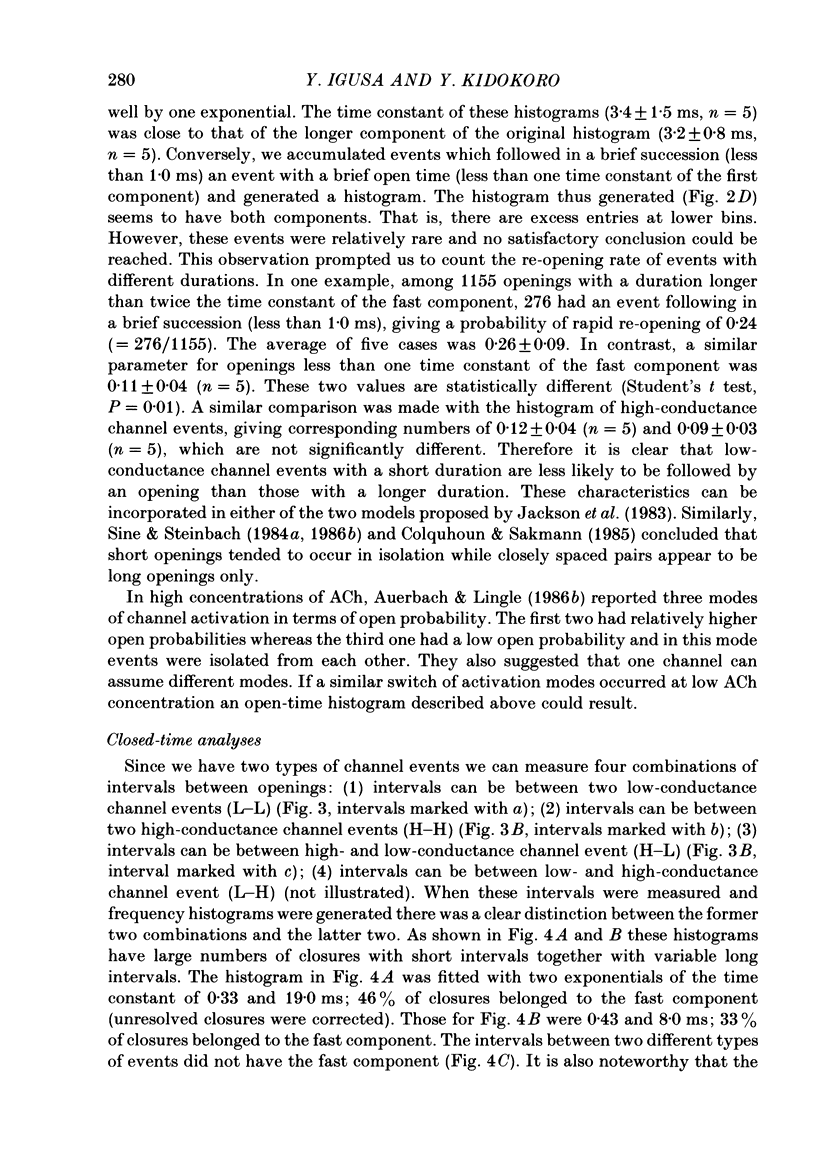
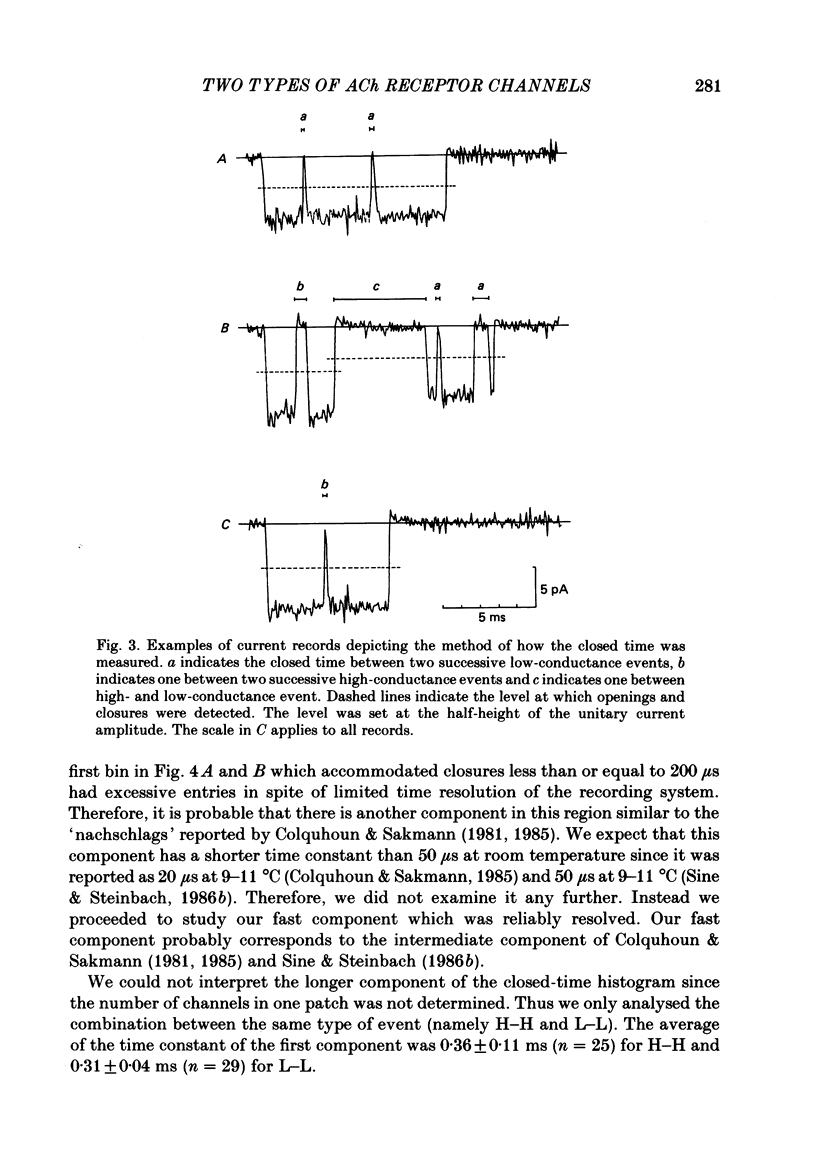
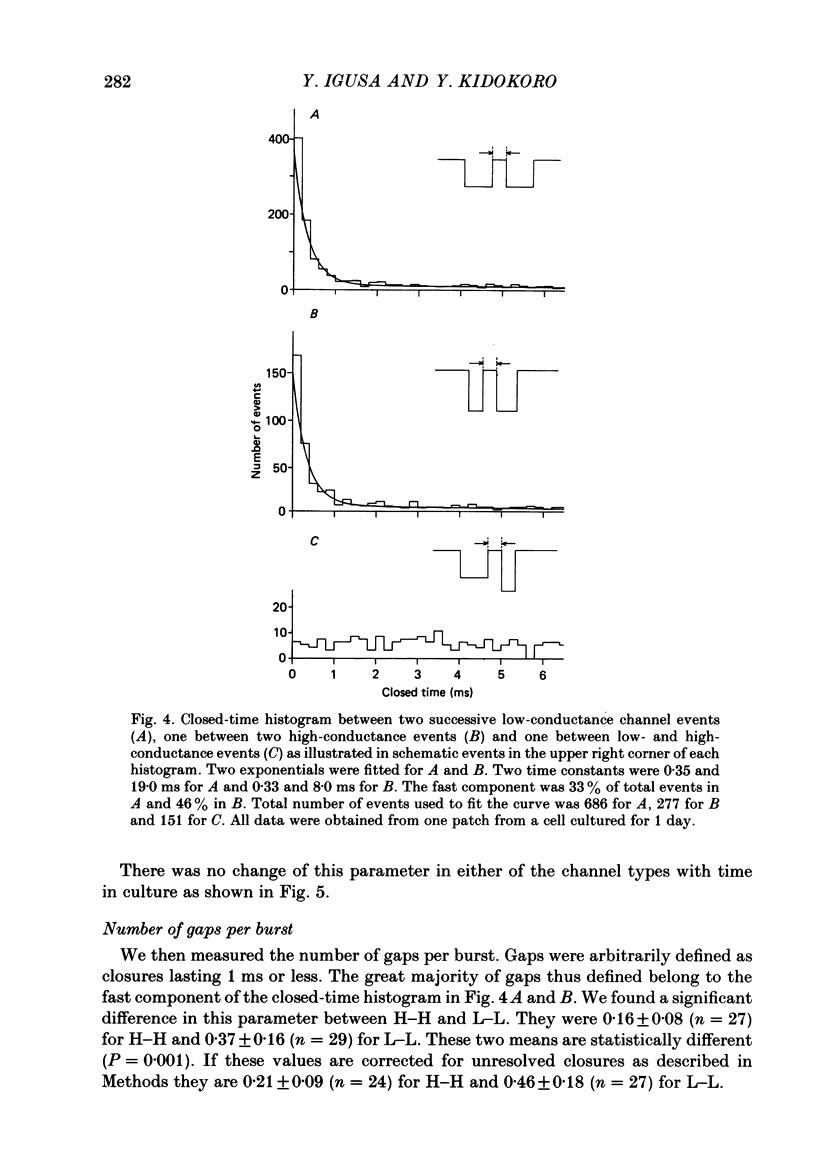
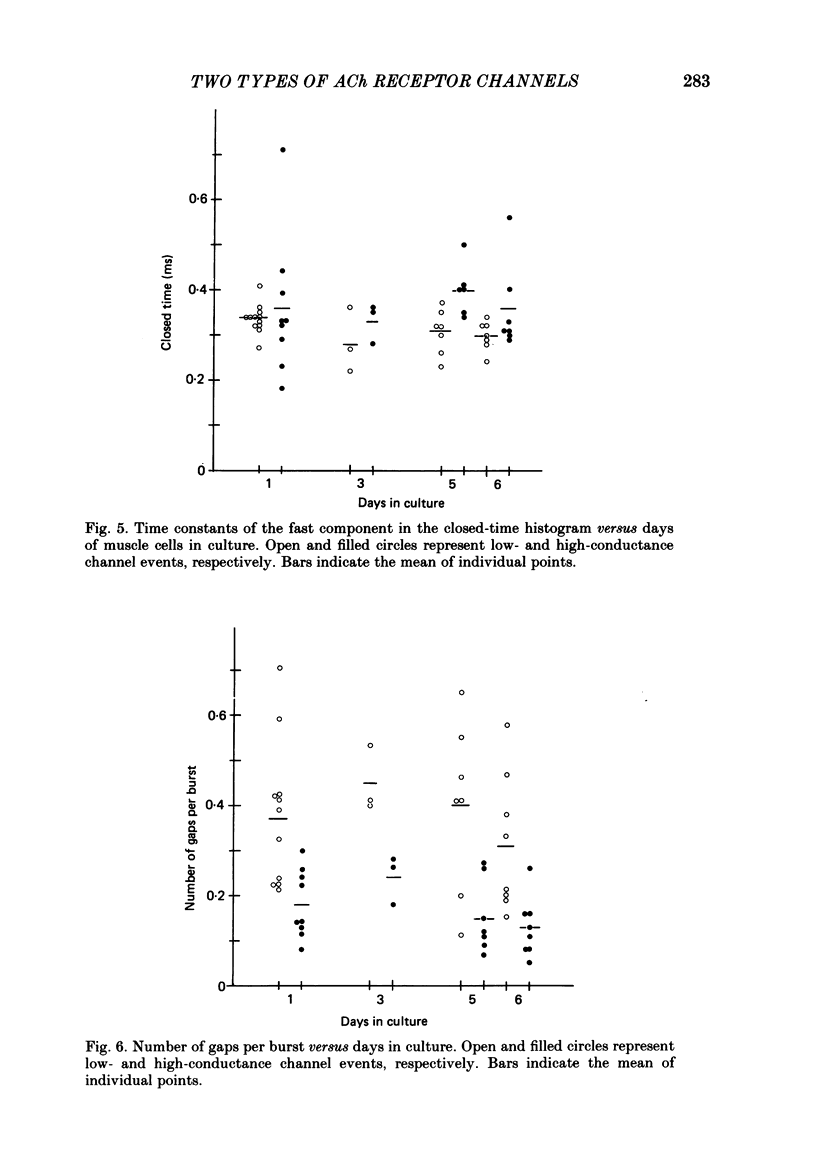
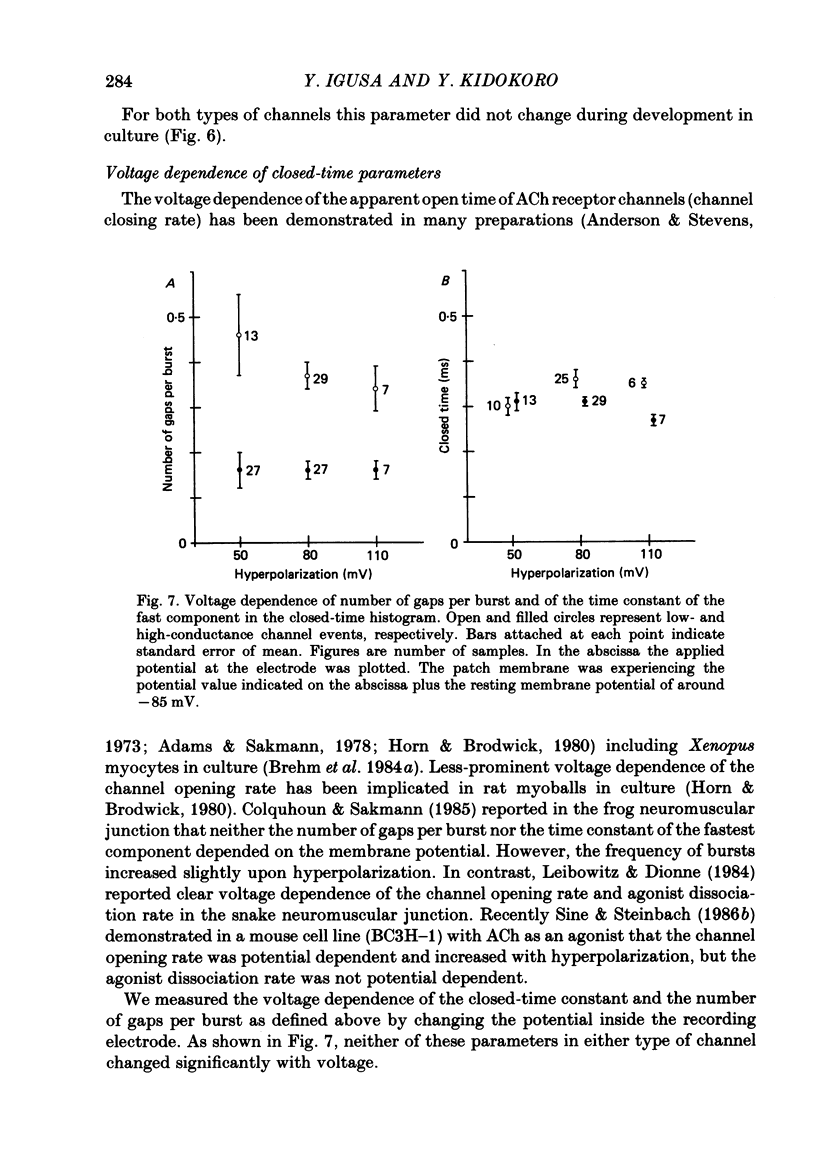
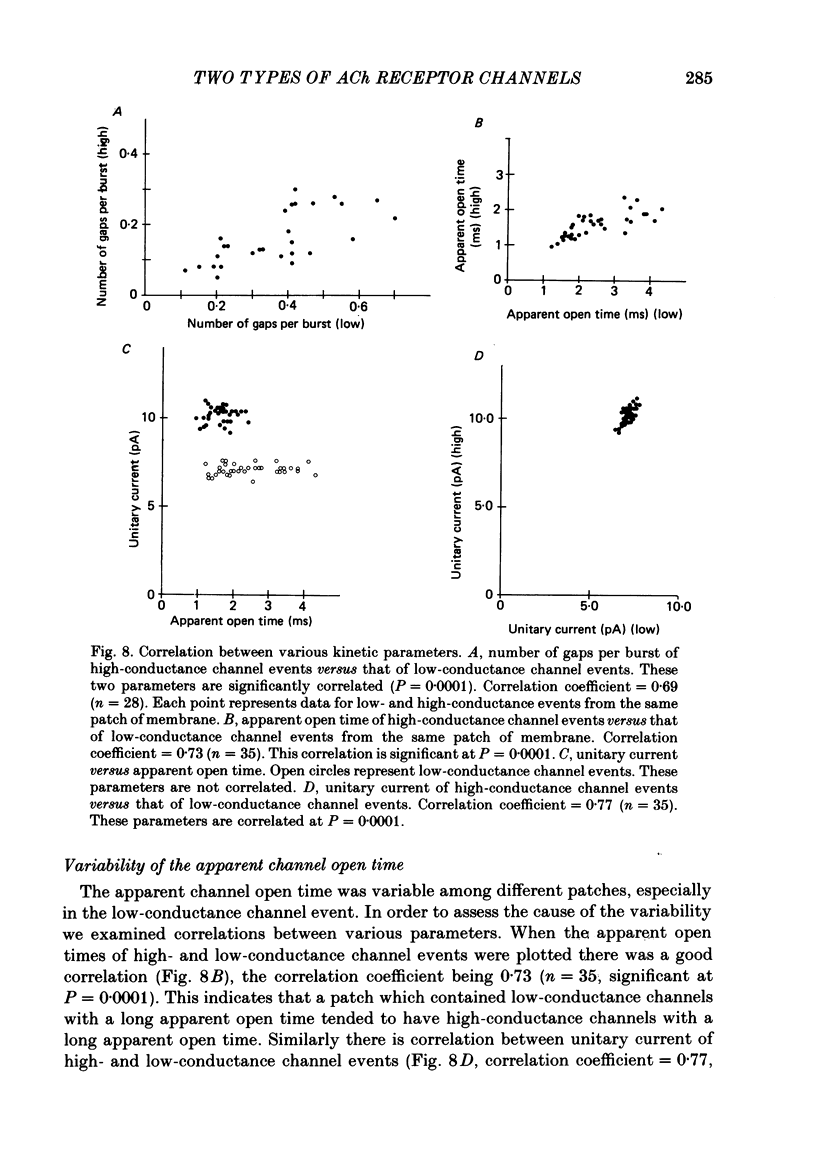
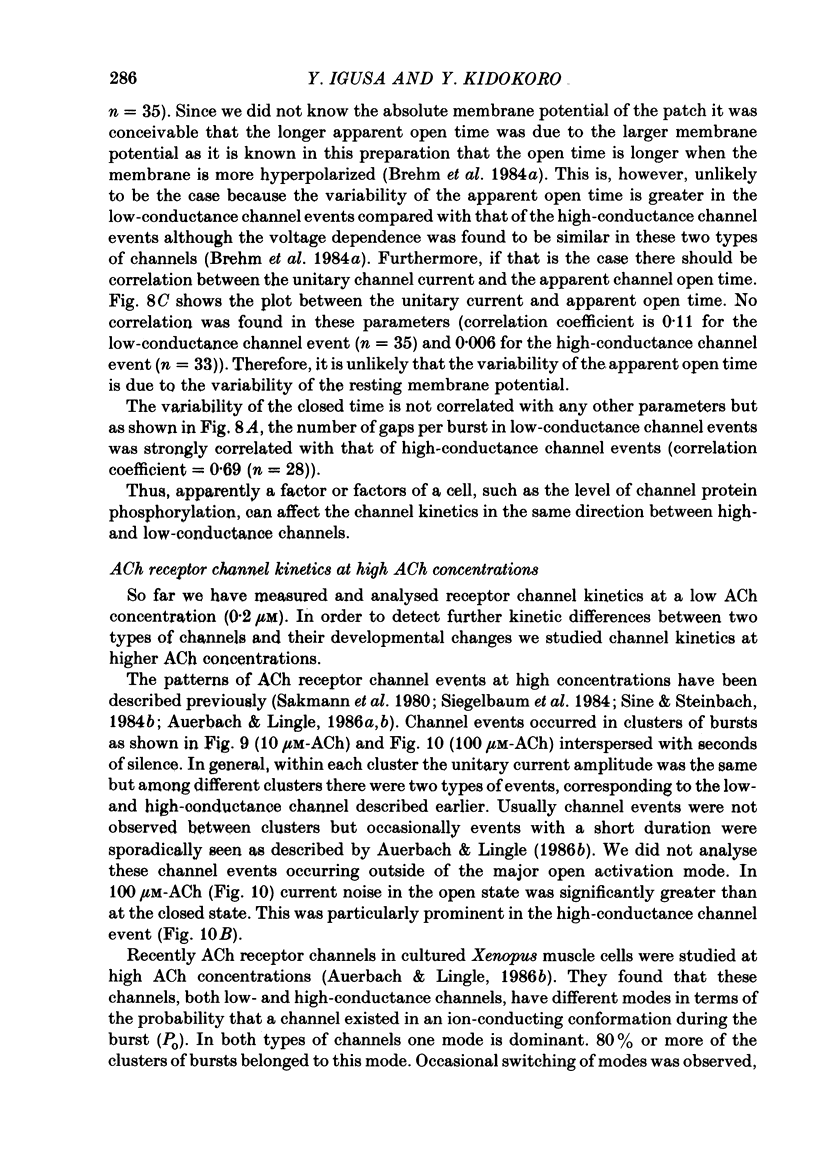
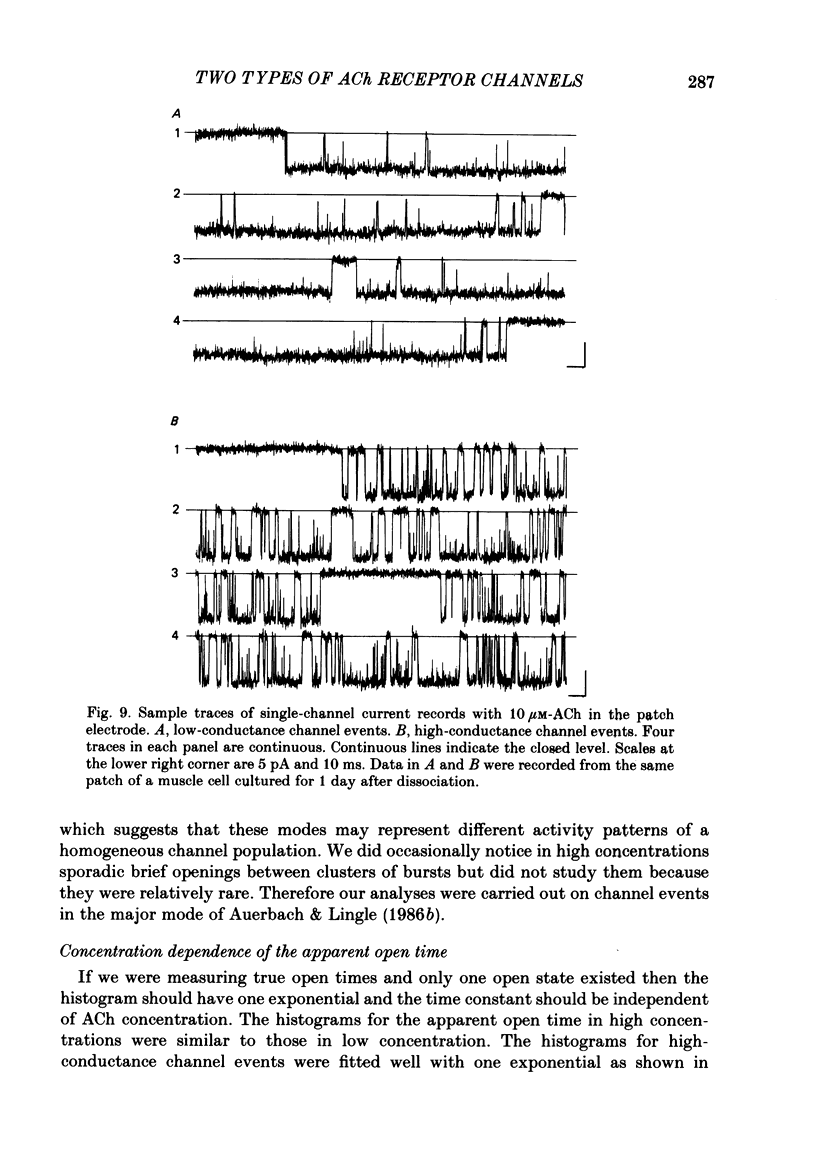
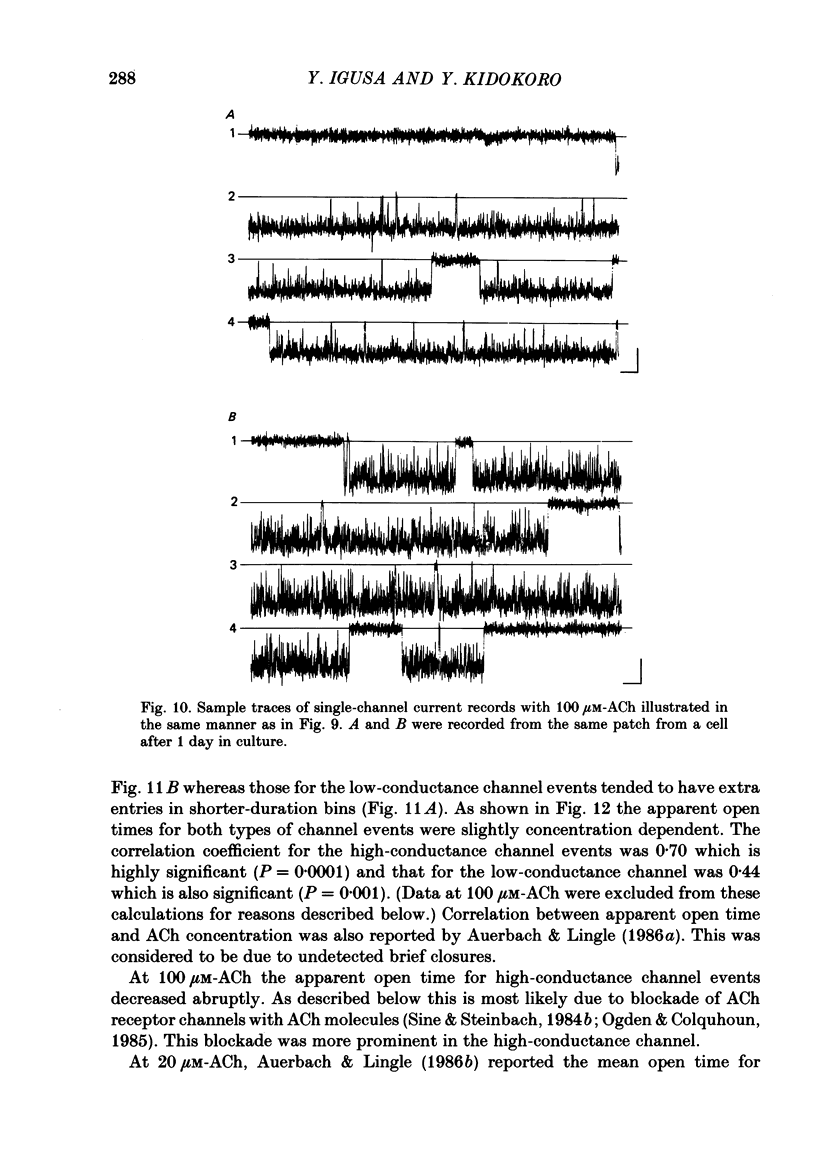
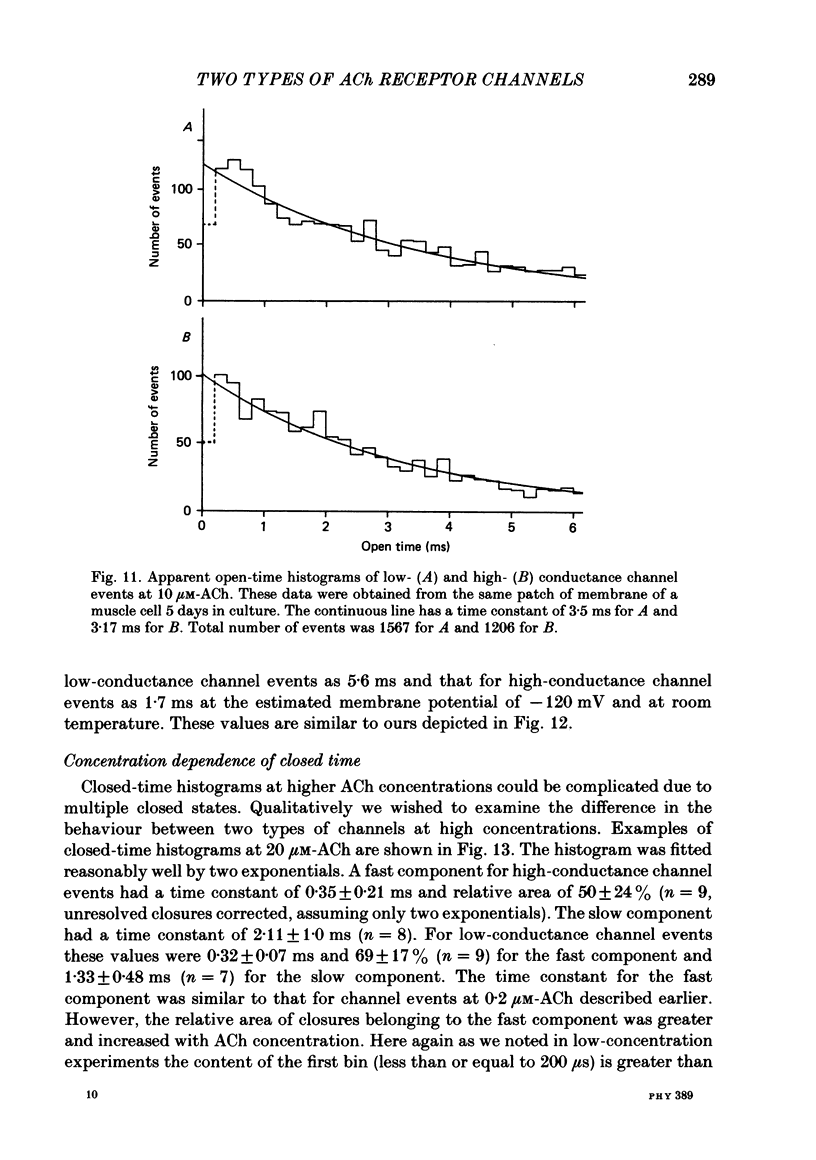
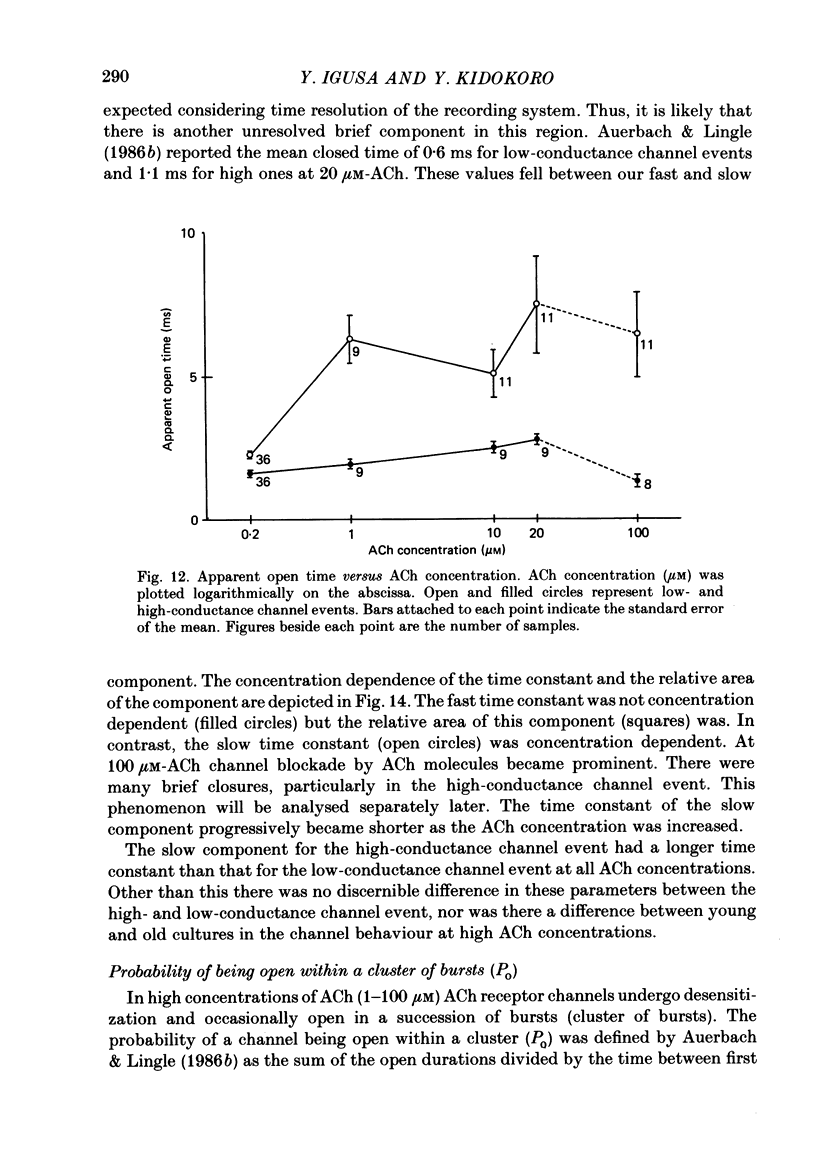
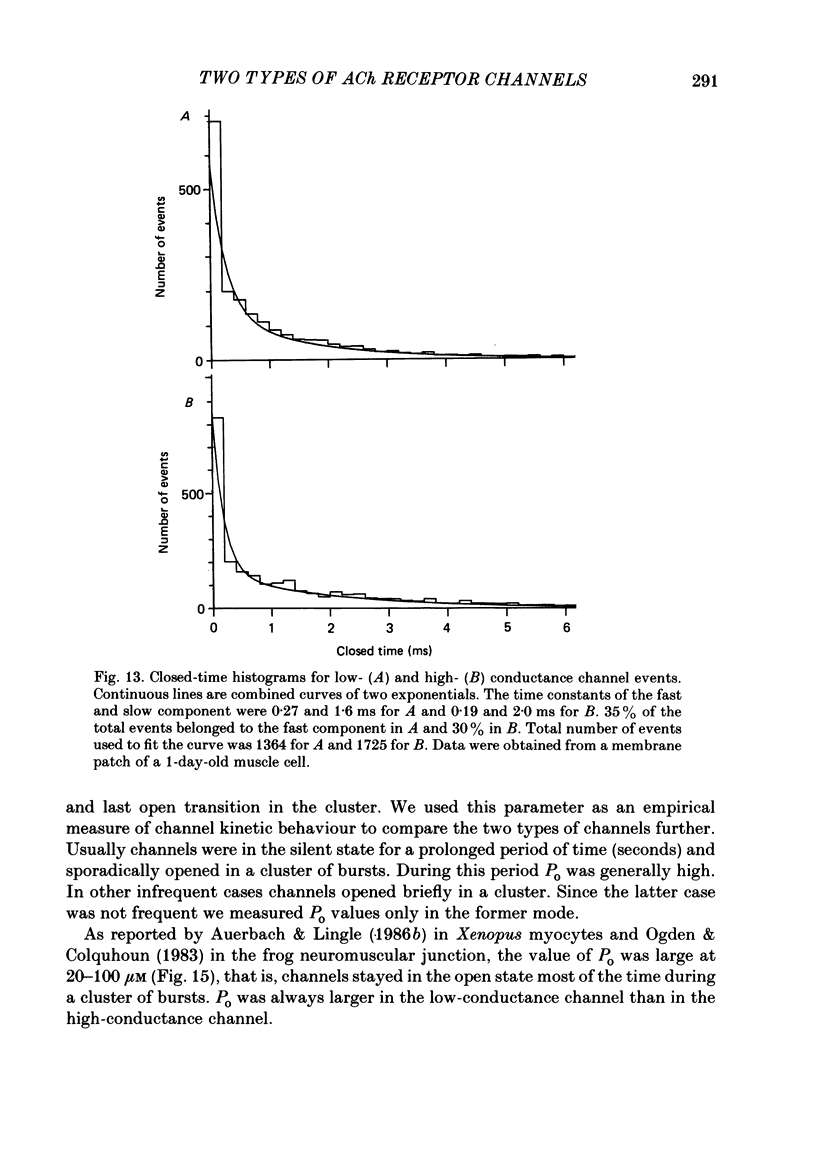
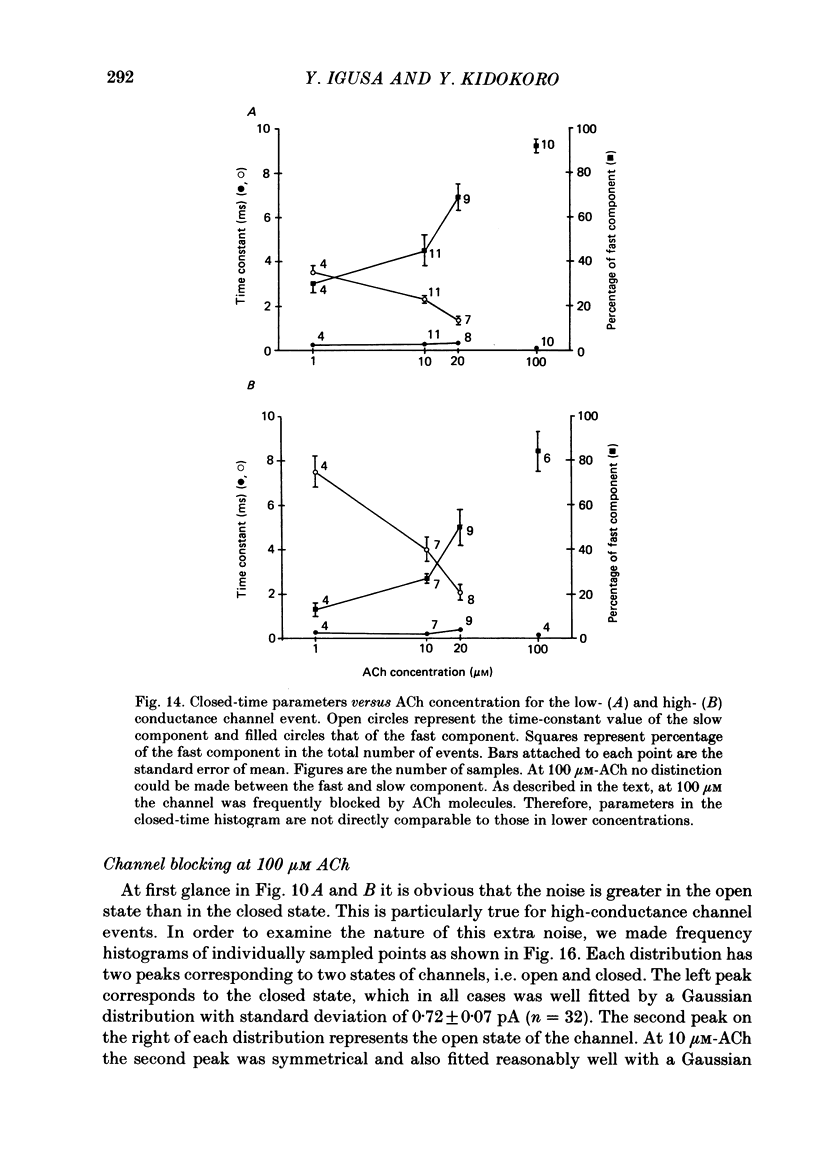
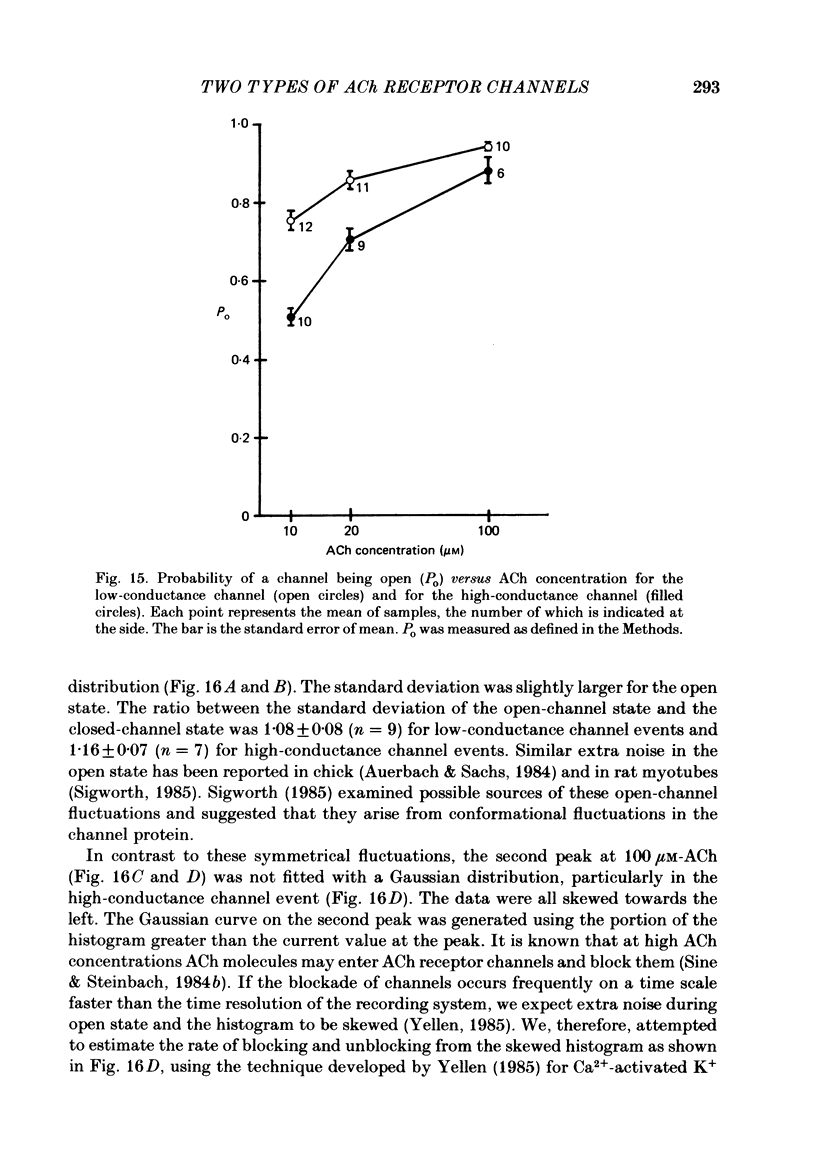
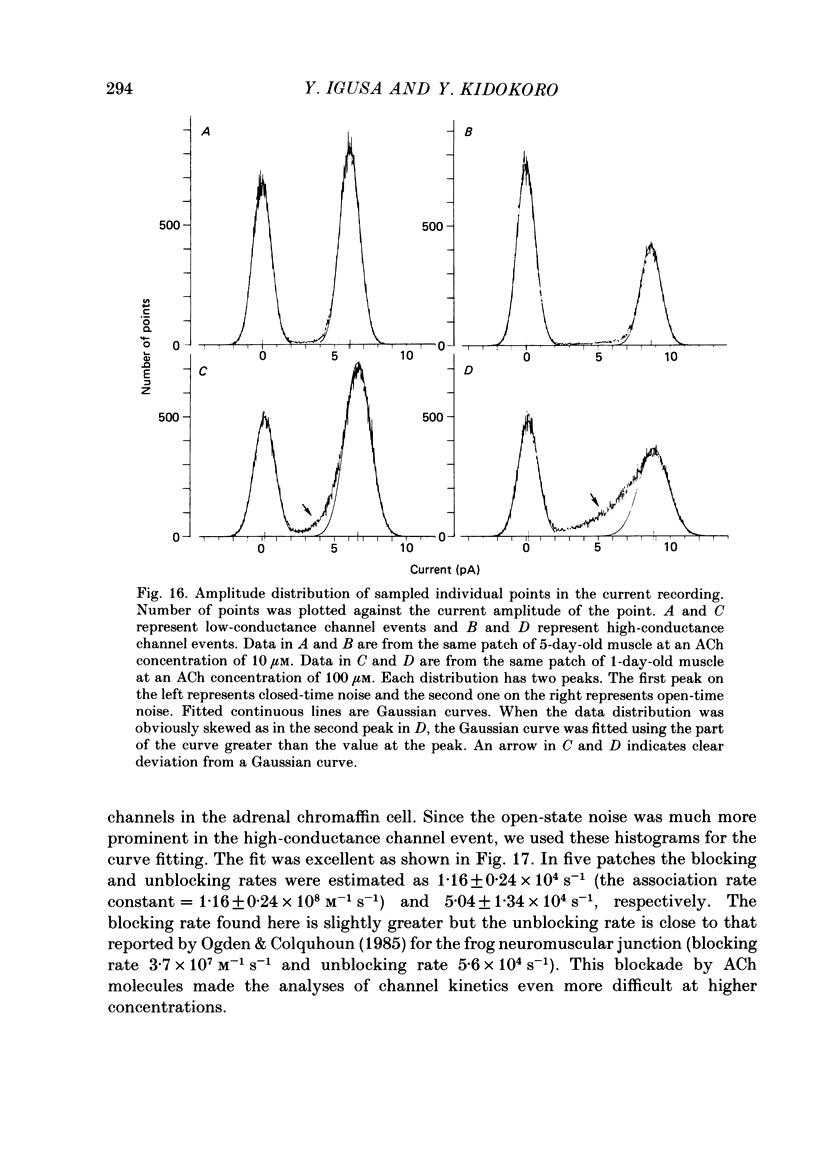
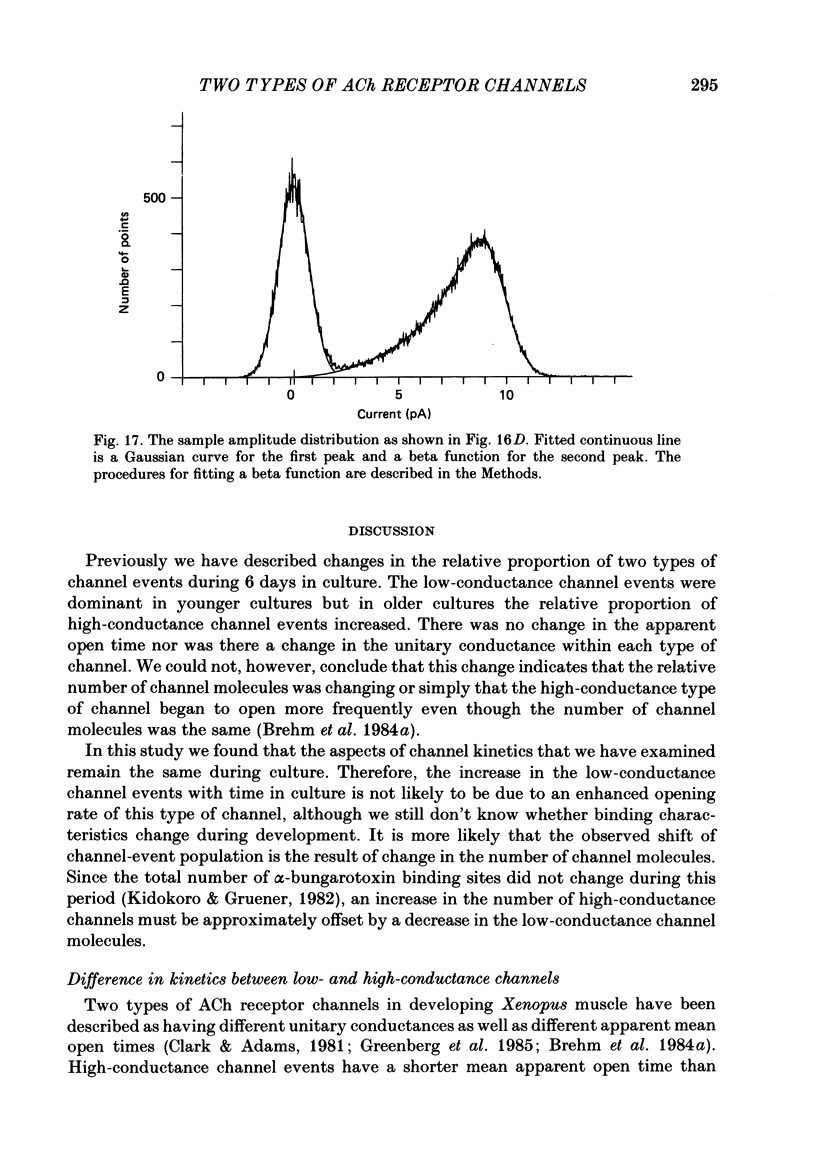
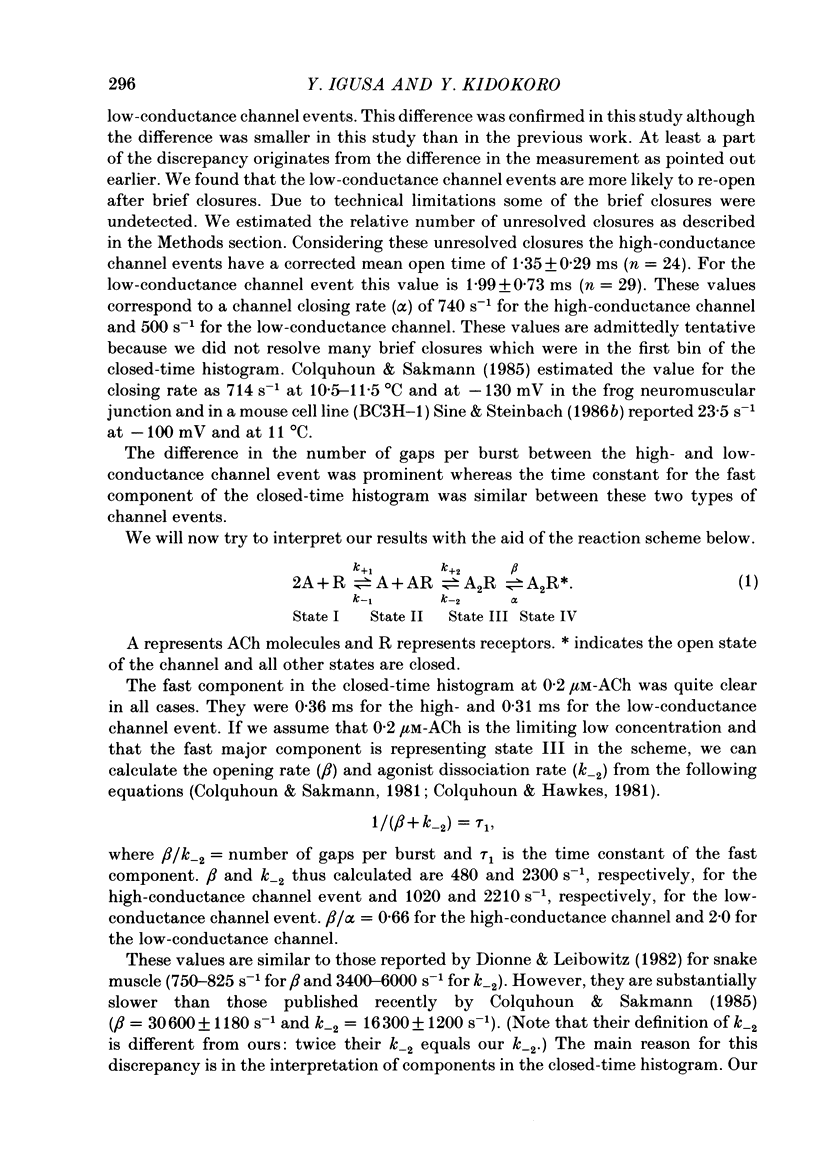
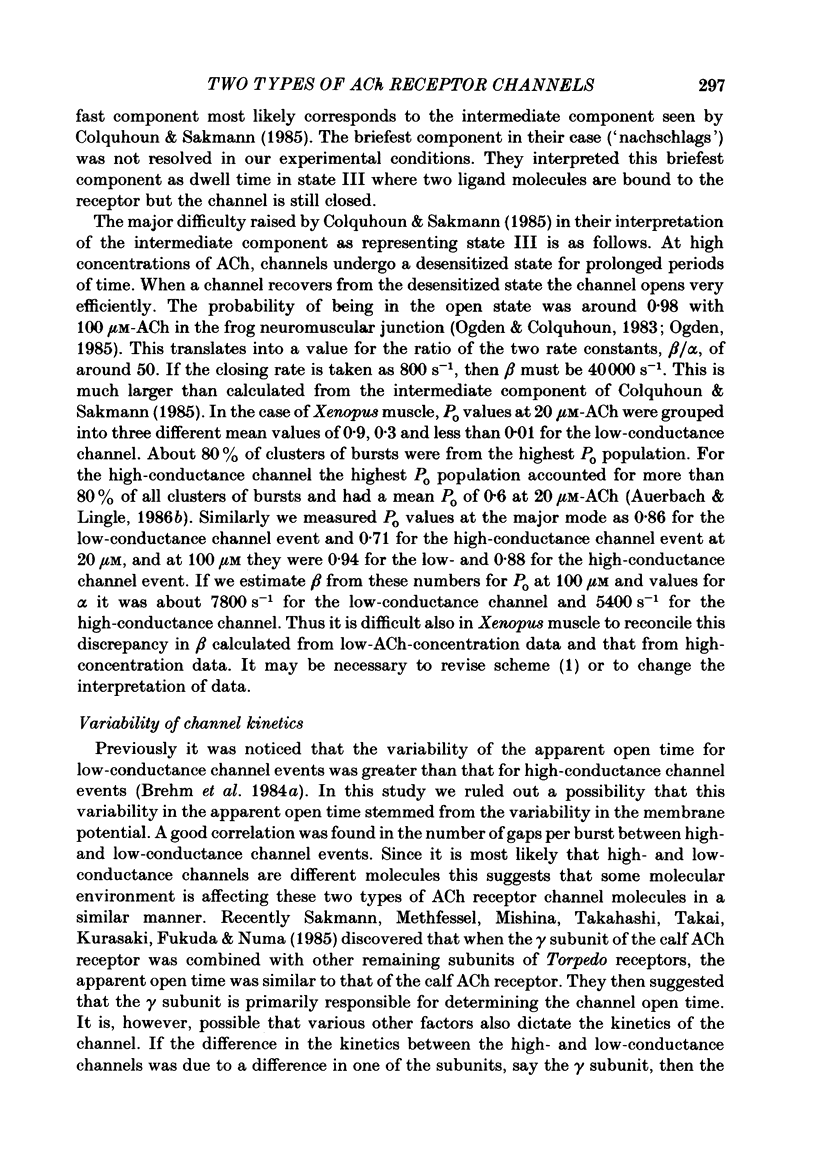
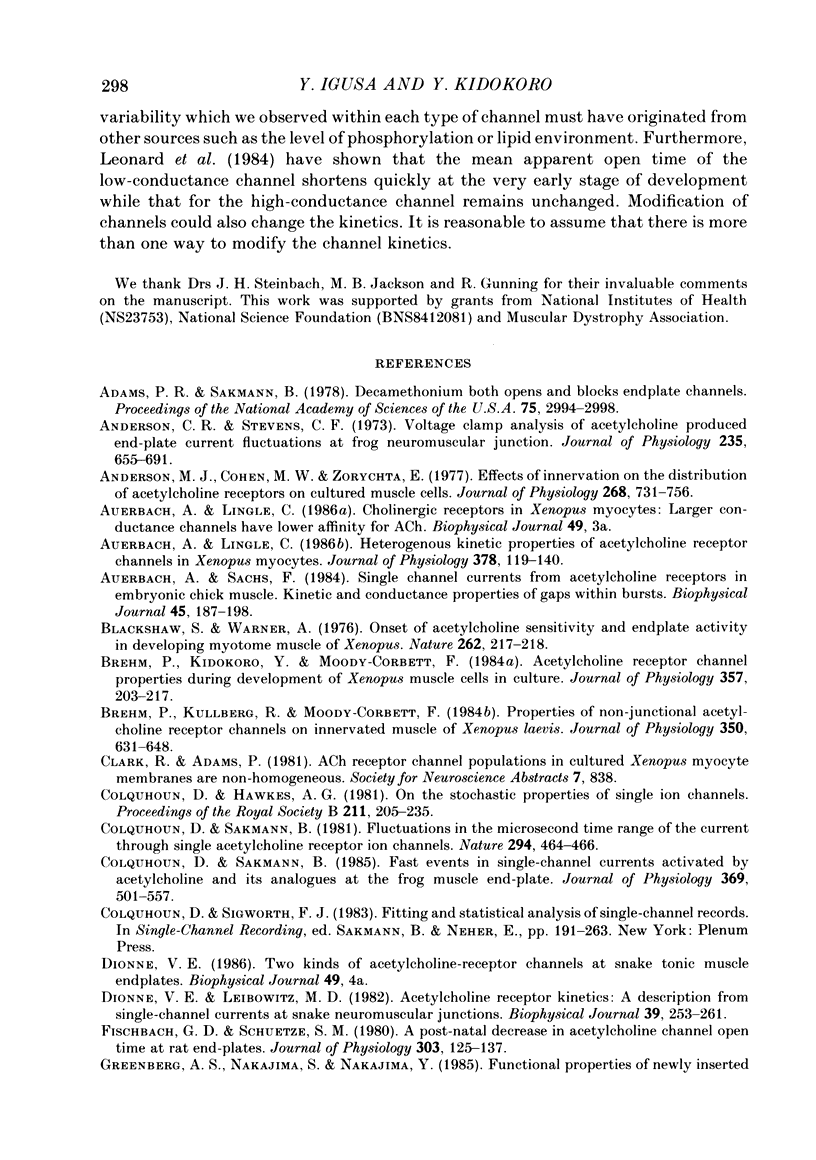
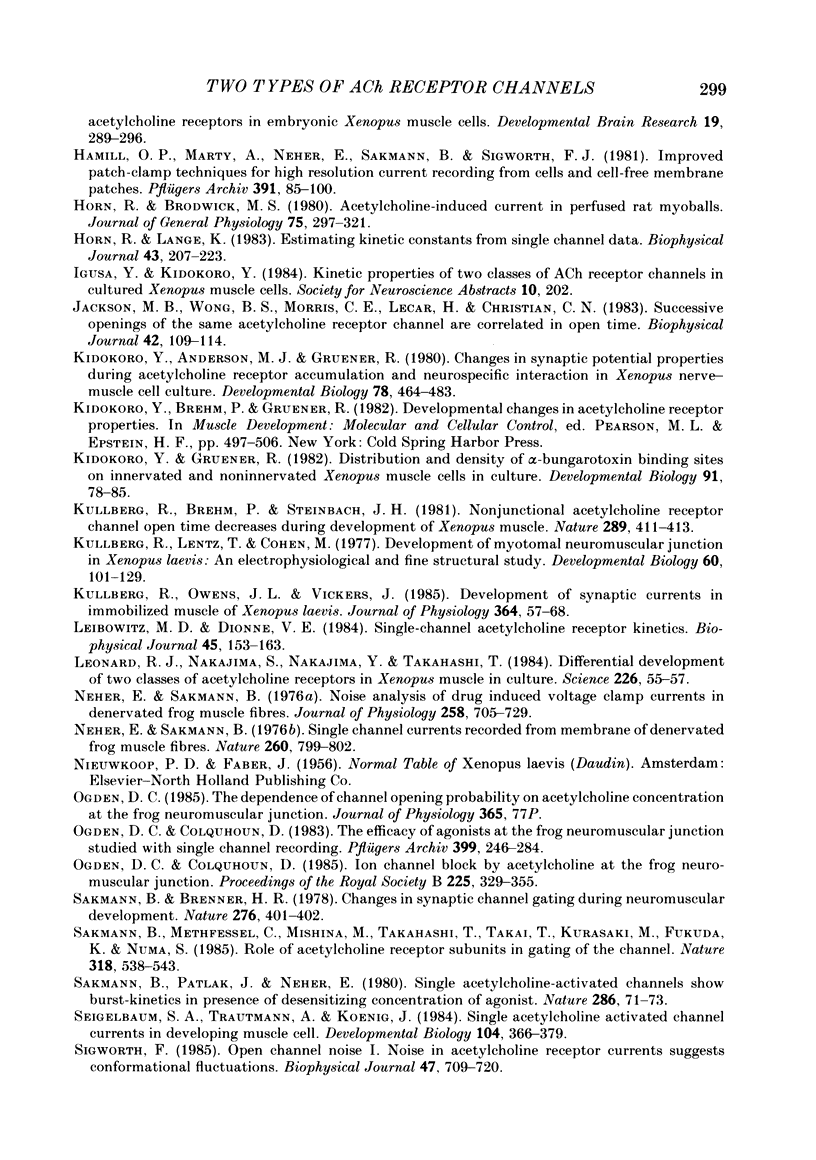
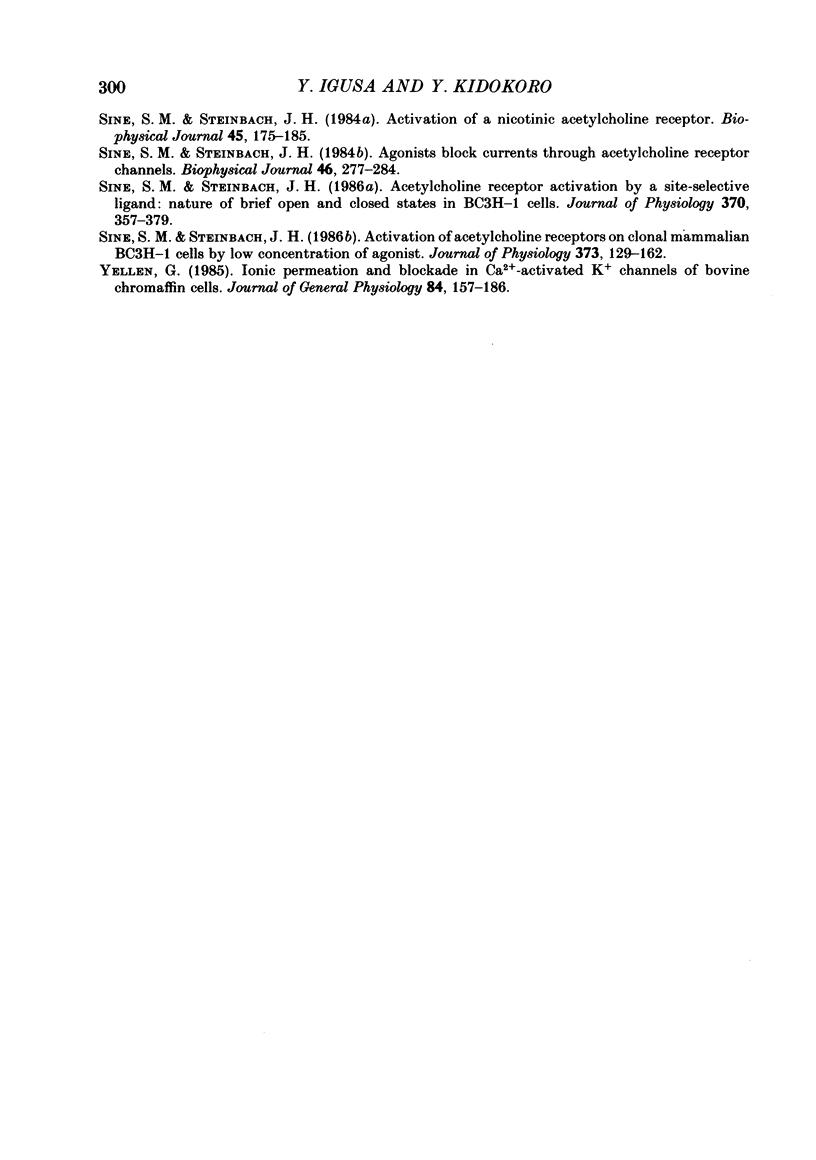
Selected References
These references are in PubMed. This may not be the complete list of references from this article.
- Adams P. R., Sakmann B. Decamethonium both opens and blocks endplate channels. Proc Natl Acad Sci U S A. 1978 Jun;75(6):2994–2998. doi: 10.1073/pnas.75.6.2994. [DOI] [PMC free article] [PubMed] [Google Scholar]
- Anderson C. R., Stevens C. F. Voltage clamp analysis of acetylcholine produced end-plate current fluctuations at frog neuromuscular junction. J Physiol. 1973 Dec;235(3):655–691. doi: 10.1113/jphysiol.1973.sp010410. [DOI] [PMC free article] [PubMed] [Google Scholar]
- Anderson M. J., Cohen M. W., Zorychta E. Effects of innervation on the distribution of acetylcholine receptors on cultured muscle cells. J Physiol. 1977 Jul;268(3):731–756. doi: 10.1113/jphysiol.1977.sp011879. [DOI] [PMC free article] [PubMed] [Google Scholar]
- Auerbach A., Lingle C. J. Heterogeneous kinetic properties of acetylcholine receptor channels in Xenopus myocytes. J Physiol. 1986 Sep;378:119–140. doi: 10.1113/jphysiol.1986.sp016211. [DOI] [PMC free article] [PubMed] [Google Scholar]
- Auerbach A., Sachs F. Single-channel currents from acetylcholine receptors in embryonic chick muscle. Kinetic and conductance properties of gaps within bursts. Biophys J. 1984 Jan;45(1):187–198. doi: 10.1016/S0006-3495(84)84147-8. [DOI] [PMC free article] [PubMed] [Google Scholar]
- Blackshaw S., Warner A. Onset of acetylcholine sensitivity and endplate activity in developing myotome muscles of Xenopus. Nature. 1976 Jul 15;262(5565):217–218. doi: 10.1038/262217a0. [DOI] [PubMed] [Google Scholar]
- Brehm P., Kidokoro Y., Moody-Corbett F. Acetylcholine receptor channel properties during development of Xenopus muscle cells in culture. J Physiol. 1984 Dec;357:203–217. doi: 10.1113/jphysiol.1984.sp015497. [DOI] [PMC free article] [PubMed] [Google Scholar]
- Brehm P., Kullberg R., Moody-Corbett F. Properties of non-junctional acetylcholine receptor channels on innervated muscle of Xenopus laevis. J Physiol. 1984 May;350:631–648. doi: 10.1113/jphysiol.1984.sp015222. [DOI] [PMC free article] [PubMed] [Google Scholar]
- Colquhoun D., Hawkes A. G. On the stochastic properties of single ion channels. Proc R Soc Lond B Biol Sci. 1981 Mar 6;211(1183):205–235. doi: 10.1098/rspb.1981.0003. [DOI] [PubMed] [Google Scholar]
- Colquhoun D., Sakmann B. Fast events in single-channel currents activated by acetylcholine and its analogues at the frog muscle end-plate. J Physiol. 1985 Dec;369:501–557. doi: 10.1113/jphysiol.1985.sp015912. [DOI] [PMC free article] [PubMed] [Google Scholar]
- Colquhoun D., Sakmann B. Fluctuations in the microsecond time range of the current through single acetylcholine receptor ion channels. Nature. 1981 Dec 3;294(5840):464–466. doi: 10.1038/294464a0. [DOI] [PubMed] [Google Scholar]
- Dionne V. E., Leibowitz M. D. Acetylcholine receptor kinetics. A description from single-channel currents at snake neuromuscular junctions. Biophys J. 1982 Sep;39(3):253–261. doi: 10.1016/S0006-3495(82)84515-3. [DOI] [PMC free article] [PubMed] [Google Scholar]
- Fischbach G. D., Schuetze S. M. A post-natal decrease in acetylcholine channel open time at rat end-plates. J Physiol. 1980 Jun;303:125–137. doi: 10.1113/jphysiol.1980.sp013275. [DOI] [PMC free article] [PubMed] [Google Scholar]
- Greenberg A. S., Nakajima S., Nakajima Y. Functional properties of newly inserted acetylcholine receptors in embryonic Xenopus muscle cells. Brain Res. 1985 Apr;351(2):289–296. doi: 10.1016/0165-3806(85)90200-7. [DOI] [PubMed] [Google Scholar]
- Hamill O. P., Marty A., Neher E., Sakmann B., Sigworth F. J. Improved patch-clamp techniques for high-resolution current recording from cells and cell-free membrane patches. Pflugers Arch. 1981 Aug;391(2):85–100. doi: 10.1007/BF00656997. [DOI] [PubMed] [Google Scholar]
- Hamm T. M., Reinking R. M., Roscoe D. D., Stuart D. G. Synchronous afferent discharge from a passive muscle of the cat: significance for interpreting spike-triggered averages. J Physiol. 1985 Aug;365:77–102. doi: 10.1113/jphysiol.1985.sp015760. [DOI] [PMC free article] [PubMed] [Google Scholar]
- Horn R., Brodwick M. S. Acetylcholine-induced current in perfused rat myoballs. J Gen Physiol. 1980 Mar;75(3):297–321. doi: 10.1085/jgp.75.3.297. [DOI] [PMC free article] [PubMed] [Google Scholar]
- Horn R., Lange K. Estimating kinetic constants from single channel data. Biophys J. 1983 Aug;43(2):207–223. doi: 10.1016/S0006-3495(83)84341-0. [DOI] [PMC free article] [PubMed] [Google Scholar]
- Jackson M. B., Wong B. S., Morris C. E., Lecar H., Christian C. N. Successive openings of the same acetylcholine receptor channel are correlated in open time. Biophys J. 1983 Apr;42(1):109–114. doi: 10.1016/S0006-3495(83)84375-6. [DOI] [PMC free article] [PubMed] [Google Scholar]
- Kidokoro Y., Anderson M. J., Gruener R. Changes in synaptic potential properties during acetylcholine receptor accumulation and neurospecific interactions in Xenopus nerve-muscle cell culture. Dev Biol. 1980 Aug;78(2):464–483. doi: 10.1016/0012-1606(80)90347-4. [DOI] [PubMed] [Google Scholar]
- Kidokoro Y., Gruener R. Distribution and density of alpha-bungarotoxin binding sites on innervated and noninnervated Xenopus muscle cells in culture. Dev Biol. 1982 May;91(1):78–85. doi: 10.1016/0012-1606(82)90010-0. [DOI] [PubMed] [Google Scholar]
- Kullberg R. W., Brehm P., Steinbach J. H. Nonjunctional acetylcholine receptor channel open time decreases during development of Xenopus muscle. Nature. 1981 Jan 29;289(5796):411–413. doi: 10.1038/289411a0. [DOI] [PubMed] [Google Scholar]
- Kullberg R. W., Lentz T. L., Cohen M. W. Development of the myotomal neuromuscular junction in Xenopus laevis: an electrophysiological and fine-structural study. Dev Biol. 1977 Oct 1;60(1):101–129. doi: 10.1016/0012-1606(77)90113-0. [DOI] [PubMed] [Google Scholar]
- Kullberg R., Owens J. L., Vickers J. Development of synaptic currents in immobilized muscle of Xenopus laevis. J Physiol. 1985 Jul;364:57–68. doi: 10.1113/jphysiol.1985.sp015729. [DOI] [PMC free article] [PubMed] [Google Scholar]
- Leibowitz M. D., Dionne V. E. Single-channel acetylcholine receptor kinetics. Biophys J. 1984 Jan;45(1):153–163. doi: 10.1016/S0006-3495(84)84144-2. [DOI] [PMC free article] [PubMed] [Google Scholar]
- Leonard R. J., Nakajima S., Nakajima Y., Takahashi T. Differential development of two classes of acetylcholine receptors in Xenopus muscle in culture. Science. 1984 Oct 5;226(4670):55–57. doi: 10.1126/science.6474189. [DOI] [PubMed] [Google Scholar]
- Neher E., Sakmann B. Noise analysis of drug induced voltage clamp currents in denervated frog muscle fibres. J Physiol. 1976 Jul;258(3):705–729. doi: 10.1113/jphysiol.1976.sp011442. [DOI] [PMC free article] [PubMed] [Google Scholar]
- Neher E., Sakmann B. Single-channel currents recorded from membrane of denervated frog muscle fibres. Nature. 1976 Apr 29;260(5554):799–802. doi: 10.1038/260799a0. [DOI] [PubMed] [Google Scholar]
- Ogden D. C., Colquhoun D. Ion channel block by acetylcholine, carbachol and suberyldicholine at the frog neuromuscular junction. Proc R Soc Lond B Biol Sci. 1985 Sep 23;225(1240):329–355. doi: 10.1098/rspb.1985.0065. [DOI] [PubMed] [Google Scholar]
- Ogden D. C., Colquhoun D. The efficacy of agonists at the frog neuromuscular junction studied with single channel recording. Pflugers Arch. 1983 Nov;399(3):246–248. doi: 10.1007/BF00656725. [DOI] [PubMed] [Google Scholar]
- Sakmann B., Brenner H. R. Change in synaptic channel gating during neuromuscular development. Nature. 1978 Nov 23;276(5686):401–402. doi: 10.1038/276401a0. [DOI] [PubMed] [Google Scholar]
- Sakmann B., Methfessel C., Mishina M., Takahashi T., Takai T., Kurasaki M., Fukuda K., Numa S. Role of acetylcholine receptor subunits in gating of the channel. Nature. 1985 Dec 12;318(6046):538–543. doi: 10.1038/318538a0. [DOI] [PubMed] [Google Scholar]
- Sakmann B., Patlak J., Neher E. Single acetylcholine-activated channels show burst-kinetics in presence of desensitizing concentrations of agonist. Nature. 1980 Jul 3;286(5768):71–73. doi: 10.1038/286071a0. [DOI] [PubMed] [Google Scholar]
- Siegelbaum S. A., Trautmann A., Koenig J. Single acetylcholine-activated channel currents in developing muscle cells. Dev Biol. 1984 Aug;104(2):366–379. doi: 10.1016/0012-1606(84)90092-7. [DOI] [PubMed] [Google Scholar]
- Sigworth F. J. Open channel noise. I. Noise in acetylcholine receptor currents suggests conformational fluctuations. Biophys J. 1985 May;47(5):709–720. doi: 10.1016/S0006-3495(85)83968-0. [DOI] [PMC free article] [PubMed] [Google Scholar]
- Sine S. M., Steinbach J. H. Acetylcholine receptor activation by a site-selective ligand: nature of brief open and closed states in BC3H-1 cells. J Physiol. 1986 Jan;370:357–379. doi: 10.1113/jphysiol.1986.sp015939. [DOI] [PMC free article] [PubMed] [Google Scholar]
- Sine S. M., Steinbach J. H. Activation of a nicotinic acetylcholine receptor. Biophys J. 1984 Jan;45(1):175–185. doi: 10.1016/S0006-3495(84)84146-6. [DOI] [PMC free article] [PubMed] [Google Scholar]
- Sine S. M., Steinbach J. H. Activation of acetylcholine receptors on clonal mammalian BC3H-1 cells by low concentrations of agonist. J Physiol. 1986 Apr;373:129–162. doi: 10.1113/jphysiol.1986.sp016039. [DOI] [PMC free article] [PubMed] [Google Scholar]
- Sine S. M., Steinbach J. H. Agonists block currents through acetylcholine receptor channels. Biophys J. 1984 Aug;46(2):277–283. doi: 10.1016/S0006-3495(84)84022-9. [DOI] [PMC free article] [PubMed] [Google Scholar]
- Yellen G. Ionic permeation and blockade in Ca2+-activated K+ channels of bovine chromaffin cells. J Gen Physiol. 1984 Aug;84(2):157–186. doi: 10.1085/jgp.84.2.157. [DOI] [PMC free article] [PubMed] [Google Scholar]